- 1Guangxi Key Laboratory of Environmental Pollution Control Theory and Technology, Guilin University of Technology, Guilin, China
- 2School of Life and Environmental Sciences, Guilin University of Electronic Technology, Guilin, China
- 3Guangxi Collaborative Innovation Center for Water Pollution Control and Water Safety in Karst Area, Guilin University of Technology, Guilin, China
- 4State Environmental Protection Key Laboratory of Simulation and Control of Groundwater Pollution, Chinese Research Academy of Environmental Sciences, Beijing, China
Biochar-amended landfill cover soil (BLCS) can promote CH4 and O2 diffusion, but it increases rainwater entry in the rainy season, which is not conducive to CH4 emission reduction. Hydrophobic biochar–amended landfill cover soil (HLCS) was prepared to investigate the changes in CH4 emission reduction and biological characteristics, and BLCS was prepared as control. Results showed that rainwater retention time in HLCS was reduced by half. HLCS had a higher CH4 reduction potential, achieving 100% CH4 removal at 25% CH4 content of landfill gas, and its main contributors to CH4 reduction were found to be at depths of 10–30 cm (upper layer) and 50–60 cm (lower layer). The relative abundances of methane-oxidizing bacteria (MOB) in the upper and lower layers of HLCS were 55.93% and 46.93%, respectively, higher than those of BLCS (50.80% and 31.40%, respectively). Hydrophobic biochar amended to the landfill cover soil can realize waterproofing, ventilation, MOB growth promotion, and efficient CH4 reduction.
1 Introduction
Methane (CH4) is an important greenhouse gas, and its global warming potential is 28-fold that of CO2 (Malyan et al., 2016). A large amount of CH4 is released during the degradation of domestic wastes in the landfill (Feng et al., 2019; Ghosh et al., 2019). Technologies that reduce CH4 emissions mainly include resource utilization and in situ emission reduction (Lou et al., 2011; Di Trapani et al., 2019). Methane oxidation of landfill cover as an in situ emission reduction technology is mostly used in small-sized, medium-sized, and aging landfills (Sadasivam and Reddy, 2014). Methane-oxidizing microorganisms play a vital role in the landfill cover (Mancinelli and McKay, 1984).
Clay is a widely used covering material because of its low price, wide source, non-toxicity, and easy construction (Christophersen et al., 2001; Chanton et al., 2009), but it is prone to crack formation, has limited diffusion of CH4 and O2, and lacks nutrients, thus restricting its application in landfill cover (Chanton et al., 2011a, 2011b; Scheutz et al., 2011a). Researchers currently use biological cover materials (Scheutz et al., 2011b; Lou et al., 2011), one of which is biochar that attracts attention due to its high porosity, large specific surface area, and high biological affinity (Sadasivam, 2015; Xu et al., 2016; Bamdad et al., 2019; Cimon et al., 2020). Yaghoubi (2011) studied the CH4 adsorption capacity of soil, biochar, and soil containing 10% and 20% biochar and found maximum values of 32, 346, 59, and 82 ml kg−1, respectively, indicating that the CH4 adsorption capacity of landfill cover soil increases with the biochar content. Reddy et al. (2014) suggested that the number of methane-oxidizing bacteria (MOB) in biochar-amended soil column is higher than that in soil alone. Huang et al. (2019) found that a 15% volume ratio for biochar amendment in landfill cover soil enhances CH4 removal efficiency, thus revealing the possible advantages of biochar amendment in terms of CH4 emission reduction, such as high porosity conducive to the diffusion of CH4 and O2 and great retention of nutrients for MOB.
In the biochar-amended cover soil, the hydraulic conductivity increases with the biochar content, 2% biochar addition increases the hydraulic conductivity by an order of magnitude (Reddy et al., 2021). An increase in hydraulic conductivity will cause a large amount of rainwater to enter the biochar-amended cover soil during rainy season (Wong et al., 2022), thereby occupying pores of the cover soil, increasing the moisture content of the cover soil and negatively affecting CH4 adsorption and oxidation (Scheutz et al., 2009; Yargicoglu and Reddy, 2017). Increasing the biochar content in the cover soil is necessary to promote the entry of CH4 from the landfill and O2 from the atmosphere into the cover soil, thereby increasing the efficiency of methane oxidation for the biochar-amended cover soil. However, rainwater can be prevented from entering by reducing the proportion of biochar, which leads to a decrease in the CH4 adsorption and oxidation efficiency of the system. Therefore, promoting the diffusion of CH4 and O2 and preventing rainwater from entering the cover layer have become the key to the application of biochar landfill cover soil technology (Cheng et al., 2018).
The development of hydrophobic biochar that can achieve waterproof and breathable properties will provide a way to solve the aforementioned problems (Sun et al., 2019; Wu et al., 2020). In this study, hydrophobic biochar replaced ordinary biochar and was added to the landfill cover soil to form hydrophobic biochar–amended landfill cover soil (HLCS). A biochar-amended landfill cover soil (BLCS) control group was also prepared. The CH4 emission reduction performance and biological characteristics of HLCS were investigated to provide a theoretical basis for the HLCS application.
2 Materials and Methods
2.1 Preparation of Hydrophobic Biochar–Amended Landfill Cover Soil
Hydrophobic biochar was made by our laboratory through modifying rice straw biochar with hydrophobic modifier silane-coupling agent KH570 (CH2=C(CH3)COOC3H6Si(OCH3)); the related preparation process have been published (Sun et al., 2019). The water absorption of hydrophobic biochar is 1.27 g (g biochar)−1, only 20% of that of rice straw biochar.
