- Department of Respiratory, Shenyang Tenth People’s Hospital, Shenyang Chest Hospital, Shenyang, China
Tuberculosis is a severe infectious disease caused by Mycobacterium tuberculosis and is a significant public health concern globally. The World Health Organization (WHO) recommends a combination regimen of several drugs, such as rifampicin (RIF), isoniazid (INH), pyrazinamide (PZA), and ethambutol (ETB), to treat tuberculosis. However, these drugs have low plasma concentrations after oral administration and require multiple high doses, which may lead to the occurrence and development of drug-resistant tuberculosis. Micro/Nanotechnology drug delivery systems have considerable potential in treating drug-resistant tuberculosis, allowing the sustained release of the drug and delivery of the drug to a specific target. These system properties could improve drug bioavailability, reduce the dose and frequency of administration, and solve the problem of non-adherence to the prescribed therapy. This study systematically reviewed the recent advances in PLGA micro/nanoparticle delivery systems as a novel therapeutic approach for drug-resistant tuberculosis.
Introduction
Human tuberculosis (TB) is one of the top 10 causes of death globally and the leading cause of death from a single infectious disease (Reid et al., 2019), which has become a global public health emergency (Figure 1). World Health Organization (WHO) estimates that approximately 9.9 million cases have contracted tuberculosis worldwide in 2020 (World Health Organization, 2021a), and there were 1.2 million deaths from tuberculosis in 2019 (World Health Organization, 2020). Pulmonary tuberculosis is the typical manifestation of tuberculosis, accounting for approximately 80% of tuberculosis cases (World Health Organization, 2020). WHO recommends a 2-month intensive regimen followed by an additional four to 6 months continuation regimen for tuberculosis treatment (Table 1). During the intensive treatment phase, patients are administered four anti-TB drugs daily, including rifampicin (RIF), isoniazid (INH), pyrazinamide (PZA), and ethambutol (ETB), while the continuation treatment phase requires daily RIF and INH (World Health Organization, 2010). Usually, poor patient adherence occurs under this administration, resulting in drug-resistant tuberculosis (DR-TB), including multidrug-resistant tuberculosis (MDR-TB) or extensively drug-resistant tuberculosis (XDR-TB) (World Health Organization, 2021b). Drug-resistant tuberculosis has become a significant public health concern in many countries (Figure 2). In recent years, the number of patients with drug-resistant tuberculosis has steadily increased globally (Lange et al., 2019). According to the WHO Tuberculosis Report 2019, there were nearly 5,00,000 new cases of rifampicin-resistant tuberculosis in the world in 2018, of which 78% were MDR-TB (World Health Organization, 2019a). Effectively controlling the epidemic of drug-resistant tuberculosis is still one of the significant challenges in the public health field of the world.
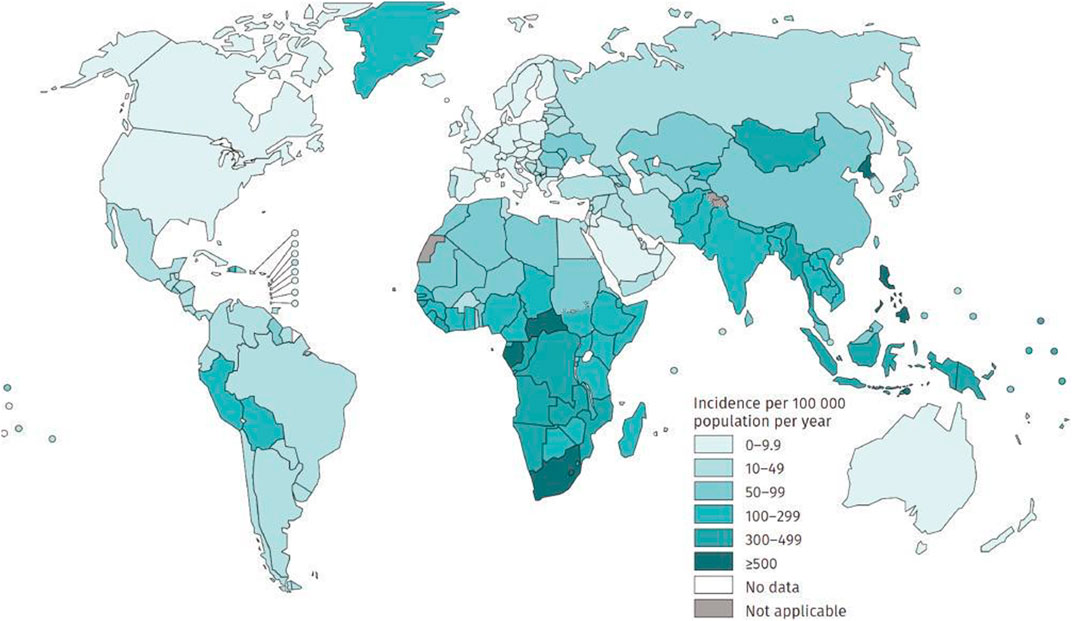
FIGURE 1. Estimated tuberculosis incidence rates, 2020 (excerpted from Global Tuberculosis Report, 2021; World Health Organization, Geneva).
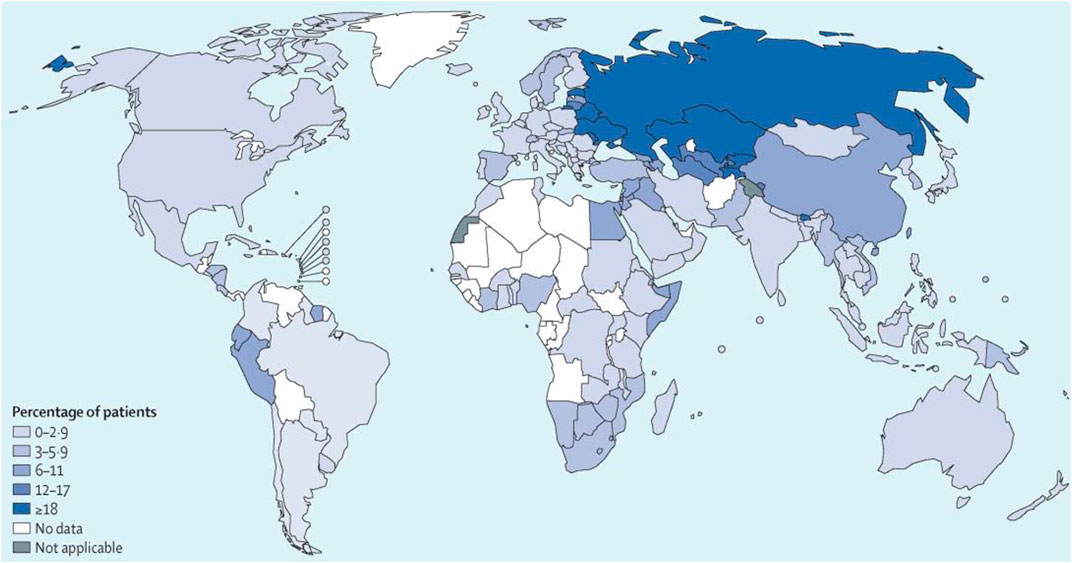
FIGURE 2. Percentages of patients with multidrug-resistant tuberculosis globally. Reproduced with permission from Lange et al. (2019). Copyright © 2019 Elsevier Ltd.
Various treatment approaches have been developed to combat this global emergence of drug-resistant tuberculosis (Patil et al., 2018). Recently, drug delivery methods which can deliver anti-tuberculosis drugs to specific sites in a controlled manner have received much attention. To make the anti-tuberculosis drug continuously released at the lesion site, the sustained-release system uses degradable polymers as the carrier comes into being, where the drug can be released for an extended period via a membrane or matrix-controlled diffusion (Kumar and Aeila, 2019; Jana et al., 2021). Such systems can reduce dose and frequency of administration to enhance patient compliance with treatment and control the distribution of drugs in tissues and the clearance rate in the blood to minimize the risk of toxicity and other side effects. The development of these advanced drug delivery systems provides an excellent alternative to addressing treatment failure due to patient non-adherence. Several micro/nanoparticles sized sustained-release systems have been designed for drug encapsulation to treat tuberculosis (Table 2).
