- 1Department of Biology, School of Science, King Mongkut's Institute of Technology Ladkrabang, Bangkok, Thailand
- 2Laboratory of Cyanobacterial Biotechnology, Department of Biochemistry, Faculty of Science, Chulalongkorn University, Bangkok, Thailand
- 3Bioenergy Research Unit, School of Science, King Mongkut's Institute of Technology Ladkrabang, Bangkok, Thailand
The halotolerant cyanobacterium Aphanothece halophytica is a potential H2 producer that induces H2 evolution under nitrogen deprivation. H2 is mainly produced via the catabolism of stored glycogen under dark anaerobic condition. H2 evolution is catalyzed by O2-sensitive bidirectional hydrogenase. The aim of this study was to improve H2 production by A. halophytica using various kinds of inhibitors. Among all types of inhibitors, simazine efficiently promoted the highest H2 production under dark conditions. High simazine concentration and long-term incubation resulted in a decrease in cell and chlorophyll concentrations. The optimal simazine concentration for H2 production by A. halophytica was 25 µM. Simazine inhibited photosynthetic O2 evolution but promoted dark respiration, resulting in a decrease in O2 level. Hence, the bidirectional hydrogenase activity and H2 production was increased. A. halophytica showed the highest H2 production rate at 58.88 ± 0.22 µmol H2 g−1 dry weight h−1 and H2 accumulation at 356.21 ± 6.04 μmol H2 g−1 dry weight after treatment with 25 µM simazine under dark anaerobic condition for 2 and 24 h, respectively. This study demonstrates the potential of simazine for the enhancement of dark fermentative H2 production by A. halophytica.
1 Introduction
Taking into consideration the concern of limited fossil fuel and the environmental impact of energy consumption, great attention has been paid to the renewable energy sources for a replacement of primitive fossil fuels. H2 is an interesting alternative renewable energy carrier. The combustion of H2 yields a high heating value of 141.6 MJ kg−1 (Perry, 1963). Due to the chemical structure of H2 as a non-carbon–based molecule, H2 burning does not emit greenhouse gases or other pollutants into the environment. H2 is mainly produced by chemical processes; however, it can be produced by various kinds of organisms via many metabolic pathways depending on the organism types (Levin et al., 2004). Cyanobacteria and green algae can generate H2 using electrons obtained from photosynthetic electron transport and/or from stored glycogen degradation during dark fermentation (Srirangan et al., 2011).
The unicellular halotolerant cyanobacterium Aphanothece halophytica is a potential H2 producer (Taikhao et al., 2013). It can grow in strong external NaCl concentrations up to 3 M NaCl (Takabe et al., 1988) and in abundant natural seawater supplemented with merely 1.76 mM NaNO3 (Taikhao et al., 2015). H2 production by A. halophytica is notably induced under nitrogen deprivation by a catabolism of stored glycogen under anaerobic condition in darkness but is hardly detected under anaerobic condition in the light (Taikhao et al., 2013, 2015). H2 evolution by A. halophytica is catalyzed by the bidirectional hydrogenase (Phunpruch et al., 2016), which is sensitive to molecular oxygen evolved by the water-splitting reaction at photosystem II during photolysis reaction (McKinlay and Harwood, 2010). Moreover, sustainable long-term H2 production is enhanced in A. halophytica cells immobilized with agar and alginate (Pansook et al., 2016; 2019a).
H2 is chemically evolved through a reduction of protons by electrons. In cyanobacteria, electrons are generated by photosynthetic pathways using water as an electron donor. Electrons can be transferred to many chemical reactions through various metabolic pathways, such as CO2 fixation, carbohydrate metabolism, and the respiratory electron transport chain (Eroglu and Melis, 2011; Srirangan et al., 2011). To enhance H2 production, using inhibitors that hinder electron transfer to other processes is a choice. Consequently, more electrons are directly transferred to bidirectional hydrogenase to produce H2. Several inhibitors with the ability to direct electrons toward H2 metabolism of cyanobacteria are photosystem II inhibitor, respiratory inhibitor, uncoupling agent of oxidative phosphorylation inhibitor, CO2 fixation inhibitor, and Krebs cycle inhibitor. In Anabaena spp. strains CA and 1F, Anabaena cylindrica, and Anabaena sp. PCC7120, H2 production increases after treatment with photosystem II inhibitor 3-(3,4-dichlorophenyl)-1,1-dimethylurea (DCMU) under light (Zhang et al., 1983; Chen et al., 2013; Chen et al., 2014). Moreover, Krebs cycle inhibitor malonate also increases H2 production in Synechocystis sp. PCC6803 and Anabaena siamensis TISTR 8012 (Burrows et al., 2011; Khetkorn et al., 2012).
In this study, screening for inhibitors of H2 production by A. halophytica were investigated. Simazine was found to be a potential inhibitor for H2 production by A. halophytica under both light and dark conditions. Then, the effect of simazine concentration on cell concentration, chlorophyll a content, and H2 and O2 production was investigated. Finally, bidirectional hydrogenase activity, PSII, and dark respiration activities by A. halophytica treated with various concentrations of simazine were also investigated.
