- Institute of Microbiology and Molecular Genetics, University of the Punjab, Lahore, Pakistan
Salmonella Gallinarum causes fowl typhoid in poultry leading to a huge economic loss to the poultry industry. The large virulence plasmid of S. gallinarum has been associated with various systemic infections in poultry. A five-gene spanning region (spvRABCD) of 7.8 kb on the large plasmid mainly confers virulence to the bacteria. However, the exact role of these genes in virulence has not been elucidated yet. SpvB exhibits delayed cell death by preventing actin polymerization followed by apoptosis during intracellular infection. The specific role of SpvB in causing the disease is not known yet. In the current study, the SpvB gene was deleted through CRISPR/Cas9 method from a large virulent plasmid of locally isolated S. gallinarum strain (SG18). The homology-directed repair method was used for complete deletion of SpvB gene using the modified pCas9 plasmid. The SpvB-deleted S. gallinarum strain (ΔSpvB_SG18), when tested for its virulence in broiler chicken showed no diseases signs and mortality. In addition, the avirulent strain does not affect the bird’s weight and was rapidly cleared from the liver after infection. However, it cleared from the intestine only after 4–5 days, which suggests that the ΔSpvB_SG18 strain is unable to invade from the intestine to the liver. This is the first study to report a complete gene deletion from the S. gallinarum virulent plasmid and its effect. This method will be useful for the deletion of virulent genes from S. gallinarum, to study their role in pathogenesis, and to prepare an effective vaccine strain for controlling fowl typhoid in poultry.
Introduction
Fowl typhoid is a severe, systemic disease of poultry caused by Salmonella enterica subsp. enterica serovar gallinarum biovar gallinarum (Shivaprasad et al., 2008). Although, S. gallinarum has been eradicated in developed countries, it is still a problem in developing countries (Andino and Hanning, 2015) and leads to a massive loss of poultry flocks (Mamman et al., 2014; Geetha and Palanivel, 2018). Pakistan’s poultry industry contributes 34% to total meat production (Islamic Markets, 2019), with a significant contribution to the national GDP (1.3%) (Hussain et al., 2015). Various virulence factors of S. gallinarum are claimed to be responsible for causing fowl typhoid; nevertheless, less is known about the genetics of S. gallinarum’s pathogenicity (Li et al., 1993). Virulent factors in enteric bacteria are often encoded by plasmids; however, the exact role of the virulent plasmids in pathogenesis is not clear yet (Singh et al., 2018). Previously, the large virulent plasmid (85 kb) of S. gallinarum has shown to be mainly involved in causing systemic infection in poultry; however, the exact function of the virulent genes in their pathogenicity has not been elucidated yet (Barrow et al., 1987). The spv locus on the large virulent plasmid harboring five genes (spvRABCD), is highly conserved among Salmonella serovars and is sufficient to restore the virulence, hence causes mortality in birds. However, the sequencing of spv genes has provided no clues about their function (Rotger and Casadesús, 1999; Jones et al., 2001). Although, the SpvB mutant of Salmonella dublin was found avirulent form in mice (Roudier et al., 1992), while another experiment has shown that SpvB together with SpvC is sufficient to confer the virulence to Salmonella typhimurium in mice (Käppeli et al., 2011; Kidwai et al., 2013). However, the specific role of the SpvB gene alone in causing the disease in poultry is not determined yet (Cheng and Wiedmann, 2019), as compared to other plasmid-encoded virulent genes, particularly considering the previous studies showing that both SpvB and SpvC are essential for mediating the toxin-associated phenotype in vivo (Matsui et al., 2001a; Guiney and Fierer, 2011). On the other hand, the serovars gallinarum does not cause systemic diseases in experimentally infected mice or other laboratory mammals (Barrow et al., 1994), which is an important exception. Therefore, the virulent role of SpvB in S. gallinarum in causing poultry infection needs to be determined.
CRISPR/Cas9 is an efficient tool for gene editing of bacterial genomes. However, the success rate of genome editing in bacterial plasmids through CRISPR/Cas9 is very low, as a single unedited plasmid (if remaining) can make their replicas, thus restoring their natural sequences (Tagliaferri et al., 2020). Recently, the CRISPR/Cas9 system has been applied for the deletion of virulent genes from genomic DNA (Jiang et al., 2013) or the curing of virulent plasmids (Wang et al., 2019; Tagliaferri et al., 2020), from clinical pathogens. Here, we describe the modified CRISPR/Cas9 system to delete the plasmid-based SpvB gene from S. gallinarum, isolated from local poultry production. The SpvB gene encodes ADP-ribosylating toxins, which destabilize cytoskeletons in host cells, playing an important role in pathogenesis of Salmonella spp. (Skyberg et al., 2006). Moreover, 9R strain of S. gallinarum (live attenuated) has been used worldwide as a vaccine since 2001; however, due to the persistent evolution of the pathogen, the vaccine strain may not provide sufficient protection against S. gallinarum (Lee et al., 2007; Kim et al., 2020). Therefore, the current study aimed to produce an avirulent strain by deleting the SpvB gene from S. gallinarum virulent plasmid through the CRISPR/Cas9 system. The deletion mutant was further analyzed for their in vivo disease-causing potential in poultry.
Materials and Methods
Bacterial Strains, Plasmids, and Chemical Reagents
S. gallinarum strains were isolated from poultry samples with fowl typhoid symptoms and identified by our laboratory [data not shown]. E. coli top 10 cells were used for transformation and propagation of recombinant plasmids. For cloning of the DNA editing template, pETT22b (+) vector was used, while pCasSA (Plasmid #98211) and pCas9 (Plasmid #42876) plasmids purchased from addgene were used for gRNA cloning. Plasmid, genomic DNA, and gel purification kits were purchased from Thermoscientific, United Kingdom. Golden gate (E1601) and HiFi assembly kits (E2621, NEB, New England Biolab) were used for the cloning of gRNA and DNA editing templates, respectively. The chemicals and the media used were purchased from Alpha Biosciences, United States. Sanger sequencing and oligos synthesis were commercially performed from Macrogen, Korea.
Oligos Designing and PCR Amplification
The gene sequence of SpvB (GenBank ID: D14490.1) was retrieved from NCBI. For targeted deletion of the SpvB gene from a large size plasmid of S. gallinarum, gRNA was designed though the CHOP CHOP online tool. The gRNA (OligoI & II) sequence showing high target efficiency was synthesized (Table 1).
