- 1Departamento de Química Fundamental, Instituto de Química, Universidade de São Paulo, São Paulo, Brazil
- 2Research Centre for Greenhouse Gas Innovation (RGCI-POLI-USP), University of São Paulo, São Paulo, Brazil
- 3Departamento de Bioquímica, Instituto de Química, Universidade de São Paulo, São Paulo, Brazil
- 4Department of Chemistry and Biochemistry, Yeshiva University, New York, NY, United States
Environmental pollutants are today a major concern and an intensely discussed topic on the global agenda for sustainable development. They include a wide range of organic compounds, such as pharmaceutical waste, pesticides, plastics, and volatile organic compounds that can be found in air, soil, water bodies, sewage, and industrial wastewater. In addition to impacting fauna, flora, and fungi, skin absorption, inhalation, and ingestion of some pollutants can also negatively affect human health. Fungi play a crucial role in the decomposition and cycle of natural and synthetic substances. They exhibit a variety of growth, metabolic, morphological, and reproductive strategies and can be found in association with animals, plants, algae, and cyanobacteria. There are fungal strains that occur naturally in soil, sediment, and water that have inherent abilities to survive with contaminants, making the organism important for bioassay applications. In this context, we reviewed the applications of fungal-based bioassays as a versatile tool for environmental monitoring.
Introduction
The expansion of the anthropogenic activities to attend to the rising global demand for a diversity of products has generated an exponential increase in the release of different pollutants into the air, soil, and aquatic compartments (Esposito et al., 2021). These pollutants, that fall into a broad category of xenobiotic compounds, are released in large quantities into the environment, very often on the fringes of the law, and, unfortunately, they are not readily degraded by indigenous microfauna and flora, being bioaccumulate and biomagnified along the food chain (Wasi et al., 2013; Zenker et al., 2014). Among the ubiquitous environmental pollutants, phenolic compounds, and transition metal cations represent some of the major toxicants present in surface water (Wasi et al., 2008, 2013; Tabrez and Ahmad, 2011).
Thereby, these pollutants change the balance of biodiversity and have led to serious health problems for humans and other living organisms (Alharbi et al., 2018; Maurya and Pachauri, 2022). Monitoring pollution levels is essential for restoration (bio)remediation, as well as it might provide the basis for other pollutants control management practices (Garg et al., 2022). There are three classes for environmental pollution monitoring: physical (such as odor, color, taste, porosity, temperature, conductivity, and aggregate stability), chemical (including parameters such as redox potential, salinity, and biological and chemical oxygen demand), and biological (microbes, plants, and animals) indicators (Maurya and Pachauri, 2022). Among these classes, bioassays using biological indicators have been extensively used to either analyze target chemicals in water, sediment, and soil samples and for evaluating the relative ecotoxicological impact of such chemicals in these matrices (Viegas, 2021). Mainly due to their sensitivity and reproducibility, bioassays have great advantages over other physical or chemical methods to detect pollutants (Maurya and Pachauri, 2022). Given their morphological and ecological diversity, exhibiting from unicellular to multicellular forms with different growth rates (Chethana et al., 2021; Hurdeal et al., 2021), besides their tolerance and ability to survive in contaminated sites (Rani et al., 2014; Zapana-Huarache et al., 2020; Hamad et al., 2021), fungi are considered promising candidates as biological indicators in bioassays.
Moreover, fungi possess an extraordinary repertoire of enzymes that makes them able to degrade a wide range of environmental pollutants, even those with complex structures, by combining different mechanisms from their intracellular and extracellular enzymatic systems (Sánchez, 2020; Naveen et al., 2022) (Figure 1). The intracellular enzymatic system, including Phase I (involved in oxidation, as cytochrome P450 family epoxidase) and Phase II enzymes (related to conjugation processes, such as transferases), serves as a detoxifying mechanism and plays an important role in fungal adaptability (Shin et al., 2018; Sánchez, 2020). Additionally, the extracellular enzymatic system, which includes hydrolases (such as the well-known cutinolytic enzymes, able to hydrolyze cuticular polymers even without undergoing interfacial activation) and nonspecific oxidoreductases, comprising the class II peroxidases, laccases, and unspecific peroxygenases, accept a wide range of substrates, acting in the degradation of complex structures and favoring their uptake by the cell (Sánchez, 2020).