2 Materials and Methods
2.1 Cyanobacterial Growth Condition
A. halophytica was grown in a 250-ml Erlenmeyer flask containing 100 ml of BG11 (pH 7.4) (Rippka et al., 1979) supplemented with Turk Island salt solution (Garlick et al., 1977). A. halophytica cells were cultivated with an initial OD730 of approximately 0.1 and shaken at 120 rpm, 30°C under a cool white-light intensity of 30 µmol photons m−2 s−1 (16 h light and 8 h dark days−1) for 7 days.
2.2 Screening of Inhibitors Affecting H2 Production by A. halophytica
A. halophytica grown as previously described for 7 days was harvested by centrifugation at 8,000 × g at 4°C for 10 min, subsequently washed twice, and finally resuspended in 100 ml of nitrogen-free BG11 (BG110) supplemented with Turk Island salt solution. Cells were shaken on a rotary shaker at 120 rpm at 30°C under a white-light intensity of 30 µmol photons m−2 s−1 for 24 h. Cells were then harvested by centrifugation, resuspended in 5 ml of BG110 supplemented with Turk Island salt solution, and transferred to a 10-ml glass vial. Various kinds of inhibitors comprising photosystem II inhibitors such as atrazine (2-chloro-4-ethylamino-6-isopropylamino-1,3,5-triazine) (Sigma, Germany), DCMU [3-(3,4-dichlorophenyl)-1,1-dimethylurea] (Sigma, Germany), glyphosate [N-(phosphonomethyl)-glycine] (Sigma, Germany), and simazine (2-chloro-4,6-diethylamino-1,3,5-triazine) (Sigma, Germany); respiration inhibitors such as malonic acid (Sigma, Germany), rotenone (Sigma, Germany), and sodium azide (Sigma, Germany); an inhibitor of uncoupling agent of oxidative phosphorylation 2,4-dinitrophenol (DNP) (Sigma, Germany); a CO2 fixation inhibitor glyceraldehyde (Sigma, Germany); and a Krebs cycle inhibitor sodium arsenate (Sigma, Germany) at a final concentration of 5 µM were added into the cell suspension. The vials were sealed with a rubber stopper and further incubated at 30°C under the light for 2 h. Then, the vials were purged with argon gas for 10 min and incubated at 30°C under light or in darkness for 2 h before H2 measurement. In this study, simazine as an effective inhibitor for H2 production by A. halophytica was selected and then the effect of simazine concentration on H2 production by A. halophytica was investigated. The concentrations of simazine were varied at 0, 0.05, 0.5, 5, 25, and 50 µM.
2.3 Measurement of Cell and Chlorophyll a Concentration
The concentrations of cell and chlorophyll a were determined after simazine treatment for 0, 2, 24, 48, 72, and 96 h. An aliquot of cell suspension samples was collected, and the cell number was counted using a hemocytometer under a compound light microscope (Nikon Eclipse Ci-L, Japan). Cell concentration was calculated as a unit of cell number per volume of cell suspension. To analyze chlorophyll a concentration, 1 mL of cell culture was harvested by centrifugation at 8,000 × g at 4°C for 10 min. Chlorophyll a was extracted by adding 1 ml of 90% (v/v) methanol to a cell pellet, subsequently vortexing and incubating at 25°C under darkness for 1 h. Chlorophyll a concentration of pigment extract was determined by measuring an absorbance at 665 nm (MacKinney, 1941).
2.4 Measurement of H2 and O2 Production
The measurement of H2 and O2 production was determined by analyzing 500 µL of gas phase in the headspace of a vial containing 5 ml of cell suspension using a gas chromatograph (Hewlett-Packard HP5890A, Japan) with a molecular sieve 5°A 60/80 mesh packed column by a thermal conductivity detector (Taikhao et al., 2013). H2 and O2 production was calculated in terms of H2 and O2 produced per dry cell weight per time (µmol H2 g−1 dry cell weight h−1 and µmol O2 g−1 dry cell weight h−1).
2.5 Bidirectional Hydrogenase Activity Assay
Bidirectional hydrogenase activity of A. halophytica was determined in the presence of sodium dithionite-reduced methyl viologen (Taikhao et al., 2015). One mL of cell culture was added to 1 ml of 25 mM phosphate buffer (pH 7.0) containing 2.5 mM methyl viologen and 10 mM sodium dithionite. The reaction mixture was incubated at 25°C under dark anaerobic conditions for 15 min before H2 measurement by gas chromatograph as previously described (Taikhao et al., 2015). Bidirectional hydrogenase activity was expressed as µmol H2 g−1 dry weight min−1.
2.6 Measurement of Photosynthetic O2 Evolution Rate and Dark Respiration Rate
Photosynthetic O2 evolution and dark respiration rates were analyzed using a Clark-type oxygen electrode (Hansatech, United Kingdom). The measurement was carried out at 25°C. For photosynthetic O2 evolution measurement, 2 mL of A. halophytica cell suspension was added to a chamber and incubated in the dark for 15 min, prior to illumination under white-light intensity of 300 µmol photons m−2 s−1 for 15 min. The O2 evolution rate of cells was expressed as µmol O2 evolved per gram of cell dry weight per min. For dark respiration measurement, 2 mL of A. halophytica cell suspension in a chamber was incubated under a white-light intensity of 30 µmol photons m−2 s−1 for 15 min. Then, cells were incubated for 15 min under dark condition. The O2 consumption rate of cells was expressed as µmol O2 consumed per gram of cell dry weight per min.