Preparation of a DNA Editing Template
In order to clone the DNA editing template in the pET22b(+) vector, one kb upstream fragment (homologous arm1) of the SpvB gene was amplified by H1F and H1R as forward and reverse primers, respectively. Similarly, one kb fragment (homologous arm2) downstream of the SpvB gene was amplified by H2F and H2R as forward and reverse primers, respectively. The homologous arms were then assembled through the HiFi kit according to the manufacturer’s protocol and the fused product of homologous arms were PCR-amplified through H1F and H2R as forward and reverse primers, respectively, followed by cloning in the pET22b(+) vector (already restricted with XbaI and XhoI), by using the HiFi assembly kit. The HiFi assembly mixture was electroporated into Top10 electrocompetent cells at 1,700 V for 5.1 ms using Eppendorp eporator (Eppendorp AG, 22,331, Hamburg). Positive transformants were confirmed through colony PCR using H1F and H2R as forward and reverse primers, respectively. The recombinant plasmid containing DNA editing template (pET_HAs) was isolated from the positive transformants and further confirmed through restriction digestion by using XbaI and XhoI and verified by Sanger sequencing.
Cloning of gRNA in pCas9 and pCasSA Vectors
In order to clone gRNA oligos (OligoI and OligoII) in pCas9 and pCasSA vectors, phosphorylation of the guide RNA oligos were performed, followed by cloning in the corresponding vectors through the golden gate assembly kit (Engler et al., 2009) using E. coli Top10 cells. The cloning of gRNA in pCas9 (pCas9-g) and pCasSA plasmid (pCasSA-g) were confirmed through colony PCR using OligoI as forward for both vectors, while C1 and C2 as reverse primers for pCas9-g and pCasSA-g, respectively, and were further confirmed through Sanger sequencing. The sequences of all the primers and oligos are given in Table 1. Details of the recombinant plasmids produced are given in Supplementary Table S2.
Electroporation of S. gallinarum With Recombinant Plasmids
The recombinant plasmids pCas9-g and pET-HAs carrying the gRNA and DNA editing templates, respectively, were co-electroporated into electrocompetent S. gallinarum and spread on an agar plate containing chloramphenicol and ampicillin. Similarly, pCasSA-g and pET-HAs electroporated into S. gallinarum were spread on kanamycin (60 μg ml−1) and ampicillin (100 μg ml−1) agar plates, followed by incubation at 37°C overnight. Plasmids without gRNA and DNA editing templates were also electroporated as negative controls.
Screening of SpvB Gene-Deleted Strains
Randomly, 25 colonies of S. gallinarum co-transformed with pCas9-g and pET-HAs plasmids and 20 colonies of S. gallinarum transformed with pCasSA-g and pET_HAs plasmids were picked and screened through colony PCR, for the successful deletion of the SpvB gene using Screen_F and Screen_R as forward and reverse primers, respectively (Table 1). These primers were designed to bind 50 bp upstream and downstream regions of the homologous arms flanking the SpvB gene, which allows the specific binding of these primers only with the large virulent plasmid of S. gallinarum. The large virulent plasmid of S. gallinarum was used as control.
Animal Trials on Poultry to Evaluate Virulence
S. gallinarum wild type and engineered strains (ΔSpvB_SG18) were used to infect chickens. Disease-free male Cobb 500 broiler chickens (n = 120) were used in this study. Day old chicks were obtained from a commercial hatchery. The birds were housed in the pre-sterile cages in an environmentally controlled room with a 16-h light period. Birds were vaccinated against ND (new castle disease) and IB (Infectious bronchitis) on the first day and distributed randomly into three groups, SG_WT (S. gallinarum positive control), SG_Healthy (not infected with any pathogens), and ΔSpvB_SG18, each with four equal sized replicates containing 10 birds. The birds were screened for Salmonella infection on day 7 through cloacal swabs or fresh fecal samples. Water and antibiotic-free feed were provided to the birds throughout the span of the experiment. At 16 days of age, the birds were inoculated orally with 0.5 ml of normal saline containing approximately 1 × 108 colony-forming units (CFU) of each strain. The birds were monitored for any gross signs and symptoms on a daily basis. The chickens were also weighed three times per week during the experiment time to document any changes in weight gain post infection. Clinical signs and postmortem examinations of the dead birds along with severely ill birds were performed for 3 weeks post infection to evaluate gross pathologies. Post mortem examination of dead and severely ill birds were performed from day 7.
Results and Discussion
Role of the SpvB Gene in Virulence
A total of 20 strains of S. gallinarum (SG1-20) isolated from poultry samples were screened for possession of the SpvB gene by using SpvB_F and SpvB_R as forward and reverse primers, respectively (Table 1). All the tested strains showed a successful amplification of the SpvB gene (Supplementary Figure S1), indicating the prevalence of the SpvB gene commonly found in S. gallinarum isolated from local poultry samples. The majority of S. gallinarum virulence genes are distributed in genomic DNA (Asten and Dijk, 2005; Blondel et al., 2010). However, there is no clear evidence available showing a single virulence gene over genome of S. gallinarum responsible for mortality in chickens (Shah et al., 2005). Many genes are responsible for the virulence of S. gallinarum in poultry. A single gene has been shown to reduce virulence significantly in S. gallinarum. For example, crp, the global transcriptional regulator is associated with virulence and deletion of crp made SG avirulent (Rosu et al., 2007; Mitra et al., 2013). Virulence plasmids are required to trigger systemic disease; however, their involvement in the enteric stage of the infection is unclear. Salmonella virulence plasmids are heterogeneous in size (50–90 kb), but they all share a 7.8 kb region, spv, required for bacterial multiplication in the reticulo endothelial system. The spv region harbors five genes, spvR, A, B, C, and D. However, it is still unclear which gene is mainly responsible for virulence, though the SpvB sequence shows a certain degree of similarity to the Ace toxin of Vibrio cholerae, which contributes to diarrhea (Trucksis et al., 1993). SpvB and C genes have been reported to cause virulence in S. typhimurium in a mouse infection model (Roudier et al., 1992; Matsui et al., 2001b). A recent study has shown that the length of the linker connecting N and C terminal domains of SpvB increases pathogenicity of S. gallinarum in chickens (Kim et al., 2020). Nevertheless, there is no evidence available of SpvB’s role in causing FT in poultry. We aimed to explore the role of SpvB gene of S. gallinarum in causing fowl typhoid and mortality in chickens, by deleting a complete SpvB gene through the engineered CRISPR/Cas9 system, as shown in the schematic diagram (Figure 1). The SpvB gene sequence retrieved from the large virulent plasmid isolated from S. gallinarum, sequenced through NGS, showed >98% homology with the previously reported SpvB gene from SG18 strain of S. gallinarum and other Salmonella Sp. (Supplementary Figure S2; Supplementary Table S1).
