- 1Key Laboratory of Prevention and Treatment of Cardiovascular and Cerebrovascular Diseases, Ministry of Education, Key Laboratory of Biomaterials and Biofabrication in Tissue Engineering of Jiangxi Province, Key Laboratory of Biomedical Sensors of Ganzhou, School of Public Health and Health Management, School of Medical and Information Engineering, Gannan Medical University, Ganzhou, China
- 2Oil-Tea in Medical Health Care and Functional Product Development Engineering Research Center in Jiangxi, The Science Research Center, School of Pharmacy, Gannan Medical University, Ganzhou, China
Coronavirus disease 19 (COVID-19) is still a major public health concern in many nations today. COVID-19 transmission is now controlled mostly through early discovery, isolation, and therapy. Because of the severe acute respiratory syndrome coronavirus 2 (SARS-CoV-2) is the contributing factor to COVID-19, establishing timely, sensitive, accurate, simple, and budget detection technologies for the SARS-CoV-2 is urgent for epidemic prevention. Recently, several electrochemical DNA biosensors have been developed for the rapid monitoring and detection of SARS-CoV-2. This mini-review examines the latest improvements in the detection of SARS-COV-2 utilizing electrochemical DNA biosensors. Meanwhile, this mini-review summarizes the problems faced by the existing assays and puts an outlook on future trends in the development of new assays for SARS-CoV-2, to provide researchers with a borrowing role in the generation of different assays.
Introduction
The severe acute respiratory syndrome coronavirus 2 (SARS-CoV-2), a new member of the β-coronavirus genus, is closely related to SARS-CoV and is also the seventh coronavirus to infect humans (Akalin et al., 2020; Wiersinga et al., 2020; Zu et al., 2020), causing severe respiratory symptoms such as fever (37.3°C), cough and expectoration, nasal obstruction or even dyspnea in humans. A few people developed gastrointestinal symptoms (GI) (Jin et al., 2020a), such as nausea, vomiting, and diarrhea. Although the 2003 SARS-CoV, 2012 Middle East Respiratory Syndrome (MERS-COV), and the current epidemic of SARS-CoV-2 are all Human coronaviruse (HCoV) strains, SARS-CoV-2 is more infectious and pathogenic. At present, the main sources of infection of the disease include patients with COVID-19 patients, asymptomatic infections, and latent infections, which can be transmitted from person to person through droplets, contact, aerosols, and other transmission routes (Anderson et al., 2020; Kalbusch et al., 2020; Lin et al., 2020; Fu et al., 2021; Huang et al., 2021; Pan et al., 2021; Yip et al., 2021). It has been reported that one COVID-19 patient can transmit to three people at the same time (Qing et al., 2020), and the population is generally susceptible, especially elderly patients with chronic diseases who are more likely to become critically ill (Adhikari et al., 2020).
The single-stranded positive-stranded RNA virus, SARS-CoV-2, is 80–220 nm in diameter and has 12–24 nm vesicular rod like spikes. (Figure 1A) (Bullock and Tamin, 2020; Huang et al., 2020c; Lu et al., 2020; Zhou et al., 2020). At the same time, it is also a single-stranded positive-stranded RNA virus with typical “coronavirus” morphological characteristics. Its homology with SARS-COV and MERS genome sequences is close to 79% and 50%, respectively (Lu et al., 2020; Zhou et al., 2020), so it can be modified to detect SARS-CoV-2 by referring to previous detection methods of SARS-COV. The genome of SARS-CoV-2 consists of two noncoding (5′-terminal noncoding region and 3′-terminal noncoding region) and five coding regions (an open reading box 1a/b (ORF1a/b), the S region encoding spinous glycoprotein (S protein), the E region encoding envelope protein (E protein), the M region encoding membrane protein (M protein) and the N region encoding nucleocapsid protein (N protein)) (Xu et al., 2020; Yang and Wang, 2020) (Figure 1B). Among them, ORF1a/b genes are responsible for viral genome replication, transcription and translation (Jalandra et al., 2020; Kirtipal et al., 2020; Udugama et al., 2020; Yang and Wang, 2020). The virus on the surface of the outer coating is mainly composed of four structural proteins, the S protein, the E protein, the M protein and the N protein (Nie et al., 2020). S protein, which enables the virus to enter the host cell, can be used for vaccine, research and development of therapeutic antibodies and diagnosis depending on its advantages. Proteins M and E are responsible for forming the envelope of the virus, whereas the N protein participates in the assembly of viruses (Jalandra et al., 2020; Udugama et al., 2020; Yang and Wang, 2020).
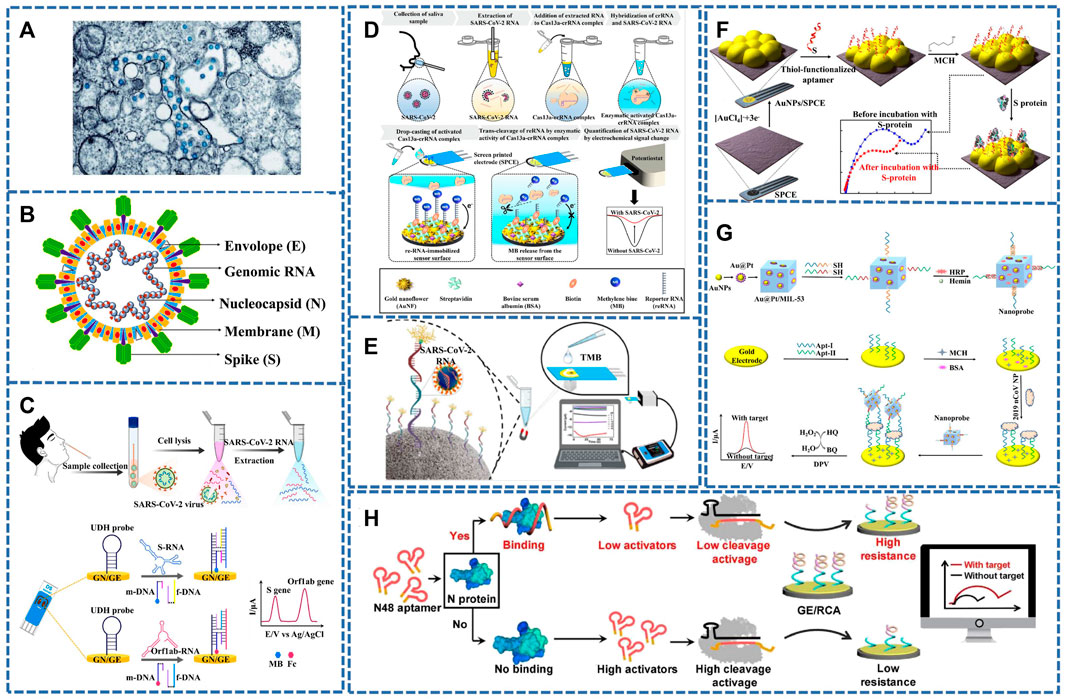
FIGURE 1. (A) TEM image of SARS-CoV-2 (the blue globules) (Bullock and Tamin, 2020). (B) SARS-CoV-2’s typical structure, S, M, E, and N proteins, encapsulates genomic RNA within virus particles (Kirtipal et al., 2020). (C) A detection workflow of SARS-CoV-2 RNA sequences from clinical samples using the electrochemical biosensor for detection of the S and Orf1ab genes (Kashefi-Kheyrabadi et al., 2022). (D) Schematic diagram of an electrochemical biosensing strategy for SARS-CoV-2 detection using CRISPR/Cas13a. (Heo et al., 2022). (E) Schematic diagram of an electrochemical gene sensor for detecting SARS-COV-2 (Cajigas et al., 2022). (F) Diagram of the preparation steps of the sensor for detecting SARS-CoV-2 S protein. (Abrego-Martinez et al., 2022). (G) Schematic diagram of constructing electrochemical sensor based on Au@Pt/MIL-53 (Tian et al., 2021). (H) Strategy and workflow of SARS-COV-2 antigen detection by CRISPR-based electrochemical sensor (Liu et al., 2022).