2.7 Long-Term H2 Production Measurement
A. halophytica grown in BG11 (pH 7.4) supplemented with Turk Island salt solution for 7 days was harvested by centrifugation at 8,000 × g at 4°C for 10 min and resuspended in BG110 supplemented with Turk Island salt solution. Cells were shaken on a rotary shaker at 120 rpm at 30°C under light for 24 h, subsequently harvested by centrifugation at 8,000 × g at 4°C for 10 min, and resuspended in 5 ml of BG110. Cell suspension was transferred into a 12-ml glass vial and treated with simazine at a final concentration of 25 µM. Cells were purged with argon gas for 10 min and then incubated at 30°C under light and dark conditions. Long-term H2 production by A. halophytica cells treated with 25 µM simazine was determined for 10 days. A. halophytica cells without 25 µM simazine treatment and 25 µM simazine without cells were used as controls.
2.8 Statistical Data Analysis
The data in this study were statistically compared by a one-way analysis of variance (ANOVA) with Duncan’s multiple range test using IBM SPSS statistic 23 (IBM Corp, United States). Significant differences between treatments were considered at a level of 0.05 (p < 0.05).
3 Results
3.1 Screening of Inhibitors Affecting H2 Production by A. halophytica
In the first study, the measurement of H2 production by A. halophytica treated with various kinds of inhibitors at a final concentration of 5 µM was performed after dark and light incubation for 2 h. The results showed that under the light, A. halophytica treated with atrazine, DCMU, glyphosate, simazine, sodium azide, and 2,4-dinitrophenol showed significantly higher H2 production rate than cells without inhibitor treatment (Table 1). On the other hand, A. halophytica treated with atrazine, glyphosate, simazine, and rotenone under dark conditions showed a significantly higher H2 production rate than cells without inhibitor treatment (Table 1). A. halophytica cells treated without and with inhibitors under darkness notably produced 4–40 folds higher H2 than those under light (Table 1). Interestingly, the highest H2 production rates at 4.88 ± 0.45 and 46.22 ± 2.20 µmol H2 g−1 dry weight h−1 were obtained in cells treated with 5 µM simazine under light and dark conditions, respectively (Table 1). H2 production of cells treated with simazine under light and darkness was approximately 9 and 3 folds higher than those without simazine treatment, respectively.
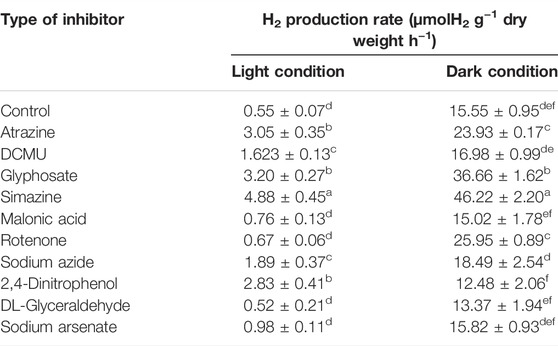
TABLE 1. Effect of various types of inhibitors on H2 production rate of Aphanothece halophytica after 2 h of incubation under the light and darkness. The concentration of all inhibitors used in this study was 5 µM. Data are presented as means ± SD (n = 3). Different letters in columns indicate a significant difference, and the same letter indicates no significant difference according to Duncan’s multiple range test at p < 0.05.
3.2 Effects of Simazine Concentration on Cell Concentration and Chlorophyll a Content
The herbicide simazine functions as a photosynthetic inhibitor, which might affect the growth and pigment content, especially chlorophyll content, in cyanobacterial strains. The results showed that after treatment with 0.05, 0.5, 5, 25, and 50 µM simazine at 30°C under the light, cell and chlorophyll concentrations of A. halophytica were slightly reduced in the first 24 h, and more reduction was observed after 48 h of treatment (Figure 1A,B). In addition, it is noted that the higher the simazine concentration, the stronger its effect on the reduction of cell concentration and chlorophyll content.
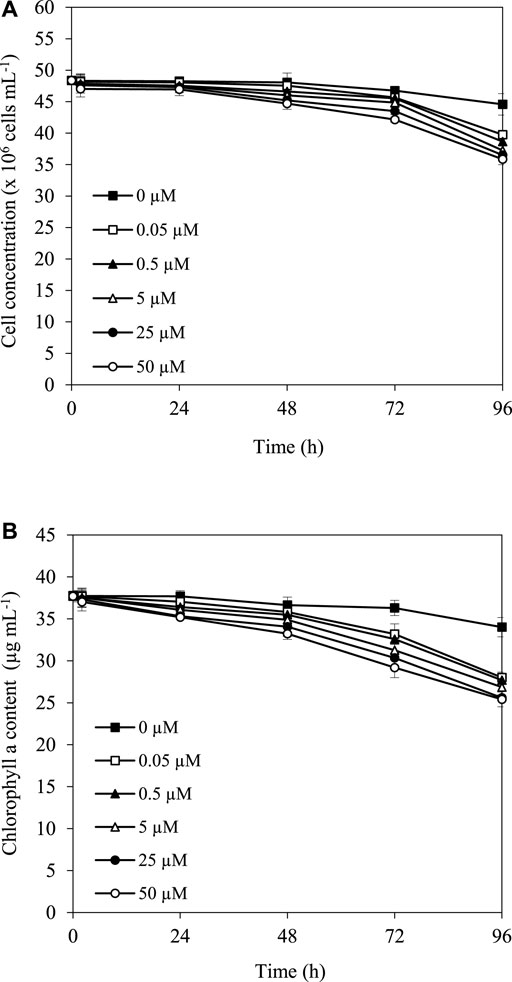
FIGURE 1. Effect of simazine concentration on cell concentration (A) and chlorophyll a content (B) of Aphanothece halophytica after various incubation durations.
