- 1Department of Botany and Microbiology, Faculty of Science, Al-Azhar University, Assiut, Egypt
- 2Food Science and Technology Department, Faculty of Agriculture, Al-Azhar University, Assiut, Egypt
- 3Department of Pharmacognosy, Faculty of Pharmacy, Al-Azhar University, Assiut, Egypt
- 4Department of Biology, College of Science and Arts, Al Bahah University, Al-Mandaq, Saudi Arabia
- 5Department of Biology, College of Science, Taif University, Taif, Saudi Arabia
- 6Colin Ratledge Center for Microbial Lipids, School of Agricultural Engineering and Food Science, Shandong University of Technology, Zibo, China
- 7Department of Genetics, Faculty of Agriculture, Menoufiya University, Sheben Al Kom, Egypt
- 8Department of Botany and Microbiology, Faculty of Science, Assiut University, Asyut, Egypt
Oxidative stress is involved in the pathophysiology of multiple health complications, and it has become a major focus in targeted research fields. As known, black seeds are rich sources of bio-active compounds and widely used to promote human health due to their excellent medicinal and pharmaceutical properties. The present study investigated the antioxidant potency of various black seeds from plants and their derived mycoendophytes, and determined the total phenolic and flavonoid contents in different extracts, followed by characterization of major constituents by HPLC analysis. Finally, in silico docking determined their binding affinities to target myeloperoxidase enzymes. Ten dominant mycoendophytes were isolated from different black seed plants. Three isolates were then selected based on high antiradical potency and further identified by ITS ribosomal gene sequencing. Those isolated were Aspergillus niger TU 62, Chaetomium madrasense AUMC14830, and Rhizopus oryzae AUMC14823. Nigella sativa seeds and their corresponding endophyte A. niger had the highest content of phenolics in their n-butanol extracts (28.50 and 24.43 mg/g), flavonoids (15.02 and 11.45 mg/g), and antioxidant activities (90.48 and 81.48%), respectively, followed by Dodonaea viscosa and Portulaca oleracea along with their mycoendophytic R. oryzae and C. madrasense. Significant positive correlations were found between total phenolics, flavonoids, and the antioxidant activities of different tested extracts. The n-butanol extracts of both black seeds and their derived mycoendophytes showed reasonable IC50 values (0.81–1.44 mg/ml) compared to the control with significant correlations among their phytochemical contents. Overall, seventeen standard phenolics and flavonoids were used, and the compounds were detected in different degrees of existence and concentration in the examined extracts through HPLC analysis. Moreover, the investigation of the molecular simulation results of detected compounds against the myeloperoxidase enzyme revealed that, as a targeted antioxidant, rutin possessed a high affinity (−15.3184 kcal/mol) as an inhibitor. Taken together, the black seeds and their derived mycoendophytes are promising bio-prospects for the broad industrial sector of antioxidants with several valuable potential pharmaceutical and nutritional applications.
1 Introduction
Oxidants, represented by reactive oxygen or nitrogen species , as an output of metabolic processes, are causative of oxidative stress. These free radicals induce structural changes in cellular biomacromolecules, such as lipids, proteins, and nucleic acids, which eventually cause harm to cells (Apak et al., 2016). Living systems have varied modes of protection against free radicals, the efficacy of which is reduced by aging (Torre et al., 2019). Due to the accumulation of oxidants along with their affirmative reduction prospecting, many familiar disorders such as cancer, Alzheimer’s, renal failure, atherosclerosis, Parkinson’s, heart diseases, and diabetes have developed. The harmful impact of free radicals can be voided by antioxidants—molecules generated endogenously (enzymatic: catalase, superoxide dismutase, and gluthatione peroxidase; nonenzymatic: vitamin A) or provided exogenously (nonenzymatic: organic natural supplements) (Charmforoshan et al., 2019; Sajjadi et al., 2019; Munteanu and Apetrei, 2021).
Bio-efficient natural products offer a valuable source of novel antioxidants and antimicrobial agents, besides their minimal side effects and virtue therapeutic prospects (Farag and Swaby, 2018). Plants are known to produce a vast number of natural secondary metabolites with unique pharmacological potential; these by-products are phenols, alkaloids, and terpenoids (Tavassoli and Djomeh, 2011). Although a wide spectrum of microorganisms produce biopotent by-products, the research deriving these secondary metabolites has pivoted principally on medicinal plants (Amer, 2018).
Mycoendophytes are fungi that subsist within plant tissues without generating any adverse symptoms (Meenatchi et al., 2016). Diverse fungal endophytes biosynthesize biopotent by-products that imitate their host plant, along with having varied nutritional, industrial and medical applications (Sharma et al., 2016). Due to a high biodiversity and biochemical evolution, mycoendophytes have the capacity to utilize sundry substrates, with a broad output of natural products (Guanatilaka, 2006; Fernandes et al., 2009). These metabolites encompass alkaloids, phenols, steroids, terpenoids, and isocoumarins (Schulz et al., 2002). There have been few studies concerning the bioactivity of black seeds and their derived mycoendophytes. Extracts derived from black seeds using various kinds of organic solvents, as well as their endophytic fungi (Mohamed et al., 2021), possess a significant antioxidant (Ashraf et al., 2011; Metwaly et al., 2019), antibacterial (Youssef et al., 2015; Sabra and Ismail, 2019), antifungal (Rizzello et al., 2009; Khattak et al., 2020), and anticancer efficacy (Abdulmyanova et al., 2016; Leporini et al., 2018).
The polyphenolic compounds, particularly flavonoids, are reported to play a significant role in antioxidant defense mechanisms and provide protection against body damage by reactive oxygen species (ROS) (Shahidi and Ambigaipalan, 2015). Despite their great beneficial effects on human health, flavonoids cannot be biosynthesized in the human body, making diet the only means of uptake (Magiera et al., 2015). Thus, their existence in natural products, especially in foods and feeds, makes them an outstanding choice as a nutraceutical. Polyphenols are widespread in plants and fungi, in which they play different roles as structural and functional metabolites (Cimmino et al., 2013). Neoflavones, naturally occurring flavonoids (Jung et al., 2018), manifest antibacterial activities (Veselinovic et al., 2014). The catechin polyphenols can act on S. aureus, through destabilization and permeabilization of the cell membrane as well as inhibition of enzymes (Ferrazzano et al., 2011). Protocatechuic acid exhibits antifungal, anti-inflammatory, antiviral, anti-hepatotoxic, apoptotic, and neuroprotective activities (Khadem and Marles, 2010).
Computer-aided drug design and in silico assay technology minimize squandered time and economic load in the drug discovery process (Dincel et al., 2020). Molecular docking has been extensively employed to resolve interactions between bio-efficient molecules and proteins and to investigate their bioactivity (Kongpichitchoke et al., 2016). N. sativa (black seeds) is a widely used medicinal plant throughout the world. According to one of the Prophet Muhammad’s (PBUH) statements, “The black seed can heal every disease, except death” [Sahih Bukhari vol. 7 book 71 # 592] (Ishtiaq et al., 2013; Ali et al., 2018). Different black seeds obtained from different plant species, along with seeds of the common N. sativa plant, were investigated to: 1) identify mycoendophytes associated with black seeds that belong to different plant species; 2) evaluate the antioxidant potential of promising isolated strains along with their host plants; 3) determine the phenolic and flavonoid components responsible for antioxidant activity using HPLC analysis, and 4) analyze these components to determine their binding affinities to target myeloperoxidase, an enzyme that catalyzes the formation of reactive intermediates.
2 Materials and Methods
2.1 Collection of Plant Seeds
Ten different healthy black seed plants, including Allium ampeloprasum, Allium cepa, Amaranthus retroflexus, Dodonaea viscose, N. sativa, Ocimum basilicum, Papaver somniferum, Piper nigrum, and Portulaca oleracea, were collected from Assiut governorate (27°10′48.4824″N, 31°11′21.4188″E), Egypt, while Pancratium maritimum mature seeds were collected from the Alexandrian coast of the Mediterranean Sea in Egypt (30°56′51ʺN, 29°30′41ʺE) (Mohamed et al., 2018) for the isolation of endophytic fungi. Plant seeds were identified by plant taxonomy staff members based on reference books with species descriptions, keys, and illustrations. Black seed plant voucher specimens were deposited at the herbarium of the Botany and Microbiology Department, Faculty of Science, Al-Azhar University, Assiut, Egypt, with voucher numbers.
2.2 Isolation of Fungal Endophytes
The seeds were surface sterilized for the isolation of endophyic fungi, as described by Geisen et al. (2017), with some modifications. The seeds were rinsed with sterile double distilled water for 5 min, and then sterilized in 5% sodium hypochlorite solution for 5 min, followed by soaking in ethanol 70% for 3 min. After that, the seeds were washed twice with sterile double distilled water and dried using filter papers under aseptic conditions. Individual seeds were inoculated into potato dextrose agar (PDA) medium, containing potato infusion 200 g/L, dextrose 20 g/L, and agar 20 g/L into 1,000 ml distilled H2O with final pH (5:6 ± 0:2), in addition to chloramphenicol and Rose Bengal, as bactericide and fast-growing fungi restrictor, respectively. In addition, 100 μl of the resulting washing water suspensions were streaked on PDA. All plates were incubated at 28 ± 2°C and monitored periodically for fungal development for up to 2 weeks. The growing fungal mycelia were picked, purified, and kept on PDA slants at 4°C for further investigation.
2.3 Identification of Fungal Isolates
The fungal cultures were characterized on the basis of their morphological features. The identified fungi were confirmed by Prof. Ahmed M. Moharram, Professor of Mycology, Botany and Microbiology Department, Faculty of Science, Assiut University, Egypt, and Head Manager of Assiut University Mycological Center (AUMC), and the most active selective isolates were confirmed using molecular biological protocols (Hassan et al., 2019; Mohamed et al., 2020), and deposited in the AUMC culture collection with institutional numbers.
2.4 Molecular Identification of the Selected Fungi
2.4.1 Isolation of Genomic DNA
Czapek-Dox broth culture medium was employed to cultivate the pure fungi for 5 days at 25–28°C. The extraction procedures of the total DNA from each isolate were performed using a specific kit “Norgen Plant/Fungi DNA Isolation Kit (Sigma, Thorold, Canada)” according to Hassan et al. (2019). DNA was kept after elution at −20°C for further studies.
