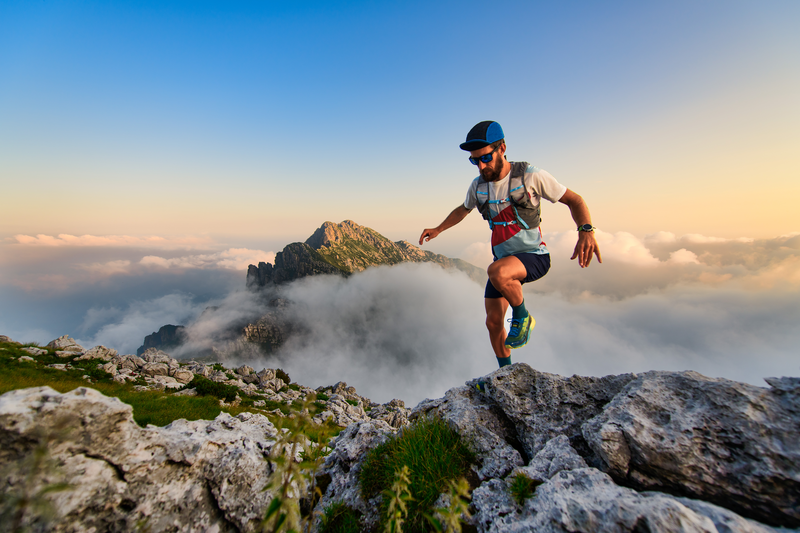
95% of researchers rate our articles as excellent or good
Learn more about the work of our research integrity team to safeguard the quality of each article we publish.
Find out more
REVIEW article
Front. Bioeng. Biotechnol. , 24 January 2023
Sec. Biomaterials
Volume 11 - 2023 | https://doi.org/10.3389/fbioe.2023.1100310
This article is part of the Research Topic Advancement of Stem Cells and Regenerative Agents in Treating Degenerations of Locomotor System View all 7 articles
Exosomes are natural extracellular vesicles with a diameter of 30–150 nm, which exist in biological fluids and contain biomolecules related to the parent cell, such as proteins, nucleic acids, lipids, etc. It has a wide range of biological functions, and participates in the regulation of important physiological and pathological activities of the body. It can be used as a biomarker for early diagnosis of ocular diseases, a potential therapeutic target, a targeted drug carrier, and has a high potential for clinical application. In this paper, we summarized the genesis mechanism, biological functions, research and application progress of exosomes, focused on the engineering strategy of exosomes, and summarized the advantages and disadvantages of common engineering exosome preparation methods. Systematically combed the role of exosomes in corneal diseases, glaucoma, and retinal diseases, to provide a reference for further understanding of the role of exosomes in the pathogenesis, diagnosis, and treatment of ocular diseases. Finally, we further summarized the opportunities and challenges of exosomes for precision medicine. The extension of exosome research to the field of ophthalmology will help advance current diagnostic and therapeutic methods. Tiny exosomes have huge potential.
Exosomes are lipid bilayer extracellular vesicles (EVs) secreted by various cells, with a diameter of 30–150 nm. They are the smallest subset of extracellular vesicles, distributed in various biological fluids, tears, aqueous humor, vitreous and blood are rich in exosomes (Liu et al., 2020). The exosomes were first discovered in sheep reticulocytes by Pan and Johnstone (1983). They were originally used to describe the membrane vesicles released by reticulocytes during differentiation, during which transferrin receptors are lost and exosomes are produced. Exosomes contain a variety of contents, the composition of which is different according to the type of cells from which they originate and the extracellular environment, resulting in a wide range of biological functions, including cellular communication, cellular waste management, inflammation regulation, immune regulation, and repair and regeneration. Meanwhile, the lipid composition of exosomes is of great significance for their stability in the extracellular space, protecting their contents from degradative enzymes or other chemicals in the extracellular environment, and has excellent stable chemical properties (Skotland et al., 2019).
Ocular diseases bring great inconvenience to people’s daily life, and some of them have insidious early symptoms. The complex anatomical structure of the eye and the existence of physiological barriers bring great challenges to the early diagnosis and treatment of eye diseases (Weng et al., 2017; Liang et al., 2018). Exosomes have been shown to be involved in the pathogenesis of ocular diseases, and sometimes the changes of exosome cargo can reflect the changes of ocular diseases, so as to be used as diagnostic markers. As a natural carrier, exosomes have higher barrier-crossing ability and safety than synthetic nano-drug carriers, and are more capable of delivering diverse drugs or bioactive molecules. In this article, we take representative ophthalmic diseases with different levels of anatomy (keratonosus, glaucoma, retinal diseases) to elucidate the roles of exosomes from different sources in their pathogenesis, diagnosis, and treatment. Exosomes can provide therapeutic effects to ocular diseases by signaling or transporting biomolecules, which can serve as ideal drug delivery vehicles for targeted therapies. We also summarized important information about exosomes, including their biogenesis, composition, biological functions, isolation and purification methods, and engineering strategies.
EVs can be classified into three types, according to their biological origin and size: exosomes (30–150 nm in diameter), microvesicles (100–1,000 nm), and apoptotic bodies (>1,000 nm) (Manukonda et al., 2022). Their diameters differ greatly, and their biogenesis is different, with different characteristics. Exosomes are derived from endosomes, the cell membrane invaginates to form early endosomes, the early endosomes mature into late endosomes and then transform into multivesicular bodies, which contain intraluminal vesicles (future exosomes). Eventually, the multivesicular bodies fuse with the cell membrane and release the exosomes into the extracellular space through exocytosis (Kalluri and LeBleu, 2020). The molecular mechanism of exosome genesis has not been fully elucidated, but studies have shown that the endosomal sorting complex required for transports (ESCRT) (Colombo et al., 2013), tetraspanins (van Niel et al., 2011), ceramides (Trajkovic et al., 2008), and lipids (Skotland et al., 2019) are all involved in exosome genesis, to achieve specific sorting of exosome contents (Zhang Y. et al., 2019).
After being secreted by the cell, exosomes can integrate their contents into the recipient cell by fusing with the cell membrane; or the various forms of endocytosis, such as endocytosis mediated by lipid raft, clathrin, caveolin, and phagocytosis, macropinocytosis, deliver the various messages to the recipient cells; it can also communicate with target cells directly through receptor-ligand interaction (Figure 1) (Gurung et al., 2021). It has not been determined whether different exosome uptake patterns in recipient cells result in different functional effects.
FIGURE 1. Biogenesis and uptake of exosomes. The parent cell membrane invaginates to form early endosome, matures to late endosome, and transforms into multivesicular body (MVB), contain intraluminal vesicles. The MVB fuse with the cell membrane and release exosomes. After exosomes are released, information can be transmitted to recipient cells through membrane fusion, direct interaction and endocytosis.
Exosomes of different cellular origins differ in size, contents, and effects on recipient cell function, showing high heterogeneity (Chiang and Chen, 2019; Kalluri and LeBleu, 2020). Exosomes contain many components of parent cells, includes growth factors, cytokines, signaling lipids, mRNA, miRNA and proteins (Phinney and Pittenger, 2017). So far, 9,769 proteins, 3,408 mRNAs, and 2,838 miRNAs have been identified in exosomes (exocarta.org). Exosomes are rich in proteins, including non-specific and specific proteins (Doyle and Wang, 2019; Mashouri et al., 2019; Gurung et al., 2021). The non-specific proteins are independent of the cellular origin of the exosomes, which are present in all exosomes, such as ESCRT proteins (Alix, TSG101), heat shock proteins (HSC70, HSC90), transport and fusion proteins (Rab protein, Annexins, Flotillin), cytoskeletal proteins (Actin, Tublin, Cofilin), can be used to detect the presence of exosomes. In contrast, specific proteins are associated with exosome-derived cells, such as MHC Class I and MHC Class II proteins, transferrin receptors, which can reflect the specificity of tissue and cell, and may mediate signaling. Exosomes contain a variety of lipids, including sphingolipid, cholesterol, ceramide, etc., which not only maintain chemical stability, but also participate in exosome biogenesis (Minciacchi et al., 2015). In addition, the exocrine cavity also contains a variety of nucleic acids, including DNA (single-stranded DNA, double-stranded DNA) and RNA (miRNA, lncRNA, mRNA, rRNA, etc.), among which miRNA is the most abundant (Figure 2) (Gurung et al., 2021). The composition of exosomes may reflect not only the composition of the parent cells to some extent, but also the existence of different sorting mechanisms during exosome biogenesis.
FIGURE 2. Diagram of exosomal molecular composition. Exosomes are composed of lipid bilayers that carry a variety of biomolecules, including proteins, DNA, RNA, lipids, etc.