At the early stage of the outbreak, metagenomic sequencing obtained the whole genome sequence of the SARS-CoV-2 virus, which provided the basis for the classification of SARS-CoV-2 as a new member of the genus β-coronavirus. Although metagenomic sequencing is costly, it not only provides technical support for the analysis of the origin and pathogenesis of SARS-CoV-2 but also lays the foundation for the development of novel SARS-CoV-2 detection methods (Lu et al., 2020; Wu et al., 2020). As detection methods have improved, the commonly used methods include polymerase chain reaction (PCR) (Yuan et al., 2020), reverse transcription PCR (RT-PCR) (Huang et al., 2020a; Hu et al., 2020; Xie et al., 2020), reverse transcription loop-mediated isothermal amplification (RT-LAMP) (Baek et al., 2020; Yan et al., 2020), regularly interspaced short palindromic repeats-CRISPR-associated (CRISPR-Cas) (Broughton et al., 2020; Ding et al., 2020) and nucleic acid biosensors (Alafeef et al., 2020; Zhu et al., 2020). Currently, Although PCR can yield results with less viral genetic material. However, we still need more sensitive, simple, accurate, and inexpensive rapid assays for SARS-CoV-2 detection.
Electrochemical biosensors could provide the possibility for rapid medical diagnosis by rapid detection of biomarkers (Chen et al., 2018; Huang et al., 2020b; Lu et al., 2021; Raza et al., 2021; Huang et al., 2022), which have been used to detect Zika virus (Afsahi et al., 2018), Ebola virus (Ilkhani and Farhad, 2018), HIV(Nandi et al., 2020), influenza virus (Krishna et al., 2016; Chowdhury et al., 2019), etc. With the advancement of COVID-19 research, electrochemical biosensors, especially electrochemical DNA biosensors, have been widely studied in the detection of SARS-CoV-2.
This mini-review covers the recent progress of the rapid detection of SARS-COV-2 by using electrochemical DNA biosensors for the first time. What’s more, this mini-review summarizes the problems faced by the existing assays and puts an outlook on future trends in the development of new assays for SARS-CoV-2, which can provide researchers with a borrowing role in the development of new assays.
Electrochemical DNA Sensor Detection of SARS-COV-2
Electrochemical DNA biosensors for the detection of viruses and bacteria are now widely used due to their advantages of higher sensitivity, less sample size, low cost, simplicity, and portability (Adam et al., 2020; Lin et al., 2021; Huang et al., 2022; Mei et al., 2022). The electrochemical DNA biosensors are based on the single-strand DNA or complementary target DNA fixed on the electrode and the target DNA through the principle of base complementarity, resulting in changes in concentration, energy, and other aspects, and this change can be converted into visual electrical signals through the appropriate conversion elements on the sensor (Huang et al., 2020b; Karimi-Maleh et al., 2021). Therefore, they combine biological specific recognition with electrochemical high sensitivity analysis to further realize the detection and analysis of the target, so that it has the potential of immediate detection and diagnosis.
Specific Detection of SARS-COV-2 Nucleic Acid
Recently, nanomaterials have been extensively applied in life science, energy science and other fields (Xu et al., 2019; Zhuang et al., 2019; Kuang et al., 2020; Wen et al., 2020; Chen et al., 2021; He et al., 2021; Liu et al., 2021; Savchenko et al., 2021; Schultz et al., 2021; Wu et al., 2021; Xu and Liu, 2021; He et al., 2022; Yi et al., 2022; Zhuang et al., 2022). At present, nano-materials in electrochemical biosensors have also been broadly concerned for gauging SARS-COV-2. Graphene (G) is considered one of the suitable materials for sensor applications due to its special good properties such as physical properties, electronics as well as oxygen-containing groups, which are introduced in the construction of biosensors and can improve the analytical parameters of electrochemical biosensors (Abdel-Haleem et al., 2021; Meng et al., 2021). Alafeef et al. developed an electrochemical biosensor chip constructed by G that can selectively recognize SARS-CoV-2 RNA (N gene) (Alafeef et al., 2020). It was obtained by immobilizing highly specific ssDNA (antisense oligonucleotides)-capped gold nanoparticles (AuNPs) probes of viral N gene onto G coated filter paper. AuNPs achieve the goal of improving the electrochemical response of the sensor to the target by enhancing the electron transfer process and providing a large surface area for the ssDNA probe. G-ssDNA-AuNPs-built monitoring platform can read the results in 5 min with a handheld reader. When combined with benchtop devices, point-of-care (POC) testing can be offered to economically lag, resource-poor areas. Zhao et al. 2021) synthesized an ultra-sensitive sandwich electrochemical sensor using calixarene functionalized go and SARS-CoV-2 targeted RNA with limit of detection (LOD) as low as 200 copies/mL for clinical samples. Based on this sensor, a smartphone can detect SARS-CoV-2. Moreover, the detection process can detect SARS-CoV-2 samples sensitively, accurately and rapidly without RNA amplification, which also provides effective suggestions for low-cost and simple POC diagnosis.
Rolling circle amplification (RCA) and catalytic hairpin assembly (CHA) in the nucleic acid amplification strategy can be combined with electrochemical analysis to accurately amplify electrochemical signals. Chaibun et al. (2021) designed the electrochemical biosensor for SARS-CoV-2 (S or N gene) RNA based on RCA. The technique can detect viruses as low as one copy/μl in 2 h by differential pulse voltammetry (DPV). It was evaluated in conjunction with quantitative RT-PCR (qRT-PCR) on 106 clinical samples, of which 41 were positive (SARS-COV-2) and nine other samples were positive for other respiratory viruses, with 100% agreement between the two methods. Peng et al. (2021) prepared an electrochemical sensor based on CHA and terminal deoxynucleotidyl transferase (TdT) induced polymerization. When the target gene (ORF1ab gene) combined with hairpin HP1 and HP2 to form a Y-type DNA structure, TdT induced polymerization was further activated. A great quantity of long single-stranded DNA products are generated in the dNTP pool, during which a large number of Ru(NH3)63+ adsorbs on the DNA phosphoric acid skeleton through strong electrostatic interaction. Therefore, this method can be used to obtain evidently boosted electrochemical signals for sensitive monitoring of SARS-COV-2. Kashefi-kheyrabadi et al. (2022) developed an electrochemical sensor without nucleic acid amplification. The detection process is shown in Figure 1C, the S and ORF1ab genes of SARS-CoV-2 can be detected simultaneously within 1 h with LOD as low as 5.0 and 6.8 ag/μl.
