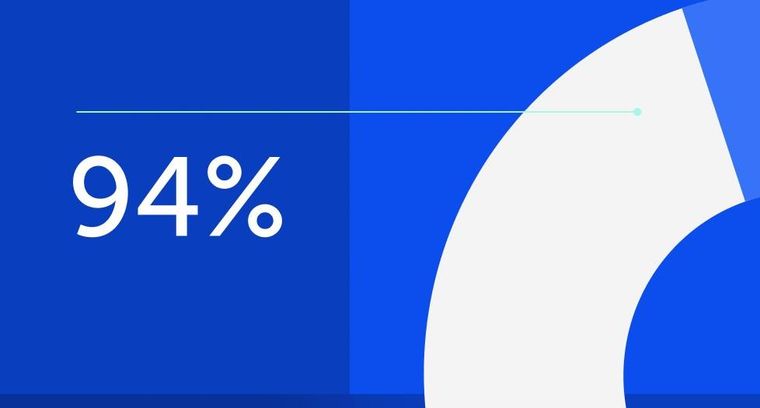
94% of researchers rate our articles as excellent or good
Learn more about the work of our research integrity team to safeguard the quality of each article we publish.
Find out more
EDITORIAL article
Front. Bioeng. Biotechnol., 22 July 2022
Sec. Nanobiotechnology
Volume 10 - 2022 | https://doi.org/10.3389/fbioe.2022.969430
This article is part of the Research TopicNano-Imaging in Translational Cancer MedicineView all 5 articles
Editorial on the Research Topic
Nano-Imaging in Translational Cancer Medicine
The use of nanomaterials has become more prevalent in modern day society since that fateful day at the California Institute of Technology in 1959 when American physicist and Nobel laureate Richard Feynman envisioned the ability to construct machines down to the molecular level during his lecture “There’s Plenty of Room at the Bottom” (Feynman, 1960). Fast forward 30 years, the discovery of carbon nanotubes (CNTs) by Iijima (1991), led to widespread applications of CNTs ranging from carbon fibers to improve the mechanical and thermal stability of polymers used in machine coatings, boat hulls, and water filters, to rechargeable batteries and thin-film electronics (De Volder et al., 2013). The emerging introduction of CNTs and other nanomaterials in biomedical applications continues to push the envelope of what is possible for improving the future of human health and well-being (reviewed in Kinnear et al., 2017) (Kinnear et al., 2017).
In this focus issue of Frontiers in Bioengineering and Biotechnology: Nano-Imaging in Translational Cancer Medicine, we present five articles that discuss a wide breadth of emerging uses of nanoparticles (NPs) for the detection and treatment of cancer. A common theme linking several manuscripts is the use of NPs as a theranostic for the detection and delivery of therapies to melanomas (Guan et al.) and pancreatic cancer (Cai et al.). Taking this one step further into the surgical oncology arena, multidisciplinary clinical perspectives on augmenting the surgical resection of melanoma (Guan et al.) and brain tumors Lam et al. using fluorescence-conjugated tumor-detecting NPs, provide a framework of how nanotechnology is being used to improve surgical outcomes and survival for cancer patients (Figure 1). These publications highlight how far the field of nanomedicine has come since the approval of the liposomal NP formulation of the chemotherapy doxorubicin (Doxil®) in 1995 (Barenholz, 2012).
A recent quantitative bibliometric analysis of cancer nanotechnology research published between the years of 2000–2021 mined over 50,000 papers, showing an exponential increase in numbers of papers on nanoparticles published since 2010. Most of these publications originated from institutions located in the United States, China, the United Kingdom, India, and Iran, with an increased ramp up between 2017 and 2021 (Millagaha Gedara et al., 2021). Topics most commonly studied included iron oxide (IONPs) or mesoporous silica NPs, nanotechnology for DNA or RNA packaging, and nanotechnology for the diagnosis and treatment of cancers, in particular, breast, lung, and prostate cancers (Millagaha Gedara et al., 2021). Further analysis of these articles across the top ten cancer types revealed the use of stem cell and xenograft models to study the pharmacodynamic and pharmacokinetics of NP delivery systems and the use of IONPs for preclinical cancer imaging. These trends are nicely recapitulated in this focus issue of Frontiers, but the translational theme of the articles also addresses some important limitations and caveats of administering NPs in humans. Kong and Dreaden’s opinion piece on the immunogenicity associated with polyethylene glycol (PEG), commonly used to encapsulate (PEGylate) different types of NPs, thereby shielding them from clearance by the reticuloendothelial system and increasing systemic circulation time, addresses why rigorous safety and quality controls are required before we will see more widespread translation of NPs for in-human use (Kong and Dreaden). The worldwide efforts to control the spread of SARS-CoV-2 (COVID-19) through global administration of a PEGylated liposomal mRNA-based vaccine uncovered a potential underlying hypersensitivity to the PEG component of the vaccine that may hinder its use in future NP formulations across different biomedical applications (de Vrieze and Pfizer, 2021). This adverse hypersensitivity reaction of PEG in the human body potentially puts into question the translatability of the safety profiles and biodistribution data of PEGylated NPs gathered from xenograft models. Case in point, Cai et al. tested the in vitro and in vivo toxicity profiles of differently PEGylated polymer NPs with fluorescence emission capabilities in the near-infrared (NIR) window in pancreatic cancer cells. Their results clearly demonstrated a wide range of in vitro toxicities and in vivo accumulation of these differentially PEGylated NPs in subcutaneous flank pancreatic tumors in nude mice (Cai et al.). However, given the recently reported immunogenicity of PEG in humans, one should use an abundance of caution in evaluating the translatability of safety data gathered using immunocompromised animal model systems and addresses the need to design NPs that minimize these adverse events.