3.3 Effect of Simazine Concentration on H2 and O2 Production
H2 and O2 production were measured in A. halophytica treated with various concentrations of simazine (0.05–50 µM) anaerobically under light and dark conditions at 30°C for 2 h. The results showed that a higher concentration of simazine increased H2 production but decreased O2 production under both light and dark conditions (Figure 2A,B). Cells treated with 25 µM simazine under light and dark conditions had an H2 production rate of 10.65 ± 0.53 and 55.23 ± 0.67 µmol H2 g−1 dry weight h−1, accounting for approximately 20 and 4 folds higher production than those without simazine treatment, respectively (Figure 2A). At 50 µM simazine, a significant decrease in H2 production rate was observed (Figure 2A). Moreover, cells treated without and with all concentrations of simazine under darkness produced higher H2 concentrations than those in light (Figure 2A).
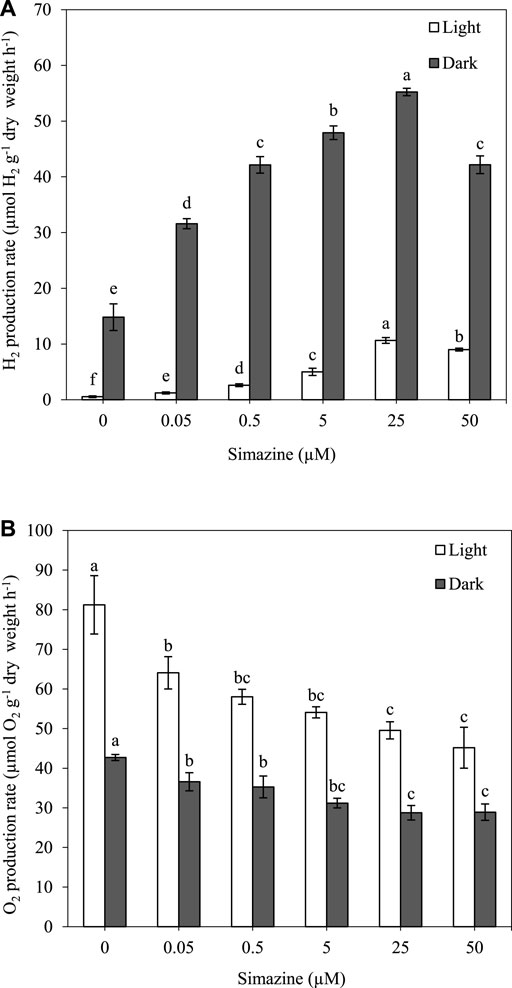
FIGURE 2. Effect of simazine concentration on H2 production rate (A) and O2 production rate (B) by Aphanothece halophytica after 2 h of incubation under the light (open square) and under darkness (solid square). Data are presented as means ± SD (n = 3). Different letters above the columns indicate a significant difference according to Duncan’s multiple range test at p < 0.05.
3.4 Effect of Simazine Concentration on Bidirectional Hydrogenase Activity, Photosynthetic O2 Evolution and Dark Respiration
The measurement of bidirectional hydrogenase activity, photosynthetic O2 evolution, and dark respiration was performed in A. halophytica cells adapted to BG110 for 24 h prior to a treatment with various concentrations of simazine under the light for 2 h. An increase in simazine concentration resulted in a significant increase in bidirectional hydrogenase activity and dark respiration rate but a decrease in photosynthetic O2 evolution (Table 2). The highest bidirectional hydrogenase activity at 53.64 ± 1.81 µmol H2 g−1 dry weight min−1 was observed in cells treated with 25 µM simazine (Table 2). Cells treated with 50 µM simazine showed lower bidirectional hydrogenase activity than those with 25 µM simazine (Table 2). This corresponded with the results of H2 production seen in Figure 2.

TABLE 2. Effect of simazine concentrations on bidirectional hydrogenase activity, photosynthetic O2 evolution, and dark respiration rate of Aphanothece halophytica after 2 h of treatment under the light. Data are presented as means ± SD (n = 3). Different letters on the columns indicate the significant difference, and the same letter indicates no significant difference according to Duncan’s multiple range test at p < 0.05.