Heo et al. (2022) combined CRISPR/Cas13a with the electrochemical biosensor. The detection process is shown in Figure 1D. When the single-stranded RNA (ssRNA) probe was recognized by the SARS-CoV-2 RNA phase, the Cas13a-crRNA complex was formed, which was then introduced into the reporter RNA (reRNA)-coupled electrochemical sensor to activate RNase, thereby cutting reRNA. In this process, redox molecules released by reRNA will cause changes in their current, thus achieving the purpose of sensitive detection. Amplification-free sensors designed by the team enable ultra-low concentration testing of SARS-CoV-2 RNA. This opens the possibility of on-site and high-speed diagnostic COVID-19 testing.
Although many sensors are available to monitor SARS-COV-2, false-positive results from its homologous viruses cannot be ruled out. Cajigas et al. (2022) reported an electrochemical biosensor that can specifically detect SARS-COV-2 and distinguish the homologous viruses of SARS-CoV, Middle East Respiratory Syndrome (MERS), and Human Coronavirus (HKU1) (Figure 1E). Biosensors with immobilized capture probes were combined with modified magnetic beads (MMBS), while the capture probes were first hybridized with the targets and then hybridized with biotinylated signal probes in a sandwich format. The biotinylated signal probe allowed interaction with one of three distinct protein-enzyme compounds containing distinct numbers of horse radish peroxidase (HRP) molecules to generate visual electrical signals by the timing current method. The prepared biosensor provides the possibility to check infected and asymptomatic patients. At the same time, it will also contribute to resisting the COVID-19 pandemic.
Specific Detection of SARS-COV-2 VIRUS/PROTEIN
With the development of nucleic acid testing, there are broad prospects for virus detection, but if the viral RNA is mutated, it can produce false-negative results, so researchers switched to detecting SARS-CoV-2-related proteins. Such as receptor-binding domain (RBD) (Jalandra et al., 2020; Udugama et al., 2020), S protein (Jin et al., 2020b; Wu et al., 2020) and N protein. Therefore, Abrego-Martinez et al. (2022) prepared a biosensor for detecting SARS-CoV-2 (S protein) by fixing probe ssDNA on gold nanoparticles (Figure 1F). Its advantages were fast detection speed, low detection limit (1.30 p.m.), and results that could be obtained in 40 min, but SARS-CoV had a certain response to it, which was negligible compared to SARS-CoV-2. The direct detection of SARS-CoV-2 was achieved by immobilizing ssDNA AuNPs on screen-printed electrode, which was also applied in a handheld potentiostat linked to a smartphone. To further reduce costs, Curti et al. prepared a biosensor targeting SARS-CoV-2 S1 by using an inexpensive and highly conductive single-wall carbon nanotube screen-printed electrode (SWCNT-SPE). The adapted ssDNA can block virus infection in vitro. It is possible to prepare multifunctional sensors (Curti et al., 2022).
Designing a highly sensitive assay is urgent for the early diagnosis and treatment of SARS-CoV-2. Tian et al. (2021) designed a diaptamer sensor for highly selective recognition of SARS-CoV-2 N protein using the metal-organic framework MIL-53 Au@Pt Nanoparticles and enzymes, as shown in the Figure 1G. The detection limit was as low as 8.33 pg/ml.
CRISPR-Cas is an efficient, simple and powerful gene targeting technology (Liu and Fan, 2014), and the nucleic acid detection of CRISPR/Cas nuclease holds great promise for the development of SARS-COV-2 diagnostics under high sensitivity, specificity and reliability (Chertow Daniel, 2018; Li et al., 2019). Liu et al. (2022) combined this method with electrochemical analysis to prepare a sensor for SARS-COV-2 virus detection (Figure 1H). the signal of electrochemical impedance spectroscopy (EIS) signal was linked to the morphology and presence of the RCA-DNA structure, which was determined by the DNA cleavage activity of Cas12a attachment regulated by target-induced competition. Therefore the sensor was also responsible for its ability to test SARS-COV-2 with high specificity.
The nucleic acid and virus/protein determinations are summarised in Table 1. Compared to the traditional testing methods (PCR, RT-PCR), electrochemical DNA sensors have demonstrated their ability to detect SARS-CoV-2 with ease of use, no need for expensive instruments, lower detection limits, higher sensitivity, and specificity. In particular, it has the advantage of shorter detection times, demonstrating its ability to detect and control outbreaks rapidly. The miniaturised electrochemical biosensor can be combined with lateral flow assay (LFA), loop-mediated isothermal amplification (LAMP), RT-LAMP approaches, clustered regularly interspaced short palindromic repeats (CRISPR) and other methods to further increase sensitivity. In fact, we also hope that by comparing the different assays, other researchers will be able to create more sensitive, rapid, economical, and accurate COVID-19 assays.
Conclusion and Perspectives
The rapid, sensitive and accurate determination of SARS-CoV-2 is crucial for the prevention and control of the epidemic. Electrochemical DNA biosensors have the advantages of high sensitivity, high selectivity, and economical portability, et al., which have been gradually applied to SARS-CoV-2 detection. In this mini-review, the latest researches on electrochemical DNA biosensors for the monitoring of SARS-CoV-2 in recent years have been summarized. Although there are many electrochemical DNA biosensors for SARS-CoV-2 detection, there are still some opportunities and challenges: 1) the preparation of working electrode materials should be simpler; 2) the stability of the electrochemical DNA biosensors should be guaranteed; 3) how to achieve simultaneous detection of multiple different genes? Of course, with the further in-depth research, the above problems will be effectively solved, which will provide the possibility for the commercial application of electrochemical biosensors for SARS-CoV-2 detection.
Author Contributions
YM: defined the focus of the review. CH, WZ, and YL: writing. CL, and ZL: editing. MY and YK: reviewing. XL and QH: writing, reviewing and editing.
Funding
This work has been supported by the National Natural Science Foundation of China (No. 82060599), the Natural Science Foundation of Jiangxi (No. 20202BABL213018), the Science and Technology Project of the Education Department of Jiangxi Province (Nos. GJJ190795 and GJJ211517), the Science and Technology Project of Jiangxi Health Committee (No. 202131033), the Research Fund of Gannan Medical University (Nos. ZD201901, YQ202003, and YB201911), the Open Fund of Fujian Provincial Key Laboratory of Modern Analytical Science and Separation Technology and the Science and Technology (No. K2017-01), the Plan Post-subsidy Project of Ganzhou (No. 2019-60-174) and the Special Fund for Graduate Innovation in Jiangxi Province (No. YC2021-S796).
Conflict of Interest
The authors declare that the research was conducted in the absence of any commercial or financial relationships that could be construed as a potential conflict of interest.
Publisher’s Note
All claims expressed in this article are solely those of the authors and do not necessarily represent those of their affiliated organizations, or those of the publisher, the editors and the reviewers. Any product that may be evaluated in this article, or claim that may be made by its manufacturer, is not guaranteed or endorsed by the publisher.