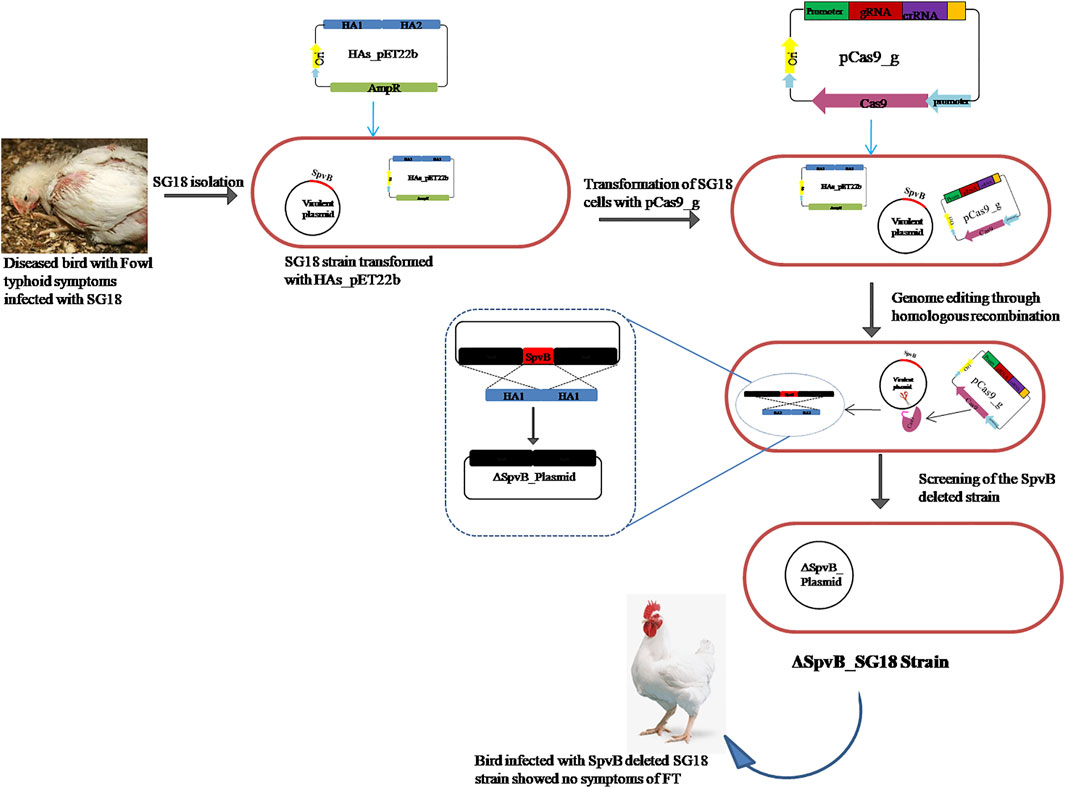
FIGURE 1. Diagrammatic representation of SpvB gene deletion from S. gallinarum large virulent plasmid through engineered CRISPR/Cas9 mediated homologous recombination.
Genome Editing Plasmid Construction
For targeted deletion of the SpvB gene, a spacer RNA targeting SpvB gene were cloned between two direct repeats in pCas9 plasmid (Jiang et al., 2013) and the plasmid was named as pCas9_g (Figure 2C). Similarly, the gRNA was also cloned in the spacer insertion site of pCasSA plasmid under cap1A promoter (Chen et al., 2017) and named as pCasSA_g (Supplementary Figure S3). The expression of the Cas9 protein in pCasSA plasmids was driven by strong rpsL promoters (Cobb et al., 2015). Cloning of gRNA in the respective plasmids was confirmed through PCR and Sanger sequencing (Figures 2A,B). The DNA-editing template containing homologous arms flanking the SpvB gene were used for homologous recombination mediated repair of the double strand DNA break (Figure 3). The gRNA was cloned through the one-step golden gate assembly reaction (Engler et al., 2009), while homology arms were cloned through the one-step HiFi assembly cloning reaction (Gibson et al., 2009) in their respective plasmids. Since, the pCas9 promoters are derived from Gram-negative bacteria, while pCasSA promoters are derived from Gram-positive bacteria, therefore, pCas9 editing efficiency may be higher in S. gallinarum than the pCasSA, while, due to the reduced expression of gRNA and Cas9 protein, the editing efficiency of pCasSA_g may be lower.
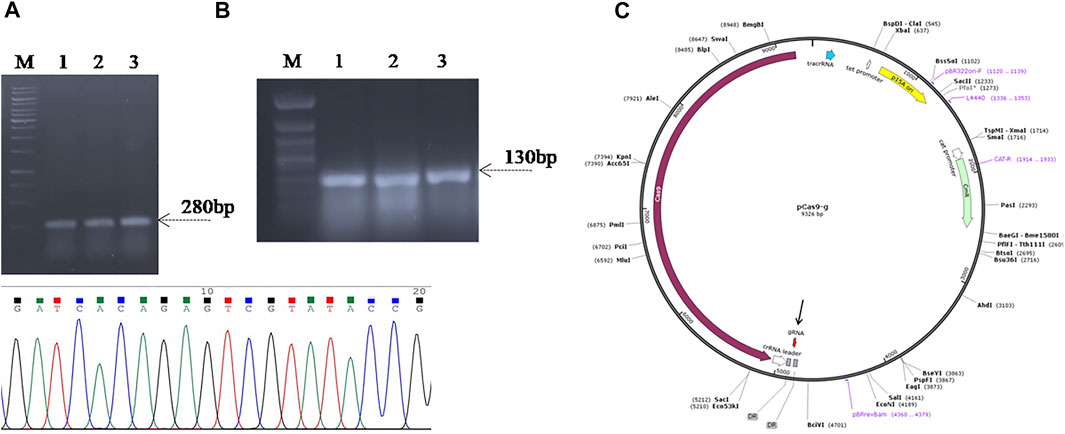
FIGURE 2. Confirmation of spacer RNA (gRNA) cloning in (A) pCas9 (B) and pCasSA vectors through PCR and Sanger sequencing. Lanes 1–3, colony PCR of the Top10 cells carrying recombinant pCas9-g showed amplification of a 180 bp fragment suggest the cloning of gRNA in pCas9. Lanes 4–6, colony PCR of the cells carrying the recombinant pCasSA-g plasmid amplified 130 bp fragment suggest the cloning of gRNA in pCasSA, which is further confirmed through Sanger sequencing shown in the chromatogram. (C) Plasmid map of recombinant pCas9-g carrying spacer RNA targeting the SpvB gene. The gRNA (colored red) is denoted with an arrow. Lane M, Gene Ruler 1 kb DNA ladder (SM0311), Lane M*, Gene Ruler 50 bp DNA ladder (SM0371) was used as the DNA ladder.
