- 1Department of Orthopaedic Surgery, The First Affiliated Hospital of Fujian Medical University, Fuzhou, China
- 2Department of Stomatology, The First Affiliated Hospital of Fujian Medical University, Fuzhou, China
- 3Department of Orthopaedics & Traumatology, The University of Hong Kong, Pokfulam, Hong Kong SAR, China
Osteoarthritis (OA) is a debilitating joint disease that affects millions of individuals. The pathogenesis of OA has not been fully elucidated. Obesity is a well-recognized risk factor for OA. Multiple studies have demonstrated adipokines play a key role in obesity-induced OA. Increasing evidence show that various adipokines may significantly affect the development or clinical course of OA by regulating the pro/anti-inflammatory and anabolic/catabolic balance, matrix remodeling, chondrocyte apoptosis and autophagy, and subchondral bone sclerosis. Several signaling pathways are involved but still have not been systematically investigated. In this article, we review the cellular and molecular mechanisms of adipokines in OA, and highlight the possible signaling pathways. The review suggested adipokines play important roles in obesity-induced OA, and exert downstream function via the activation of various signaling pathways. In addition, some pharmaceuticals targeting these pathways have been applied into ongoing clinical trials and showed encouraging results. However, these signaling pathways are complex and converge into a common network with each other. In the future work, more research is warranted to further investigate how this network works. Moreover, more high quality randomised controlled trials are needed in order to investigate the therapeutic effects of pharmaceuticals against these pathways for the treatment of OA. This review may help researchers to better understand the pathogenesis of OA, so as to provide new insight for future clinical practices and translational research.
1 Introduction
Osteoarthritis (OA) is the most prevalent arthritis worldwide and a leading cause of pain and physical disability in nearly 10% of males and 18% of females aged 60 years and older (Glyn-Jones et al., 2015). OA is a degenerative disease of the entire joint and occurs most commonly in the hip and knee, characterized by a gradual loss of articular cartilage and remodeling of the subchondral bone (Hochberg et al., 2013). The symptoms and signs of OA include pain, stiffness, deformity and disability, which significantly affect the quality of life of patients (Sharma, 2021). It was estimated that from 1990 to 2019, the number of people affected by OA increased by 48% (Hunter et al., 2020). It should also be noted that the incidence of OA is rising even among young and physically active people (Long et al., 2020).
The pathogenesis of OA has not yet been fully elucidated. Old age, female sex and obesity are primary risk factors of OA (Collins et al., 2020). Obesity is one of the modifiable risk factors for OA, and was conventionally believed to cause OA via the increased mechanical loading on weight-bearing joints, leading to “wear and tear” (Zhang et al., 2018). However, recent evidence showed that this might not be the only pathogenesis. Studies have demonstrated that OA is also common in non-weight bearing joints, such as hand joints, and is more prominent in obese patients, which indicates that OA is not simply a “wear and tear” disease (Neumann et al., 2016; Favero et al., 2022) (Figure 1). Epidemiology study has shown that hand OA was seen in ∼60% of North American and European adults ≥65 years of age—far greater than the ∼33% found in the knee and ∼5% in the hip (Plotz et al., 2021). Increasing evidence suggests that there are multiple subtypes of OA that reflect a complex and multifactorial nature, in which obesity-induced OA has been proposed as a new phenotype of OA that displays a unique characteristic (Sun et al., 2021).
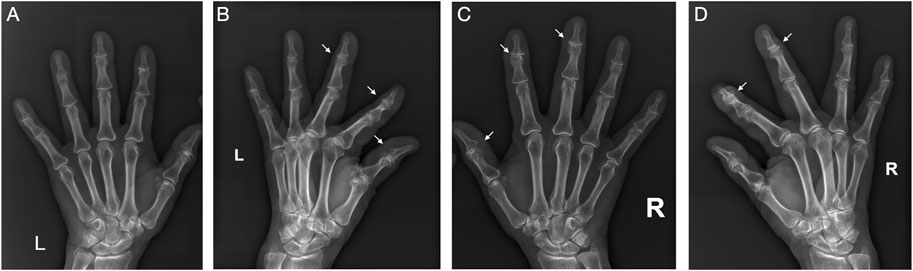
FIGURE 1. Typical X-ray films of OA in hand. This is the X-ray films of both hands of a 71-year-old obese woman (BMI 28.0). Significant manifestations of OA (narrowing of joint space, osteophyte formation, and subchondral bone sclerosis) can be seen in these non-weight-bearing joints. (A) Frontal view of left hand; (B) Lateral view of left hand; (C) Frontal view of right hand; (D) Lateral view of right hand.
The low-grade chronic inflammation seen in obesity-induced OA might be the main characteristic which are different from other phenotypes of OA (Sun et al., 2021). In obese patients, the cytokines produced and released by adipose tissues, which are also termed as “adipokines,” might play important roles (Conde et al., 2015). In this process, adipose tissue secretes various adipokines (leptin, adiponectin, resistin, visfatin, omentin, vaspin, retinol binding protein 4, etc.) and cytokines [interleukin (IL)-1, IL-6, IL-8, tumor necrosis factor (TNF)-α, etc.], and contribute to the degeneration of chondrocyte and breakdown of extracellular matrix (Urban and Little, 2018; Giardullo et al., 2021). The synovial adipokines might come from the secretion of the infrapatellar fat pad itself or from the blood circulation system permeating the synovial membrane and entering the joint cavity. It’s close association with OA have been widely reported in literature (Collins et al., 2020). Clinical data has shown that there was a positive correlation between synovial adipokine level and OA activity index in elderly women with knee OA (Eldjoudi et al., 2022; Ilia et al., 2022).
Several mechanisms have been proposed. First, studies have shown that the single nucleotide polymorphism rs182052 in the ADIPOQ gene encoding adiponectin may modify individual susceptibility to knee OA (Jiang et al., 2021). In addition, dipokines regulate the metabolic balance of joints by regulating cytokines, chemokines, matrix degrading enzymes, and cell growth/differentiation factors (Xie and Chen, 2019). Many studies have shown that adipokines such as leptin and adiponectin released from the synovium, infrapatellar fat pad and osteophytes can upregulate the levels of inflammatory cytokines such as prostaglandin E2 (PGE2), IL-6, IL-8, vascular cell adhesion molecule (VCAM)-1, and TNF-α in knee synovial fluid, and even infiltrating cartilage and activating the degenerative cascade (Ushiyama et al., 2003; Tang et al., 2007; Tong et al., 2008; Moilanen et al., 2009; Conde et al., 2012; Yang et al., 2013; Tsuchida et al., 2014). During the process, multiple signaling pathways were associated, including AMP-activated protein kinase (AMPK)/mammalian target of rapamycin (mTOR), nuclear factor-κB (NF-κB), mitogen-activated phosphokinase (MAPK), etc.
The detailed pathophysiology by which adipokines lead to the onset and progression of OA is not fully understood. To our knowledge, no review has examined the adipokine signaling pathways in OA. In this context, this review aims to summarize the signaling pathways associated with adipokine in OA. We searched for articles containing the key terms “osteoarthritis,” “obesity,” “adipokine,” and “signaling pathway.” A total of over 200 publications were reviewed. Specifically, the reports on the signaling pathways involving in adipokine-associated OA were carefully reviewed. Publications on other phenotypes of OA, such as traumatic OA, were excluded to avoid selection bias. In addition, a structured search in the clinicaltrials.gov database was performed, and all phase II and phase III trials with pharmaceuticals against signaling pathways for the treatment of OA were selected. The purpose of this review is to help to reveal the underlying biological mechanism, so as to develop new therapeutic targets or biomarkers, and eventually provide insights for the development of preventive strategies and effective treatments.
2 Adipokine Signaling Pathways in Osteoarthritis
2.1 Main Signaling Pathways
2.1.1 AMP-Activated Protein Kinase/Mammalian Target of Rapamycin Signaling Pathway
AMPK is a highly conserved cell energy metabolism regulator that plays an important role in the regulation of cell growth and survival and energy metabolism in the body (Hardie, 2014; Garcia and Shaw, 2018). mTOR belongs to the phosphatidylinositol kinase-related kinase family. It is an atypical serine/threonine protein kinase that is highly evolutionarily conserved and is mainly involved in regulating cell growth, proliferation, apoptosis, and autophagy (Chen and Zhou, 2020). There are two types of mTOR protein complexes: mTORC1 and mTORC2.
AMPK/mTOR was also found to be associated with the pathogenesis of OA. When the AMPK signaling pathway is activated, the phosphorylation of AMPK blocks the phosphorylation of the mTOR signaling pathway, inhibits the IL-1β-stimulated catabolic response, downregulates the expression of MMPs and phospho-NF-κB, decreases the levels of apoptotic markers, and eventually regulates the progression of OA (Zhou et al., 2017). The AMPK/mTOR signaling pathway is involved in cartilage degeneration and chondrocyte aging (Feng et al., 2020; Zheng et al., 2021). The activation of AMPK promotes autophagy of chondrocytes and inhibits the production of inflammatory cytokines, such as IL-6 and TNF-α, in OA (Zhao et al., 2018).