References
Abdel-Haleem, F. M., Gamal, E., Rizk, M. S., Madbouly, A., El Nashar, R. M., Anis, B., et al. (2021). Molecularly Imprinted Electrochemical Sensor-Based Fe2O3@MWCNTs for Ivabradine Drug Determination in Pharmaceutical Formulation, Serum, and Urine Samples. Front. Bioeng. Biotechnol. 9, 648704. doi:10.3389/fbioe.2021.648704
Abrego-Martinez, J. C., Jafari, M., Chergui, S., Pavel, C., Che, D., and Siaj, M. (2022). Aptamer-based Electrochemical Biosensor for Rapid Detection of SARS-CoV-2: Nanoscale Electrode-Aptamer-SARS-CoV-2 Imaging by Photo-Induced Force Microscopy. Biosens. Bioelectron. 195, 113595. doi:10.1016/j.bios.2021.113595
Adam, N., Schlicht, S., Han, Y., Bechelany, M., Bachmann, J., and Perner, M. (2020). Metagenomics Meets Electrochemistry: Utilizing the Huge Catalytic Potential from the Uncultured Microbial Majority for Energy-Storage. Front. Bioeng. Biotechnol. 8, 567. doi:10.3389/fbioe.2020.00567
Adhikari, S. P., Meng, S., Wu, Y.-J., Mao, Y.-P., Ye, R.-X., Wang, Q.-Z., et al. (2020). Epidemiology, Causes, Clinical Manifestation and Diagnosis, Prevention and Control of Coronavirus Disease (COVID-19) during the Early Outbreak Period: a Scoping Review. Infect. Dis. Poverty 9, 29. doi:10.1186/s40249-020-00646-x
Afsahi, S., Lerner, M. B., Goldstein, J. M., Lee, J., Tang, X., Bagarozzi, D. A., et al. (2018). Novel Graphene-Based Biosensor for Early Detection of Zika Virus Infection. Biosens. Bioelectron. 100, 85–88. doi:10.1016/j.bios.2017.08.051
Akalin, E., Azzi, Y., Bartash, R., Seethamraju, H., Parides, M., Hemmige, V., et al. (2020). Covid-19 and Kidney Transplantation. N. Engl. J. Med. 382 (25), 2475–2477. doi:10.1056/NEJMc2011117
Alafeef, M., Dighe, K., Moitra, P., and Pan, D. (2020). Rapid, Ultrasensitive, and Quantitative Detection of SARS-CoV-2 Using Antisense Oligonucleotides Directed Electrochemical Biosensor Chip. ACS Nano 14 (12), 17028–17045. doi:10.1021/acsnano.0c06392
Amouzadeh Tabrizi, M., and Acedo, P. (2022). An Electrochemical Impedance Spectroscopy-Based Aptasensor for the Determination of SARS-CoV-2-RBD Using a Carbon Nanofiber–Gold Nanocomposite Modified Screen-Printed Electrode. Biosensors 12 (3), 142. doi:10.3390/bios12030142
Anderson, R. M., Hollingsworth, T. D., Baggaley, R. F., Maddren, R., and Vegvari, C. (2020). COVID-19 Spread in the UK: the End of the Beginning? Lancet 396 (10251), 587–590. doi:10.1016/S0140-6736(20)31689-5
Ang, W. L., Lim, R. R. X., Ambrosi, A., and Bonanni, A. (2022). Rapid Electrochemical Detection of COVID-19 Genomic Sequence with Dual-Function Graphene Nanocolloids Based Biosensor. FlatChem 32, 100336. doi:10.1016/j.flatc.2022.100336
Avelino, K. Y. P. S., Dos Santos, G. S., Frías, I. A. M., Silva-Junior, A. G., Pereira, M. C., Pitta, M. G. R., et al. (2021). Nanostructured Sensor Platform Based on Organic Polymer Conjugated to Metallic Nanoparticle for the Impedimetric Detection of SARS-CoV-2 at Various Stages of Viral Infection. J. Pharm. Biomed. Analysis 206, 114392. doi:10.1016/j.jpba.2021.114392
Baek, Y. H., Um, J., Antigua, K. J. C., Park, J.-H., Kim, Y., Oh, S., et al. (2020). Development of a Reverse Transcription-Loop-Mediated Isothermal Amplification as a Rapid Early-Detection Method for Novel SARS-CoV-2. Emerg. Microbes Infect. 9 (1), 998–1007. doi:10.1080/22221751.2020.1756698
Broughton, J. P., Deng, X., Yu, G., Fasching, C. L., Servellita, V., Singh, J., et al. (2020). CRISPR-Cas12-based Detection of SARS-CoV-2. Nat. Biotechnol. 38 (7), 870–874. doi:10.1038/s41587-020-0513-4
Bullock, H. A., and Tamin, A. (2020). Transmission Electron Microscopic Image of an Isolate from the First U.S. Case of COVID-19, Formerly Known as 2019-nCoV. The Spherical Viral Particles, Colorized Blue, Contain Cross-Sections through the Viral Genome, Seen as Black Dots. CDC 2020. Available at: https://phil.cdc.gov/Details.aspx?pid=23354.
Cajigas, S., Alzate, D., Fernández, M., Muskus, C., and Orozco, J. (2022). Electrochemical Genosensor for the Specific Detection of SARS-CoV-2. Talanta 245, 123482. doi:10.1016/j.talanta.2022.123482
Chaibun, T., Puenpa, J., Ngamdee, T., Boonapatcharoen, N., Athamanolap, P., O’Mullane, A. P., et al. (2021). Rapid Electrochemical Detection of Coronavirus SARS-CoV-2. Nat. Commun. 12 (1), 802. doi:10.1038/s41467-021-21121-7
Chen, B., Chen, D., Li, F., Lin, X., and Huang, Q. (2018). Graphitic Porous Carbon: Efficient Synthesis by a Combustion Method and Application as a Highly Selective Biosensor. J. Mat. Chem. B 6 (46), 7684–7691. doi:10.1039/c8tb02139b
Chen, Z., Liao, T., Wan, L., Kuang, Y., Liu, C., Duan, J., et al. (2021). Dual-stimuli Responsive Near-Infrared Emissive Carbon Dots/hollow Mesoporous Silica-Based Integrated Theranostics Platform for Real-Time Visualized Drug Delivery. Nano Res. 14 (11), 4264–4273. doi:10.1007/s12274-021-3624-4
Chertow, D. S. (2018). Next-generation Diagnostics with CRISPR. Science 360 (6387), 381–382. doi:10.1126/science.aat4982
Chowdhury, A. D., Takemura, K., Li, T.-C., Suzuki, T., and Park, E. Y. (2019). Electrical Pulse-Induced Electrochemical Biosensor for Hepatitis E Virus Detection. Nat. Commun. 10 (1), 3737. doi:10.1038/s41467-019-11644-5
Cui, J., Kan, L., Cheng, F., Liu, J., He, L., Xue, Y., et al. (2022). Construction of Bifunctional Electrochemical Biosensors for the Sensitive Detection of the SARS-CoV-2 N-Gene Based on Porphyrin Porous Organic Polymers. Dalton Trans. 51 (5), 2094–2104. doi:10.1039/D1DT03869A