The biological functions of exosomes are extensive, and the understanding of their biological functions helps to study the role of exosomes in health and disease. 1) Signal transduction: the most basic and important biological function of exosomes. As carriers of cellular communication, exosomes can transport their contents to nearby or distant cells, thus regulating various physiological and pathological processes (Wei et al., 2021). 2) Inflammatory regulation: exosomes can promote/inhibit the activation of inflammasomes (Noonin and Thongboonkerd, 2021). Luo Z. W. et al. (2021); Luo et al. (2022a) demonstrated that exosomes secreted by cells in an inflammatory environment can induce M1 polarization and inhibit M2 polarization of macrophages, which in turn promote the inflammatory response. 3) Immunomodulation: immune cell-derived exosomes can promote/inhibit the immune response, depending on the composition of exosomes and the induced cellular environment, allowing exosomes to play an important role in immune response and immune privilege (Li Q. et al., 2019). 4) Repair and regeneration: mesenchymal stem cell (MSC) derived exosomes play a protective and therapeutic role in wound healing and tissue regeneration (Bakhtyar et al., 2017; Chen W. et al., 2021). 5) Autophagy regulation: exosomes from different sources can promote/inhibit autophagy (Hassanpour et al., 2020). In addition, exosomes can be used as biomarkers and therapeutic carriers of diseases. The composition of functional molecules in exosomes varies according to their secreting cells and is influenced by the cellular microenvironment. Different functional molecular compositions allow for a wide range of exosome biological functions (Gurunathan et al., 2019).
Exosomes are widely found in various biological fluids as well as cell culture supernatants (Yanez-Mo et al., 2015). Common techniques for exosome isolation include ultracentrifugation, density gradient centrifugation, ultrafiltration, size exclusion chromatography, chemical precipitation, immunoaffinity capture, and microfluidic techniques (Chiang and Chen, 2019; Sun et al., 2022). Ultracentrifugation is the most common technique and the gold standard for exosome separation (Li et al., 2017). Density gradient centrifugation can be considered as an advanced version of ultracentrifugation, which can obtain exosomes with high purity. However, this method is cumbersome and has a relatively low yield (Van Deun et al., 2014). Ultrafiltration, one of the simplest separation techniques, passes exosomes through filtration membranes with different molecular weight cutoff. Size exclusion chromatography is similar to ultrafiltration (Chen J. et al., 2021). The principle of chemical precipitation is protein coagulation, which is easy to operate. However, the exosomes obtained are of low purity and often contain macromolecular protein impurities which are not conducive to proteomic analysis of exosomes. The principle of immunoaffinity capture is an antigen-antibody-specific reaction. The exosomes obtained by this method have high purity but low activity (Greening et al., 2015). Microfluidic chip separates exosomes according to physical and biochemical characteristics (Chen W. et al., 2021). This method is characterized by high speed, high throughput, and strong specificity. It can rapidly detect exosomes from a large number of clinical samples, which is a new diagnostic technology with promising clinical applications.
Different methods of isolation and purification have their advantages and disadvantages, but there is no technology available to isolate exosomes to absolute purity (Thery et al., 2018). In addition to developing new technologies, the combined application of the above techniques may help to overcome the shortcomings of individual technology, and give consideration to the yield and purity as much as possible without damaging the bioactivity of exosomes. With the continuous development of exosome isolation and purification technology, the application of nanotechnology and microfluidic chips can optimize the study of exosomes. Standardization and new exosome isolation protocols can help improve the consistency of exosome studies, and the application of exosomes in the field of ophthalmology will continue to expand.
Natural exosomes still face some challenges as therapeutic molecular delivery systems (Jafari et al., 2020). The therapeutic effect of natural exosomes is limited due to the absence of pharmaceutical components. Animal experiments have demonstrated that natural exosomes have poor tissue and cell targeting ability (Tian et al., 2018), and their cargo will be non-selectively transported to the recipient cells. Fortunately, exosomes are highly engineerable, namely engineered exosomes, to further improve their targeting, safety, therapeutic efficiency, and loading of more effective functional molecules to suit clinical needs (Zhang et al., 2021).
Currently, exosome engineering strategies are divided into “cell engineering” and “exosome engineering” in terms of time (Figure 3) (Lu et al., 2018). “Cell engineering” is to use genetic engineering and co-incubation to transform parent cells, transfect nucleotides or import proteins, and load them into exosomes. While “exosome engineering” is the modification of exosomes directly after they are secreted. It also can be divided into exosome membrane modification and content loading in space (Xu et al., 2020). The main purpose of outer membrane modification is to enhance the targeting of exosomes, reduce the clearance of exosomes, increase the concentration of exosomes at the disease site, maximize the therapeutic effect and reduce cytotoxicity and adverse reactions (Liang et al., 2021). While content loading can make exosomes load specific nucleic acids, proteins, small molecule drugs, etc. Zhang et al. (2022) used extrusion and incubation methods to treat neutrophil exosomes, and constructed engineered exosomes modified with superparamagnetic iron oxide nanoparticles and loaded with chemotherapeutic drugs. Under the action of the external magnetic field, the exosomes were targeted and enriched at tumor sites, achieving a dual effect of tumor treatment, almost completely eliminating tumor growth, and greatly prolonging the survival time of tumor mice. In addition, biocompatible scaffolds loaded with exosomes can be developed to construct exosome carriers, which can deliver exosomes in targeted batches. Achieve sustained and controlled release of exosomes, and enhance its therapeutic effect (Mishra et al., 2021). In another study, three different loading procedures, incubation, sonication, and electroporation, were used and the results proved that sonication was the most efficient (Kim et al., 2016). The engineering strategy of exosomes and the construction of exosome carriers, combining low immunogenicity, nanoparticles, targeted drug delivery, and drug delivery systems, are helpful to improve the half-life of exosomes and enable targeted enrichment, which is important for biomedical research and clinical translation of exosomes. Further, Table 1 summarizes the advantages and disadvantages of common engineering strategies.
FIGURE 3. Diagram of engineered exosome. The engineering strategies of exosomes include cell engineering and exosome engineering. Hydrophobic drugs, hydrophilic drugs and targeted ligands can be loaded onto exosomes through different methods.
The cornea is the outermost anatomical structure of the eye, exposed to the external environment. Corneal-related diseases are mainly corneal trauma caused by chemical or physical damage, associated with inflammation, ulceration, corneal scar, and neovascularization (Whitcher et al., 2001). If not treated effectively in time, blindness may result. The pathogenesis of corneal fibrosis/scar formation is characterized by excessive production of extracellular matrix (ECM) and deposition in the stroma layer. ECM remodeling can lead to corneal fibrosis, accompanied by inflammation and neovascularization (Janin-Manificat et al., 2012; Wilson et al., 2012). Exosomes derived from corneal fibroblasts can transport matrix metalloproteinase 14 to vascular endothelial cells, thus mediating corneal angiogenesis (Han et al., 2015). The role of exosomes in the physiological and pathological reactions of corneal wound healing and scar formation has been extensively studied (Samaeekia et al., 2018; McKay et al., 2020; Yeung et al., 2022). In vitro experiments have demonstrated that mouse corneal epithelium-derived exosomes fuse with corneal stromal cells and induce myofibroblast transformation, leading to the formation of corneal scar (Han et al., 2017). These results suggest that exosomes are important mediators of corneal wound healing, play a role in the intercellular communication between epithelium and stroma, and may serve as therapeutic targets for corneal tissue regeneration.
High IOP has long been considered as the most important risk factor for the development and progression of glaucoma (Vidal-Sanz et al., 2012). Exosomes can participate in regulating the function of trabecular meshwork cells, including involvement in transporting the glaucoma-associated secretory protein myocilin (Stamer et al., 2011), interfering with the classical Wnt signaling pathway (Tabak et al., 2018), regulating ECM turnover and remodeling (Dismuke et al., 2016), regulating atrial aqueous dynamics, thereby affecting intraocular pressure, thus participating in the development of glaucoma. Further exploration of the exosome pathway will help to find new targets for glaucoma treatment targeting the aqueous humor drainage system. Exosome-mediated inflammation also plays an important role in the pathogenesis of glaucoma (Aires et al., 2020). When intraocular pressure increases, exosomes derived from retinal microglia interact with receptor microglia cells to induce neuroinflammation, inducing oxidative stress and retinal ganglion cells (RGCs) death, leading to retinal degeneration of glaucoma.
Diabetic retinopathy (DR) is a microvascular complication of diabetes mellitus. It is caused by long-term chronic hyperglycemia that leads to leakage and obstruction of the retinal microvascular, resulting in fundus lesions. DR has a complex pathogenesis and can result in irreversible vision loss. Exosome levels in blood were higher in diabetic rats and diabetic patients than in normal controls (Huang et al., 2018; Mazzeo et al., 2018), and the increased number of exosomes reflects the increased secretory activity of cells in the state of diabetes, which may help accelerate the progression of diabetic retinopathy (Ogata et al., 2005). In addition, exosomes transport inflammatory factors, activate endothelial cells, and participate in angiogenesis and diabetic microangiopathy under certain conditions.