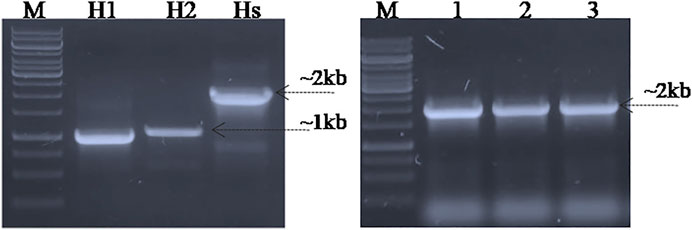
FIGURE 3. PCR amplification and cloning of homologous arms in the pET22b (+) vector. Lane H1; homologous arm1, Lane H2; homologous arm 2, Lane Hs; fused homologous arms, Lane P; PCR amplified fused homologous arms was used as positive control, Lane 1–3; Colony PCR of Top10 cells carrying fused homologous arms as the DNA editing template cloned in the pET22b (+) vector (pET_HAs).
Electroporation of S. gallinarum With Recombinant Plasmids
In order to delete the SpvB gene from the large virulent plasmid of S. gallinarum, the SG18 strain was co-electroporated with the pCas9_g and pCasSA_g vectors, in combination with the pET_HAs vector. The transformed cells showed growth on an agar plate containing all respective antibiotics. The gRNA directs the Cas9 endonuclease to the SpvB gene locus that is adjacent to a PAM, which produces a double-stranded nick at the gRNA bound locus of the plasmid genome. The cell repair machinery repairs the double-stranded break. However, the presence of DNA editing templates delete the targeted SpvB gene by integrating the homologous arms flanking the SpvB gene through homologous recombination (Chen et al., 2017). Furthermore, to test the toxicity of pCas9, empty pCas9 and pCasSA plasmids were electroporated into SG18 cells, which resulted in more than 300 colonies per plate, suggesting no off-target cleavage of bacterial genomes. More than 100 colonies were obtained after electroporation with the pCas9_g plasmids, while >200 colonies were obtained when the cells were electroporated with the pCasSA_g plasmid, suggesting a lower DNA editing efficiency of the pCasSA-g plasmid in S. gallinarum.
Screening for SpvB Gene Deletion in S. gallinarum
In order to screen the SpvB gene deletion in pCasSA_g and pCas9_g transformed cells, colony PCR was performed using SpvB screening primers. The cells transformed with pCasSA_g and pET_HAs plasmids showed partial deletion of the SpvB gene in 10/25 colonies. Similarly, cells transformed with pCas9_g and pET_HAs plasmids also showed partial deletion of the SpvB gene in 25/25 colonies (Figure 4). The partial deletion may be due to either a low copy number of the DNA editing template or a rapid replication of uncut plasmids. To increase the copy number of the DNA editing template, we first electroporated the SG18 cells with the DNA editing template and confirmed that the isolate carrying pET_HAs plasmid were subsequently electroporated with pCas9_g. The colonies appearing showed 13/25 partial deletion while 12/25 showed a complete deletion of the SpvB gene, confirmed through colony PCR (Figure 5). This 48% complete deletion efficiency was obtained due to subsequent electroporation of DNA-editing plasmids followed by gRNA plasmids. The Sanger sequencing further confirmed that the SpvB gene was completely deleted in the ΔSpvB_SG18 strain, with no mutation in the up and downstream regions of the deleted gene, suggesting that SpvC and A were intact and should be functional in the ΔSpvB_SG18 strain (Supplementary Figures S6, S7). Interestingly, the SG18 cells co-electroporated with two spacer RNAs (pCas9_g and pCas9_g*) targeting distinct regions in the SpvB gene did not show any amplification through the colony PCR (Supplementary Figure S4), which suggests that the double-stranded break produced at two distinct positions in the SpvB gene may not induce effective recombination to achieve the double-stranded break repair, hence it degraded the plasmid (Wang et al., 2016). However, the plasmid isolated from the same colonies showed SpvB amplification, which suggests that, the uncut plasmid replicated to restore their copies, which is contrary to a previous study that claimed gene deletion from plasmid was confirmed through negative colony PCR (Liu et al., 2020). Our results suggest that screening the bacterial colonies for plasmid editing solely through colony PCR may give false-positive results. We further cured the virulent plasmid from SG18 cells, after subsequent rounds of overnight incubation at 42, 43, 44, and 45°C. The plasmid-cured strain was also tested for its virulence in chickens.
