- 1Faculty of Food Technology Osijek, Josip Juraj Strossmayer University of Osijek, Osijek, Croatia
- 2Faculty of Chemistry and Chemical Technology, University of Ljubljana, Ljubljana, Slovenia
- 3Energy Institute Hrvoje Požar, Zagreb, Croatia
- 4Czech Academy of Sciences (ASCR), Prague, Czechia
- 5Lab of Biorefinery, Shanghai Advanced Research Institute, Chinese Academy of Sciences, Pudong, China
Brewer’s spent grain (BSG) accounts for approximately 85% of the total mass of solid by-products in the brewing industry and represents an important secondary raw material of future biorefineries. Currently, the main application of BSG is limited to the feed and food industry. There is a strong need to develop sustainable pretreatment and fractionation processes to obtain BSG hydrolysates that enable efficient biotransformation into biofuels, biomaterials, or biochemicals. This paper aims to provide a comprehensive insight into the availability of BSG, chemical properties, and current and potential applications juxtaposed with the existing and emerging markets of the pyramid of bio-based products in the context of sustainable and circular bioeconomy. An economic evaluation of BSG for the production of highly valuable products is presented in the context of sustainable and circular bioeconomy targeting the market of Central and Eastern European countries (BIOEAST region).
Introduction
The circular and sustainable bioeconomy are gaining increasing attention as a means to address climate changes and defosillisation, to increase resource efficiency, and to create new opportunities for sustainable, long-term economic growth (Birner, 2018; European Commission 2018). In the search for innovative solutions to return wastes and by-products to the production cycle, the bioeconomy often relies on bioprocesses involving cells or their constituents. Biorefineries replace fossil carbon with renewable carbon from various lignocellulosic biomass resources to produce a range of bio-based products (Tišma et al., 2021a; Karp et al., 2021). The trend towards innovative biotechnological solutions for the valorization of lignocellulosic side-streams in the food and beverage industry is evident also from six projects funded by Bio-based Industries Joint Undertaking in 2020 (Bio-based Industries Joint Undertaking, 2020)1: VAMOS, HYPERBIOCOAT, IFERMENTER, VEHICLE, CAFIPLA, FIRST2RUN, and BIOSUPPACK, the latter considering also BSG.
Lignocellulosic materials are renewable raw materials derived from natural sources or bio-based chemical/biotechnological processes. They are mostly used directly or indirectly as feed or as a bioenergy source, but there is growing interest in using them as a substitute for fossil carbon in the production of various products including high-value chemicals and biomaterials (Tišma et al., 2021a). The main structural components of lignocellulosic materials are polymers, namely cellulose, hemicellulose, and lignin. The composition varies greatly depending on the type of raw material, harvesting and growth conditions, as well as handling and storage (Tišma et al., 2021a).
BSG is a lignocellulosic material that, along with hot trub and residual brewer’s yeast, accounts for the majority (approximately 85%) of solid by-products in the brewing industry (Lynch et al., 2016). In the beer production chain, the grain (mostly barley) is converted into malt in the malting process (steeping, germination and kilning), which takes place in the malting factory. Then, the malted barley (or other raw materials, alone or in combination, depending on the type of beer produced) goes through the mashing phase in the brewery, where milled malt and water are mixed and the hydrolytic enzymes responsible for breaking down the starch and proteins are activated. At the end of these processes, a mixture of undegraded and extracted ingredients in water is obtained. The aqueous solution containing the extract is called wort, while BSG is the solid phase that serves as a filter for the wort, which is further processed before being used as a medium for fermentation. The mass of wet BSG obtained per 1 hl of beer produced is about 20 kg (Gupta et al., 2010).
BSG is mainly used in feed and food production but the trend of converting BSG into products in the upper part of the bio-based value pyramid is evident from recent reviews (Lynch et al., 2016; Ikram et al., 2017; Abd El-Hack et al., 2019; Lao et al., 2020; Jaeger et al., 2021; Marcus and Fox, 2021; Puligundla and Mok, 2021). The establishment of a circular green process for BSG management is based on substantial and detailed experimental research of each process. Examples include the dehydration process of BSG, oil extraction, ethanol production and anaerobic digestion on a common basis, as a concept of an integrated multi-product biorefinery (Kavalopoulos et al., 2021).
This review aims to outline the availability of BSG with a focus on the EU and the 11 member states of The Central-Eastern European Initiative BIOEAST (2021),2. The chemical composition and current application of BSG are discussed, followed by the possibilities of biotransformation to achieve higher economic value. Generally, great emphasis has been placed on waste recovery through the development of high value-added products in order to provide new economic opportunities through the commercialization of products. The novelty of this paper is reflected in the current and forecasted assessment of market size of high-value products based on new BSG valorization research in the context of sustainable and circular bioeconomy.
Systematic Review Protocol/Strategy
It is known that a detailed systematic literature search and strategy setting provides an explanation, hypothesis and planned order of the review paper. Protocol preparation ensures consistency, review, research integrity, and transparency of completed work (Moher et al., 2015). The systematic search strategy in this paper was undertaken following Systematic Review in Conservation and Environmental Management guidelines established by Pullin and Stewart (Pullin and Stewart, 2007) and at the beginning of a systematic search, a question-setting composed: a) subject, b) intervention, and c) research outcome was set. The guidelines of the model Preferred Reporting Items for Systematic Review and Meta-Analysis Protocols (PRISMA-P) established by Moher et al. (Moher et al., 2009) were also followed. The protocol of this systematic search is divided into three sections. First, a systematic literature search was conducted to collect all relevant data on the worldwide availability of BSG, followed by the chemical composition of BSG and its current use. Then, a systematic literature search was conducted to collect information on the potential use of BSG in the circular economy: a) the treatment of BSG with microorganisms or enzymes, b) the use of BSG as a substrate for the cultivation of microroganisms for enzyme production, c) the use of BSG as a substrate for the cultivation of microorganisms and for the production of high-value products. In the last section of the paper, the market size of high-value products was analyzed.
Worldwide Beer Production
In 2020, the global annual beer production was estimated at 1.82 billion hL, with North and South America leading in production (615.28 million hL), followed by Asia (550.88 million hL), Europe (500.93 million hL), Africa (131.51 million hL) and Australia/Oceania (20.99 million hL) (Statista, 2021)3. Based on the global beer production quantities, about 36.4 million tons of BSG would be available worldwide. Table 1 presents global beer and BSG production by region.
The Central-Eastern European Initiative BIOEAST supports transition to circular and sustainable bioeconomies comprising sustainable production and processing of residual biomass. BIOEAST member countries produce about 26% of the total EU27 beer production, meaning that BSG is an abundant resource to consider when creating a regional and national bioeconomical strategy (BIOEAST, 2021). Among the BIOEAST countries, the largest potential for BSG processing to higher added-value bio-based products is in Poland with annual BSG production of 816 kt, followed by Czech Republic (403 kt) and Romania (359 kt). Other member countries are Hungary (120 kt), Bulgaria (96 kt), Croatia (65 kt), Lithuania (63 kt), Slovakia (34 kt), Estonia (28 kt), Slovenia (19 kt) and Latvia (15 kt) in 2019. According to 2021 data from European Beer Association, there are currently around 11,000 active breweries in the EU producing around 400 million hL of beer per year. Calculated based on market movements of the last 5-year period, in 2030 the EU will produce about 425 million hL of beer and 8.5 million tonnes of BSG per year, which makes BSG an interesting biomass resource for the future biorefineries (The Brewers of Europe, 2019)4.
Chemical Composition of BSG
The chemical composition of BSG depends on several factors, primarily on the type of barley (or other raw materials) used in malting, the time and technique of harvesting, the quality of the malt, the additives applied in the mashing stage, etc. (Lynch et al., 2016). For example, it has been shown that BSG produced from light malt has a higher concentration of phenolic compounds compared to dark malt obtained by malt roasting. Total amount of ferullic acid (FA) and p-coumaric acid (p-CA) was four fold higher in BSG obtained from light malt than in those from dark malt. The highest levels obtained for the light malt type were 1809.5 μg FA/gDM and 686.6 μg p-CA/gDM, while those for the dark malt type were 404.7 μg FA/gDM and 185.3 μg p-CA/gDM (Birsan et al., 2019). During beer production, various additives such as gum arabic, propylene glycol alginate, polypeptides, zinc, iron, nickel and other elements are added that can improve foaming, cap hanging and foam stability. The addition of additives leads to hydrolysis of β-glucans, removal of oxalic acid, and polyphenols reduction, which also affects the final chemical composition of BSG (Li et al., 2010). In addition, the BSG storage process also causes changes in chemical composition. It was investigated that frozen samples had a higher content of protein and fat compared to lyophilized and oven-dried samples, but lower sugar content, especially arabinose (Johnson et al., 2010).
BSG is lignocellulosic material. The main components of BSG are hemicellulose, cellulose, lignin, proteins, and polysaccharides (Parchami et al., 2021). Among them, hemicellulose is the most abundant (19%–20%DM) (Tišma et al., 2018; Giacobbe et al., 2019) and in some cases reaches up to 41.3% (Assefa and Jabasingh, 2020), followed by cellulose (15.2%–28.7%DM) (Rojas-Chamorro et al., 2019; Assefa and Jabasingh, 2020). The average content of lignin is 11.41%, but it can vary from 3.35% (Castro and Colpini, 2021) to 21% (Giacobbe et al., 2019). The amount of arabinoxylans, the main components of hemicellulose, in BSG ranges from 2.67% (Bravi et al., 2021) to 21.9% (Lynch et al., 2021). β-glucans are present in an amount of 1% (Bravi et al., 2021). Total proteins range from 18.5% to 24.7% (Almeida et al., 2017; Nazzaro et al., 2020). The other presented components are lipids (8.4%) (Nazzaro et al., 2020), starch (5.3%) (Rojas-Chamorro et al., 2019) and ash (3.7%) (Sibhatu et al., 2021).