2.4.2 PCR Amplification and Nucleotide Sequence Analysis
Specific universal primers ITS-1 (5′-TCC GTA GGT GAA CCT GCG G-3′) and ITS-4 (5′-TCC TCC GCT TAT TGA TAT GC-3′) were used in the present investigation to amplify the ITS region of the selected strains as previously suggested by Mohamed et al. (2020). PCR reaction was achieved as reported by Hassan et al. (2019), and the mixture of PCR tubes contained 25 μl, viz., 12.5 μl of PCR master mix, 1 μl from both forward and reverse primers (approx. 20 pmol), genomic DNA 1 and 9.5 μl of ddH2O. The amplification of DNA was carried out in Thermocycler C1000 Touch™ (Bio-Rad, Germany). The PCR products obtained were resolved on agarose gel 1.5% using TBE buffer, and visualized through a gel documentation system. Then the gel extraction kit (Omega Bio-tek) was applied to purify the PCR product based on the manufacturer’s instructions. All PCR products were sequenced with the gene analyzer 3121 sequencing service (Macrogen Co., Seoul, South Korea). The sequences of selected strains were aligned with the BLAST search tool at (NCBI) to detect sequence similarity. The obtained sequences were checked and analyzed using BioEdit software program version No. 7.2.5, and the homology search was carried out by comparison to isolates from the sequencing databases using a BLAST search algorithm of the GenBank (http://www.ncbi.nlm.nih.gov/BLAST).
2.5 Preparation of Extracts
2.5.1 Propagation and Extraction of Fungal Metabolites
Propagation of isolated fungi was carried out by solid state fermentation (Al Mousa et al., 2021) on autoclaved 100 g rice and 100 ml H2O in 1L Erlenmeyer flasks. After cooling, 2 ml spore suspensions (105 cfu/ml) were inoculated into flasks, and incubated at 28°C for 1 month. After that, the fermented rice was defatted by petroleum ether, and then sequential extraction of fungal metabolites was employed using gradual polar solvents, namely n-butanol, acetone and methanol, respectively, for 24 h at room temperature (Al-Fatimi et al., 2007). Each extract was filtered and dried up with a rotavapor (BÜCHI R-114, Switzerland).
2.5.2 Preparation of Seeds Extracts
Dried seeds were powdered using an electric mill, and 30 g was defatted and extracted as described above. Each extract was filtered and dried up with a rotavapor (BÜCHI R-114, Switzerland) to yield the crude extract that was collected in a vial for further investigation.
2.6 Estimation of Total Phenolic Content
Total phenolic content assay was assessed using modified procedures of Suleria et al. (2012). In brief, 0.5 ml of the specimen (10 mg/ml) was added to the same volume of Folin–Ciocalteu’s phenol reagent, followed by the addition to the reaction solution of 1 ml of 10% Na2CO3 after 3 min. Incubation of the mixture proceeded under shaking and dark conditions at 180 rpm for 60 min at 25°C. Measurement of the absorbance was at 750 nm. Phenolic content was declared as gallic acid equivalent (GAE) (mg/g) through the later equation due to the standardization curve: y = 0.0169x − 0.1172, R2 = 0.9588. The standard curve of gallic acid was linear between 0.5 and 100 μg/ml.
2.7 Determination of Total Flavonoid Content
A previous method reported by Quettier-Deleu et al. (2000) was conducted to measured total flavonoid content; 0.5 ml of each extract (10 mg/ml) was carefully mixed with 1.0 ml of a 2% (v/v) AlCl3.6H2O ethanolic solution, and the absorbance was measured at 430 nm after 10 min. The total flavonoid content was explicated as quercetin equivalent (QE) (mg/g) by employing the later equation due to the standardization curve: y = 0.0208x − 0.2381, R2 = 0.9678. The calibration curve of quercetin was linear between 0.5 and 100 μg/ml.
2.8 Antioxidant Activity Assay
2.8.1 DPPH Scavenging Activity (%) Assay
The antioxidant properties of the test samples were measured for scavenging activity or hydrogen donating form based on the procedure reported before (Brand-Williams et al., 1995). While the DPPH radical was scavenged, the color changed from purple to yellow with a 517 nm absorbance decrease. Then, 1.8 ml of 0.1 mM DPPH (Sigma-Aldrich, Germany) (4 mg/100 ml of methanol) mixed solution was added to 0.2 ml of the tested samples in absolute methanol at various concentrations (1, 0.8, 0.6, 0.4, 0.2, 0.1, and 0.05 mg/ml) beside the blank. The mixture was left aside at room temperature (shaken vigorously in-between) for 30 min and absorbance was determined by spectrophotometer (Jenway 7315) at 517 nm. Butylated hydroxytoluene (BHT) was employed as a positive control and all measurements were performed in triplicate. The following formula was used to determine the capacity to scavenge the DPPH radical
where A is the negative control absorbance (methanol and DPPH) and B is the sample absorbance (DPPH, methanol and sample). The IC50 was obtained by interpolation from linear regression analysis, where the obtained regression equation was y = ax + b, IC50 was calculated as IC50 = (0.5 − b)/a. The IC50 value denotes the level of the antioxidant capacity of the tested extracts. The IC50 value is inversely proportional to the free radical scavenging property of the sample.
2.8.2 Hydrogen Peroxide Scavenging Activity Assay
The IC50 values of the most potent extracts were investigated according to the method described by Ruch et al. (1989), where the extracts at various concentrations (1, 0.8, 0.6, 0.4, 0.2, 0.1, and 0.05 mg/ml) beside the blank were added to a hydrogen peroxide solution (0.6 ml, 40 mM) and the absorbance at 230 nm was determined 10 min later against a blank solution containing the phosphate buffer. The percentage of hydrogen peroxide scavenging of the extracts were calculated: % Scavenged [H2O2] = [(A0 − A1)/A0] × 100, where A0 was the control absorbance and A1 the absorbance of the sample.
2.8.3 Nitric Oxide Scavenging Activity Assay
The scavenging impact of the most potent extracts on nitric oxide (NO) was determined according to Sreejayan and Rao (1997). Sodium nitroprusside (10 mM) was mixed with extracts (1, 0.8, 0.6, 0.4, 0.2, 0.1, and 0.05 mg/ml) and incubated for 150 min. After that, the test was mixed with 0.5 ml of Griess reagent and then measured at 546 nm. In the control, sample extract was substituted by PBS. The capability of scavenging NO was calculated using the following equation: Scavenging effect (%) = [1 − (A sample/A control)] × 100.
2.9 High Performance Liquid Chromatography Analysis of Flavonoid and Phenolic Contents
The principal flavonoids and phenolic compounds were detected and identified using an Agilent 1260 series HPLC system with an Eclipse C18 column (4.6 mm × 250 mm i.d., 5 μm) and water (A) and 0.05% trifluoroacetic acid in acetonitrile (B) as the mobile phase at a flow rate of 1 ml/min. The mobile phase elution linear gradient was used as follows: 0 min (82% A:18% B); 0–5 min (80% A:20% B); 5–8 min (60% A:40% B); 8–12 min (60% A:40% B); and 12–20 min (82% A:18% B). The multi-wavelength detector used was monitored at a wavelength of 280 nm. The injection volume for each sample was 5 μl and the column temperature was maintained at 40°C. Each sample’s phenolic and flavonoid composition was determined by comparing their retention times and spectral reference data with the external standard controls. All standards, namely gallic acid, chlorogenic acid, catechin, methyl gallate, caffeic acid, syringic acid, pyrocatechol, rutin, ellagic acid, coumaric acid, vanillin, ferulic acid, naringenin, querectin, cinnamic acid, kaempferol, and hesperetin were purchased from Sigma-Aldrich (Khalil et al., 2020; Abdel-Aziz et al., 2021).
2.10 Molecular Docking Simulations With Antioxidant Target Protein
Molecular Operating Environment software (MOE 2019.01) was utilized for optimization of both examined receptors and compounds for docking study. The inhibition of in vivo production of reactive oxygen species (ROS) by the seventeen detected compounds was assessed by evaluating their ligand-protein binding patterns and interactions with myeloperoxidase enzymatic protein (1DNU), retrieved from the Protein Data Bank (https://www.rcsb.org/pdb) (Vadabingi et al., 2020).
The target protein was prepared for docking by Quickprep function and removing unnecessary water molecules and all co-crystallized ligands and metals. The active site for the interactions was selected by site finder built-in function. Further, the detected compounds were subjected to 3D generation, 3D potentiation energy minimization using Merck Molecular Forcefield (MMFF94x), and stochastic conformational search. The conformers with minimum energy were selected and a virtual ligand database was generated. The rigid receptor-flexible ligand was adopted as the docking procedure using Triangle Matcher placement and rigid receptor refinement with London dG as the scoring and rescoring algorithm retaining 10 poses. The docking score and root mean square deviation (RSMD) were recorded. 2D and 3D interaction figures were generated by the MOE visualization tool (Gabr et al., 2019).
2.11 Data Analysis
All experiments and measurements were performed thrice. Using the SPSS, software program (version No. 16), and one-way ANOVA, the values were expressed as the mean ± SE at the 0.05 significance level.
3 Results
3.1 Fungal Isolates and Their Sequence Data
Based on the phenotypic and microscopic cultural characteristics, ten dominant endophytic fungi belonged to four genera and seven species were isolated from black seeds of ten different plant taxa including: Aspergillus amstelodami AUMC14827 from O. basilicum; A. niger AUMC14825 and 14829 from A. ampeloprasum and N. sativa, respectively; A. versicolor AUMC14828 from P. nigrum; C. madrasense AUMC14830 from P. oleracea; Penicillium chrysogenum AUMC14822, 14831 and 14826 from A. cepa, P. maritimum, and P. somniferum, respectively; P. citrinum AUMC14824 from A. retroflexus; and R. oryzae AUMC14823 from D. viscose (Table 1).
Identification of the most potent isolates was done by performing the ITS region sequencing, and the obtained sequences were further subjected to a BLAST search at NCBI database. The isolates were confirmed as A. niger TU 62 (GenBank accession no. OL411611), C. madrasense AUMC14830 (GenBank accession no. OL588256), and R. oryzae AUMC14823 (GenBank accession no. MZ723403) (Figure 1). Nucleotide comparisons of the ITS regions among Aspergillus strains and other similar strains retrieved from NCBI revealed 100% identity between A. niger TU 62 and each of A. niger MW186672 and A. niger MT729862 from GenBank. Moreover, C. madrasense AUMC14830 showed 99.12%–100% identity with several strains of C. madrasense, including the type strain CBS315.74 with GenBank accession no. NR_144834. At the same time, the sequenced R. oryzae AUMC14823 showed 100% identity and 100% coverage with several strains of R. oryzae, including the type material R. oryzae CBS 112.07 with GenBank accession no. 103595.
