- 1Department of Medicine and Medical Specialties, Faculty of Health Sciences, Universidad Alcalá de Henares, Madrid, Spain
- 2Department of Surgery, Instituto de Investigación Biomédica de Salamanca (IBSAL), University of Salamanca, Salamanca, Spain
- 3Department of Dentistry, Universidad Federico Henríquez y Carvajal, Santo Domingo, Dominican Republic
- 4Faculty of Dentistry, Universidad Alfonso X El Sabio, Madrid, Spain
Titanium and alloy osseointegrated implants are used to replace missing teeth; however, some fail and are removed. Modifications of the implant surface with biologically active substances have been proposed. MEDLINE [via Pubmed], Embase and Web of Science were searched with the terms “titanium dental implants”, “surface properties”, “bioactive surface modifications”, “biomolecules”, “BMP”, “antibacterial agent”, “peptide”, “collagen”, “grown factor”, “osseointegration”, “bone apposition”, “osteogenic”, “osteogenesis”, “new bone formation”, “bone to implant contact”, “bone regeneration” and “in vivo studies”, until May 2022. A total of 10,697 references were iden-tified and 26 were included to analyze 1,109 implants, with follow-ups from 2 to 84 weeks. The ARRIVE guidelines and the SYRCLE tool were used to evaluate the methodology and scientific evidence. A meta-analysis was performed (RevMan 2020 software, Cochane Collaboration) with random effects that evaluated BIC at 4 weeks, with subgroups for the different coatings. The heterogeneity of the pooled studies was very high (95% CI, I2 = 99%). The subgroup of BMPs was the most favorable to coating. Surface modification of Ti implants by organic bioactive molecules seems to favor osseointegration in the early stages of healing, but long-term studies are necessary to corroborate the results of the experimental studies.
1 Introduction
Since the introduction of dental implants by Brånemark in the 1960s, titanium (Ti) and some Ti alloys (Ti6Al4V) have been used in edentulous patients to replace missing teeth (Osman and Swain, 2015), their long-term success depending mainly on their osseointegration. However, despite the high success rates recorded, some of them have to be removed due to failure (Moraschini et al., 2015; Alghamdi and Jansen, 2020).
Recently, the attention of researchers has been focused on chemical and topographical modifications of dental implant surfaces and surface coatings with biologically active materials (Le Guéhennec et al., 2007).
These materials, in addition to provoking a response in living tissue, would have the capacity to achieve a faster, higher quality and more durable osseointegration, reducing the waiting time for prosthetic rehabilitations and solving the problems of poor bone quality (Stanford, 2008). Currently, bioceramics, ions and biomolecules are applied for bioactive purposes (Ellingsen et al., 2004; Cooper et al., 2006; Badr and Hadary, 2007; Zagury et al., 2007). The latter include biomacromolecules (lipids, proteins, polynucleic acids and polysaccharides) and biomicromolecules (oligopeptides, deoxyribonucleotides, amino acids, monosaccharides and metabolic products), which are of extraordinary importance for physiological processes and homeostasis (Fischer et al., 2020).
The ability to adhere to bone tissue and the chemical similarity with this tissue have led to great interest in calcium phosphate (CaP) coatings on the surface of implants, precisely because they increase the biochemical anchorage between the bone and the surface materials (Bosco et al., 2013). Similarly, protein coatings have been used in recent years because they accelerate the bone regeneration process at the bone-implant interface and improve osseointegration (Raphel et al., 2016).
Bone morphogenetic protein (BMP) and collagen have been proposed as bone regeneration stimulating materials. Collagen is an important component in bone composition, leading to increased tissue vascularization and decreased inflammation by curbing macrophage and osteoclast activity (Lee et al., 2014). In turn, BMPs play an important role in osteogenesis by regulating the differentiation of bone mesenchymal stem cells (MSCs) and osteogenic cells (Dolanmaz et al., 2015a).
Synthetic peptides have been shown to stimulate bone formation by enhancing the binding of osteoblast cell adhesion receptors (e.g., integrins, selectins, and cadherins). Binding of osteoblast integrin receptors to these bioactive molecules stimulates their interaction with their extracellular matrix (ECM) and promotes cell proliferation and mineralization (Garcia and Reyes, 2005).
Studies have shown that biofunctionalization of implant surfaces with biomimetic peptides would result in a greater increase in the bone-to-implant contact surface (BIC) and an increase in bone density around the implant (Lutz et al., 2010a). However, the process of peptide immobilization on Ti implant surfaces can be a complex process, despite the fact that, in recent years, specific methods have been developed to achieve this goal (Narai and Nagahata, 2003; Russell et al., 2008; Viera-Negron et al., 2008). Also, it has been observed that the biological activity of certain peptides would be reduced by the immobilization process. The surface density, together with the length of the spacers and the orientation, would condition the bactericidal effect of the peptides (Giro et al., 2008). Moussa and Aparicio demonstrated in vitro that bacterial abundance on peptide-coated hydroxyapatite (HA) discs was significantly lower than in controls (Andrea et al., 2018a). Makihira et al. tested in edentulous dog mandibles, the osseointegrative capacity of Ti implants coated with a histatin-derived peptide, demonstrating, by histological and micro-CT analysis, increased trabecular bone formation around the coated implants (Riool et al., 2017). Their observations suggest that antimicrobial peptides on Ti implants would decrease bacterial colonization on the implant surface and facilitate osseointegration (Silva et al., 2016; Zhang et al., 2018).
Despite the existence in the literature of reviews to evaluate the effects of different implant surface modifications on peri-implant bone formation and osseointegration (Makihira et al., 2011; Andrea et al., 2018b; Moussa and Aparicio, 2020; Siwakul et al., 2021) and the known benefit on osseointegration of the use of bioactive molecules (Junker et al., 2009), we have not found meta-analyses that investigate the results in depth, so the aim of our study was to evaluate the role and efficacy of bioactive surfaces on osseointegration. Our meta-analysis limited the research interest to titanium dental implants coated with biomolecules, i.e. organic molecules produced by a living organism.
2 Materials and methods
2.1 Registration
This systematic review was registered at INPLASY, registration number INPLASY202260076.
2.2 PICOS and focused question
Supplementary Table S1: PRISMA Checklist]. According to the PRISMA guidelines for Systematic Reviews and Meta-Analyses (Hutton et al., 2016), a specific question was formulated based on the PICOS principle (Participants, Interventions, Control, Outcomes, and Study Design). The focused question was, “Does the bioactive surface of titanium dental implants, based on biomolecules, influence osseointegration?“.
P) Participants: Subjects received endosseous implantation.
I) Interventions: Implants with incorporated bioactive surfaces based on biomolecules.
C) Control: Implants with conventional etched surfaces (SLA type).
O) Outcome: Bone to Implant Contact (BIC).
S) Study design: Preclinical studies in unmodified experimental animal models.
2.3 Search strategy
The electronic databases PubMed/MEDLINE, WOS and EMBASE were searched until May 2022, with the terms Medical Subject Headings (MeSH): “titanium dental implants”, “surface properties”, “bioactive surface modifications”, “biomolecules”, “BMP”, “antibacterial agent”, “peptide”, “collagen”, “grown factor”, in combination with “osseointegration”, “bone apposition”, “osteogenic”, “osteogenesis”, “new bone formation”, “bone to implant contact”, “bone regeneration” and “in vivo studies”. The Boolean operators AND/OR were used to refine the search. In addition, relevant studies in the gray literature and reference lists of included studies were also examined (cross-referenced). The search strategy and the PICOS strategy are shown in Table 1.
2.4 Inclusion and exclusion criteria
2.4.1 Inclusion criteria
1) Studies regarding Ti implant surfaces coated with biomolecules; b) Studies reporting evaluation of the effect of biomolecular coatings on bone formation or osseointegration; 3) Studies published in English.
2.4.2 Exclusion criteria
1) In vitro studies; b) Studies using modified animals; 3) Narrative reviews and systematic reviews; 4) Irrelevant and duplicate studies and those that did not meet the established inclusion criteria.