BSG is also comprised of phenolic compounds (Tišma et al., 2018), which are distributed among different parts of the barley kernel. Their concentration varies depending on the genotype of malt used, growing environment, and their interaction during production process (Rahman et al., 2021). According to Sajib et al., the most common phenolic compounds in BSG are ferulic acid (1,219.40 μg/g) and p-coumaric acid (488.51 μg/g). Smaller amounts of catechin,4-hydroxybenzoic acid, sinapic acid, syringic acid, protocatechuic acid and caffeic acid are also found (Birsan et al., 2019). The fatty acids found in BSG are palmitic acid (1.805 mg/g), stearic acid (0.596 mg/g), oleic acid (0.041 mg/g), linoleic acid (0.445 mg/g) (Tan et al., 2019). The amount of total amino acids in BSG is 0.859 mg/g, in the largest amount is proline (0.349 mg/g), then glutamic acid (0.340 (mg/g). In a smaller amount there are presence of aspartic acid, achenylalanine, serine, threonine, lysine and tyrosine (Tan et al., 2019). The most abundant sugars are glucose (37.06%), xylose (10.25%), arabinose (4.50%), mannose (1.18%) and galactose (0.24%) (Sajib et al., 2018). Various minerals found in ash are phosphorus (4,882.7 mg/kg), potassium (1,570.9 mg/kg), iron (210 mg/kg), calcium (81.60 mg/kg), zinc (67.2 mg/kg), manganese (34.3 mg/kg) (Almeida et al., 2017). BSG contains a significant amount of vitamins including vitamin B1 (25 mg/kg), vitamin B2 (25 mg/kg), vitamin B2 (9 mg/kg) and vitamin K (4.5 mg/kg) (Nagy and Diosi, 2021). Table 2 presents the chemical composition of BSG collected from literature, expressed as the mean value with indicated standard deviations.
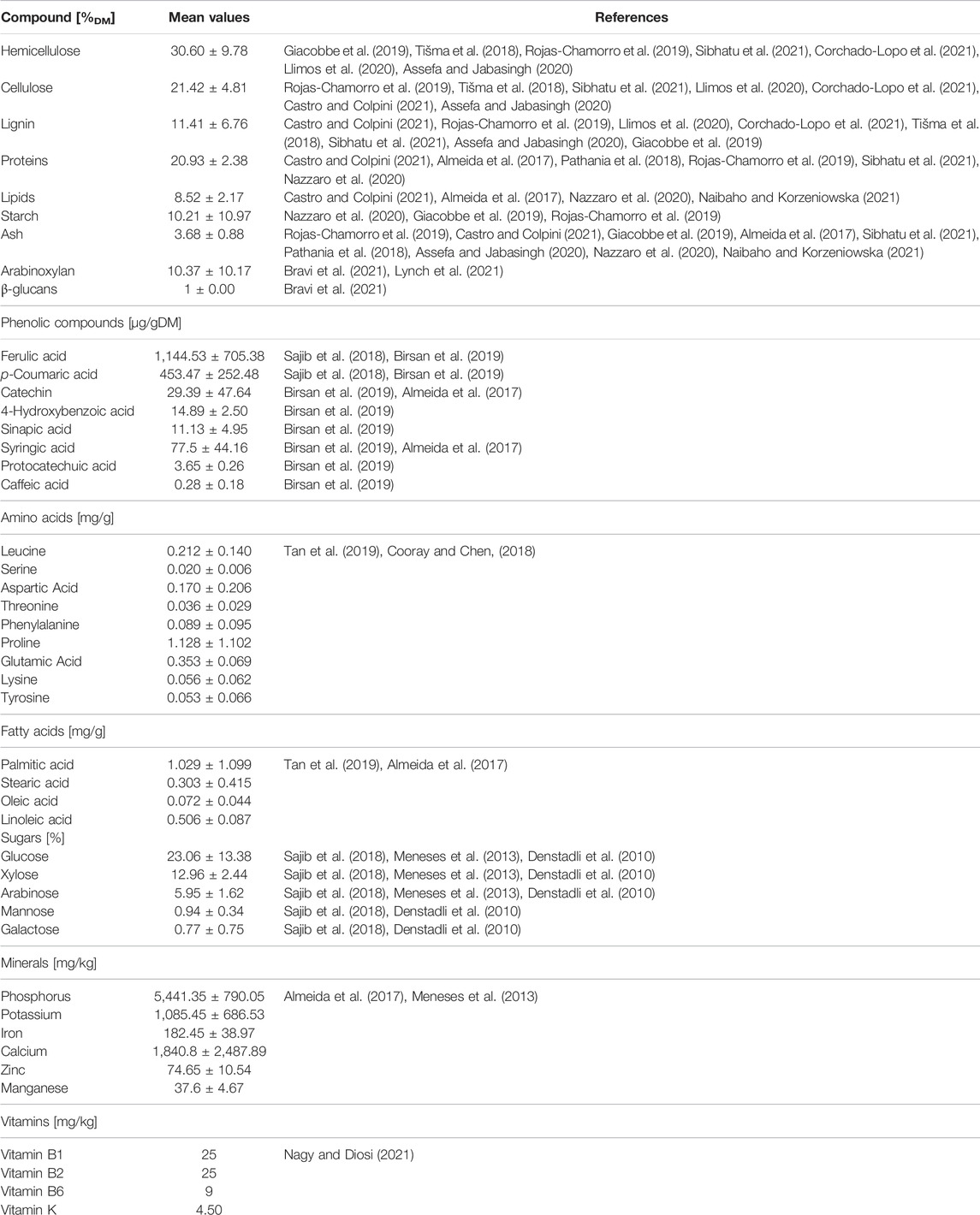
TABLE 2. Estimated mean values of BSG primary components calculated from the literature data stated in the table. The values are expressed as a percentage of dry matter (%DM), or with an appropriate unit of measurement, with indicated standard deviations.
Conventional techniques for determining the chemical composition of BSG are complex and time consuming, so novel techniques that are both fast and precise are requested. A promising, cost-effective, and widely used technique for rapid determination of chemical composition can be near-infrared spectroscopy (NIR). NIR is used to characterize the chemical composition by providing qualitative and quantitative information on complex samples, in BSG, the presence of cellulose, hemicellulose and lignin was investigated by this method (Amirvaresi et al., 2020; Castro and Colpini, 2021). Thermogravimetry (TG) is a modern and fast technique that provides accurate and fast measurements. TG is used to characterize the composition and moisture content of lignocellulosic material by continuous monitoring a decrease or increase in mass as a function of temperature applied (Rego et al., 2019). The amount and distribution of cellulose, hemicellulose, and lignin to submicroscopic level can be elucidated using the Raman scattering microscopy technique. A potential problem with spectroscopic techniques is the accurate determination of the peaks associated with the component of interest due to the intricate nature of the basic spectrum (Krasznai et al., 2017).
The Most Common Use of BSG
At present, BSG is used for various purposes in wet form, directly after filtration, or in dried form after drying. Traditionally it is used in feed and food production, but recently BSG also plays a role in bioenergy production and waste management. The cost of transportation (especially of BSG in wet form), the cost of drying, and need for pretreatment process to reduce its recalcitrance, are some of the barrier to wider application (Pabbathi et al., 2022).
Its use in animal feed is related to its high fiber and protein content. However, the high fiber content has positive effects on cows and negative effects on poultry. Feeding cows with BSG improves digestibility and has a positive effect on milk production efficiency and profitability, but may also reduce dry matter intake, body weight, protein and milk fat (Faccenda et al., 2017). Dried BSG contains the nutrients needed in poultry feed formulation, but its use may be limited due to high fiber content, resulting in reduced digestibility (Abd El-Hack et al., 2019). BSG is also used in pig and lamb feeding and, contributes to weight gain and meat quality (Amoah et al., 2017; Radzik-Rant et al., 2018).
Recent studies show that BSG is a potential source of prebiotics that optimize the balance and activity of microbes in the gut. When domestic animals ingest arabinoxylans and β-glucans from BSG, the activity of beneficial bacteria, especially Bifidobacterium, Enterococcus and Lactobacillus, is stimulated. In addition, increased degradation of dietary fiber accelerates the production of short-chain fatty acids, which are the primary energy source for anaerobic microbes. With appropriate considerations and a BSG strategy as a dietary supplement, the overall health and breeding of domestic animals can be improved (Lao et al., 2020).
In the food industry, BSG is used for the production of bread (Neylon et al., 2021), cookies (Farcas et al., 2021), muffins (Combest et al., 2020), pasta (Schettino et al., 2021), cereal bars (Stelick et al., 2021), chips (Garrett et al., 2021) and yogurt (Naibaho et al., 2022). The increased content of dietary fiber helps in the elimination of cholesterol and fats and improves the symptoms of ulcerative colitis. Moreover, the presence of phenolic compounds, which are considered as natural antioxidants, is associated with the prevention of chronic, cardiovascular, and neurogenerative diseases, certain cancers, and diabetes (Ikram et al., 2017). Additionally, BSG is a suitable medium for the growth of various fungi, bacteria and other microorganisms due to its chemical composition, particle size, and water retention capacity (Xiros and Christakopoulos, 2012; Malomo et al., 2013).
BSG can also be used as a vermicompost/soil improver, after bioconversion with Eisenia fetida in combination with microorganisms. The suitability of BSG as a substrate for the growth of these worms is demonstrated by the results of reduced total organic carbon, increased total nitrogen and total humus matter, which induces enhanced mineralization and stabilization (Saba et al., 2019).
The Potential Use of BSG in Circular Bioeconomy
The concept of circular bioeconomy is mainly aimed at the recovery of all products from the resources without generating waste. Lignocellulosic biorefineries are enablers of circular bioeconomy, while through integrated biorefinery processes, multiple end products could be produced. However, to meet the sustainability requirements, development and implementation of sustainable technologies in conversion of BSG and other lignocellulosic materials into bio-basaed products are urgently needed (Sganzerla et al., 2021a). Sganzerla et al. (2021b) made techno-economic assessment of bioenergy and fertilizer production by anaerobic digestion of BSG with simulations performed by integrating the production of biomethane, electricity, thermal energy, and fertilizer, which is one of examples of application of brewer’s spent grains following biorefinery concept.
Biochemical Transformation of BSG for Improved Applicability in Production and Environmental Processes
As mentioned above, the current use of BSG in wet or dry form shows some disadvantages in certain applications. Therefore, considerable efforts are being made to improve the BSG applicability through various pretreatment processes. Supplementary Table S1 provides an overview of the methods used to treat BSG for its further use in energy production, waste management, biofertilizer, feed and food production.