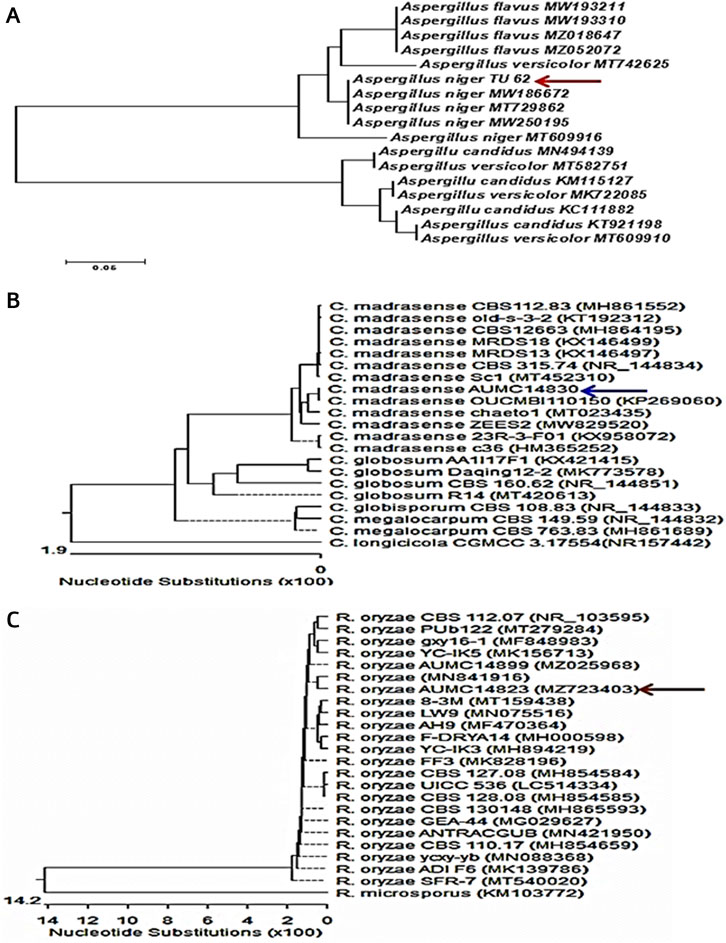
FIGURE 1. Neighbor-joining phylogenetic tree showing the genetic diversity of fungal isolates based on ITS sequences of tested fungi aligned with closely-related strains accessed from GenBank: (A) A. niger TU62; (B) C. madrasense AUMC14830; (C) R. oryzae AUMC14823.
3.2 Determination of Total Phenolics, Flavonoids, and Antioxidant Activity of Extracts
The present study aimed to determine the antioxidant activity of n-butanol, acetone, and methanol extracts obtained from ten black seed plants and their associated endophytic fungi. Total phenolics and flavonoids, which are closely correlated to antioxidant potency, were investigated in all extracts. The results indicated that butanolic extracts of both the seeds and their endophytic fungi had higher content of total phenolics, flavonoids, and antioxidant activity than acetonic and methanolic extracts except for O. basilicum, P. maritimum, and A. retroflexus along with their fungal endophytes. The highest content of phenolics in butanolic extracts was found in N. sativa and D. viscosa (28.50 and 26.51 mg/g, respectively), while their corresponding endophytes A. niger and R. oryzae contained 24.43and 24.40 mg/g, respectively. Elevated content of total flavonoids were recorded in the n-butanol extracts of N. sativa and P. oleracea (15.02 and 10.46 mg/g) and their associated endophytic A. niger and C. madrasense (11.45 and 9.23 mg/g), respectively. Moreover, N. sativa and its endophytic A. niger exhibited the best antioxidant activities (90.48 and 81.48%), followed by D. viscosa and its accompanying R. oryzae (89.21 and 81.71%), whereas P. oleracea and its partner C. madrasense got 81.42 and 78.55%, respectively, followed by P. somniferum and derived P. chrysogenum (Table 2). Regarding Pearson’s correlation of total phenolics, flavonoids, and the antioxidant activities of different organic solvent extracts from plant seeds and their derived fungi, positive correlations were revealed along with significant correlations between some parameters (Figure 2).
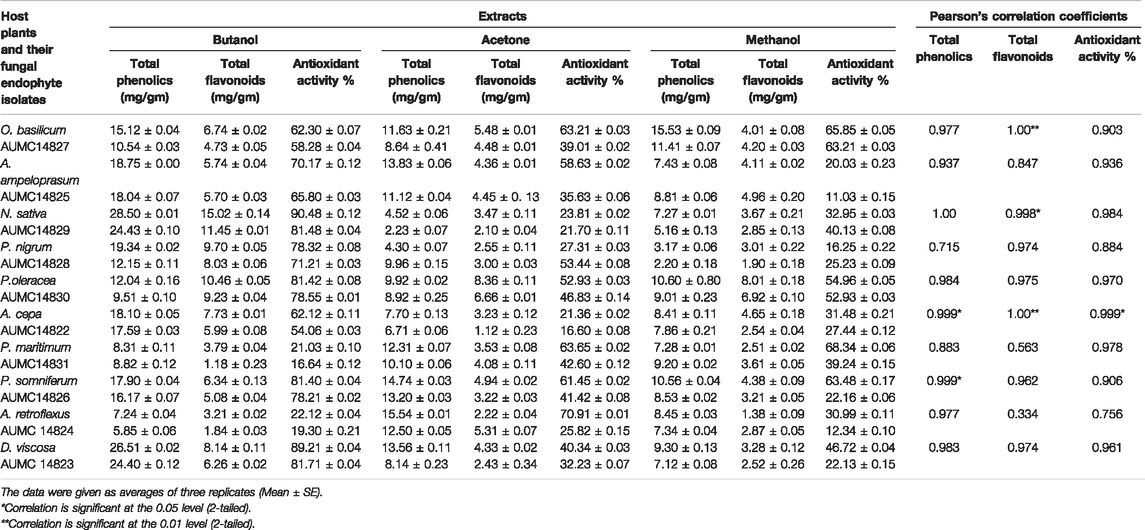
TABLE 2. Pearson’s correlation coefficients of total phenolics, flavonoids, and antioxidant activities by DPPH assay of n-butanol, acetone and methanol extracts from the investigated host, black seed plants, and their endophytic fungal isolates.
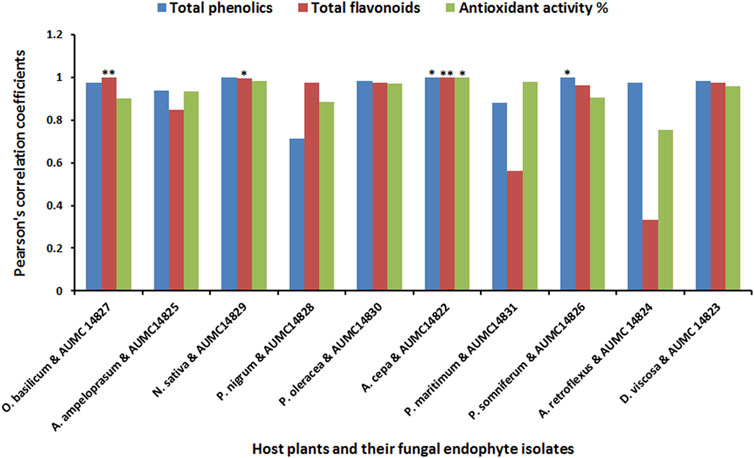
FIGURE 2. Pearson’s correlation coefficients of total phenolics, flavonoids, and the antioxidant activities of different extracts from black seed plants and their mycoendophytic isolates.
The IC50 values determined for n-butanol extracts showed the highest antioxidant potency of both the seeds and their companion endophytic fungi using DPPH, H2O2 and nitric oxide assays. The positive control (BHT) presented IC50 values of 0.34, 0.41, and 0.33 mg/ml for DPPH, H2O2, and nitric oxide radicals, respectively. The data presented in Table 3 report that assayed n-butanol extracts demonstrated significant IC50 values for N. sativa along with its endophyte A. niger, introducing IC50 values toward DPPH free radicals of 0.81 and 1.16 mg/ml, followed by IC50 values of D. viscosa and its accompanying R. oryzae (1.11 and 1.28 mg/ml), whereas P. oleracea and its partner C. madrasense got 1.16 and 1.31 mg/ml, respectively. IC50 values induced by N. sativa and A. niger against H2O2 were 1.03 and 1.31 mg/ml, followed by D. viscosa and R. oryzae (1.31 and 1.44 mg/ml), while P. oleracea and C. madrasense attained IC50 values of 1.44 and 1.48 mg/ml, respectively. Moreover, the N. sativa and A. niger butanol extract versus nitric oxide radicals achieved IC50 values of 0.81 and 1.33 mg/ml, tailed by P. oleracea and C. madrasense (1.30 and 1.36 mg/ml), and D. viscosa along with R. oryzae (1.33 and 1.44 mg/ml), respectively. When compared with BHT, the N. sativa extract presented good IC50 values for antiradical activity within all assays, followed by the rest of the extracts which showed reasonable values. Our results showed significant positive correlations between different antioxidant activity assays of n-butanol extracts from seeds and associated mycoendophytic strains.
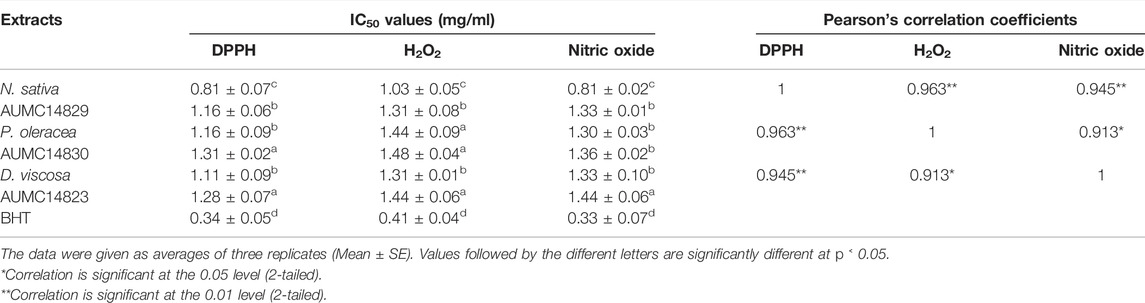
TABLE 3. IC50 values (mg/ml) and Pearson’s correlation coefficients of three antioxidant activity assays of butanol extracts from three black seed plants and associated endophytic fungal strains.
3.3 HPLC Analysis of Flavonoid and Phenolic Contents
HPLC analysis for identification of the chemical constituents of the n-butanol extract of N. sativa, P. oleracea, and D. viscosa, and their derived endophytic fungi (A. niger, C. madrasense, and R. oryzae, respectively) was performed to correlate their potential antioxidant activities with these chemical ingredients. Seventeen standard phenolics and flavonoids, namely gallic acid, chlorogenic acid, catechin, methyl gallate, caffeic acid, syringic acid, pyrocatechol, rutin, ellagic acid, coumaric acid, vanillin, ferulic acid, naringenin, querectin, cinnamic acid, kaempferol, and hesperetin, were used in our study and the detected compounds are illustrated in Figure 3. The HPLC analysis results (Table 4; Figures 4A–F) depict the presence of varying phenolics and flavonoid amounts based on the above-mentioned standards in the tested extracts.