HLCS was prepared based on the results of our previous study for BLCS (Qin et al., 2021), by mixing the hydrophobic biochar with the landfill cover soil at a volume ratio of 1:4. A control group BLCS was also provided. Physical and chemical properties of BLCS and HLCS as specified in Table 1. There were no significant differences in maximum compaction dry density and nutritional indicators (such as N content, K content, and organic matter content) of BLCS and HLCS. The plasticity index of HLCS was obviously lower than that of BLCS, indicating that HLCS contained fewer hydrophilic groups (Soltani-Jigheh et al., 2019).

TABLE 1. Physical and chemical properties of biochar-amended and hydrophobic biochar–amended landfill cover soils.
2.2 Experimental Device
Two landfill cover–simulated columns (CB and CH) made of plexiglass with 100-cm height and 15-cm diameter were set up for the CH4 emission reduction experiment (Figure 1). Air was blown in from the top of the column by a blower (flow rate = 50 ml min−1), and simulated landfill gas composed of CH4, CO2, and N2 in a certain proportion was pumped into the bottom of the column (flow rate = 15 ml min−1) (Reddy et al., 2014). Air and landfill gas were humidified into the column to add moisture. The simulated columns were composed of cover, gravel, and permeable layers from top to bottom. The height of cover layer was 60 cm (Yargicoglu and Reddy, 2018). For the two simulated columns CB and CH, the cover materials consisted of biochar-amended and hydrophobic biochar–amended landfill cover soils, respectively. The relative compaction of two columns was approximately 80% (Reddy et al., 2014). The initial moisture content of two columns was 20%. In total, nine sampling ports were set on one side of the cover layer to collect gas and soil samples numbered 1–9 from top to bottom, and the interval between each sampling port was 5 cm. The height of the gravel layer was 10 cm to support the cover layer. The permeable layer with a height of 15 cm can discharge the excess moisture of the cover layer.
2.3 Operation Method
The simulated columns of CB and CH were operated by increasing the content of CH4 in the simulated landfill gas. The whole operation process consisted of the following three stages: stage Ⅰ (days 1–30) with CH4 content of 5%, stage Ⅱ (days 31–60) with CH4 content of 15%, and stage Ⅲ (days 61–95) with CH4 content of 25%. The experiment was finished after the CH4 was almost completely removed.
2.4 Sampling and Analysis Methods
The gas inlet and outlet of simulation columns were closed during sampling. Gas samples at each stage were periodically collected by syringes from the outlets, 1# (upper cover layer, 10 cm depth), 5# (middle cover layer, 30 cm depth), and 9# (lower cover layer, 50 cm depth) of CB and CH into collection bags for CH4 content analysis. Each gas sample included 20 ml of gas in a collection bag. The degree of contribution of CH4 removal at different depths in the simulation column depends on the amount of CH4 removal at that depth, that is, the differential between the CH4 content at the inlet and outlet of that depth. The total CH4 removal rate was determined by measuring the differential between the CH4 content of the inlet and outlet of the simulation column at regular time intervals.
where Cin and Cout are the CH4 content of the inlet and outlet of the simulation column (%), respectively.
Soil samples were collected by sterile spoons from the 1#, 5#, and 9# sampling ports of simulation columns at the beginning of the operation (day 1) and the end of stages Ⅰ, Ⅱ, and Ⅲ (days 30, 60, and 95, respectively) into sterile tubes to study the changes in microbial community structure. Each soil sample included 2 g of soil in a sterile tube. The soil samples collected from the 1#, 5#, and 9# sampling ports at the beginning (day 1) of CB were named B1.1, B1.5, and B1.9, and those collected at the end of the stage I were named B2.1, B2.5, and B2.9. The naming rules of the soil samples collected from CH were the same as those of CB. The collected soil samples were qualified by DNA extraction, PCR amplification, product purification, library preparation, and library inspection and then sequenced on NovaSeq6000 (Beijing Nuohe Zhiyuan Technology Co., Ltd.). The primers used for PCR amplification of bacteria were 341F_806R and Arch519F_915R for archaea. R and Origin software were used to visualize the data sets of the microbial community characteristics after quality control, OTU clustering, and species annotation.
At the end of stage Ⅲ, 2 g of soil samples from each of the upper, middle, and lower cover layers of CB and CH were taken with sterilized spoons for fluorescence in situ hybridization (FISH). The probes used in the FISH experiment are shown in Table 2.
3 Results and Discussion
3.1 Methane Emission Reduction of the Landfill Cover
CB and CH were operated by continuously increasing the CH4 content of simulated landfill gas. The whole process was divided into three stages and lasted for 95 days. Changes in CH4 content at each sampling port of CB and CH during the operation are shown in Figure 2.
In stage Ⅰ (days 1–30), the CH4 content in the simulated gas for CB and CH was 5%. The CH4 content of each sampling port gradually decreased with the operation of simulated column. On day 5, the CH4 contents of outlet of CB and CH were 1.12% and 3.90%, respectively, and the total removal rate of CH4 of CB and CH were 77.60% and 22.00%, respectively. The CH4 removal rate of CH was lower at the initial stage compared with that of CB possibly because the microorganisms have not fully adapted to the hydrophobic biochar environment. The CH4 removal efficiency of CB and CH was remarkably improved with further operation of the simulated column. On day 30, the total removal rates of both CB and CH reached over 98%, but CH was still slightly lower than CB. The CH4 content in the simulated landfill gas was then further increased to 15%, and the experiment entered stage Ⅱ (days 31–60). The CH4 removal efficiency at the beginning of stage Ⅱ was lower compared with that in stage Ⅰ possibly because the microorganisms have not adapted to the high CH4 content. The CH4 removal efficiency of each simulated column was remarkably improved with further domestication. At the end of the stage Ⅱ (day 60), the CH4 contents of 9#, 5#, 1#, and outlet of CB were 11.21%, 10.01%, 6.93%, and 3.54%, respectively, and the CH4 contents of 9#, 5#, 1#, and outlet of CH were 11.58%, 10.16%, 5.98%, and 3.94%, respectively. The main contributors of CB and CH to CH4 removal were extended from depth 50–60 cm (below nine# sampling port) in stage Ⅰ to the depths 50–60 cm and 10–30 cm (between 1# and 5# sampling ports) in stage Ⅱ, while the depth 0–30 cm of the traditional landfill cover soil is the main part responsible for CH4 removal due to the limited of oxygen (Feng et al., 2019). It can be seen that the CH4 removal of CB and CH was not limited by oxygen with the addition of high-porosity biochar (Huang et al., 2019). The total CH4 removal rate of CH (73.73%) was still slightly lower than that of CB (76.40%). But the CH4 content of 1# sampling port of CH was significantly lower than that of CB in the later stage Ⅱ (days 45–60), and the CH4 removal efficiency at the depth 10–30 cm of CH was higher than that of CB (Figure 2), indicating that the CH4 oxidation performance of CH was further improved.