In terms of vascular injury, endothelial cells are directly exposed to blood, and several studies have shown that endothelial cells can take up circulating exosomes (Zhang et al., 2018; Yao et al., 2019). Hang et al. demonstrated that the complement content of plasma exosomes increased in patients with diabetic retinopathy, and exosomes rich in IgG could promote the activation of the diabetes-induced classical complement pathway, and the up-regulation of pro-inflammatory factors and chemokines, thus leading to vascular damage and eventually causing an increase in retinal microvascular leakage. Conversely, IgG-deficient exosomes reduce retinal vascular leakage, suggesting that a complement cascade that inhibits exosomal activation may prevent the progression of diabetic retinopathy (Huang et al., 2018). Through proteomic analysis, Zhang et al. (2018) found a significant increase in Arginase 1 content in serum exosomes of diabetic mice. These exosomes rich in Arginase 1 were taken up by endothelial cells, inhibiting the production of nitric oxide, and damaging the function of vascular endothelial cells.
In terms of neovascularization, Tokarz et al. (2015) compared plasma EVs with plasma without EVs in diabetic patients, and found that cytokines and angiogenic factors were significantly increased in EVs in diabetic patients, suggesting that exosomes promote angiogenesis, by transporting pro-angiogenic factors to accelerate the progression of diabetic retinopathy. On the other hand, some studies have shown that plasma exosomes from diabetic patients also contain components that inhibit angiogenesis. Xiong et al. (2020) extracted exosomes from the blood of patients with type 2 diabetes and observed that miR-20b-5p was significantly up-regulated. The miRNA could inhibit the angiogenesis of human umbilical vein endothelial cells by regulating Wnt9b/β-catenin signaling.
These results suggest that the content or composition of exosomes changes with diabetes, and plays an important role in the retinal vascular disease of diabetes. Given that blood is a kind of tissue that can reach the whole body, the cellular origin of plasma exosomes is relatively complex, and the heterogeneity of exosomes may be one of the reasons for their different effects. Further studies are needed to determine how exosomes precisely regulate vascular injury and neovascularization under specific conditions.
The progressive degeneration and death of retinal pigment epithelial (RPE) cells are considered as the initial pathology of age-related macular degeneration (AMD), and oxidative stress is considered as an important factor leading to RPE injury and degeneration. Early AMD is characterized by the formation of drusen, which is closely related to the RPE cells. Zhang et al. (2019a) showed that after oxidative stress injury induced by blue light, the RPE can secrete exosomes rich in IL-1β, IL-18, and caspase-1, which in turn can activate NLRP3 inflammasome and further aggravate the oxidative stress response of RPE. Compared with normal RPE, RPE damaged by oxidative stress secreted increased exosomes and carried vascular endothelial growth factor (VEGF) receptors 1 and 2, which promoted endothelial cell neovascularization (Atienzar-Aroca et al., 2016; Tong et al., 2016). Complement factor H (CFH) dysfunction is associated with AMD. Wang et al. proposed that in aging retinal tissue, CFH dysfunction in the complement pathway can lead to RPE-derived exosomes containing C3 molecules becoming targets of invading leukocytes and being attacked by leukocytes, leading to instability of the exosome membrane and release of intracellular proteins, thus promoting the formation of drusen. In addition, they also found increased expression of exosome markers CD63, CD8, and LAMP2 in AMD patients with drusen, but not in age-matched healthy controls (Wang et al., 2009). The above findings indicate that RPE-derived exosomes are involved in the development of AMD, suggesting that exosomes interact with the complement pathway, and RPE-derived exosomes may be targets of the complement system.
Early symptoms of primary glaucoma are insidious, and nearly half of Chinese glaucoma patients have missed the best time for treatment when diagnosed (Liang et al., 2018). In addition to conventional diagnostic methods, exosomes can be used as risk predictors to supplement the existing glaucoma diagnostic strategies. Sacca et al. (2012) found through proteomic analysis that compared with healthy controls, the expression levels of several exosome proteins in aqueous humor of glaucoma patients were significantly increased. Among them, heat shock protein 90 played a key role in the release of exosomes (Lauwers et al., 2018). Dismuke et al. (2015) detected miRNAs involved in aqueous humor generation and drainage tissue communication, such as miR-204, miR-191, and miR-148a, suggesting that glaucoma can be diagnosed by identifying the content of specific miRNAs in aqueous exosomes.
Exosomes are also important for the diagnosis of DR. The change of exosome contents is earlier than the appearance of fundus lesions, and has high specificity. It is an ideal biological marker for early diagnosis of DR, prediction of disease progress, and prognosis. Sadeghzadeh et al. (2020) proposed that the plasma expression levels of miR-222 and miR-15a can be used to distinguish pre-diabetes from normal people. Compared with diabetes, the expression of miR-15a in pre-diabetes patients is significantly downregulated. Compared with healthy controls, the miRNA expression profile of plasma EVs in diabetes was different. Mazzeo et al. (2018) found that the expression of miR-150-5p, miR-21-3p, and miR-30b-5p in the diabetes group was increased, which can be used as a potential biomarker of diabetes retinopathy. Further, Fan et al. analyzed the mRNA of plasma exosomes in type 1 diabetes patients and healthy controls, and found four differentially expressed mRNA with statistical significance, indicating that plasma-derived exosomes mRNAs can be used as a new minimally invasive diagnostic tool for diabetes.
Several studies have demonstrated the therapeutic effect of stem cell-derived exosomes on the corneal injury (Hertsenberg et al., 2017; Shojaati et al., 2019; Ma et al., 2022). In the corneal injury mouse model, combined with an autophagy activator, human umbilical cord MSC-derived exosomes (MSC-exos) activated the autophagy AMPK-mTOR-ULK1 signaling pathway, reduced the levels of the apoptotic markers, Bax and cleaved Caspase-3, and decreased the expression of the inflammatory response products, TNF-α, IL-1β, IL-6, and CXCL-2, thereby reducing corneal epithelial defects and stromal opacity (Figure 4). In vitro experiments showed that the combination treatment significantly increased the viability of human corneal epithelial cells and promoted cell proliferation (Ma et al., 2022). Hertsenberg et al. (2017) demonstrated that exosomes from human corneal stromal stem cells through a TSG-6-dependent pathway, down-regulate the expression of the fibrosis markers myostatin C, Acta2, and Col3a1, inhibit neutrophil infiltration, inhibit corneal inflammation, stimulate regeneration of transparent stroma, and reduce corneal scar formation and fibrosis. Further experiments demonstrated that the above effects were produced by miRNA-dependent mechanisms. Gene knockdown using siRNA reduced miRNA in exosomes by 85%, and the effect of exosomes to prevent corneal scar formation was subsequently lost (Shojaati et al., 2019). Based on microfluidic technology, Yu et al. (2022) introduced human corneal-derived cells, simulated the environment of corneal injury with microfluidics, designed and constructed an in vitro bionic corneal chip, and found that stem cell exosomes could significantly promote the recovery of corneal epithelium, reduce the expression of matrix metallopeptidase-2 protein, inhibit corneal inflammation and neovascularization, and facilitate the process of injury repair. The above studies demonstrated that stem cell exosomes promote scar-free repair of corneal injury and help maintain corneal transparency. Meanwhile, different engineering strategies can be used to load exosomes with therapeutic components [such as miRNA, lncRNA (Sun Y. et al., 2018)] and construct exosome scaffolds to further enhance their therapeutic effects.
Stem cell-derived exosomes can be an effective neuroprotective and regenerative therapy (Doeppner et al., 2015). Using bone marrow MSC-exos, Mead et al. demonstrated that exosomes preserve RGCs function and promote RGCs survival and axonal regeneration through miRNA-dependent mechanisms. Exosomes deliver miRNA to RGCs for expression, leading to the translation of new proteins. After the key effector molecule Argonaute-2 of miRNA is knocked out, the therapeutic effect of exosomes is weakened (Mead and Tomarev, 2017); Liu et al. (2022) demonstrated that exosomes inhibit microglial activation and its associated neuroinflammation, and up-regulate synapse-associated protein and brain-derived neurotrophic factor protein expression. In summary, bone marrow MSC-exos can act as neuroprotective agents in glaucoma, promoting RGCs survival, inhibiting neuroinflammatory damage, and delivering neurotrophic proteins. In addition, umbilical cord MSC-exos (Pan et al., 2019) and human embryonic stem cell-derived exosomes (Seyedrazizadeh et al., 2020) also showed neuroprotective effects.
In the treatment of diabetic retinopathy, MSC-exos inhibit the inflammatory response and the formation of new blood vessels. Pakravan et al. (2017) demonstrated that MSC-exos significantly inhibited VEGF expression and secretion in a dose-dependent manner. Moreover, MSC-exos inhibit hyperglycemia-induced inflammatory responses, and MSC-exos overexpressing miR-126 significantly reduced HMGB1 expression and NLRP3 inflammasome activity in hyperglycemia-treated retinal endothelial cells (Zhang et al., 2019b), suggesting that miR-126 in MSC-exos reduces hyperglycemic-induced retinal inflammation by down-regulating the HMGB1 signaling pathway.