To improve the quality of BSG for use as feed, BSG can be treated with enzymes (Denstadli et al., 2010) or microorganisms. Solid-state fermentation (SSF) technology is a promising technology for the treatment of lignocellulosic materials for various applications (Tišma et al., 2021b). Xylanase pretreatment BSG for chicken feed reduced the reduced the concentration of polymeric arabinose and xylose by 15%–30% (Denstadli et al., 2010), and the inclusion of BSG treated with SSF by Aspergillus ibericus in fish diet increased dry matter digestibility and energy (Fernandes et al., 2021). SSF was used for BSG treatment for the production of food, wherein the properties of whole-wheat bread with the addition of fermented BSG by Aspergillus awamory increase the amount of ferulic acid by 198% (Dos Santos Costa et al., 2020). SSF with Bacillus subtilis resulted in improved nutritional composition of BSG. There was an increase in total amino acids by 2 fold, 1.7 fold of unsaturated fatty acids and 5.8 fold of antioxidants compared to unfermented BSG (Tan et al., 2019). In particular, the bioconversion of BSG via SSF improves the nutritional profile and indicates the potential use of BSG in a diet enriched with proteins and bioactive compounds. Thus, SSF by Pleurotus ostreatus resulted in an increase in the proportion of protein and 1,3/1,6-β-glucans in fermented BSG (Eliopoulos et al., 2021). Also, the implementation of SSF by Rhizopus sp. led to an 11 fold increase in total polyphenolic compounds and enrichment of BSG with amino acids (Ibarruri et al., 2019). BSG has proven to be a good substrate for the production of biocontrol fertilizer by fungal SSF (Qiu et al., 2019) and vermicompost enriched with bacteria and fungi (Bianco et al., 2022).
However, most studies on BSG pretreatment are devoted to its use for bioenergy production. One study considers silver nitrate pretreatment of BSG prior to pyrolysis (Ashman et al., 2020), while the majority of studies is dedicated to biogas (Panjičko et al., 2017; Dudek et al., 2019), bioethanol (Caetano et al., 2013), or biobutanol (Giacobbe et al., 2019) production. A two-stage biogas production process from BSG as a mono-substrate was developed, where methanogenesis was performed in a granular biomass reactor, while microbiological hydrolysis and acidogenesis were performed in a solid-state anaerobic digestion reactor. After adaptation of the microbial community, the process exhibited long-term stable operation and showed a high degradation efficiency and biogas/methane production capacity (Panjičko et al., 2017). Improvement of yields and increase of biogas production by anaerobic digestion of BSG can be achieved by adding biochar produced by the process of torefication, also from BSG. The maximum biogas production without the addition of biochar was 92.3 dm3/kg of dry organic matter (DOM), while it increased to 122 dm3/kgDOM with the addition of 5% biochar. The addition of a high percentage of biochar (20%–50%) can inhibit biogas production (Dudek et al., 2019). In the work of Ortiz et al. (2019) it is shown that by gasification of BSG it is possible to achieve a net economic saving of 22%, if the produced gas would be used for heat production in breweries, with the advantage of minimizing BSG as waste.
The process of bioethanol production typically involves pretreatment of lignocellulosic material to enhance carbohydrate hydrolysis, further fermentation of simple sugars into ethanol, and final distillation for product recovery (Caetano et al., 2013). Widely used pretreatment techniques comprise acid hydrolysis, alkaline wet oxidation and steam explosion, which involve high temperatures and pressures and often lead to the formation of compounds that negatively affect the further fermentation of sugars into ethanol. In contrast, enzymatic hydrolysis, which is carried out under mild conditions, does not generate hazardous by-products (Heredia-Olea et al., 2015). For example, application of two laccase preparations from Pleurotus ostreatus on milled BSG resulted in up to 94% of phenols reduction. Moreover, the formation of other inhibitory compounds was avoided, so further fermentation to acetone-butanol-ethanol using Clostridium acetobutilycum was enhanced (Giacobbe et al., 2019). The high yield of biobutanol obtained in this study is noteworthy because butanol has a higher energy value, lower vapor pressure, lower corrosivity, and lower tendency to mix with water compared to ethanol, and can be transported through existing pipelines (Bellido et al., 2014). The use of hydrolysate from various lignocellulosic materials has been investigated a lot, suggesting that there is a need for exploring the use of BSG for isobutanol production. An engineered Escherichia coli strain yielded 3.7 g/L of biobutanol when grown on cedar hydrolyzate (Akita et al., 2015). This microorganism was also used by Minty et al. (2013) on corn stover, without costly nutrient supplementation, 1.88 g/L of isobutanol was produced. A synthetic fungal-bacterial consortia was developed, in which the fungus secretes cellulase enzymes to hydrolyze lignocellulosic material, and E. coli metabolizes soluble saccharides into isobutanol. An isobutanol producing strain that use Clostridium thermocellum as the host produced 5.4 g/L of isobutanol from cellulose (Lin et al., 2015).
In waste management, BSG can be used as a low-cost adsorbent to remove heavy metals such as silver and iron (Li et al., 2010; Izinyon et al., 2016), or to remove dyes such as Congo Red, Methylene Blue and Malachite Green dyes in the wastewater system (Kezerle et al., 2018; Chanzu et al., 2019). The modification of BSG is beneficial for the removal of silver from aqueous solutions. Various agents such as oxidizing agents, mineral and organic solutions, bases and acids have been used to improve the adsorption capacity of BSG (Li et al., 2010).
In an effort to reduce the production of synthetic materials and increase plastic recycling, BSG is a potential raw material for biofilm production. Biofilms used in food products and medicines must have thermal stability which has been successfully achieved with the arabinoxylan-rich fraction from BSG (Jaguey–Hernández et al., 2022). Also, due to the rich protein profile of BSG, Proaño et al. (2020) have used protein dispersion from BSG in the production of biofilms with potential application in active food packaging. Protein films showed balanced mechanical properties, water resistance and antioxidant capacity.
BSG as a Resource for the Production of Enzymes and Other Value-Added Products
Biocatalysis and continuous processing were identified as crucial enabling technologies for the development of cost-efficient manufacturing with high-quality products and low waste generation, following the 12 principles of green chemistry (Žnidaršič-Plazl, 2021a; Žnidaršič-Plazl, 2021b). Enzymes are environmentally friendly catalysts that operate under mild conditions with high regio-, stereo-and reaction selectivity, making them key tools for single-step biotransformations up to total chemo- or multi-enzymatic syntheses (Wohlgemuth, 2021). Enzymes can be produced by submerged fermentation (SmF) or SSF, the latter being more suitable when the substrate for microbial growth and enzyme production is lignocellulose. The advantages and disadvantages of both fermentation techniques were described in recent review papers (Tišma et al., 2021b; Leite et el., 2021).
The possibilities of using BSG as a substrate for cultivation of various microorganisms in SSF to produce a variety of enzymes are shown in Table 3. Information about the microorganism, the type of cultivation and the enzyme activities obtained is indicated. The use of BSG as fermentation medium component typically results in high xylanase activities, which is to be expected considering that hemicellulose represents the largest portion of all polymers in BSG. In addition, many other enzymatic activities are involved in the complex degradation of lignocellulosic material (Šelo et al., 2021; Chilakamarry et al., 2022) e.g., various glucanases, cellobiohydrolases, esterases and pectinases.
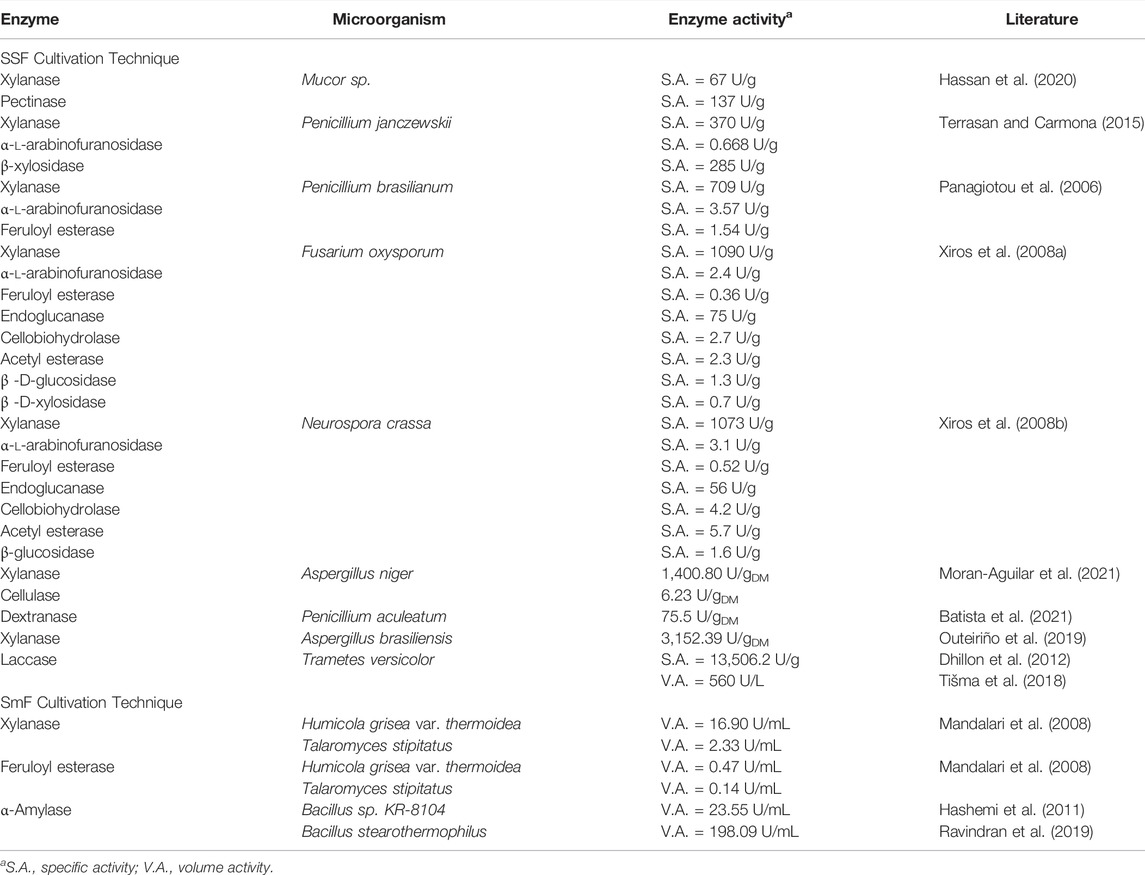
TABLE 3. BSG as a substrate for the production of different enzymes by different microorganisms in SSF or SmF conditions.