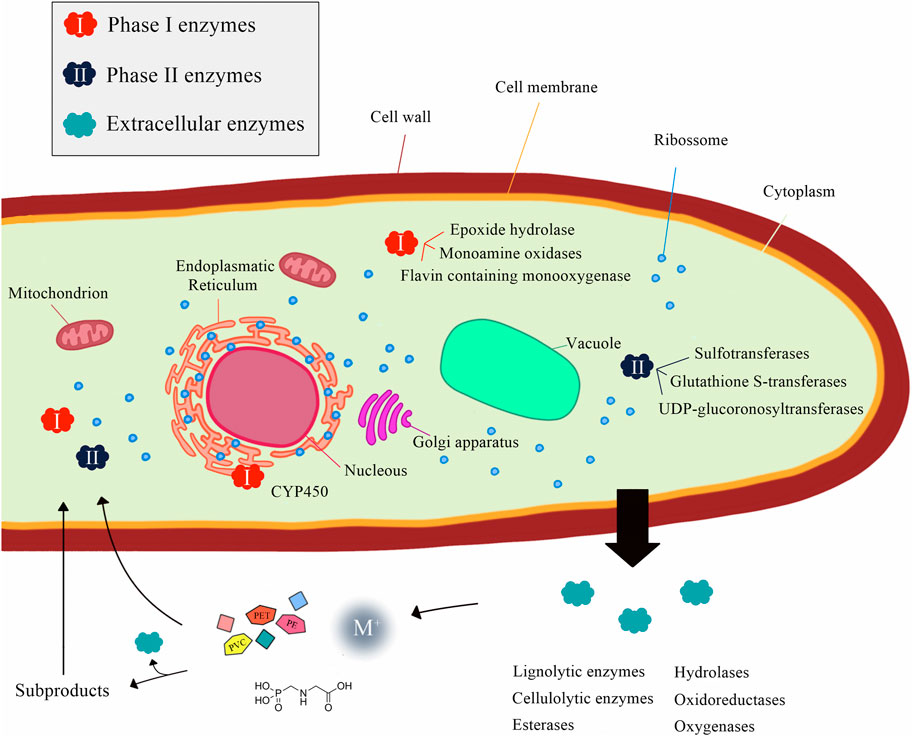
FIGURE 1. Intra- and extracellular enzymatic systems in fungi related to the biodegradation of environmental pollutants.
A great diversity and combination of these enzymatic complexes can be found in several fungal species as, for instance, some wood-degrading basidiomycetes, which produce lignin peroxidases, manganese peroxidases, and laccases, along with oxygenases, oxidases/dehydrogenases, and cellulolytic enzymes (Schwartz et al., 2018; Naveen et al., 2022). In this scenario, the comprehension of the molecular pathways used for fungi exposed to different environmental pollutants could expand their implementation in bioassays for the detection and management of contaminated areas (Sánchez, 2020; Naveen et al., 2022). Herein, we review the application of yeasts, other ascomycetes, and basidiomycetes in ecotoxicological studies to predict the toxicity of environmental pollutants.
Fungal bioassays for environmental monitoring
Fungi, together with bacteria, microalga, and protozoa, are among the frontline biota exposed to environmental pollutants, which makes them an interesting model to be exploited in ecotoxicological assays (Viegas, 2021). Indeed, several fungal species have been employed in bioassays for environmental pollutants monitoring (Table 1).
Most reports regarding the use of fungi in bioassays show the effect of soil pollutants or pure substances, including metal cations, organochlorine, and phenolic compounds, on the growth of mycelium cultures using methodologies based on biomass quantification, enzymatic activity, bioluminescence, and plate occupation diameters (Mendes and Stevani, 2010; Chan-Cheng et al., 2020; Ventura et al., 2020) (Figure 2).
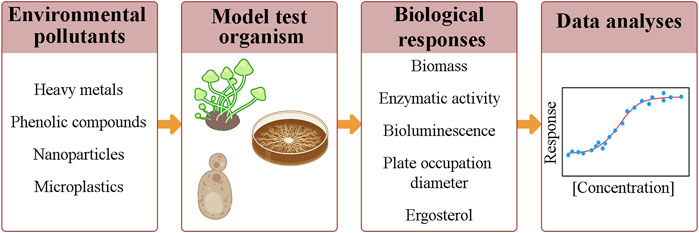
FIGURE 2. Common steps in ecotoxicological assays, which include: the exposure of a model test organism to different concentrations of a chemical compound or dilutions of an environmental sample; the monitoring of biological responses; and the obtention of a concentration-response curve.