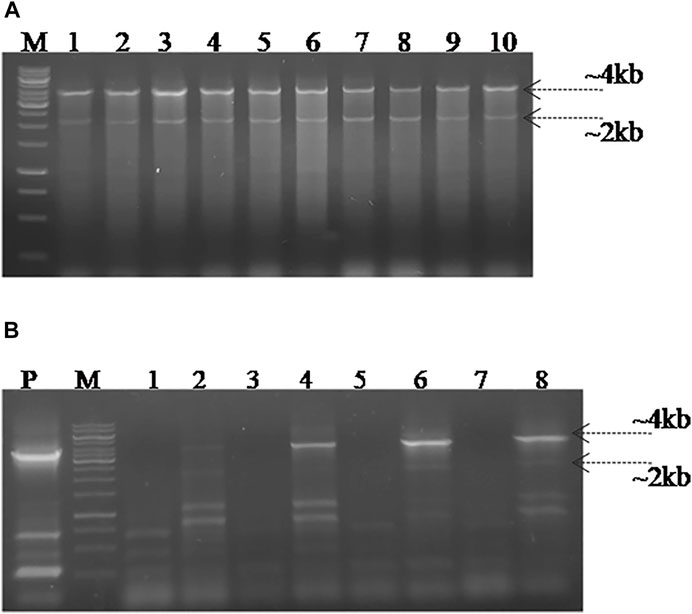
FIGURE 4. Screening for SpvB deletion in SG18 cells co-transformed with pCas9_g and pCasSA_g plasmids in combination with the DNA editing template carrying vector. (A) All the pCas9-g transformed SG18 colonies screened showed a partial deletion of the SpvB gene, while (B) pCasSA-g transformed SG18 cells showed a partial deletion of the SpvB gene in 40% of total cells screened. Lanes 1–10 are the colonies electroporated with corresponding vectors, Lane P; large virulent plasmids isolated from SG18 cells were used as positive control, showing amplification of the SpvB gene (4 kb) through screening primers.
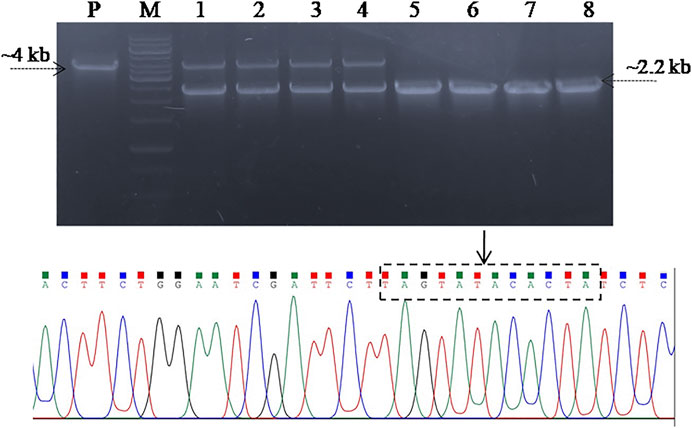
FIGURE 5. Screening SG18 cells for SpvB gene deletion. The cells were primarily electroporated with the pET_HAs vector and after confirmation, subsequently; the DNA editing template-carrying cells were electroporated with pCas9_g vector. PCR-based screening showed that almost 50% cells screened showed a complete deletion of the SpvB gene, while the rest showed a partial deletion as shown in the aforementioned figure. The deleted mutant further confirmed through Sanger sequencing showed successful deletion of the SpvB gene from the large virulent plasmid of S. gallinarum, as shown in the aforementioned chromatogram. The encircled area (denoted by arrow) showing fused homology arms1 and 2 with no SpvB gene.
Effect of SpvB Deletion on S. gallinarum in Mediating Virulence in Chickens
We further determined the effect of SpvB gene deletion from SG18 cells on chicken mortality upon infection. No symptoms of the fowl typhoid disease and mortality were observed in the chickens infected with the ΔSpvB_SG18 strain throughout the 36 days of experimentation, which are in agreement with a previously reported study showing that mutations in SP-2 of S. gallinarum resulted in a loss of virulence in chickens (Jones et al., 2001). However, a total of 52% mortality rate was observed in the group infected with the wild-type SG18 strain after 36 days of post infection (Figure 6), which agreed with previous reports showing 50% mortality caused by S. gallinarum in domestic fowls (Shivaprasad, 2000). Necropsy of dead birds along with severely ill birds infected with SG18 showed classical signs of fowl typhoid (Figure 7) such as anorexia, lethargy, yellowish diarrhea with pasting, liver enlargement (Saha et al., 2012), catarrhal enteritis with splenomegaly (Shivaprasad, 2000), and pericarditis characterized by thickening of pericardium due to fibrinous exudations along with an enlarged spleen were evident (Supplementary Figure S5). Hemorrhagic edematous lungs with a peculiar yellow color were also a common finding in the birds infected with SG18 (Kumari et al., 2013). In contrary, ΔSpvB_SG18 and virulent plasmid-cured SG18-infected chickens showed no mortality or morbidity with no gross pathological lesions and signs of fowl typhoid throughout the experiment. Interestingly, the weight gain of the birds that survived after the challenge with ΔSpvB_SG18 cells was almost similar to the negative control group pointing out the non-pathogenic behavior of the ΔSpvB_SG18 strain, while birds challenged with wild-type SG18 cells showed a marked decrease in weight gain (Figures 6A,B).
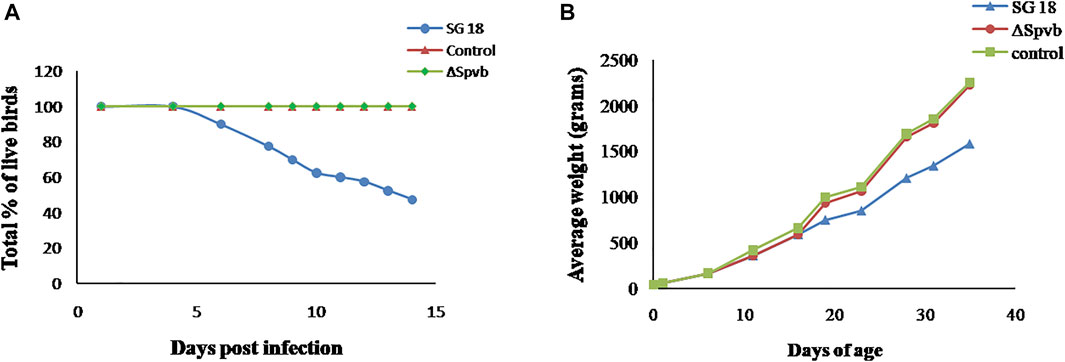
FIGURE 6. (A) Rate of mortality in chickens observed during 15 days after infection with SG18 and ΔSpvB_SG18 strains. (B) Effect of SpvB deletion from S. gallinarum on poultry weight during the 36 days of experiment.