BSG is considered as a cheap and promising raw material for the production of high-value products (Xiros and Christakopoulos, 2012). The studies on the use of BSG for the production of lactic acid, ferulic acid, p-coumaric acid, xylitol, poly-3-hidroxybutyrate, natural red pigment, 2,3-butanediol, gibberellic acid, citric acid, ascorbic acid and cordycepin are summarized in Table 4. Table 4 provides information of pretreatment used to produce BSG hydrolyzate, including the techniques to concentrate simple sugars, and the data on the fermentation conditions comprising the microorganisms used to produce the product of interest together with the yield obtained.
The widespread use of lactic acid in the food, pharmaceutical, chemical, and textile industries requires industrial manufacturing on a ton scale. Its production from bio-based materials has increased significantly in the last decade due to the increasing use of (poly) lactic acid, a bio-based and biodegradable biopolymer used in many disposable packaging (Datta and Henry, 2006). Pretreatment is required to remove the lignin barrier from BSG, followed by saccharification of polysaccharides with a cellulolytic enzyme cocktail, and fermentation of the resulting sugars using microorganisms (Liguori et al., 2015). Citric acid is an important organic acid produced in tons as it is widely used in food industry and household. It can be produced from BSG by SmF using Aspergillus niger (Pathania et al., 2018). da Silva et al. (2021) published that BSG can be used as a growth medium for the cultivation of Fusarium fujikuroi to produce gibberellic acid (GA3), which has a promising application in the agroindustrial sector as it is related to plant growth.
The most common phenolic acids that can be isolated from lignin of BSG are ferulic and p-coumaric acid. Since these phenolic acids are precursors in the biocatalytic production of aromatic natural value-added compounds, BSG can be used as a source of these compounds (Mussatto et al., 2007a; Mussatto et al., 2007b). Xylitol is a functional sweetener that is usually produced chemically, but can also be produced from xylose-rich hydrolysates that can be obtained by lignocellulose degradation. BSG is a potential and cost-effective raw material for xylitol production without the need to add nutrients to the medium and detoxify the hydrolysate (Puligundla and Mok, 2021). An innovative approach to reducing the environmental impact of BSG processing in xylitol, ethanol, and polyhydroxybutyrate production in biorefineries using a heat integration strategy suggested that the overall production costs could be reduced by 43% (Dávila et al., 2016).
BSG also occupies a place in pharmaceutical use. It was used as a culture medium for Cordyceps militaris for the production of cordycepin (3′-deoxyadenosine), which has anti-tumor, anti-metastatic, anti-bacterial, anti-proliferative and insecticidal action (Gregori, 2014). 2,3-butanediol is a microbially synthesized metabolite with versatile use in the food, chemical and pharmaceutical industries with great market potential. The high cost of the substrate in the conventional production of this compound, gives preference to renewable raw materials such as BSG (Amraoui et al., 2022). A natural red pigment was also produced during SmF with BSG hydrolysate, where sodium glutamate was used as an additional nitrogen source that also stimulate the production of the pigment by Monascus purpureus. Maximum red pigment production of 22.25 UA500 was achieved at pH 6.5, 350 rpm shake speed, 50 ml volume and inoculation ratio 2% (v/v) (Silbir and Göksungur, 2019).
Among other alternatives associated with the use of BSG, it is worth mentioning the production of fatty acids (Castilla-Archilla et al., 2020) and the production of biodegradable plastics (e.g. polyhydroxyalkanoates, PHA) (Llimós et al., 2020), leading to fulfilment of the aspirations for a sustainable manufacturing and circular bioeconomy.
Existing Market Size and Growth Rate of Selected Value-Added Products From BSG
While BSG has numerous promising pathways from waste to high value added product, it is useful to contrast the research potential with the potential market size where such novel product will be placed. Another valuable information provides the approximate unit price of the novel BSG product to size if the effort invested could be covered with the unit price achieved. While the lab scale work is much different than the industrial production, a researcher can navigate through market strata with the achieved yield of the desired component per BSG unit (Table 4), lab costs and approximated unit market price. BSG provides numerous opportunities to increase resource efficiency and sustainability, yet those opportunities seldom stay within the original, beer production. Sizing market opportunities to increase national resource efficiency relies much on recognising and forming strategic partnerships described in circular business models (Salvador et al., 2021). When deciding on the circularity pathway of BSG in the emerging circular and sustainable bioeconomies, matching available intellectual capital and BSG availability with bio-based alternatives to fossil components demand, it is worth examining the market potentials. Figure 1 illustrates the relative sizes of selected potential products of BSG valorization in world markets, and the corresponding compound annual growth rate (CAGR). The idea behind Figures 1–3 is to size and compare different potential markets for novel products as well as to align unit prices to recognise and form strategic partnerships between the BSG owner (i.e., brewery) and novel BSG-based product demand industry, whether to form market penetration strategy or to develop a circular business model.
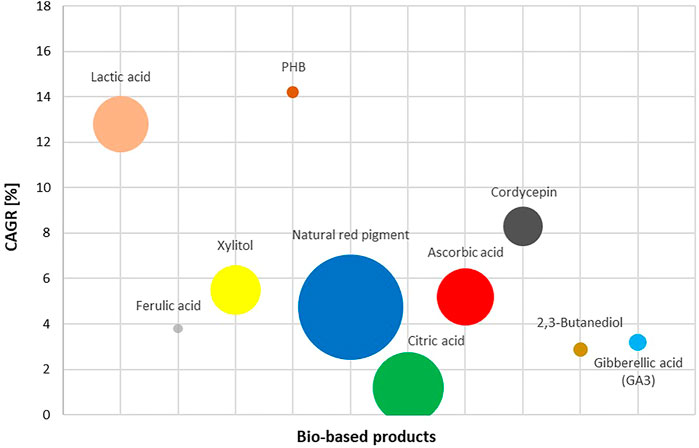
FIGURE 1. Market size and related growth rate (CAGR) of selected value-added products from BSG hydrolisates (PHB, poly-3-hydroxybutyrate (as a part of the PHA group); Natural red pigment as a part of natural pigment group). The size of the bubble represents the relative market size in 2020.
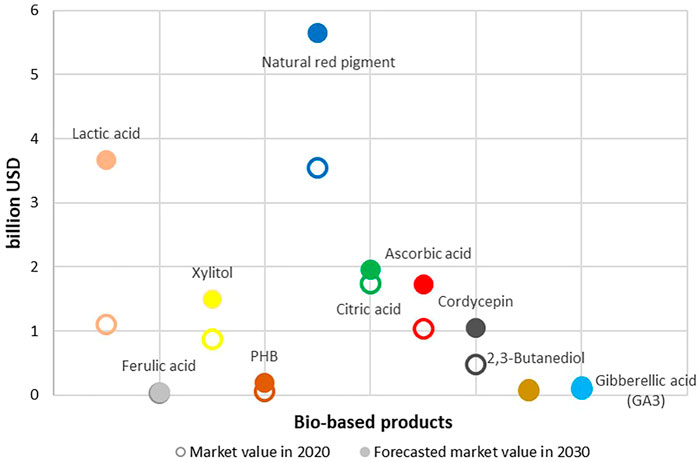
FIGURE 2. Market shares of high-value BSG-based products (PHB as a part of the PHA group; Natural red pigment as a part of natural pigment group) in 2020 and estimation for 2030.
Market size data presented in Figure 2 of selected bio-based products is retrieved from numerous websites that report on market shares and forecast future values based on the CAGR without preferring any of the sources, such as: Markets and Markets (Lactic acid); Market Watch (Ferulic acid; 2,3—Butanediol; Gibberellic acid); Expert Market Research (Xylitol, Citric acid), Business Wire (PHB), Market Research Future (Natural Red Pigment), Reports and Data (Ascorbic acid), Grand View Research (Cordycepin).
The highest annual growth rate (Figure 1) is forecast for lactic acid, which is also expected to be the largest single market for BSG-based products in 2030 (Figure 2). Natural red pigment is shown as a part of the natural pigments group, which has a large existing market worth 3.5 billion USD and a projected CAGR of 4.75% by 2025. Other uses of BSG are in a range of 1-2 billion USD, with the exception of ferulic acid and PHB.
However, when looked at the unit price (derived by triangulation of an average wholesale price at Alibaba.com) ratio, Gibberellic acid is by far carrying the greatest unit value per kg. Figure 3 illustrates ratios between unit prices among the highly valued bio-based products from BSG.
Considering the current linear structure of bioeconomies in the BIOEAST macro-region (Kulisic et al., 2020), the use of lactic acid from BSG in the existing food and beverage, pharmaceutical and cosmetics industries, as well as for biopolymers production allows a rapid transition to bio-based industry by giving BSG circularity in the macro-region. Most effects from strategic partnerships with brewery industry in lactic acid, but not limited to it, are expected in countries with high beer production and potential demand from the industry to replace fossil carbon with renewable one, supplied by short supply chains: Poland, Romania, Czech Republic, followed by Bulgaria, Croatia, Lithuania and Slovakia. In Bulgaria, Croatia, Lithuania and Romania, beer production belongs to the top 10 industrial products with their contributions to the gross value added. In those countries, circularity of BSG might fortify the competitiveness of the beer industry.
Conclusion and Future Research Directions
Although there are a large number of publications dealing with the application of BSG for the production of biofuels (biogas, bioethanol, biobutanol), biofertilizers, value-added products such as enzymes (mainly xylanase), lactic acid, ascorbic acid, citric acid, gibberellic acid, ferulic acid, xylitol, etc.), its use in biorefinery is still rare.
Future directions should aim to a) develop rapid and accurate methods to determine the chemical composition of BSG, b) cascade utilization of BSG based on its composition to produce value-added products and biofuels, c) develop sustainable methods for pretreatment of BSG, fractionation of polymers, and isolation of value-added products, d) implement methods to assess sustainability of all process phases involved in BSG utilization within biorefinery to follow the concept of circular and sustainable bioeconomy.
In this way, keeping BSG in the production cycle by producing value-added biobased products could be a readily available option for the industry to transition to a sustainable and circular bioeconomy, recognize, expand and define their future strategic partnerships with the brewing industry.