3.5 Long-Term Dark Fermentative H2 Production
Long-term dark fermentative H2 production was determined in A. halophytica cells treated with and without 25 µM simazine for 10 days. The results showed that A. halophytica had maximum H2 accumulation with 356.21 ± 6.04 µmol H2 g−1 dry weight when treating cells with 25 µM simazine under dark anaerobic condition at 24 h (Figure 3). The maximum H2 accumulation was approximately 4 folds higher than that of cells without simazine treatment. No H2 production was observed in the negative control containing only 25 µM simazine.
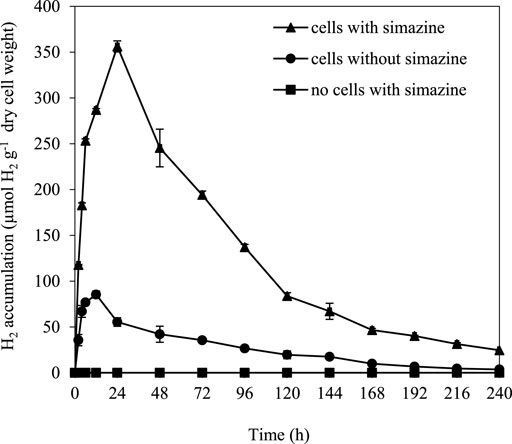
FIGURE 3. Long-term H2 accumulation of Aphanothece halophytica treated with and without 25 µM simazine during 10 days of dark anaerobic incubation. Aphanothece halophytica grown in BG11 for 7 days was harvested by centrifugation and suspended in BG110. Cells were incubated in BG110 under the light for 24 h before treatment with 25 µM simazine. Cells were purged with argon for 10 min and incubated at 30°C for 10 days under darkness. Cells without simazine treatment and simazine without cells were used as controls.
4 Discussion
Simazine has been shown as a popular algicide for controlling the growth of both unicellular and attached filamentous algae (Snow, 1963). It can inhibit photosynthetic electron transport by binding to the plastoquinone B (QB) binding site on the D1 protein of PS II, resulting in an interruption of CO2 fixation and production of ATP and NAD(P)H (Ahrens, 1994). Until now, only few studies have reported on the effect of simazine on H2 production by cyanobacteria. Previously, the N2-fixing filamentous cyanobacterium Nostoc muscorum treated with 2 µM simazine in the light produced approximately 2 folds higher H2 than the untreated cells. However, simazine did not affect acetylene reduction or nitrogenase activity in this cyanobacterium. The increased H2 production was due to the partial lowering of O2 in the cell, thus preventing oxidative H2 consumption (Spiller et al., 1978).
In this study, other inhibitors for photosynthetic electron transport such as atrazine and glyphosate could also induce H2 production by A. halophytica under both light and dark conditions (Table 1), indicating that they could inhibit electron transport in photosynthetic and/or other metabolic pathways. This reduces the number of electrons in photosystem II activity, resulting in a decrease in O2 evolution and finally promoting H2 production. Atrazine inhibits photosynthetic activity by blocking electron transport during the Hill reaction of PSII (Suresh Kumar et al., 2014). However, atrazine affects algae with a wide variety of responses depending on concentrations, duration of exposure, and type of algal species (Tang et al., 1998). Similarly, glyphosate was found to interrupt the photosynthetic electron transport and O2 evolution in both wild-type and mutant cells of Anabaena doliolum (Shikha and Singh, 2004). Although these inhibitors play a similar role in interrupting photosynthesis, they show such a difference in affinity for binding substrate and inhibitory activity.
Interestingly, DCMU induced H2 production by A. halophytica only upon illumination (Table 1). On the other hand, a known photosystem II inhibitor, carbonyl cyanide m-chlorophenyl hydrazine (CCCP), has been reported to induce H2 production by A. halophytica under both light and dark conditions (Pansook et al., 2019b). This is because photosystem II, a target of inhibition by DCMU, is functional upon illumination, whereas CCCP acts not only as a photosystem II inhibitor but also as an uncoupling agent of oxidative phosphorylation, which takes place under both illumination and darkness. The previous study showed that H2 production by Oscillatoria chalybea and Synechocystis sp. PCC6803 was enhanced in cells treated with 5 µM CCCP (Abdel-Basset and Bader, 1998), whereas the marine green alga Platymonas helgolandica var. tsingtaoensis increased H2 production due to the complete PSII inhibition by 50 µM DCMU (Zhang et al., 2012). Likewise, the cyanobacterium Anabaena cylindrica treated with 1.0 µM DCMU enhanced H2 production, which was partly due to the low level of O2 content (Chen et al., 2013), and DCMU at 10 mM increased 1.5-fold H2 production by the cyanobacterium Desertifilum sp. IPPAS B-1220 (Kossalbayev et al., 2020). Other inhibitors including the respiration inhibitor malonic acid, a CO2 fixation inhibitor glyceraldehyde, and a Krebs cycle inhibitor sodium arsenate did not induce H2 production rate by A. halophytica, suggesting no involvement of these inhibitors in H2 metabolism. Some inhibitors, such as a respiration inhibitor sodium azide and an inhibitor of uncoupling agent of oxidative phosphorylation 2,4-dinitrophenol (DNP), induced H2 production only under light condition, whereas a respiration inhibitor rotenone induced H2 production only under dark condition (Table 1). It was previously reported that Cyanothece sp. could increase the H2 production rate in cells treated with 50 µM 2,4-dinitrophenol under darkness (Skizim et al., 2012).