Recently, the application of fungi bioluminescence system has been demonstrated to respond, albeit not specifically, to several metal cations such as Cd(II) and Cu(II), and phenolic compounds by bioluminescent basidiomycetes Neonothopanus gardneri, Gerronema viridilucens, Armillaria mellea, and Mycena citricolor (Weitz et al., 2002; Mendes et al., 2010; Mendes and Stevani, 2010; Stevani et al., 2013; Ventura et al., 2020, 2021). In an agar medium, the bioassay with the fungus N. gardneri, which responds in a more sensitive and repeatable way, can be conducted by exposing the mycelium for 30 min or 24 h to an aqueous solution containing the toxicant. Bioluminescence is a precocious endpoint that can indicate the toxic effect of the sample. There is evidence that the decrease of light emission in the presence of the toxicant is related to the uncoupling of mitochondrial oxidative phosphorylation, leading to the impairment of ATP biosynthesis (Ventura et al., 2020). Likewise, other bioluminescent microorganisms, like bacteria, respond to toxic substances by decreasing light emission proportionally to concentration of the toxicant (Hollis et al., 2000; Weitz et al., 2002; Mendes and Stevani, 2010; Ventura et al., 2020, 2021).
Besides detecting air and soil pollutants, different fungal species have also been employed as water bioindicators to sense the quality of the environment (Maurya and Pachauri, 2022). Despite fungi respond to environmental pollution, bioassays using aquatic fungi are scarce, even though they would better mirror the impact of hazardous pollutants in freshwater ecosystems (Ortiz-Vera et al., 2018). The main issue with the use of aquatic fungi in bioassays is the challenge to determine work experimental conditions that are close to the natural ones (Ittner et al., 2018). There are some reports on the use of classical growth-based methodologies, but with fungi isolated from polluted waters (Duddridge and Wainwright, 1980; Chandrashekar and Kaveriappa, 1989; Jaeckel et al., 2005). Nevertheless, it is more common to find metagenomic studies from aquatic fungi instead of ecotoxicological bioassays, once this tool allows the assessment of the impact of a toxic agent in a broader way at the community level (Selvarajan et al., 2019; Usharani, 2019; Ogwugwa et al., 2021; Zhang et al., 2022).
Usually, the fungal communities are evaluated by the quantification of ergosterol, an alternative way to measure growth (Bundschuh et al., 2011; Dimitrov et al., 2014; Zubrod et al., 2015; Donnadieu et al., 2016; Gessner, 2020). Ergosterol is a major membrane component on fungi, with similar functions to cholesterol in animal cells but being absent in animal and plant membranes. Hence, its quantification is a useful method because the mycelium is not easily separated from the leaf tissue and, therefore, an analysis method focusing on specific fungal cell constituents, like chitin or ergosterol, is required for this type of bioassay (Gessner, 2020; Baudy et al., 2021). Additionally, leaf mass loss can be used to measure fungal biomass in aquatic hyphomycetes, a group of saprotrophic fungi adapted to submerged leaf litter (Baudy et al., 2021). Generally, there is a strong linear association between leaf mass loss and the cumulative activity of fungal enzymes (Flores et al., 2014; Baudy et al., 2021).
Due to their high sensitivity to air pollutants, such as nitrogen oxides (NOx) or SO2, fungal associations with roots (mycorrhiza) or algae (lichens) are considered good indicators of air quality (Hage-Ahmed et al., 2019; Castro e Silva et al., 2020; Anderson et al., 2022). Considering that fungal-based air pollution bioassays are scarce, this property becomes promising for developing bioassays for the detection of atmospheric pollutants based on fungal associations. Indeed, most recent studies have focused on correlating air pollution with sporulation or biodiversity of fungal communities present in particulate matter, usually comparing fungal and bacterial diversity by DNA sequencing (Du et al., 2018; Fan et al., 2019; Stevens et al., 2021). However, evidence suggests that spores concentration in air is more affected by meteorological factors than by pollution, a finding that is difficult to confirm due to the strong relationship between air pollution and meteorological factors (Pyrri and Kapsanaki-Gotsi, 2017).