Author Contributions
All authors gave their maximum, direct and intellectual contribution during the writing of the paper. AZ-P, MT, MP, and PŽ-P set the structure and topic of the review work, developed the theory, tables and figures. BK and GS participated in the assessment and development of graphs for market size and growth rate of high value products. JH gave his expert contribution in the environmental processing section. All authors read, reviewed, and approved the final manuscript prior to submission.
Funding
This work was supported by the European Regional Development Fund (ERDF) (Grant KK.01.1.1.04.0107). PŽ-P was supported through the Slovenian Research Agency (ARRS), Slovenia (Grants P2-0191, N2- 0067 and J4-1775).
Conflict of Interest
The authors declare that the research was conducted in the absence of any commercial or financial relationships that could be construed as a potential conflict of interest.
Publisher’s Note
All claims expressed in this article are solely those of the authors and do not necessarily represent those of their affiliated organizations, or those of the publisher, the editors and the reviewers. Any product that may be evaluated in this article, or claim that may be made by its manufacturer, is not guaranteed or endorsed by the publisher.
Supplementary Material
The Supplementary Material for this article can be found online at: https://www.frontiersin.org/articles/10.3389/fbioe.2022.870744/full#supplementary-material
Footnotes
1https://www.bbi.europa.eu/search/node/lignocellulosic%20type%3Aprojects%20%22food.
3https://www.statista.com/statistics/202417/beer-output-volumes-of-the-different-continents-in-2010/
4https://brewersofeurope.org/site/countries/key-facts-figures.php
References
Abd El-Hack, M. E., Alagawany, M., Patra, A., Abdel-Latif, M., Ashour, E. A., Arif, M., et al. (2019). Use of Brewers Dried Grains as an Unconventional Feed Ingredient in the Diets of Broiler Chickens: A Review. Adv. Anim. Vet. Sci. 7, 218–224. doi:10.17582/journal.aavs/2019/7.3.218.224
A. Dávila, J., Rosenberg, M., Rosenberg, M., and A. Cardona, C. (2016). A Biorefinery Approach for the Production of Xylitol, Ethanol and Polyhydroxybutyrate from Brewer's Spent Grain. AIMS Agric. Food 1, 52–66. doi:10.3934/AGRFOOD.2016.1.52
Akita, H., Nakashima, N., and Hoshino, T. (2015). Bacterial Production of Isobutanol without Expensive Reagents. Appl. Microbiol. Biotechnol. 99, 991–999. doi:10.1007/s00253-014-6173-x
Almeida, A. D. R., Geraldo, M. R. F., Ribeiro, L. F., Silva, M. V., Maciel, M. V. D. O. B., and Haminiuk, C. W. I. (2017). Bioactive Compounds from Brewer's Spent Grain: Phenolic Compounds, Fatty Acids and In Vitro Antioxidant Capacity. Acta Sci. Technol. 39, 269–277. doi:10.4025/actascitechnol.v39i3.28435
Amirvaresi, A., Nikounezhad, N., Amirahmadi, M., Daraei, B., and Parastar, H. (2021). Comparison of Near-Infrared (NIR) and Mid-infrared (MIR) Spectroscopy Based on Chemometrics for Saffron Authentication and Adulteration Detection. Food Chem. 344, 128647. doi:10.1016/j.foodchem.2020.128647
Amoah, K. O., Asiedu, P., Wallace, P., Bumbie, G. Z., and Rhule, S. W. A. (2017). The Performance of Pigs at Different Phases of Growth on Sun-Dried Brewers Spent Grain. Livest. Res. Rural. Dev. 29, e90.
Amraoui, Y., Prabhu, A. A., Narisetty, V., Coulon, F., Kumar Chandel, A., Willoughby, N., et al. (2022). Enhanced 2,3-Butanediol Production by Mutant Enterobacter Ludwigii Using Brewers' Spent Grain Hydrolysate: Process Optimization for a Pragmatic Biorefinery Loom. Chem. Eng. J. 427, 130851. doi:10.1016/j.cej.2021.130851
Ashman, C. H., Gao, L., and Goldfarb, J. L. (2020). Silver Nitrate In Situ Upgrades Pyrolysis Biofuels from Brewer's Spent Grain via Biotemplating. J. Anal. Appl. Pyrolysis 146, 104729. doi:10.1016/j.jaap.2019.104729
Assefa, Y., and Jabasingh, S. A. (2020). Lactic Acid Production from Brewer’s Spent Grain by Lactobacillus Plantarum ATCC 8014. J. Sci. Ind. Res. 79, 10–613.
Banjo, T., Kareem, S., Popoola, T., and Akinloye, O. (2018). Microbial Production of Ascorbic Acid from Brewery Spent Grain (BSG). Food Appl. Biosci. J. 6, 93–105. doi:10.14456/fabj.2018.9
Bellido, C., Loureiro Pinto, M., Coca, M., González-Benito, G., and García-Cubero, M. T. (2014). Acetone-butanol-ethanol (ABE) Production by Clostridium Beijerinckii from Wheat Straw Hydrolysates: Efficient Use of Penta and Hexa Carbohydrates. Bioresour. Technol. 167, 198–205. doi:10.1016/j.biortech.2014.06.020
Bianco, A., Fancello, F., Garau, M., Deroma, M., Atzori, A. S., Castaldi, P., et al. (2022). Microbial and Chemical Dynamics of Brewers' Spent Grain during a Low-Input Pre-vermicomposting Treatment. Sci. Total Environ. 802, 149792. doi:10.1016/j.scitotenv.2021.14910.1016/j.scitotenv.2021.149792
Bio-based Industries Joint Undertaking (2020). Bio-based Industries Joint Undertaking. https://www.bbi.europa.eu/search/node/lignocellulosic%20type%3Aprojects%20%22food (Accessed June 15, 2021).
BIOEAST (2021). The Central-Eastern European Initiative for Knowledge-Based Agriculture, Aquaculture and Forestry in the Bioeconomy. https://bioeast.eu/home/(Accessed April 14, 2021).
Birner, R. (2018). “Bioeconomy Concepts,” in Bioeconomy. Editor I. Lewandowski (Cham: Springer), 17–38. doi:10.1007/978-3-319-68152-8_3
Birsan, R. I., Wilde, P., Waldron, K. W., and Rai, D. K. (2019). Recovery of Polyphenols from Brewer's Spent Grains. Antioxidants 8, 380. doi:10.3390/antiox8090380
Bravi, E., Francesco, G. D., Sileoni, V., Perretti, G., Galgano, F., and Marconi, O. (2021). Brewing By-Product Upcycling Potential: Nutritionally Valuable Compounds and Antioxidant Activity Evaluation. Antioxidants 10, 165. doi:10.3390/antiox10020165
Caetano, N. S., Moura, R. F., Meireles, S., Mendes, A. M., and Mata, T. M. (2013). Bioethanol from Brewer’s Spent Grains: Acid Pretreatment Optimization. Chem. Eng. Trans. 35, 1021–1026. doi:10.3303/CET1335170
Castilla-Archilla, J., Papirio, S., and Lens, P. N. L. (2021). Two Step Process for Volatile Fatty Acid Production from Brewery Spent Grain: Hydrolysis and Direct Acidogenic Fermentation Using Anaerobic Granular Sludge. Process Biochem. 100, 272–283. doi:10.1016/j.procbio.2020.10.011
Castro, L. E. N., and Colpini, L. M. S. (2021). All-around Characterization of Brewers' Spent Grain. Eur. Food Res. Technol. 247, 3013–3021. doi:10.1007/s00217-021-03860-5
Chanzu, H. A., Onyari, J. M., and Shiundu, P. M. (2019). Brewers' Spent Grain in Adsorption of Aqueous Congo Red and Malachite Green Dyes: Batch and Continuous Flow Systems. J. Hazard. Mater. 380, 120897. doi:10.1016/j.jhazmat.2019.120897
Chilakamarry, C. R., Mimi Sakinah, A. M., Zularisam, A. W., Sirohi, R., Khilji, I. A., Ahmad, N., et al. (2022). Advances in Solid-State Fermentation for Bioconversion of Agricultural Wastes to Value-Added Products: Opportunities and Challenges. Bioresour. Technol. 343, 126065. doi:10.1016/j.biortech.2021.126065
Combest, S., Warren, C., and Patterson, M. (2020). Upcycling Brewers' Spent Grain: The Development of Muffins and Biomarker Response after Consuming Muffins for 8-Weeks in Healthy Adults from Randomized-Controlled Trial. Curr. Dev. Nutr. 4, 745. doi:10.1093/cdn/nzaa052_014
Cooray, S. T., and Chen, W. N. (2018). Valorization of Brewer's Spent Grain Using Fungi Solid-State Fermentation to Enhance Nutritional Value. J. Funct. Foods 42, 85–94. doi:10.1016/j.jff.2017.12.027
Corchado-Lopo, C., Martínez-Avila, O., Marti, E., Llimós, J., Busquets, A. M., Kucera, D., et al. (2021). Brewer's Spent Grain as a No-Cost Substrate for Polyhydroxyalkanoates Production: Assessment of Pretreatment Strategies and Different Bacterial Strains. New Biotechnol. 62, 60–67. doi:10.1016/j.nbt.2021.01.009
Datta, R., and Henry, M. (2006). Lactic Acid: Recent Advances in Products, Processes and Technologies - a Review. J. Chem. Technol. Biotechnol. 81, 1119–1129. doi:10.1002/jctb.1486
Denstadli, V., Westereng, B., Biniyam, H. G., Ballance, S., Knutsen, S. H., and Svihus, B. (2010). Effects of Structure and Xylanase Treatment of Brewers' Spent Grain on Performance and Nutrient Availability in Broiler Chickens. Br. Poult. Sci. 51, 419–426. doi:10.1080/00071668.2010.495745
Dhillon, G. S., Kaur, S., and Brar, S. K. (2012). In-vitro Decolorization of Recalcitrant Dyes through an Ecofriendly Approach Using Laccase from Trametes versicolor Grown on Brewer's Spent Grain. Int. Biodeterior. Biodegrad. 72, 67–75. doi:10.1016/j.ibiod.2012.05.012
Directorate-General for Research and Innovation (European Commission) (2018). A Sustainable Bioeconomy for Europe: Strengthening the Connection between Economy, Society and the Environment: Updated Bioeconomy Strategy. Brussels: Publications Office of the European Union.