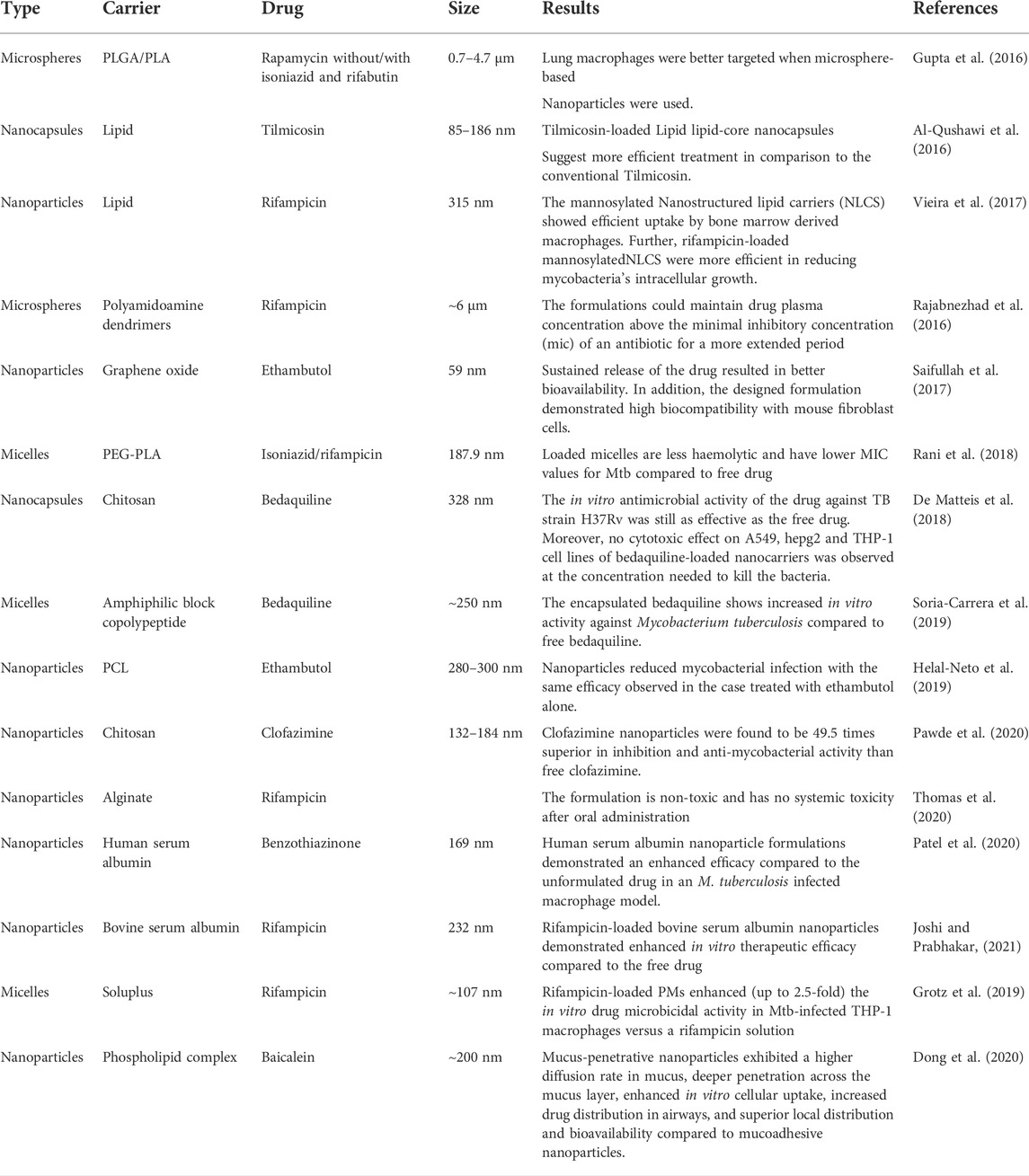
TABLE 2. Several micro/nanoparticles sized sustained-release systems for drug encapsulation to treat tuberculosis.
Poly (lactic-co-glycolic acid) and poly (lactic-co-glycolic acid) micro/nanoparticles
Poly (lactic-co-glycolic acid) (PLGA) is one of the most successfully developed biodegradable polymers with a wide range of degradation times that can be tuned by its molecular weight and copolymer ratio. PLGA is soluble in common solvents and can be processed into virtually any shape and size. Therefore, PLGA polymers have been primarily tested as delivery vehicles for drugs, proteins, and other macromolecules such as DNA, RNA, and peptides. In addition, the polymers chemical composition and molecular weight, and the physical properties of PLGA nanocarriers, such as size, shape, surface area to volume ratio, etc., can be “tuned” to obtain the desired release profile. With excellent biocompatibility, tunable degradation and release properties and high versatility have been approved for a variety of biomedical applications, PLGA is approved by Food and Drug Administration for human use and has been widely used in sustained-release drug delivery systems (Figure 3) and tissue engineering (Gentile et al., 2014; Mironov et al., 2017; Ding and Zhu, 2018; Lam et al., 2018; Lai et al., 2019; Park et al., 2019; Ghitman et al., 2020; Lagreca et al., 2020; Koerner et al., 2021). PLGA particles are the most widely applied type of particles; the extensive use of PLGA micro/nanoparticle-based drug delivery systems is promising due to their higher efficiency and fewer adverse effects (Chereddy et al., 2016). Table 3 shows the current clinical trials/status of PLGA-based micro/nanoparticles therapy and diagnostics.
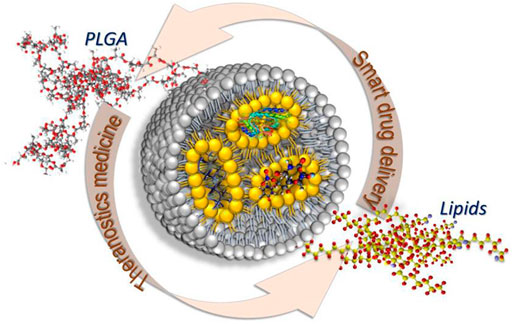
FIGURE 3. PLGA-lipid hybrid nanocarriers. Reproduced with permission from Ghitman et al. (2020). Copyright © 2020 the Authors.
Poly (lactic-co-glycolic acid) micro/nanoparticles as novel therapeutic approaches for drug-resistant tuberculosis
In recent years, great efforts have been devoted to the development of PLGA Micro/nanoparticle delivery systems for treating drug-resistant tuberculosis, and the current research achievements have been listed in Table 4.
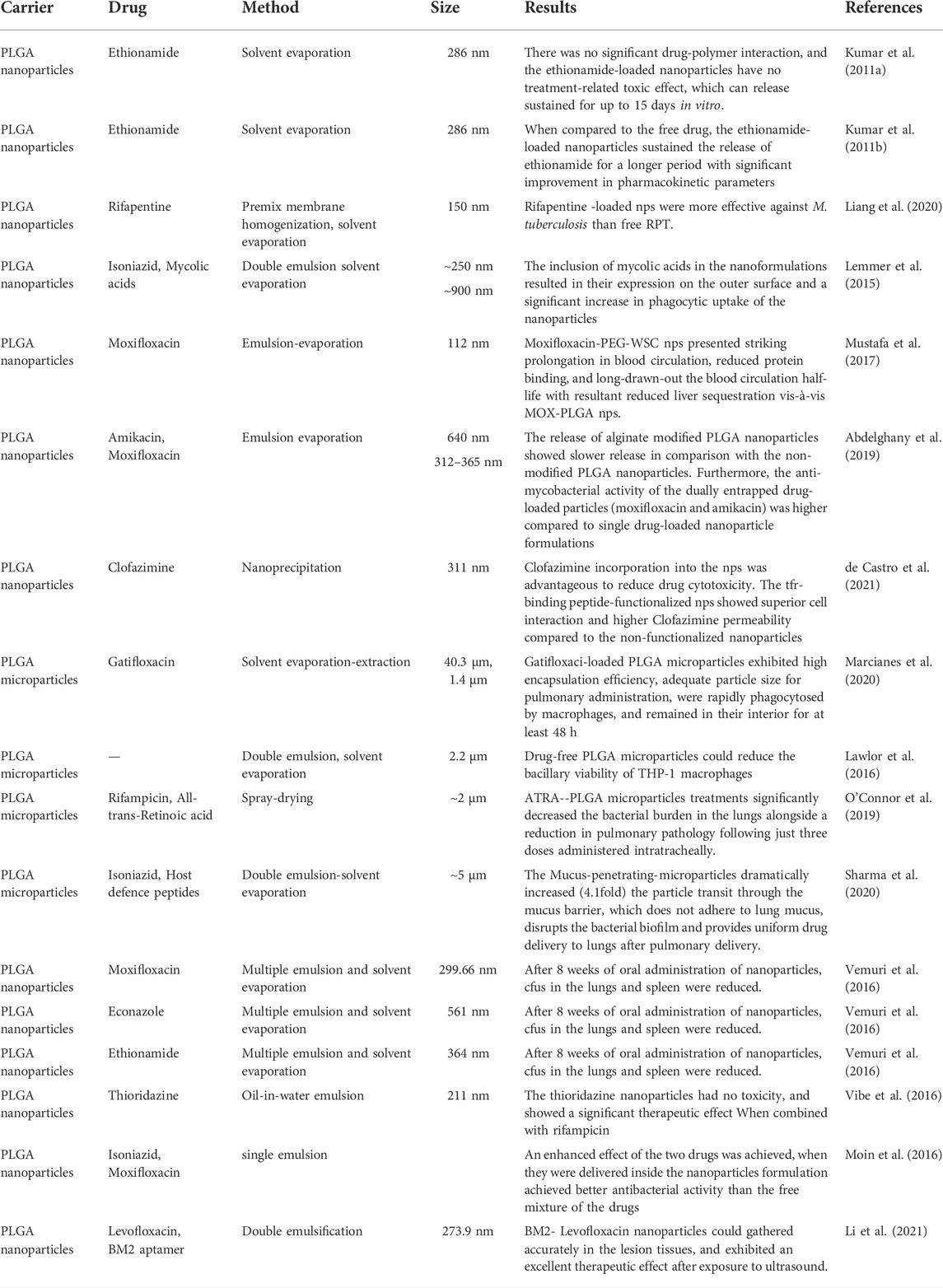
TABLE 4. Current research achievements of PLGA Micro/nanoparticle delivery systems for treating drug-resistant tuberculosis
Kumar et al. (2011a) developed ethionamide-loaded PLGA nanoparticles by solvent evaporation method to achieve prolonged drug release for the treatment of MDR-TB and improve patient compliance. In vitro release studies showed that ethionamide was sustainedly released for 15 days in various media. In vivo results showed that the ethionamide-loaded PLGA nanoparticles did not show any statistically significant treatment-related effects on weight gain and clinical signs. Likewise, no treatment-related toxic effects were found in haematology, clinical chemistry, and histopathology. The results demonstrate that orally safe ethionamide-loaded PLGA nanoparticles with sustained-release properties offer excellent potential for further preclinical and clinical studies. The authors also investigated ethionamide’s pharmacokinetics and tissue distribution in mice (Kumar et al., 2011b). The sustained release of ethionamide in plasma for 6 days was detected for ethionamide-loaded PLGA nanoparticles compared to 6 h for free ethionamide. Furthermore, ethionamide was detected in organs (lungs, liver and spleen) for up to 5–7 days while maintaining drug levels above the MIC for 5 days, whereas free ethionamide was cleared within 12 h in vivo (Kumar et al., 2011b). Ethionamide-loaded PLGA nanoparticles showed significant improvement in pharmacokinetic parameters. Hence, the ethionamide-based PLGA nanoparticles have great potential for reducing the dosing frequency of ethionamide in treating MDR-TB. PLGA-based nanoparticles could also act as a sustained-release delivery vehicle for rifapentine to prolong drug release, alter pharmacokinetics, increase anti-tuberculosis activity, and reduce toxicity, allowing for low dose and frequency (Liang et al., 2020). Future studies on toxicity studies of drug-loaded nanoparticles and the chemotherapeutic potential of ethionamide-loaded nanoparticles against Mycobacterium tuberculosis (Mtb) in clinics should be performed.