In stage Ⅲ (days 61–95), the CH4 content in the simulated landfill gas of CB and CH were elevated to 25%. The CH4 removal efficiency of CB and CH was not remarkably affected by the increase in CH4 content and even showed a growing trend, indicating that the simulation column has gradually stabilized and is not easily affected by load mutation. The CH4 removal efficiency at different depths of CB and CH were further improved and then stabilized with further domestication. At the end of the experiment (day 95), the CH4 contents of 9#, 5#, 1#, and outlet of CB were 9.28%, 8.61%, 1.35%, and 0.02%, respectively, and the total removal rate of CB was 99.90%, which was higher than that of traditional soil covers (average is 35%) (Christophersen et al., 2001) and woody biochar soil covers (46%–56%) (Reddy et al., 2014; Yargicoglu and Reddy, 2017; Huang et al., 2019), indicating that biochar can provide convenient conditions for the oxidation and adsorption of CH4, and different biochar materials may affect the efficiency of CH4 reduction (Liu et al., 2015; Wong et al., 2017; He et al., 2018). The CH4 contents of 9#, 5#, 1#, and outlet of CH at the 95th day were 9.67%, 9.25%, 0.70%, and 0%, respectively. The total removal rate of CH was 100%, and the CH4 removal efficiency at the depth 10–30 cm of CH was significantly higher than that of CB, representing the potential to reduce CH4 with higher concentration for CH. The CH4 removal of CB and CH were mainly performed at depths of 50–60 and 10–30 cm, respectively, indicating these depths have relatively high methane oxidation activity (Yargicoglu and Reddy. 2018; Reddy et al., 2019) and abundance of MOB (as described in 3.3). As seen before, hydrophobic biochar instead of ordinary biochar in the landfill cover soil can also achieve efficient CH4 removal.
3.2 Moisture Content and Methane Emission Reduction of Landfill Cover After Simulated Rainfall
A simulated rainfall experiment was conducted to study the waterproof and breathability performance of hydrophobic biochar at the end of stage Ⅲ. Approximately, 2.5 L of water was added to CB and CH through the sprinkler head at the top of columns. The residence time of rainwater in the cover layer was the time between the spraying of sprinkler head and the continuous outflow of water in the permeable layer. The residence time of rainwater was 101.23 s in CB and was shortened by half to 50.55 s in CH, indicating that rainwater stayed in the CH for a shorter time and HLCS has good hydrophobic property.
The 4 g soil samples and 20 ml gas samples were collected from each of sampling ports 1#, 5#, and 9# of CB and CH after water addition to measure moisture content and CH4 removal efficiency, respectively. The changes of moisture and CH4 content of CB and CH are shown in Figure 3. After the water addition, the soil moisture contents of 1#, 5#, and 9# of CH were all lower than CB, the CH4 removal efficiency of CH was less affected than that of CB, especially for the soil moisture contents of upper layer of CH(1#, 39.75%) was significantly lower than that of CB(1#, 41.15%), and the CH4 content of 1# of CH(10.46%) was also significantly lower than that of CB(13.07%). It is worth noting that although HLCS improves the waterproof and breathable properties of the cover layer to reduce CH4 emission on rainy days, due to the hydrophobic properties of its material, rainwater is not retained in the cover layer after rainfall, but flows into the landfill layer quickly, resulting in a large amount of leachate generation instantly. Therefore, it is necessary to consider a certain slope and install drainage pipes at the bottom of the slope during the construction of hydrophobic biochar soil landfill cover. In addition, the assessment of the biosafety of KH570 in leachate is also important.
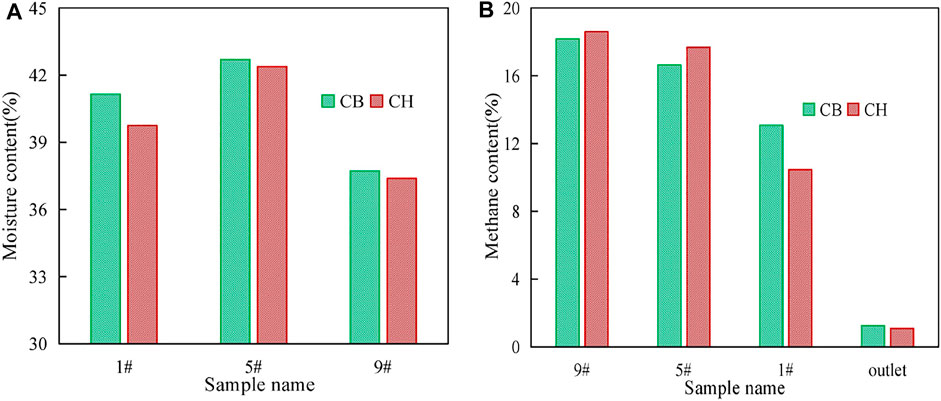
FIGURE 3. Changes of moisture content (A) and methane content (B) in CB and CH after simulated rainfall.