Curti, F., Fortunati, S., Knoll, W., Giannetto, M., Corradini, R., Bertucci, A., et al. (2022). A Folding-Based Electrochemical Aptasensor for the Single-step Detection of the SARS-CoV-2 Spike Protein. ACS Appl. Mat. Interfaces 14 (17), 19204–19211. doi:10.1021/acsami.2c02405
Damiati, S., Sopstad, S., Peacock, M., Akhtar, A. S., Pinto, I., Soares, R. R. G., et al. (2021). Flex Printed Circuit Board Implemented Graphene-Based DNA Sensor for Detection of SARS-CoV-2. IEEE Sensors J. 21 (12), 13060–13067. doi:10.1109/JSEN.2021.3068922
Deng, Y., Peng, Y., Wang, L., Wang, M., Zhou, T., Xiang, L., et al. (2022). Target-triggered Cascade Signal Amplification for Sensitive Electrochemical Detection of SARS-CoV-2 with Clinical Application. Anal. Chim. Acta 1208, 339846. doi:10.1016/j.aca.2022.339846
Ding, X., Yin, K., Li, Z., Lalla, R. V., Ballesteros, E., Sfeir, M. M., et al. (2020). Ultrasensitive and Visual Detection of SARS-CoV-2 Using All-In-One Dual CRISPR-Cas12a Assay. Nat. Commun. 11 (1), 4711. doi:10.1038/s41467-020-18575-6
Fan, Z., Yao, B., Ding, Y., Xu, D., Zhao, J., and Zhang, K. (2022). Rational Engineering the DNA Tetrahedrons of Dual Wavelength Ratiometric Electrochemiluminescence Biosensor for High Efficient Detection of SARS-CoV-2 RdRp Gene by Using Entropy-Driven and Bipedal DNA Walker Amplification Strategy. Chem. Eng. J. 427, 131686. doi:10.1016/j.cej.2021.131686
Fan, Z., Yao, B., Ding, Y., Zhao, J., Xie, M., and Zhang, K. (2021). Entropy-driven Amplified Electrochemiluminescence Biosensor for RdRp Gene of SARS-CoV-2 Detection with Self-Assembled DNA Tetrahedron Scaffolds. Biosens. Bioelectron. 178, 113015. doi:10.1016/j.bios.2021.113015
Farzin, L., Sadjadi, S., Sheini, A., and Mohagheghpour, E. (2021). A Nanoscale Genosensor for Early Detection of COVID-19 by Voltammetric Determination of RNA-dependent RNA Polymerase (RdRP) Sequence of SARS-CoV-2 Virus. Microchim. Acta 188 (4), 121. doi:10.1007/s00604-021-04773-6
Fu, L., Liu, X., Su, Y., Ma, J., and Hong, K. (2021). Prevalence and Impact of Cardiac Injury on COVID ‐19: A Systematic Review and Meta‐analysis. Clin. Cardiol. 44 (2), 276–283. doi:10.1002/clc.23540
Gutiérrez-Gálvez, L., del Caño, R., Menéndez-Luque, I., García-Nieto, D., Rodríguez-Peña, M., Luna, M., et al. (2022). Electrochemiluminescent Nanostructured DNA Biosensor for SARS-CoV-2 Detection. Talanta 240, 123203. doi:10.1016/j.talanta.2021.123203
Han, C., Li, W., Li, Q., Xing, W., Luo, H., Ji, H., et al. (2022). CRISPR/Cas12a-Derived Electrochemical Aptasensor for Ultrasensitive Detection of COVID-19 Nucleocapsid Protein. Biosens. Bioelectron. 200, 113922. doi:10.1016/j.bios.2021.113922
Hatamluyi, B., Rezayi, M., Amel Jamehdar, S., Rizi, K. S., Mojarrad, M., Meshkat, Z., et al. (2022). Sensitive and Specific Clinically Diagnosis of SARS-CoV-2 Employing a Novel Biosensor Based on Boron Nitride Quantum Dots/flower-like Gold Nanostructures Signal Amplification. Biosens. Bioelectron. 207, 114209. doi:10.1016/j.bios.2022.114209
He, C., Lin, X., Mei, Y., Luo, Y., Yang, M., Kuang, Y., et al. (2022). Recent Advances in Carbon Dots for In Vitro/Vivo Fluorescent Bioimaging: A Mini-Review. Front. Chem. 10, 905475. doi:10.3389/fchem.2022.905475
He, S., Huang, Q., Zhang, Y., Zhang, H., Xu, H., Li, X., et al. (2021). Magnetic Beads-Based Multicolor Colorimetric Immunoassay for Ultrasensitive Detection of Aflatoxin B1. Chin. Chem. Lett. 32 (4), 1462–1465. doi:10.1016/j.cclet.2020.09.047
Heo, W., Lee, K., Park, S., Hyun, K.-A., and Jung, H.-I. (2022). Electrochemical Biosensor for Nucleic Acid Amplification-free and Sensitive Detection of Severe Acute Respiratory Syndrome Coronavirus 2 (SARS-CoV-2) RNA via CRISPR/Cas13a Trans-cleavage Reaction. Biosens. Bioelectron. 201, 113960. doi:10.1016/j.bios.2021.113960
Hu, X., Zhu, L., Luo, Y., Zhao, Q., Tan, C., Chen, X., et al. (2020). Evaluation of the Clinical Performance of Single-, Dual-, and Triple-Target SARS-CoV-2 RT-qPCR Methods. Clin. Chim. Acta 511, 143–148. doi:10.1016/j.cca.2020.10.008
Huang, P., Liu, T., Huang, L., Liu, H., Lei, M., Xu, W., et al. (2020a). Use of Chest CT in Combination with Negative RT-PCR Assay for the 2019 Novel Coronavirus but High Clinical Suspicion. Radiology 295 (1), 22–23. doi:10.1148/radiol.2020200330
Huang, Q., Lin, X., Chen, D., and Tong, Q.-X. (2022). Carbon Dots/α-Fe2O3-Fe3O4 Nanocomposite: Efficient Synthesis and Application as a Novel Electrochemical Aptasensor for the Ultrasensitive Determination of Aflatoxin B1. Food Chem. 373, 131415. doi:10.1016/j.foodchem.2021.131415
Huang, Q., Lin, X., Tong, L., and Tong, Q.-X. (2020b). Graphene Quantum Dots/Multiwalled Carbon Nanotubes Composite-Based Electrochemical Sensor for Detecting Dopamine Release from Living Cells. ACS Sustain. Chem. Eng. 8 (3), 1644–1650. doi:10.1021/acssuschemeng.9b06623
Huang, Y., Liu, G. D., and Zhang, X. J. (2020c). Detection and Diagnosis of COVID-19. Prog. Chem. 32 (9), 1241–1251. doi:10.7536/PC200522
Huang, Z., Huang, P., Du, B., Kong, L., Zhang, W., Zhang, Y., et al. (2021). Prevalence and Clinical Outcomes of Cardiac Injury in Patients with COVID-19: A Systematic Review and Meta-Analysis. Nutr. Metabolism Cardiovasc. Dis. 31 (1), 2–13. doi:10.1016/j.numecd.2020.09.004
Idili, A., Parolo, C., Alvarez-Diduk, R., and Merkoçi, A. (2021). Rapid and Efficient Detection of the SARS-CoV-2 Spike Protein Using an Electrochemical Aptamer-Based Sensor. ACS Sens. 6 (8), 3093–3101. doi:10.1021/acssensors.1c01222