It is well known that tuberculosis is a chronic infectious disease caused by Mtb (Khawbung et al., 2021). Resistant strains of Mtb cause a significant proportion of drug-resistant tuberculosis cases. There is an urgent need to develop new and innovative approaches for treatment. Lemmer et al. (2015) studied mycolic acids as a promising mycobacterial ligand for drug targeting using isoniazid PLGA nanoparticles. The results showed that the phagocytic uptake of mycobacterial acid-coated nanoparticles by mycobacterial-infected macrophages was significantly increased. Therefore, mycolic acid can be further explored as a potential target ligand for various nanoformulations to treat tuberculosis effectively.
Moxifloxacin (MOX) is an Mtb DNA gyrase inhibitor. Due to its strong hydrophilicity, MOX is cleared from the body within 24 h and requires repeated administration, leading to hepatotoxicity and acquisition of MOX-resistant tuberculosis associated with use. To overcome the limitations above, Mustafa et al. (2017) developed PLGA nanoparticles to act as an efficient carrier for the controlled delivery of MOX. The authors performed the affixation of polyethylene glycol (PEG) to MOX-PLGA nanoparticles and adsorption of water-soluble chitosan (WSC) to the particle surface to achieve a substantial extension in blood circulation. They investigated the in vivo pharmacokinetics and in vivo biodistribution after oral administration of the resulting surface-modified nanoparticles (MOX-PEG-WSC NPs), finding that the NPs surface charge was close to neutral +4.76 mV and was significantly affected by the WSC coating. MOX-PEG-WSC NPs significantly prolong blood circulation, reduce protein binding, and prolong blood circulation half-life compared with MOX-PLGA NPs. Therefore, these studies demonstrate that the MOX-PEG-WSC NPs exhibit sustained-release behaviour for controlled drug delivery and prolong the circulation time in the bloodstream for extended periods, thereby minimizing the frequency of dosing and avoiding the occurrence of MOX-resistant tuberculosis. Abdelghany et al. (2019) have entrapped amikacin and moxifloxacin into alginate modified-PLGA nanoparticles using two water-oil-water (w/o/w) emulsion strategies (Figure 4), targeting the treatment of MDR. The authors assessed the anti-mycobacterial activity of the resulting PLGA nanoparticles using Mtb-infected macrophages. The dually entrapped nanoparticles showed bacterial viability of 0.6% relative to the untreated group, compared to 6.49% for amikacin alone nanoparticles and 3.27% for moxifloxacin alone nanoparticles, revealing an enhanced inhibition of viable bacterial count due to the synergistic effect of moxifloxacin and amikacin in the PLGA nanoparticles (Abdelghany et al., 2019). The amikacin-moxifloxacin alginate entrapped PLGA nanoparticles have the potential to reduce the dose of these drugs, thereby improving patient compliance with treatment and potentially reducing adverse dose-related side effects. However, further in vivo studies are urgently required to confirm this prospect.
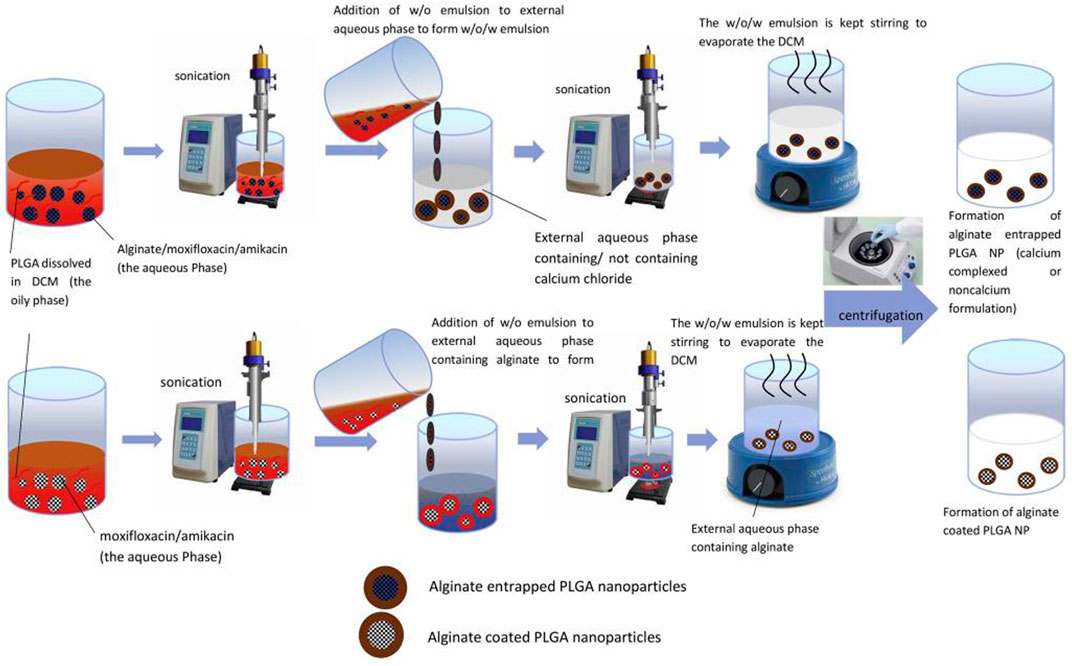
FIGURE 4. Schematic presentation of (A) alginate entrapped PLGA nanoparticles and (B) alginate coated PLGA nanoparticles. Reproduced with permission from Abdelghany et al. (2019). Copyright © 2019 Elsevier B. V.
Clofazimine (CFZ) exhibits high activity against multidrug-resistant strains of Mtb in vitro (Nugraha et al., 2021; Mashele et al., 2022). However, its poor water solubility and high lipophilicity cause low and erratic drug bioavailability, high plasma protein binding and fatty tissue accumulation, limiting the therapeutic efficacy after oral administration (World Health Organization, 2019b). To solve this problem, de Castro et al. (2021) developed PLGA-PEG nanoparticles loaded with CFZ and functionalized with a transferrin receptor-binding peptide (Figure 5) to develop brain drug delivery to treat the central nervous system tuberculosis. In vitro studies in brain endothelial hCMEC/D3 cells showed that incorporating CFZ into nanoparticles significantly reduced drug cytotoxicity. TfR-binding peptide-functionalized nanoparticles exhibited better cellular interactions and higher CFZ permeability in hCMEC/D3 cell monolayers than non-functionalized NP controls. The functionalized PLGA-PEG nanoparticles demonstrate suitability for CFZ biological administration, suggested with low plasma protein binding, off-target biodistribution and precise delivery of CFZ towards the brain parenchyma.
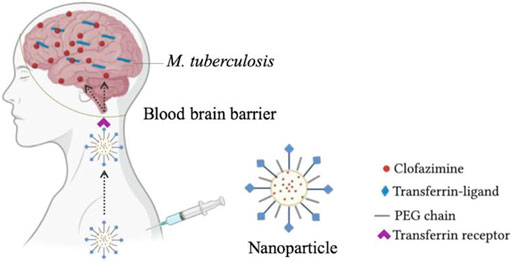
FIGURE 5. PLGA-PEG nanoparticles loaded with CFZ and functionalized with a transferrin receptor-binding peptide for brain drug delivery. Reproduced with permission from de Castro et al. (2021) Copyright © 2021 Elsevier B. V.
Mtb can survive and replicate in alveolar macrophages (Ufimtseva et al., 2019), evading host defence mechanisms and developing the latent disease. Considering that phagocytes can localize and internalize foreign substances, such as polymeric particles, this fact could guide particles to the interior of macrophages, leading to an exciting approach to the treatment of intracellular infections affecting the mononuclear phagocytic system. Marcianes et al. (2020) developed a new biodegradable PLGA microparticle for pulmonary administration of gatifloxacin, using labrafil as a surface modifier to actively target alveolar macrophages, thereby allowing gain access of the drug to Mtb. Cell phagocytosis was studied in raw 264.7 mouse macrophage cell lines after incubating 3, 5, 24, and 48 h with the microparticles. The results showed that labrafil enhanced the uptake rate of PLGA 502H microparticles by macrophages (Figure 6). Gatifloxacin-loaded PLGA microparticles using PLGA 502H and labrafil exhibited high encapsulation efficiency (89.6% ± 0.2%), rapid phagocytosis by macrophages (3 h), and remained inside the cells for at least 48 h, resulting in a suitable carrier to potentially treat MDR-TB. These results are promising, but regarding future perspectives, further immunogenicity studies of the developed systems and phagocytosis in Mtb-infected macrophages should be conducted, and the safety of the formulations should be evaluated in an animal model of tuberculosis.