Among fungi, the budding yeast Saccharomyces cerevisiae is considered a relevant animal-alternative tool in ecotoxicological studies, mainly due to its non-pathogenicity, experimental amenability, cost-effectiveness, and the public availability of genomics and bioinformatics resources which aid the interpretation of its biological processes (Viegas, 2021). For instance, the exposure of an engineered strain of Saccharomyces cerevisiae expressing the firefly luciferase gene (luc) from Photinus pyralis to herbicides like mecoprop, diuron, and the Cu ions interferes with cell membrane integrity. This damage leads to defense responses to neutralize the toxic agent that consumes ATP and compete with the ATP-dependent bioluminescence, which ultimately decreases light emission (Martin-Yken, 2020).
Conclusion and perspectives
Fungi are multi or unicellular organisms that have been widely applied as a biological indicator responding to several toxicants. Several reports have shown the efficient use of fungi to assess the toxicity of metals, organic compounds, and inorganic contaminants in bioassays using the inhibition of enzymatic activity, mycelium growth, ergosterol measurement, and bioluminescence intensity as parameters. Additionally, the current genetic engineering approaches in yeasts, such as S. cerevisiae, make possible the expression of optimized-recombinant proteins which, combined with a specific detection system, can expand both the type and the intensity of the response signal (Peltola et al., 2005; Matsuura et al., 2013; Li et al., 2016; Sun and Wang, 2021). Finally, the efficient use of modern techniques of molecular biology such as genome sequencing, heterologous expression of enzymes, the transformation of filamentous fungi, and genetic modifications using the CRISPR/Cas system (Li et al., 2017; Song et al., 2019) can harness tools to simplify the construction of new fungal sensors for more accurate bioassays.
Author contributions
DS, DP, CZ, BN, MB, GR, PL, and AP developed a concept for the mini-review and collected the literature. DS, DP, CZ, BN, MB, GR, PL, and AP wrote the manuscript with input from EB, AO, RF, and CS. All authors contributed to the article and approved the submitted version.
Funding
Financial support of this work was provided by the Fundação de Amparo à Pesquisa do Estado de São Paulo (FAPESP) under grant numbers 2020/16000-3 (BN), 2019/21782-3 (CZ), 2017/22501-2 (EB, CS, AO), and 2019/12605-0 (DS).
Acknowledgments
DP, RF, and CS gratefully acknowledge the support of the RCGI—Research Centre for Gas Innovation, hosted by the University of São Paulo (USP) and sponsored by FAPESP and Shell Brazil, and the strategic importance of the support given by ANP (Brazil’s National Oil, Natural Gas, and Biofuels Agency) through the R&D levy regulation. We also thank the National Council for Scientific and Technological Development (CNPq) for the scientific research awards of EJHB (306460/2016-5), RF (305355/2019-8), CS (303525/2021-5), and the GR’s scholarship (163018/2021-9). AP thanks the Coordination for the Improvement of Higher Education Personnel (CAPES) for grant number 88887613616/2021-00.
Conflict of interest
The authors declare that the research was conducted in the absence of any commercial or financial relationships that could be construed as a potential conflict of interest.
Publisher’s note
All claims expressed in this article are solely those of the authors and do not necessarily represent those of their affiliated organizations, or those of the publisher, the editors and the reviewers. Any product that may be evaluated in this article, or claim that may be made by its manufacturer, is not guaranteed or endorsed by the publisher.