3.3 Biological Characteristics of Landfill Cover
3.3.1 Composition of Bacterial Community in the Landfill Cover
In total, 24 samples at different depths and stages of CB and CH were analyzed by high-throughput sequencing. The bacterial community composition at genus level (top 30) is shown in Figure 4. At the end of stage Ⅰ and stage Ⅱ, Luteimonas had the highest abundance in CB, and then decreased to the lowest in the end of stage Ⅲ (Figure 4A). Luteimonas has a strong ability of organic matter degradation (Takashi et al., 2004). The high relative abundance of Luteimonas in the early stage may be due to the presence of rich organic matter in the cover that was generated from the decay of some microorganisms that were not suitable for environmental change. The continuous decrease in the relative abundance of some bacteria (Castellaniella, Ciceribacter, Proteiniphilum, Petrimonas, etc) in the early stage also confirmed this speculation. The change trend of Luteimonas in CH was the same as that of CB, but the relative abundance of Luteimonas was significantly lower than CB (Figure 4B). The hydrophobic modifier KH570 was speculated to be an environmentally friendly material (Yang et al., 2012), whereas biochar modified by KH570 was added to soil cover, provided nutrients and suitable moisture for the growth of microorganisms, protected the flora from environmental threats, and reduced the decline of microorganisms (only 10 species of CH declined, while 15 species of CB in the top 30 genera). Some Common bacteria such as Sphingomonas, Bacillus, Truepera, Bryobacter and Terrimonas were enriched in CB and CH, but the bacteria enriched in different depths of the landfill cover were different, and some different genera were observed with the enrichment in different columns, indicating that different covering materials and different depths would significantly affect the distribution of the bacteria in the landfill cover (Cole et al., 2019; Huang et al., 2019; Wong et al., 2019).
The MOB was enriched with the increase of CH4 content of simulated landfill gas in CB and CH. The distribution of MOB in each landfill cover at the end of the experiment is shown in Table 3. The FISH detection results of CB and CH are shown in Figure 5. Six types of main MOB in CB and CH, including Type Ⅰ Methylobacter, Methylocaldum, and Methylloccus, Type Ⅱ Methylocystis, and other types of Methylobacillus and Methyloversatilis, were detected (Table 3). Methylocaldum and Methylloccus were also classified among them as Type X (Kalyuzhnaya et al., 2015). The dominant MOB in the upper layer of CB and CH were Type Ⅰ Methylobacter, which is the major abundant methanotrophs reported in other studies of landfill cover soil (Chi et al., 2015; Reddy et al., 2019). The green fluorescence representing Type I MOB in the upper layer of CH was more and brighter than that of CB (Figures 5A,B), and the total abundance of MOB in the upper layer of CH(55.93%) was higher than that of CB(50.80%), which was consistent with changes of the CH4 removal efficiency in this depth (Figure 2). The dominant MOB in the lower layer of CB were Methylobacter and Methylocaldum, and for the CH was Methylobacter. The relative abundance of total MOB in the lower layer of CH (46.93%) was higher than that for the same depth of CB(31.40%), and with more and brighter green fluorescence in CH (Figures 5E,F). The abundance of total MOB in the lower layers of both CH and CB were lower than that in the upper layers, but the CH4 removal rates were significantly higher than those in the upper layers, indicating that the high CH4 content in the lower layers were also critical for the improvement of CH4 removal efficiency. The dominant MOB in the middle layers of CB and CH were Methylobacter. The green fluorescence in the middle layer of CH was weaker and less than that of CB (Figures 5C,D). The relative abundance of total MOB in the middle layer of CH(13.02%) was visibly less than that for the same depth of CB(42.67%), and with little difference in the inlet CH4 content (9#) in this layer, it is presumed to be a lower level of immediate CH4 production in the middle layer of CH, supported by the lower abundance of methanogenic archaea in the middle and lower layers of CH(as described in Section 3.3.2).

TABLE 3. Relative abundance of methane-oxidizing bacteria of different cover layers at the end of the experiment.
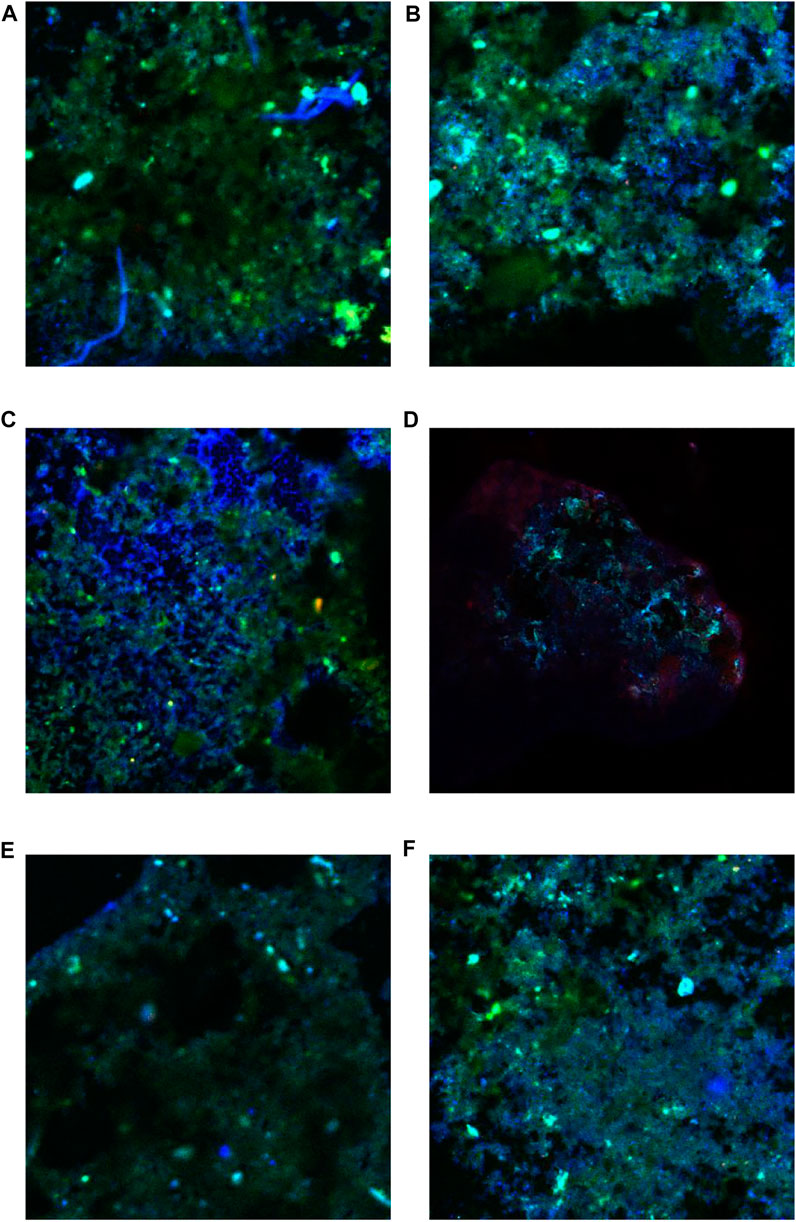
FIGURE 5. Fluorescence in situ hybridization diagram of methane-oxidizing bacteria at each layer of CB and CH. (A) Upper layer of CB. (B) Upper layer of CH. (C) Middle layer of CB. (D) Middle layer of CH. (E) Lower layer of CB. (F) Lower layer of CH.