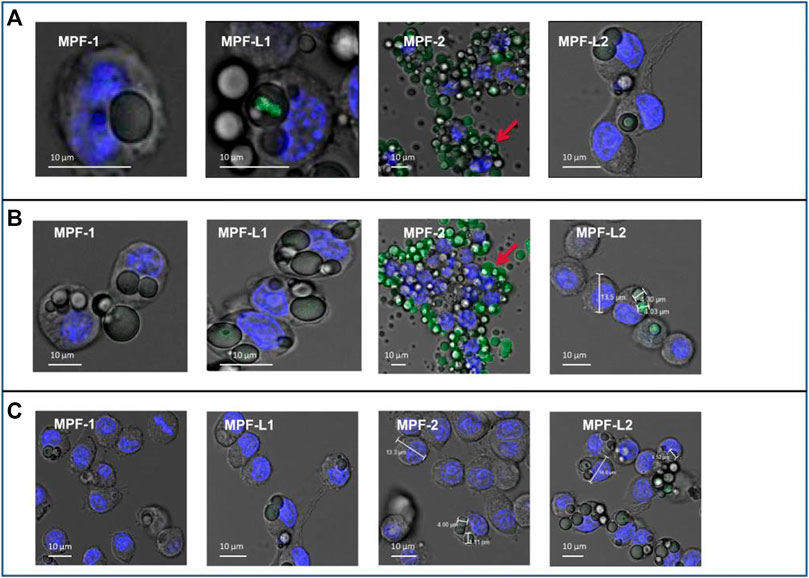
FIGURE 6. Confocal images of phagocytosis obtained at 3 h (A), 5 h (B), and 24 h (C). MPF-1, fluorescein-loaded PLGA 502 MPs; MPF-2, fluorescein-loaded PLGA 502H MPs; MPF-L1, labrafil-modified fluorescein-loaded PLGA 502 MPs; MPF-L2, labrafil-modified fluorescein-loaded PLGA 502H MPs. Reproduced with permission from Marcianes et al. (2020). Copyright © 2022 Springer Nature Switzerland AG.
Pulmonary drug delivery systems are gaining popularity because of their ability to achieve high drug concentrations at the site of infection and minimize systemic toxicity for the treatment of various lung diseases, including tuberculosis. Lawlor et al. (2016) designed PLGA microparticles (MPs) carrying anti-tuberculosis drugs that human alveolar macrophages could successfully take up. They demonstrated how MPs affect macrophage function in Mtb infection by treating Mtb H37Ra or H37Rv-infected THP-1 macrophages with MPs. It was found that the activity of NF kappa B was increased in MPs-treated macrophages, although cytokine secretion was unaltered, and the induction of autophagy was demonstrated via the Confocal microscopy of immortalized murine bone marrow-derived macrophages expressing GFP-tagged LC3 (Figure 7). Inhibition of caspases did not influence the MP-induced restriction of bacillary growth, however, blockade of NF kappa B or autophagy with pharmacological inhibitors reversed this MP effect on macrophage function. These data support using the inhaled PLGA MP drug delivery system as a vehicle for immunotherapeutic agents and targeted drug delivery, exploiting the generation of inhaled vaccines and inhaled MDR-TB therapeutics.
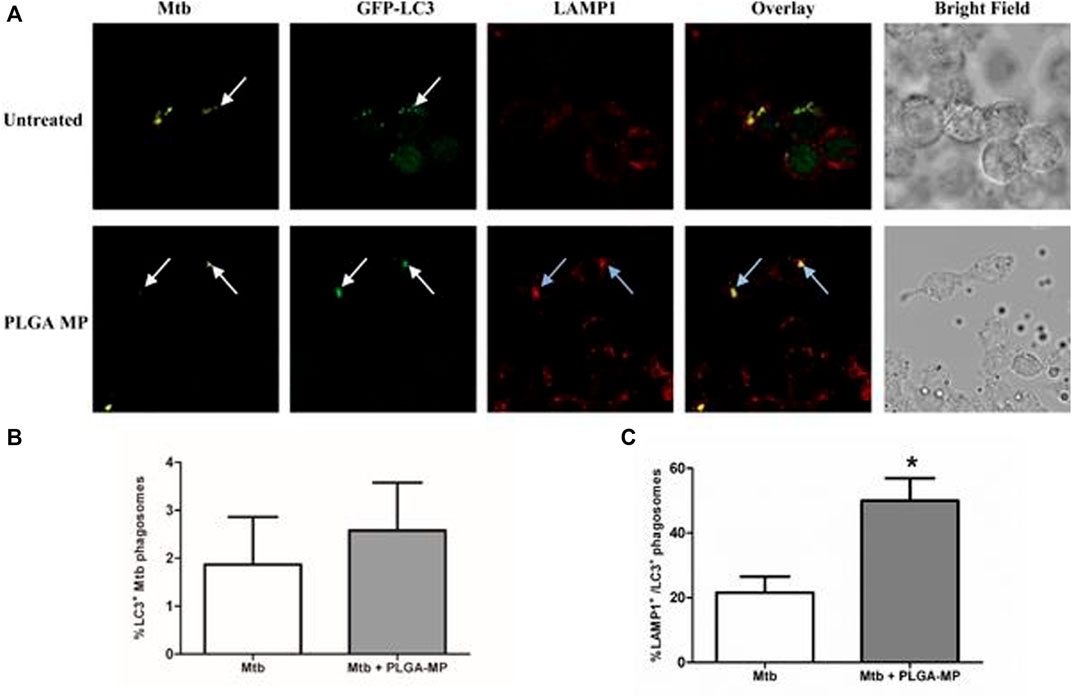
FIGURE 7. PLGA MPs trigger autophagic flux in Mtb-infected macrophages. (A) After a total of 24 h, infected macrophages were fixed and stained with anti-LAMP1_and anti-Mtb antibodies, and then observed by laser scanning confocal microscopy. The proportion of (B) LC3 positive Mtb phagosomes and (C) LC3 positive phagosomes which were also positive for LAMP1 were counted. Data represent the mean ± SEM of three independent experiments in which more than 100 phagosomes were counted for each condition. *p < 0.05. The white arrows indicate localization of Mtb phagosomes with GFP-LC3, blue arrows indicate co-localization of Mtb, GFP-LC3, and LAMP1. The results shown are the means of three independent experiments. Reproduced with permission from Lawlor et al. (2016). Copyright © 2016 the authors.
O’Connor et al. (2019) prepared inhalable PLGA microparticles loaded with trans-Retinoic acid (ATRA) to establish the effect of targeted ATRA treatment on Mtb viability. The results showed that 70.5% ± 2.3% of encapsulated ATRA was targeted and delivered to the site of action within the alveolar macrophage, indicating the efficient cellular delivery of ATRA. Furthermore, the results of the BACT/ALERT® system and enumeration of colony-forming units showed a reduction in Mtb (H37Ra) growth in THP-1 derived macrophages, confirming the good antibacterial effect of ATRA-loaded PLGA microparticles. The ATRA-loaded PLGA microparticles could also significantly decrease the bacterial burden in the lungs alongside a reduction in pulmonary pathology, as shown in the in vivo antibacterial assessment results in Mtb (H37Rv) strain infected BALB/c mice (Figure 8). This study is the first to treat tuberculosis with controlled release of ATRA via the pulmonary route in vivo, providing an alternative to traditional oral supplements that have been ineffective in clinical studies. It provides a new and tried strategy for treating drug-resistant tuberculosis and has broad clinical application prospects.
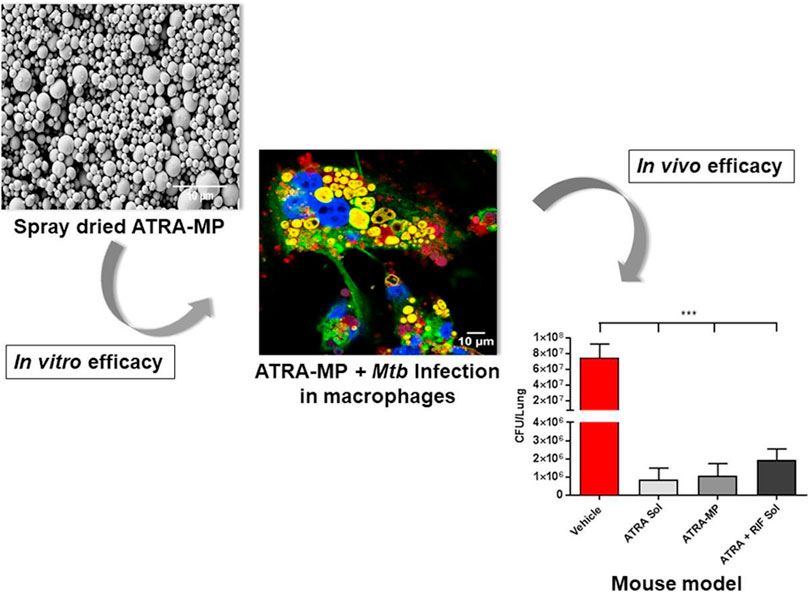
FIGURE 8. In vitro and in vivo efficacy of the inhalable PLGA microparticles loaded with trans-Retinoic acid (ATRA). Reproduced with permission from O’Connor et al. (2019). Copyright © 2018 Elsevier B. V.
In tuberculosis treatment, antibiotics become less effective, and bacteria develop resistance over time due to the formation of some barriers around microorganisms, such as lung mucus and biofilms. Traditional respirable microparticles are mainly trapped in dense, chaotic networks of mucins and are rapidly cleared by mucociliary clearance. Therefore, if the anti-tuberculosis activity of drug-loaded inhalable polymer particles can synergize with the mucus-penetrating and biofilm-disrupting properties, it would be a significant advantage of anti-tuberculosis microparticles, helping to improve the therapeutic effect. Sharma et al. encapsulated IDR-1018 peptide with an anti-tuberculosis drug in N-acetyl cysteine (NAC) decorated porous PLGA microspheres (Sharma et al., 2020), developing Mucus-penetrating-microparticles (NAC/PLGA-MPP). The multiple tracking techniques showed that NAC coating on the porous PLGA microspheres significantly increased (4.1-fold) the passage of particles through the mucus barrier. The designed inhalable NAC/PLGA-MPP does not adhere to lung mucus, does not disrupt bacterial biofilms, and provides uniform drug delivery to the lungs after pulmonary delivery. The activity of the NAC/PLGA-MPP against Mtb in macrophage cultures and in mice model infected with a low-dose bacterial aerosol was evaluated. After 6 weeks of administration of the daily dose, the inhalation of NAC/PLGA-MPP encapsulated with IDR-1018 significantly reduced (p < 0.05) bacterial load and inflammation in the lungs in a mouse model of tuberculosis. The histopathological results also validate the compelling chemotherapeutic outcome of inhaled formulations (Figure 9). This data supports the potential of using mucus-penetrating inhalable drug delivery systems as a strategy for targeted pulmonary delivery, which may benefit drug-resistant tuberculosis treatment.