References
Alharbi, O. M. L., Basheer, A. A., Khattab, R. A., and Ali, I. (2018). Health and environmental effects of persistent organic pollutants. J. Mol. Liq. 263, 442–453. doi:10.1016/j.molliq.2018.05.029
Anderson, J., Lévesque, N., Caron, F., Beckett, P., and Spiers, G. A. (2022). A review on the use of lichens as a biomonitoring tool for environmental radioactivity. J. Environ. Radioact. 243, 106797. doi:10.1016/j.jenvrad.2021.106797
Baudy, P., Zubrod, J. P., Konschak, M., Röder, N., Nguyen, T. H., Schreiner, V. C., et al. (2021). Environmentally relevant fungicide levels modify fungal community composition and interactions but not functioning. Environ. Pollut. 285, 117234. doi:10.1016/j.envpol.2021.117234
Bundschuh, M., Zubrod, J. P., Kosol, S., Maltby, L., Stang, C., Duester, L., et al. (2011). Fungal composition on leaves explains pollutant-mediated indirect effects on amphipod feeding. Aquat. Toxicol. 104, 32–37. doi:10.1016/j.aquatox.2011.03.010
Castro e Silva, D. de M., Marcusso, R. M. N., Barbosa, C. G. G., Gonçalves, F. L. T., and Cardoso, M. R. A. (2020). Air pollution and its impact on the concentration of airborne fungi in the megacity of São Paulo, Brazil. Heliyon 6, e05065. doi:10.1016/j.heliyon.2020.e05065
Chan-Cheng, M., Cambronero-Heinrichs, J. C., Masís-Mora, M., and Rodríguez-Rodríguez, C. E. (2020). Ecotoxicological test based on inhibition of fungal laccase activity: Application to agrochemicals and the monitoring of pesticide degradation processes. Ecotoxicol. Environ. Saf. 195, 110419. doi:10.1016/j.ecoenv.2020.110419
Chandrashekar, K. R., and Kaveriappa, K. M. (1989). Effect of pesticides on the growth of aquatic hyphomycetes. Toxicol. Lett. 48, 311–315. doi:10.1016/0378-4274(89)90058-1
Chethana, K. W. T., Manawasinghe, I. S., Hurdeal, V. G., Bhunjun, C. S., Appadoo, M. A., Gentekaki, E., et al. (2021). What are fungal species and how to delineate them? Fungal Divers. 109, 1–25. doi:10.1007/s13225-021-00483-9
Dimitrov, M. R., Kosol, S., Smidt, H., Buijse, L., Van den Brink, P. J., Van Wijngaarden, R. P. A., et al. (2014). Assessing effects of the fungicide tebuconazole to heterotrophic microbes in aquatic microcosms. Sci. Total Environ. 490, 1002–1011. doi:10.1016/j.scitotenv.2014.05.073
Donnadieu, F., Besse-Hoggan, P., Forestier, C., and Artigas, J. (2016). Influence of streambed substratum composition on stream microbial communities exposed to the fungicide tebuconazole. Freshw. Biol. 61, 2026–2036. doi:10.1111/fwb.12679
Du, P., Du, R., Ren, W., Lu, Z., Zhang, Y., and Fu, P. (2018). Variations of bacteria and fungi in PM2.5 in Beijing, China. Atmos. Environ. X. 172, 55–64. doi:10.1016/j.atmosenv.2017.10.048
Duddridge, J. E., and Wainwright, M. (1980). Heavy metal accumulation by aquatic fungi and reduction in viability of Gammarus pulex fed Cd2+ contaminated mycelium. Water Res. 14, 1605–1611. doi:10.1016/0043-1354(80)90065-2
Esposito, M. C., Corsi, I., Russo, G. L., Punta, C., Tosti, E., and Gallo, A. (2021). The era of nanomaterials: A safe solution or a risk for marine environmental pollution? Biomolecules 11, 441. doi:10.3390/biom11030441
Fan, X.-Y., Gao, J.-F., Pan, K.-L., Li, D.-C., Dai, H.-H., and Li, X. (2019). More obvious air pollution impacts on variations in bacteria than fungi and their co-occurrences with ammonia-oxidizing microorganisms in PM2.5. Environ. Pollut. 251, 668–680. doi:10.1016/j.envpol.2019.05.004
Flores, L., Banjac, Z., Farré, M., Larrañaga, A., Mas-Martí, E., Muñoz, I., et al. (2014). Effects of a fungicide (imazalil) and an insecticide (diazinon) on stream fungi and invertebrates associated with litter breakdown. Sci. Total Environ. 476–477, 532–541. doi:10.1016/j.scitotenv.2014.01.059