2.5 Data extraction and analysis
Studies that did not refer to the research question were eliminated and only the titles and abstracts of the selected articles were considered and entered into an Excel spreadsheet. Two reviewers (N.L.-V. and A.L.-V.) selected the titles and abstracts independently. Discrepancies between the two reviewers were discussed until a consensus was reached for inclusion of the studies. The full texts of the selected studies were then obtained for inclusion and analysis.
2.6 Risk of bias of included articles
An adapted version of the Cochrane RoB tool with specific biases in animal studies (SYRCLE) was used to assess the scientific evidence in all selected studies (Hooijmans et al., 2014).
2.7 Quality of the reports of the included studies
Two reviewers N.L.-V. and A.L.-V evaluated the included studies according to the ARRIVE (Animal Research: Reporting of In Vivo Experiments) guidelines (Stadlinger et al., 2012a), which include a total of 23 items. Each item was scored by 0 (not reported) or 1 (reported), with a complete count of all included studies.
2.8 Statistical analysis
The meta-analysis was performed using RevMan software [Review Manager (RevMan) (Computer program). Version 5.4.1, The Cochrane Collaboration, 2020].
A meta-analysis based on Odds Ratio (OR) with 95% confidence intervals (CI) was performed for adverse event outcomes. Mean difference (MD) and standard deviation (SD) were used to estimate effect size. The random-effects model was selected because of the expected methodological heterogeneity in the included studies; furthermore, heterogeneity was interpreted as significant when the I2 value was >50%. The threshold for statistical significance was defined as p < 0.05. A funnel plot was used to assess publication bias.
3 Results
3.1 Selection and description of the studies
Among the available literature, three categories of biomolecular coatings have been evaluated in this review: 1) peptides, 2) BMPs and 3) ECM. The initial electronic search yielded 10,697 references. After eliminating duplicates and irrelevant articles based on their title and abstracts, 84 articles were selected, of which, after eliminating those that did not meet inclusion criteria (in vitro studies, systematic reviews, modified animals...), 26 full texts were selected (Anitua, 2006; Germanier et al., 2006; Wikesjö et al., 2008a; Wikesjö et al., 2008b; Wikesjö et al., 2008c; Stadlinger et al., 2008; Anitua et al., 2009; Barros et al., 2009; Ishibe et al., 2009; Yang et al., 2009; Lutz et al., 2010b; Polimeni et al., 2010; Susin et al., 2010; Ramazanoglu et al., 2011; Stadlinger et al., 2012b; Sverzut et al., 2012; Jiang et al., 2013; Cecconi et al., 2014; Korn et al., 2014; Kim et al., 2015; Yoo et al., 2015; Cardoso et al., 2017; Bae et al., 2018; Cho et al., 2019; Cho et al., 2021; Pang et al., 2021). The concordance between reviewers (N.L-V., A.L-V.) was 100% with a Cohen’s kappa index of 1 (total concordance). (Figure 1. Flow Diagram).
Table 2 provides the evaluation of the ARRIVE criteria in animal studies, with a mean rating of 16.5 ± 1.5. All studies provided adequate information in terms of title, abstract, introduction, ethical statement, species, surgical procedure, outcome assessment and statistical analysis. Items 5 (Rationale for animal models), 19 (3Rs, Replace, Reduce and Refine), 20 (Adverse events), were not reported in any of the included studies. Item 11 (Accommodation and handling of animals) was reported by only five studies (Anitua, 2006; Anitua et al., 2009; Ishibe et al., 2009; Lutz et al., 2010b; Korn et al., 2014) and item 21 (Study limitations) was reported by six studies (Jiang et al., 2013; Korn et al., 2014; Kim et al., 2015; Yoo et al., 2015; Cardoso et al., 2017; Bae et al., 2018).
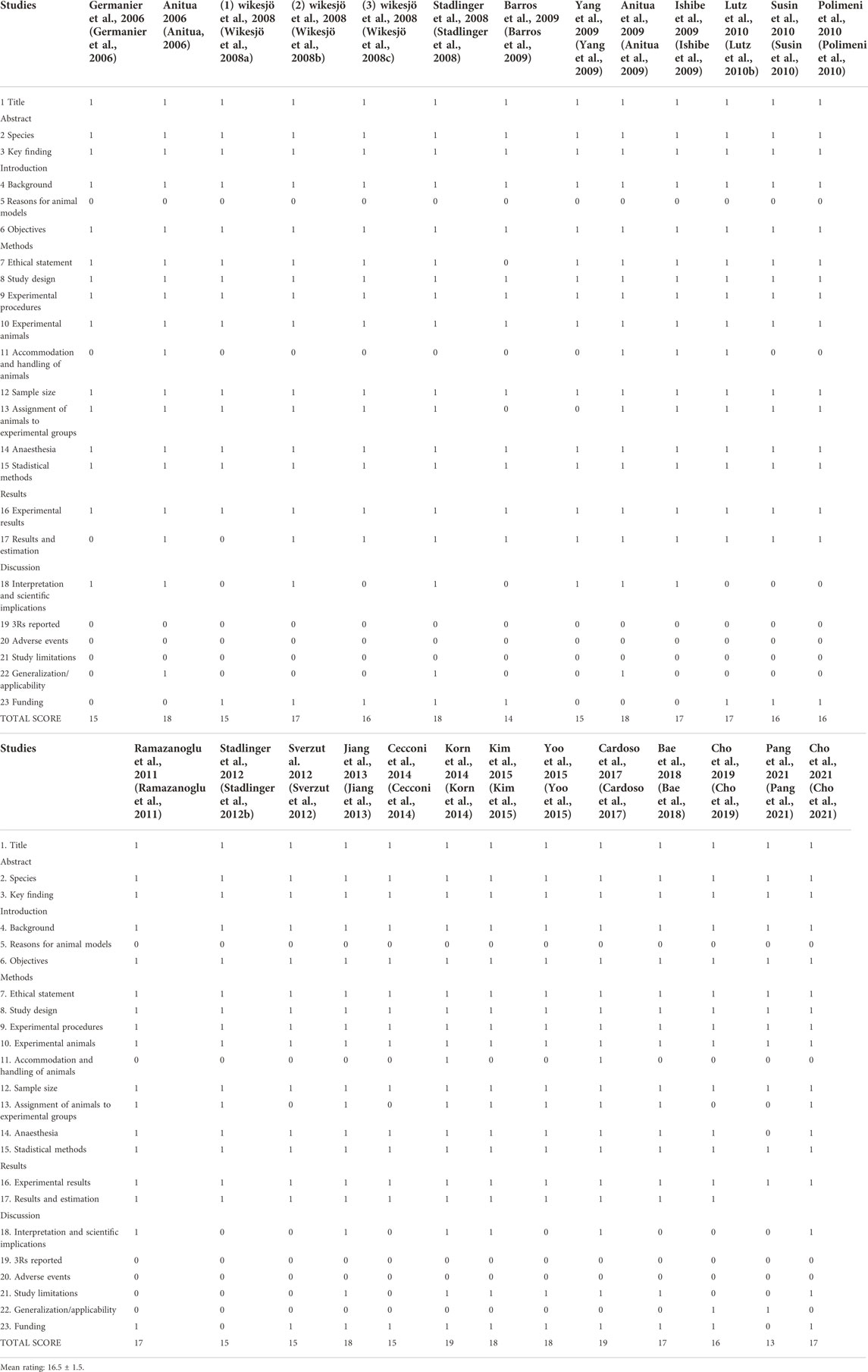
TABLE 2. Checklist of ARRIVE criteria reported by the included studies. Each item was judged as “0” (not reported) or “1” (reported).
3.2 Risk of bias assessment
The Random sequence generation domain was the most frequently mentioned (60%). Blinding of participants and personnel and Blinding of outcome assessment were the least mentioned domains. The domains Incomplete outcome data and Selective reporting were the least clear. The lack of information resulted in a high and unclear risk of bias for most of the included studies (Figure 2).