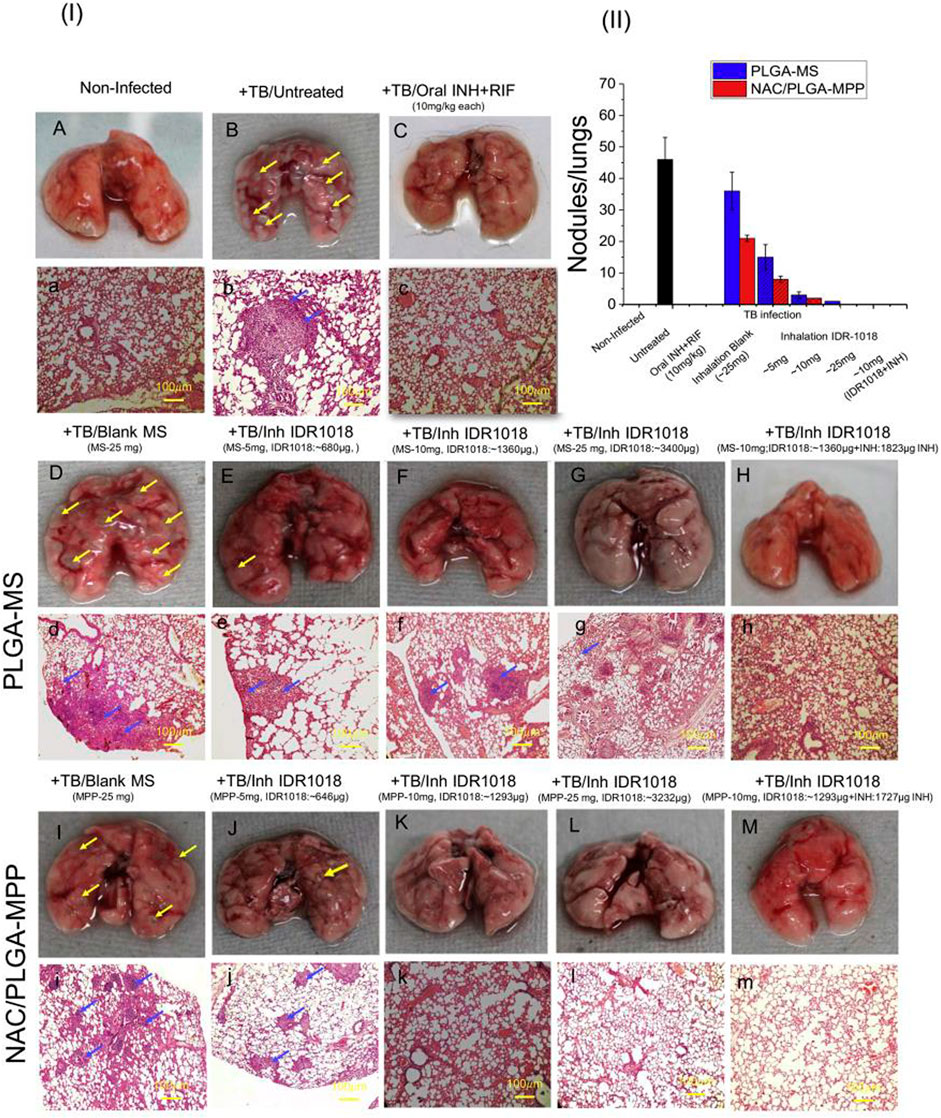
FIGURE 9. (I) Morphological and histopathological changes in the lungs of mice post Mtb infection and treatment. Representative images showing gross anatomic morphology of whole lungs of Balb/c mice infected with virulent Mtb (H37Rv) and treated with various formulations (A–I,K–M) (scale bar: 10 mm). Yellow arrowheads indicate grey-white coloured tubercular nodules (lesion). Histological sections of (H,E) stained lungs of normal, infected and treated mice (a–h,j–m,l). Scale bar: 100 μm. Gross pathology photomicrograph showed granulomas (blue arrowheads) in the lungs (n = 6 animals/group). Graph show quantitative results of macroscopically detectable tubercular nodules per lung. (II) CFU counting in lungs from mice infected with Mtb. Tissue homogenates of each individual mouse were cultured in agar plates and CFU were counted and averaged. Reproduced with permission from Sharma et al. (2020). Copyright © 2020 Elsevier B. V.
Combination therapy has been demonstrated as a potentially effective treatment for MDR-TB. Vemuri et al. (2016) encapsulated moxifloxacin (MOX), econazole (ECZ) and ethionamide (ETH) into PLGA nanoparticles to treat the MDR-TB infected mice. Eight weeks of oral administration of individual nanoformulations (PLGA-NP-ECZ/PLGA-NP-MOX/PLGA-NP-ETH) showed limited reduction of CFUs in lungs and spleen, while with 8 doses of a combination of the three nanoformulations (PLGA-NP-ECZ+PLGA-NPMOX+PLGA-NP-ETH) there was a significant reduction in CFUs in lungs as well as in spleen. Corroborating the results with histopathology revealed that the combination of 3-drugs loaded nanoparticles decreased lung congestion to 50%. This is the first report on the potential efficacy of a combination of ECZ, MOX and ETH nanoparticles against MDR-TB. Similar results were reported by Vibe et al. (2016) that when combined with rifampicin nanoparticles, the PLGA nanoparticles loaded with thioridazine gave a modest increase in the killing of both Mycobacterium Bovis BCG and Mtb in macrophages. The thioridazine nanoparticles showed a significant therapeutic effect combined with rifampicin in the zebrafish, enhancing embryo survival and reducing mycobacterial infection. The results show that thioridazine nanoparticle therapy can improve the antibacterial effect of rifampicin in vivo. Moin et al. (2016) also developed a dual drug conjugate PLGA nanoparticle using isoniazid (INH) and moxifloxacin (MOXI) to combat the multi-drug resistance exhibited by mycobacterial species. The drug-conjugate-loaded PLGA nanoparticles are rapidly hydrolyzed into individual parent molecules at the pH of macrophages and function with different mechanisms of action at the same site in macrophages, thereby preventing the development of drug resistance and the development of tuberculosis. From the results of the experimental work, it can be concluded that incorporating two or more drugs into tuberculosis with the same carrier and target can be an effective strategy against MDR-TB.
Sonodynamic antibacterial chemotherapy (SACT) combined with sonosensitizer-loaded nanoparticles with targeted therapeutic capabilities promises to eliminate bacteria to treat MDR-TB. Li et al. (2021) developed levofloxacin-loaded PLGA-PEG nanoparticles (BM2-LVFX-NPs) with BM2 aptamer conjugated on the surface using cross-linking agents 1-ethyl-3-(3-dimethylaminopropyl) carbodiimide (EDC) and N-hydroxysuccinimide (NHS), to study the antibacterial activity underlying mechanisms of PLGA nanoparticles with targeted therapeutic function against Bacillus Calmette-Guérin bacteria (BCG, an Mtb model in the presence of ultrasound stimulations (Figure 10). PLGA nanoparticles were specifically recognized BCG in vitro and accurately accumulated in the lesion tissue (Figure 11), and the drugs with ultrasound-responsive properties loaded in PLGA nanoparticles were effectively released. Furthermore, PLGA nanoparticles exhibited significant SACT efficiency and higher ROS production levels, resulting in efficient bacterial elimination in vitro. Meanwhile, in vivo experiments, PLGA nanoparticles showed excellent ultrasound therapeutic effects in a BCG-infected rat model (Figure 12). The results show that PLGA nanoparticles containing levofloxacin can effectively transfer therapeutic drugs into cells and improve the bactericidal effect under ultrasound, which may be a targeted therapy strategy for Mtb infection with high biosafety.
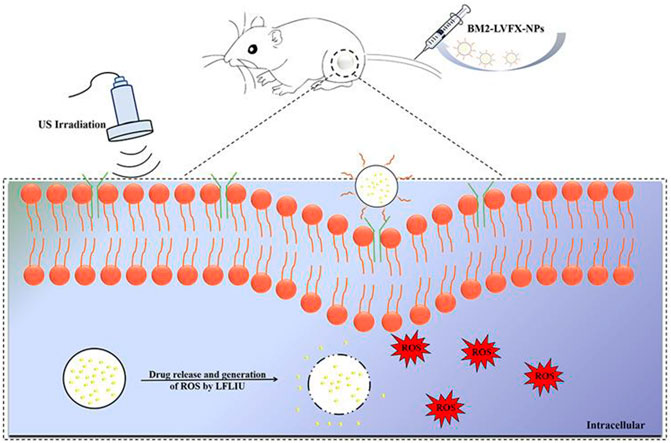
FIGURE 10. BM2-LVFX-NPs for sonodynamic antimicrobial chemotherapy for BCG infection. Reproduced with permission from Li et al. (2021). Copyright © 2021 the authors.