Choroidal neovascularization (CNV) is an important cause of blindness in AMD patients and causes mechanical compression of RPE (Farjood et al., 2020). Hajrasouliha et al. (2013) found that subconjunctiva injection of retinal astrocyte-derived exosomes (RAC-exos) targeted both macrophages and vascular endothelial cells, inhibited their infiltration and migration, and had anti-angiogenic effects, whereas exosomes from RPE or non-retinal tissue cells did not show such anti-angiogenic effects. Although intravitreal injection of anti VEGF is an effective treatment, subconjunctival injection is less invasive than intravitreal injection (Kim and Woo, 2021). Meanwhile, subconjunctival exosomes injection allows for rapid drug delivery to the choroid and retina, which may be used for anti angiogenesis treatment of wet AMD in the future. The above experiments suggest that subconjunctival exosome injection may be a safer and more effective way of drug delivery. Zhu et al. (2020) further showed that normal RAC-exos were able to significantly inhibit angiogenesis, whereas under oxidative stress conditions, RAC autophagy levels were elevated and exosomes derived from them had the opposite effect, manifesting as promotion of human umbilical vein endothelial cell metastasis and angiogenesis.
Stem cell-derived exosomes have also been shown to have therapeutic effects. He et al. (2018) demonstrated that cord blood MSC-exos downregulated VEGF-A, alleviating blue light and laser-induced retinal pigment epithelial damage. On the other hand, mouse neural progenitor cell-derived exosomes can inhibit microglia activation. The miRNAs carried by exosomes inhibit inflammatory signaling pathways in microglia by reducing the expression of TNF-α, IL-1β, and COX-2, thus reducing photoreceptor apoptosis in vivo or in vitro, delaying photoreceptor degeneration and preserving visual function (Bian et al., 2020).
It should be emphasized that exosomes are not only involved in the above-mentioned ocular diseases. The biological role of exosomes in other ocular diseases is summarized in Table 2.
MSCs have promising clinical translational applications (Luo Z. et al., 2021; Luo et al., 2022b). MSCs exosomes are safe, stable, easy to store, non-immunogenic, small in size, easily penetrate the human biological barrier, can be modified and engineered to act efficiently on target cells, and may serve as a non-cellular therapeutic biologic drug, and in the long run, a new alternative to MSC therapy (EL Andaloussi et al., 2013; Guillamat-Prats, 2021). Several studies mentioned above demonstrated that exosomes carry and transfer their contents such as miRNAs, proteins for intercellular communication and generate the corresponding biological functions. As the content of protein in the exosomes reaches the therapeutic dose and has sufficient functional activity, the ability of protein to initiate biological activity is widely recognized (Toh et al., 2018). Quantitative analysis of the stoichiometry of exosomal miRNAs showed that there is less than one copy per exosome (Chevillet et al., 2014). This implies that natural endogenous exosomes in a physiological setting lack the potential to act as therapeutic potential for miRNA transfer mediators. However, studies of exosome function often use large excesses of exosomes. Stevanato et al. (2016) demonstrated that exosomes can be mesoenriched with certain selected miRNAs up to 10 copies per exosome, and that exosomal agents can transfer certain miRNAs at a functional level to mediate biological effects.
As a natural nanoscale vesicle secreted by cells, exosomes are considered to be drug delivery vehicles with minimal immune response. Based on the exosome engineering strategy, hydrophobic substances can be inserted into the bilayer lipid membrane of the exosome, ensuring the therapeutic activity of the drug without chemical modification. In addition, natural exosomes derived from M2 type macrophages have anti-inflammatory properties. Li et al. (2022) engineered the exosomes derived from M2 type macrophages, transfected plasmids, and loaded with anti-inflammatory drugs. Through the synergistic effect of multiple mechanisms, macrophages were induced to transform from pro-inflammatory M1 type to anti-inflammatory M2 type. Engineered exosomes showed good inflammatory inhibition and targeted enrichment of inflammatory sites in inflammatory diseases, which could be used as a reference for inflammatory diseases in ophthalmology. In terms of targeted therapy, engineered exosomes, as targeting agents, have been proven to directly and specifically target oncogenes, significantly inhibit cancer cell proliferation, minimize cytotoxicity, and prolong the survival of pancreatic cancer mice (Kamerkar et al., 2017). Because the eye is a very small organ and its special anatomy, intravenous drug delivery has low drug utilization, and the application of engineered exosomes for targeted research in tumors has important implications for targeted therapy in ophthalmology.
At the same time, the nanoscale size allows exosomes to penetrate the human biological barrier, which has good retinal penetration. They can be injected through the vitreous cavity to reach the inner and outer layers of the retina (Pollalis et al., 2022), and can also be injected through the peribulbar quickly cross the wall of the eye to reach the eye (Hajrasouliha et al., 2013), with a better therapeutic effect than systemic intravenous injection (Safwat et al., 2018). Biocompatible scaffolds such as hydrogel dressings can greatly increase the retention rate and stability of exosomes in vivo. Tang et al. (2022) developed an exocrine sustained-release system based on thermosensitive chitosan hydrogel, which proved that it could effectively promote the repair and regeneration of damaged corneal epithelium and stroma, accelerate the corneal wound healing process, and reduce the formation of corneal scar. The above studies suggest that exosomes have great potential as intraocular drug delivery carriers, and the construction of exosome carriers can realize pulse therapy with a high concentration of drugs in the eye, reducing adverse effects and improving therapeutic efficacy.
While exosomes are significantly heterogeneous (Chiang and Chen, 2019), and quality control is a pressing challenge. But there is a lack of unified research standards, and there is an urgent need for more standard isolation and purification, analytical methods, and engineering strategies to obtain highly pure and consistent exosomes, to deeply explore valuable information about their biological functions. In addition, achieving mass production of therapeutic exosomes for clinical use is a challenge (van der Meel et al., 2014). The use of exosomes in the diagnosis of eye diseases is rarely studied, and false negative results may be caused by low concentrations of exosomes in body fluids (Sun Z. et al., 2018). However, microfluidic technology, which can rapidly and sensitively analyze exosomes, provides a miniaturized, integrated, and automated diagnostic method. With the development of modern technology and high-throughput sequencing facilities, exosomes as specific markers of eye diseases will be more widely studied and applied.
Exosomes play an important role in various biological processes, affecting various physiological and pathological processes. It has been proved that exosomes are involved in the pathogenesis, diagnosis, and treatment of ophthalmopathy from the molecular mechanism. In the future, the construction of an exosome delivery and treatment platform based on engineered exosomes is conducive to the realization of precision medicine for ocular diseases.
ZL and HL contributed to the conception and design of the study. XF, ZP, and LY designed and wrote the whole manuscript. ZP and ZL completed subsequent revisions of the manuscript. XF, MJ, HH, XP, YW, and CZ collected the references and prepared figures of the manuscript. All authors contributed to manuscript revision, and approved the final version of manuscript.
National Natural Science Foundation of China (82060181), Natural Science Foundation of Jiangxi Provincial Education Department (GJJ210201).
The authors declare that the research was conducted in the absence of any commercial or financial relationships that could be construed as a potential conflict of interest.
All claims expressed in this article are solely those of the authors and do not necessarily represent those of their affiliated organizations, or those of the publisher, the editors and the reviewers. Any product that may be evaluated in this article, or claim that may be made by its manufacturer, is not guaranteed or endorsed by the publisher.