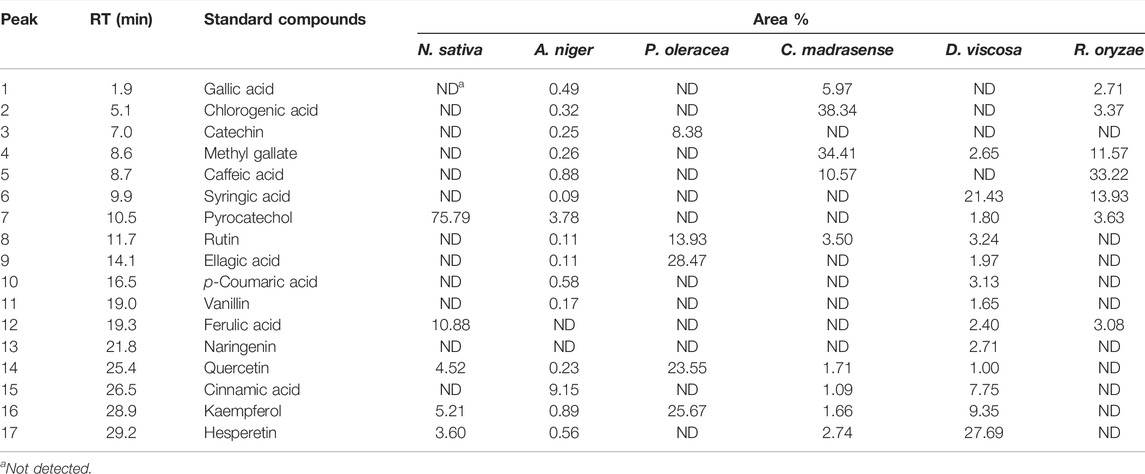
TABLE 4. Retention time (Rt), and area percentage of detected phenolic and flavonoid compounds derived from selected black seeds and their associated fungi.
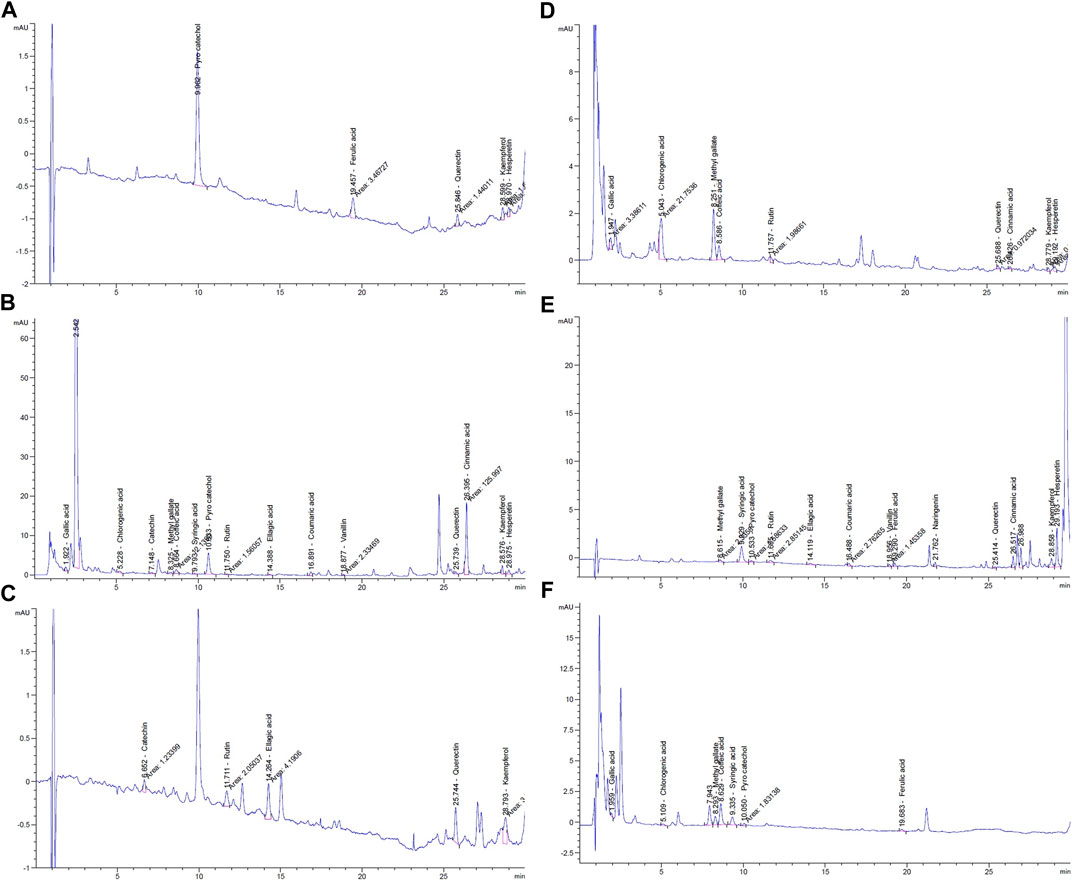
FIGURE 4. HPLC chromatograms of phenolic components in n-butanol extracts from: (A) N. sativa seeds; (B) endophytic fungus A. niger; (C) P. oleracea seeds; (D) endophytic fungus C. madrasense; (E) D. viscosa seeds; (F) endophytic fungus R. oryzae.
In this experiment, the N. sativa extract revealed the presence of pyrocatechol as a major compound (75.79%), reported for the first time in N. sativa, while its endophytic fungus A. niger showed its presence in a small amount (3.78%) and cinnamic acid was the predominant compound in the A. niger extract. As presented in Table 4, a total of five polyphenols were identified from seeds of P. oleracea using HPLC, namely; catechin, rutin, ellagic acid, quercetin, and kaempferol. The chemical analysis revealed the presence of ellagic acid 28.47%, kaempferol (25.67%), and quercetin (23.55%) as the main components, whereas chlorogenic acid (38.34%) and methyl gallate (34.41%) were found in C. madrasense endophyte and not detected in the host plant, indicating that it may be produced through biotransformation of host metabolites. On the other hand, methyl gallate, syringic acid, pyrocatechol, and ferulic acid were found in different proportions in both extracts of D. viscosa and its derived endophytic fungus R. oryzae. Hesperetin and syringic acid were the major detected compounds (27.69 and 21.43%, respectively) in the D. viscosa butanolic extract, while the endophytic fungi R. oryzae extract showed the presence of caffeic acid (33.22%) as a major compound not detected in the host plant.
3.4 In Silico Molecular Docking of Identified Compounds
According to the HPLC analysis, the different extracts of host plants and their endophytic fungi contained 17 polyphenolic compounds (Table 4). The docking studies for those compounds were analyzed to determine their binding affinities to target protein as antioxidants. Myeloperoxidase is an enzyme that catalyzes the formation of reactive oxygen intermediates and the generation of reactive nitrating and halogenating agents. It acts as a local tissue damage mediator in many inflammatory diseases. The investigation of molecular simulation results of detected phytomolecules against myeloperoxidase enzymatic protein (1DNU chain A & C) revealed that these compounds possess various affinities with the enzyme for acting as inhibitors, ranging from −15.3184 kcal/mol (rutin) to −6.1162 kcal/mol (vanillin) (Table 5). Therefore, they could have antioxidant effect by preventing reactive species release. The top scoring compounds were rutin, chlorogenic acid, quercetin, and kaempferol with pose scores of −15.3184 (RMSD = 0.99 Å), −12.1866 (RMSD = 0.64 Å), −10.6613 (RMSD = 0.76 Å), and −10.6037 (RMSD = 0.70 Å) kcal/mol, respectively.
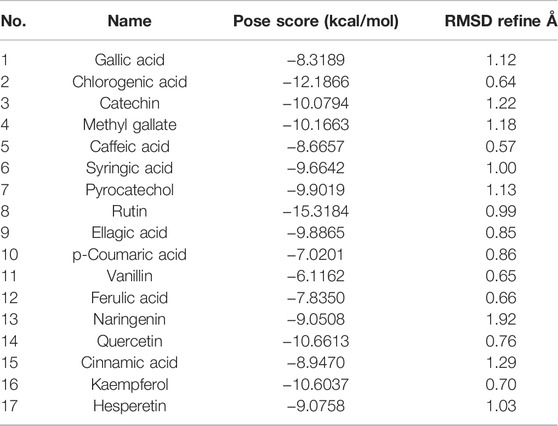
TABLE 5. Pose score results of detected compound interactions with myeloperoxidase antioxidant target proteins.
The interactions of the highest affinity compound (rutin) showed the presence of hydrogen bond interaction as H-donar with Glu A102 and Asp A98 and H-acceptor with His C 336 amino acid residues (Figure 5). In addition, the 2D and 3D interaction models showed the involvement of hydrophobic interactions with Phe A99, Phe C366, Phe C407, Leu C406, Leu C415, Leu C416, and Leu C420 amino acid residues.
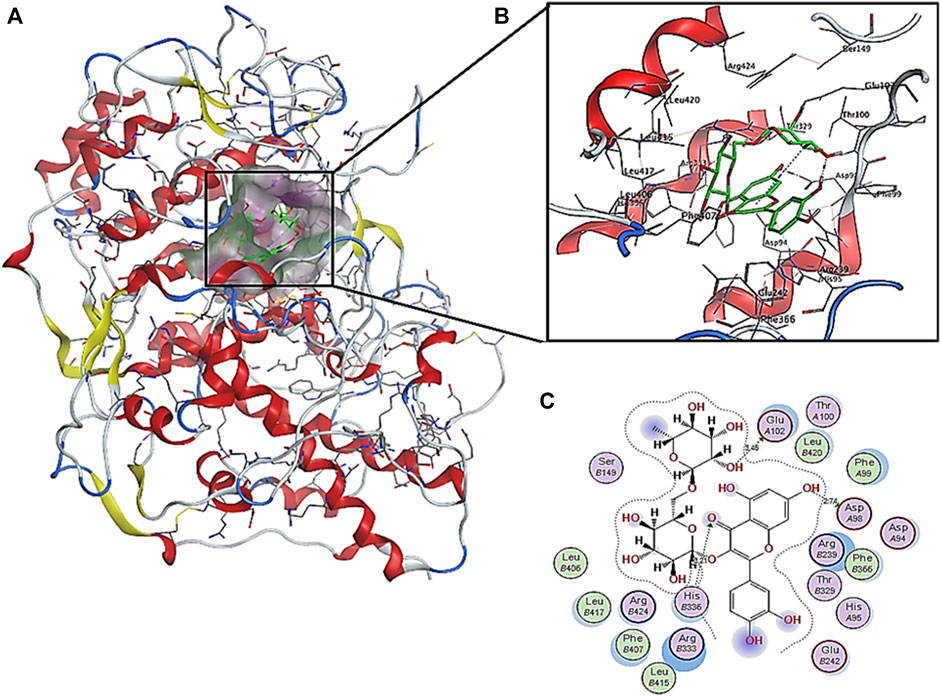
FIGURE 5. Predicted binding pose interactions with the residues of myeloperoxidase antioxidant target proteins: (A) whole protein binding site; (B) 3D interaction model; (C) 2D interaction model.