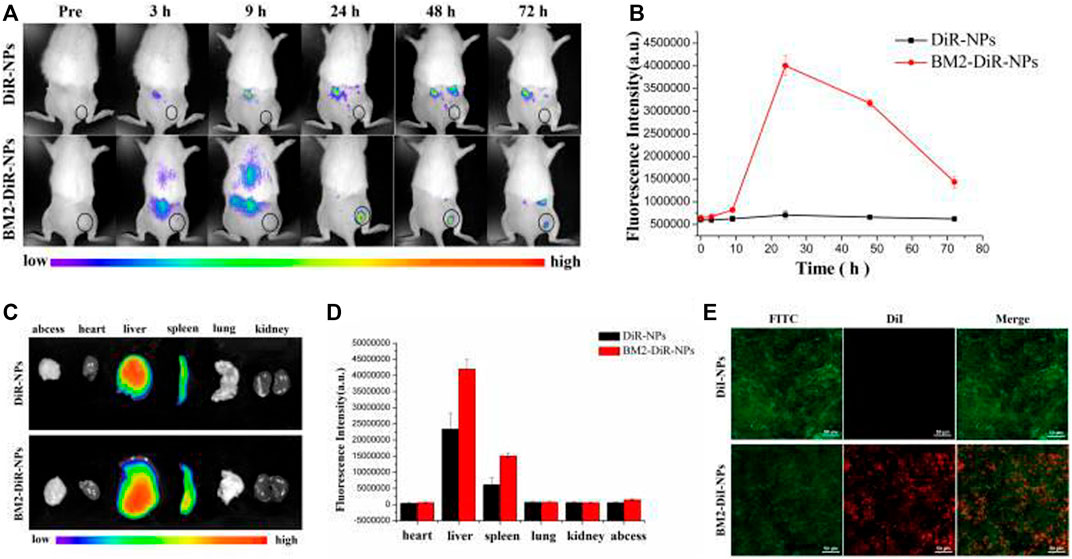
FIGURE 11. Targeting ability of BM2-modified nanoparticles in vivo. (A) Fluorescence images of a BCG-infected rat at 3, 9, 24, 48, and 72 h post injection of DiR-labelled nanoparticles. (B) Quantitative fluorescence intensity (n = 3) of abscess tissue at different time points. (C) Biodistribution of DiR-labeled nanoparticles in major organs extracted from rats at 72 h post injection. (D) Quantitative analysis of fluorescence intensity (n = 3) in major organs. (E) CLSM images of Frozen section of abscess tissues at 24 h post-injection of DiR-loaded nanoparticles. The scale bar is 50 μm. Reproduced with permission from Li et al. (2021). Copyright © 2021 the authors.
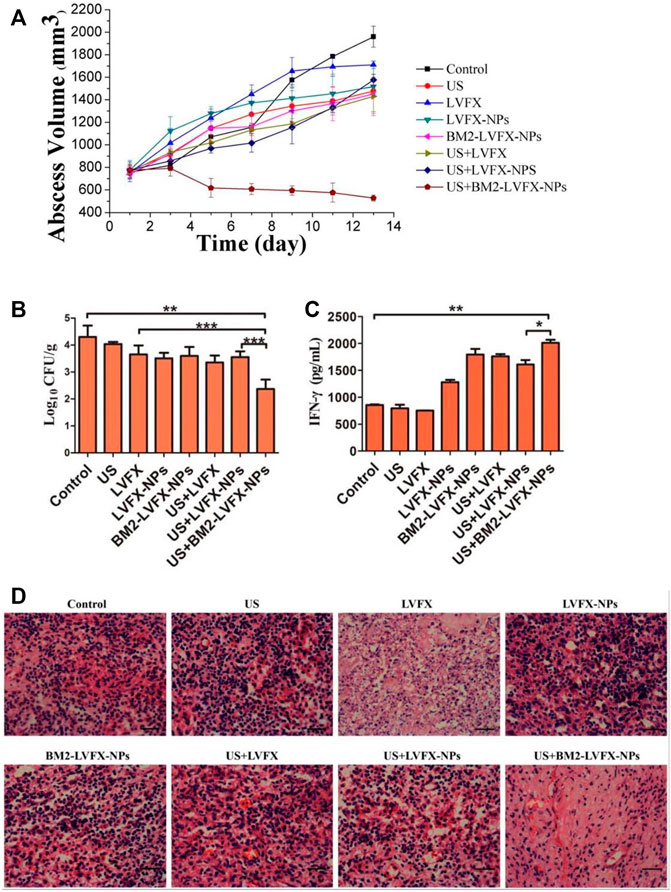
FIGURE 12. In vivo SACT efficacy of BM2-LVFX-NPs combined with ultrasound. (A) The time-dependent abscess volume curves of infected rats in each group. (B) Colony counting analysis (Log10 CFU) of bacterial cultures from the abscess tissue in the rats after a 14-day treatment. **p < 0.01, ***p < 0.001. (C) Serum IFN-γ level of BCG-infected rats on Day 14 after treatment. (D) Histopathologic observation of the infected tissues of every group after being treated in various ways. The scale bar is 50 μm. Reproduced with permission from Li et al. (2021). Copyright © 2021 the authors.
Shortcomings and limitations
Despite recent advances in PLGA micro/nanoparticle drug delivery systems in tuberculosis treatment, many issues still need further addressed. First, it is not an easy task to develop a lyophilization process for dry powder of PLGA micro/nanoparticles, and it is necessary to ensure that the lyophilized powder is easy to redisperse, avoiding aggregation and drug precipitation; Second, the total amount of biomaterial and lyoprotector to be inhaled over time should be evaluated in terms ofchronic lung toxicity. The total amount of materials and lyoprotectants, and thus nanomedicines for tuberculosis treatment, should be optimized to reduce the daily dose of excipients administered to patients. It is also necessary to emphasize the importance of reproducibility and stability (drug loading, encapsulation efficiency and physicochemical properties) in producing drug-loaded PLGA micro/nanoparticles during mass production. The lack of appropriate and specific regulatory guidelines on characterization, study design, and statistical analysis is a common obstacle to the clinical translation of nanoformulations. There is also a need to optimize shared practices to facilitate the translation of nanotechnology from experimental success to clinical practice. In addition, more in vivo data are needed. Only a few studies have shown that the PLGA micro/nanoparticles drug delivery system is effective in preclinical models of infected tuberculosis. This is a severe disadvantage as future clinical studies will depend on available preclinical data. The research on PLGA micro/nano drug delivery systems for the treatment of tuberculosis is still at an early stage, and more investment and capacity are required to make it possible to obtain commercially available micro/nano formulations.
Conclusion and prospect
Drug-resistant tuberculosis is a significant global disease with high morbidity and mortality and remains a major health problem. As current treatment strategies are inadequate, innovative strategies are needed to improve treatment and reduce mortality. The PLGA micro/nanoparticles can be loaded with single or combined drugs with additive/synergistic effects, allowing lower doses of drugs with reduced side effects while improving antituberculosis efficacy of first- and second-line antituberculosis drugs. PLGA micro/nanoparticles offer great potential for more efficient delivery of tuberculosis drugs to lesion sites to improve their efficacy, and the introduction of potent, novel and repurposed drugs will increase the effectiveness of such systems. Although the results obtained so far are too preliminary, it is still believed that PLGA micro/nanoparticles have great potential as a novel therapeutic approach for drug-resistant tuberculosis and reduce the risk of drug-resistant tuberculosis impacting human health. In order to promote the safe and extensive application of the drug-loaded PLGA micro/nanoparticles in the clinical treatment of drug-resistant tuberculosis, the following issues should be paid attention to in the future in-depth research: 1) A reliable animal model is necessarily required to examine the safety of PLGA micro/nanoparticles in vivo because the number of in vivo studies of these pharmaceutical formulations is minimal and requires careful study for human use; 2) Addressing the processing/manufacturing issues for large scale production at an affordable cost will be a fundamental issue in the future; 3) Furthermore, to further realize the progress and efficient delivery of nanomedicines in the lesion sites for precise drug delivery, active participation and cooperation in the fields of nanotechnology, biomedicine, bioengineering and computer analysis are required to make sure nano-drugs will not face any issues during application in clinical trials.
Author contributions
LS wrote this article; HL revised this article; SS checked and review this article.
Funding
This work was supported by the program of the Liaoning Natural Science Foundation (2019-ZD-0976).
Conflict of interest
The authors declare that the research was conducted in the absence of any commercial or financial relationships that could be construed as a potential conflict of interest.
Publisher’s note
All claims expressed in this article are solely those of the authors and do not necessarily represent those of their affiliated organizations, or those of the publisher, the editors and the reviewers. Any product that may be evaluated in this article, or claim that may be made by its manufacturer, is not guaranteed or endorsed by the publisher.
References
Abdelghany, S., Parumasivam, T., Pang, A., Roediger, B., Tang, P., Jahn, K., et al. (2019). Alginate modified-PLGA nanoparticles entrapping amikacin and moxifloxacin as a novel host-directed therapy for multidrug-resistant tuberculosis. J. Drug Deliv. Sci. Technol. 52, 642–651. doi:10.1016/j.jddst.2019.05.025
Al-Qushawi, A., Rassouli, A., Atyabi, F., Peighambari, S. M., Esfandyari-Manesh, M., Shams, G. R., et al. (2016). Preparation and characterization of three tilmicosin-loaded lipid nanoparticles: Physicochemical properties and in-vitro antibacterial activities. Iran. J. Pharm. Res. 15 (4), 663–676.