Normally, simazine concentrations used for controlling algal and cyanobacterial growth range from 0.5 to 1.0 mg L−1 or from 2.5 to 5 µM (Suresh Kumar et al., 2014). In this study, a high concentration of simazine and long-term incubation resulted in a decrease in cell and chlorophyll concentrations (Figure 1A,1B). These results indicated the toxicity of simazine due to the inhibition of electron transport in photosynthesis, thus resulting in a decrease in cell concentration and intracellular pigment contents, especially chlorophyll a. These results are in line with the previous study on filamentous cyanobacteria Anabaena circinalis and Anabaena variabilis; and green algae Protosiphon botryoides and Spirogyra jurgensii, showing that simazine inhibited their growth and reduced chlorophyll content (O’Neal and Lembi, 1983; Millie et al., 1992; Kobbia et al., 2001). In this study, simazine exhibited toxicity to cell growth and affected intracellular pigments of A. halophytica under long-term treatment.
Our results indicated the importance of simazine concentration on photosynthetic activity and H2 metabolism of A. halophytica cells. A high concentration of simazine caused a decrease in O2 concentration due to O2 evolution via oxygenic photosynthesis and an activation of dark respiration rate. The decrease in O2 concentration promoted bidirectional hydrogenase activity, leading to an increase in H2 production. Under the light, simazine was shown to interrupt photosynthetic electron transport at photosystem II by displacing QB from its binding site on the D1 protein (O’Neal and Lembi, 1983), resulting in an inhibition of photosynthetic activity or photosynthetic O2 evolution. This caused a reduction in O2 concentration, thereafter promoting bidirectional hydrogenase activity. Under dark anaerobic conditions, where photosynthetic activity is inactive, simazine promoted the dark respiration rate, providing higher O2 consumption and higher level of NAD(P)H from carbohydrate degradation. The lower O2 concentration activated bidirectional hydrogenase activity, and NAD(P)H gave electrons to hydrogenase, finally resulting in higher H2 production by A. halophytica. Since A. halophytica produces H2 under dark anaerobic conditions via a catabolism of storage glycogen (Taikhao et al., 2015), the effect of simazine on glycogen content was investigated. Unfortunately, no significant differences in the glycogen content of cells treated and untreated with 25 µM simazine were found (data not shown). Apart from being a photosystem II inhibitor, simazine might affect other metabolisms, including H2 metabolism. In Protosiphon botryoides and Anabaena variabilis, simazine treatment was shown to increase respiration rate (Kobbia et al., 2001). In oat plant (Avena sativia L. var. Seminole), simazine treatment showed a decrease in protein synthesis under darkness (Singh and West, 1967). However, too high a concentration of simazine (50 µM) is likely to be toxic to A. halophytica cells. This study confirmed the capability of the effective inhibitor simazine for enhancement of dark fermentative H2 production by A. halophytica. However, during long-term incubation after 24 h of simazine treatment, cells reduced dark fermentative H2 production due to the decreased action of simazine and the toxicity of simazine to cyanobacterial growth and cellular metabolism (O’Neal and Lembi, 1983).
5 Conclusion
The photobiological H2 production by A. halophytica was significantly enhanced by treatment with atrazine, DCMU, glyphosate, simazine, sodium azide, and 2,4-dinitrophenol, whereas the dark fermentative H2 production was significantly increased in cells treated with atrazine, glyphosate, simazine, and rotenone. Among all the inhibitors, simazine is the best inhibitor to enhance H2 production by A. halophytica under both light and dark conditions. High simazine concentration and long-term incubation reduced cell concentration and chlorophyll content due to its cell toxicity. The optimal concentration of simazine for H2 production by A. halophytica was 25 µM. Simazine treatment reduced photosynthetic O2 evolution, resulting in an increase in bidirectional hydrogenase activity. In addition, simazine induced O2 consumption by enhancing the dark respiration rate. These incidences promoted H2 production in A. halophytica.
Data Availability Statement
The original contributions presented in the study are included in the article/Supplementary Material; further inquiries can be directed to the corresponding author.
Author Contributions
SaP contributed to conception and design of the study. SaP received the research grant. SuP performed the experiments and statistical analysis. SuP wrote the first draft of the manuscript. SuP and SaP wrote sections of the manuscript. All authors contributed to manuscript revision, and read and approved the submitted version.
Funding
This work was supported by the School of Science, King Mongkut's Institute of Technology Ladkrabang (KMITL) (Grant Number 2562-01-05-34).
Conflict of Interest
The authors declare that the research was conducted in the absence of any commercial or financial relationships that could be construed as a potential conflict of interest.
Publisher’s Note
All claims expressed in this article are solely those of the authors and do not necessarily represent those of their affiliated organizations, or those of the publisher, the editors, and the reviewers. Any product that may be evaluated in this article, or claim that may be made by its manufacturer, is not guaranteed or endorsed by the publisher.
References
Abdel-Basset, R., and Bader, K. P. (1998). Physiological Analyses of the Hydrogen Gas Exchange in Cyanobacteria. J. Photochem. Photobiol. B Biol. 43 (2), 146–151. doi:10.1016/s1011-1344(98)00097-9
Ahrens, W. (1994). Herbicide Handbook of the Weed Science Society of America. Champaign, IL: Weed Science Society of America, 270–274.