3.3 Characteristics of the included studies
Qualitative synthesis. A total of 1,109 implants were evaluated. Most of the studies employed commercial Ti and Ti alloy implant models, with the exception of two studies in rat tibias (Ishibe et al., 2009; Bae et al., 2018) that used rods and microscrews, respectively. The implants featured either a re-coated or uncoated surface with peptides in five studies (Germanier et al., 2006; Barros et al., 2009; Yang et al., 2009; Lutz et al., 2010b; Cho et al., 2019), BMPs in fourteen studies (Anitua, 2006; Wikesjö et al., 2008a; Wikesjö et al., 2008b; Wikesjö et al., 2008c; Anitua et al., 2009; Ishibe et al., 2009; Polimeni et al., 2010; Susin et al., 2010; Ramazanoglu et al., 2011; Kim et al., 2015; Yoo et al., 2015; Cardoso et al., 2017; Pang et al., 2021), or ECM products in seven studies (Stadlinger et al., 2008; Stadlinger et al., 2012b; Sverzut et al., 2012; Cecconi et al., 2014; Korn et al., 2014; Bae et al., 2018; Cho et al., 2019). Follow-up periods ranged from 2 to 16 weeks, except for the study by Bae et al. (Bae et al., 2018) that the follow-up period was extended to 84 weeks. The most commonly used experimental models were the dog (Wikesjö et al., 2008a; Wikesjö et al., 2008b; Barros et al., 2009; Polimeni et al., 2010; Susin et al., 2010; Sverzut et al., 2012; Kim et al., 2015; Cho et al., 2019) and the pig (Germanier et al., 2006; Stadlinger et al., 2008; Lutz et al., 2010b; Susin et al., 2010; Ramazanoglu et al., 2011; Stadlinger et al., 2012b; Korn et al., 2014; Cardoso et al., 2017). The jaw and tibia were the most commonly used bones for implantation and all included studies evaluated the BIC; six studies evaluated BA (Yang et al., 2009; Sverzut et al., 2012; Yoo et al., 2015; Cho et al., 2019; Cho et al., 2021; Pang et al., 2021) and nine studies evaluated BD (Wikesjö et al., 2008b; Wikesjö et al., 2008c; Stadlinger et al., 2008; Barros et al., 2009; Lutz et al., 2010b; Polimeni et al., 2010; Susin et al., 2010; Ramazanoglu et al., 2011; Korn et al., 2014). The main characteristics of the studies are shown in the tables below (Tables 3–5).
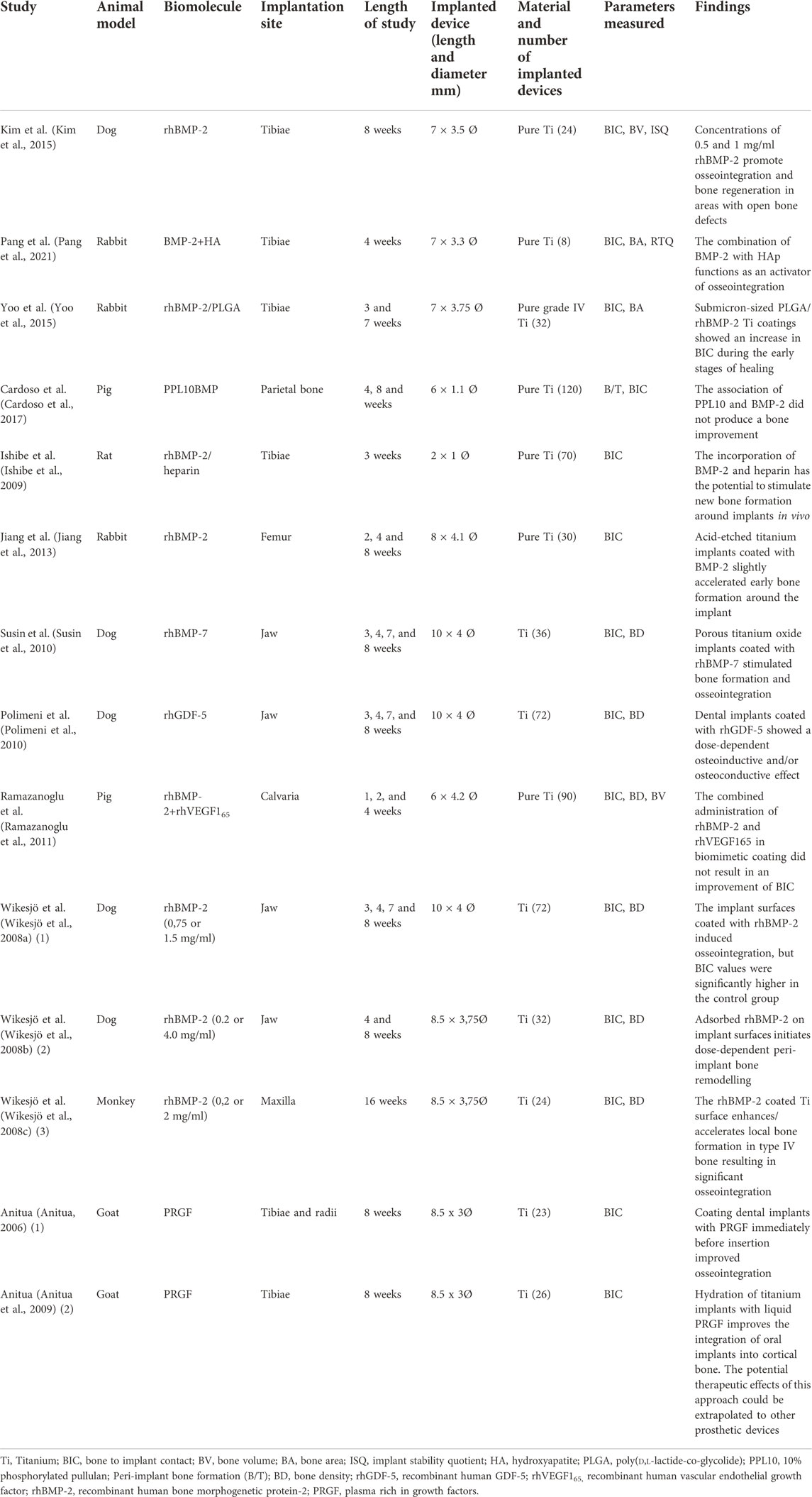
TABLE 4. Surface modification with Bone Morphogenetic Proteins (BMPs). Characteristics of the included studies.
3.4 Quantitative synthesis (meta-analysis)
The same studies included in the qualitative synthesis were used to perform a meta-analysis comparing Ti implants coated with different biomolecules, with Ti implants etched. Meta-analysis of adverse outcomes could not be performed due to lack of data. All included studies (Anitua, 2006; Germanier et al., 2006; Wikesjö et al., 2008a; Wikesjö et al., 2008b; Wikesjö et al., 2008c; Stadlinger et al., 2008; Anitua et al., 2009; Barros et al., 2009; Ishibe et al., 2009; Yang et al., 2009; Lutz et al., 2010b; Polimeni et al., 2010; Susin et al., 2010; Ramazanoglu et al., 2011; Stadlinger et al., 2012b; Sverzut et al., 2012; Jiang et al., 2013; Cecconi et al., 2014; Korn et al., 2014; Kim et al., 2015; Yoo et al., 2015; Cardoso et al., 2017; Bae et al., 2018; Cho et al., 2019; Cho et al., 2021; Pang et al., 2021) evaluated bone-to-implant contact (BIC), using measurement 4 weeks after placement. The heterogeneity of the grouped studies was very high (I2 = 99%) (Figure 3). Only one result favorable to coating, was found in the BMPs subgroup. Analysis of the grouped studies showed no significant differences between coatings and controls.