From this it is clear that hydrophobic biochar was conducive to MOB growth in upper and lower layers and thereby achieved high-efficiency CH4 removal, and the dominant MOB in different cover materials was varied. Some researchers suggested that the high CH4 oxidation rates and high MOB abundances were present in the upper layers of amended soil (0–30 cm depth) where the O2 availability was high (Reddy et al., 2014; Yargicoglu and Reddy, 2017; Feng et al., 2019). While in this study, the high CH4 oxidation rates and high MOB abundances were also present in the lower layer of HLCS. It can be seen that some MOB (such as Methylobacter) can also survive in low oxygen conditions (Grinsven et al., 2020), and higher MOB enrichment at the lower layer can be achieved by gradually increasing CH4 content of the inlet gas and with long-term acculturation (Reddy et al., 2014).
3.3.2 Composition of Archaea Community in the Landfill Cover
Aerobic methane oxidation in the landfill cover was considered to be the main way to achieve CH4 emission reduction (Chen et al., 2018; Wong et al., 2019), while anaerobic methane oxidation was ignored (Zhang et al., 2019). The low volume fraction of oxygen in the middle and lower layers of the landfill cover provided favorable conditions for the growth of anaerobic methanotrophic archaea (ANME) (Dong et al., 2015). The ANME with relatively high abundance detected in this study was Methanosarcinales and Methanomicrobiales (Figure 6). With the increase of CH4 concent in inlet, the relative abundance of Methanosarcinales in each sampling ports of CB and CH showed a decreasing trend (the color in the heat map gradually changed from red to blue (Figure 6), showing that it was not suitable for the environment of landfill cover–simulated columns. Methanomicrobiales was gradually decreased in the upper and lower layers of CB and CH, while it was enriched in the middle layers of CB and CH, indicating that ANME contributed to CH4 emission reduction in the middle layers of the landfill covers, but the addition of hydrophobic biochar had little impact on the abundance of ANME. In addition, some methanogenic archaea, such as Methanobacteriales and Methanomassiliicoccales, were found in both CH and CB, and mainly distributed in the middle and lower layers of CH and CB. The relative abundances of methanogenic archaea in the middle and lower layers of CH were 86.3 and 65.5%, respectively, both lower than 87.4 and 89.5% in CB. With these suggested that the middle and lower layers were the main methanogenic regions, where the methanogenic process of CH might be weaker than that of CB. It is evident that the cover layer simulation column is a dynamic CH4 production and consumption system. The reinforcement of ANME and suppression of methanogenic archaea are also essential for CH4 reduction.
3.4 Biological Mechanism of Hydrophobic Biochar–Amended Landfill Cover Soil
The hydrophobic biochar replaced ordinary biochar was added to the landfill cover soil to form HLCS, the rainwater retention time in HLCS was reduced by half and an efficient CH4 reduction was achieved. Thus, HLCS can solve the contradiction between promoting the diffusion of CH4 and O2 and preventing rainwater from entering the cover layer, the biological mechanism as shown in Figure 7. The upper layer and lower layer of HLCS were the main parts responsible for CH4 emission reduction. The dominant MOB in HLCS was Methylobacter, and with high abundance in the lower and upper layers of HLCS. In addition, there was a certain abundance of ANME in the middle layer of HLCS, and some methanogenic archaea were also discovered in HLCS. It can be seen that the aerobic methane oxidation, anaerobic methane oxidation and methane production were all existed in HLCS. HLCS was a dynamic CH4 generation and consumption system, and the suppression of methanogenic archaea in HLCS is essential for CH4 emission reduction.
In addition, compared with BLCS, HLCS had an efficient CH4 reduction potential and higher abundance of MOB. These may be related to the higher zeta potential of hydrophobic biochar than ordinary biochar facilitated the adsorption of MOB (Afrooz et al., 2018; Ren et al., 2020). Meanwhile, the organic long chain (alkoxy group) of hydrophobic modifier KH570 grafted on biochar surface induced extensive agglomeration (Yang et al., 2012; Sun et al., 2019), which is conducive to the clustering of MOB and the capture of gas molecules such as CH4 and O2 (Zhang et al., 2019). More importantly, the large specific surface area and the appropriate moisture content of hydrophobic biochar provided good conditions for MOB growth (Yang et al., 2012; Reddy et al., 2014). Therefore, the cover layer of hydrophobic biochar soil had high abundance of MOB and thus showed relatively high CH4 oxidation efficiency.