Anselmo, A. C., and Mitragotri, S. (2014). An overview of clinical and commercial impact of drug delivery systems. J. Control. Release 190, 15–28. doi:10.1016/j.jconrel.2014.03.053
Bobo, D., Robinson, K. J., Islam, J., Thurecht, K. J., and Corrie, S. R. (2016). Nanoparticle-based medicines: A review of FDA-approved materials and clinical trials to date. Pharm. Res. 33 (10), 2373–2387. doi:10.1007/s11095-016-1958-5
Cao, Y., Samy, K. E., Bernards, D. A., and Desai, T. A. (2019). Recent advances in intraocular sustained-release drug delivery devices. Drug Discov. today 24 (8), 1694–1700. doi:10.1016/j.drudis.2019.05.031
Chaurasiya, A., Patra, P., Thathireddy, P., and Gorajiya, A. (2021). “PLGA-based micro- and nano-particles,” in Micro-and nanotechnologies-based product development (Boca Raton: CRC Press), 83–94. doi:10.1201/9781003043164-6
Chen, Y. C., Gad, S. F., Chobisa, D., Li, Y., and Yeo, Y. (2021). Local drug delivery systems for inflammatory diseases: Status quo, challenges, and opportunities. J. Control. Release 330, 438–460. doi:10.1016/j.jconrel.2020.12.025
Chereddy, K. K., Vandermeulen, G., and Préat, V. (2016). PLGA based drug delivery systems: Promising carriers for wound healing activity. Wound Rep Reg 24 (2), 223–236. doi:10.1111/wrr.12404
de Castro, R. R., do Carmo, F. A., Martins, C., Simon, A., de Sousa, V. P., Rodrigues, C. R., et al. (2021). Clofazimine functionalized polymeric nanoparticles for brain delivery in the tuberculosis treatment. Int. J. Pharm. 602, 120655. doi:10.1016/j.ijpharm.2021.120655
De Matteis, L., Jary, D., Lucía, A., García-Embid, S., Serrano-Sevilla, I., Pérez, D., et al. (2018). New active formulations against M. tuberculosis: Bedaquiline encapsulation in lipid nanoparticles and chitosan nanocapsules. Chem. Eng. J. 340, 181–191. doi:10.1016/j.cej.2017.12.110
Dean, R. L. (2005). The preclinical development of Medisorb Naltrexone, a once a month long-acting injection, for the treatment of alcohol dependence. Front. Biosci. 10 (1), 643. doi:10.2741/1559
Ding, D., and Zhu, Q. (2018). Recent advances of PLGA micro/nanoparticles for the delivery of biomacromolecular therapeutics. Mater. Sci. Eng. C 92, 1041–1060. doi:10.1016/j.msec.2017.12.036
Dong, W., Ye, J., Zhou, J., Wang, W., Wang, H., Zheng, X., et al. (2020). Comparative study of mucoadhesive and mucus-penetrative nanoparticles based on phospholipid complex to overcome the mucus barrier for inhaled delivery of baicalein. Acta Pharm. Sin. B 10 (8), 1576–1585. doi:10.1016/j.apsb.2019.10.002
Fineman, M., Flanagan, S., Taylor, K., Aisporna, M., Shen, L. Z., Mace, K. F., et al. (2011). Pharmacokinetics and pharmacodynamics of exenatide extended-release after single and multiple dosing. Clin. Pharmacokinet. 50 (1), 65–74. doi:10.2165/11585880-000000000-00000
Gentile, P., Chiono, V., Carmagnola, I., and Hatton, P. (2014). An overview of poly(lactic-co-glycolic) acid (PLGA)-Based biomaterials for bone tissue engineering. Int. J. Mol. Sci. 15 (3), 3640–3659. doi:10.3390/ijms15033640
Ghitman, J., Biru, E. I., Stan, R., and Iovu, H. (2020). Review of hybrid PLGA nanoparticles: Future of smart drug delivery and theranostics medicine. Mater. Des. 193, 108805. doi:10.1016/j.matdes.2020.108805
Grotz, E., Tateosian, N. L., Salgueiro, J., Bernabeu, E., Gonzalez, L., Manca, M. L., et al. (2019). Pulmonary delivery of rifampicin-loaded soluplus micelles against Mycobacterium tuberculosis. J. Drug Deliv. Sci. Technol. 53, 101170. doi:10.1016/j.jddst.2019.101170
Gupta, A., Sharma, D., Meena, J., Pandya, S., Sachan, M., Kumar, S., et al. (2016). Preparation and preclinical evaluation of inhalable particles containing rapamycin and anti-tuberculosis agents for induction of autophagy. Pharm. Res. 33 (8), 1899–1912. doi:10.1007/s11095-016-1926-0
Helal-Neto, E., Rocha Pinto, S., Portilho, F. L., da Costa, M. D., Pereira, J. X., Nigro, F., et al. (2019). Development and biological evaluation of a new nanotheranostic for tuberculosis. Drug Deliv. Transl. Res. 9 (1), 97–105. doi:10.1007/s13346-018-0577-6
Hrkach, J., and Langer, R. (2020). From micro to nano: Evolution and impact of drug delivery in treating disease. Drug Deliv. Transl. Res. 10 (3), 567–570. doi:10.1007/s13346-020-00769-6
Jain, A., Kunduru, K. R., Basu, A., Mizrahi, B., Domb, A. J., and Khan, W. (2016). Injectable formulations of poly(lactic acid) and its copolymers in clinical use. Adv. drug Deliv. Rev. 107, 213–227. doi:10.1016/j.addr.2016.07.002
Jana, P., Shyam, M., Singh, S., Jayaprakash, V., and Dev, A. (2021). Biodegradable polymers in drug delivery and oral vaccination. Eur. Polym. J. 142, 110155. doi:10.1016/j.eurpolymj.2020.110155
Joshi, M., and Prabhakar, B. (2021). Development of respirable rifampicin loaded bovine serum albumin formulation for the treatment of pulmonary tuberculosis. J. Drug Deliv. Sci. Technol. 61, 102197. doi:10.1016/j.jddst.2020.102197
Khawbung, J. L., Nath, D., and Chakraborty, S. (2021). Drug resistant tuberculosis: A review. Comp. Immunol. Microbiol. Infect. Dis. 74, 101574. doi:10.1016/j.cimid.2020.101574
Koerner, J., Horvath, D., Herrmann, V. L., MacKerracher, A., Gander, B., Yagita, H., et al. (2021). PLGA-particle vaccine carrying TLR3/RIG-I ligand Riboxxim synergizes with immune checkpoint blockade for effective anti-cancer immunotherapy. Nat. Commun. 12 (1), 2935. doi:10.1038/s41467-021-23244-3
Kumar, A. R., and Aeila, A. S. S. (2019). Sustained release matrix type drug delivery system: An overview. World J. Pharma Pharm. Sci. 8 (12), 470–480. doi:10.20959/wjpps20201-15241
Kumar, G., Malhotra, S., Shafiq, N., Pandhi, P., Khuller, G. K., and Sharma, S. (2011a). In vitrophysicochemical characterization and short termin vivotolerability study of ethionamide loaded PLGA nanoparticles: Potentially effective agent for multidrug resistant tuberculosis. J. Microencapsul. 28 (8), 717–728. doi:10.3109/02652048.2011.615948
Kumar, G., Sharma, S., Shafiq, N., Pandhi, P., Khuller, G. K., and Malhotra, S. (2011b). Pharmacokinetics and tissue distribution studies of orally administered nanoparticles encapsulated ethionamide used as potential drug delivery system in management of multi-drug resistant tuberculosis. Drug Deliv. 18 (1), 65–73. doi:10.3109/10717544.2010.509367
Lagreca, E., Onesto, V., Di Natale, C., La Manna, S., Netti, P. A., and Vecchione, R. (2020). Recent advances in the formulation of PLGA microparticles for controlled drug delivery. Prog. Biomater. 9 (4), 153–174. doi:10.1007/s40204-020-00139-y
Lai, Y., Li, Y., Cao, H., Long, J., Wang, X., Li, L., et al. (2019). Osteogenic magnesium incorporated into PLGA/TCP porous scaffold by 3D printing for repairing challenging bone defect. Biomaterials 197, 207–219. doi:10.1016/j.biomaterials.2019.01.013
Lam, S. J., Wong, E. H. H., Boyer, C., and Qiao, G. G. (2018). Antimicrobial polymeric nanoparticles. Prog. Polym. Sci. 76, 40–64. doi:10.1016/j.progpolymsci.2017.07.007
Lange, C., Dheda, K., Chesov, D., Mandalakas, A. M., Udwadia, Z., and Horsburgh, C. R. (2019). Management of drug-resistant tuberculosis. Lancet 394 (10202), 953–966. doi:10.1016/s0140-6736(19)31882-3
Lawlor, C., O’Connor, G., O’Leary, S., Gallagher, P. J., Cryan, S. A., Keane, J., et al. (2016). Treatment of Mycobacterium tuberculosis-infected macrophages with poly(lactic-Co-glycolic acid) microparticles drives NFκB and autophagy dependent bacillary killing. PLoS One 11 (2), e0149167. doi:10.1371/journal.pone.0149167
Lemmer, Y., Kalombo, L., Pietersen, R. D., Jones, A. T., Semete-Makokotlela, B., Van Wyngaardt, S., et al. (2015). Mycolic acids, a promising mycobacterial ligand for targeting of nanoencapsulated drugs in tuberculosis. J. Control. release 211, 94–104. doi:10.1016/j.jconrel.2015.06.005
Li, G., Li, J., Hou, Y., Xie, S., Xu, J., Yang, M., et al. (2021). Levofloxacin-loaded nanosonosensitizer as a highly efficient therapy for Bacillus calmette-guérin infections based on bacteria-specific labeling and sonotheranostic strategy. Int. J. Nanomedicine 16, 6553–6573. doi:10.2147/ijn.s321631
Liang, Q., Xiang, H., Li, X., Luo, C., Ma, X., Zhao, W., et al. (2020). Development of rifapentine-loaded PLGA-based nanoparticles: In vitro characterisation and in vivo study in mice. Int. J. Nanomedicine 15, 7491–7507. doi:10.2147/ijn.s257758
Marcianes, P., Negro, S., Barcia, E., Montejo, C., and Fernández-Carballido, A. (2020). Potential active targeting of gatifloxacin to macrophages by means of surface-modified PLGA microparticles destined to treat tuberculosis. AAPS PharmSciTech 21 (1), 15. doi:10.1208/s12249-019-1552-3