4 Discussion
Oxidative stress has been implicated in the development and prognosis of multiple diseases. To avoid oxidative stress, exogenous natural supplements can help to support the demand for endogenous antioxidants (Suleria et al., 2015). Mycoendophytes are well known for producing a variety of valuable bioactive by-products as well as their host plant. The majority of available synthesized antioxidants have been considered carcinogenic and cause liver injury (Yuan et al., 2008). By contrast, natural antioxidants produced by plants and their fungal endophytes, have not been detrimental (Caicedo et al., 2019). Polyphenols have hydroxyl groups and play a vital role in antioxidation, scavenging free radicals, and other pharmacological activities (Gangwar et al., 2014). Therefore, the discovery of polyphenol-producing endophytic fungi could help meet the needs of the pharmaceutical and food industry (Tang et al., 2021).
In the current study, ten dominant fungal endophytes were derived from black seeds belonging to ten different plant taxa. These isolates were A. amstelodami from O. basilicum, A. niger from A. ampeloprasum and N. sativa, A. versicolor from P. nigrum, C. madrasense from P. oleracea, P. chrysogenum from A. cepa, P. maritimum and P. somniferum, P. citrinum from A. retroflexus, and R. oryzae from D. viscose. Our results were in agreement with Metwaly et al. (2019), who isolated Aspergillus tubingensis which belongs to section Nigri, from N. sativa seeds. Similarly, Mandlaa et al. (2019) isolated endophytic C. globosum from P. oleracea roots. Metwaly et al. (2019) derived A. parasiticus, Eurotium pseudoglaucus, and Alternaria pluriseptata from N. sativa seeds, while Penicillium, Alternaria, and Cladosporium endophytic genera were identified by Gopane et al. (2021) from N. sativa seeds. Moreover, endophytic Purpureocillium lilacinum was obtained from seeds of P. somniferum (Pandey et al., 2016), and endophytic Alternaria spp. was recovered from Amaranthus hybridus seeds (Blodjett et al., 2000). However, Sreeja et al. (2019) reported that the endocarp of black pepper seed is free from fungal endophytes.
The most potent anti-free radical isolates were identified as A. niger TU 62, C. madrasense AUMC14830, and R. oryzae AUMC14823 by utilizing the ITS region sequencing. The ITS locus is a mostly credible region for identifying strains at species level. Molecular identification of Aspergillus strains to species level by ITS region sequencing was recently introduced as an effective alternative method for their accurate identification (Mazrou et al., 2020).
The present results proved that the obtained n-butanol extracts of both black seeds and their derived-mycoendophytes had higher content of total phenolics, flavonoids, and antioxidant capacity than acetone and methanol extracts, except O. basilicum, P. maritimum, and A. retroflexus, along with their fungal endophytes. The function of an antioxidant is to intercept and react with free radicals at a rate faster than the substrate (Garcia-Estan, 2019). The reduction activity of phenolic and flavonoid compounds depends on the number of free hydroxyl groups in the molecular structure, which would be strengthened by steric hindrance (Saxena et al., 2012; Platzer et al., 2022). The alcoholic extract of P. oleracea seeds exhibited good antiradical efficiency, and can ameliorate the H2O2 that induces death in hepatic cells (Mousavi et al., 2015; Al-Sheddi et al., 2016). Furthermore, Guo et al. (2016) reported that the oil from P. oleracea seed oil can be utilized as an alternate to synthetic antioxidants in food preservation and also as an ingredient in cosmetics. At the same time, Ercisli et al. (2008) and Alam et al. (2014) reported a potent antiradical activity of purslane leaves’ methanolic extract.
Our study found positive correlations as well as significant correlations between total phenolics, flavonoids, and the antioxidant activities of different organic solvent extracts from plant seeds and their derived mycoendophytes. In the same context, extraction of endophytic P. oxalicum, Simplilium sp., and Colletotrichum sp. culture filtrates derived from Ligusticum chuanxiong using different solvents (ethyl acetate, n-butanol, petroleum ether, and chloroform) showed a variation in total phenolic contents. P. oxalicum and Simplicillium sp. ethyl acetate extract was the most effective solvent for polyphenol extraction (94.58 and 58.64 mg GAE/g, respectively), while Colletotrichum sp. n-butanol was the more effective solvent for the extraction of polyphenols with 58.96 GAE/g polyphenols. In addition, antioxidant activities seemed to be correlated with total phenolic content (Tang et al., 2021). On the other hand, the methanolic extract of Cochliobolus sp. AL24 endophytic fungus from Aerva lanata exerts potent antioxidant effect, while a moderate activity was found in the dichloromethane extract followed by the butanol extract. The antioxidant activities were proportionally correlated with their total phenolic and flavonoid contents (Shoba and Sathiavelu, 2018). Conversely, Lim et al. (2021) found incompatible correlation between antioxidant potency and phenolic content.
Polar and medium polarity solvents are more favorable to the extraction of low and high molecular weight polyphenols in endophytic fungus fermentation broth than nonpolar solvents (Tang et al., 2021). Successive n-butanol fraction, viz., n-hexane, chloroform and ethyl acetate fractions obtained from the Trichoderma viride culture filtrate proved the most effective in retarding the growth of pathogenic Alternaria alternata afforded by n-butanol fraction. When these organic solvents were successively employed, organic compounds from fungal metabolites with different polarities were dissolved in different solvents and thus became separated. The disparity in bioactivity of extracts in different solvents may be ascribed to the different chemical nature of the solvents. It is the dissolution of different types of chemicals in different solvents that ensured the variable activity of metabolites of the same source in different solvents (Shafique et al., 2019). The antioxidant activity for the n-butanol and ethyl acetate extracts of Senna italic against the ABTS radical was 85.7 and 82.9%, respectively, compared to ascorbic acid with 89.2% inhibition. The structure elucidation of the isolated compounds from the n-butanol extract showed the presence of quercetin and rutin (Khalaf et al., 2019). Alkaloids and flavonoids present in high concentration in the n-butanol extract of Apium graveolens seeds act as an antioxidant in streptozotocin-induced diabetic mature male rats (Middleton et al., 2000; Al-Saaidi et al., 2012).
The IC50 values were determined for n-butanol extracts and showed the highest antioxidant potency of both seeds and their companion endophytic fungi using DPPH, H2O2 and nitric oxide assays. Our results showed significant positive correlations between different antioxidant activity assays of n-butanol extracts from plant seeds and associated mycoendophytic strains. Endophytic A. tubingensis from N. sativa seeds revealed antioxidant activity with IC50 value of 8 μg/ml determined by the DCFH-DA (20,70-dichlorofluorescein diacetate) method in myelomonocytic HL-60 cells (Metwaly et al., 2019). The ethanol extract of P. maritimum fruits showed an IC50 value of 6.9
According to the HPLC analysis, the different extracts of host plants and their endophytic fungi contained 17 polyphenolic compounds. The N. sativa extract revealed the presence of pyrocatechol as a major compound (75.79%), reported for the first time in N. sativa, while its endophytic fungus A. niger showed the presence of catechol in a small amount (3.78%), and cinnamic acid was the predominant compound in the A. niger extract. A HPLC–UV–MS study by Toma et al. (2015) reported the presence of ferulic acid, quercetin, and kaempferol in the N. sativa extract which supports our results. The chemical analysis revealed the presence of ellagic acid 28.47%, kaempferol (25.67%), and quercetin (23.55%) as main components beside rutin and catechin for the first time from the extract of P. oleracea. Chlorogenic acid (38.34%) and methyl gallate (34.41%) were found in C. madrasense endophyte and not detected in the host plant, indicating that these may be produced through biotransformation of host metabolites. Kaempferol and apigenin were previously reported from leaf and stem, while luteolin, myricetin and quercetin were isolated from the whole P. oleracea plant (Zhou et al., 2015). Salucci et al. (2002) reported that dietary flavonoids like epicatechin, galate, gallic acid, and quercetin-3-glucoside exhibit strong antioxidant activity. The dietary flavonol, quercetin, is a potent antioxidant because it has all the right structural features for free radical scavenging activity (Formica and Regelson, 1995). Quercetin, kaempferol, morin, myricetin and rutin, by acting as antioxidants, exhibited beneficial effects such as anti-inflammatory, antiallergic, antiviral, as well as anticancer activity (Saxena et al., 2012).
Our results revealed that hesperetin and syringic acid were the major detected compounds (27.69 and 21.43%, respectively) in the D. viscosa n-butanol extract, while the endophytic fungi R. oryzae extract showed the presence of caffeic acid (33.22%) as a major compound, which was not detected in the host plant. Previous studies on D. viscosa reported the presence of alkaloids, flavonoids, phenols, steroids, glycosidic cyanide, saponins and tannins in which flavonoids and phenols were the major components in accordance with our results (Alzandi et al., 2021). Ghisalberti (1998) identified 23 flavones from seeds, bark, flowers and leaves of D. viscosa, characterized at c-3 and in almost 50% of cases, methoxylation at c-6. It is noteworthy that plant-endophyte metabolisms can interact by inducing each other’s metabolisms or metabolizing secondary compounds from each other via different biosynthetic and biotransformation pathways (Ludwig-Muller, 2015). These reports could explain the variance between the detected compounds in the host plant and their derived mycoendophytes in our study.
The binding interaction and conformation of each phytocompound with each target protein was ranked based on lowest energy and lowest RMSD, respectively, according to previous studies that indicated that the lower the binding energy score found, the better the protein–ligand binding stability (Imana et al., 2020). The investigation of molecular simulation results of detected phytomolecules against myeloperoxidase enzymatic protein (1DNU chain A & C) revealed that rutin possess high affinity for the enzyme (−15.3184 kcal/mol) to act as inhibitor. Rutin (7 ± 1 mg/kg dw) was determined in A. retroflexus seeds (Kalinova and Dadakova, 2009). Rutin is a flavonol that has exhibited a variety of pharmacological efficacies including antioxidant, cytoprotective, anticarcinogenic, neuroprotective, and cardioprotective activities (Ganeshpurkar and Saluja, 2017; Enogieru et al., 2018). Flavonoids have the hydroxyl groups and functional substitutions that provide their bioactivity and have an important role in non-covalent interactions such as electrostatic interactions, hydrogen bonds, van der Waals interactions, and hydrophobic interactions with their targets in drug-enzyme interactions and are critical in drug design (Comakli et al., 2020). Phenolic structures often have the potential to strongly interact with proteins, due to their hydrophobic benzenoid rings and the hydrogen-bonding potential of the phenolic hydroxyl groups. This gives phenolics the ability to act as antioxidants also by virtue of their capacity to inhibit some enzymes involved in radical generation (Pereira et al., 2009).