Aires, I. D., Ribeiro-Rodrigues, T., Boia, R., Catarino, S., Girao, H., Ambrosio, A. F., et al. (2020). Exosomes derived from microglia exposed to elevated pressure amplify the neuroinflammatory response in retinal cells. Glia 68 (12), 2705–2724. doi:10.1002/glia.23880
Atienzar-Aroca, S., Flores-Bellver, M., Serrano-Heras, G., Martinez-Gil, N., Barcia, J. M., Aparicio, S., et al. (2016). Oxidative stress in retinal pigment epithelium cells increases exosome secretion and promotes angiogenesis in endothelial cells. J. Cell Mol. Med. 20 (8), 1457–1466. doi:10.1111/jcmm.12834
Bai, L., Shao, H., Wang, H., Zhang, Z., Su, C., Dong, L., et al. (2017). Effects of mesenchymal stem cell-derived exosomes on experimental autoimmune uveitis. Sci. Rep. 7 (1), 4323. doi:10.1038/s41598-017-04559-y
Bakhtyar, N., Jeschke, M. G., Mainville, L., Herer, E., and Amini-Nik, S. (2017). Acellular gelatinous material of human umbilical cord enhances wound healing: A candidate remedy for deficient wound healing. Front. Physiol. 8, 200. doi:10.3389/fphys.2017.00200
Bian, B., Zhao, C., He, X., Gong, Y., Ren, C., Ge, L., et al. (2020). Exosomes derived from neural progenitor cells preserve photoreceptors during retinal degeneration by inactivating microglia. J. Extracell. Vesicles 9 (1), 1748931. doi:10.1080/20013078.2020.1748931
Chen, C. F., Hua, K., Woung, L. C., Lin, C. H., Chen, C. T., Hsu, C. H., et al. (2019). Expression profiling of exosomal miRNAs derived from the aqueous humor of myopia patients. Tohoku J. Exp. Med. 249 (3), 213–221. doi:10.1620/tjem.249.213
Chen, J., Li, P., Zhang, T., Xu, Z., Huang, X., Wang, R., et al. (2021). Review on strategies and technologies for exosome isolation and purification. Front. Bioeng. Biotechnol. 9, 811971. doi:10.3389/fbioe.2021.811971
Chen, W., Sun, Y., Gu, X., Cai, J., Liu, X., Zhang, X., et al. (2021). Conditioned medium of human bone marrow-derived stem cells promotes tendon-bone healing of the rotator cuff in a rat model. Biomaterials 271, 120714. doi:10.1016/j.biomaterials.2021.120714
Chevillet, J. R., Kang, Q., Ruf, I. K., Briggs, H. A., Vojtech, L. N., Hughes, S. M., et al. (2014). Quantitative and stoichiometric analysis of the microRNA content of exosomes. Proc. Natl. Acad. Sci. U. S. A. 111 (41), 14888–14893. doi:10.1073/pnas.1408301111
Chiang, C. Y., and Chen, C. (2019). Toward characterizing extracellular vesicles at a single-particle level. J. Biomed. Sci. 26 (1), 9. doi:10.1186/s12929-019-0502-4
Colombo, M., Moita, C., van Niel, G., Kowal, J., Vigneron, J., Benaroch, P., et al. (2013). Analysis of ESCRT functions in exosome biogenesis, composition and secretion highlights the heterogeneity of extracellular vesicles. J. Cell Sci. 126 (24), 5553–5565. doi:10.1242/jcs.128868
Cortes-Troncoso, J., Jang, S. I., Perez, P., Hidalgo, J., Ikeuchi, T., Greenwell-Wild, T., et al. (2020). T cell exosome-derived miR-142-3p impairs glandular cell function in Sjogren's syndrome. JCI Insight 5 (9), e133497. doi:10.1172/jci.insight.133497
Dismuke, W. M., Challa, P., Navarro, I., Stamer, W. D., and Liu, Y. (2015). Human aqueous humor exosomes. Exp. Eye Res. 132, 73–77. doi:10.1016/j.exer.2015.01.019
Dismuke, W. M., Klingeborn, M., and Stamer, W. D. (2016). Mechanism of fibronectin binding to human trabecular meshwork exosomes and its modulation by dexamethasone. PLoS One 11 (10), e0165326. doi:10.1371/journal.pone.0165326
Doeppner, T. R., Herz, J., Gorgens, A., Schlechter, J., Ludwig, A. K., Radtke, S., et al. (2015). Extracellular vesicles improve post-stroke neuroregeneration and prevent postischemic immunosuppression. Stem Cells Transl. Med. 4 (10), 1131–1143. doi:10.5966/sctm.2015-0078
Doyle, L. M., and Wang, M. Z. (2019). Overview of extracellular vesicles, their origin, composition, purpose, and methods for exosome isolation and analysis. Cells 8 (7), 727. doi:10.3390/cells8070727
EL Andaloussi, S., Mager, I., Breakefield, X. O., and Wood, M. J. (2013). Extracellular vesicles: Biology and emerging therapeutic opportunities. Nat. Rev. Drug Discov. 12 (5), 347–357. doi:10.1038/nrd3978
Elbay, A., Ercan, C., Akbas, F., Bulut, H., and Ozdemir, H. (2019). Three new circulating microRNAs may be associated with wet age-related macular degeneration. Scand. J. Clin. Lab. Invest. 79 (6), 388–394. doi:10.1080/00365513.2019.1637931
Fan, W., Pang, H., Shi, X., Li, J., Wang, Y., Luo, S., et al. (2022). Plasma-derived exosomal mRNA profiles associated with type 1 diabetes mellitus. Front. Immunol. 13, 995610. doi:10.3389/fimmu.2022.995610
Farjood, F., Ahmadpour, A., Ostvar, S., and Vargis, E. (2020). Acute mechanical stress in primary porcine RPE cells induces angiogenic factor expression and in vitro angiogenesis. J. Biol. Eng. 14, 13. doi:10.1186/s13036-020-00235-4
Greening, D. W., Xu, R., Ji, H., Tauro, B. J., and Simpson, R. J. (2015). A protocol for exosome isolation and characterization: Evaluation of ultracentrifugation, density-gradient separation, and immunoaffinity capture methods. Methods Mol. Biol. 1295, 179–209. doi:10.1007/978-1-4939-2550-6_15
Guillamat-Prats, R. (2021). The role of MSC in wound healing, scarring and regeneration. Cells 10 (7), 1729. doi:10.3390/cells10071729
Gurunathan, S., Kang, M. H., Jeyaraj, M., Qasim, M., and Kim, J. H. (2019). Review of the isolation, characterization, biological function, and multifarious therapeutic approaches of exosomes. Cells 8 (4), 307. doi:10.3390/cells8040307
Gurung, S., Perocheau, D., Touramanidou, L., and Baruteau, J. (2021). The exosome journey: From biogenesis to uptake and intracellular signalling. Cell Commun. Signal 19 (1), 47. doi:10.1186/s12964-021-00730-1
Hajrasouliha, A. R., Jiang, G., Lu, Q., Lu, H., Kaplan, H. J., Zhang, H. G., et al. (2013). Exosomes from retinal astrocytes contain antiangiogenic components that inhibit laser-induced choroidal neovascularization. J. Biol. Chem. 288 (39), 28058–28067. doi:10.1074/jbc.M113.470765
Han, K. Y., Dugas-Ford, J., Seiki, M., Chang, J. H., and Azar, D. T. (2015). Evidence for the involvement of MMP14 in MMP2 processing and recruitment in exosomes of corneal fibroblasts. Invest. Ophthalmol. Vis. Sci. 56 (9), 5323–5329. doi:10.1167/iovs.14-14417
Han, K. Y., Tran, J. A., Chang, J. H., Azar, D. T., and Zieske, J. D. (2017). Potential role of corneal epithelial cell-derived exosomes in corneal wound healing and neovascularization. Sci. Rep. 7, 40548. doi:10.1038/srep40548
Hassanpour, M., Rezabakhsh, A., Rezaie, J., Nouri, M., and Rahbarghazi, R. (2020). Exosomal cargos modulate autophagy in recipient cells via different signaling pathways. Cell Biosci. 10, 92. doi:10.1186/s13578-020-00455-7
He, G. H., Zhang, W., Ma, Y. X., Yang, J., Chen, L., Song, J., et al. (2018). Mesenchymal stem cells-derived exosomes ameliorate blue light stimulation in retinal pigment epithelium cells and retinal laser injury by VEGF-dependent mechanism. Int. J. Ophthalmol. 11 (4), 559–566. doi:10.18240/ijo.2018.04.04
Hertsenberg, A. J., Shojaati, G., Funderburgh, M. L., Mann, M. M., Du, Y., and Funderburgh, J. L. (2017). Corneal stromal stem cells reduce corneal scarring by mediating neutrophil infiltration after wounding. PLoS One 12 (3), e0171712. doi:10.1371/journal.pone.0171712
Hiratsuka, I., Yamada, H., Munetsuna, E., Hashimoto, S., and Itoh, M. (2016). Circulating MicroRNAs in graves' disease in relation to clinical activity. Thyroid 26 (10), 1431–1440. doi:10.1089/thy.2016.0062
Huang, C., Fisher, K. P., Hammer, S. S., Navitskaya, S., Blanchard, G. J., and Busik, J. V. (2018). Plasma exosomes contribute to microvascular damage in diabetic retinopathy by activating the classical complement pathway. Diabetes 67 (8), 1639–1649. doi:10.2337/db17-1587
Jafari, D., Shajari, S., Jafari, R., Mardi, N., Gomari, H., Ganji, F., et al. (2020). Designer exosomes: A new platform for Biotechnology therapeutics. BioDrugs 34 (5), 567–586. doi:10.1007/s40259-020-00434-x
Janin-Manificat, H., Rovere, M. R., Galiacy, S. D., Malecaze, F., Hulmes, D. J., Moali, C., et al. (2012). Development of ex vivo organ culture models to mimic human corneal scarring. Mol. Vis. 18, 2896–2908.