Garg, A., Yadav, B. K., Das, D. B., and Wood, P. J. (2022). Improving the assessment of polluted sites using an integrated bio-physico-chemical monitoring framework. Chemosphere 290, 133344. doi:10.1016/j.chemosphere.2021.133344
Gessner, M. O. (2020). “Ergosterol as a measure of fungal biomass,” in Methods to study litter decomposition (Cham: Springer International Publishing), 247–255. doi:10.1007/978-3-030-30515-4_27
Hage-Ahmed, K., Rosner, K., and Steinkellner, S. (2019). Arbuscular mycorrhizal fungi and their response to pesticides. Pest Manag. Sci. 75, 583–590. doi:10.1002/ps.5220
Hamad, A. A., Moubasher, H. A., Moustafa, Y. M., Mohamed, N. H., and Abd-el rhim, E. H. (2021). Petroleum hydrocarbon bioremediation using native fungal isolates and consortia. Sci. World J. 2021, 1–13. doi:10.1155/2021/6641533
Hollis, R. P., Killham, K., and Glover, L. A. (2000). Design and application of a biosensor for monitoring toxicity of compounds to eukaryotes. Appl. Environ. Microbiol. 66, 1676–1679. doi:10.1128/AEM.66.4.1676-1679.2000
Hurdeal, V. G., Gentekaki, E., Hyde, K. D., and Jeewon, R. (2021). Where are the basal fungi? Current status on diversity, ecology, evolution, and taxonomy. Biologia 76, 421–440. doi:10.2478/s11756-020-00642-4
Ittner, L. D., Junghans, M., and Werner, I. (2018). Aquatic fungi: A disregarded trophic level in ecological risk assessment of organic fungicides. Front. Environ. Sci. 6. doi:10.3389/fenvs.2018.00105
Jaeckel, P., Krauss, G.-J., and Krauss, G. (2005). Cadmium and zinc response of the fungi Heliscus lugdunensis and Verticillium cf. alboatrum isolated from highly polluted water. Sci. Total Environ. 346, 274–279. doi:10.1016/j.scitotenv.2004.12.082
Li, D., Tang, Y., Lin, J., and Cai, W. (2017). Methods for genetic transformation of filamentous fungi. Microb. Cell. Fact. 16, 168. doi:10.1186/s12934-017-0785-7
Li, J., Liu, Y., Kong, D., Ren, S., and Li, N. (2016). T-screen and yeast assay for the detection of the thyroid-disrupting activities of cadmium, mercury, and zinc. Environ. Sci. Pollut. Res. 23, 9843–9851. doi:10.1007/s11356-016-6095-5
Li, Z., Xie, Y., Zeng, Y., Zhang, Z., Song, Y., Hong, Z., et al. (2022). Plastic leachates lead to long-term toxicity in fungi and promote biodegradation of heterocyclic dye. Sci. Total Environ. 806, 150538. doi:10.1016/j.scitotenv.2021.150538
Mahanty, S., Tudu, P., Ghosh, S., Chatterjee, S., Das, P., Bhattacharyya, S., et al. (2021). Chemometric study on the biochemical marker of the manglicolous fungi to illustrate its potentiality as a bio indicator for heavy metal pollution in Indian Sundarbans. Mar. Pollut. Bull. 173, 113017. doi:10.1016/j.marpolbul.2021.113017
Martin-Yken, H. (2020). Yeast-based biosensors: Current applications and new developments. Biosensors 10, 51. doi:10.3390/bios10050051
Matsuura, H., Yamamoto, Y., Muraoka, M., Akaishi, K., Hori, Y., Uemura, K., et al. (2013). Development of surface-engineered yeast cells displaying phytochelatin synthase and their application to cadmium biosensors by the combined use of pyrene-excimer fluorescence. Biotechnol. Prog. 29, 1197–1202. doi:10.1002/btpr.1789
Maurya, G. K., and Pachauri, S. (2022). “Fungi: The indicators of pollution,” in Freshwater mycology (Elsevier), 277–296. doi:10.1016/B978-0-323-91232-7.00012-X
Mendes, L. F., Bastos, E. L., and Stevani, C. V. (2010). Prediction of metal cation toxicity to the bioluminescent fungus Gerronema viridilucens. Environ. Toxicol. Chem. 29, 2177–2181. doi:10.1002/etc.283
Mendes, L. F., and Stevani, C. V. (2010). Evaluation of metal toxicity by a modified method based on the fungus Gerronema viridilucens bioluminescence in agar medium. Environ. Toxicol. Chem. 29, 320–326. doi:10.1002/etc.42