5 Conclusion
In this study, ten mycoendophytes associated with black seed plants, and their n-butanol, acetone, and methanol crude extracts, demonstrated different levels of total phenolics and flavonoids, in addition to antiradical bioactivity. A. niger TU 62, C. madrasense AUMC14830, and R. oryzae AUMC14823 derived from N. sativa, P. oleracea, and D. viscosa seeds, respectively, as well as the n-butanol extracts, showed the highest antiradical potency. Moreover, assayed n-butanol extracts demonstrated significant IC50 values as well as significant positive correlations between different antioxidant activity assays. In HPLC analysis of the most antiradical efficient n-butanol extracts, multiple phenolic and flavonoid compounds were detected in different degrees of existence and concentration. Furthermore, molecular docking of the investigated molecules demonstrated that rutin held high affinity against myeloperoxidase and could act as an inhibitor. Concerning the obtained results, the mycoendophytes associated with black seed plants might be deemed valuable sources for the high-scale industrial production of antioxidants, given their virtue therapeutic prospects and lack of any adverse effects.
Data Availability Statement
The raw data supporting the conclusion of this article will be made available by the authors, without undue reservation.
Author Contributions
Conceptualization, AH and MA; methodology, SH, MFA, and TT; software, MA and MH; validation, AH and HM; formal analysis, MA; investigation, AH and HM; resources, SH, MFA, TT, and HM; data curation, SH, MFA, and TT; writing—original draft preparation, HM, SH, MFA, and TT; writing—review and editing, AH and MA; visualization, AE, MHH, and NA; supervision, AH and MA; project administration, AH; funding acquisition, null. All authors have read and agreed to the published version of the manuscript.
Conflict of Interest
The authors declare that the research was conducted in the absence of any commercial or financial relationships that could be construed as a potential conflict of interest.
Publisher’s Note
All claims expressed in this article are solely those of the authors and do not necessarily represent those of their affiliated organizations, or those of the publisher, the editors and the reviewers. Any product that may be evaluated in this article, or claim that may be made by its manufacturer, is not guaranteed or endorsed by the publisher.
References
Abdel-Aziz, A.-W., Elwan, N., Abdallah, m., Shaaban, R., Osman, N., and Mohamed, M. (2021). High-Performance Liquid Chromatography-Fingerprint Analyses, In Vitro Cytotoxicity, Antimicrobial and Antioxidant Activities of the Extracts of Ceiba Speciosa Growing in Egypt. Egypt. J. Chem. 64 (4), 2–3. doi:10.21608/EJCHEM.2021.58716.3267
Abdulmyanova, L. I., Fayzieva, F. K., Ruzieva, D. M., Rasulova, G. A., Sattarova, R. S., and Gulyamova, T. G. (2016). Bioactivity of Fungal Endophytes Associating with Allium Plants Growing in Uzbekistan. Int. J. Curr. Microbiol. App. Sci. 5 (9), 769–778. doi:10.20546/ijcmas.2016.509.088
Abukawsar, M. M., Saleh‐e‐In, M. M., Ahsan, M. A., Rahim, M. M., Bhuiyan, M. N. H., Roy, S. K., et al. (2018). Chemical, Pharmacological and Nutritional Quality Assessment of Black Pepper (Piper nigrum L.) Seed Cultivars. J. Food Biochem. 42 (6), e12590. doi:10.1111/jfbc.12590
Al-Fatimi, M., Wurster, M., Schröder, G., and Lindequist, U. (2007). Antioxidant, Antimicrobial and Cytotoxic Activities of Selected Medicinal Plants from Yemen. J. Ethnopharmacol. 111 (3), 657–666. doi:10.1016/j.jep.2007.01.018
Al Mousa, A. A., Mohamed, H., Hassane, A. M. A., and Abo-Dahab, N. F. (2021). Antimicrobial and Cytotoxic Potential of an Endophytic Fungus Alternaria tenuissima AUMC14342 Isolated from Artemisia judaica L. Growing in Saudi Arabia. J. King Saud Univ. - Sci. 33, 101462. doi:10.1016/j.jksus.2021.101462
Al-Saaidi, A., Alrodhan, M. N. A., and Ismael, A. K. (2012). Antioxidant Activity of N-Butanol Extract of Celery (Apium graveolens) Seed in Streptozotocin-Induced Diabetic Male Rats. Res. Pharm. Biotech. 4 (2), 24–29. doi:10.5897/RPB12.002
Al-Sheddi, E. S., Farshori, N. N., Al-Oqail, M. M., Al- Massarani, S. M., Al Salem, A. M., Musarrat, J., et al. (2016). Portulaca oleracea Linn Seed Extract Ameliorates Hydrogen Peroxide-Induced Cell Death in Human Liver Cells by Inhibiting Reactive Oxygen Species Generation and Oxidative Stress. Trop. J. Pharm. Res. 15 (8), 1643–1649. doi:10.4314/tjpr.v15i8.7
Alam, M. A., Juraimi, A. S., Rafii, M. Y., Abdul Hamid, A., Aslani, F., Hasan, M. M., et al. (2014). Evaluation of Antioxidant Compounds, Antioxidant Activities, and Mineral Composition of 13 Collected Purslane (Portulaca oleracea L.) Accessions. BioMed Res. Int. 2014, 1–10. doi:10.1155/2014/296063
Ali, S. A., Parveen, N., and Ali, A. S. (2018). Links between the Prophet Muhammad (PBUH) Recommended Foods and Disease Management: A Review in the Light of Modern Superfoods. Int. J. Health Sci. (Qassim) 12 (2), 61–69.
Alzandi, A. A., Taher, E. A., Al-Sagheer, N. A., Al-Khulaidi, A. W., Azizi, M., and Naguib, D. M. (2021). Phytochemical Components, Antioxidant and Anticancer Activity of 18 Major Medicinal Plants in Albaha Region, Saudi Arabia. Biocatal. Agric. Biotechnol. 34, 102020. doi:10.1016/j.bcab.2021.102020
Amer, A. M. (2018). Antimicrobial Effects of Egyptian Local Chicory, Cichorium endivia subsp. pumilum. Int. J. Microbiol. 2018, 1–6. doi:10.1155/2018/6475072
Apak, R., Özyürek, M., Güçlü, K., and Çapanoğlu, E. (2016). Antioxidant Activity/capacity Measurement. 1. Classification, Physicochemical Principles, Mechanisms, and Electron Transfer (ET)-based Assays. J. Agric. Food Chem. 64 (5), 997–1027. doi:10.1021/acs.jafc.5b04739
Ashraf, S. S., Rao, M. V., Kaneez, F. S., Qadri, S., Al-Marzouqi, A. H., Chandranath, I. S., et al. (2011). Nigella sativa Extract as a Potent Antioxidant for Petrochemical-Induced Oxidative Stress. J. Chromatogr. Sci. 49 (4), 321–326. doi:10.1093/chrsci/49.4.321
Atiphasaworn, P., Monggoot, S., Gentekaki, E., Brooks, S., and Pripdeevech, P. (2017). Antibacterial and Antioxidant Constituents of Extracts of Endophytic Fungi Isolated from Ocimum basilicum Var. thyrsiflora Leaves. Curr. Microbiol. 74 (10), 1185–1193. doi:10.1007/s00284-017-1303-1
Blodgett, J. T., Swart, W. J., Louw, S. v., and Weeks, W. J. (2000). Species Composition of Endophytic Fungi in Amaranthus hybridus Leaves, Petioles, Stems, and Roots. Mycologia 92 (5), 853–859. doi:10.1080/00275514.2000.12061230
Brand-Williams, W., Cuvelier, M. E., and Berset, C. (1995). Use of a Free Radical Method to Evaluate Antioxidant Activity. LWT - Food Sci. Technol. 28 (1), 25–30. doi:10.1016/S0023-6438(95)80008-5
Caicedo, N. H., Davalos, A. F., Puente, P. A., Rodríguez, A. Y., and Caicedo, P. A. (2019). Antioxidant Activity of Exo‐metabolites Produced by Fusarium oxysporum: An Endophytic Fungus Isolated from Leaves of Otoba Gracilipes. MicrobiologyOpen 8 (10), e903. doi:10.1002/mbo3.903
Charmforoshan, E., Karimi, E., Oskoueian, E., Es-Haghi, A., and Iranshahi, M. (2019). Inhibition of Human Breast Cancer Cells (MCF-7 Cell Line) Growth via Cell Proliferation, Migration, and Angiogenesis by Auraptene of Ferula szowitsiana Root Extract. Food Meas. 13, 2644–2653. doi:10.1007/s11694-019-00185-6
Cimmino, A., Andolfi, A., Abouzeid, M., and Evidente, A. (2013). Polyphenols as Fungal Phytotoxins, Seed Germination Stimulants and Phytoalexins. Phytochem. Rev. 12 (4), 653–672. doi:10.1007/s11101-013-9277-5
Comakli, V., Adem, S., Oztekin, A., and Demirdag, R. (2020). Screening Inhibitory Effects of Selected Flavonoids on Human Recombinant Aldose Reductase Enzyme: In Vitro and In Silico Study. Archives Physiology Biochem. 28, 1–7. doi:10.1080/13813455.2020.1771377
de Torre, M. P., Cavero, R. Y., Calvo, M. I., and Vizmanos, J. L. (2019). A Simple and a Reliable Method to Quantify Antioxidant Activity In Vivo. Antioxidants 8 (5), 142. doi:10.3390/antiox8050142
Dincel, E. D., Gürsoy, E., Yilmaz-Ozden, T., and Ulusoy-Güzeldemirci, N. (2020). Antioxidant Activity of Novel Imidazo[2,1-B]thiazole Derivatives: Design, Synthesis, Biological Evaluation, Molecular Docking Study and In Silico ADME Prediction. Bioorg. Chem. 103, 104220. doi:10.1016/j.bioorg.2020.104220
Enogieru, A. B., Haylett, W., Hiss, D. C., Bardien, S., and Ekpo, O. E. (2018). Rutin as a Potent Antioxidant: Implications for Neurodegenerative Disorders. Oxidative Med. Cell. Longev. 2018 (1), 1–17. doi:10.1155/2018/6241017
Ercisli, S., Coruh, I., Gormez, A., and Sengul, M. (2008). Antioxidant and Antibacterial Activities of Portulaca oleracea L. Grown Wild in Turkey. Ital. J. Food Sci. 20 (4), 533–542.