Jo, W., Jeong, D., Kim, J., Cho, S., Jang, S. C., Han, C., et al. (2014). Microfluidic fabrication of cell-derived nanovesicles as endogenous RNA carriers. Lab. Chip 14 (7), 1261–1269. doi:10.1039/c3lc50993a
Johnsen, K. B., Gudbergsson, J. M., Skov, M. N., Christiansen, G., Gurevich, L., Moos, T., et al. (2016). Evaluation of electroporation-induced adverse effects on adipose-derived stem cell exosomes. Cytotechnology 68 (5), 2125–2138. doi:10.1007/s10616-016-9952-7
Kalluri, R., and LeBleu, V. S. (2020). The biology, function, and biomedical applications of exosomes. Science 367 (6478), eaau6977. doi:10.1126/science.aau6977
Kamerkar, S., LeBleu, V. S., Sugimoto, H., Yang, S., Ruivo, C. F., Melo, S. A., et al. (2017). Exosomes facilitate therapeutic targeting of oncogenic KRAS in pancreatic cancer. Nature 546 (7659), 498–503. doi:10.1038/nature22341
Kang, G. Y., Bang, J. Y., Choi, A. J., Yoon, J., Lee, W. C., Choi, S., et al. (2014). Exosomal proteins in the aqueous humor as novel biomarkers in patients with neovascular age-related macular degeneration. J. Proteome Res. 13 (2), 581–595. doi:10.1021/pr400751k
Kang, M., Choi, J. K., Jittayasothorn, Y., and Egwuagu, C. E. (2020). Interleukin 35-producing exosomes suppress neuroinflammation and autoimmune uveitis. Front. Immunol. 11, 1051. doi:10.3389/fimmu.2020.01051
Katome, T., Namekata, K., Mitamura, Y., Semba, K., Egawa, M., Naito, T., et al. (2015). Expression of intraocular peroxisome proliferator-activated receptor gamma in patients with proliferative diabetic retinopathy. J. Diabetes Complicat. 29 (2), 275–281. doi:10.1016/j.jdiacomp.2014.10.010
Kim, H. M., and Woo, S. J. (2021). Ocular drug delivery to the retina: Current innovations and future perspectives. Pharmaceutics 13 (1), 108. doi:10.3390/pharmaceutics13010108
Kim, M. S., Haney, M. J., Zhao, Y., Mahajan, V., Deygen, I., Klyachko, N. L., et al. (2016). Development of exosome-encapsulated paclitaxel to overcome MDR in cancer cells. Nanomedicine 12 (3), 655–664. doi:10.1016/j.nano.2015.10.012
Lauwers, E., Wang, Y. C., Gallardo, R., Van der Kant, R., Michiels, E., Swerts, J., et al. (2018). Hsp90 mediates membrane deformation and exosome release. Mol. Cell 71 (5), 689–702.e9. doi:10.1016/j.molcel.2018.07.016
Lee, T. S., Kim, Y., Zhang, W., Song, I. H., and Tung, C. H. (2018). Facile metabolic glycan labeling strategy for exosome tracking. Biochim. Biophys. Acta Gen. Subj. 1862 (5), 1091–1100. doi:10.1016/j.bbagen.2018.02.001
Li, F., Liu, Z., Zhang, B., Jiang, S., Wang, Q., Du, L., et al. (2020). Circular RNA sequencing indicates circ-IQGAP2 and circ-ZC3H6 as noninvasive biomarkers of primary Sjogren's syndrome. Rheumatol. Oxf. 59 (9), 2603–2615. doi:10.1093/rheumatology/keaa163
Li, H., Feng, Y., Zheng, X., Jia, M., Mei, Z., Wang, Y., et al. (2022). M2-type exosomes nanoparticles for rheumatoid arthritis therapy via macrophage re-polarization. J. Control Release 341, 16–30. doi:10.1016/j.jconrel.2021.11.019
Li, N., Zhao, L., Wei, Y., Ea, V. L., Nian, H., and Wei, R. (2019). Recent advances of exosomes in immune-mediated eye diseases. Stem Cell Res. Ther. 10 (1), 278. doi:10.1186/s13287-019-1372-0
Li, P., Kaslan, M., Lee, S. H., Yao, J., and Gao, Z. (2017). Progress in exosome isolation techniques. Theranostics 7 (3), 789–804. doi:10.7150/thno.18133
Li, Q., Wang, H., Peng, H., Huyan, T., and Cacalano, N. A. (2019). Exosomes: Versatile nano mediators of immune regulation. Cancers (Basel) 11 (10), 1557. doi:10.3390/cancers11101557
Liang, Y., Duan, L., Lu, J., and Xia, J. (2021). Engineering exosomes for targeted drug delivery. Theranostics 11 (7), 3183–3195. doi:10.7150/thno.52570
Liang, Y., Jiang, J., Ou, W., Peng, X., Sun, R., Xu, X., et al. (2018). Effect of community screening on the demographic makeup and clinical severity of glaucoma patients receiving care in urban China. Am. J. Ophthalmol. 195, 1–7. doi:10.1016/j.ajo.2018.07.013
Liu, J., Jiang, F., Jiang, Y., Wang, Y., Li, Z., Shi, X., et al. (2020). Roles of exosomes in ocular diseases. Int. J. Nanomedicine 15, 10519–10538. doi:10.2147/IJN.S277190
Liu, S., Fan, M., Xu, J. X., Yang, L. J., Qi, C. C., Xia, Q. R., et al. (2022). Exosomes derived from bone-marrow mesenchymal stem cells alleviate cognitive decline in AD-like mice by improving BDNF-related neuropathology. J. Neuroinflammation 19 (1), 35. doi:10.1186/s12974-022-02393-2
Lu, M., Xing, H., Xun, Z., Yang, T., Zhao, X., Cai, C., et al. (2018). Functionalized extracellular vesicles as advanced therapeutic nanodelivery systems. Eur. J. Pharm. Sci. 121, 34–46. doi:10.1016/j.ejps.2018.05.001
Luo, Z., Lin, J., Sun, Y., Wang, C., and Chen, J. (2021). Bone marrow stromal cell-derived exosomes promote muscle healing following contusion through macrophage polarization. Stem Cells Dev. 30 (3), 135–148. doi:10.1089/scd.2020.0167
Luo, Z., Qi, B., Sun, Y., Chen, Y., Lin, J., Qin, H., et al. (2022a). Engineering bioactive M2 macrophage-polarized, anti-inflammatory, miRNA-based liposomes for functional muscle repair: From exosomal mechanisms to biomaterials. Small 18 (34), e2201957. doi:10.1002/smll.202201957
Luo, Z., Sun, Y., Qi, B., Lin, J., Chen, Y., Xu, Y., et al. (2022b). Human bone marrow mesenchymal stem cell-derived extracellular vesicles inhibit shoulder stiffness via let-7a/Tgfbr1 axis. Bioact. Mater 17, 344–359. doi:10.1016/j.bioactmat.2022.01.016
Luo, Z. W., Sun, Y. Y., Lin, J. R., Qi, B. J., and Chen, J. W. (2021). Exosomes derived from inflammatory myoblasts promote M1 polarization and break the balance of myoblast proliferation/differentiation. World J. Stem Cells 13 (11), 1762–1782. doi:10.4252/wjsc.v13.i11.1762
Ma, S., Yin, J., Hao, L., Liu, X., Shi, Q., Diao, Y., et al. (2022). Exosomes from human umbilical cord mesenchymal stem cells treat corneal injury via autophagy activation. Front. Bioeng. Biotechnol. 10, 879192. doi:10.3389/fbioe.2022.879192
Manukonda, R., Attem, J., Yenuganti, V. R., Kaliki, S., and Vemuganti, G. K. (2022). Exosomes in the visual system: New avenues in ocular diseases. Tumour Biol. 44 (1), 129–152. doi:10.3233/TUB-211543
Mashouri, L., Yousefi, H., Aref, A. R., Ahadi, A. M., Molaei, F., and Alahari, S. K. (2019). Exosomes: Composition, biogenesis, and mechanisms in cancer metastasis and drug resistance. Mol. Cancer 18 (1), 75. doi:10.1186/s12943-019-0991-5
Mazzeo, A., Beltramo, E., Lopatina, T., Gai, C., Trento, M., and Porta, M. (2018). Molecular and functional characterization of circulating extracellular vesicles from diabetic patients with and without retinopathy and healthy subjects. Exp. Eye Res. 176, 69–77. doi:10.1016/j.exer.2018.07.003
McKay, T. B., Hutcheon, A. E. K., Zieske, J. D., and Ciolino, J. B. (2020). Extracellular vesicles secreted by corneal epithelial cells promote myofibroblast differentiation. Cells 9 (5), 1080. doi:10.3390/cells9051080
Mead, B., and Tomarev, S. (2017). Bone marrow-derived mesenchymal stem cells-derived exosomes promote survival of retinal ganglion cells through miRNA-dependent mechanisms. Stem Cells Transl. Med. 6 (4), 1273–1285. doi:10.1002/sctm.16-0428
Minciacchi, V. R., Freeman, M. R., and Di Vizio, D. (2015). Extracellular vesicles in cancer: Exosomes, microvesicles and the emerging role of large oncosomes. Semin. Cell Dev. Biol. 40, 41–51. doi:10.1016/j.semcdb.2015.02.010