Adiponectin is the most abundant adipocytokine secreted by adipocytes and a critical member in glucose metabolism and energy balance (Chen et al., 2015). It has strong anti-inflammatory and anti-apoptotic properties, which are closely related to the development and progression of OA. Adiponectin receptors 1 and 2 (AdipoR 1 and AdipoR 2) are two major transmembrane receptors that interact with adiponectin (Harasymowicz et al., 2021). Kang et al. (2010) reported that adiponectin increased the expression of matrix metalloproteinase (MMP)-1, MMP-3, MMP-13, and inducible nitric oxide synthase (iNOS) in human OA chondrocytes through the AdipoR1/2 and AMPK signaling pathways, resulting in the degradation of cartilage matrix. Berendoncks et al. (2013) and Tong et al. (2011) also found that adiponectin may activate AMPK through AdipoR1, which affects the MMP-3 promoter and leads to cartilage destruction. In addition to its effects on chondrocytes, adiponectin can stimulate the proliferation, differentiation and mineralization of osteoblasts in autocrine and/or paracrine fashion through the AMPK signaling pathway (Kanazawa et al., 2007). Chen et al. (2015) further demonstrated that adiponectin promotes osteogenic differentiation of human adipose-derived stem cells by activating the AMPK pathway. Furthermore, adiponectin can also induce intercellular adhesion molecule-1 (ICAM-1) expression via AMPK to promote the adhesion of monocytes to human OA synovial fibroblasts (Chen et al., 2014). Moreover, AMPK plays an important role in the expression of vascular cell adhesion molecule-1 (VCAM-1) in human and mouse chondrocytes induced by adiponectin and leptin and makes cartilage degradation permanent (Conde et al., 2012).
Leptin is a ubiquitous fat factor produced by fat and other tissues that regulates food intake and energy consumption. The increased expression of leptin and the enhanced effect of leptin on the infrapatellar fat pad, synovium, articular cartilage and bone are also involved in the pathogenesis of OA (Gao et al., 2020). The role of AMPK/mTOR in leptin-induced OA has not been widely reported. Huang et al. (2016) found that the increase in leptin levels in patients with OA significantly stimulated the expression of lysyl oxidase-like 3. Increased lysyl oxidase-like 3 expression induces chondrocyte apoptosis, activates mTORC1 and inhibits chondrocyte autophagy (Huang et al., 2016).
Resistin induces the expression of proinflammatory factors and chemokines in human cartilage, thus inhibiting the synthesis of cartilage matrix. Epidemiological studies have found that resistin levels in serum and synovial fluid are positively correlated with the severity of OA (Song et al., 2016). The role of AMPK/mTOR in resistin-induced OA is also unknown. Su et al. (2017) studied the mechanism by which low shear stress (2 dyn/cm2) regulates the catabolism of resistin in human OA chondrocytes and found that preshear activated the AMPK/sirtuin 1 (SIRT1) signal, but postshear inhibited this signal and regulated the cyclooxygenase-2 expression in human OA chondrocytes induced by resistin.
2.1.2 Nuclear Factor-κB Signaling Pathway
NF-κB is an ubiquitously expressed transcription factor that plays an important role in cell survival, differentiation, proliferation, aging, inflammation, immune response, and apoptosis (Hayden and Ghosh, 2012). Meanwhile, NF-κB has also been proven to be involved in the occurrence and development of OA, including chondrocyte survival and catabolism, as well as synovial inflammation (Roman-Blas and Jimenez, 2006; Rigoglou and Papavassiliou, 2013; Saito and Tanaka, 2017; Choi et al., 2019).
The destruction of cartilage matrix integrity in OA is caused by the increase in chondrocyte catabolism and apoptosis and the decrease in chondrocyte anabolism (Hwang and Kim, 2015; Zheng et al., 2021). Studies have shown that adipokines can induce the expression of matrix-degrading enzymes and/or proinflammatory mediators in chondrocytes through the NF-κB signaling pathway (Choi et al., 2019). Studies have shown that leptin increases the expression of PGE2, IL-6, IL-8, MMP-1, MMP-3, and MMP-13, a disintegrin and metalloproteinase with thrombospondin motifs (ADAMTS)-4, ADAMTS-5, and ADAMTS-9 genes through the NF-κB signaling pathway (Tong et al., 2008; Moilanen et al., 2009; Koskinen et al., 2011; Yaykasli et al., 2015). The increased production of MMPs and ADAMTSs is a marker of the destruction of cartilage homeostasis and the initiator and promoter of OA (Mobasheri et al., 2017). Additionally, adiponectin activates p38 and AMPK through AdipoR1, thereby activating NF-κB on the MMP-3 promoter and leading to cartilage breakdown (Tong et al., 2011). Huang et al. (2010) and Tang et al. (2007) also found that the NF-κB signaling pathway may play a critical role in adiponectin promoting the expression of bone morphogenetic protein (BMP)-2 in osteoblasts and IL-6 in human synovial fibroblasts. Moreover, in one study by Li et al. (2017), resistin was found to combine with toll-like receptor-4 through the p38 MAPK and NF-κB signaling pathways, increasing the expression of chemokine ligand 4 in nucleus pulposus cells and leading to macrophage infiltration. Su et al. (2017) found that human OA chondrocytes exposed to different low shear stress modes have an opposite effect on resistin-induced catabolic cyclooxygenase-2 expression, which is closely related to the NF-κB signaling pathway. That is, preshearing over a short duration inhibits the NF-κB-p65 subunit and cAMP response element binding protein to attenuate the resistin effect. However, postshear for a longer duration enhances the resistin effect by activating only the NF-κB-p65 subunit (Su et al., 2017). Furthermore, resistin can mediate osteoclastogenesis by activating NF-κB (Thommesen et al., 2006). Osteoclastogenesis is also an important pathophysiological change of subchondral bone in OA, as OA is usually associated with subchondral bone sclerosis and remodeling. Another common adipokine, visfatin, was found to induce the expression of MMP-3, MMP-12, MMP-13, IL-6, monocyte chemokine protein 1, and keratinocyte chemokine in osteoblasts and chondrocytes via NF-κB (Laiguillon et al., 2014; Yang et al., 2015).
Lipocalin 2, an adipokine initially isolated from neutrophil granules, has been considered a factor that may damage chondrocyte phenotype, cartilage homeostasis, and growth-plate development (Conde et al., 2017). Studies have demonstrated that NF-κB is a key member of lipocalin 2-induced OA (Guo et al., 2014; Conde et al., 2016). A novel adipokine, Nesfatin-1, was also found to stimulate NF-κB pathway (Lee et al., 2021).
2.1.3 Janus Kinase/Signal Transducer and Activator of Transcription Signaling Pathway
The Janus Kinase (JAK)/Signal Transducer and Activator of Transcription (STAT) signaling pathway is the process by which STAT is phosphorylated and dimerized by JAK and then transported to the nucleus through the nuclear membrane to regulate the expression of related genes (Xin et al., 2020). It is an ubiquitously expressed intracellular signal transduction pathway that participates in many important biological processes, including cell proliferation, differentiation, apoptosis, and immune regulation (Rawlings et al., 2004).
Leptin, in one study, has been proven to induce chondrocyte apoptosis and produce MMP-3, MMP-13, reactive oxygen species, iNOS, NO, and VCAM-1 through the JAK/STAT signaling pathway (Otero et al., 2003, 2005; Koskinen et al., 2011; Conde et al., 2012; Zhang et al., 2016). Ben-Eliezer et al. (2007) also demonstrated that the effects of leptin on growth-plate chondrocytes are specifically mediated by STAT 3. Pallu et al. (2010) further found that 500 ng/ml leptin triggers signal transduction through the STAT signaling pathway and thus induces the expression of insulin growth factor (IGF)-1, type 2 collagen, tissue inhibitors of metalloproteinase (TIMP)-2 and MMP-13. Although leptin at 100 ng/ml failed to activate STAT 3, it could induce STAT 1α phosphorylation in the chondrocytes of obese patients (Pallu et al., 2010). Leptin receptor activation initiated by leptin binding was reported to activate multiple signaling pathways including JAK/STAT (Eldjoudi et al., 2022).
Lipocalin 2 is a novel adipokine that has a negative effect on articular cartilage and triggers the catabolism and inflammatory response of chondrocytes in OA. Guo et al. (2014) suggested that lipocalin 2 is a regulator of macrophage polarization and STAT 3 signaling pathway activation. Studies have also shown that IL-1 induces the expression of lipocalin 2 in chondrocytes through JAK2 (Conde et al., 2017).
2.1.4 Mitogen-Activated Phosphokinase Signaling Pathway
MAPK is an important pathway of eukaryotic signal transduction. It regulates many cell biological processes in OA, including proliferation, differentiation, migration and apoptosis (Lan et al., 2021). MAPK signal transduction is carried out via a three-level kinase cascade. First, MAPK kinase (MAPKKK) is activated by mitogen-stimulated phosphorylation. On this basis, MAPKKK phosphorylates and activates MAPK kinase (MAPKK). Last, MAPKK phosphorylates MAPK to activate it and then transfers it into the nucleus. MAPK is a proline-directed protein kinase that can phosphorylate serine or threonine residues adjacent to proline. Thus, MAPKs activate many protein kinases, transcription factors, and nuclear proteins, resulting in downstream signal transduction (Cuschieri and Maier, 2005).