Farag, R., and Swaby, S. (2018). Antimicrobial Effects of Wasp (Vespa orientalis) Venom. Egypt. Pharm. J. 17 (3), 218–222. doi:10.4103/epj.epj_39_18
Fernandes, M. d. R. V., Silva, T. A. C. e., Pfenning, L. H., Costa-Neto, C. M. d., Heinrich, T. A., Alencar, S. M. d., et al. (2009). Biological Activities of the Fermentation Extract of the Endophytic Fungus Alternaria alternata Isolated from Coffea Arabica L. Braz. J. Pharm. Sci. 45 (4), 677–685. doi:10.1590/S1984-82502.00900.0400010
Ferrazzano, G., Amato, I., Ingenito, A., Zarrelli, A., Pinto, G., and Pollio, A. (2011). Plant Polyphenols and Their Anti-cariogenic Properties: A Review. Molecules 16 (2), 1486–1507. doi:10.3390/molecules16021486
Formica, J. V., and Regelson, W. (1995). Review of the Biology of Quercetin and Related Bioflavonoids. Food Chem. Toxicol. 33 (12), 1061–1080. doi:10.1016/0278-6915(95)00077-1
Gabr, S. K., Bakr, R. O., Mostafa, E. S., El-Fishawy, A. M., and El-Alfy, T. S. (2019). Antioxidant Activity and Molecular Docking Study of Erythrina × Neillii Polyphenolics. South Afr. J. Bot. 121, 470–477. doi:10.1016/j.sajb.2018.12.011
Ganeshpurkar, A., and Saluja, A. K. (2017). The Pharmacological Potential of Rutin. Saudi Pharm. J. 25 (2), 149–164. doi:10.1016/j.jsps.2016.04.025
Gangwar, M., Gautam, M. K., Sharma, A. K., Tripathi, Y. B., Goel, R. K., and Nath, G. (2014). Antioxidant Capacity and Radical Scavenging Effect of Polyphenol Rich Mallotus philippenensis Fruit Extract on Human Erythrocytes: An In Vitro Study. Sci. World J. 2014, 1–12. doi:10.1155/2014/279451
Geisen, S., Kostenko, O., Cnossen, M. C., ten Hooven, F. C., Vreš, B., and van der Putten, W. H. (2017). Seed and Root Endophytic Fungi in a Range Expanding and a Related Plant Species. Front. Microbiol. 8, 1645. doi:10.3389/fmicb.2017.01645
Ghisalberti, E. L. (1998). Ethnopharmacology and Phytochemistry of Dodonaea Species. Fitoterapia 69 (2), 99–113.
Giuseppe Rizzello, C., Coda, R., De Angelis, M., Di Cagno, R., Carnevali, P., and Gobbetti, M. (2009). Long-term Fungal Inhibitory Activity of Water-Soluble Extract from Amaranthus spp. Seeds during Storage of Gluten-free and Wheat Flour Breads. Int. J. Food Microbiol. 131 (2-3), 189–196. doi:10.1016/j.ijfoodmicro.2009.02.025
Gopane, B., Kaptchouang Tchatchouang, C. D., Regnier, T., Ateba, C. N., and Manganyi, M. C. (2021). Community Diversity and Stress Tolerance of Culturable Endophytic Fungi from Black Seed (Nigella sativa L.). South Afr. J. Bot. 137, 272–277. doi:10.1016/j.sajb.2020.10.026
Gunatilaka, A. A. L. (2006). Natural Products from Plant-Associated Microorganisms: Distribution, Structural Diversity, Bioactivity, and Implications of Their Occurrence. J. Nat. Prod. 69 (3), 509–526. doi:10.1021/np058128n
Guo, G., Yue, L., Fan, S., Jing, S., and Yan, L. (2016). Antioxidant and Antiproliferative Activities of Purslane Seed Oil. J. Hypertens. (Los Angel) 05 (2), 218. doi:10.4172/2167-1095.1000218
Hassan, M. M., Farid, M. A., and Gaber, A. (2019). Rapid Identification of Trichoderma koningiopsis and Trichoderma longibrachiatum Using Sequence-Characterized Amplified Region Markers. Egypt. J. Biol. Pest control. 29, 13. doi:10.1186/s41938-019-0113-0
Imana, S. N., Ningsih, E. G., and Tambunan, U. S. F. (2020). In Silico identification of Peptide as Epidermal Growth Factor Receptor Tyrosine Kinase Inhibitors in Lung Cancer Treatment. Pak. J. Biol. Sci. Biol. Sci. 23 (4), 567–574. doi:10.3923/pjbs.2020.567.574
Ishtiaq, S., Ashraf, M., Hayat, M. Q., and Asrar, M. (2013). Phytochemical Analysis of Nigella sativa and its Antibacterial Activity against Clinical Isolates Identified by Ribotyping. Int. J. Agric. Biol. 15 (6), 1151–1156.
Jung, J.-W., Kim, N.-J., Yun, H., and Han, Y. (2018). Recent Advances in Synthesis of 4-Arylcoumarins. Molecules 23, 2417. doi:10.3390/molecules23102417
Kalinova, J., and Dadakova, E. (2009). Rutin and Total Quercetin Content in Amaranth (Amaranthus spp.). Plant Foods Hum. Nutr. 64, 68–74. doi:10.1007/s11130-008-0104-x
Khadem, S., and Marles, R. J. (2010). Monocyclic Phenolic Acids; Hydroxy- and Polyhydroxybenzoic Acids: Occurrence and Recent Bioactivity Studies. Molecules 15 (11), 7985–8005. doi:10.3390/molecules15117985
Khalaf, O. M., Ghareeb, M. A., Saad, A. M., Madkour, H. M. F., El-Ziaty, A. K., and Abdel-Aziz, M. S. (2019). Phenolic Constituents, Antimicrobial, Antioxidant, and Anticancer Activities of Ethyl Acetate and N-Butanol Extracts of Senna italica. Acta Chromatogr. 31 (2), 138–145. doi:10.1556/1326.2018.00412
Khalil, S. R., Elhakim, Y. A., Abd El-fattah, A. H., Ragab Farag, M., Abd El-Hameed, N. E., and El-Murr, A. E. (2020). Dual Immunological and Oxidative Responses in Oreochromis niloticus Fish Exposed to Lambda Cyhalothrin and Concurrently Fed with Thyme Powder (Thymus vulgaris L.): Stress and Immune Encoding Gene Expression. Fish Shellfish Immunol. 100, 208–218. doi:10.1016/j.fsi.2020.03.009
Kongpichitchoke, T., Chiu, M.-T., Huang, T.-C., and Hsu, J.-L. (2016). Gallic Acid Content in Taiwanese Teas at Different Degrees of Fermentation and its Antioxidant Activity by Inhibiting PKCδ Activation: In Vitro and In Silico Studies. Molecules 21, 1346. doi:10.3390/molecules21101346
Leporini, M., Catinella, G., Bruno, M., Falco, T., Tundis, R., and Loizzo, M. R. (2018). Investigating the Antiproliferative and Antioxidant Properties of Pancratium maritimum L. (Amaryllidaceae) Stems, Flowers, Bulbs, and Fruits Extracts. Evidence-Based Complementary Altern. Med. 2018, 9301247. doi:10.1155/2018/9301247
Lim, S. M., Agatonovic-Kustrin, S., Lim, F. T., and Ramasamy, K. (2021). High-performance Thin Layer Chromatography-Based Phytochemical and Bioactivity Characterisation of Anticancer Endophytic Fungal Extracts Derived from Marine Plants. J. Pharm. Biomed. Analysis 193, 113702. doi:10.1016/j.jpba.2020.113702
López, J. G.-E. (2019). Flavonoids in Health and Disease. Cmc 26 (39), 6972–6975. doi:10.2174/092986732639191213095405
Ludwig-Müller, J. (2015). Plants and Endophytes: Equal Partners in Secondary Metabolite Production? Biotechnol. Lett. 37 (7), 1325–1334. doi:10.1007/s10529-015-1814-4
Magiera, S., Baranowska, I., and Lautenszleger, A. (2015). UHPLC-UV Method for the Determination of Flavonoids in Dietary Supplements and for Evaluation of Their Antioxidant Activities. J. Pharm. Biomed. Analysis 102, 468–475. doi:10.1016/j.jpba.2014.10.004
Mazrou, Y. S., Makhlouf, A. H., Makhlouf, A. H., Elbealy, E. R., Salem, M. A., Farid, M. A., et al. (2020). Molecular Characterization of Phosphate Solubilizing Fungi Aspergillus niger and its Correlation to Sustainable Agriculture. Jeb 41 (3), 592–599. doi:10.22438/jeb/41/3/MRN-1298
Meenatchi, A., Ramesh, V., Bagyalakshmi, K. R., Shanmugaiah, V., and Rajendran, A. (2016). Diversity of Endophytic Fungi from the Ornamental Plant - Adenium obesum. Stud. Fungi 1, 34–42. doi:10.5943/sif/1/1/3
Metwaly, A., Ashour, M. A., Khan, S., Ma, G., Kadry, H. A., El-Hela, A. A., et al. (2019). Comparative Biological Evaluation of Four Endophytic Fungi Isolated from Nigella sativa Seeds. Al-Azhar J. Pharm. Sci. 59 (1), 123–136. doi:10.21608/ajps.2019.64111
Middleton, E., Kandaswami, C., and Theoharides, T. C. (2000). The Effects of Plant Flavonoids on Mammalian Cells: Implications for Inflammation, Heart Disease, and Cancer. Pharmacol. Rev. 52 (4), 673–751.
Mohamed, E., Kasem, A. M. M., and Farghali, K. A. (2018). Seed Germination of Egyptian Pancratium Maritimum under Salinity with Regard to Cytology, Antioxidant and Reserve Mobilization Enzymes, and Seed Anatomy. Flora 242, 120–127. doi:10.1016/j.flora.2018.03.011
Mohamed, H., El-Shanawany, A.-R., Shah, A. M., Nazir, Y., Naz, T., Ullah, S., et al. (2020). Comparative Analysis of Different Isolated Oleaginous Mucoromycota Fungi for Their γ-Linolenic Acid and Carotenoid Production. BioMed Res. Int. 2020, 3621543. doi:10.1155/2020/3621543
Mohamed, H., Hassane, A., Atta, O., and Song, Y. (2021). Deep Learning Strategies for Active Secondary Metabolites Biosynthesis from Fungi: Harnessing Artificial Manipulation and Application. Biocatal. Agric. Biotechnol. 38, 102195. doi:10.1016/j.bcab.2021.102195
Mousavi, S. R. J., Niazmand, R., and Shahidi, M. S. (2015). Antioxidant Activity of Purslane (Portulaca oleracea L.) Seed Hydro-Alcoholic Extract on the Stability of Soybean Oil. J. Agr. Sci. Tech. 17 (6), 1473–1480.