4 Conclusion
Hydrophobic biochar amended to the landfill cover soil can realize waterproofing, ventilation, MOB growth promotion, and efficient CH4 reduction. The rainwater retention time in HLCS was reduced by half compared with BLCS. A completed removal of CH4 was achieved in HLCS with 25% CH4 content of the landfill gas. The main contributors to the CH4 removal in HLCS were found in depths 10–30 (upper layer) and 50–60 cm (lower layer), and the CH4 removal in its upper layer of HLCS was more effective than that in BLCS. The dominant MOB in HLCS was Methylobacter; the relative abundances of the MOB in the upper and lower layers of HLCS were significantly higher than those of BLCS. The ANME and methanogenic archaea were also discovered in HLCS, and the reinforcement of ANME and suppression of methanogenic archaea are also essential for CH4 reduction.
Data Availability Statement
The original contributions presented in the study are included in the article; further inquiries can be directed to the corresponding authors.
Author Contributions
XS, YQ, and CX contributed to conception and design of the study. YQ and CX organized the database. YQ and BW performed the statistical analysis. YQ wrote the first draft of the manuscript. XS, BX, and HZ wrote sections of the manuscript. All authors contributed to manuscript revision, read, and approved the submitted version.
Funding
This research was funded by the Natural Science Foundation of Guangxi (No. 2018GXNSFGA281001), National Natural Science Foundation of China (No. 51668014), Guangxi Science and Technology Planning Project (No. GuiKe-AD18126018), and Research Foundation of Young and Middle-aged Teachers in Guangxi Universities (No. 2021KY0204).
Conflict of Interest
The authors declare that the research was conducted in the absence of any commercial or financial relationships that could be construed as a potential conflict of interest.
Publisher’s Note
All claims expressed in this article are solely those of the authors and do not necessarily represent those of their affiliated organizations, or those of the publisher, the editors, and the reviewers. Any product that may be evaluated in this article, or claim that may be made by its manufacturer, is not guaranteed or endorsed by the publisher.
References
Afrooz, A. R. M. N., Pitol, A. K., Kitt, D., and Boehm, A. B. (2018). Role of Microbial Cell Properties on Bacterial Pathogen and Coliphage Removal in Biochar-Modified Stormwater Biofilters. Environ. Sci. Water Res. Technol. 4 (12), 2160–2169. doi:10.1039/c8ew00297e
Bamdad, H., Hawboldt, K., Macquarrie, S., and Papari, S. (2019). Application of Biochar for Acid Gas Removal: Experimental and Statistical Analysis Using CO2. Environ. Sci. Pollut. Res. 26, 10902–10915. doi:10.1007/s11356-019-04509-3
Chanton, J., Abichou, T., Langford, C., Hater, G., Green, R., Goldsmith, D., et al. (2011a). Landfill Methane Oxidation across Climate Types in the U.S. Environ. Sci. Technol. 45 (1), 313–319. doi:10.1021/es101915r
Chanton, J., Abichou, T., Langford, C., Spokas, K., Hater, G., Green, R., et al. (2011b). Observations on the Methane Oxidation Capacity of Landfill Soils. Waste Manag. 31 (5), 914–925. doi:10.1016/j.wasman.2010.08.028
Chanton, J. P., Powelson, D. K., and Green, R. B. (2009). Methane Oxidation in Landfill Cover Soils, Is a 10% Default Value Reasonable? J. Environ. Qual. 38 (2), 654–663. doi:10.2134/jeq2008.0221
Chen, X. W., Wong, J. T. F., Chen, Z. T., Tang, T. W. L., Guo, H. W., Leung, A. O. W., et al. (2018). Effects of Biochar on the Ecological Performance of a Subtropical Landfill. Sci. Total Environ. 644, 963–975. doi:10.1016/j.scitotenv.2018.06.379
Cheng, Q., Guo, H., Feng, T., and Lu, Y. (2018). Volume Changes of Biochar-Amended Landfill Cover Soil under a Thermal Cycle. Waste Manag. Res. 36 (12), 1223–1227. doi:10.1177/0734242x18790356
Chi, Z.-F., Lu, W.-J., and Wang, H.-T. (2015). Spatial Patterns of Methane Oxidation and Methanotrophic Diversity in Landfill Cover Soils of Southern china. J. Microbiol. Biotechnol. 25 (4), 423–430. doi:10.4014/jmb.1408.08055
Christophersen, M., Kjeldsen, P., Holst, H., and Chanton, J. (2001). Lateral Gas Transport in Soil Adjacent to an Old Landfill: Factors Governing Emissions and Methane Oxidation. Waste Manag. Res. 19 (6), 595–612. doi:10.1177/0734242x0101900616
Cimon, C., Kadota, P., and Eskicioglu, C. (2020). Effect of Biochar and Wood Ash Amendment on Biochemical Methane Production of Wastewater Sludge from a Temperature Phase Anaerobic Digestion Process. Bioresour. Technol. 297, 122440. doi:10.1016/j.biortech.2019.122440