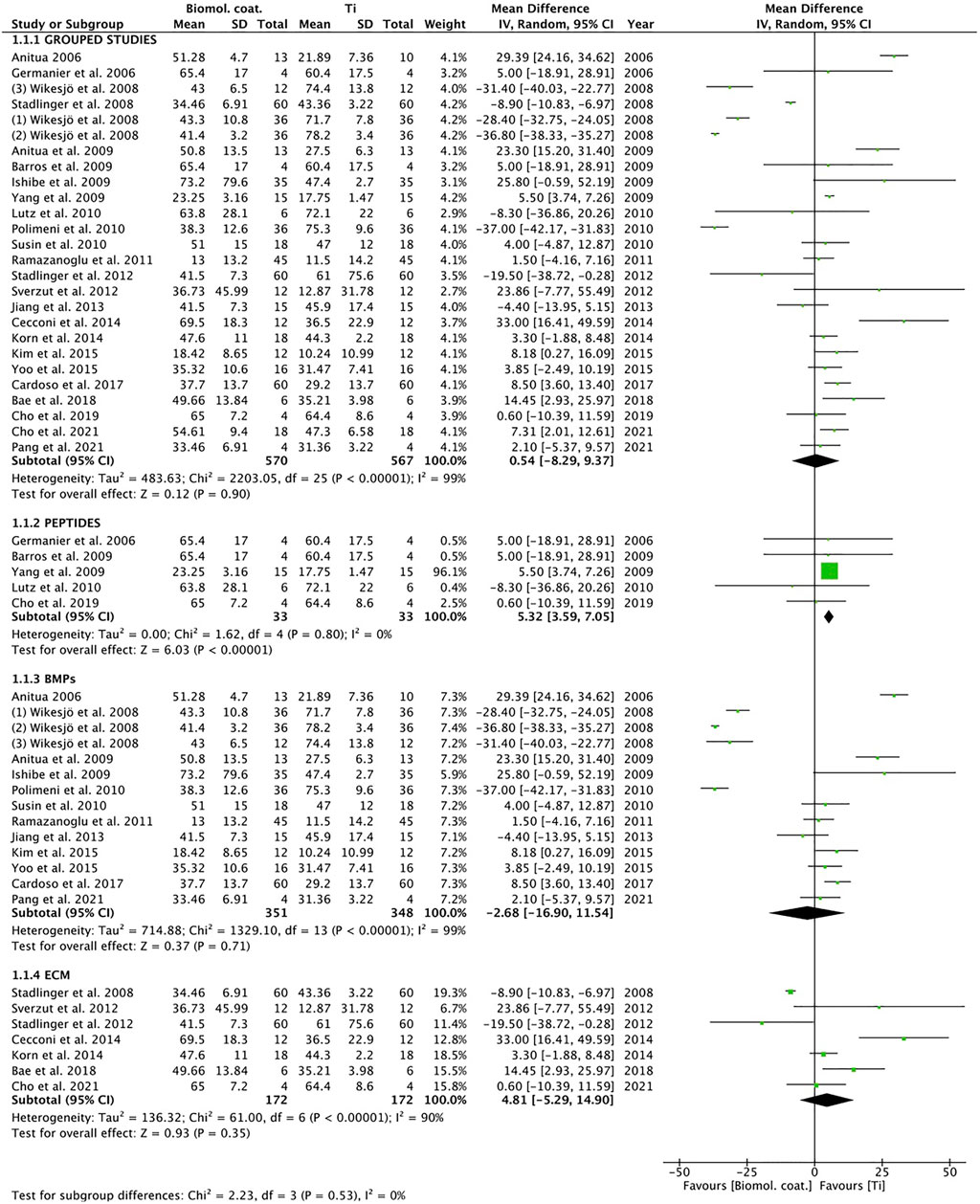
FIGURE 3. Forest plot for meta-analysis of studies evaluating BIC at 4 weeks after placement, assuming a random-effects model. SD, standard deviation; CI, confidence interval.
3.5 Publication bias and heterogeneity
The grouped studies show graphic signs of publication bias (Figure 4).
4 Discussion
The purpose of the present study was to answer the following clinical question: “Does the bioactive surface of titanium dental implants, based on biomolecules, influence osseointegration?”.
Osseointegration is the stable anchorage of an implant through direct bone-to-implant contact (Albrektsson and Johansson, 2001).
The main objective of surface modifications of endosseous implants is to modulate the response of the host bone tissue to achieve better osseointegration.
This review focused on BIC analysis in three categories of biomolecular Ti implant coatings: peptides, BMPs and ECM and identified 26 preclinical research articles that used BIC analysis to assess peri-implant bone formation in different animal models. The included studies found that coatings with bioactive molecules increased bone values around the implant; only the study by Ramazanoglu et al. (2011) found no difference in BIC in the rhBMP-2 coating.
After insertion of an endosseous implant, a series of events occur between the host and the implant surface. During the intercommunication of the implant surface and the blood of the recipient, ligands and proteins are dynamically adsorbed at the implant surface and through a subsequent inflammatory process are released from it, followed by bone formation around the bioactive surface, reaching the maximum degree of organization and biomechanical properties through several remodeling cycles (Lemons, 2004; Goiato et al., 2009). Due to the dynamic nature of the bone-biomaterial interface, biomaterials for endosseous dental implants must have short- and long-term biocompatible and biofunctional properties (Xuereb et al., 2015). It was Puleo and Nanci (Puleo and Nanci, 1999), in 1999, who first indicated that “biochemical surface modification strives to utilize current knowledge of the biology and biochemistry of cell function and differentiation".
Since then, and especially in recent years, surface modifications of Ti and Ti6Al4V implants, using methods based on the immobilization of biologically active organic molecules, have aroused particular interest among researchers, with the aim of increasing cell migration and adhesion to the substrate and avoiding nonspecific addition of proteins, to improve the healing process (Panayotov et al., 2015). (Drexelius and Neundorf, 2021) Antimicrobial peptides have evolved as reliable alternatives to commonly used antibiotics and are positioned as candidates for antimicrobial surface coatings of implants. A review by Drexelius and Neundorf concluded that they have excellent in vitro and in vivo antimicrobial activity (Drexelius and Neundorf, 2021). Kang et al. (Kang et al., 2013) in a mixed in vitro and in vivo study used a laminin-2-derived peptide capable of promoting initial cell adhesion and propagation of osteoblast-like cells in vitro, acting as an accelerator of osseointegration of implant materials and determining its positive effect, in vivo, on BIC values.
Plasma and extracellular matrix proteins (type I collagen, fibronectin, vitronectin, osteopontin, and bone sialoprotein), which contain at binding sites the RGD (Arg-Gly-Asp) sequence, together with receptor integrins, constitute an important recognition system for cell adhesion (Ruoslahti, 1996). Two of the selected studies (Germanier et al., 2006; Yang et al., 2009) investigated the effect of RGD coating by a layered self-assembly technique on porous surface implants, concluding that the peptides possess potential to transmit particular cell adhesion properties to Ti surfaces and are able to enhance cell-material interactions. Kroese-Deutman et al. (Kroese-Deutman et al., 2005) used a porous Ti fiber mesh implant coated with the RGD peptide in the rabbit skull and compared it with porous Ti fiber mesh disks without the RGD sequence. Histological and histomorphometric examinations after 4 and 8 weeks showed a significant increase in bone growth in the RGD-Ti group compared to the control group.