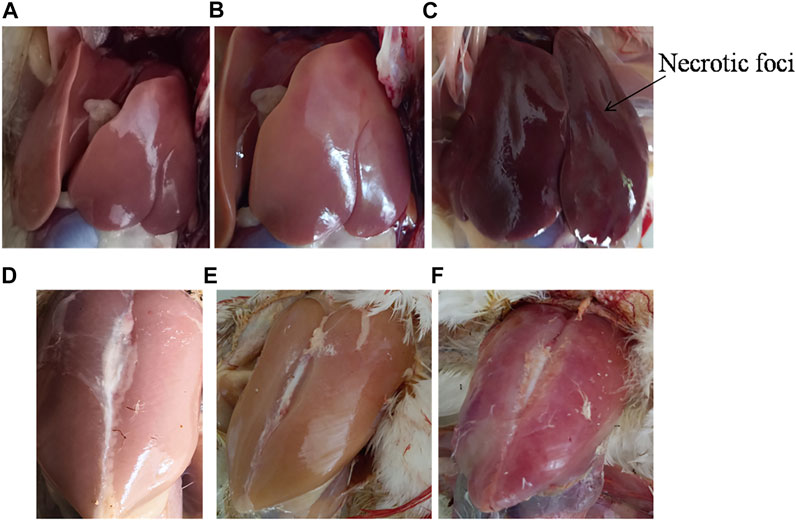
FIGURE 7. Gross pathology of dead poultry birds exhibiting typical lesions of fowl typhoid. (A) Liver of control and (B) ΔSpvB_SG18 infected birds showing no signs of fowl typhoid. In contrary, (C) the wild type strain (SG18) infected birds showing enlarged livers with necrotic foci, which are typical features of fowl typhoid. Similarly, the gross pathology of (D) the control and (E) ΔSpvB_SG18-infected birds showed no apparent lesion of disease, suggesting that these chickens were clinically healthy, while (F) the SG18-infected birds showed signs of fever and inflammation caused by S. gallinarum.
SpvB-Deleted Strains Are Rapidly Cleared After Infection
In order to determine the systemic infection, the infected strains were isolated from visceral organs of the birds, primarily liver and ileum, after 7 and 14 days post infection. The SG18 strain recovered from the liver and intestine of infected birds (positive control group) showed 3.0 × 105 (log10 = 5.4) and 1.6 × 108 (log 10 = 8.2) CFU/g after 7 and 14 days, respectively, post infection. Our results are in agreement with the previously reported study showing the highest number of viable counts that were isolated from the liver after 14 days post infection with S. gallinarum (Alves Batista et al., 2018). In contrary, no Salmonella were recovered from ΔSpvB_SG18 infected liver and ileum samples, after 4, 7, and 14 days post infection. However, very low counts were obtained from the intestine after 4 days of post infection, suggesting their rapid clearance after infection. Our results suggest that the non-virulent strains (ΔSpvB_SG18) were unable to invade from the intestine to the liver, which is contrary to previously reported avirulent SsaU mutant of S. gallinarum, found slightly invasive from the intestine to the liver (Jones et al., 2001). A complete deletion of the SpvB gene from S. gallinarum resulted in attenuation in vivo; however, further deletion of other virulent genes from the ΔSpvB_SG18 strain will make this strain a suitable candidate for vaccine design.
Conclusion
These results established an appropriate method for the complete deletion of virulent genes from plasmid DNA through CRISPR/Cas9, to produce an attenuated strain of S. gallinarum. The SpvB deleted strain of S. gallinarum was found completely avirulent in chickens, with no effect on chicken growth rate and weight. This is the first report showing gene editing in Salmonella Sp. through CRISPR/Cas9. The procedure opens a path for deletion of other virulent genes from the S. gallinarum genome to make the strain completely avirulent. The attenuated strain can then be used as a vaccine for controlling fowl typhoid disease in poultry.
Data Availability Statement
The original contributions presented in the study are included in the article/Supplementary Material; further inquiries can be directed to the corresponding authors.
Ethics Statement
The animal study was reviewed and approved by the Ethical committee of the Institute of Microbiology and Molecular Genetics, University of the Punjab, Lahore, Pakistan.
Author Contributions
AB: investigation, designing, and writing—original draft. HTah: investigation. ZH, HTar, and AU: writing—review and editing. SR: supervision, designing, and writing—review and editing.
Funding
This work was carried out with financial support from the UK government, Department of Health and Social Care (DHSC), Global AMR Innovation Fund (GAMRIF), and the International Development Research Centre Ottawa, Canada (Grant No. 109051-002). The views expressed herein do not necessarily represent those of IDRC or its Board of Governors.
Conflict of Interest
The authors declare that the research was conducted in the absence of any commercial or financial relationships that could be construed as a potential conflict of interest.
Publisher’s Note
All claims expressed in this article are solely those of the authors and do not necessarily represent those of their affiliated organizations, or those of the publisher, the editors, and the reviewers. Any product that may be evaluated in this article, or claim that may be made by its manufacturer, is not guaranteed or endorsed by the publisher.