Cole, E. J., Zandvakili, O. R., Blanchard, J., Xing, B., Hashemi, M., and Etemadi, F. (2019). Investigating Responses of Soil Bacterial Community Composition to Hardwood Biochar Amendment Using High-Throughput PCR Sequencing. Appl. Soil Ecol. 136, 80–85. doi:10.1016/j.apsoil.2018.12.010
Di Trapani, D., Volpe, M., Di Bella, G., Messineo, A., Volpe, R., and Viviani, G. (2019). Assessing Methane Emission and Economic Viability of Energy Exploitation in a Typical Sicilian Municipal Solid Waste Landfill. Waste Biomass Valor 10 (10), 3173–3184. doi:10.1007/s12649-018-0321-y
Dong, J., Ding, L., Wang, X., Chi, Z., and Lei, J. (2015). Vertical Profiles of Community Abundance and Diversity of Anaerobic Methanotrophic Archaea (ANME) and Bacteria in a Simple Waste Landfill in North China. Appl. Biochem. Biotechnol. 175, 2729–2740. doi:10.1007/s12010-014-1456-3
Feng, S., Leung, A. K., Liu, H. W., Ng, C. W. W., Zhan, L. T., and Chen, R. (2019). Effects of Thermal Boundary Condition on Methane Oxidation in Landfill Cover Soil at Different Ambient Temperatures. Sci. Total Environ. 692, 490–502. doi:10.1016/j.scitotenv.2019.07.108
Ghosh, P., Shah, G., Chandra, R., Sahota, S., Kumar, H., Vijay, V. K., et al. (2019). Assessment of Methane Emissions and Energy Recovery Potential from the Municipal Solid Waste Landfills of Delhi, India. Bioresour. Technol. 272, 611–615. doi:10.1016/j.biortech.2018.10.069
Grinsven, S., Sinninghe Damsté, J. S., Abdala Asbun, A., Engelmann, J. C., Harrison, J., and Villanueva, L. (2020). Methane Oxidation in Anoxic Lake Water Stimulated by Nitrate and Sulfate Addition. Environ. Microbiol. 22, 766–782. doi:10.1111/1462-2920.14886
He, X., Yin, H., Sun, X., Han, L., and Huang, G. (2018). Effect of Different Particle-Size Biochar on Methane Emissions during Pig Manure/wheat Straw Aerobic Composting: Insights into Pore Characterization and Microbial Mechanisms. Bioresour. Technol. 268, 633–637. doi:10.1016/j.biortech.2018.08.047
Huang, D., Yang, L., Ko, J. H., and Xu, Q. (2019). Comparison of the Methane-Oxidizing Capacity of Landfill Cover Soil Amended with Biochar Produced Using Different Pyrolysis Temperatures. Sci. Total Environ. 693, 133594. doi:10.1016/j.scitotenv.2019.133594
Kalyuzhnaya, M. G., Puri, A. W., and Lidstrom, M. E. (2015). Metabolic Engineering in Methanotrophic Bacteria. Metab. Eng. 29, 142–152. doi:10.1016/j.ymben.2015.03.010
Liu, W.-J., Jiang, H., and Yu, H.-Q. (2015). Development of Biochar-Based Functional Materials: Toward a Sustainable Platform Carbon Material. Chem. Rev. 115 (22), 12251–12285. doi:10.1021/acs.chemrev.5b00195
Lou, Z., Wang, L., and Zhao, Y. (2011). Consuming Un-captured Methane from Landfill Using Aged Refuse Bio-Cover. Bioresour. Technol. 102 (3), 2328–2332. doi:10.1016/j.biortech.2010.10.086
Malyan, S. K., Bhatia, A., Kumar, A., Gupta, D. K., Singh, R., Kumar, S. S., et al. (2016). Methane Production, Oxidation and Mitigation: a Mechanistic Understanding and Comprehensive Evaluation of Influencing Factors. Sci. Total Environ. 572, 874–896. doi:10.1016/j.scitotenv.2016.07.182
Mancinelli, R. L., and McKay, C. P. (1984). “Methane Oxidizing Bacteria in Sanitary Landfills,” in Proceedings First Symposium Biotechnological Advances in Processing Municipal Wastes for Fuels and Chemicals, 437–449.
Qin, Y. L., Sun, X. J., Wang, C. L., Wu, B. B., Xue, C. N., and Zhang, H. X. (2021). Methane Emission Reduction and Biological Characteristics Induced by the Landfill Cover Soil Amended with Biochar. China Environ. Sci. 41 (1), 254–262. doi:10.19674/j.cnki.issn1000-6923.2021.0031
Reddy, K. R., Yargicoglu, E. N., Yue, D., and Yaghoubi, P. (2014). Enhanced Microbial Methane Oxidation in Landfill Cover Soil Amended with Biochar. J. Geotechnical Geoenvironmental Eng. 140 (9), 1–7. doi:10.1061/(asce)gt.1943-5606.0001148
Reddy, K. R., Rai, R. K., Green, S. J., and Chetri, J. K. (2019). Effect of Temperature on Methane Oxidation and Community Composition in Landfill Cover Soil. J. Industrial Microbiol. Biotechnol. 46, 1283–1295. doi:10.1007/s10295-019-02217-y
Reddy, K. R., Yargicoglu, E. N., and Chetri, J. K. (2021). Effects of Biochar on Methane Oxidation and Properties of Landfill Cover Soil: Long-Term Column Incubation Tests. J. Environ. Eng. 147 (1), 04020144. doi:10.1061/(asce)ee.1943-7870.0001829
Ren, H.-Y., Wei, Z.-J., Wang, Y., Deng, Y.-P., Li, M.-Y., and Wang, B. (2020). Effects of Biochar Properties on the Bioremediation of the Petroleum-Contaminated Soil from a Shale-Gas Field. Environ. Sci. Pollut. Res. 27, 36427–36438. doi:10.1007/s11356-020-09715-y
Sadasivam, B. Y. (2015). Biochar-based Cover System for Landfill Methane Mitigation: Quantifying Adsorption, Transport & Oxidation. Chicago, Illinois, USA: University of Illinois at Chicago. Ph.D. Thesis.