Increased MAPK activity has been found in human OA. Currently, p38, c-Jun N-Terminal Kinase (JNK), and Extracellular Signal-Regulated Kinase (ERK)½ in the MAPK family have been investigated and found to be involved in the pathogenesis of OA (Saklatvala, 2007). The p38 and JNK MAPK signaling pathways are important signals involved in the regulation of the inflammatory response. A variety of external stressors, such as cytokines and hypoxia, can cause the phosphorylation of JNK and p38 and lead to the chain reaction of intracellular protein kinase (Shi et al., 2016; Sun et al., 2017). The ERK½ MAPK signal transduction pathway is the key factor determining cell fate when stimulated extracellularly. Its main function is to promote proliferation and regulate cell terminal differentiation (Cuschieri and Maier, 2005).
2.1.4.1 p38 Mitogen-Activated Phosphokinase Signaling Pathway
p38 MAPK plays a pivotal role in cartilage destruction in adipokine associated OA. Researchers have found that leptin dose-dependently stimulates the proliferation of abnormal OA osteoblasts and increases the levels of phosphorylated p38 and ERK½ MAPK (Ben-Eliezer et al., 2007; Mutabaruka et al., 2010). However, inhibition of the p38 MAPK signaling pathway fails to block the effect of leptin on the expression of type X collagen in ATDC5 chondrogenic cells (Ben-Eliezer et al., 2007). Additionally, studies have shown that leptin can increase the expression of PGE2, IL-6, IL-8, nitric oxide synthase (NOS) type II, ADAMTS-4, ADAMTS-5, ADAMTS-9, MMP-1, and MMP-13 in human chondrocytes through the p38 MAPK signaling pathway (Otero et al., 2005, 2007; Moilanen et al., 2009; Koskinen et al., 2011; Yaykasli et al., 2015). Adiponectin, another key adipokine, increases the expression of BMP-2 in osteoblasts, MMP-3 in human chondrocytes, and IL-6 in human synovial fibroblasts via the p38 MAPK signaling pathway (Tang et al., 2007; Huang et al., 2010; Tong et al., 2011).
2.1.4.2 c-Jun N-Terminal Kinase Mitogen-Activated Phosphokinase Signaling Pathway
JNK was initially known as a 54-kDa stress-activated protein kinase (SAPK), which is responsible for immune reactions and cellular functions, such as cell growth, differentiation, survival, and apoptosis (Chen et al., 2001; Ge et al., 2017). Many studies have indicated that JNK is phosphorylated and activated in OA and is closely associated with cartilage destruction in the pathogenesis of obesity-induced OA. Wang et al. (2016) demonstrated that dual specificity protein phosphatase 19, a downstream target of leptin, inhibited chondrocyte apoptosis by dephosphorylating JNK. In another study, Lee et al. (2015) found that leptin suppresses TNF-α-induced chondrocyte death through the JNK MAPK signaling pathway in rat articular chondrocytes. Additionally, studies have shown that leptin-induced NO, PGE2, IL-6, and IL-8 production was reduced by inhibitors of JNK (Moilanen et al., 2009). On the other hand, adiponectin leads to the degradation of the OA cartilage matrix and increases the expression of MMPs and iNOS in human OA chondrocytes through the JNK MAPK pathway (Kang et al., 2010). Luo et al. (2005) also found that adiponectin could stimulate human osteoblast proliferation and differentiation through p38 and JNK but not the ERK ½ MAPK signaling pathway. Furthermore, the JNK MAPK signaling pathway is related to adiponectin increasing the expression of ICAM-1 in human OA synovial fluid and promoting the adhesion between monocytes and OA synovial fluid (Chen et al., 2014).
2.1.4.3 Extracellular Signal-Regulated Kinase½ Mitogen-Activated Phosphokinase Signaling Pathway
Although ERK½ MAPK has no direct impact on the degradation of the extracellular matrix like p38 MAPK and JNK MAPK, it mainly mediates the proliferation and hypertrophic differentiation of chondrocytes, leading to cartilage calcification and osteophyte formation (Lin et al., 2021). As the only cell of articular cartilage, the fate of chondrocytes determines the formation and development of adipokine-associated OA.
Leptin, as a proinflammatory fat factor, was found to play a key role in cartilage metabolism by inducing the upregulation of MMP1 and MMP13 expression with concomitant activation of the STAT, MAPK (p38, JNK, and ERK), Akt, and NF-κB signaling pathways. Otero et al. (2005), Otero et al. (2007) strongly suggested that ERK½ MAPK and p38 MAPK play pivotal roles in the activation of leptin-mediated NOS type II. Moreover, ERK has also been reported to be involved in activating lipocalin 2 by IL-1α during OA progression (Conde et al., 2017). Collagen II expression was also found to be stimulated by leptin-medicated MAPK/ERK signaling pathway in an in vivo study (Wang et al., 2022).
Chemerin is a novel adipokine identified in 2007. It is highly expressed in the synovial fluid and synovial membrane of patients with OA and is positively correlated with the severity of OA (Huang et al., 2012; Ma et al., 2015). Berg et al. (2010) suggested that the stimulation of chondrocytes with chemerin could lead to the phosphorylation of ERK½ MAPK and Atk. Additionally, the levels of proinflammatory cytokines, such as IL-1β, IL-6, IL-8, TNF-α, MMP-1, MMP-2, MMP-3, MMP-8, and MMP-13, are significantly increased (Berg et al., 2010).
2.1.5 Activating Protein-1 (AP-1) Signaling Pathway
Activating Protein-1 (AP-1) is most often defined as a collective term, which refers to dimeric transcription factors composed of Jun (v-Jun, c-Jun, JunB, and JunD), Fos (v-Fos, c-Fos, FosB, Fral-1, and Fra2), activating transcription factor (ATF-2, ATF-3/LRF1, ATF-4, ATF-5, ATF-6B, ATF-7, BATF, BATF-2, BATF-3, and JDP2), and MAF (c-MAF, MAFA, MAFA-B, MAFA-F, MAFA-G, MAFA-K, and Nrl) subunits, which bind to a common DNA site (Bejjani et al., 2019).
The AP-1 signaling pathway may be the signaling pathway of leptin-induced IL-6 production in OA synovial fibroblasts. Yang et al. (2013) found that leptin activated IRS-1/PI3K/Akt signaling and increased the production of IL-6 by binding with the OBRI receptor, thus enhancing the transcriptional activity of AP-1 and eventually leading to the transcription of IL-6. There are three known cis-regulatory elements in the promoter region of the IL-6 gene, including AP-1, NF-κB, and C/EBP-β. Yang et al. (2013) confirmed that leptin-stimulated luciferase activity was eliminated by AP-1 binding site mutation, and an AP-1 inhibitor (curcumin) and c-jun siRNA could antagonize the increase in IL-6 expression mediated by leptin. In addition, the stimulation of OA synovial fibroblasts by leptin increased c-Jun phosphorylation in a time-dependent manner. Moreover, leptin increased the binding of c-Jun to the AP-1 (-312 to -39) element in the IL-6 promoter (Yang et al., 2013).
AP-1 is a critical transcription factor that controls ICAM-1 production and cell movement during the progression of adiponectin-induced OA. By exploring the effect of adiponectin on the expression of ICAM-1 in synovial fibroblasts, researchers found that adiponectin led to the transactivation of ICAM-1 expression by enhancing the binding of the AP-1 transcription factor and ICAM-1 promoter and promoted the adhesion of monocytes to human OA synovial fibroblasts through the AP-1 pathway (Chen et al., 2014).
2.1.6 Insulin Receptor Substrate-1 Signaling Pathway
Insulin Receptor Substrate-1 (IRS-1) is the main substrate of receptor tyrosine kinase for insulin and insulin-like growth factor 1, and it is also a substrate of IL-4-activated tyrosine kinase and has been associated with adipokine associated OA pathophysiology (Myers et al., 1994). Specifically, leptin in the synovium may play a proinflammatory role in the pathogenesis of OA through the IRS-1 signaling pathway (Gao et al., 2020). Studies have shown that leptin induces the production of IL-8 in OA synovial fibroblasts via an IRS-1/PI3K/Akt/NF-κB-dependent pathway (Tong et al., 2008). Yang et al. and Tang et al. found that leptin also increased the production of IL-6 via the activation of the IRS-1/PI3K/Akt/NF-κB signaling pathway (Yang et al., 2013).