Naveen, K. V., Saravanakumar, K., Zhang, X., Sathiyaseelan, A., and Wang, M.-H. (2022). Impact of environmental phthalate on human health and their bioremediation strategies using fungal cell factory- A review. Environ. Res. 214, 113781. doi:10.1016/j.envres.2022.113781
Ogwugwa, V. H., Oyetibo, G. O., and Amund, O. O. (2021). Taxonomic profiling of bacteria and fungi in freshwater sewer receiving hospital wastewater. Environ. Res. 192, 110319. doi:10.1016/j.envres.2020.110319
Ortiz-Vera, M. P., Olchanheski, L. R., da Silva, E. G., de Lima, F. R., Martinez, L. R., del, P. R., et al. (2018). Influence of water quality on diversity and composition of fungal communities in a tropical river. Sci. Rep. 8, 14799. doi:10.1038/s41598-018-33162-y
Peltola, P., Ivask, A., Åström, M., and Virta, M. (2005). Lead and Cu in contaminated urban soils: Extraction with chemical reagents and bioluminescent bacteria and yeast. Sci. Total Environ. 350, 194–203. doi:10.1016/j.scitotenv.2005.01.029
Pyrri, I., and Kapsanaki-Gotsi, E. (2017). Functional relations of airborne fungi to meteorological and pollution factors in a Mediterranean urban environment. Fungal Ecol. 30, 48–54. doi:10.1016/j.funeco.2017.08.007
Rani, B., Kumar, V., Singh, J., Bisht, S., Teotia, P., Sharma, S., et al. (2014). Bioremediation of dyes by fungi isolated from contaminated dye effluent sites for bio-usability. Braz. J. Microbiol. 45, 1055–1063. doi:10.1590/S1517-83822014000300039
Sánchez, C. (2020). Fungal potential for the degradation of petroleum-based polymers: An overview of macro- and microplastics biodegradation. Biotechnol. Adv. 40, 107501. doi:10.1016/j.biotechadv.2019.107501
Schwartz, M., Perrot, T., Aubert, E., Dumarçay, S., Favier, F., Gérardin, P., et al. (2018). Molecular recognition of wood polyphenols by phase II detoxification enzymes of the white rot Trametes versicolor. Sci. Rep. 8, 8472. doi:10.1038/s41598-018-26601-3
Seena, S., Graça, D., Bartels, A., and Cornut, J. (2019). Does nanosized plastic affect aquatic fungal litter decomposition? Fungal Ecol. 39, 388–392. doi:10.1016/j.funeco.2019.02.011
Selvarajan, R., Sibanda, T., Sekar, S., and Nel, W. (2019). Industrial effluents harbor a unique diversity of fungal community structures as revealed by high-throughput sequencing analysis. Pol. J. Environ. Stud. 28, 2353–2362. doi:10.15244/pjoes/90791
Shin, J., Kim, J.-E., Lee, Y.-W., and Son, H. (2018). Fungal cytochrome P450s and the P450 complement (CYPome) of Fusarium graminearum. Toxins (Basel). 10, 112. doi:10.3390/toxins10030112
Song, R., Zhai, Q., Sun, L., Huang, E., Zhang, Y., Zhu, Y., et al. (2019). CRISPR/Cas9 genome editing technology in filamentous fungi: Progress and perspective. Appl. Microbiol. Biotechnol. 103, 6919–6932. doi:10.1007/s00253-019-10007-w
Stevani, C. V., Oliveira, A. G., Mendes, L. F., Ventura, F. F., Waldenmaier, H. E., Carvalho, R. P., et al. (2013). Current status of research on fungal bioluminescence: Biochemistry and prospects for ecotoxicological application. Photochem. Photobiol. 89, 1318–1326. doi:10.1111/php.12135
Stevens, V., Thijs, S., Bongaerts, E., Nawrot, T., Marchal, W., Van Hamme, J., et al. (2021). Ambient air pollution shapes bacterial and fungal ivy leaf communities. Microorganisms 9, 2088. doi:10.3390/microorganisms9102088
Sun, A., and Wang, W.-X. (2021). Adenine deficient yeast: A fluorescent biosensor for the detection of labile Zn(II) in aqueous solution. Biosens. Bioelectron. X. 179, 113075. doi:10.1016/j.bios.2021.113075
Tabrez, S., and Ahmad, M. (2011). Some enzymatic/nonenzymatic antioxidants as potential stress biomarkers of trichloroethylene, heavy metal mixture, and ethyl alcohol in rat tissues. Environ. Toxicol. 26, 207–216. doi:10.1002/tox.20548
Usharani, B. (2019). Metagenomics study of the microbes in constructed wetland system treating sewage. Int. Lett. Nat. Sci. 74, 26–48. doi:10.18052/www.scipress.com/ILNS.74.26