Supplementary Material
The Supplementary Material for this article can be found online at: https://www.frontiersin.org/articles/10.3389/fbioe.2022.885227/full#supplementary-material
References
Alves Batista, D. F., de Freitas Neto, O. C., Maria de Almeida, A., Maboni, G., de Carvalho, T. F., de Carvalho, T. P., et al. (2018). Evaluation of Pathogenicity of Salmonella Gallinarum Strains Harbouring Deletions in Genes Whose Orthologues Are Conserved Pseudogenes in S. Pullorum. PLoS ONE 13 (7), e0200585. doi:10.1371/journal.pone.0200585
Andino, A., and Hanning, I. (2015). Salmonella enterica: Survival, Colonization, and Virulence Differences Among Serovars. ScientificWorldJournal 2015, 520179. doi:10.1155/2015/520179
Asten, A. J. A. M., and Dijk, J. E. (2005). Distribution of  Classicâ Virulence Factors amongSalmonellaspp. FEMS Immunology Med. Microbiology 44 (3), 251–259. doi:10.1016/j.femsim.2005.02.002
Barrow, P. A., Huggins, M. B., and Lovell, M. A. (1994). Host Specificity of Salmonella Infection in Chickens and Mice Is Expressed In Vivo Primarily at the Level of the Reticuloendothelial System. Infect. Immun. 62 (10), 4602–4610. doi:10.1128/iai.62.10.4602-4610.1994
Barrow, P. A., Simpson, J. M., Lovell, M. A., and Binns, M. M. (1987). Contribution of Salmonella Gallinarum Large Plasmid toward Virulence in Fowl Typhoid. Infect. Immun. 55 (2), 388–392. doi:10.1128/iai.55.2.388-392.1987
Blondel, C. J., Yang, H.-J., Castro, B., Chiang, S., Toro, C. S., Zaldívar, M., et al. (2010). Contribution of the Type VI Secretion System Encoded in SPI-19 to Chicken Colonization by Salmonella enterica Serotypes Gallinarum and Enteritidis. PLoS One 5 (7), e11724. doi:10.1371/journal.pone.0011724
Chen, W., Zhang, Y., Yeo, W.-S., Bae, T., and Ji, Q. (2017). Rapid and Efficient Genome Editing in Staphylococcus aureus by Using an Engineered CRISPR/Cas9 System. J. Am. Chem. Soc. 139 (10), 3790–3795. doi:10.1021/jacs.6b13317
Cheng, R. A., and Wiedmann, M. (2019). The ADP-Ribosylating Toxins of Salmonella. Toxins 11 (7), 416. doi:10.3390/toxins11070416
Cobb, R. E., Wang, Y., and Zhao, H. (2015). High-Efficiency Multiplex Genome Editing of Streptomyces Species Using an Engineered CRISPR/Cas System. ACS Synth. Biol. 4, 723–728. doi:10.1021/sb500351f
Engler, C., Gruetzner, R., Kandzia, R., and Marillonnet, S. (2009). Golden Gate Shuffling: a One-Pot DNA Shuffling Method Based on Type IIs Restriction Enzymes. PloS one 4 (5), e5553. doi:10.1371/journal.pone.0005553
Geetha, M., and Palanivel, K. M. (2018). A Brief Review on Salmonellosis in Poultry. Int. J. Curr. Microbiol. App. Sci. 7 (05), 1269–1274. doi:10.20546/ijcmas.2018.705.153
Gibson, D. G., Young, L., Chuang, R. Y., Venter, J. C., Hutchison, C. A., and Smith, H. O. (2009). Enzymatic Assembly of DNA Molecules up to Several Hundred Kilobases. Nat. Methods 6 (6), 343–345. doi:10.1038/nmeth.1318
Guiney, D. G., and Fierer, J. (2011). The Role of the Spv Genes in Salmonella Pathogenesis. Front. Microbiol. 2 (129), 129. doi:10.3389/fmicb.2011.00129
Hussain, J., Rabbani, I., Aslam, S., and Ahmad, H. A. (2015). An Overview of Poultry Industry in Pakistan. World's Poult. Sci. J. 71 (4), 689–700. doi:10.1017/s0043933915002366
Islamic Markets (2019). Survey of Pakistan 2018-2019 Agriculture. Islamabad City, Pakistan: Government of Pakistan, 29.
Jiang, W., Bikard, D., Cox, D., Zhang, F., and Marraffini, L. A. (2013). RNA-guided Editing of Bacterial Genomes Using CRISPR-Cas Systems. Nat. Biotechnol. 31 (3), 233–239. doi:10.1038/nbt.2508
Jones, M. A., Wigley, P., Page, K. L., Hulme, S. D., and Barrow, P. A. (2001). Salmonella enterica Serovar Gallinarum Requires the Salmonella Pathogenicity Island 2 Type III Secretion System but Not the Salmonella Pathogenicity Island 1 Type III Secretion System for Virulence in Chickens. Infect. Immun. 69 (9), 5471–5476. doi:10.1128/iai.69.9.5471-5476.2001
Käppeli, R., Kaiser, P., Stecher, B., and Hardt, W.-D. (2011). Roles of spvB and spvC in S. Typhimurium Colitis via the Alternative Pathway. Int. J. Med. Microbiology 301 (2), 117–124. doi:10.1016/j.ijmm.2010.08.017
Kidwai, A. S., Mushamiri, I., Niemann, G. S., Brown, R. N., Adkins, J. N., and Heffron, F. (2013). Diverse Secreted Effectors Are Required for Salmonella Persistence in a Mouse Infection Model. PLOS ONE 8 (8), e70753. doi:10.1371/journal.pone.0070753
Kim, K., Yoon, S., Kim, Y. B., and Lee, Y. J. (2020). Virulence Variation of Salmonella Gallinarum Isolates through SpvB by CRISPR Sequence Subtyping, 2014 to 2018. Animals 10 (12), 2346. doi:10.3390/ani10122346
Kumari, D., Mishra, S. K., and Lather, D. (2013). Pathomicrobial Studies on Salmonella Gallinarum Infection in Broiler Chickens. Vet. World 6 (10), 725–729. doi:10.14202/vetworld.2013.725-729
Lee, Y. J., Mo, I. P., and Kang, M. S. (2007). Protective Efficacy of liveSalmonella gallinarum9R Vaccine in Commercial Layer Flocks. Avian Pathology 36 (6), 495–498. doi:10.1080/03079450701691278
Li, J., Smith, N. H., Nelson, K., Crichton, P. B., Old, D. C., Whittam, T. S., et al. (1993). Evolutionary Origin and Radiation of the Avian-Adapted Non-motile Salmonellae. J. Med. Microbiology 38 (2), 129–139. doi:10.1099/00222615-38-2-129
Liu, H., Li, H., Liang, Y., Du, X., Yang, C., Yang, L., et al. (2020). Phage-delivered Sensitisation with Subsequent Antibiotic Treatment Reveals Sustained Effect against Antimicrobial Resistant Bacteria. Theranostics 10 (14), 6310–6321. doi:10.7150/thno.42573
Mamman, P. H., Kazeem, H. M., Raji, M. A., Nok, A. J., and Kwaga, J. K. P. (2014). Isolation and Characterization of Salmonella Gallinarum from Outbreaks of Fowl Typhoid in Kaduna State, Nigeria. Int. J. Public Health Epidmiol 3 (10), 82–88. https://www.internationalscholarsjournals.com/articles/isolation-and-characterization-of-salmonella-gallinarum-from-outbreaks-of-fowl-typhoid-in-kaduna-state-nigeria.pdf.