Dos Santos Costa, R., de Almeida, S. S., Cavalcanti, E. d. A. C., Freire, D. M. G., Moura-Nunes, N., Monteiro, M., et al. (2021). Per Rone, DEnzymes Produced by Solid State Fermentation of Agro-Industrial By-Products Release Ferulic Acid in Bioprocessed Whole-Wheat Breads. Food Res. Int. 140, 109843. doi:10.1016/j.foodres.2020.109843
Dudek, M., Świechowski, K., Manczarski, P., Koziel, J. A., and Białowiec, A. (2019). The Effect of Biochar Addition on the Biogas Production Kinetics from the Anaerobic Digestion of Brewers' Spent Grain. Energies 12, 1518. doi:10.3390/en12081518
Eliopoulos, C., Arapoglou, D., Chorianopoulos, N., Markou, G., and Haroutounian, S. A. (2021). Conversion of Brewers' Spent Grain into Proteinaceous Animal Feed Using Solid State Fermentation. Environ. Sci. Pollut. Res. 29, 29562–29569. doi:10.1007/s11356-021-15495-w
Faccenda, A., Zambom, M. A., Castagnara, D. D., Avila, A. S. d., Fernandes, T., Eckstein, E. I., et al. (2017). Use of Dried Brewers' Grains Instead of Soybean Meal to Feed Lactating Cows. R. Bras. Zootec. 46, 39–46. doi:10.1590/S1806-92902017000100007
Farcas, A. C., Socaci, S. A., Chiș, M. S., Pop, O. L., Fogarasi, M., Păucean, A., et al. (2021). Reintegration of Brewers Spent Grains in the Food Chain: Nutritional, Functional and Sensorial Aspects. Plants 10, 2504. doi:10.3390/plants10112504
Femi-Ola, T. O., and Atere, V. A. (2012). Citric Acid Production from Brewers Spent Grain by Aspergillus niger and Saccharomyces cerevisiae. Int. J. Biosci. 2, 30–36.
Fernandes, H., Moyano, F., Castro, C., Salgado, J., Martínez, F., Aznar, M., et al. (2021). Solid-state Fermented Brewer's Spent Grain Enzymatic Extract Increases In Vitro and In Vivo Feed Digestibility in European Seabass. Sci. Rep. 11, 1–15. doi:10.1038/s41598-021-02393-x
Garrett, R., Bellmer, D., McGlynn, W., and Rayas-Duarte, P. (2021). Development of New Chip Products from Brewer's Spent Grain. J. Food Qual. 2021, 1–6. doi:10.1155/2021/5521746
Giacobbe, S., Piscitelli, A., Raganati, F., Lettera, V., Sannia, G., Marzocchella, A., et al. (2019). Butanol Production from Laccase-Pretreated Brewer's Spent Grain. Biotechnol. Biofuels. 12, e47. doi:10.1186/s13068-019-1383-1
Gregori, A. (2014). Cordycepin Production by Cordyceps Militaris Cultivation on Spent Brewery Grains. Acta Biol. Slov. 57, 45–52.
Gupta, M., Abu-Ghannam, N., and Gallaghar, E. (2010). Barley for Brewing: Characteristic Changes during Malting, Brewing and Applications of its By-Products. Compr. Rev. Food Sci. Food Saf. 9, 318–328. doi:10.1111/j.1541-4337.2010.00112.x
Hashemi, M., Razavi, S. H., Shojaosadati, S. A., and Mousavi, S. M. (2011). The Potential of Brewer's Spent Grain to Improve the Production of α-amylase by Bacillus Sp. KR-8104 in Submerged Fermentation System. New Biotechnol. 28, 165–172. doi:10.1016/j.nbt.2010.10.009
Hassan, S. S., Tiwari, B. K., Williams, G. A., and Jaiswal, A. K. (2020). Bioprocessing of Brewers' Spent Grain for Production of Xylanopectinolytic Enzymes by Mucor Sp. Bioresour. Technol. Rep. 9, 100371. doi:10.1016/j.biteb.2019.100371
Heredia-Olea, E., Pérez-Carrillo, E., and Serna-Saldívar, S. O. (2015). Effect of Extrusion Conditions and Hydrolysis with Fiber-Degrading Enzymes on the Production of C5 and C6 Sugars from Brewers' Spent Grain for Bioethanol Production. Biofuel Res. J. 5, 203–208. doi:10.18331/BRJ2015.2.1.6
Ibarruri, J., Cebrián, M., and Hernández, I. (2019). Solid State Fermentation of Brewer's Spent Grain Using Rhizopus Sp. To Enhance Nutritional Value. Waste Biomass Valor 10, 3687–3700. doi:10.1007/s12649-019-00654-5
Ikram, S., Huang, L., Zhang, H., Wang, J., and Yin, M. (2017). Composition and Nutrient Value Proposition of Brewers Spent Grain. J. Food Sci. 82, 2232–2242. doi:10.1111/1750-3841.13794
Izinyon, O., Nwosu, O., Akhigbe, L., and Ilaboya, I. (2016). Performance Evaluation of Fe (Iii) Adsorption onto Brewers' Spent Grain. Nig. J. Tech. 35, 970. doi:10.4314/njt.v35i4.36
Jaeger, A., Zannini, E., Sahin, A. W., and Arendt, E. K. (2021). Barley Protein Properties, Extraction and Applications, with a Focus on Brewers' Spent Grain Protein. Foods 10, 1389. doi:10.3390/foods10061389
Jaguey-Hernández, Y., Tapia-Ignacio, C., Aguilar-Arteaga, K., González-Olivares, L. G., Castañeda-Ovando, E. P., Cruz-Cansino, N., et al. (2022). Thermoplastic Biofilms Obtained from an Arabinoxylan-Rich Fraction from Brewers' Spent Grain: Physicochemical Characterization and Thermal Analysis. Biomass Conv. bioref. 2022, 1–13. doi:10.1007/s13399-021-02288-x
Johnson, P., Paliwal, J., and Cenkowski, S. (2010). Issues with Utilisation of Brewers' Spent Grain. Stewart Postharvest Rev. 6, 1–8. doi:10.2212/spr.2010.4.2
Karp, S. G., Bittencourt Sydney, E., Lorenci Woiciechowski, A., Letti, L. A. J., de Carvalho, J. C., Zevallos Torres, L. A., et al. (2021). “Lignocellulosic Biorefinery for Value-Added Products: the Emerging Bioeconomy,” in Circular Bioeconomy - Current Status and Future Outlook. Editors A. Pandey, R.D. Tyagi, and S. Varjani (Elsevier), 291–321. doi:10.1016/b978-0-12-821878-5.00002-7
Kavalopoulos, M., Stoumpou, V., Christofi, A., Mai, S., Barampouti, E. M., Moustakas, K., et al. (2021). Sustainable Valorisation Pathways Mitigating Environmental Pollution from Brewers' Spent Grains. Environ. Pollut. 270, 116069. doi:10.1016/j.envpol.2020.116069
Kezerle, A., Velić, N., Hasenay, D., and Kovačević, D. (2018). Lignocellulosic Materials as Dye Adsorbents: Adsorption of Methylene Blue and Congo Red on Brewers' Spent Grain. Croat. Chem. Acta 91, 53–64. doi:10.5562/cca3289
Krasznai, D. J., Champagne Hartley, R., Roy, H. M., Champagne, P., and Cunningham, M. F. (2017). Compositional Analysis of Lignocellulosic Biomass: Conventional Methodologies and Future Outlook. Crit. Rev. Biotechnol. 38, 199–217. doi:10.1080/07388551.2017.1331336
Kulisic, B., Lier, M., Perović, M., Matijašević, N., Mandarić, A., and Sauvula-Seppälä, T. (2020). D 1.2: Report on Analysis of BIOEAST National Bioeconomy Related Sectors. Horizon 2020 Project: Advancing Sustainable Circular Bioeconomy in Central and Eastern European Countries. BIOEASTsUP.