2.1.7 Phosphatidyl Inositol 3 Kinase-Protein Kinase B Signaling Pathway
The Phosphatidyl Inositol 3 Kinase (PI3K)-Protein Kinase B (Akt) signaling pathway functions in many cellular processes that are essential for homeostasis, including the cell cycle, survival, metabolism, inflammation, and apoptosis (Tang et al., 2018). Molecules, such as adipokines and cytokines, can activate receptor tyrosine kinases and G protein-coupled receptors and then activate PI3K to generate phospholipase (De Santis et al., 2019). Akt, as a downstream effector of PI3K, is subsequently activated by these signals. Activated Akt is transferred to other cellular compartments to activate various downstream substrates, including metabolic enzymes, protein kinases, small G protein regulators, E3 ubiquitin ligases, and transcription factors (Manning and Toker, 2017). The PI3K-Akt signaling pathway plays an important regulatory role in cartilage homeostasis, subchondral bone dysfunction and synovitis (Sun et al., 2020). A previous study suggested that the PI3K-Akt signaling pathway is downregulated in human cartilage with OA compared with normal cartilage (Rosa et al., 2011). Specifically, inhibition of the PI3K-Akt signaling pathway can promote the proliferation, apoptosis, and autophagy of articular chondrocytes and reduce the inflammatory response in OA (Xue et al., 2017; Sun et al., 2020). Many studies have shown that adipokines can regulate the progression of OA through the PI3K-Akt signaling pathway.
Visfatin is also a newly discovered proinflammatory adipokine produced by visceral white adipose tissue, which can be found in muscle, bone, synovium, and cartilage (Franco-Trepat et al., 2019). Vascular endothelial growth factor and endothelial progenitor cells are critical factors that promote angiogenesis of the pannus in OA (Kiewisz et al., 2016; Patel et al., 2016; MacDonald et al., 2018). Studies have shown that visfatin inhibits the synthesis of miRNA-485-5p through the PI3K-Akt signaling pathway, which affects the expression of vascular endothelial growth factor in OA synovial fluid and the angiogenesis of endothelial progenitor cells (Tsai et al., 2020).
Vaspin (visceral adipose tissue-derived serine protease inhibitor), a novel adipokine, is produced by skeletal muscle and participates in bone metabolism in an obesity-dependent manner (Tarabeih et al., 2020). Researchers have declared that vaspin can inhibit osteogenic differentiation by activating the PI3K-Akt signaling pathway. During this process, PI3K-Akt and miRNA-34c constitute a modulation loop and control the expression of each other (Liu et al., 2016). Another study has also indicated that PI3K-Akt is critical in Vaspin-induced proliferation of BMSc in OA (Wang et al., 2021).
Apelin is an adipokine involved in the pathogenesis and angiogenesis of OA (Wang et al., 2020). It plays a catabolic role in cartilage metabolism. Specifically, apelin could stimulate chondrocyte proliferation and significantly increase the catabolic factors MMP-1, MMP-3, MMP-9, ADAMTS-4, ADAMTS-5, and the proinflammatory cytokine IL-1β as well as reduce the level of type II collagen (Hu et al., 2010). Chang et al. first proved that apelin stimulates IL-1β expression by activating the PI3K and ERK signaling pathways and inhibiting the downstream expression of miRNA-144-3p in OA synovial fibroblasts (Chang et al., 2020).
Omentin-1, also known as intelectin-1, is a newly identified anti-inflammatory adipokine involved in lipid metabolism (Chai et al., 2020). It has been reported that omentin-1 can stimulate the proliferation and inhibit the differentiation of mouse osteoblasts (Xie et al., 2011, 2012). Wu et al. (2013) found that omentin-1 could induce human osteoblast proliferation through the PI3K-Akt signaling pathway.
Furthermore, stimulation of OA synovial fluid with leptin leads to time-dependent phosphorylation of Akt (Yang et al., 2013). Additionally, leptin can promote the expression of IL-6 in human OA synovial fluid and IL-8 in human synovial fibroblasts through the PI3K-Akt signaling pathway (Tong et al., 2008; Yang et al., 2013). In addition, PI3K was demonstrated to be involved in leptin and adiponectin increasing the expression of VCAM-1 in human and mouse chondrocytes (Conde et al., 2012). Moreover, PI3K could induce the expression of lipocalin 2 in cartilage and affect cartilage homeostasis (Guo et al., 2014). Chemerin can also induce Akt phosphorylation in chondrocytes and increase the levels of proinflammatory cytokines, including IL-6, IL-8, IL-1β, TNF-α, and MMPs (Berg et al., 2010).
2.1.8 Peroxisome Proliferator-Activated Receptor Signaling Pathway
Peroxisome Proliferator-Activated Receptor (PPAR) is a ligand-activated transcription factor that plays a key regulatory role in lipid metabolism and energy homeostasis (Huang et al., 2021). There are three PPAR isotypes, namely, α, γ and β/δ (Dubois et al., 2017).
PPARs regulate the homeostasis of articular cartilage via various pathways and reduce the inflammatory response of OA cartilage. First, PPAR-γ, known as the main adipogenesis regulator, may affect the deposition of fat in skeletal muscle and connective tissue. Fat deposition is an important risk factor for knee OA. The main adipose tissue of the knee joint is the infrapatellar fat pad, which can produce inflammatory cytokines and adipokines. Therefore, the activation of the PPAR-γ signaling pathway may promote adipogenesis, which could be related to the pathological changes in the infrapatellar fat pad in OA (Cordani et al., 2013; Reggio et al., 2019, 2020; Cerquone Perpetuini et al., 2020). Meanwhile, the lack of PPAR-γ in articular cartilage may accelerate the cartilage destruction and progression of OA by reducing chondrocyte differentiation and proliferation and increasing catabolic activity (Monemdjou et al., 2012; Vasheghani et al., 2013, 2015). Studies have shown that stimulation of adiponectin leads to increased PPAR-α ligand activity, fatty acid oxidation and glucose uptake in skeletal muscle (Kanazawa et al., 2007). It was also reported that the PPAR-γ coactivator (PGC)-1α critically mediates anti-catabolic activity in human knee OA chondrocytes (Wang et al., 2015). In addition, the activation of PPAR was found to significantly reduce the synthesis of key adipokine-associated OA mediators, such as MMPs, ADAMTS-5, TNF-α, PGE2, IL1-β, IL-6 and nitric oxide (NO), and inhibit the activation of the ERK½ MAPK, p38, AP-1, and NF-κB signaling pathways (Boileau et al., 2007; Clockaerts et al., 2011; Fahmi et al., 2011; Scirpo et al., 2015). Ratneswaran et al. (2015) also reported that the activation of PPAR-δ could induce the upregulation of proteolytic active genes in cartilage principal extracellular matrix as well as increase the degradation of aggrecan and the release of glycosaminoglycan in knee joint explants. Cartilage-specific PPAR-δ knockout mice showed a strong protective effect on cartilage degeneration in the destabilization of the medial meniscus model of post-traumatic OA (Ratneswaran et al., 2015). Moreover, activated PPAR can inhibit the activation of NF-κB induced by adipokines or promote its inactivation through the following mechanisms, leading to the inhibition of inflammation. PPAR interferes with the activation of NF-κB in the inflammatory response mainly by upregulating the expression of IκBα, sirtuin 1, and phosphatase and tensin homolog (Korbecki et al., 2019). A possible mechanism of PPAR promoting NF-κB inactivation is that PPAR, as an E3 ubiquitin ligase, can physically interact with p65 NF-κB, which leads to inactivation and ubiquitination of p65 NF-κB and finally results in the proteolytic degradation of p65 NF-κB (Hou et al., 2012). Furthermore, adiponectin could selectively activate human monocytes into anti-inflammatory M2 macrophages through the PPAR-α/γ signaling pathway, thus controlling the progression of OA (Lovren et al., 2010).
2.1.9 Wnt/β-Catenin Signaling Pathway
Wnt is an extracellularly secreted glycoprotein. Its signal transduction involves 19 Wnt genes and various Wnt receptors that regulate the canonical β-catenin-dependent signaling pathway (Wang et al., 2019). The overactivation of the Wnt/β-catenin signaling pathway is related to the degradation process of OA (Huang et al., 2020; Palma and Nalesso, 2021). Wang et al. (2017) investigated the effects of adiponectin on osteogenic differentiation and bone formation of bone mesenchymal stem cells (BMSCs). They conducted both in vivo and in vitro studies and found higher gene and protein expression levels of osteogenesis-related genes and Wnt/β-catenin pathway-related factors β-catenin and cyclin D1 in adiponectin transgenic BMSCs and rats. Therefore, adiponectin can facilitate osteogenic differentiation and bone formation of BMSCs via the Wnt/β-catenin signaling pathway (Wang et al., 2017).
2.2 Other Pathways
2.2.1 RhoA/ROCK Signaling Pathway
Rho kinase (ROCK), a serine/threonine kinase, is a downstream effector of member A of the Ras homologous gene family (RhoA) and is involved in regulating cell migration, proliferation and survival (Cai et al., 2021). The abnormal activation of RhoA/ROCK signaling is involved in the early response to abnormal mechanical stimulation, which is considered to be a promoter of the progression of OA. RhoA/ROCK interacts with OA pathological factors, including β-catenin, transforming growth factor (TGF), epidermal growth factor receptor (EGFR), IL-1, insulin-like growth factor-1 (IGF-1) and leptin, and induces cartilage degeneration through the degradation of chondrocyte extracellular matrix (Deng et al., 2019). Liang et al. (2011) proved that leptin mediates cartilage cytoskeleton remodeling through the RhoA/ROCK signaling pathway and its downstream mediators LIMK1 and cofilin-2.