BMPs belong to the transforming growth factor beta (TGF-β) family and are biological factors with a strong ability to induce bone, cartilage and connective tissue formation through the differentiation of bone mesenchymal stem cells (Dolanmaz et al., 2015b). They have been investigated as one of the growth factors (GF) that stimulate undifferentiated cells to become osteoblasts, with a certain ability to attract undifferentiated mesenchymal cells, regulating angiogenesis, chemotaxis and cell multiplication (Chang et al., 2010; Öncü and Alaaddinoğlu, 2015; Öncü et al., 2016). Numerous studies have reported that the use of BMPs improves the process of osteogenesis, osteoblast activity and osseointegration after dental implantation (Chen et al., 2004; Halloran et al., 2020). Nine of the reviewed studies (Wikesjö et al., 2008a; Wikesjö et al., 2008b; Wikesjö et al., 2008c; Ishibe et al., 2009; Ramazanoglu et al., 2011; Jiang et al., 2013; Kim et al., 2015; Yoo et al., 2015; Pang et al., 2021) used BMP-2 as a Ti implant coating. Wikesjö et al. used recombinant human bone morphogenetic protein-2 (rhBMP-2) in three studies and in different experimental models (Wikesjö et al., 2008a; Wikesjö et al., 2008b; Wikesjö et al., 2008c); in one study with non-human primates (Wikesjö et al., 2008c), they found that Ti surface coated with rhBMP-2 accelerated type IV bone formation; another study, in a canine model (Wikesjö et al., 2008b), based peri-implant bone remodeling on rhBMP-2 doses, reporting that sites receiving implants coated with rhBMP-2 at 3 mg/ml, showed increased formation of immature trabecular bone. On the contrary, the same authors in a third study, also on a canine model (Wikesjö et al., 2008a), demonstrated that rh BMP-2 at doses of 0.75 or 1.5 mg/ml, despite inducing osseointegration, did not increase BIC values, resulting significantly higher in the control group (uncoated Ti). Similarly, Ramazanoglu et al. (Ramazanoglu et al., 2011) found no increase in BIC in Ti implants with rhBMP-2 biomimetic coatings, despite inducing an improvement in peri-implant bone density.
Anitua et al. (Anitua et al., 2007; Anitua et al., 2009) proposed implant wetting with autologous growth factors, obtaining significant improvements in osseointegration. Lee et al. (Lee et al., 2010) reported that Ti porous oxide implants coated with rhBMP-2 significantly induce bone formation and remodeling, although they did not find significant effects according to the application techniques.
The ECM is a three-dimensional network, with an abundance of macromolecules, such as type I collagen, proteoglycans, laminin and fibronectin, which provides biochemical and structural support to surrounding cells (Daley and Yamada, 2013). It has been highlighted that ECM could affect the differentiation, survival and potentiality of mesenchymal stem cells (MSCs) by modulating the activity of growth factors and affecting cell behavior (Assis-Ribas et al., 2018). Feng et al. in a recent investigation (Feng et al., 2020) studied the behavior of MSC laminates, obtained by a decellularization process, on SLA-surfaced implants and demonstrated that they promoted adhesion, proliferation and osteogenic differentiation of bone marrow mesenchymal stem cells (BMSCs) in vitro, and improved osseointegration of implants in vivo. Shekaran and Garcia in a review study (Shekaran and García, 2011) highlighted the functionalization of implants with ECM peptides or proteins, to modulate host cell responses to the implant material and to enhance osseointegration and bone formation. They also observed that surfaces presenting the peptide Gly-Phe-Hyp-Gly-Glu-Arg (GFOGER), from the α1 chain of type I collagen, promote osteoblastic differentiation of primary bone marrow cells in vitro, and that GFOGER-functionalized titanium implants would improve implant integration in a rat cortical model by enhancing peri-implant bone formation and implant attachment to bone. Despite this, studies such as those by Hennessy et al. (Hennessy et al., 2009) disagree with these results, suggesting that collagen mimetic peptides would exclusively stimulate osteoblastic differentiation and that the beneficial effects would be due to the role of these peptides as differentiation rather than adhesion factors. Stadlinger et al. (Stadlinger et al., 2008; Stadlinger et al., 2012b) in two in vivo studies did not obtain variations in BIC at 4 and 8 weeks after cycloaddition in collagen-coated implants, finding only a slight increase in bone-to-implant contact around the implants that incorporated CS in the coating and observing that the additional inclusion of a low amount of rhBMP-4 had a detrimental 4meta-analysis had several limitations: first, different experimental models were used, suggesting different bone formation dynamics, especially in early healing times (Pearce et al., 2007; Wancket, 2015). These factors may influence the observed BIC values. Second, this meta-analysis focused only on three biomolecular coatings (peptides, BMPs, and ECMs), leaving out other bioactive coatings; moreover, the coatings in the different studies were not single coatings, but most resorted to combined coatings. Thirdly, the discrepant follow-up periods (2–84 weeks) and differences in the number of animals in the studies, could condition the results. Fourth, the various investigations analyzed several parameters indicative of bone neoformation and in our meta-analysis only BIC was chosen as a measure indicative of osseointegration (Albrektsson and Johansson, 2001; Gehrke et al., 2020).
5 Conclusion
In summary, the present meta-analysis revealed that the use of certain bioactive organic molecules seems to promote peri-implant bone formation, which could influence osseointegration during the early stages of healing; however, different factors make comparison between studies difficult and complicate the interpretation of the results on peri-implant bone formation. Nevertheless, in order to confirm the clinical applicability of these findings, in addition to a greater number of preclinical studies on suitable experimental models, clinical trials with prolonged follow-up periods would be necessary, since the results of preclinical experiments do not necessarily reflect the human clinical reality.
Data availability statement
The original contributions presented in the study are included in the article/Supplementary Material, further inquiries can be directed to the corresponding author.
Author contributions
Conceptualization, NL-V and JA; methodology, NL-V; formal analysis, NQ-L and AL-V; investigation, NL-V and JA; writing—original draft preparation AL-V; data curation, JA and CR; supervision, JA and AL-V. All authors have read and agreed to the published version of the manuscript. All authors have read and agreed to the published version of the manuscript.
Conflict of interest
The authors declare that the research was conducted in the absence of any commercial or financial relationships that could be construed as a potential conflict of interest.
Publisher’s note
All claims expressed in this article are solely those of the authors and do not necessarily represent those of their affiliated organizations, or those of the publisher, the editors and the reviewers. Any product that may be evaluated in this article, or claim that may be made by its manufacturer, is not guaranteed or endorsed by the publisher.