Ilkhani, H., and Farhad, S. (2018). A Novel Electrochemical DNA Biosensor for Ebola Virus Detection. Anal. Biochem. 557, 151–155. doi:10.1016/j.ab.2018.06.010
Jalandra, R., Yadav, A. K., Verma, D., Dalal, N., Sharma, M., Singh, R., et al. (2020). Strategies and Perspectives to Develop SARS-CoV-2 Detection Methods and Diagnostics. Biomed. Pharmacother. 129, 110446. doi:10.1016/j.biopha.2020.110446
Jiang, C., Mu, X., Liu, S., Liu, Z., Du, B., Wang, J., et al. (2022). A Study of the Detection of SARS-CoV-2 ORF1ab Gene by the Use of Electrochemiluminescent Biosensor Based on Dual-Probe Hybridization. Sensors 22 (6), 2402. doi:10.3390/s22062402
Jin, X., Lian, J.-S., Hu, J.-H., Gao, J., Zheng, L., Zhang, Y.-M., et al. (2020a). Epidemiological, Clinical and Virological Characteristics of 74 Cases of Coronavirus-Infected Disease 2019 (COVID-19) with Gastrointestinal Symptoms. Gut 69 (6), 1002–1009. doi:10.1136/gutjnl-2020-320926
Jin, Y.-H., Cai, L., Cai, L., Cheng, Z.-S., Cheng, H., Deng, T., et al. (2020b). A Rapid Advice Guideline for the Diagnosis and Treatment of 2019 Novel Coronavirus (2019-nCoV) Infected Pneumonia (Standard Version). Mil. Med. Res. 7 (1), 4. doi:10.1186/s40779-020-0233-6
Kalbusch, A., Henning, E., Brikalski, M. P., Luca, F. V. d., and Konrath, A. C. (2020). Impact of Coronavirus (COVID-19) Spread-Prevention Actions on Urban Water Consumption. Resour. Conservation Recycl. 163, 105098. doi:10.1016/j.resconrec.2020.105098
Karimi-Maleh, H., Alizadeh, M., Orooji, Y., Karimi, F., Baghayeri, M., Rouhi, J., et al. (2021). Guanine-Based DNA Biosensor Amplified with Pt/SWCNTs Nanocomposite as Analytical Tool for Nanomolar Determination of Daunorubicin as an Anticancer Drug: A Docking/Experimental Investigation. Ind. Eng. Chem. Res. 60 (2), 816–823. doi:10.1021/acs.iecr.0c04698
Kashefi-Kheyrabadi, L., Nguyen, H. V., Go, A., Baek, C., Jang, N., Lee, J. M., et al. (2022). Rapid, Multiplexed, and Nucleic Acid Amplification-free Detection of SARS-CoV-2 RNA Using an Electrochemical Biosensor. Biosens. Bioelectron. 195, 113649. doi:10.1016/j.bios.2021.113649
Kim, H. E., Schuck, A., Lee, S. H., Lee, Y., Kang, M., and Kim, Y.-S. (2021). Sensitive Electrochemical Biosensor Combined with Isothermal Amplification for Point-Of-Care COVID-19 Tests. Biosens. Bioelectron. 182, 113168. doi:10.1016/j.bios.2021.113168
Kirtipal, N., Bharadwaj, S., and Kang, S. G. (2020). From SARS to SARS-CoV-2, Insights on Structure, Pathogenicity and Immunity Aspects of Pandemic Human Coronaviruses. Infect. Genet. Evol. 85, 104502. doi:10.1016/j.meegid.2020.104502
Krishna, V. D., Wu, K., Perez, A. M., and Wang, J.-P. (2016). Giant Magnetoresistance-Based Biosensor for Detection of Influenza A Virus. Front. Microbiol. 7, 400. doi:10.3389/fmicb.2016.00400
Kuang, Y., Zhang, J., Xiong, M., Zeng, W., Lin, X., Yi, X., et al. (2020). A Novel Nanosystem Realizing Curcumin Delivery Based on Fe3O4@Carbon Dots Nanocomposite for Alzheimer's Disease Therapy. Front. Bioeng. Biotechnol. 8, 614906. doi:10.3389/fbioe.2020.614906
Kumar, M. S., Nandeshwar, R., Lad, S. B., Megha, K., Mangat, M., Butterworth, A., et al. (2021). Electrochemical Sensing of SARS-CoV-2 Amplicons with PCB Electrodes. Sensors Actuators B Chem. 343, 130169. doi:10.1016/j.snb.2021.130169
Kurnia Sari, A., Yeni Wahyuni Hartati, Y. W., Shabarni Gaffar, S., Isa Anshori, I., Darmawan Hidayat, D., and Hesti Lina Wiraswati, H. L. (2022). The Optimization of an Electrochemical Aptasensor to Detect RBD Protein S SARS-CoV-2 as a Biomarker of COVID-19 Using Screen-Printed Carbon electrode/AuNP. J. Electrochem. Sci. Eng. 12 (1), 219–235. doi:10.5599/jese.1206
Li, Y., Li, S., Wang, J., and Liu, G. (2019). CRISPR/Cas Systems towards Next-Generation Biosensing. Trends Biotechnol. 37 (7), 730–743. doi:10.1016/j.tibtech.2018.12.005
Lin, L., Hu, K., Cai, S., Deng, X., Shao, X., Liang, Y., et al. (2020). Hypoproteinemia Is an Independent Risk Factor for the Prognosis of Severe COVID-19 Patients. J. Clin. Biochem. Nutr. 67 (2), 126–130. doi:10.3164/jcbn.20-75
Lin, X., Mei, Y., He, C., Luo, Y., Yang, M., Kuang, Y., et al. (2021). Electrochemical Biosensing Interface Based on Carbon Dots-Fe3O4 Nanomaterial for the Determination of Escherichia coli O157:H7. Front. Chem. 9 (903), 769648. doi:10.3389/fchem.2021.769648
Liu, L., and Fan, X.-D. (2014). CRISPR-cas System: a Powerful Tool for Genome Engineering. Plant Mol. Biol. 85 (3), 209–218. doi:10.1007/s11103-014-0188-7
Liu, N., Liu, R., and Zhang, J. (2022). CRISPR-Cas12a-mediated Label-free Electrochemical Aptamer-Based Sensor for SARS-CoV-2 Antigen Detection. Bioelectrochemistry 146, 108105. doi:10.1016/j.bioelechem.2022.108105
Liu, Z., Du, Y., Zhang, P., Zhuang, Z., and Wang, D. (2021). Bringing Catalytic Order Out of Chaos with Nitrogen-Doped Ordered Mesoporous Carbon. Matter 4 (10), 3161–3194. doi:10.1016/j.matt.2021.07.019
Lu, R., Zhao, X., Li, J., Niu, P., Yang, B., Wu, H., et al. (2020). Genomic Characterisation and Epidemiology of 2019 Novel Coronavirus: Implications for Virus Origins and Receptor Binding. Lancet 395 (10224), 565–574. doi:10.1016/S0140-6736(20)30251-8
Lu, Y., Zhou, Q., and Xu, L. (2021). Non-Invasive Electrochemical Biosensors for TNF-α Cytokines Detection in Body Fluids. Front. Bioeng. Biotechnol. 9, 701045. doi:10.3389/fbioe.2021.701045
Martínez-Periñán, E., García-Mendiola, T., Enebral-Romero, E., del Caño, R., Vera-Hidalgo, M., Vázquez Sulleiro, M., et al. (2021). A MoS2 Platform and Thionine-Carbon Nanodots for Sensitive and Selective Detection of Pathogens. Biosens. Bioelectron. 189, 113375. doi:10.1016/j.bios.2021.113375