2.2.2 Sirtuin-1 Signaling Pathway
SIRT-1 is a histone deacetylase. SIRT-1 regulates gene expression and protein function by deacetylating lysine residues in histones and nonhistones (Matsuzaki et al., 2014). It is reported that SIRT-1 regulates aging and age-related diseases in simple eukaryotes (Chen et al., 2020).
It is widely recognized that SIRT-1 activity plays an anti-catabolic role in chondrocytes in the development of OA (Gabay et al., 2013; Matsuzaki et al., 2014; Goldring and Berenbaum, 2015). Researchers have found that SIRT-1 is involved in the pathogenesis of OA by regulating chondrocyte gene expression and hypertrophy (Fujita et al., 2011). SIRT-1 increases the expression of the gene encoding cartilage extracellular matrix in human chondrocytes and improves the survival rate of human OA chondrocytes by inhibiting apoptosis (Takayama et al., 2009; Gagarina et al., 2010; Goldring and Berenbaum, 2015). Moreover, SIRT-1 could also exert anti-inflammatory effects in different tissues by inhibiting the transcription of pro-inflammatory genes (Michan and Sinclair, 2007). Studies have shown that SIRT-1 inhibits the expression of cartilage-degrading enzymes induced by IL-1β and TNF-α by regulating the NF-κB pathway (Matsushita et al., 2013; Moon et al., 2013). In particular, Su et al. (2017) reported that low shear stress regulates the expression of resistin-induced catabolic cyclooxygenase-2 in human OA chondrocytes via the AMPK/SIRT-1 signaling pathway and then NF-κB and cAMP response element binding protein transcription factors. Specifically, AMPK activity and SIRT-1 expression in human OA chondrocytes decrease under resistin-induced cyclooxygenase-2 expression. In addition, the attenuation of the preshear effect and the enhancement of the postshear effect of resistin-induced cyclooxygenase-2 expression are caused by the regulation of AMPK and then SIRT-1 signaling (Su et al., 2017). Furthermore, Patel et al. suggested that omentin-1 reduced the expression of IL-1β-induced senescent factors (including caveolin-1, p21 and PAI-1) and p53 acetylation by ameliorating SIRT1 reduction (Chai et al., 2020).
2.2.3 p53/p21 Signaling Pathway
The p53/p21 signaling pathway is implicated in the progression and severity of OA (Xu et al., 2020). In knee OA cases, the protein and mRNA expression of p53 is significantly higher than that in healthy controls (Zhu et al., 2016). Xu et al. (2020) found that chondrocyte apoptosis and p53 increased during the progression of OA, while the expression of SIRT1 decreased in human cartilage. The deletion of SIRT1 in cartilage leads to the acceleration of OA through the abnormal activation of senescence-associated secretory phenotype, hypertrophy, and apoptosis mediated by p53/p21 (Xu et al., 2020). Zhao et al. (2016) further confirmed that high-dose leptin induces cell cycle arrest and senescence in chondrogenic progenitor cells by activating the p53/p21 signaling pathway and inhibiting the SIRT1 signaling pathway.
2.2.4 Calcium Calmodulin-Dependent Kinase II Signaling Pathway
Calcium Calmodulin-Dependent Kinase II (CaMKII) is a serine/threonine protein kinase and a general integrator of Ca2+ signaling (Wei et al., 2018). The upregulation of CaMKII is highly correlated with the pathogenesis and progression of OA and the reactivation of articular cartilage hypertrophy (Saitta et al., 2019; Cai et al., 2021). Studies have demonstrated that activated phosphorylated-CaMKII may play a key role in chondrocyte apoptosis through the MAPK and Akt/mTOR signaling pathways (Wei et al., 2018). Researchers have proven that adiponectin can promote ICAM-1 expression and monocyte adhesion in synovial fibroblasts through the CaMKII signaling pathway (Chen et al., 2014).
2.2.5 Protein Kinase C and Protein Kinase A Signaling Pathways
Many studies have indicated that Protein Kinase C (PKC) and Protein Kinase A (PKA) have a close relationship with adipokine-induced OA. Thommesen et al. revealed that resistin stimulates the proliferation of preosteoblasts (MC3T3-E1) and the differentiation of preosteoclasts (RAW 264.7) through the PKC and PKA signaling pathways (Thommesen et al., 2006). Studies have also shown that vaspin inhibits TNF-α-induced ICAM-1 expression via activation of PKC, thereby inhibiting the inflammatory state of vascular smooth muscle cells during OA progression (Phalitakul et al., 2011). In addition, PKC is involved in leptin-induced MMP production (Koskinen et al., 2011).
2.2.6 ATF4/RANKL Signaling Pathway
ATF4 is an osteoblast-specific member of the cyclic AMP response-binding protein (CREB) family. It has been reported that leptin regulates osteoclast differentiation and osteoblast proliferation by using ATF4 and its target gene RANKL as transcription medium through sympathetic signals (Fu et al., 2005). In addition, adipokine-induced IL-6 also triggers osteoclast formation and bone resorption through the ATF4/RANKL signaling pathway (Xie and Chen, 2019).
2.2.7 Bone Morphogenetic Protein-2 Signaling Pathway
BMP-2 is an important growth factor promoting osteogenesis and plays a pivotal role in osteoblastic differentiation and bone formation. It has been found to be expressed at low levels in the synovial fluid of OA patients (Yang et al., 2018). Studies have shown that adiponectin affects the progression of OA by enhancing the expression level of BMP-2 in osteoblasts (Huang et al., 2010).
2.2.8 CCAAT/Enhancer-Binding Protein-β Signaling Pathway
The CCAAT/Enhancer-Binding Protein (C/EBP-β) pathway is a key signaling pathway that regulates the downstream target genes of osteoblastogenesis. The expression of C/EBP-β is a reliable output for measuring the activity of osteoblastogenesis at the cellular level (Wang et al., 2018). It has been confirmed that resistin can activate the C/EBP-β signaling pathway and thus upregulate proinflammatory cytokines and chemokines (Zhao et al., 2019).
2.2.9 Notch Signaling Pathway
Notch is a single-pass transmembrane cell surface receptor that plays a key role in cell fate by regulating cell differentiation and apoptosis. The direct transcription targets downstream of Notch are Hes1, Hes5, Hes7, Hey1, Hey2, and HeyL, of which only Hes1 is highly expressed in articular chondrocytes (Hosaka et al., 2013; Sugita et al., 2015). Activation of the Notch signaling pathway affects the occurrence of OA by enhancing the production of inflammation-related molecules in OA synovial cells and chondrocytes (Saito and Tanaka, 2017). Leptin can stimulate the expression of IL-6 in serum and OA synovial fluid. The upregulated IL-6 then reduces the synthesis of bone proteoglycans and increases the expression of the decomposition factor MMP13 through the Notch signaling pathway, resulting in the degradation of proteoglycans and the suppression of cartilage formation (Zanotti and Canalis, 2013; Yan et al., 2018).
3 Signaling Pathways of Osteoarthritis as Diagnostic Biomarker and Therapeutic Targets
Signaling pathway as a diagnostic biomarker of OA is rarely reported in the literature. Most studies on diagnostic biomarkers still focus on collagenous markers of cartilage decomposition, metabolic mediators (mainly adipokines) and inflammatory mediators (mainly cytokines) (Kumavat et al., 2021). Only a few literatures have reported the potential of signaling pathway as a diagnostic biomarker of OA, mainly by means of high throughout sequencing and bioinformatics analysis (Zhang et al., 2020). Therefore, in the future work, whether the signal pathway or its key components can be used for early diagnosis of OA needs further research.
To date, the key management strategies for OA have included non-pharmacological (e.g., education and self-management, exercises, weight loss if overweight), pharmacological (e.g., NSAIDs and intra-articular injection of corticosteroids), and surgical approaches. (Sun et al., 2020). There are currently no approved disease-modifying treatments for osteoarthritis. Although many preclinical studies have reported various signaling pathways involved in OA, as reviewed above, there are still few drugs applied in clinical trials. (Table 1) Moreover, some studies have even been terminated. Our review can provide ideas and directions for more clinical trials in the future.
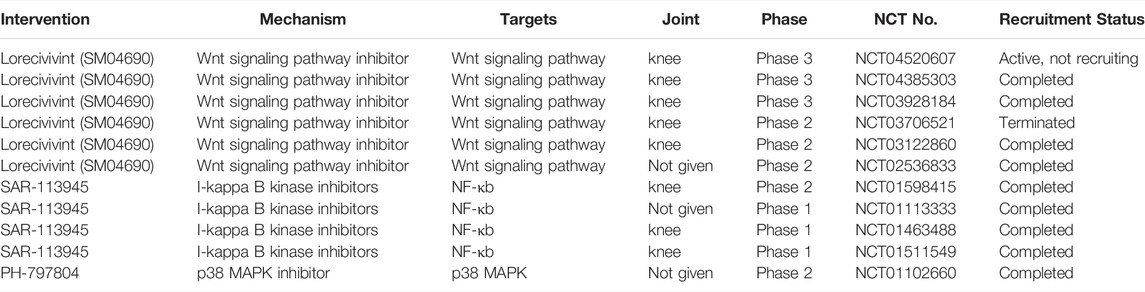
TABLE 1. Ongoing clinical trials investigating inhibitors of signaling pathways as treatment for OA.