Supplementary material
The Supplementary Material for this article can be found online at: https://www.frontiersin.org/articles/10.3389/fbioe.2022.986112/full#supplementary-material
References
Albrektsson, T., and Johansson, C. (2001). Osteoinduction, osteoconduction and osseointegration. Eur. Spine J. 2 (2), S96–S101. doi:10.1007/s005860100282
Alghamdi, H. S., and Jansen, J. A. (2020). The development and future of dental implants. Dent. Mat. J. 39, 167–172. doi:10.4012/dmj.2019-140
Andrea, A., Molchanova, N., and Jenssen, H. (2018). Antibiofilm peptides and peptidomimetics with focus on surface immobilization. Biomolecules 8, 27. doi:10.3390/biom8020027
Andrea, A., Molchanova, N., and Jenssen, H. (2018). Antibiofilm peptides and peptidomimetics with focus on surface immobilization. Biomolecules 8, 27. doi:10.3390/biom8020027
Anitua, E., Orive, G., Pla, R., Roman, P., Serrano, V., and Andía, I. (2009). The effects of PRGF on bone regeneration and on titanium implant osseointegration in goats: A histologic and histomorphometric study. J. Biomed. Mat. Res. A 91, 158–165. doi:10.1002/jbm.a.32217
Anitua, E., Sánchez, M., Orive, G., and Andía, I. (2007). The potential impact of the preparation rich in growth factors (PRGF) in different medical fields. Biomaterials 28, 4551–4560. doi:10.1016/j.biomaterials.2007.06.037
Anitua, E. A. (2006). Enhancement of osseointegration by generating a dynamic implant surface. J. Oral Implantol. 32, 72–76. doi:10.1563/736.1
Assis-Ribas, T., Forni, M. F., Winnischofer, S. M. B., Sogayar, M. C., and Trombetta-Lima, M. (2018). Extracellular matrix dynamics during mesenchymal stem cells differentiation. Dev. Biol. N. Y. 1985. 437, 63–74. doi:10.1016/j.ydbio.2018.03.002
Badr, N., and Hadary, A. (2007). Hydroxyapatite-electroplated cp-titanium implant and its bone integration potentiality: An in vivo study. Implant Dent. 16, 297–308. doi:10.1097/ID.0b013e31805d7dc4
Bae, E. B., Yoo, J. H., Jeong, S. I., Kim, M. S., Lim, Y. M., Ahn, J. J., et al. (2018). Effect of titanium implants coated with radiation-crosslinked collagen on stability and osseointegration in rat tibia. Materials 11 (12), 2520. doi:10.3390/ma11122520
Barros, R. R., Novaes, A. B., Papalexiou, V., Souza, S. L., Taba, M., Palioto, D. B., et al. (2009). Effect of biofunctionalized implant surface on osseointegration: A histomorphometric study in dogs. Braz. Dent. J. 20, 91–98. doi:10.1590/s0103-64402009000200001
Bosco, R., Edreira, E. R. U., Wolke, J. G., Leeuwenburgh, S. C., van den Beucken, J. J., and Jansen, J. A. (2013). Instructive coatings for biological guidance of bone implants. Surf. Coat. Technol. 233, 91–98. doi:10.1016/j.surfcoat.2013.02.039
Cardoso, M. V., de Rycker, J., Chaudhari, A., Coutinho, E., Yoshida, Y., Van Meerbeek, B., et al. (2017). Titanium implant functionalization with phosphate-containing polymers may favour in vivo osseointegration. J. Clin. Periodontol. 44, 950–960. doi:10.1111/jcpe.12736
Cecconi, S., Mattioli-Belmonte, M., Manzotti, S., Orciani, M., Piccioli, A., and Gigante, A. (2014). Bone-derived titanium coating improves in vivo implant osseointegration in an experimental animal model. J. Biomed. Mat. Res. 102, 303–310. doi:10.1002/jbm.b.33008
Chang, P. C., Lang, N. P., and Giannobile, W. V. (2010). Evaluation of functional dynamics during osseointegration and regeneration associated with oral implants. Clin. Oral Implants Res. 21, 1–12. doi:10.1111/j.1600-0501.2009.01826.x
Chen, D., Zhao, M., and Mundy, G. R. (2004). Bone morphogenetic proteins. Growth factors. 22, 233–241. doi:10.1080/08977190412331279890
Cho, C. B., Jung, S. Y., Park, C. Y., Kang, H. K., Yeo, I. L., and Min, B. M. (2019). A vitronectin-derived bioactive peptide improves bone healing capacity of SLA titanium surfaces. Mater. (Basel) 12 (20), 3400. doi:10.3390/ma12203400
Cho, W. T., Kim, S. Y., Jung, S. I., Kang, S. S., Kim, S. E., Hwang, S. H., et al. (2021). Effects of gamma radiation-induced crosslinking of collagen type I coated dental titanium implants on osseointegration and bone regeneration. Mater. (Basel) 14 (12), 3268. doi:10.3390/ma14123268
Cooper, L., Zhou, Y., Takebe, J., Guo, J., Abron, A., Holmen, A., et al. (2006). Fluoride modification effects on osteoblast behavior and bone formation at TiO grit-blasted c.p. titanium endosseous implants. Biomaterials 27, 926–936. doi:10.1016/j.biomaterials.2005.07.009
Daley, W. P., and Yamada, K. M. (2013). ECM-modulated cellular dynamics as a driving force for tissue morphogenesis. Curr. Opin. Genet. Dev. 23, 408–414. doi:10.1016/j.gde.2013.05.005
Dolanmaz, D., Saglam, M., Inan, O., Dundar, N., Alniacık, G., Gursoy Trak, B., et al. (2015). Monitoring bone morphogenetic protein-2 and -7, soluble receptor activator of nuclear factor-κB ligand and osteoprotegerin levels in the peri-implant sulcular fluid during the osseointegration of hydrophilic-modified sandblasted acid-etched and sandblaste. J. Periodontal Res. 50, 62–73. doi:10.1111/jre.12182
Drexelius, M. G., and Neundorf, I. (2021). Application of antimicrobial peptides on biomedical implants: Three ways to pursue peptide coatings. Int. J. Mol. Sci. 22, 13212. doi:10.3390/ijms222413212
Ellingsen, J., Johansson, C., Wennerberg, A., and Holmén, A. (2004). Improved retention and bone-tolmplant contact with fluoride-modified titanium implants. Int. J. Oral Maxillofac. Implants 19, 659–666.
Feng, Y., Jiang, Z., Zhang, Y., Miao, X., Yu, Q., Xie, Z., et al. (2020). Stem-cell-derived ECM sheet-implant complexes for enhancing osseointegration. Biomater. Sci. 8, 6647–6656. doi:10.1039/d0bm00980f
Fischer, N. G., Münchow, E. A., Tamerler, C., Bottino, M. C., and Aparicio, C. (2020). Harnessing biomolecules for bioinspired dental biomaterials. J. Mat. Chem. B 8, 8713–8747. doi:10.1039/d0tb01456g
Garcia, A. J., and Reyes, C. D. (2005). Bio-adhesive surfaces to promote osteoblast differentiation and bone formation. J. Dent. Res. 84, 407–413. doi:10.1177/154405910508400502
Gehrke, S. A., AramburúJúnior, J., Pérez-Díaz, L., do Prado, T. D., Dedavid, B. A., Mazon, P., et al. (2020). Can changes in implant macrogeometry accelerate the osseointegration process?: An in vivo experimental biomechanical and histological evaluations. PLoS One 15 (5), e0233304. doi:10.1371/journal.pone.0233304
Germanier, Y., Tosatti, S., Broggini, N., Textor, M., and Buser, D. (2006). Enhanced bone apposition around biofunctionalized sandblasted and acid-etched titanium implant surfaces. A histomorphometric study in miniature pigs. Clin. Oral Implants Res. 17, 251–257. doi:10.1111/j.1600-0501.2005.01222.x
Giro, G., Gonçalves, D., Sakakura, C. E., Pereira, R. M., Marcantonio Júnior, E., and Orrico, S. R. (2008). Influence of estrogen deficiency and its treatment with alendronate and estrogen on bone density around osseointegrated implants: Radiographic study in female rats. Oral Surg. Oral Med. Oral Pathology Oral Radiology Endodontology 105, 162–167. doi:10.1016/j.tripleo.2007.06.010
Goiato, M. C., Pellizzer, E. P., dos Santos, D. M., Barão, V. A., de Carvalho, B. M., Magro-Filho, O., et al. (2009). Clinical viability of immediate loading of dental implants: Part I-factors for success. J. Craniofac. Surg. 20, 2 139–2142. doi:10.1097/SCS.0b013e3181bec71a