Burrows, E. H., Chaplen, F. W. R., and Ely, R. L. (2011). Effects of Selected Electron Transport Chain Inhibitors on 24-h Hydrogen Production by Synechocystis Sp. PCC 6803. Bioresour. Technol. 102 (3), 3062–3070. doi:10.1016/j.biortech.2010.10.042
Chen, M., Zhang, Z., Wang, C., Zhang, L., Li, J., Chang, S., et al. (2013). Improving Conversion Efficiency of Solar Energy to Electricity in Cyanobacterial PEMFC by High Levels of Photo-H2 Production. Int. J. Hydrogen Energy 38 (31), 13556–13563. doi:10.1016/j.ijhydene.2013.08.020
Chen, M., Li, J., Zhang, L., Chang, S., Liu, C., Wang, J., et al. (2014). Auto-flotation of Heterocyst Enables the Efficient Production of Renewable Energy in Cyanobacteria. Sci. Rep. 4, 3998. doi:10.1038/srep03998
Eroglu, E., and Melis, A. (2011). Photobiological Hydrogen Production: Recent Advances and State of the Art. Bioresour. Technol. 102 (18), 8403–8413. doi:10.1016/j.biortech.2011.03.026
Garlick, S., Oren, A., and Padan, E. (1977). Occurrence of Facultative Anoxygenic Photosynthesis Among Filamentous and Unicellular Cyanobacteria. J. Bacteriol. 129 (2), 623–629. doi:10.1128/jb.129.2.623-629.1977
Khetkorn, W., Baebprasert, W., Lindblad, P., and Incharoensakdi, A. (2012). Redirecting the Electron Flow towards the Nitrogenase and Bidirectional Hox-Hydrogenase by Using Specific Inhibitors Results in Enhanced H2 Production in the Cyanobacterium Anabaena Siamensis TISTR 8012. Bioresour. Technol. 118, 265–271. doi:10.1016/j.biortech.2012.05.052
Kobbia, I. A., Battah, M. G., Shabana, E. F., and Eladel, H. M. (2001). Chlorophyll a Fluorescence and Photosynthetic Activity as Tools for the Evaluation of Simazine Toxicity to Protosiphon Botryoides and Anabaena Variabilis. Ecotoxicol. Environ. Saf. 49 (2), 101–105. doi:10.1006/eesa.2000.1955
Kossalbayev, B. D., Tomo, T., Zayadan, B. K., Sadvakasova, A. K., Bolatkhan, K., Alwasel, S., et al. (2020). Determination of the Potential of Cyanobacterial Strains for Hydrogen Production. Int. J. Hydrogen Energy 45 (4), 2627–2639. doi:10.1016/j.ijhydene.2019.11.164
Levin, D., Pitt, L., and Love, M. (2004). Biohydrogen Production: Prospects and Limitations to Practical Application. Int. J. Hydrogen Energy 29 (2), 173–185. doi:10.1016/s0360-3199(03)00094-6
MacKinney, G. (1941). Absorption of Light by Chlorophyll Solutions. J. Biol. Chem. 140 (2), 315–322. doi:10.1016/s0021-9258(18)51320-x
McKinlay, J. B., and Harwood, C. S. (2010). Photobiological Production of Hydrogen Gas as a Biofuel. Curr. Opin. Biotechnol. 21 (3), 244–251. doi:10.1016/j.copbio.2010.02.012
Millie, D. F., Hersh, C. M., and Dionigi, C. P. (1992). Simazine-induced Inhibition in Photoacclimated Populations of Anabaena Circinalis (Cyanophyta)1. J. Phycol. 28 (1), 19–26. doi:10.1111/j.0022-3646.1992.00019.x
O'Neal, S. W., and Lembi, C. A. (1983). Effect of Simazine on Photosynthesis and Growth of Filamentous Algae. Weed Sci. 31 (6), 899–903. doi:10.1017/s0043174500070958
Pansook, S., Incharoensakdi, A., and Phunpruch, S. (2016). Hydrogen Production by Immobilized Cells of Unicellular Halotolerant Cyanobacterium Aphanothece Halophytica in Alginate Beads. KKU Res. J. 22 (1), 248–255. doi:10.14456/kkurj.2016.34
Pansook, S., Incharoensakdi, A., and Phunpruch, S. (2019a). Enhanced Dark Fermentative H2 Production by Agar-Immobilized Cyanobacterium Aphanothece Halophytica. J. Appl. Phycol. 31, 2869–2879. doi:10.1007/s10811-019-01822-9
Pansook, S., Incharoensakdi, A., and Phunpruch, S. (2019b). Effects of the Photosystem II Inhibitors CCCP and DCMU on Hydrogen Production by the Unicellular Halotolerant Cyanobacterium Aphanothece Halophytica. Sci. World J. 2019, 1–10. doi:10.1155/2019/1030236
Phunpruch, S., Taikhao, S., and Incharoensakdi, A. (2016). Identification of Bidirectional Hydrogenase Genes and Their Co-transcription in Unicellular Halotolerant Cyanobacterium Aphanothece Halophytica. J. Appl. Phycol. 28 (2), 967–978. doi:10.1007/s10811-015-0664-8
Rippka, R., Stanier, R. Y., Deruelles, J., Herdman, M., and Waterbury, J. B. (1979). Generic Assignments, Strain Histories and Properties of Pure Cultures of Cyanobacteria. Microbiology 111 (1), 1–61. doi:10.1099/00221287-111-1-1
Shikha , , and Singh, D. P. (2004). Influence of Glyphosate on Photosynthetic Properties of Wild Type and Mutant Strains of Cyanobacterium Anabaena Doliolum. Curr. Sci. 86 (4), 571–576.