4 Summary and Future Perspectives
In this review, we summarize the possible adipokine signalling pathways in OA reported in the current literature, including the ones that are common and classic, such as AMPK/mTOR and NF-κB, and some novel pathways, such as C/EBP-β and ATF4/RANKL. Various studies have suggested adipokines play important roles in obesity-induced OA, and exert downstream function via the activation of these signaling pathways. In addition, based on these findings, some pharmaceuticals targeting these pathways (such as Wnt, NF-κB, and p38 MAPK) have been applied into ongoing clinical trials and showed encouraging results. Inhibitors or antibodies against some novel pathways have demonstrated excellent results in alleviating OA in preclinical studies. These data might provide novel therapeutic strategies for the treatment of OA, especially obesity-induced OA.
However, these signaling pathways are complex and converge into a common network with each other (Figure 2). It has to be noted that none of the adipokines mediates the downstream reaction via a single signaling pathway, and a specific signaling pathway is also the common target of several different adipokines. Therefore, in the future work, more research is warranted to further investigate how this network works. Moreover, more high quality randomised controlled trials are needed in order to investigate the therapeutic effects of pharmaceuticals against these pathways for the treatment of OA, as these drugs may serve as disease-modifying drugs. Our review may help researchers to better understand the pathogenesis of OA, so as to provide new insight for future clinical practices and translational research.
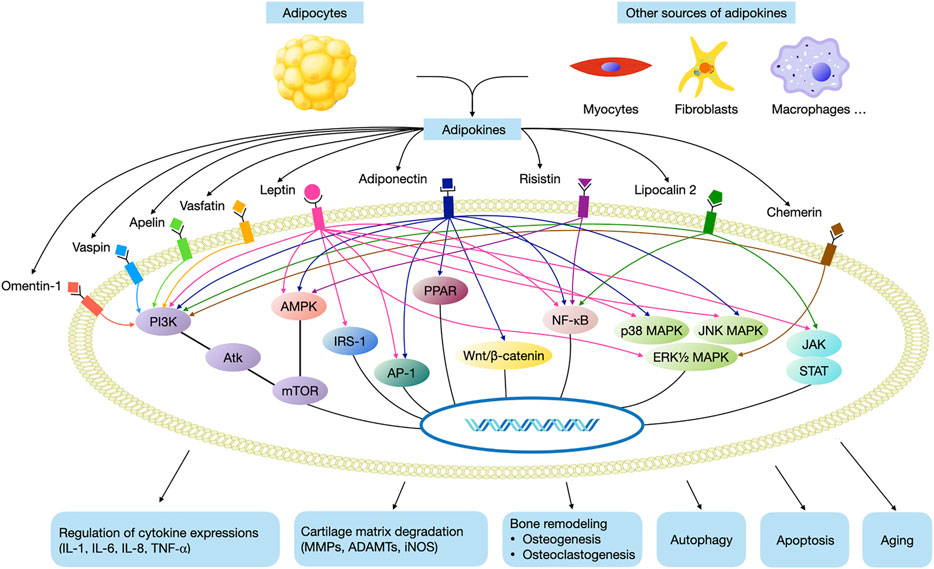
FIGURE 2. Main adipokine signaling pathways in OA. (AMPK, AMP-activated protein kinase; mTOR, mammalian target of rapamycin; NF-κB, nuclear factor-κB, JAK, janus kinases; STAT, signal transducer and activator of transcription, MAPK, mitogen activated phosphokinase; AP-1, activating protein-1; IRS-1, insulin receptor substrate-1; PI3K, phosphatidyl inositol 3 kinase; Akt, protein kinase B; PPAR, peroxisome proliferator-activated receptor).
Author Contributions
All authors listed have made a substantial and direct contribution to the work, and approved it for publication.
Funding
This work was supported by National Natural Science Foundation of China (No. 82102621), Foreign Cooperation Project of Science and Technology, Fujian Province (No. 2020I0015), Joint Funds for the Innovation of Science and Technology, Fujian Province (No. 2019Y9122), Fujian Provincial Health Technology Project (No. 2019-ZQN-57), Startup Fund for Scientific Research, Fujian Medical University (No. 2018QH1053), and 2019–2020 Middle-aged Young Experts with Outstanding Contributions to Health in Fujian Province.
Conflict of Interest
The authors declare that the research was conducted in the absence of any commercial or financial relationships that could be construed as a potential conflict of interest.
Publisher’s Note
All claims expressed in this article are solely those of the authors and do not necessarily represent those of their affiliated organizations, or those of the publisher, the editors and the reviewers. Any product that may be evaluated in this article, or claim that may be made by its manufacturer, is not guaranteed or endorsed by the publisher.
References
Bejjani, F., Evanno, E., Zibara, K., Piechaczyk, M., and Jariel-Encontre, I. (2019). The AP-1 Transcriptional Complex: Local Switch or Remote Command? Biochim. Biophys. Acta (Bba) - Rev. Cancer 1872, 11–23. doi:10.1016/j.bbcan.2019.04.003
Ben-Eliezer, M., Phillip, M., and Gat-Yablonski, G. (2007). Leptin Regulates Chondrogenic Differentiation in ATDC5 Cell-Line through JAK/STAT and MAPK Pathways. Endocr 32, 235–244. doi:10.1007/s12020-007-9025-y
Berendoncks, A. M. V., Stensvold, D., Garnier, A., Fortin, D., Sente, T., Vrints, C. J., et al. (2013). Disturbed Adiponectin - AMPK System in Skeletal Muscle of Patients with Metabolic Syndrome. Eur. J. Prev. Cardiol. 22, 203–205. doi:10.1177/2047487313508034
Berg, V., Sveinbjörnsson, B., Bendiksen, S., Brox, J., Meknas, K., and Figenschau, Y. (2010). Human Articular Chondrocytes Express ChemR23 and Chemerin; ChemR23 Promotes Inflammatory Signalling upon Binding the Ligand Chemerin21-157. Arthritis Res. Ther. 12, R228. doi:10.1186/ar3215
Boileau, C., Martel-Pelletier, J., Fahmi, H., Mineau, F., Boily, M., and Pelletier, J. P. (2007). The Peroxisome Proliferator-Activated Receptor γ Agonist Pioglitazone Reduces the Development of Cartilage Lesions in an Experimental Dog Model of Osteoarthritis: In Vivo Protective Effects Mediated through the Inhibition of Key Signaling and Catabolic Pa. Arthritis Rheum. 56, 2288–2298. doi:10.1002/art.22726
Cai, R., Wang, Y., Huang, Z., Zou, Q., and Pu, Y. (2021). Role of RhoA/ROCK Signaling in Alzheimer ’ S Disease. Behav. Brain Res. 414, 113481. doi:10.1016/j.bbr.2021.113481
Cerquone Perpetuini, A., Giuliani, G., Reggio, A., Cerretani, M., Santoriello, M., Stefanelli, R., et al. (2020). Janus Effect of Glucocorticoids on Differentiation of Muscle Fibro/adipogenic Progenitors. Sci. Rep. 10, 1–18. doi:10.1038/s41598-020-62194-6
Chai, B., Zheng, Z. H., Liao, X., Li, K. Y., Liang, J. S., Huang, Y. X., et al. (2020). The Protective Role of Omentin-1 in IL-1β-induced Chondrocyte Senescence. Artif. Cells Nanomedicine Biotechnol. 48, 8–14. doi:10.1080/21691401.2019.1699803
Chang, T. K., Wang, Y. H., Kuo, S. J., Wang, S. W., Tsai, C. H., Fong, Y. C., et al. (2020). Apelin Enhances IL-1β Expression in Human Synovial Fibroblasts by Inhibiting miR-144-3p through the PI3K and ERK Pathways. Aging (Albany. NY) 12, 9224–9239. doi:10.18632/aging.103195
Chen, C., Zhou, M., Ge, Y., and Wang, X. (2020). SIRT1 and Aging Related Signaling Pathways. Mech. Ageing Dev. 187, 111215. doi:10.1016/j.mad.2020.111215
Chen, H. Te., Tsou, H. K., Chen, J. C., Shih, J. M. K., Chen, Y. J., and Tang, C. H. (2014). Adiponectin Enhances Intercellular Adhesion Molecule-1 Expression and Promotes Monocyte Adhesion in Human Synovial Fibroblasts. PLoS One 9, 1–10. doi:10.1371/journal.pone.0092741
Chen, T., Wu, Y. W., Lu, H., Guo, Y., and Tang, Z. H. (2015). Adiponectin Enhances Osteogenic Differentiation in Human Adipose-Derived Stem Cells by Activating the APPL1-AMPK Signaling Pathway. Biochem. Biophys. Res. Commun. 461, 237–242. doi:10.1016/j.bbrc.2015.03.168
Chen, Y., and Zhou, X. (2020). Research Progress of mTOR Inhibitors. Eur. J. Med. Chem. 208, 112820. doi:10.1016/j.ejmech.2020.112820