Ventura, F. F., Soares, D. M. M., Bayle, K., Oliveira, A. G., Bechara, E. J. H., Freire, R. S., et al. (2021). Toxicity of metal cations and phenolic compounds to the bioluminescent fungus Neonothopanus gardneri. Environ. Adv. 4, 100044. doi:10.1016/j.envadv.2021.100044
Ventura, F. F., Mendes, L. F., Oliveira, A. G., Bazito, R. C., Bechara, E. J. H., Freire, R. S., et al. (2020). Evaluation of phenolic compound toxicity using a bioluminescent assay with the fungus Gerronema viridilucens. Environ. Toxicol. Chem. 39, 1558–1565. doi:10.1002/etc.4740
Viegas, C. A. (2021). Microbial bioassays in environmental toxicity testing. Adv Appl Microbiol. 115, 115–158. doi:10.1016/bs.aambs.2021.03.002
Wasi, S., Jeelani, G., and Ahmad, M. (2008). Biochemical characterization of a multiple heavy metal, pesticides and phenol resistant Pseudomonas fluorescens strain. Chemosphere 71, 1348–1355. doi:10.1016/j.chemosphere.2007.11.023
Wasi, S., Tabrez, S., and Ahmad, M. (2013). Toxicological effects of major environmental pollutants: An overview. Environ. Monit. Assess. 185, 2585–2593. doi:10.1007/s10661-012-2732-8
Weitz, H. J., Campbell, C. D., and Killham, K. (2002). Development of a novel, bioluminescence-based, fungal bioassay for toxicity testing. Environ. Microbiol. 4, 422–429. doi:10.1046/j.1462-2920.2002.00315.x
Zapana-Huarache, S. V., Romero-Sánchez, C. K., Gonza, A. P. D., Torres-Huaco, F. D., and Rivera, A. M. L. (2020). Chromium (VI) bioremediation potential of filamentous fungi isolated from Peruvian tannery industry effluents. Braz. J. Microbiol. 51, 271–278. doi:10.1007/s42770-019-00209-9
Zenker, A., Cicero, M. R., Prestinaci, F., Bottoni, P., and Carere, M. (2014). Bioaccumulation and biomagnification potential of pharmaceuticals with a focus to the aquatic environment. J. Environ. Manage. 133, 378–387. doi:10.1016/j.jenvman.2013.12.017
Zhang, M., Zhou, Z., Zhang, J., Yu, Y., Sun, L., Lu, T., et al. (2022). Metagenomic ecotoxicity assessment of trace difenoconazole on freshwater microbial community. Chemosphere 294, 133742. doi:10.1016/j.chemosphere.2022.133742
Zubrod, J. P., Feckler, A., Englert, D., Koksharova, N., Rosenfeldt, R. R., Seitz, F., et al. (2015). Inorganic fungicides as routinely applied in organic and conventional agriculture can increase palatability but reduce microbial decomposition of leaf litter. J. Appl. Ecol. 52, 310–322. doi:10.1111/1365-2664.12393
Keywords: ascomycete, basidiomycete, bioluminescence, ecotoxicology, metal cation, organic pollutant
Citation: Soares DMM, Procópio DP, Zamuner CK, Nóbrega BB, Bettim MR, de Rezende G, Lopes PM, Pereira ABD, Bechara EJH, Oliveira AG, Freire RS and Stevani CV (2022) Fungal bioassays for environmental monitoring. Front. Bioeng. Biotechnol. 10:954579. doi: 10.3389/fbioe.2022.954579
Received: 27 May 2022; Accepted: 02 August 2022;
Published: 25 August 2022.
Edited by:
Ranjit Gurav, Konkuk University, South KoreaReviewed by:
Maruti Dhanavade, Bharati Vidyapeeth’s Dr. Patangrao Kadam Mahavidyalaya Sangli, IndiaMayur B. Kurade, Hanyang University, South Korea
Copyright © 2022 Soares, Procópio, Zamuner, Nóbrega, Bettim, de Rezende, Lopes, Pereira, Bechara, Oliveira, Freire and Stevani. This is an open-access article distributed under the terms of the Creative Commons Attribution License (CC BY). The use, distribution or reproduction in other forums is permitted, provided the original author(s) and the copyright owner(s) are credited and that the original publication in this journal is cited, in accordance with accepted academic practice. No use, distribution or reproduction is permitted which does not comply with these terms.
*Correspondence: Cassius V. Stevani, c3RldmFuaUBpcS51c3AuYnI=