Another recurring theme in this issue of Frontiers is the use of NPs for the imaging and detection of tumors using NIR fluorescence imaging. These articles are timely as NIR fluorescence-guided surgery (FGS) using the NIR dye indocyanine green (ICG) is increasingly being used for aiding surgical oncologists across multiple specialties in the operating room for visualization of tumor cells that are difficult to discern using conventional white light operating microscopes (Cho et al., 2018; Quan et al., 2020). The review by Faiz et al. outlines the use of IONPs to increase contrast enhancement of high-grade brain tumors on magnetic resonance imaging (MRI) sequences (Faiz et al., 2022), which can then be used in the operating room by neurosurgeons in conjunction with FGS using ICG to better visualize tumor cells (Lam et al.). However, FGS still remains in its infancy and will require rigorous validation before it can be adopted as standard of care practice (Vahrmeijer et al., 2013).
In closing, this focus issue of Frontiers showcases emerging and approved nanotechnologies that are poised to help clinical and surgical oncologists offer patients better detection of their tumor burden and delivery of precision cancer therapies. Careful characterization of the toxicity profiles of NPs and adherence to good lab and manufacturing practices for upscale manufacturing will improve the translational value of preclinical phase nanomedicines.
FCL conceived, wrote, and edited the manuscript.
The authors declare that the research was conducted in the absence of any commercial or financial relationships that could be construed as a potential conflict of interest.
All claims expressed in this article are solely those of the authors and do not necessarily represent those of their affiliated organizations, or those of the publisher, the editors and the reviewers. Any product that may be evaluated in this article, or claim that may be made by its manufacturer, is not guaranteed or endorsed by the publisher.
Barenholz, Y. (2012). Doxil - the First FDA-Approved Nano-Drug: Lessons Learned. J. Control. Release 160, 117–134. doi:10.1016/j.jconrel.2012.03.020
Cho, S. S., Zeh, R., Pierce, J. T., Salinas, R., Singhal, S., and Lee, J. Y. K. (2018). Comparison of Near-Infrared Imaging Camera Systems for Intracranial Tumor Detection. Mol. Imaging Biol. 20, 213–220. doi:10.1007/s11307-017-1107-5
De Volder, M. F. L., Tawfick, S. H., Baughman, R. H., and Hart, A. J. (2013). Carbon Nanotubes: Present and Future Commercial Applications. Science 339, 535–539. doi:10.1126/science.1222453339/6119/535
de Vrieze, J., and Pfizer, J. (2021). Pfizer's Vaccine Raises Allergy Concerns. Science 371, 10–11. doi:10.1126/science.371.6524.10
Faiz, K., Lam, F. C., Chen, J., Kasper, E. M., and Salehi, F. (2022). The Emerging Applications of Nanotechnology in Neuroimaging: A Comprehensive Review. Front. Bioeng. Biotechnol. 10, 855195. doi:10.3389/fbioe.2022.885195
Iijima, S. (1991). Helical Microtubules of Graphitic Carbon. Nature 354, 56–58. doi:10.1038/354056a0
Kinnear, C., Moore, T. L., Rodriguez-Lorenzo, L., Rothen-Rutishauser, B., and Petri-Fink, A. (2017). Form Follows Function: Nanoparticle Shape and its Implications for Nanomedicine. Chem. Rev. 117, 11476–11521. doi:10.1021/acs.chemrev.7b00194
Millagaha Gedara, N. I., Xu, X., DeLong, R., Aryal, S., and Jaberi-Douraki, M. (2021). Global Trends in Cancer Nanotechnology: A Qualitative Scientific Mapping Using Content-Based and Bibliometric Features for Machine Learning Text Classification. Cancers 13, 4417. doi:10.3390/cancers13174417
Quan, Y. H., Oh, C. H., Jung, D., Lim, J.-Y., Choi, B. H., Rho, J., et al. (2020). Evaluation of Intraoperative Near-Infrared Fluorescence Visualization of the Lung Tumor Margin with Indocyanine Green Inhalation. JAMA Surg. 155, 732–740. doi:10.1001/jamasurg.2020.1314
Keywords: nanotechnology, cancer therapeutic, diagnostic imaging, theranostic, fluorescence guided surgery
Citation: Lam FC (2022) Editorial: Nano-Imaging in Translational Cancer Medicine. Front. Bioeng. Biotechnol. 10:969430. doi: 10.3389/fbioe.2022.969430
Received: 15 June 2022; Accepted: 21 June 2022;
Published: 22 July 2022.
Edited and Reviewed by:
Gianni Ciofani, Italian Institute of Technology (IIT), ItalyCopyright © 2022 Lam. This is an open-access article distributed under the terms of the Creative Commons Attribution License (CC BY). The use, distribution or reproduction in other forums is permitted, provided the original author(s) and the copyright owner(s) are credited and that the original publication in this journal is cited, in accordance with accepted academic practice. No use, distribution or reproduction is permitted which does not comply with these terms.
*Correspondence: Fred C. Lam, ZnJlZGxhbUBtaXQuZWR1
Disclaimer: All claims expressed in this article are solely those of the authors and do not necessarily represent those of their affiliated organizations, or those of the publisher, the editors and the reviewers. Any product that may be evaluated in this article or claim that may be made by its manufacturer is not guaranteed or endorsed by the publisher.
Research integrity at Frontiers
Learn more about the work of our research integrity team to safeguard the quality of each article we publish.