Chen, Z., Gibson, T. B., Robinson, F., Silvestro, L., Pearson, G., Xu, B., et al. (2001). MAP Kinases. Chem. Rev. 101, 2449–2476. doi:10.1021/cr000241p
Choi, M-C., Jo, J., Park, J., Kang, H. K., and Park, Y. (2019). NF-B Signaling Pathways in Osteoarthritic Cartilage Destruction. Cells 8, 734. doi:10.3390/cells8070734
Clockaerts, S., Bastiaansen-Jenniskens, Y. M., Feijt, C., Verhaar, J. A. N., Somville, J., De Clerck, L. S., et al. (2011). Peroxisome Proliferator Activated Receptor Alpha Activation Decreases Inflammatory and Destructive Responses in Osteoarthritic Cartilage. Osteoarthr. Cartil. 19, 895–902. doi:10.1016/j.joca.2011.03.010
Collins, K. H., Lenz, K. L., Pollitt, E. N., Ferguson, D., Hutson, I., Springer, L. E., et al. (2020). Adipose Tissue Is a Critical Regulator of Osteoarthritis. Proc. Natl. Acad. Sci. U. S. A. 118, 1–12. doi:10.1073/pnas.2021096118
Conde, J., Lazzaro, V., Scotece, M., Abella, V., Villar, R., López, V., et al. (2017). Corticoids Synergize with IL-1 in the Induction of LCN2. Osteoarthr. Cartil. 25, 1172–1178. doi:10.1016/j.joca.2017.01.017
Conde, J., Otero, M., Scotece, M., Abella, V., López, V., Pino, J., et al. (2016). E74-like Factor 3 and Nuclear Factor-Κb Regulate Lipocalin-2 Expression in Chondrocytes. J. Physiol. 594, 6133–6146. doi:10.1113/JP272240
Conde, J., Scotece, M., Abella, V., Gómez, R., López, V., Villar, R., et al. (2015). Identification of Novel Adipokines in the Joint. Differential Expression in Healthy and Osteoarthritis Tissues. PLoS One 10, e0123601. doi:10.1371/journal.pone.0123601
Conde, J., Scotece, M., López, V., Gómez, R., Lago, F., Pino, J., et al. (2012). Adiponectin and Leptin Induce VCAM-1 Expression in Human and Murine Chondrocytes. PLoS One 7, 1–7. doi:10.1371/journal.pone.0052533
Cordani, N., Pisa, V., Pozzi, L., Sciorati, C., and Clementi, E. (2013). Nitric Oxide Controls Fat Deposition in Dystrophic Skeletal Muscle by Regulating Fibro-Adipogenic Precursor Differentiation. Tissue-Specific Stem Cells 32, 874–875. doi:10.1002/stem.1587
Cuschieri, J., and Maier, R. V. (2005). Mitogen-activated Protein Kinase (MAPK). Crit. Care Med. 33, 417–419. doi:10.1097/01.CCM.0000191714.39495.A6
De Santis, M. C., Gulluni, F., Campa, C. C., Martini, M., and Hirsch, E. (2019). Targeting PI3K Signaling in Cancer: Challenges and Advances. Biochim. Biophys. Acta - Rev. Cancer 1871, 361–366. doi:10.1016/j.bbcan.2019.03.003
Deng, Z., Jia, Y., Liu, H., He, M., Yang, Y., Xiao, W., et al. (2019). RhoA/ROCK Pathway: Implication in Osteoarthritis and Therapeutic Targets. Am. J. Transl Res. 11, 5324–5331.
Dubois, V., Eeckhoute, J., Lefebvre, P., and Staels, B. (2017). Distinct but Complementary Contributions of PPAR Isotypes to Energy Homeostasis. J. Clin. Invest. 127, 1202–1214. doi:10.1172/JCI88894
Eldjoudi, D. A., Barreal, A. C., Ruiz-fern, C., Farrag, Y., Farrag, M., Lago, F., et al. (2022). Leptin in Osteoarthritis and Rheumatoid Arthritis : Player or Bystander? Int. J. Mol. Sci. 23. doi:10.3390/ijms23052859
Fahmi, H., Martel-Pelletier, J., Pelletier, J. P., and Kapoor, M. (2011). Peroxisome Proliferator-Activated Receptor Gamma in Osteoarthritis. Mod. Rheumatol. 21, 1–9. doi:10.1007/s10165-010-0347-x
Favero, M., Belluzzi, E., Ortolan, A., Lorenzin, M., Oliviero, F., Doria, A., et al. (2022). Erosive Hand Osteoarthritis: Latest Findings and Outlook. Nat. Rev. Rheumatol. 18, 171–183. doi:10.1038/s41584-021-00747-3
Feng, X., Pan, J., Li, J., Zeng, C., Qi, W., Shao, Y., et al. (2020). Metformin Attenuates Cartilage Degeneration in an Experimental Osteoarthritis Model by Regulating AMPK/mTOR. Aging (Albany. NY) 12, 1087–1103. doi:10.18632/aging.102635
Franco-Trepat, E., Guillán-Fresco, M., Alonso-Pérez, A., Jorge-Mora, A., Francisco, V., Gualillo, O., et al. (2019). Visfatin Connection: Present and Future in Osteoarthritis and Osteoporosis. J. Clin. Med. 8, 12–15. doi:10.3390/jcm8081178
Fu, L., Patel, M. S., Bradley, A., Wagner, E. F., and Karsenty, G. (2005). The Molecular Clock Mediates Leptin-Regulated Bone Formation. Cell 122, 803–815. doi:10.1016/j.cell.2005.06.028
Fujita, N., Matsushita, T., Ishida, K., Kubo, S., Matsumoto, T., Takayama, K., et al. (2011). Potential Involvement of SIRT1 in the Pathogenesis of Osteoarthritis through the Modulation of Chondrocyte Gene Expressions. J. Orthop. Res. 29, 511–515. doi:10.1002/jor.21284
Gabay, O., Sanchez, C., Dvir-Ginzberg, M., Gagarina, V., Zaal, K. J., Song, Y., et al. (2013). Sirtuin 1 Enzymatic Activity Is Required for Cartilage Homeostasis In Vivo in a Mouse Model. Arthritis Rheum. 65, 159–166. doi:10.1002/art.37750
Gagarina, V., Gabay, O., Dvir-Ginzberg, M., Lee, E-J., Brady, J. K., Quon, M. J., et al. (2010). SirT1 Enhances Survival of Human Osteoarthritic Chondrocytes by Repressing PTP1B and Activating the IGF Receptor Pathway. Arthritis Rheum. 62, 1383–1392. doi:10.1002/art.27369
Gao, Y. H., Zhao, C. W., Liu, B., Dong, N., Ding, L., Li, Y. R., et al. (2020). An Update on the Association between Metabolic Syndrome and Osteoarthritis and on the Potential Role of Leptin in Osteoarthritis. Cytokine 129, 155043. doi:10.1016/j.cyto.2020.155043
Garcia, D., and Shaw, R. J. (2018). AMPK: Mechanisms of Cellular Energy Sensing and Restoration of Metabolic Balance. Mol. Cell 66, 789–800. doi:10.1016/j.molcel.2017.05.032
Ge, H. X., Zou, F. M., Li, Y., Liu, A. M., and Tu, M. (2017). JNK Pathway in Osteoarthritis: Pathological and Therapeutic Aspects. J. Recept. Signal. Transduct. 37, 431–436. doi:10.1080/10799893.2017.1360353
Giardullo, L., Corrado, A., Maruotti, N., Cici, D., Mansueto, N., and Cantatore, F. P. (2021). Adipokine Role in Physiopathology of Inflammatory and Degenerative Musculoskeletal Diseases. Int. J. Immunopathol. Pharmacol. 35. doi:10.1177/20587384211015034
Glyn-Jones, S., Palmer, A. J. R., Agricola, R., Price, A. J., Vincent, T. L., Weinans, H., et al. (2015). Osteoarthritis. Lancet 386, 376–387. doi:10.1016/S0140-6736(14)60802-3
Goldring, M. B., and Berenbaum, F. (2015). Emerging Targets in Osteoarthritis Therapy Mary. Physiol. Behav. 22, 51–63. doi:10.1016/j.coph.2015.03.004
Guo, H., Jin, D., and Chen, X. (2014). Lipocalin 2 Is a Regulator of Macrophage Polarization and NF-Κb/stat3 Pathway Activation. Mol. Endocrinol. 28, 1616–1628. doi:10.1210/me.2014-1092
Harasymowicz, N. S., Azfer, A., Burnett, R., Simpson, H., and Salter, D. M. (2021). Chondrocytes from Osteoarthritic Cartilage of Obese Patients Show Altered Adiponectin Receptors Expression and Response to Adiponectin. J. Orthop. Res. 39, 2333–2339. doi:10.1002/jor.24993