Halloran, D., Durbano, H. W., and Nohe, A. (2020). Bone morphogenetic protein-2 in development and bone homeostasis. J. Dev. Biol. 8, 19. doi:10.3390/jdb8030019
Hennessy, K. M., Pollot, B. E., Clem, W. C., Phipps, M. C., Sawyer, A. A., Culpepper, B. K., et al. (2009). The effect of collagen I mimetic peptides on mesenchymal stem cell adhesion and differentiation, and on bone formation at hydroxyapatite surfaces. Biomaterials 30, 1898–1909. doi:10.1016/j.biomaterials.2008.12.053
Hooijmans, C. R., Rovers, M. M., de Vries, R. B., Leenaars, M., Ritskes-Hoitinga, M., and Langendam, M. W. (2014). SYRCLE’s risk of bias tool for animal studies. BMC Med. Res. Methodol. 14, 43. doi:10.1186/1471-2288-14-43
Hubbell, J. A. (1999). Bioactive biomaterials. Curr. Opin. Biotechnol. 10, 123–129. doi:10.1016/s0958-1669(99)80021-4
Hutton, B., Catalá-López, F., and Moher, D. (2016). The PRISMA statement extension for systematic reviews incorporating network meta-analysis: PRISMA-NMA. Med. Clin. 147 (6), 262–266. doi:10.1016/j.medcli.2016.02.025
Ishibe, T., Goto, T., Kodama, T., Miyazaki, T., Kobayashi, S., and Takahashi, T. (2009). Bone formation on apatite-coated titanium with incorporated BMP-2/heparin in vivo. Oral Surg. Oral Med. Oral Pathology Oral Radiology Endodontology 108, 867–875. doi:10.1016/j.tripleo.2009.06.039
Jiang, Q. H., Liu, L., Peel, S., Yang, G. L., Zhao, S. F., and He, F. M. (2013). Bone response to the multilayer BMP-2 gene coated porous titanium implant surface. Clin. Oral Implants Res. 24, 853–861. doi:10.1111/j.1600-0501.2011.02383.x
Junker, R., Dimakis, A., Thoneick, M., and Jansen, J. A. (2009). Effects of implant surface coatings and composition on bone integration: A systematic review. Clin. Oral Implants Res. 20 (4), 185–206. doi:10.1111/j.1600-0501.2009.01777.x
Kang, H. K., Kim, O. B., Min, S. K., Jung, S. Y., Jang, D. H., Kwon, T. K., et al. (2013). The effect of the DLTIDDSYWYRI motif of the human laminin α2 chain on implant osseointegration. Biomaterials 34, 4027–4037. doi:10.1016/j.biomaterials.2013.02.023
Kim, N. H., Lee, S. H., Ryu, J. J., Choi, K. H., and Huh, J. B. (2015). Effects of rhBMP-2 on sandblasted and acid etched titanium implant surfaces on bone regeneration and osseointegration: Spilt-mouth designed pilot study. Biomed. Res. Int. 2015, 1–11. doi:10.1155/2015/459393
Korn, P., Schulz, M. C., Hintze, V., Range, U., Mai, R., Eckelt, U., et al. (2014). Chondroitin sulfate and sulfated hyaluronan-containing collagen coatings of titanium implants influence peri-implant bone formation in a minipig model. J. Biomed. Mat. Res. A 102, 2334–2344. doi:10.1002/jbm.a.34913
Kroese-Deutman, H. C., van den Dolder, J., Spauwen, P. H., and Jansen, J. A. (2005). Influence of RGD-loaded titanium implants on bone formation in vivo. Tissue Eng. 11 (11-12), 1867–1875. doi:10.1089/ten.2005.11.1867
Le Guéhennec, L., Soueidan, A., Layrolle, P., and Amouriq, Y. (2007). Surface treatments of titanium dental implants for rapid osseointegration. Dent. Mat. 23, 844–854. doi:10.1016/j.dental.2006.06.025
Lee, J., Decker, J. F., Polimeni, G., Cortella, C. A., Rohrer, M. D., Wozney, J. M., et al. (2010). Evaluation of implants coated with rhBMP-2 using two different coating strategies: A critical-size supraalveolar peri-implant defect study in dogs. J. Clin. Periodontol. 37, 582–590. doi:10.1111/j.1600-051X.2010.01557.x
Lee, S. W., Hahn, B. D., Kang, T. Y., Lee, M. J., Choi, J. Y., Kim, M. K., et al. (2014). Hydroxyapatite and collagen combination-coated dental implants display better bone formation in the peri-implant area than the same combination plus bone morphogenetic protein-2-coated implants, hydroxyapatite only coated implants, and uncoated implants. J. Oral Maxillofac. Surg. 72, 53–60. doi:10.1016/j.joms.2013.08.031
Lemons, J. E. (2004). Biomaterials, biomechanics, tissue healing, and immediate-function dental implants. J. Oral Implantol. 30, 318–324. doi:10.1563/0712.1
Lutz, R., Srour, S., Nonhoff, J., Weisel, T., Damien, C. J., and Schlegel, K. A. (2010). Biofunctionalization of titanium implants with a biomimetic active peptide (P-15) promotes early osseointegration. Clin. Oral Implants Res. 21, 726–734. doi:10.1111/j.1600-0501.2009.01904.x
Lutz, R., Srour, S., Nonhoff, J., Weisel, T., Damien, C. J., and Schlegel, K. A. (2010). Biofunctionalization of titanium implants with a biomimetic active peptide (P-15) promotes early osseointegration. Clin. Oral Implants Res. 21, 726–734. doi:10.1111/j.1600-0501.2009.01904.x
Makihira, S., Nikawa, H., Shuto, T., Nishimura, M., Mine, Y., Tsuji, K., et al. (2011). Evaluation of trabecular bone formation in a canine model surrounding a dental implant fixture immobilized with an antimicrobial peptide derived from histatin. J. Mat. Sci. Mat. Med. 22, 2765–2772. doi:10.1007/s10856-011-4440-2
Moraschini, V., Poubel, L. A., Ferreira, V. F., and Barboza Edos, S. (2015). Evaluation of survival and success rates of dental implants reported in longitudinal studies with a follow-up period of at least 10 years: A systematic review. Int. J. Oral Maxillofac. Surg. 44, 377–388. doi:10.1016/j.ijom.2014.10.023
Moussa, D. G., and Aparicio, C. (2020). Targeting the oral plaque microbiome with immobilized anti-biofilm peptides at tooth-restoration interfaces. PLoS One 15 (7), e0235283. doi:10.1371/journal.pone.0235283
Narai, S., and Nagahata, S. (2003). Effects of alendronate on the removal torque of implants in rats with induced osteoporosis. Int. J. Oral Maxillofac. Implants 18, 218–223.
Öncü, E., and Alaaddinoğlu, E. E. (2015). The effect of platelet-rich fibrin on implant stability. Int. J. Oral Maxillofac. Implants 30, 578–582. doi:10.11607/jomi.3897
Öncü, E., Bayram, B., Kantarci, A., Gülsever, S., and Alaaddinoğlu, E. E. (2016). Positive effect of platelet rich fibrin on osseointegration. Med. Oral Patol. Oral Cir. Bucal 21 (5), e601–e607. doi:10.4317/medoral.21026
Osman, R. B., and Swain, M. V. (2015). A critical review of dental implant materials with an emphasis on titanium versus zirconia. Mater. (Basel) 8, 932–958. doi:10.3390/ma8030932
Panayotov, I. V., Vladimirov, B. S., Dutilleul, P. Y., Levallois, B., and Cuisinier, F. (2015). Strategies for immobilization of bioactive organic molecules on titanium implant surfaces - a review. Folia Med. Plovdiv. 57, 11–18. doi:10.1515/folmed-2015-0014
Pang, K., Seo, Y. K., and Lee, J. H. (2021). Effects of the combination of bone morphogenetic protein-2 and nano-hydroxyapatite on the osseointegration of dental implants. J. Korean Assoc. Oral Maxillofac. Surg. 47, 454–464. doi:10.5125/jkaoms.2021.47.6.454
Pearce, A. I., Richards, R. G., Milz, S., Schneider, E., and Pearce, S. G. (2007). Animal models for implant biomaterial research in bone: A review. Eur. Cell. Mat. 13, 1–10. doi:10.22203/ecm.v013a01
Polimeni, G., Wikesjö, U. M., Susin, C., Qahash, M., Shanaman, R. H., Prasad, H. S., et al. (2010). Alveolar ridge augmentation using implants coated with recombinant human growth/differentiation factor-5: Histologic observations. J. Clin. Periodontol. 37, 759–768. Epub 2010 May 25. doi:10.1111/j.1600-051X.2010.01579.x
Puleo, D. A., and Nanci, A. (1999). Understanding and controlling the bone-implant interface. Biomaterials 20 (23-24), 2311–2321. doi:10.1016/s0142-9612(99)00160-x