Sadasivam, B. Y., and Reddy, K. R. (2014). Landfill Methane Oxidation in Soil and Bio-Based Cover Systems: a Review. Rev. Environ. Sci. Biotechnol. 13 (1), 79–107. doi:10.1007/s11157-013-9325-z
Scheutz, C., Fredenslund, A. M., Chanton, J., Pedersen, G. B., and Kjeldsen, P. (2011a). Mitigation of Methane Emission from Fakse Landfill Using a Biowindow System. Waste Manag. 31, 1018–1028. doi:10.1016/j.wasman.2011.01.024
Scheutz, C., Kjeldsen, P., Bogner, J. E., De Visscher, A., Gebert, J., Hilger, H. A., et al. (2009). Microbial Methane Oxidation Processes and Technologies for Mitigation of Landfill Gas Emissions. Waste Manag. Res. 27 (5), 409–455. doi:10.1177/0734242x09339325
Scheutz, C., Pedicone, A., Pedersen, G. B., and Kjeldsen, P. (2011b). Evaluation of Respiration in Compost Landfill Biocovers Intended for Methane Oxidation. Waste Manag. 31 (5), 895–902. doi:10.1016/j.wasman.2010.11.019
Soltani-Jigheh, H., Bagheri, M., and Amani-Ghadim, A. R. (2019). Use of Hydrophilic Polymeric Stabilizer to Improve Strength and Durability of Fine-Grained Soils. Cold Regions Sci. Technol. 157, 187–195. doi:10.1016/j.coldregions.2018.10.011
Sun, X. J., Qin, Y. L., Li, Q., Wu, B. B., Li, J., and Xue, C. N. (2019). Optimization of Hydrophobic Properties of Biochar Modified by Silane Coupling Agent. Environ. Sci. Technol. 42 (12), 68–73. doi:10.19674/j.cnki.issn1000-6923.2021.0031 (in chinese)
Takashi, N., Satoru, T., and Akira, H. (2004). Activity and Phylogenetic Composition of Proteolytic Bacteria in Mesophilic Fed-Batch Garbage Composters. Microbes Environ. 19 (4), 292–300. doi:10.1264/jsme2.19.292
Wong, J. T. F., Chen, X., Deng, W., Chai, Y., Ng, C. W. W., and Wong, M. H. (2019). Effects of Biochar on Bacterial Communities in a Newly Established Landfill Cover Topsoil. J. Environ. Manag. 236, 667–673. doi:10.1016/j.jenvman.2019.02.010
Wong, J. T. F., Chen, Z., Chen, X., Ng, C. W. W., and Wong, M. H. (2017). Soil-water Retention Behavior of Compacted Biochar-Amended Clay: a Novel Landfill Final Cover Material. J. Soils Sediments 17, 590–598. doi:10.1007/s11368-016-1401-x
Wong, J. T. F., Chow, K. L., Chen, X. W., Ng, C. W. W., and Wong, M. H. (2022). Effects of Biochar on Soil Water Retention Curves of Compacted Clay during Wetting and Drying. Biochar 4, 4. doi:10.1007/s42773-021-00125-y
Wu, B., Xi, B., He, X., Sun, X., Li, Q., Ouche, Q., et al. (2020). Methane Emission Reduction Enhanced by Hydrophobic Biochar-Modified Soil Cover. Processes 8 (2), 162. doi:10.3390/pr8020162
Xu, X., Wu, Z., Dong, Y., Zhou, Z., and Xiong, Z. (2016). Effects of Nitrogen and Biochar Amendment on Soil Methane Concentration Profiles and Diffusion in a Rice-Wheat Annual Rotation System. Sci. Rep. 6, 38688. doi:10.1038/srep38688
Yaghoubi, P. (2011). Development of Biochar-Amended Landfill Cover for Landfill Gas Mitigation. Chicago, Illinois, USA: University of Illinois at Chicago. Ph.D. Thesis.
Yang, W., Miao, J., Xia, R., Qian, J., and Zhang, Y. (2012). On Surface Modification of Nano-TiN with Graft Copolymer LMPB-G-Kh570. J. Dispersion Sci. Technol. 33 (6), 827–834. doi:10.1080/01932691.2011.590119
Yargicoglu, E. N., and Reddy, K. R. (2018). Biochar-amended Soil Cover for Microbial Methane Oxidation: Effect of Biochar Amendment Ratio and Cover Profile. J. Geotech. Geoenviron. Eng. 144 (3), 04017123. doi:10.1061/(asce)gt.1943-5606.0001845
Yargicoglu, E. N., and Reddy, K. R. (2017). Effects of Biochar and Wood Pellets Amendments Added to Landfill Cover Soil on Microbial Methane Oxidation: A Laboratory Column Study. J. Environ. Manag. 193, 19–31. doi:10.1016/j.jenvman.2017.01.068
Keywords: landfill cover, biochar, hydrophobic, methane emission reduction, biological characteristics
Citation: Qin Y, Xi B, Sun X, Zhang H, Xue C and Wu B (2022) Methane Emission Reduction and Biological Characteristics of Landfill Cover Soil Amended With Hydrophobic Biochar. Front. Bioeng. Biotechnol. 10:905466. doi: 10.3389/fbioe.2022.905466
Received: 27 March 2022; Accepted: 28 April 2022;
Published: 08 June 2022.
Edited by:
Liyan Song, Anhui University, ChinaReviewed by:
Ziyang Lou, Shanghai Jiao Tong University, ChinaYuyang Long, Zhejiang Gongshang University, China
Copyright © 2022 Qin, Xi, Sun, Zhang, Xue and Wu. This is an open-access article distributed under the terms of the Creative Commons Attribution License (CC BY). The use, distribution or reproduction in other forums is permitted, provided the original author(s) and the copyright owner(s) are credited and that the original publication in this journal is cited, in accordance with accepted academic practice. No use, distribution or reproduction is permitted which does not comply with these terms.
*Correspondence: Xiaojie Sun, c3VueGlhb2ppZUBnbHV0LmVkdS5jbg==; Hongxia Zhang, emh4NzVAZ2x1dC5lZHUuY24=