Mashele, S. A., Steel, H. C., Matjokotja, M. T., Rasehlo, S. S., Anderson, R., and Cholo, M. C. (2022). Assessment of the efficacy of clofazimine alone and in combination with primary agents against Mycobacterium tuberculosis in vitro. J. Glob. Antimicrob. Resist. 29, 343–352. doi:10.1016/j.jgar.2022.03.008
McKeage, K. (2015). Pasireotide in acromegaly: A review. Drugs 75 (9), 1039–1048. doi:10.1007/s40265-015-0413-y
Mironov, A. V., Grigoryev, A. M., Krotova, L. I., Skaletsky, N. N., Popov, V. K., and Sevastianov, V. I. (2017). 3D printing of PLGA scaffolds for tissue engineering. J. Biomed. Mat. Res. 105 (1), 104–109. doi:10.1002/jbm.a.35871
Moin, A., Raizaday, A., Hussain, T., and Nagshubha, B. (2016). Development and optimization of dual drugs (Isoniazid and moxiflox-acin) loaded functional PLGA nanoparticles for the synergistic treatment of tuberculosis. Curr. Drug Deliv. 13 (7), 1034–1052. doi:10.2174/1567201813666160502124811
Molavi, F., Barzegar-Jalali, M., and Hamishehkar, H. (2020). Polyester based polymeric nano and microparticles for pharmaceutical purposes: A review on formulation approaches. J. Control. Release 320, 265–282. doi:10.1016/j.jconrel.2020.01.028
Mustafa, S., Devi, V. K., and Pai, R. S. (2017). Effect of PEG and water-soluble chitosan coating on moxifloxacin-loaded PLGA long-circulating nanoparticles. Drug Deliv. Transl. Res. 7 (1), 27–36. doi:10.1007/s13346-016-0326-7
Nkanga, C. I., Fisch, A., Rad-Malekshahi, M., Romic, M. D., Kittel, B., Ullrich, T., et al. (2020). Clinically established biodegradable long acting injectables: An industry perspective. Adv. drug Deliv. Rev. 167, 19–46. doi:10.1016/j.addr.2020.11.008
Nugraha, R. V., Yunivita, V., Santoso, P., Aarnoutse, R. E., and Ruslami, R. (2021). Clofazimine as a treatment for multidrug-resistant tuberculosis: A review. Sci. Pharm. 89 (2), 19. doi:10.3390/scipharm89020019
O'Connor, G., Krishnan, N., Fagan-Murphy, A., Cassidy, J., O'Leary, S., Robertson, B. D., et al. (2019). Inhalable poly(lactic-co-glycolic acid) (PLGA) microparticles encapsulating all-trans-Retinoic acid (ATRA) as a host-directed, adjunctive treatment for Mycobacterium tuberculosis infection. Eur. J. Pharm. Biopharm. 134, 153–165. doi:10.1016/j.ejpb.2018.10.020
Park, K., Skidmore, S., Hadar, J., Garner, J., Park, H., Otte, A., et al. (2019). Injectable, long-acting PLGA formulations: Analyzing PLGA and understanding microparticle formation. J. Control. Release 304, 125–134. doi:10.1016/j.jconrel.2019.05.003
Patel, A., Redinger, N., Richter, A., Woods, A., Neumann, P. R., Keegan, G., et al. (2020). In vitro and in vivo antitubercular activity of benzothiazinone-loaded human serum albumin nanocarriers designed for inhalation. J. Control. Release 328, 339–349. doi:10.1016/j.jconrel.2020.08.022
Patil, K., Bagade, S., Bonde, S., Sharma, S., and Saraogi, G. (2018). Recent therapeutic approaches for the management of tuberculosis: Challenges and opportunities. Biomed. Pharmacother. 99, 735–745. doi:10.1016/j.biopha.2018.01.115
Pawde, D. M., Viswanadh, M. K., Mehata, A. K., Sonkar, R., Haldiya, N. K., Poddar, S., et al. (2020). Mannose receptor targeted bioadhesive chitosan nanoparticles of clofazimine for effective therapy of tuberculosis. Saudi Pharm. J. 28 (12), 1616–1625. doi:10.1016/j.jsps.2020.10.008
Rajabnezhad, S., Casettari, L., Lam, J. K. W., Nomani, A., Torkamani, M. R., Palmieri, G. F., et al. (2016). Pulmonary delivery of rifampicin microspheres using lower generation polyamidoamine dendrimers as a carrier. Powder Technol. 291, 366–374. doi:10.1016/j.powtec.2015.12.037
Rani, S., Gothwal, A., Khan, I., Pachouri, P. K., Bhaskar, N., Gupta, U. D., et al. (2018). Smartly engineered PEGylated di-block nanopolymeric micelles: Duo delivery of isoniazid and rifampicin against Mycobacterium tuberculosis. AAPS PharmSciTech 19 (7), 3237–3248. doi:10.1208/s12249-018-1151-8
Reid, M. J. A., Arinaminpathy, N., Bloom, A., Bloom, B. R., Boehme, C., Chaisson, R., et al. (2019). Building a tuberculosis-free world: The Lancet Commission on tuberculosis. Lancet 393 (10178), 1331–1384. doi:10.1016/S0140-6736(19)30024-8
Rentzepis, P. J., Kurian, M. J., Carracher, A. M., and Close, K. L. (2018). Practical ways to achieve targets in diabetes care. J. Diabetes 10 (12), 911–915. doi:10.1111/1753-0407.12842
Saifullah, B., Chrzastek, A., Maitra, A., Naeemullah, B., Fakurazi, S., Bhakta, S., et al. (2017). Novel anti-tuberculosis nanodelivery formulation of ethambutol with graphene oxide. Molecules 22 (10), 1560. doi:10.3390/molecules22101560
Sharma, A., Vaghasiya, K., Gupta, P., Singh, A. K., Gupta, U. D., and Verma, R. K. (2020). Dynamic mucus penetrating microspheres for efficient pulmonary delivery and enhanced efficacy of host defence peptide (HDP) in experimental tuberculosis. J. Control. release 324, 17–33. doi:10.1016/j.jconrel.2020.05.013
Soria-Carrera, H., Lucía, A., Matteis, L., Aínsa, J. A., la Fuente, J. M., and Martín‐Rapún, R. (2019). Polypeptidic micelles stabilized with sodium alginate enhance the activity of encapsulated bedaquiline. Macromol. Biosci. 19 (4), 1970012. doi:10.1002/mabi.201970012
Thomas, D., KurienThomas, K., and Latha, M. S. (2020). Preparation and evaluation of alginate nanoparticles prepared by green method for drug delivery applications. Int. J. Biol. Macromol. 154, 888–895. doi:10.1016/j.ijbiomac.2020.03.167
Ufimtseva, E., Eremeeva, N., Bayborodin, S., Umpeleva, T., Vakhrusheva, D., and Skornyakov, S. (2019). Mycobacterium tuberculosis with different virulence reside within intact phagosomes and inhibit phagolysosomal biogenesis in alveolar macrophages of patients with pulmonary tuberculosis. Tuberculosis 114, 77–90. doi:10.1016/j.tube.2018.12.002
Vemuri, N., K. Khuller, G., Prabhakar, T., Pal, N., Gupta, P., and Gupta, U. (2015). Nanoformulations of moxifloxacin, econozole and ethionamide as novel treatment regimens against MDR TB - an experimental study. Curr. Nanosci. 12 (1), 110–117. doi:10.2174/1573413711666150901203515
Vibe, C. B., Fenaroli, F., Pires, D., Wilson, S. R., Bogoeva, V., Kalluru, R., et al. (2016). Thioridazine in PLGA nanoparticles reduces toxicity and improves rifampicin therapy against mycobacterial infection in zebrafish. Nanotoxicology 10 (6), 680–688. doi:10.3109/17435390.2015.1107146
Vieira, A. C. C., Magalhães, J., Rocha, S., Cardoso, M. S., Santos, S. G., Borges, M., et al. (2017). Targeted macrophages delivery of rifampicin-loaded lipid nanoparticles to improve tuberculosis treatment. Nanomedicine 12 (24), 2721–2736. doi:10.2217/nnm-2017-0248
World Health Organization (2010). Global tuberculosis report 2010. Geneva: World Health Organization.
World Health Organization (2019a). Global tuberculosis report 2019. Geneva: World Health Organization.
World Health Organization (2020). Global tuberculosis report 2020. Geneva: World Health Organization.
World Health Organization (2021a). Global tuberculosis report 2021. Geneva: World Health Organization.
World Health Organization (2021b). Rapid communication on updated guidance on the management of tuberculosis in children and adolescents. Geneva: World Health Organization.
World Health Organization (2019b). WHO consolidated guidelines on drug-resistant tuberculosis treatment. Geneva: World Health Organization.
Keywords: PLGA microparticles, PLGA nanoparticles, drug-resistant tuberculosis, Mycobacterium tuberculosis, combination therapy, inhalable therapy
Citation: Shao L, Shen S and Liu H (2022) Recent advances in PLGA micro/nanoparticle delivery systems as novel therapeutic approach for drug-resistant tuberculosis. Front. Bioeng. Biotechnol. 10:941077. doi: 10.3389/fbioe.2022.941077
Received: 11 May 2022; Accepted: 29 June 2022;
Published: 22 July 2022.
Edited by:
Jianshe Hu, Northeastern University, ChinaReviewed by:
Padmani Sandhu, Chandigarh University, IndiaAngel León-Buitimea, Universidad Autonoma de Nuevo Leon, Mexico
Nibedita Mahata, National Institute of Technology, India
Copyright © 2022 Shao, Shen and Liu. This is an open-access article distributed under the terms of the Creative Commons Attribution License (CC BY). The use, distribution or reproduction in other forums is permitted, provided the original author(s) and the copyright owner(s) are credited and that the original publication in this journal is cited, in accordance with accepted academic practice. No use, distribution or reproduction is permitted which does not comply with these terms.
*Correspondence: Shu Shen, c2hlbnNodTU1QDE2My5jb20=