Matsui, H., Bacot, C. M., Garlington, W. A., Doyle, T. J., Roberts, S., and Gulig, P. A. (2001). Virulence Plasmid-Borne SpvB and spvC Genes Can Replace The 90-Kilobase Plasmid In Conferring Virulence To Salmonella enterica Serovar Typhimurium In Subcutaneously Inoculated Mice. J. Bacteriol. 183 (15), 4652–4658. doi:10.1128/jb.183.15.4652-4658.2001
Matsui, H., Bacot, C. M., Garlington, W. A., Doyle, T. J., Roberts, S., and Gulig, P. A. (2001). Virulence Plasmid-Borne spvB and spvC Genes Can Replace the 90-kilobase Plasmid in Conferring Virulence to Salmonella enterica Serovar Typhimurium in Subcutaneously Inoculated Mice. J. Bacteriol. 183 (15), 4652–4658. doi:10.1128/jb.183.15.4652-4658.2001
Mitra, A., Loh, A., Gonzales, A., Łaniewski, P., Willingham, C., Curtiss III, R., et al. (2013). Safety and Protective Efficacy of Live Attenuated Salmonella Gallinarum Mutants in Rhode Island Red Chickens. Vaccine 31 (7), 1094–1099. doi:10.1016/j.vaccine.2012.12.021
Rosu, V., Chadfield, M. S., Santona, A., Christensen, J. P., Thomsen, L. E., Rubino, S., et al. (2007). Effects of Crp Deletion in Salmonella enterica Serotype Gallinarum. Acta Vet. Scand. 49 (1), 14–17. doi:10.1186/1751-0147-49-14
Rotger, R., and Casadesús, J. (1999). The Virulence Plasmids of Salmonella. Int. Microbiol. 2, 177–184.
Roudier, C., Fierer, J., and Guiney, D. G. (1992). Characterization of Translation Termination Mutations in the Spv Operon of the Salmonella Virulence Plasmid pSDL2. J. Bacteriol. 174 (20), 6418–6423. doi:10.1128/jb.174.20.6418-6423.1992
Saha, A. K., Sufian, M. A., Hossain, M. I., and Hossain, M. M. (2012). Salmonellosis in Layer Chickens: Pathological Features and Isolation of Bacteria from Ovaries and Inner Content of Laid Eggs. J. Bangladesh Agric. Univ. 10 (1), 61–67. doi:10.3329/jbau.v10i1.12095
Shah, D. H., Lee, M.-j., Park, J.-h., Lee, J.-h., Eo, S.-k., Kwon, J.-t., et al. (2005). Identification of Salmonella Gallinarum Virulence Genes in a Chicken Infection Model Using PCR-Based Signature-Tagged Mutagenesis. Microbiology 151 (12), 3957–3968. doi:10.1099/mic.0.28126-0
Shivaprasad, H., and Barrow, P. (2008). in Pullorum Disease and Fowl Typhoid. Diseases of Poultry. Editors YM Saif, AM Fadley, JR Glisson, LR McDougald, LK Nolan, and DE Swayne. 12th ed. (Ames, IA: Blackwell Publishing), 620–634.
Shivaprasad, H. L. (2000). Fowl Typhoid and Pullorum Disease. Rev. Sci. Tech. OIE 19 (2), 405–424. doi:10.20506/rst.19.2.1222
Singh, Y., Saxena, A., Kumar, R., and Saxena, M. K. (2018). Virulence System of Salmonella with Special Reference to Salmonella enterica. London, UK: IntechOpen Limited, 41–53.
Skyberg, J. A., Logue, C. M., and Nolan, L. K. (2006). Virulence Genotyping of Salmonella Spp. With Multiplex PCR. Avian Dis. 50 (1), 77–81. doi:10.1637/7417.1
Tagliaferri, T. L., Guimarães, N. R., Pereira, M. P. M., Vilela, L. F. F., Horz, H. P., Dos Santos, S. G., et al. (2020). Exploring the Potential of CRISPR-Cas9 under Challenging Conditions: Facing High-Copy Plasmids and Counteracting Beta-Lactam Resistance in Clinical Strains of Enterobacteriaceae. Front. Microbiol. 11 (578), 578. doi:10.3389/fmicb.2020.00578
Trucksis, M., Galen, J. E., Michalski, J., Fasano, A., and Kaper, J. B. (1993). Accessory Cholera Enterotoxin (Ace), the Third Toxin of a Vibrio cholerae Virulence Cassette. Proc. Natl. Acad. Sci. U.S.A. 90 (11), 5267–5271. doi:10.1073/pnas.90.11.5267
Wang, P., He, D., Li, B., Guo, Y., Wang, W., Luo, X., et al. (2019). Eliminating Mcr-1-Harbouring Plasmids in Clinical Isolates Using the CRISPR/Cas9 System. J. Antimicrob. Chemother. 74 (9), 2559–2565. doi:10.1093/jac/dkz246
Wang, Y., Zhang, Z.-T., Seo, S.-O., Lynn, P., Lu, T., Jin, Y.-S., et al. (2016). Bacterial Genome Editing with CRISPR-Cas9: Deletion, Integration, Single Nucleotide Modification, and Desirable "Clean" Mutant Selection in Clostridium Beijerinckii as an Example. ACS Synth. Biol. 5 (7), 721–732. doi:10.1021/acssynbio.6b00060
Keywords: CRISPR/Cas9, Salmonella gallinarum, fowl typhoid, poultry, virulent plasmid, SpvB
Citation: Basit A, Tahir H, Haider Z, Tariq H, Ullah A and Rehman SU (2022) CRISPR/Cas9-Based Deletion of SpvB Gene From Salmonella gallinarum Leads to Loss of Virulence in Chicken. Front. Bioeng. Biotechnol. 10:885227. doi: 10.3389/fbioe.2022.885227
Received: 27 February 2022; Accepted: 25 April 2022;
Published: 13 June 2022.
Edited by:
Alberto Rodriguez, Sandia National Laboratories (DOE), United StatesReviewed by:
Junjie Yue, Beijing Institute of Biotechnology, ChinaArindam Mitra, Adamas University, India
Copyright © 2022 Basit, Tahir, Haider, Tariq, Ullah and Rehman. This is an open-access article distributed under the terms of the Creative Commons Attribution License (CC BY). The use, distribution or reproduction in other forums is permitted, provided the original author(s) and the copyright owner(s) are credited and that the original publication in this journal is cited, in accordance with accepted academic practice. No use, distribution or reproduction is permitted which does not comply with these terms.
*Correspondence: Abdul Basit, basitbch@gmail.com; Shafiq Ur Rehman , shafiq.mmg@pu.edu.pk
†These authors have contributed equally to this work and share first authorship