Singh, R. P., and West, S. H. (1967). Influence of Simazine on Chloroplast Ribonucleic Acid, and Protein Metabolism. Weeds 15 (1), 31–34. doi:10.2307/4041062
Skizim, N. J., Ananyev, G. M., Krishnan, A., and Dismukes, G. C. (2012). Metabolic Pathways for Photobiological Hydrogen Production by Nitrogenase- and Hydrogenase-Containing Unicellular Cyanobacteria Cyanothece. J. Biol. Chem. 287 (4), 2777–2786. doi:10.1074/jbc.m111.302125
Snow, J. R. (1963). A Preliminary Report on the Control of Pithophora with Simazine. Proc. So. Weed Conf. 16, 329–335.
Spiller, H., Ernst, A., Kerfin, W., and Böger, P. (1978). Increase and Stabilization of Photoproduction of Hydrogen in Nostoc Muscorum by Photosynthetic Electron Transport Inhibitors. Z. für Naturforsch. C 33 (7-8), 541–547. doi:10.1515/znc-1978-7-815
Srirangan, K., Pyne, M. E., and Perry Chou, C. (2011). Biochemical and Genetic Engineering Strategies to Enhance Hydrogen Production in Photosynthetic Algae and Cyanobacteria. Bioresour. Technol. 102 (18), 8589–8604. doi:10.1016/j.biortech.2011.03.087
Suresh Kumar, K., Dahms, H.-U., Lee, J.-S., Kim, H. C., Lee, W. C., and Shin, K.-H. (2014). Algal Photosynthetic Responses to Toxic Metals and Herbicides Assessed by Chlorophyll a Fluorescence. Ecotoxicol. Environ. Saf. 104, 51–71. doi:10.1016/j.ecoenv.2014.01.042
Taikhao, S., Junyapoon, S., Incharoensakdi, A., and Phunpruch, S. (2013). Factors Affecting Biohydrogen Production by Unicellular Halotolerant Cyanobacterium Aphanothece Halophytica. J. Appl. Phycol. 25 (2), 575–585. doi:10.1007/s10811-012-9892-3
Taikhao, S., Incharoensakdi, A., and Phunpruch, S. (2015). Dark Fermentative Hydrogen Production by the Unicellular Halotolerant Cyanobacterium Aphanothece Halophytica Grown in Seawater. J. Appl. Phycol. 27 (1), 187–196. doi:10.1007/s10811-014-0292-8
Takabe, T., Incharoensakdi, A., Arakawa, K., and Yokota, S. (1988). CO2 Fixation Rate and RuBisCO Content Increase in the Halotolerant Cyanobacterium, Aphanothece Halophytica, Grown in High Salinities. Plant Physiol. 88, 1120–1124. doi:10.1104/pp.88.4.1120
Tang, J., Hoagland, K. D., and Siegfried, B. D. (1998). Uptake and Bioconcentration of Atrazine by Selected Freshwater Algae. Environ. Toxicol. Chem. 17 (6), 1085–1090. doi:10.1002/etc.5620170614
Zhang, X. K., Haskell, J. B., Tabita, F. R., and Van Baalen, C. (1983). Aerobic Hydrogen Production by the Heterocystous Cyanobacteria Anabaena Spp. Strains CA and 1F. J. Bacteriol. 156 (3), 1118–1122. doi:10.1128/JB.156.3.1118-1122.1983
Keywords: H2 production, cyanobacteria, Aphanothece halophytica, inhibitor, simazine
Citation: Pansook S, Incharoensakdi A and Phunpruch S (2022) Simazine Enhances Dark Fermentative H2 Production by Unicellular Halotolerant Cyanobacterium Aphanothece halophytica. Front. Bioeng. Biotechnol. 10:904101. doi: 10.3389/fbioe.2022.904101
Received: 25 March 2022; Accepted: 13 June 2022;
Published: 15 July 2022.
Edited by:
Susana Rodriguez-Couto, LUT University, FinlandReviewed by:
Takashi Osanai, Meiji University, JapanAshwani Rai, Banaras Hindu University, India
Hakuto Kageyama, Meijo University, Japan
Copyright © 2022 Pansook, Incharoensakdi and Phunpruch. This is an open-access article distributed under the terms of the Creative Commons Attribution License (CC BY). The use, distribution or reproduction in other forums is permitted, provided the original author(s) and the copyright owner(s) are credited and that the original publication in this journal is cited, in accordance with accepted academic practice. No use, distribution or reproduction is permitted which does not comply with these terms.
*Correspondence: Saranya Phunpruch, saranya.ph@kmitl.ac.th