Lao, E. J., Dimoso, N., Raymond, J., and Mbega, E. R. (2020). The Prebiotic Potential of Brewers' Spent Grain on Livestock's Health: a Review. Trop. Anim. Health Prod. 52, 461–472. doi:10.1007/s11250-019-02120-9
Leite, P., Sousa, D., Fernandes, H., Ferreira, M., Costa, A. R., Filipe, D., et al. (2021). Recent Advances in Production of Lignocellulolytic Enzymes by Solid-State Fermentation of Agro-Industrial Wastes. Curr. Opin. Green Sustain. Chem. 27, 100407. doi:10.1016/j.cogsc.2020.100407
Li, Q., Chai, L., Yang, Z., Wang, Q., and Wang, Y. (2010). A Comparative Study of Ag(I) Adsorption on Raw and Modified Spent Grain: Kinetic and Thermodynamic Aspects. Water Environ. Res. 82, 2290–2296. doi:10.2175/106143010x12681059116978
Liguori, R., Soccol, C. R., Vandenberghe, L. P. d. S., Woiciechowski, A. L., Marcolongo, L., et al. (2015). Selection of the StrainLactobacillus acidophilusATCC 43121 and its Application to Brewers' Spent Grain Conversion into Lactic Acid. BioMed Res. Int. 2015, 9. doi:10.1155/2015/240231
Lin, P. P., Mi, L., Morioka, A. H., Yoshino, K. M., Konishi, S., Xu, S. C., et al. (2015). Consolidated Bioprocessing of Cellulose to Isobutanol Using Clostridium Thermocellum. Metab. Eng. 31, 44–52. doi:10.1016/j.ymben.2015.07.001
Llimós, J., Martínez-Avila, O., Marti, E., Corchado-Lopo, C., Llenas, L., Gea, T., et al. (2020). Brewer's Spent Grain Biotransformation to Produce Lignocellulolytic Enzymes and Polyhydroxyalkanoates in a Two-Stage Valorization Scheme. Biomass Conv. bioref. doi:10.1007/s13399-020-00918-4
Lynch, K. M., Steffen, E. J., and Arendt, E. K. (2016). Brewers' Spent Grain: a Review with an Emphasis on Food and Health. J. Inst. Brew. 122, 553–568. doi:10.1002/jib.363
Lynch, K. M., Strain, C. R., Johnson, C., Patangia, D., Stanton, C., Koc, F., et al. (2021). Extraction and Characterisation of Arabinoxylan from Brewers Spent Grain and Investigation of Microbiome Modulation Potential. Eur. J. Nutr. 60, 4393–4411. doi:10.1007/s00394-021-02570-8
Machado, C. M. M., Soccol, C. R., de Oliveira, B. H., and Pandey, A. (2021). Gibberellic Acid Production by Solid-State Fermentation in Coffee Husk. Abab 102-103, 179–192. doi:10.1385/abab:102-103:1-6:179
Malomo, O., Daniels, A. O., Olajiga, O., Femi- Ola, T. O., and Alamu, A. E. (2013). The Use of Brewer′S Spent Grains in the Cultivation of Some Fungal Isolates. Ijnfs 2, 5–9. doi:10.11648/j.ijnfs.20130201.12
Mandalari, G., Bisignano, G., Lo Curto, R. B., Waldron, K. W., and Faulds, C. B. (2008). Production of Feruloyl Esterases and Xylanases by Talaromyces stipitatus and Humicola Grisea Var. Thermoidea on Industrial Food Processing By-Products. Bioresour. Technol. 99, 5130–5133. doi:10.1016/j.biortech.2007.09.022
Marcus, A., and Fox, G. (2021). Fungal Biovalorization of a Brewing Industry Byproduct, Brewer's Spent Grain: A Review. Foods 10, 2159. doi:10.3390/foods10092159
Mata, T. M., Tavares, T. F., Meireles, S., and Caetano, N. S. (2015). Bioethanol from Brewers’ Spent Grain: Pentose Fermentation. Chem. Eng. Trans. 43, 241–246. doi:10.3303/CET1543041
Meneses, N. G. T., Martins, S., Teixeira, J. A., and Mussatto, S. I. (2013). Influence of Extraction Solvents on the Recovery of Antioxidant Phenolic Compounds from Brewer's Spent Grains. Sep. Purif. Technol. 108, 152–158. doi:10.1016/j.seppur.2013.02.015
Minty, J. J., Singer, M. E., Scholz, S. A., Bae, C.-H., Ahn, J.-H., Foster, C. E., et al. (2013). Design and Characterization of Synthetic Fungal-Bacterial Consortia for Direct Production of Isobutanol from Cellulosic Biomass. Proc. Natl. Acad. Sci. U.S.A. 110, 14592–14597. doi:10.1073/pnas.1218447110
Moher, D., Liberati, A., Tetzlaff, J., and Altman, D. G. (2009). Preferred Reporting Items for Systematic Reviews and Meta-Analyses: The PRISMA Statement. PLoS Med. 6, e1000097. doi:10.1371/journal.pmed.1000097
Moher, D., Shamseer, L., Shamseer, L., Clarke, M., Ghersi, D., Liberati, A., et al. (2015). Preferred Reporting Items for Systematic Review and Meta-Analysis Protocols (PRISMA-P) 2015 Statement. Syst. Rev. 4, 1. doi:10.1186/2046-4053-4-1
Moran-Aguilar, M. G., Costa-Trigo, I., Calderón-Santoyo, M., Domínguez, J. M., and Aguilar-Uscanga, M. G. (2021). Production of Cellulases and Xylanases in Solid-State Fermentation by Different Strains of Aspergillus niger Using Sugarcane Bagasse and Brewery Spent Grain. Biochem. Eng. J. 172, 108060. doi:10.1016/j.bej.2021.108060
Mussatto, S. I., Dragone, G., and Roberto, I. C. (2007a). Ferulic and P-Coumaric Acids Extraction by Alkaline Hydrolysis of Brewer's Spent Grain. Industrial Crops Prod. 25, 231–237. doi:10.1016/j.indcrop.2006.11.001
Mussatto, S. I., Fernandes, M., Dragone, G., Mancilha, I. M., and Roberto, I. C. (2007b). Brewer's Spent Grain as Raw Material for Lactic Acid Production by Lactobacillus Delbrueckii. Biotechnol. Lett. 29, 1973–1976. doi:10.1007/s10529-007-9494-3
Mussatto, S. I., and Roberto, I. C. (2005). Acid Hydrolysis and Fermentation of Brewer's Spent Grain to Produce Xylitol. J. Sci. Food Agric. 85, 2453–2460. doi:10.1002/jsfa.2276
Nagy, V., and Diósi, G. (2021). Using Brewer's Spent Grain as a Byproduct of the Brewing Industry in the Bakery Industry. Évik 67, 3339–3350. doi:10.52091/EVIK-2021/1-5-ENG
Naibaho, J., Butula, N., Jonuzi, E., Korzeniowska, M., Laaksonen, O., Föste, M., et al. (2022). Potential of Brewers' Spent Grain in Yogurt Fermentation and Evaluation of its Impact in Rheological Behaviour, Consistency, Microstructural Properties and Acidity Profile during the Refrigerated Storage. Food Hydrocoll. 125, 107412. doi:10.1016/j.foodhyd.2021.107412
Naibaho, J., and Korzeniowska, M. (2021). The Variability of Physico-Chemical Properties of Brewery Spent Grain from 8 Different Breweries. Heliyon 7, e06583. doi:10.1016/j.heliyon.2021.e06583
Nazzaro, J., Martin, D. S., Perez-Vendrell, A. M., Padrell, L., Iñarra, B., Orive, M., et al. (2021). Apparent Digestibility Coefficients of Brewer's By-Products Used in Feeds for Rainbow Trout (Oncorhynchus mykiss) and Gilthead Seabream (Sparus aurata). Aquaculture 530, 735796. doi:10.1016/j.aquaculture.2020.735796
Neylon, E., Arendt, E. K., Zannini, E., and Sahin, A. W. (2021). Fermentation as a Tool to Revitalise Brewers' Spent Grain and Elevate Techno-Functional Properties and Nutritional Value in High Fibre Bread. Foods 10, 1639. doi:10.3390/foods10071639
Olugbenga, A. O., and Ibiyemi, I. O. (2011). Bioethanol Production from Brewer’s Spent Grain, Bread Wastes and Corn Fiber. Afr. J. Food Sci. 5, 148–155.
Ortiz, I., Torreiro, Y., Molina, G., Maroño, M., and Sánchez, J. M. (2019). A Feasible Application of Circular Economy: Spent Grain Energy Recovery in the Beer Industry. Waste Biomass Valor 10, 3809–3819. doi:10.1007/s12649-019-00677-y
Outeiriño, D., Costa-Trigo, I., Pinheiro de Souza Oliveira, R., Pérez Guerra, N., and Domínguez, J. M. (2019). A Novel Approach to the Biorefinery of Brewery Spent Grain. Process Biochem. 85, 135–142. doi:10.1016/j.procbio.2019.06.007
Pabbathi, N. P. P., Velidandi, A., Pogula, S., Gandam, P. K., Baadhe, R. R., Sharma, M., et al. (2022). Brewer's Spent Grains-Based Biorefineries: A Critical Review. Fuel 317, 123435. doi:10.1016/j.fuel.2022.123435
Panagiotou, G., Granouillet, P., and Olsson, L. (2006). Production and Partial Characterization of Arabinoxylan-Degrading Enzymes by Penicillium brasilianum under Solid-State Fermentation. Appl. Microbiol. Biotechnol. 72, 1117–1124. doi:10.1007/s00253-006-0394-6
Panjičko, M., Zupančič, G. D., Fanedl, L., Logar, R. M., Tišma, M., and Zelić, B. (2017). Biogas Production from Brewery Spent Grain as a Mono-Substrate in a Two-Stage Process Composed of Solid-State Anaerobic Digestion and Granular Biomass Reactors. J. Clean. Prod. 166, 519–529. doi:10.1016/j.jclepro.2017.07.197
Parchami, M., Ferreira, J. A., and Taherzadeh, M. J. (2021). Starch and Protein Recovery from Brewer's Spent Grain Using Hydrothermal Pretreatment and Their Conversion to Edible Filamentous Fungi - A Brewery Biorefinery Concept. Bioresour. Technol. 337, 125409. doi:10.1016/j.biortech.2021.125409
Pathania, S., Sharma, S., and Kumari, K. (2018). Solid State Fermentation of BSG for Citric Acid Production. Indian J. Nat. Prod. Resour. 9, 70–74.