Munteanu, I. G., and Apetrei, C. (2021). Analytical Methods Used in Determining Antioxidant Activity: A Review. Ijms 22 (7), 3380. doi:10.3390/ijms22073380
Pandey, S. S., Singh, S., Babu, C. S. V., Shanker, K., Srivastava, N. K., and Kalra, A. (2016). Endophytes of Opium Poppy Differentially Modulate Host Plant Productivity and Genes for the Biosynthetic Pathway of Benzylisoquinoline Alkaloids. Planta 243 (5), 1097–1114. doi:10.1007/s00425-016-2467-9
Pereira, D., Valentão, P., Pereira, J., and Andrade, P. (2009). Phenolics: From Chemistry to Biology. Molecules 14, 2202–2211. doi:10.3390/molecules14062202
Platzer, M., Kiese, S., Tybussek, T., Herfellner, T., Schneider, F., Schweiggert-Weisz, U., et al. (2022). Radical Scavenging Mechanisms of Phenolic Compounds: A Quantitative Structure-Property Relationship (QSPR) Study. Front. Nutr. 9, 882458. doi:10.3389/fnut.2022.882458
Quettier-Deleu, C., Gressier, B., Vasseur, J., Dine, T., Brunet, C., Luyckx, M., et al. (2000). Phenolic Compounds and Antioxidant Activities of Buckwheat (Fagopyrum esculentum Moench) Hulls and Flour. J. Ethnopharmacol. 72 (1-2), 35–42. doi:10.1016/S0378-8741(00)00196-3
Ramesha, A., Venkataramana, M., Nirmaladevi, D., Gupta, V. K., Chandranayaka, S., and Srinivas, C. (2015). Cytotoxic Effects of Oosporein Isolated from Endophytic Fungus Cochliobolus kusanoi. Front. Microbiol. 6, 870. doi:10.3389/fmicb.2015.00870
Rasul Suleria, H. A., Sadiq Butt, M., Muhammad Anjum, F., Saeed, F., Batool, R., and Nisar Ahmad, A. (2012). Aqueous Garlic Extract and its Phytochemical Profile; Special Reference to Antioxidant Status. Int. J. Food Sci. Nutr. 63 (4), 431–439. doi:10.3109/09637486.2011.634786
Rehman, H. u., Rehman, H., Khurshid, A., and Ijaz, B. (2020). Biological Screening of Endophytic Fungi Extracted from Different Parts of Medicinal Plants. Pab 9 (1), 989–998. doi:10.19045/bspab.2020.90104
Ruch, R. J., Cheng, S.-j., and Klaunig, J. E. (1989). Prevention of Cytotoxicity and Inhibition of Intercellular Communication by Antioxidant Catechins Isolated from Chinese Green Tea. Carcinogenesis 10 (6), 1003–1008. doi:10.1093/carcin/10.6.1003
Sabra, S. M. M., and Ismail, A. B. M. (2019). Habak Al-Madinah Al-Munawarah"; (Ocimum basilicum) Endophytic-Ability against Pathogenic-Bacteria Proven Successuse-Constantly in Saudi-society, Taif, KSA. Int. J. Res. Stud. Biosci. 7 (10), 26–30. doi:10.20431/2349-0365.0710004
Sajjadi, M., Karimi, E., Oskoueian, E., Iranshahi, M., and Neamati, A. (2019). Galbanic Acid: Induced Antiproliferation in Estrogen Receptor‐negative Breast Cancer Cells and Enhanced Cellular Redox State in the Human Dermal Fibroblasts. J. Biochem. Mol. Toxicol. 33, e22402. doi:10.1002/jbt.22402
Salucci, M., Stivala, L. A., Maiani, G., Bugianesi, R., and Vannini, V. (2002). Flavonoids Uptake and Their Effect on Cell Cycle of Human Colon Adenocarcinoma Cells (Caco2). Br. J. Cancer 86 (10), 1645–1651. doi:10.1038/sj.bjc.6600295
Saxena, M., Saxena, J., and Pradhan, A. (2012). Flavonoids and Phenolic Acids as Antioxidants in Plants and Human Health. Int. J. Pharm. Sci. Rev. Res. 16 (2), 130–134.
Schulz, B., Boyle, C., Draeger, S., Römmert, A.-K., and Krohn, K. (2002). Endophytic Fungi: A Source of Novel Biologically Active Secondary Metabolites. Mycol. Res. 106 (9), 996–1004. doi:10.1017/S0953.75620.2006342
Shafique, S., Shafique, S., Javed, A., Akhtar, N., and Bibi, S. (2019). Analysis of Antagonistic Potential of Secondary Metabolites and Organic Fractions of Trichoderma Species against Alternaria alternata. Biocontrol Sci. 24 (2), 81–88. doi:10.4265/bio.24.81
Shahidi, F., and Ambigaipalan, P. (2015). Phenolics and Polyphenolics in Foods, Beverages and Spices: Antioxidant Activity and Health Effects - A Review. J. Funct. Foods 18 (B), 820–897. doi:10.1016/j.jff.2015.06.018
Sharma, D., Pramanik, A., and Agrawal, P. K. (2016). Evaluation of Bioactive Secondary Metabolites from Endophytic Fungus Pestalotiopsis neglecta BAB-5510 Isolated from Leaves of Cupressus Torulosa D.Don. 3 Biotech. 6 (2), 210. doi:10.1007/s13205-016-0518-3
Shoba, S., and Sathiavelu, M. (2018). Biological Activities of Endophytic Fungus Cochliobolus sp. AL24 Isolated from Aerva lanata L. Indian J. Pharm. Educ. Res. 52 (2), 277–283. doi:10.5530/ijper.52.2.32
Sreeja, K., Anandaraj, M., and Suseela Bhai, R. (2019). Documentation of Fungal Endophytes of Black Pepper (Piper nigrum L.) and Their Seed Transmission Studies. J. Spices Arom Crops 28 (2), 113–121. doi:10.25081/josac.2019.v28.i2.6074
Sreejayan,, , and Rao, M. N. A. (2011). Nitric Oxide Scavenging by Curcuminoids. J. Pharm. Pharmacol. 49, 105–107. doi:10.1111/j.2042-7158.1997.tb06761.x
Suleria, H. A. R., Butt, M. S., Anjum, F. M., Saeed, F., and Khalid, N. (2015). Onion: Nature Protection against Physiological Threats. Crit. Rev. Food Sci. Nutr. 55 (1), 50–66. doi:10.1080/10408398.2011.646364
Tang, Z., Qin, Y., Chen, W., Zhao, Z., Lin, W., Xiao, Y., et al. (2021). Diversity, Chemical Constituents, and Biological Activities of Endophytic Fungi Isolated from Ligusticum chuanxiong Hort. Front. Microbiol. 12, 771000. doi:10.3389/fmicb.2021.771000
Tavassoli, S., and Djomeh, Z. E. (2011). Total Phenols, Antioxidant Potential and Antimicrobial Activity of Methanol Extract of Rosemary (Rosmarinus officinalis L.). Glob. Vet. 7 (4), 337–341.
Toma, C.-C., Olah, N.-K., Vlase, L., Mogoșan, C., and Mocan, A. (2015). Comparative Studies on Polyphenolic Composition, Antioxidant and Diuretic Effects of Nigella sativa L. (Black Cumin) and Nigella damascena L. (Lady-in-a-mist) Seeds. Molecules 20 (6), 9560–9574. doi:10.3390/molecules20069560
Vadabingi, N., Avula, V. K. R., Zyryanov, G. V., Vallela, S., Anireddy, J. S., Pasupuleti, V. R., et al. (2020). Multiple Molecular Targets Mediated Antioxidant Activity, Molecular Docking, ADMET, QSAR and Bioactivity Studies of Halo Substituted Urea Derivatives of α-Methyl- -DOPA. Bioorg. Chem. 97, 103708. doi:10.1016/j.bioorg.2020.103708
Veselinović, J. B., Veselinović, A. M., Nikolić, G. M., Pešić, S. Z., Stojanović, D. B., Matejić, J. S., et al. (2014). Antibacterial Potential of Selected 4-phenyl Hydroxycoumarins: Integrated In Vitro and Molecular Docking Studies. Med. Chem. Res. 24, 1626–1634. doi:10.1007/s00044-014-1245-0
Youssef, M. S., Saber, S. M., Hassane, A. M. A., and Arafa, R. F. (2015). Evaluation of Antibacterial Activities of Some Egyptian Medicinal Plant Extracts. J. Ecol. Health Environ. 3 (3), 49–57.
Yuan, J.-F., Zhang, Z.-Q., Fan, Z.-C., and Yang, J.-X. (2008). Antioxidant Effects and Cytotoxicity of Three Purified Polysaccharides from Ligusticum chuanxiong Hort. Carbohydr. Polym. 74 (4), 822–827. doi:10.1016/j.carbpol.2008.04.040
Zhou, Y.-X., Xin, H.-L., Rahman, K., Wang, S.-J., Peng, C., and Zhang, H. (2015). Portulaca oleracea L.: A Review of Phytochemistry and Pharmacological Effects. BioMed Res. Int. 2015, 925631. doi:10.1155/2015/925631
Keywords: black seed plants, mycoendophytes, phenolics, flavonoids, antioxidant, HPLC, molecular docking
Citation: Hassane AMA, Hussien SM, Abouelela ME, Taha TM, Awad MF, Mohamed H, Hassan MM, Hassan MHA, Abo-Dahab NF and El-Shanawany A-RA (2022) In Vitro and In Silico Antioxidant Efficiency of Bio-Potent Secondary Metabolites From Different Taxa of Black Seed-Producing Plants and Their Derived Mycoendophytes. Front. Bioeng. Biotechnol. 10:930161. doi: 10.3389/fbioe.2022.930161
Received: 27 April 2022; Accepted: 06 June 2022;
Published: 19 July 2022.
Edited by:
Claudia Botelho, University of Minho, PortugalReviewed by:
Ehsan Karimi, Islamic Azad University of Mashhad, IranMustafa A. Fawzy, Assiut University, Egypt
Serhat Keser, Firat University, Turkey
Copyright © 2022 Hassane, Hussien, Abouelela, Taha, Awad, Mohamed, Hassan, Hassan, Abo-Dahab and El-Shanawany. This is an open-access article distributed under the terms of the Creative Commons Attribution License (CC BY). The use, distribution or reproduction in other forums is permitted, provided the original author(s) and the copyright owner(s) are credited and that the original publication in this journal is cited, in accordance with accepted academic practice. No use, distribution or reproduction is permitted which does not comply with these terms.
*Correspondence: Abdallah M. A. Hassane, YWJkYWxsYWhoYXNzYW5lQGF6aGFyLmVkdS5lZw==; Mohamed E. Abouelela, bV9hYm91ZWxlbGFAYXpoYXIuZWR1LmVn