Mishra, A., Singh, P., Qayoom, I., Prasad, A., and Kumar, A. (2021). Current strategies in tailoring methods for engineered exosomes and future avenues in biomedical applications. J. Mater Chem. B 9 (32), 6281–6309. doi:10.1039/d1tb01088c
Noonin, C., and Thongboonkerd, V. (2021). Exosome-inflammasome crosstalk and their roles in inflammatory responses. Theranostics 11 (9), 4436–4451. doi:10.7150/thno.54004
Ogata, N., Imaizumi, M., Nomura, S., Shozu, A., Arichi, M., Matsuoka, M., et al. (2005). Increased levels of platelet-derived microparticles in patients with diabetic retinopathy. Diabetes Res. Clin. Pract. 68 (3), 193–201. doi:10.1016/j.diabres.2004.10.010
Pakravan, K., Babashah, S., Sadeghizadeh, M., Mowla, S. J., Mossahebi-Mohammadi, M., Ataei, F., et al. (2017). MicroRNA-100 shuttled by mesenchymal stem cell-derived exosomes suppresses in vitro angiogenesis through modulating the mTOR/HIF-1α/VEGF signaling axis in breast cancer cells. Cell Oncol. (Dordr) 40 (5), 457–470. doi:10.1007/s13402-017-0335-7
Pan, B. T., and Johnstone, R. M. (1983). Fate of the transferrin receptor during maturation of sheep reticulocytes in vitro: Selective externalization of the receptor. Cell 33 (3), 967–978. doi:10.1016/0092-8674(83)90040-5
Pan, D., Chang, X., Xu, M., Zhang, M., Zhang, S., Wang, Y., et al. (2019). UMSC-derived exosomes promote retinal ganglion cells survival in a rat model of optic nerve crush. J. Chem. Neuroanat. 96, 134–139. doi:10.1016/j.jchemneu.2019.01.006
Phinney, D. G., and Pittenger, M. F. (2017). Concise review: MSC-derived exosomes for cell-free therapy. Stem Cells 35 (4), 851–858. doi:10.1002/stem.2575
Pollalis, D., Kim, D., Nair, G. K. G., Kang, C., Nanda, A. V., and Lee, S. Y. (2022). Intraocular RGD-engineered exosomes and active targeting of choroidal neovascularization (CNV). Cells 11 (16), 2573. doi:10.3390/cells11162573
Ragusa, M., Barbagallo, C., Statello, L., Caltabiano, R., Russo, A., Puzzo, L., et al. (2015). miRNA profiling in vitreous humor, vitreal exosomes and serum from uveal melanoma patients: Pathological and diagnostic implications. Cancer Biol. Ther. 16 (9), 1387–1396. doi:10.1080/15384047.2015.1046021
Sacca, S. C., Centofanti, M., and Izzotti, A. (2012). New proteins as vascular biomarkers in primary open angle glaucomatous aqueous humor. Invest. Ophthalmol. Vis. Sci. 53 (7), 4242–4253. doi:10.1167/iovs.11-8902
Sadeghzadeh, S., Dehghani Ashkezari, M., Seifati, S. M., Vahidi Mehrjardi, M. Y., Dehghan Tezerjani, M., Sadeghzadeh, S., et al. (2020). <p>Circulating miR-15a and miR-222 as Potential Biomarkers of Type 2 Diabetes</p>. Diabetes Metab. Syndr. Obes. 13, 3461–3469. doi:10.2147/DMSO.S263883
Safwat, A., Sabry, D., Ragiae, A., Amer, E., Mahmoud, R. H., and Shamardan, R. M. (2018). Adipose mesenchymal stem cells-derived exosomes attenuate retina degeneration of streptozotocin-induced diabetes in rabbits. J. Circ. Biomark. 7, 184945441880782. doi:10.1177/1849454418807827
Samaeekia, R., Rabiee, B., Putra, I., Shen, X., Park, Y. J., Hematti, P., et al. (2018). Effect of human corneal mesenchymal stromal cell-derived exosomes on corneal epithelial wound healing. Invest. Ophthalmol. Vis. Sci. 59 (12), 5194–5200. doi:10.1167/iovs.18-24803
Sato, Y. T., Umezaki, K., Sawada, S., Mukai, S. A., Sasaki, Y., Harada, N., et al. (2016). Engineering hybrid exosomes by membrane fusion with liposomes. Sci. Rep. 6, 21933. doi:10.1038/srep21933
Seyedrazizadeh, S. Z., Poosti, S., Nazari, A., Alikhani, M., Shekari, F., Pakdel, F., et al. (2020). Extracellular vesicles derived from human ES-MSCs protect retinal ganglion cells and preserve retinal function in a rodent model of optic nerve injury. Stem Cell Res. Ther. 11 (1), 203. doi:10.1186/s13287-020-01702-x
Shojaati, G., Khandaker, I., Funderburgh, M. L., Mann, M. M., Basu, R., Stolz, D. B., et al. (2019). Mesenchymal stem cells reduce corneal fibrosis and inflammation via extracellular vesicle-mediated delivery of miRNA. Stem Cells Transl. Med. 8 (11), 1192–1201. doi:10.1002/sctm.18-0297
Skotland, T., Hessvik, N. P., Sandvig, K., and Llorente, A. (2019). Exosomal lipid composition and the role of ether lipids and phosphoinositides in exosome biology. J. Lipid Res. 60 (1), 9–18. doi:10.1194/jlr.R084343
Stamer, W. D., Hoffman, E. A., Luther, J. M., Hachey, D. L., and Schey, K. L. (2011). Protein profile of exosomes from trabecular meshwork cells. J. Proteomics 74 (6), 796–804. doi:10.1016/j.jprot.2011.02.024
Stevanato, L., Thanabalasundaram, L., Vysokov, N., and Sinden, J. D. (2016). Investigation of content, stoichiometry and transfer of miRNA from human neural stem cell line derived exosomes. PLoS One 11 (1), e0146353. doi:10.1371/journal.pone.0146353
Stickney, Z., Losacco, J., McDevitt, S., Zhang, Z., and Lu, B. (2016). Development of exosome surface display technology in living human cells. Biochem. Biophys. Res. Commun. 472 (1), 53–59. doi:10.1016/j.bbrc.2016.02.058
Sun, Y., Luo, Z., Chen, Y., Lin, J., Zhang, Y., Qi, B., et al. (2022). si-Tgfbr1-loading liposomes inhibit shoulder capsule fibrosis via mimicking the protective function of exosomes from patients with adhesive capsulitis. Biomater. Res. 26 (1), 39. doi:10.1186/s40824-022-00286-2
Sun Y., Y., Sun, X., Liu, S., Liu, L., and Chen, J. (2018). The overlap between regeneration and fibrosis in injured skeletal muscle is regulated by phosphatidylinositol 3-kinase/Akt signaling pathway - a bioinformatic analysis based on lncRNA microarray. Gene 672, 79–87. doi:10.1016/j.gene.2018.06.001
Sun, Z., Yang, S., Zhou, Q., Wang, G., Song, J., Li, Z., et al. (2018). Emerging role of exosome-derived long non-coding RNAs in tumor microenvironment. Mol. Cancer 17 (1), 82. doi:10.1186/s12943-018-0831-z
Tabak, S., Schreiber-Avissar, S., and Beit-Yannai, E. (2018). Extracellular vesicles have variable dose-dependent effects on cultured draining cells in the eye. J. Cell Mol. Med. 22 (3), 1992–2000. doi:10.1111/jcmm.13505
Tang, Q., Lu, B., He, J., Chen, X., Fu, Q., Han, H., et al. (2022). Exosomes-loaded thermosensitive hydrogels for corneal epithelium and stroma regeneration. Biomaterials 280, 121320. doi:10.1016/j.biomaterials.2021.121320
Thery, C., Witwer, K. W., Aikawa, E., Alcaraz, M. J., Anderson, J. D., Andriantsitohaina, R., et al. (2018). Minimal information for studies of extracellular vesicles 2018 (MISEV2018): A position statement of the international society for extracellular vesicles and update of the MISEV2014 guidelines. J. Extracell. Vesicles 7 (1), 1535750. doi:10.1080/20013078.2018.1535750
Tian, T., Zhang, H. X., He, C. P., Fan, S., Zhu, Y. L., Qi, C., et al. (2018). Surface functionalized exosomes as targeted drug delivery vehicles for cerebral ischemia therapy. Biomaterials 150, 137–149. doi:10.1016/j.biomaterials.2017.10.012
Toh, W. S., Lai, R. C., Zhang, B., and Lim, S. K. (2018). MSC exosome works through a protein-based mechanism of action. Biochem. Soc. Trans. 46 (4), 843–853. doi:10.1042/BST20180079
Tokarz, A., Szuscik, I., Kusnierz-Cabala, B., Kapusta, M., Konkolewska, M., Zurakowski, A., et al. (2015). Extracellular vesicles participate in the transport of cytokines and angiogenic factors in diabetic patients with ocular complications. Folia Med. Cracov 55 (4), 35–48.