Plaza, P. E., Gallego-Morales, L. J., Peñuela-Vásquez, M., Lucas, S., García-Cubero, M. T., and Coca, M. (2017). Biobutanol Production from Brewer's Spent Grain Hydrolysates by Clostridium Beijerinckii. Bioresour. Technol. 244, 166–174. doi:10.1016/j.biortech.2017.07.139
Proaño, J. L., Salgado, P. R., Cian, R. E., Mauri, A. N., and Drago, S. R. (2020). Physical, Structural and Antioxidant Properties of Brewer's Spent Grain Protein Films. J. Sci. Food Agric. 100, 5458–5465. doi:10.1002/jsfa.10597
Puligundla, P., and Mok, C. (2021). Recent Advances in Biotechnological Valorization of Brewers' Spent Grain. Food Sci. Biotechnol. 30, 341–353. doi:10.1007/s10068-021-00900-4
Pullin, A. S., and Stewart, G. B. (2007). Guidelines for Systematic Review in Conservation and Environmental Management. Conserv. Biol. 20, 1647–1656. doi:10.1111/j.1523-1739.2006.00485.x
Qiu, L., Li, J.-J., Li, Z., and Wang, J.-J. (2019). Production and Characterization of Biocontrol Fertilizer from Brewer's Spent Grain via Solid-State Fermentation. Sci. Rep. 9, e480. doi:10.1038/s41598-018-36949-1
Radzik-Rant, A., Rant, W., Niżnikowski, R., Świątek, M., Szymańska, Ż., Ślęzak, M., et al. (2018). The Effect of the Addition of Wet Brewers Grain to the Diet of Lambs on Body Weight Gain, Slaughter Valueand Meat Quality. Arch. Anim. Breed. 61, 245–251. doi:10.5194/aab-61-245-2018
Rahman, M. J., Malunga, L. N., Eskin, M., Eck, P., Thandapilly, S. J., and Thiyam-Hollander, U. (2021). Valorization of Heat-Treated Brewers' Spent Grain through the Identification of Bioactive Phenolics by UPLC-PDA and Evaluation of Their Antioxidant Activities. Front. Nutr. 8, 519. doi:10.3389/fnut.2021.634519
Ravindran, R., Williams, G. A., and Jaiswal, A. K. (2019). Evaluation of Brewer's Spent Grain Hydrolysate as a Substrate for Production of Thermostable α-amylase by Bacillus Stearothermophilus. Bioresour. Technol. Rep. 5, 141–149. doi:10.1016/j.biteb.2019.01.004
Rego, F., Soares Dias, A. P., Casquilho, M., Rosa, F. C., and Rodrigues, A. (2019). Fast Determination of Lignocellulosic Composition of Poplar Biomass by Thermogravimetry. Biomass Bioenergy 122, 375–380. doi:10.1016/j.biombioe.2019.01.037
Rojas-Chamorro, J. A., Romero, I., López-Linares, J. C., and Castro, E. (2020). Brewer's Spent Grain as a Source of Renewable Fuel through Optimized Dilute Acid Pretreatment. Renew. Energy 148, 81–90. doi:10.1016/j.renene.2019.12.030
Saba, S., Zara, G., Bianco, A., Garau, M., Bononi, M., Deroma, M., et al. (2019). Comparative Analysis of Vermicompost Quality Produced from Brewers' Spent Grain and Cow Manure by the Red Earthworm Eisenia fetida. Bioresour. Technol. 293, 122019. doi:10.1016/j.biortech.2019.122019
Sajib, M., Falck, P., Sardari, R. R. R., Mathew, S., Grey, C., Karlsson, E. N., et al. (2018). Valorization of Brewer's Spent Grain to Prebiotic Oligosaccharide: Production, Xylanase Catalyzed Hydrolysis, In-Vitro Evaluation with Probiotic Strains and in a Batch Human Fecal Fermentation Model. J. Biotechnol. 268, 61–70. doi:10.1016/j.jbiotec.2018.01.005
Salvador, R., Puglieri, F. N., Halog, A., Andrade, F. G. d., Piekarski, C. M., and De Francisco, A. C. (2021). Key Aspects for Designing Business Models for a Circular Bioeconomy. J. Clean. Prod. 278, 124341. doi:10.1016/j.jclepro.2020.124341
Schettino, R., Verni, M., Acin-Albiac, M., Vincentini, O., Krona, A., Knaapila, A., et al. (2021). Bioprocessed Brewers' Spent Grain Improves Nutritional and Antioxidant Properties of Pasta. Antioxidants 10, 742. doi:10.3390/antiox10050742
Šelo, G., Planinić, M., Tišma, M., Tomas, S., Koceva Komlenić, D., and Bucić-Kojić, A. (2021). A Comprehensive Review on Valorization of Agro-Food Industrial Residues by Solid-State Fermentation. Foods 10, 927. doi:10.3390/foods10050927
Sganzerla, W. G., Ampese, L. C., Mussatto, S. I., and Forster‐Carneiro, T. (2021a). A Bibliometric Analysis on Potential Uses of Brewer's Spent Grains in a Biorefinery for the Circular Economy Transition of the Beer Industry. Biofuels, Bioprod. Bioref. 15, 1965–1988. doi:10.1002/bbb.2290
Sganzerla, W. G., Buller, L. S., Mussatto, S. I., and Forster-Carneiro, T. (2021b). Techno-economic Assessment of Bioenergy and Fertilizer Production by Anaerobic Digestion of Brewer's Spent Grains in a Biorefinery Concept. J. Clean. Prod. 297, 126600. doi:10.1016/j.jclepro.2021.126600
Sibhatu, H. K., Anuradha Jabasingh, S., Yimam, A., and Ahmed, S. (2021). Ferulic Acid Production from Brewery Spent Grains, an Agro-Industrial Waste. Lwt 135, 110009. doi:10.1016/j.lwt.2020.110009
Silbir, S., and Goksungur, Y. (2019). Natural Red Pigment Production by Monascus Purpureus in Submerged Fermentation Systems Using a Food Industry Waste: Brewer's Spent Grain. Foods 8, 161. doi:10.3390/foods8050161
Silva, E. G., Borges, A. S., Maione, N. R., Castiglioni, G. L., Suarez, C. A. G., and Montano, I. D. C. (2019). Fermentation of Hemicellulose Liquor from Brewer's Spent Grain Using Scheffersomyces Stipitis and Pachysolen Tannophilus for Production of 2G Ethanol and Xylitol. Biofuels, Bioprod. Bioref. 14, 127–137. doi:10.1002/bbb.2072
Statista (2021). Beer Production Worldwide in 2020, by Region (In Million Hectoliters). https://www.statista.com/statistics/202417/beer-output-volumes-of-the-different-continents-in-2010/(Accessed March 12, 2022).
Stelick, A., Sogari, G., Rodolfi, M., Dando, R., and Paciulli, M. (2021). Impact of Sustainability and Nutritional Messaging on Italian Consumers' Purchase Intent of Cereal Bars Made with Brewery Spent Grains. J. Food Sci. 86, 531–539. doi:10.1111/1750-3841.15601
Tan, Y. X., Mok, W. K., Lee, J., Kim, J., and Chen, W. N. (2019). Solid State Fermentation of Brewers' Spent Grains for Improved Nutritional Profile Using Bacillus Subtilis WX-17. Fermentation 5, 52. doi:10.3390/fermentation5030052
Tanoue Batista, M. C., Soccol, C. R., Spier, M. R., Libardi Junior, N., and Porto de Souza Vandenberghe, L. (2021). Potential Application of Dextranase Produced by Penicillium aculeatum in Solid-State Fermentation from Brewer's Spent Grain in Sugarcane Process Factories. Biocatal. Agric. Biotechnol. 35, 102086. doi:10.1016/j.bcab.2021.102086
Terrasan, C. R. F., and Carmona, E. C. (2015). Solid-state Fermentation of Brewer's Spent Grain for Xylanolytic Enzymes Production by Penicillium janczewskii and Analyses of the Fermented Substrate. Biosci. J. 31, 1826–1836. doi:10.14393/BJ-v31n6a2015-30044
The Brewers of Europe (2019). Country Profiles. https://brewersofeurope.org/site/countries/key-facts-figures.php (Accessed April 14, 2021).
Tišma, M., Bucić-Kojić, A., and Planinić, M. (2021a). Bio-based Products from Lignocellulosic Waste Biomass. Chem. Biochem. Eng. Q. (Online) 35, 139–156. doi:10.15255/CABEQ.2021.1931
Tišma, M., Jurić, A., Bucić-Kojić, A., Panjičko, M., and Planinić, M. (2018). Biovalorization of Brewers' Spent Grain for the Production of Laccase and Polyphenols. J. Inst. Brew. 124, 182–186. doi:10.1002/jib.479
Tišma, M., Žnidaršič-Plazl, P., Šelo, G., Tolj, I., Šperanda, M., Bucić-Kojić, A., et al. (2021b). Trametes versicolor in Lignocellulose-Based Bioeconomy: State of the Art, Challenges and Opportunities. Bioresour. Technol. 330, 124997. doi:10.1016/j.biortech.2021.124997
Wilkinson, S., Smart, K. A., and Cook, D. J. (2014). Optimisation of Alkaline Reagent Based Chemical Pre-treatment of Brewers Spent Grains for Bioethanol Production. Industrial Crops Prod. 62, 219–227. doi:10.1016/j.indcrop.2014.08.036
Wohlgemuth, R. (2021). Biocatalysis - Key Enabling Tools from Biocatalytic One-step and Multi-step Reactions to Biocatalytic Total Synthesis. New Biotechnol. 60, 113–123. doi:10.1016/j.nbt.2020.08.006
Wolters, N., Schabronath, C., Schembecker, G., and Merz, J. (2016). Efficient Conversion of Pretreated Brewer's Spent Grain and Wheat Bran by Submerged Cultivation of Hericium erinaceus. Bioresour. Technol. 222, 123–129. doi:10.1016/j.biortech.2016.09.121
Xiros, C., and Christakopoulos, P. (2012). Biotechnological Potential of Brewers Spent Grain and its Recent Applications. Waste Biomass Valor 3, 213–232. doi:10.1007/s12649-012-9108-8
Xiros, C., Topakas, E., Katapodis, P., and Christakopoulos, P. (2008a). Evaluation of Fusarium Oxysporum as an Enzyme Factory for the Hydrolysis of Brewer's Spent Grain with Improved Biodegradability for Ethanol Production. Industrial Crops Prod. 28, 213–224. doi:10.1016/j.indcrop.2008.02.004
Xiros, C., Topakas, E., Katapodis, P., and Christakopoulos, P. (2008b). Hydrolysis and Fermentation of Brewer's Spent Grain by Neurospora Crassa. Bioresour. Technol. 99, 5427–5435. doi:10.1016/j.biortech.2007.11.010
Žnidaršič-Plazl, P. (2021a). Let the Biocatalyst Flow. Acta Chim. Slov. 68, 1–16. doi:10.17344/acsi.2020.64
Keywords: Brewer’s spent grain, bio-based products, circular bioeconomy, sustainability, biochemical transformation
Citation: Zeko-Pivač A, Tišma M, Žnidaršič-Plazl P, Kulisic B, Sakellaris G, Hao J and Planinić M (2022) The Potential of Brewer’s Spent Grain in the Circular Bioeconomy: State of the Art and Future Perspectives. Front. Bioeng. Biotechnol. 10:870744. doi: 10.3389/fbioe.2022.870744
Received: 07 February 2022; Accepted: 17 May 2022;
Published: 17 June 2022.
Edited by:
Isabel Belo, University of Minho, PortugalReviewed by:
Joachim Venus, Leibniz Institute for Agricultural Engineering and Bioeconomy (ATB), GermanyElsa Vieira, Chemistry and Technology Network (REQUIMTE), Portugal
Copyright © 2022 Zeko-Pivač, Tišma, Žnidaršič-Plazl, Kulisic, Sakellaris, Hao and Planinić. This is an open-access article distributed under the terms of the Creative Commons Attribution License (CC BY). The use, distribution or reproduction in other forums is permitted, provided the original author(s) and the copyright owner(s) are credited and that the original publication in this journal is cited, in accordance with accepted academic practice. No use, distribution or reproduction is permitted which does not comply with these terms.
*Correspondence: Marina Tišma, bWFyaW5hLnRpc21hQHB0Zm9zLmhy