Ramazanoglu, M., Lutz, R., Ergun, C., von Wilmowsky, C., Nkenke, E., and Schlegel, K. A. (2011). The effect of combined delivery of recombinant human bone morphogenetic protein-2 and recombinant human vascular endothelial growth factor 165 from biomimetic calcium-phosphate-coated implants on osseointegration. Clin. Oral Implants Res. 22 (12), 1433–1439. doi:10.1111/j.1600-0501.2010.02133.x
Raphel, J., Karlsson, J., Galli, S., Wennerberg, A., Lindsay, C., Haugh, M. G., et al. (2016). Engineered protein coatings to improve the osseointegration of dental and orthopaedic implants. Biomaterials 83, 269–282. doi:10.1016/j.biomaterials.2015.12.030
Riool, M., de Breij, A., Drijfhout, J. W., Nibbering, P. H., and Zaat, S. A. J. (2017). Antimicrobial peptides in biomedical device manufacturing. Front. Chem. 5, 63. doi:10.3389/fchem.2017.00063
Ruoslahti, E. (1996). RGD and other recognition sequences for integrins. Annu. Rev. Cell Dev. Biol. 12, 697–715. doi:10.1146/annurev.cellbio.12.1.697
Russell, R. G. G., Watts, N. B., Ebetino, F. H., and Rogers, M. J. (2008). Mechanisms of action of bisphosphonates: Similarities and differences and their potential influence on clinical efficacy. Osteoporos. Int. 19, 733–759. doi:10.1007/s00198-007-0540-8
Shekaran, A., and García, A. J. (2011). Extracellular matrix-mimetic adhesive biomaterials for bone repair. J. Biomed. Mat. Res. A 96, 261–272. doi:10.1002/jbm.a.32979
Silva, R. R., Avelino, K. Y., Ribeiro, K. L., Franco, O. L., Oliveira, M. D., and Andrade, C. A. (2016). Chemical immobilization of antimicrobial peptides on biomaterial surfaces. Front. Biosci. 8, 453–142. doi:10.2741/s453
Siwakul, P., Sirinnaphakorn, L., Suwanprateep, J., Hayakawa, T., and Pugdee, K. (2021). Cellular responses of histatin-derived peptides immobilized titanium surface using a tresyl chloride-activated method. Dent. Mat. J. 40, 307–941. doi:10.4012/dmj.2020-307
Stadlinger, B., Hintze, V., Bierbaum, S., Möller, S., Schulz, M. C., Mai, R., et al. (2012). Biological functionalization of dental implants with collagen and glycosaminoglycans-A comparative study. J. Biomed. Mat. Res. 100 (2), 331–341. doi:10.1002/jbm.b.31953
Stadlinger, B., Pilling, E., Huhle, M., Mai, R., Bierbaum, S., Scharnweber, D., et al. (2008). Evaluation of osseointegration of dental implants coated with collagen, chondroitin sulphate and BMP-4: An animal study. Int. J. Oral Maxillofac. Surg. 37, 54–59. doi:10.1016/j.ijom.2007.05.024
Stadlinger, B., Pourmand, P., Locher, M. C., and Schulz, M. C. (2012). Systematic review of animal models for the study of implant integration, assessing the influence of material, surface and design. J. Clin. Periodontol. 39, 28–36. doi:10.1111/j.1600-051x.2011.01835.x
Stanford, C. M. (2008). Surface modifications of dental implants. Aust. Dent. J. 53 (1), S26–S33. doi:10.1111/j.1834-7819.2008.00038.x
Susin, C., Qahash, M., Polimeni, G., Lu, P. H., Prasad, H. S., Rohrer, M. D., et al. (2010). Alveolar ridge augmentation using implants coated with recombinant human bone morphogenetic protein-7 (rhBMP-7/rhOP-1): Histological observations. J. Clin. Periodontol. 37, 574–581. doi:10.1111/j.1600-051X.2010.01554.x
Sverzut, A. T., Crippa, G. E., Morra, M., de Oliveira, P. T., Beloti, M. M., and Rosa, A. L. (2012). Effects of type I collagen coating on titanium osseointegration: Histomorphometric, cellular and molecular analyses. Biomed. Mat. 7 (3), 035007. doi:10.1088/1748-6041/7/3/035007
Viera-Negron, Y. E., Ruan, W. H., Winger, J. N., Hou, X., Sharawy, M. M., and Borke, J. L. (2008). Effect of ovariectomy and alendronate on implant osseointegration in rat maxillary bone. J. Oral Implantol. 34, 76–82. doi:10.1563/1548-1336(2008)34[76:EOOAAO]2.0.CO;2
Wancket, L. M. (2015). Animal models for evaluation of bone implants and devices: Comparative bone structure and common model uses. Vet. Pathol. 52, 842–850. doi:10.1177/0300985815593124
Wikesjö, U. M., Huang, Y. H., Xiropaidis, A. V., Sorensen, R. G., Rohrer, M. D., Prasad, H. S., et al. (2008). Bone formation at recombinant human bone morphogenetic protein-2-coated titanium implants in the posterior maxilla (Type IV bone) in non-human primates. J. Clin. Periodontol. 35, 992–1000. doi:10.1111/j.1600-051X.2008.01322.x
Wikesjö, U. M., Qahash, M., Polimeni, G., Susin, C., Shanaman, R. H., Rohrer, M. D., et al. (2008). Alveolar ridge augmentation using implants coated with recombinant human bone morphogenetic protein-2: Histologic observations. J. Clin. Periodontol. 35, 1001–1010. doi:10.1111/j.1600-051X.2008.01321.x
Wikesjö, U. M., Xiropaidis, A. V., Qahash, M., Lim, W. H., Sorensen, R. G., Rohrer, M. D., et al. (2008). Bone formation at recombinant human bone morphogenetic protein-2-coated titanium implants in the posterior mandible (Type II bone) in dogs. J. Clin. Periodontol. 35, 985–991. doi:10.1111/j.1600-051X.2008.01318.x
Xuereb, M., Camilleri, J., and Attard, N. J. (2015). Systematic review of current dental implant coating materials and novel coating techniques. Int. J. Prosthodont. 28, 51–59. doi:10.11607/ijp.4124
Yang, G. L., He, F. M., Yang, X. F., Wang, X. X., and Zhao, S. F. (2009). In vivo evaluation of bone-bonding ability of RGD-coated porous implant using layer-by-layer electrostatic self-assembly. J. Biomed. Mat. Res. A 90, 175–185. doi:10.1002/jbm.a.32055
Yoo, S. Y., Kim, S. K., Heo, S. J., Koak, J. Y., Lee, J. H., and Heo, J. M. (2015). Biochemical responses of anodized titanium implants with a poly(lactide-co-glycolide)/bone morphogenetic protein-2 submicron particle coating. Part 2: An in vivo study. Int. J. Oral Maxillofac. Implants 30, 754–760. doi:10.11607/jomi.3701b
Zagury, R., Harari, N., Conz, M., Soares, A., and Vidigal, G. (2007). Histomorphometric analyses of bone interface with titanium-aluminum-vanadium and hydroxyapatite- coated implants by biomimetic process. Implant Dent. 16, 290–296. doi:10.1097/ID.0b013e3180e9d9ed
Keywords: titanium dental implants, bioactive surface modifications, biomolecules, peptides, bone morphogenetic protein, grown factor, components of the extracellular matrix, osteointegration
Citation: López-Valverde N, Aragoneses J, López-Valverde A, Quispe-López N, Rodríguez C and Aragoneses JM (2022) Effectiveness of biomolecule-based bioactive surfaces, on os-seointegration of titanium dental implants: A systematic review and meta-analysis of in vivo studies. Front. Bioeng. Biotechnol. 10:986112. doi: 10.3389/fbioe.2022.986112
Received: 04 July 2022; Accepted: 12 September 2022;
Published: 26 September 2022.
Edited by:
Min Jiang, Nanjing Tech University, ChinaReviewed by:
Miguel Ángel Garcés-Villalá, Catholic University San Antonio of Murcia, SpainPaolo Canepa, University of Genoa, Italy
Copyright © 2022 López-Valverde, Aragoneses, López-Valverde, Quispe-López, Rodríguez and Aragoneses. This is an open-access article distributed under the terms of the Creative Commons Attribution License (CC BY). The use, distribution or reproduction in other forums is permitted, provided the original author(s) and the copyright owner(s) are credited and that the original publication in this journal is cited, in accordance with accepted academic practice. No use, distribution or reproduction is permitted which does not comply with these terms.
*Correspondence: Antonio López-Valverde, YWxvcGV6dmFsdmVyZGVAdXNhbC5lcw==
†These authors have contributed equally to this work