Mei, Y., He, C., Zeng, W., Luo, Y., Liu, C., Yang, M., et al. (2022). Electrochemical Biosensors for Foodborne Pathogens Detection Based on Carbon Nanomaterials: Recent Advances and Challenges. Food Bioprocess Technol. 15 (3), 498–513. doi:10.1007/s11947-022-02759-7
Meng, S., Liu, Y., Wang, L., Ji, X., Chen, Y., Zheng, T., et al. (2021). Graphene-Based Flexible Sensors for Simultaneous Detection of Ascorbic Acid, Dopamine, and Uric Acid. Front. Bioeng. Biotechnol. 9, 726071. doi:10.3389/fbioe.2021.726071
Najjar, D., Rainbow, J., Timilsina, S. S., Jolly, P., de Puig, H., Yafia, M., et al. (2021). Lab-on-a-chip Multiplexed Electrochemical Sensor Enables Simultaneous Detection of SARS-CoV-2 RNA and Host Antibodies. MedRxiv. doi:10.1101/2021.09.01.21262387
Nandi, S., Mondal, A., Roberts, A., and Gandhi, S. (2020). Biosensor Platforms for Rapid HIV Detection. Adv. Clin. Chem. 98, 1–34. doi:10.1016/bs.acc.2020.02.001
Nie, J., Li, Q., Wu, J., Zhao, C., Hao, H., Liu, H., et al. (2020). Establishment and Validation of a Pseudovirus Neutralization Assay for SARS-CoV-2. Emerg. Microbes Infect. 9 (1), 680–686. doi:10.1080/22221751.2020.1743767
Pan, L., Huang, P., Xie, X., Xu, J., Guo, D., and Jiang, Y. (2021). Metabolic Associated Fatty Liver Disease Increases the Severity of COVID-19: A Meta-Analysis. Dig. Liver Dis. 53 (2), 153–157. doi:10.1016/j.dld.2020.09.007
Pang, S.-N., Lin, Y.-L., Yu, K.-J., Chiou, Y.-E., Leung, W.-H., and Weng, W.-H. (2021). An Effective SARS-CoV-2 Electrochemical Biosensor with Modifiable Dual Probes Using a Modified Screen-Printed Carbon Electrode. Micromachines 12 (10), 1171. doi:10.3390/mi12101171
Peng, Y., Pan, Y., Sun, Z., Li, J., Yi, Y., Yang, J., et al. (2021). An Electrochemical Biosensor for Sensitive Analysis of the SARS-CoV-2 RNA. Biosens. Bioelectron. 186, 113309. doi:10.1016/j.bios.2021.113309
Qing, W., Chen, K., Yang, Y., Wang, Y., and Liu, X. (2020). Cu2+-doped Carbon Dots as Fluorescence Probe for Specific Recognition of Cr(VI) and its Antimicrobial Activity. Microchem. J. 152, 104262. doi:10.1016/j.microc.2019.104262
Ramírez-Chavarría, R. G., Castillo-Villanueva, E., Alvarez-Serna, B. E., Carrillo-Reyes, J., Ramírez-Zamora, R. M., Buitrón, G., et al. (2022). Loop-mediated Isothermal Amplification-Based Electrochemical Sensor for Detecting SARS-CoV-2 in Wastewater Samples. J. Environ. Chem. Eng. 10 (3), 107488. doi:10.1016/j.jece.2022.107488
Raza, T., Qu, L., Khokhar, W. A., Andrews, B., Ali, A., and Tian, M. (2021). Progress of Wearable and Flexible Electrochemical Biosensors with the Aid of Conductive Nanomaterials. Front. Bioeng. Biotechnol. 9, 761020. doi:10.3389/fbioe.2021.761020
Savchenko, A., Yin, R. T., Kireev, D., Efimov, I. R., and Molokanova, E. (2021). Graphene-Based Scaffolds: Fundamentals and Applications for Cardiovascular Tissue Engineering. Front. Bioeng. Biotechnol. 9, 797340. doi:10.3389/fbioe.2021.797340
Schultz, A., Knoll, T., Urban, A., Schuck, H., Von Briesen, H., Germann, A., et al. (2021). Novel Cost-Efficient Graphene-Based Impedance Biosensor for the Analysis of Viral Cytopathogenicity and the Effect of Antiviral Drugs. Front. Bioeng. Biotechnol. 9, 718889. doi:10.3389/fbioe.2021.718889
Song, Z., Ma, Y., Chen, M., Ambrosi, A., Ding, C., and Luo, X. (2021). Electrochemical Biosensor with Enhanced Antifouling Capability for COVID-19 Nucleic Acid Detection in Complex Biological Media. Anal. Chem. 93 (14), 5963–5971. doi:10.1021/acs.analchem.1c00724
Tian, J., Liang, Z., Hu, O., He, Q., Sun, D., and Chen, Z. (2021). An Electrochemical Dual-Aptamer Biosensor Based on Metal-Organic Frameworks MIL-53 Decorated with Au@Pt Nanoparticles and Enzymes for Detection of COVID-19 Nucleocapsid Protein. Electrochimica Acta 387, 138553. doi:10.1016/j.electacta.2021.138553
Udugama, B., Kadhiresan, P., Kozlowski, H. N., Malekjahani, A., Osborne, M., Li, V. Y. C., et al. (2020). Diagnosing COVID-19: The Disease and Tools for Detection. ACS Nano 14 (4), 3822–3835. doi:10.1021/acsnano.0c02624
Wen, L., Wen, C., Zhang, F., Wang, K., Yuan, H., and Hu, F. (2020). siRNA and Chemotherapeutic Molecules Entrapped into a Redox-Responsive Platform for Targeted Synergistic Combination Therapy of Glioma. Nanomedicine Nanotechnol. Biol. Med. 28, 102218. doi:10.1016/j.nano.2020.102218
Wiersinga, W. J., Rhodes, A., Cheng, A. C., Peacock, S. J., and Prescott, H. C. (2020). Pathophysiology, Transmission, Diagnosis, and Treatment of Coronavirus Disease 2019 (COVID-19). JAMA 324 (8), 782–793. doi:10.1001/jama.2020.12839
Wu, F., Zhao, S., Yu, B., Chen, Y.-M., Wang, W., Song, Z.-G., et al. (2020). A New Coronavirus Associated with Human Respiratory Disease in China. Nature 579 (7798), 265–269. doi:10.1038/s41586-020-2008-3
Wu, J., Wang, Q., Dong, X., Xu, M., Yang, J., Yi, X., et al. (2021). Biocompatible AIEgen/p-Glycoprotein siRNA@reduction-Sensitive Paclitaxel Polymeric Prodrug Nanoparticles for Overcoming Chemotherapy Resistance in Ovarian Cancer. Theranostics 11 (8), 3710–3724. doi:10.7150/thno.53828
Xie, X., Zhong, Z., Zhao, W., Zheng, C., Wang, F., and Liu, J. (2020). Chest CT for Typical Coronavirus Disease 2019 (COVID-19) Pneumonia: Relationship to Negative RT-PCR Testing. Radiology 296 (2), E41–E45. doi:10.1148/radiol.2020200343
Xu, L., Li, D., Ramadan, S., Li, Y., and Klein, N. (2020). Facile Biosensors for Rapid Detection of COVID-19. Biosens. Bioelectron. 170, 112673. doi:10.1016/j.bios.2020.112673