Tong, Y., Zhou, Y. L., Wang, Y. X., Zhao, P. Q., and Wang, Z. Y. (2016). Retinal pigment epithelium cell-derived exosomes: Possible relevance to CNV in wet-age related macular degeneration. Med. Hypotheses 97, 98–101. doi:10.1016/j.mehy.2016.10.027
Trajkovic, K., Hsu, C., Chiantia, S., Rajendran, L., Wenzel, D., Wieland, F., et al. (2008). Ceramide triggers budding of exosome vesicles into multivesicular endosomes. Science 319 (5867), 1244–1247. doi:10.1126/science.1153124
van der Meel, R., Fens, M. H., Vader, P., van Solinge, W. W., Eniola-Adefeso, O., and Schiffelers, R. M. (2014). Extracellular vesicles as drug delivery systems: Lessons from the liposome field. J. Control Release 195, 72–85. doi:10.1016/j.jconrel.2014.07.049
Van Deun, J., Mestdagh, P., Sormunen, R., Cocquyt, V., Vermaelen, K., Vandesompele, J., et al. (2014). The impact of disparate isolation methods for extracellular vesicles on downstream RNA profiling. J. Extracell. Vesicles 3, 24858. doi:10.3402/jev.v3.24858
van Niel, G., Charrin, S., Simoes, S., Romao, M., Rochin, L., Saftig, P., et al. (2011). The tetraspanin CD63 regulates ESCRT-independent and -dependent endosomal sorting during melanogenesis. Dev. Cell 21 (4), 708–721. doi:10.1016/j.devcel.2011.08.019
Vidal-Sanz, M., Salinas-Navarro, M., Nadal-Nicolas, F. M., Alarcon-Martinez, L., Valiente-Soriano, F. J., de Imperial, J. M., et al. (2012). Understanding glaucomatous damage: Anatomical and functional data from ocular hypertensive rodent retinas. Prog. Retin Eye Res. 31 (1), 1–27. doi:10.1016/j.preteyeres.2011.08.001
Wan, Y., Wang, L., Zhu, C., Zheng, Q., Wang, G., Tong, J., et al. (2018). Aptamer-conjugated extracellular nanovesicles for targeted drug delivery. Cancer Res. 78 (3), 798–808. doi:10.1158/0008-5472.CAN-17-2880
Wang, A. L., Lukas, T. J., Yuan, M., Du, N., Tso, M. O., and Neufeld, A. H. (2009). Autophagy and exosomes in the aged retinal pigment epithelium: Possible relevance to drusen formation and age-related macular degeneration. PLoS One 4 (1), e4160. doi:10.1371/journal.pone.0004160
Wang, Z., Mo, H., He, Z., Chen, A., and Cheng, P. (2022). Extracellular vesicles as an emerging drug delivery system for cancer treatment: Current strategies and recent advances. Biomed. Pharmacother. 153, 113480. doi:10.1016/j.biopha.2022.113480
Wei, H., Chen, Q., Lin, L., Sha, C., Li, T., Liu, Y., et al. (2021). Regulation of exosome production and cargo sorting. Int. J. Biol. Sci. 17 (1), 163–177. doi:10.7150/ijbs.53671
Weng, Y., Liu, J., Jin, S., Guo, W., Liang, X., and Hu, Z. (2017). Nanotechnology-based strategies for treatment of ocular disease. Acta Pharm. Sin. B 7 (3), 281–291. doi:10.1016/j.apsb.2016.09.001
Whitcher, J. P., Srinivasan, M., and Upadhyay, M. P. (2001). Corneal blindness: A global perspective. Bull. World Health Organ 79 (3), 214–221.
Wilson, S. L., El Haj, A. J., and Yang, Y. (2012). Control of scar tissue formation in the cornea: Strategies in clinical and corneal tissue engineering. J. Funct. Biomater. 3 (3), 642–687. doi:10.3390/jfb3030642
Xiong, Y., Chen, L., Yan, C., Zhou, W., Endo, Y., Liu, J., et al. (2020). Circulating exosomal miR-20b-5p inhibition restores Wnt9b signaling and reverses diabetes-associated impaired wound healing. Small 16 (3), e1904044. doi:10.1002/smll.201904044
Xu, M., Yang, Q., Sun, X., and Wang, Y. (2020). Recent advancements in the loading and modification of therapeutic exosomes. Front. Bioeng. Biotechnol. 8, 586130. doi:10.3389/fbioe.2020.586130
Xu, W., Wu, Y., Hu, Z., Sun, L., Dou, G., Zhang, Z., et al. (2019). Exosomes from microglia attenuate photoreceptor injury and neovascularization in an animal model of retinopathy of prematurity. Mol. Ther. Nucleic Acids 16, 778–790. doi:10.1016/j.omtn.2019.04.029
Yanez-Mo, M., Siljander, P. R., Andreu, Z., Zavec, A. B., Borras, F. E., Buzas, E. I., et al. (2015). Biological properties of extracellular vesicles and their physiological functions. J. Extracell. Vesicles 4, 27066. doi:10.3402/jev.v4.27066
Yao, Y., Sun, W., Sun, Q., Jing, B., Liu, S., Liu, X., et al. (2019). Platelet-derived exosomal MicroRNA-25-3p inhibits coronary vascular endothelial cell inflammation through Adam10 via the NF-κB signaling pathway in ApoE−/− mice. Front. Immunol. 10, 2205. doi:10.3389/fimmu.2019.02205
Yeung, V., Zhang, T. C., Yuan, L., Parekh, M., Cortinas, J. A., Delavogia, E., et al. (2022). Extracellular vesicles secreted by corneal myofibroblasts promote corneal epithelial cell migration. Int. J. Mol. Sci. 23 (6), 3136. doi:10.3390/ijms23063136
Yu, Z., Hao, R., Du, J., Wu, X., Chen, X., Zhang, Y., et al. (2022). A human cornea-on-a-chip for the study of epithelial wound healing by extracellular vesicles. iScience 25 (5), 104200. doi:10.1016/j.isci.2022.104200
Zhang, H., Liu, J., Qu, D., Wang, L., Wong, C. M., Lau, C. W., et al. (2018). Serum exosomes mediate delivery of arginase 1 as a novel mechanism for endothelial dysfunction in diabetes. Proc. Natl. Acad. Sci. U. S. A. 115 (29), E6927–E6936. doi:10.1073/pnas.1721521115
Zhang, J., Ji, C., Zhang, H., Shi, H., Mao, F., Qian, H., et al. (2022). Engineered neutrophil-derived exosome-like vesicles for targeted cancer therapy. Sci. Adv. 8 (2), eabj8207. doi:10.1126/sciadv.abj8207
Zhang, W., Ma, Y., Zhang, Y., Yang, J., He, G., and Chen, S. (2019a). Photo-oxidative blue-light stimulation in retinal pigment epithelium cells promotes exosome secretion and increases the activity of the NLRP3 inflammasome. Curr. Eye Res. 44 (1), 67–75. doi:10.1080/02713683.2018.1518458
Zhang, W., Wang, Y., and Kong, Y. (2019b). Exosomes derived from mesenchymal stem cells modulate miR-126 to ameliorate hyperglycemia-induced retinal inflammation via targeting HMGB1. Invest. Ophthalmol. Vis. Sci. 60 (1), 294–303. doi:10.1167/iovs.18-25617
Zhang, X., Zhang, H., Gu, J., Zhang, J., Shi, H., Qian, H., et al. (2021). Engineered extracellular vesicles for cancer therapy. Adv. Mater 33 (14), e2005709. doi:10.1002/adma.202005709
Zhang Y., Y., Liu, Y., Liu, H., and Tang, W. H. (2019). Exosomes: Biogenesis, biologic function and clinical potential. Cell Biosci. 9, 19. doi:10.1186/s13578-019-0282-2
Keywords: exosome, extracellular vesicle, stem cell, ocular disease, engineered exosome, treatment
Citation: Feng X, Peng Z, Yuan L, Jin M, Hu H, Peng X, Wang Y, Zhang C, Luo Z and Liao H (2023) Research progress of exosomes in pathogenesis, diagnosis, and treatment of ocular diseases. Front. Bioeng. Biotechnol. 11:1100310. doi: 10.3389/fbioe.2023.1100310
Received: 16 November 2022; Accepted: 10 January 2023;
Published: 24 January 2023.
Edited by:
Qihui Zhou, Qingdao University, ChinaReviewed by:
Wei Seong Toh, National University of Singapore, SingaporeCopyright © 2023 Feng, Peng, Yuan, Jin, Hu, Peng, Wang, Zhang, Luo and Liao. This is an open-access article distributed under the terms of the Creative Commons Attribution License (CC BY). The use, distribution or reproduction in other forums is permitted, provided the original author(s) and the copyright owner(s) are credited and that the original publication in this journal is cited, in accordance with accepted academic practice. No use, distribution or reproduction is permitted which does not comply with these terms.
*Correspondence: Hongfei Liao, bGhmemZAMTI2LmNvbQ==; Zhiwen Luo, emhpd2VuLmx1b19mdWRhbkBob3RtYWlsLmNvbQ==
†These authors have contributed equally to this work and share first authorship
Disclaimer: All claims expressed in this article are solely those of the authors and do not necessarily represent those of their affiliated organizations, or those of the publisher, the editors and the reviewers. Any product that may be evaluated in this article or claim that may be made by its manufacturer is not guaranteed or endorsed by the publisher.
Research integrity at Frontiers
Learn more about the work of our research integrity team to safeguard the quality of each article we publish.