Xu, Z., He, H., Zhang, S., Wang, B., Jin, J., Li, C., et al. (2019). Mechanistic Studies on the Antibacterial Behavior of Ag Nanoparticles Decorated with Carbon Dots Having Different Oxidation Degrees. Environ. Sci. Nano 6 (4), 1168–1179. doi:10.1039/C8EN01090K
Xu, Z., and Liu, Y. (2021). The Behavior of Carbonized Polymer Dots at the Nano‐Bio Interface and Their Luminescent Mechanism: A Physical Chemistry Perspective. Chin. J. Chem. 39 (2), 265–273. doi:10.1002/cjoc.202000334
Yan, C., Cui, J., Huang, L., Du, B., Chen, L., Xue, G., et al. (2020). Rapid and Visual Detection of 2019 Novel Coronavirus (SARS-CoV-2) by a Reverse Transcription Loop-Mediated Isothermal Amplification Assay. Clin. Microbiol. Infect. 26 (6), 773–779. doi:10.1016/j.cmi.2020.04.001
Yang, P., and Wang, X. (2020). COVID-19: a New Challenge for Human Beings. Cell Mol. Immunol. 17 (5), 555–557. doi:10.1038/s41423-020-0407-x
Yao, B., Zhang, J., Fan, Z., Ding, Y., Zhou, B., Yang, R., et al. (2021). Rational Engineering of the DNA Walker Amplification Strategy by Using a Au@Ti3C2@PEI-Ru(dcbpy)32+ Nanocomposite Biosensor for Detection of the SARS-CoV-2 RdRp Gene. ACS Appl. Mat. Interfaces 13 (17), 19816–19824. doi:10.1021/acsami.1c04453
Yi, X., Zeng, W., Wang, C., Chen, Y., Zheng, L., Zhu, X., et al. (2022). A Step-by-step Multiple Stimuli-Responsive Metal-Phenolic Network Prodrug Nanoparticles for Chemotherapy. Nano Res. 15 (2), 1205–1212. doi:10.1007/s12274-021-3626-2
Yip, T. L., Huang, Y., and Liang, C. (2021). Built Environment and the Metropolitan Pandemic: Analysis of the COVID-19 Spread in Hong Kong. Build. Environ. 188, 107471. doi:10.1016/j.buildenv.2020.107471
Yuan, J., Kou, S., Liang, Y., Zeng, J., Pan, Y., and Liu, L. (2020). Polymerase Chain Reaction Assays Reverted to Positive in 25 Discharged Patients with COVID-19. Clin. Infect. Dis. 71 (16), 2230–2232. doi:10.1093/cid/ciaa398
Zhang, K., Fan, Z., Ding, Y., and Xie, M. (2022a). A pH-Engineering Regenerative DNA Tetrahedron ECL Biosensor for the Assay of SARS-CoV-2 RdRp Gene Based on CRISPR/Cas12a Trans-activity. Chem. Eng. J. 429, 132472. doi:10.1016/j.cej.2021.132472
Zhang, K., Fan, Z., Ding, Y., Zhu, S., Xie, M., and Hao, N. (2022b). Exploring the Entropy-Driven Amplification Reaction and Trans-cleavage Activity of CRISPR-Cas12a for the Development of an Electrochemiluminescence Biosensor for the Detection of the SARS-CoV-2 RdRp Gene in Real Samples and Environmental Surveillance. Environ. Sci. Nano 9 (1), 162–172. doi:10.1039/D1EN00645B
Zhang, K., Fan, Z., Huang, Y., Ding, Y., and Xie, M. (2022c). A Strategy Combining 3D-DNA Walker and CRISPR-Cas12a Trans-cleavage Activity Applied to MXene Based Electrochemiluminescent Sensor for SARS-CoV-2 RdRp Gene Detection. Talanta 236, 122868. doi:10.1016/j.talanta.2021.122868
Zhang, K., Fan, Z., Huang, Y., Ding, Y., Xie, M., and Wang, M. (2022d). Hybridization Chain Reaction Circuit-Based Electrochemiluminescent Biosensor for SARS-Cov-2 RdRp Gene Assay. Talanta 240, 123207. doi:10.1016/j.talanta.2022.123207
Zhao, H., Liu, F., Xie, W., Zhou, T.-C., Ouyang, J., Jin, L., et al. (2021). Ultrasensitive Supersandwich-type Electrochemical Sensor for SARS-CoV-2 from the Infected COVID-19 Patients Using a Smartphone. Sensors Actuators B Chem. 327, 128899. doi:10.1016/j.snb.2020.128899
Zhou, P., Yang, X.-L., Wang, X.-G., Hu, B., Zhang, L., Zhang, W., et al. (2020). A Pneumonia Outbreak Associated with a New Coronavirus of Probable Bat Origin. Nature 579 (7798), 270–273. doi:10.1038/s41586-020-2012-7
Zhu, X., Wang, X., Han, L., Chen, T., Wang, L., Li, H., et al. (2020). Multiplex Reverse Transcription Loop-Mediated Isothermal Amplification Combined with Nanoparticle-Based Lateral Flow Biosensor for the Diagnosis of COVID-19. Biosens. Bioelectron. 166, 112437. doi:10.1016/j.bios.2020.112437
Zhuang, Z., Li, Y., Huang, J., Li, Z., Zhao, K., Zhao, Y., et al. (2019). Sisyphus Effects in Hydrogen Electrochemistry on Metal Silicides Enabled by Silicene Subunit Edge. Sci. Bull. 64 (9), 617–624. doi:10.1016/j.scib.2019.04.005
Zhuang, Z., Li, Y., Yu, R., Xia, L., Yang, J., Lang, Z., et al. (2022). Reversely Trapping Atoms from a Perovskite Surface for High-Performance and Durable Fuel Cell Cathodes. Nat. Catal. 5 (4), 300–310. doi:10.1038/s41929-022-00764-9
Keywords: COVID-19, SARS-CoV-2, electrochemical DNA biosensors, nucleic acid detection, virus/protein detection
Citation: Mei Y, Lin X, He C, Zeng W, Luo Y, Liu C, Liu Z, Yang M, Kuang Y and Huang Q (2022) Recent Progresses in Electrochemical DNA Biosensors for SARS-CoV-2 Detection. Front. Bioeng. Biotechnol. 10:952510. doi: 10.3389/fbioe.2022.952510
Received: 25 May 2022; Accepted: 10 June 2022;
Published: 15 July 2022.
Edited by:
Youbin Zheng, Technion Israel Institute of Technology, IsraelReviewed by:
Jia-Ming Liu, Minnan Normal University, ChinaBoya Liu, Boston Children’s Hospital and Harvard Medical School, United States
Copyright © 2022 Mei, Lin, He, Zeng, Luo, Liu, Liu, Yang, Kuang and Huang. This is an open-access article distributed under the terms of the Creative Commons Attribution License (CC BY). The use, distribution or reproduction in other forums is permitted, provided the original author(s) and the copyright owner(s) are credited and that the original publication in this journal is cited, in accordance with accepted academic practice. No use, distribution or reproduction is permitted which does not comply with these terms.
*Correspondence: Xiaofeng Lin, Linxf@gmu.edu.cn; Qitong Huang, hqtblue@163.com