Hardie, D. G. (2014). AMPK – Sensing Energy while Talking to Other Signaling Pathways. Cell Metabolism. 20, 939–952. doi:10.1016/j.cmet.2014.09.013
Hayden, M. S., and Ghosh, S. (2012). NF-κB, the First quarter-century: Remarkable Progress and Outstanding Questions. Genes Dev. 26, 203–234. doi:10.1101/gad.183434.111
Hochberg, M. C., Yerges-Armstrong, L., Yau, M., and Mitchell, B. D. (2013). Genetic Epidemiology of Osteoarthritis: Recent Developments and Future Directions. Curr. Opin. Rheumatol. 25, 192–197. doi:10.1097/BOR.0b013e32835cfb8e
Hosaka, Y., Saito, T., Sugita, S., Hikata, T., Kobayashi, H., Fukai, A., et al. (2013). Notch Signaling in Chondrocytes Modulates Endochondral Ossification and Osteoarthritis Development. Proc. Natl. Acad. Sci. U. S. A. 110, 1875–1880. doi:10.1073/pnas.1207458110
Hou, Y., Moreau, F., and Chadee, K. (2012). PPARγ Is an E3 Ligase that Induces the Degradation of NFκB/p65. Nat. Commun. 3, 1300–1311. doi:10.1038/ncomms2270
Hu, P.-F., Chen, W.-P., Tang, J.-L., Bao, J.-P., and Wu, L.-D. (2010). Apelin Plays a Catabolic Role on Articular Cartilage: In Vivo and In Vitro Studies. Int. J. Mol. Med. 26, 357–363. doi:10.3892/ijmm_00000473
Huang, C. Y., Lee, C. Y., Chen, M. Y., Tsai, H. C., Hsu, H. C., and Tang, C. H. (2010). Adiponectin Increases BMP-2 Expression in Osteoblasts via AdipoR Receptor Signaling Pathway. J. Cel. Physiol. 224, 475–483. doi:10.1002/jcp.22145
Huang, G., Jiang, W., Weiyong, X. I. E., Wei, L. U., Weimin, Z. H. U., and Deng, Z. (2021). Role of Peroxisome Proliferator-Activated Receptors in Osteoarthritis (Review). Mol. Med. Rep. 23, 4–6. doi:10.3892/mmr.2020.11798
Huang, J., Chen, C., Liang, C., Luo, P., Xia, G., Zhang, L., et al. (2020). Dysregulation of the Wnt Signaling Pathway and Synovial Stem Cell Dysfunction in Osteoarthritis Development. Stem Cell Dev 29, 401–413. doi:10.1089/scd.2019.0260
Huang, K., Du, G., Li, L., Liang, H., and Zhang, B. (2012). Association of Chemerin Levels in Synovial Fluid with the Severity of Knee Osteoarthritis. Biomarkers 17, 16–20. doi:10.3109/1354750x.2011.634028
Huang, Z. M., Du, S. H., Huang, L. G., Li, J. H., Xiao, L., and Tong, P. (2016). Leptin Promotes Apoptosis and Inhibits Autophagy of Chondrocytes through Upregulating Lysyl Oxidase-like 3 during Osteoarthritis Pathogenesis. Osteoarthr. Cartil. 24, 1246–1253. doi:10.1016/j.joca.2016.02.009
Hunter, D. J., March, L., and Chew, M. (2020). Osteoarthritis in 2020 and beyond: a Lancet Commission. Lancet 396, 1711–1712. doi:10.1016/S0140-6736(20)32230-3
Hwang, H. S., and Kim, H. A. (2015). Chondrocyte Apoptosis in the Pathogenesis of Osteoarthritis. Int. J. Mol. Sci. 16, 26035–26054. doi:10.3390/ijms161125943
Ilia, I., Nitusca, D., and Marian, C. (2022). Adiponectin in Osteoarthritis: Pathophysiology, Relationship with Obesity and Presumptive Diagnostic Biomarker Potential. Diagnostics 12, 1–10. doi:10.3390/diagnostics12020455
Jiang, L., Zhu, X., Rong, J., Xing, B., Wang, S., Liu, A., et al. (2021). Obesity, Osteoarthritis and Genetic Risk the Rs182052 Polymorphism in the Adipoq Gene Is Potentially Associated with Risk of Knee Osteoarthritis. Bone Jt. Res. 7, 494–500. doi:10.1302/2046-3758.77.BJR-2017-0274.R1
Kanazawa, I., Yamaguchi, T., Yano, S., Yamauchi, M., Yamamoto, M., and Sugimoto, T. (2007). Adiponectin and AMP Kinase Activator Stimulate Proliferation, Differentiation, and Mineralization of Osteoblastic MC3T3-E1 Cells. BMC Cell Biol 8, 51. doi:10.1186/1471-2121-8-51
Kang, E. H., Lee, Y. J., Kim, T. K., Chang, C. B., Chung, J.-H., Shin, K., et al. (2010). Adiponectin Is a Potential Catabolic Mediator in Osteoarthritis Cartilage. Arthritis Res. Ther. 12, R231. doi:10.1186/ar3218
Kiewisz, J., Kaczmarek, M. M., Pawlowska, A., Kmiec, Z., and Stompor, T. (2016). Endothelial Progenitor Cells Participation in Cardiovascular and Kidney Diseases: A Systematic Review. Acta Biochim. Pol. 63, 475–482. doi:10.18388/abp.2016_1284
Korbecki, J., Bobiński, R., and Dutka, M. (2019). Self-regulation of the Inflammatory Response by Peroxisome Proliferator-Activated Receptors. Inflamm. Res. 68, 443–458. doi:10.1007/s00011-019-01231-1
Koskinen, A., Vuolteenaho, K., Nieminen, R., Moilanen, T., and Moilanen, E. (2011). Leptin Enhances MMP-1, MMP-3 and MMP-13 Production in Human Osteoarthritic Cartilage and Correlates with MMP-1 and MMP-3 in Synovial Fluid from OA Patients. Clin. Exp. Rheumatol. 29, 57–64. Available at: http://www.ncbi.nlm.nih.gov/pubmed/21345293 (Accessed January 13, 2017).
Kumavat, R., Kumar, V., Malhotra, R., Pandit, H., Jones, E., Ponchel, F., et al. (2021). Biomarkers of Joint Damage in Osteoarthritis: Current Status and Future Directions. Mediators Inflamm. 2021, 5574582. doi:10.1155/2021/5574582
Laiguillon, M.-C., Houard, X., Bougault, C., Gosset, M., Nourissat, G., Sautet, A., et al. (2014). Expression and Function of Visfatin (Nampt), an Adipokine-Enzyme Involved in Inflammatory Pathways of Osteoarthritis. Arthritis Res. Ther. 16, R38. doi:10.1186/ar4467
Lan, C. N., Cai, W. J., Shi, J., and Yi, Z. J. (2021). MAPK Inhibitors Protect against Early-Stage Osteoarthritis by Activating Autophagy. Mol. Med. Rep. 24, 1–9. doi:10.3892/mmr.2021.12469
Lee, K. T., Chen, B. C., Liu, S. C., Lin, Y. Y., Tsai, C. H., Ko, C. Y., et al. (2021). Nesfatin-1 Facilitates IL-1β Production in Osteoarthritis Synovial Fibroblasts by Suppressing miR-204-5p Synthesis through the AP-1 and NF-kB Pathways. Aging (Albany. NY) 13, 22490–22501. doi:10.18632/aging.203559
Lee, S. W., Rho, J. H., Lee, S. Y., Kim, J. H., Cheong, J. H., Kim, H. Y., et al. (2015). Leptin Protects Rat Articular Chondrocytes from Cytotoxicity Induced by TNF-α in the Presence of Cyclohexamide. Osteoarthr. Cartil. 23, 2269–2278. doi:10.1016/j.joca.2015.06.005
Li, Z., Wang, X., Pan, H., Yang, H., Li, X., Zhang, K., et al. (2017). Resistin Promotes CCL4 Expression through Toll-like Receptor-4 and Activation of the P38-MAPK and NF-Κb Signaling Pathways: Implications for Intervertebral Disc Degeneration. Osteoarthr. Cartil. 25, 341–350. doi:10.1016/j.joca.2016.10.002
Liang, J., Feng, J., Wu, W. K. K., Xiao, J., Wu, Z., Han, D., et al. (2011). Leptin-mediated Cytoskeletal Remodeling in Chondrocytes Occurs via the RhoA/ROCK Pathway. J. Orthop. Res. 29, 369–374. doi:10.1002/jor.21257
Lin, Z., Miao, J., Zhang, T., He, M., Wang, Z., Feng, X., et al. (2021). JUNB-FBXO21-ERK axis Promotes Cartilage Degeneration in Osteoarthritis by Inhibiting Autophagy. Aging Cell 20, 1–15. doi:10.1111/acel.13306
Liu, Y., Xu, F., Pei, H. X., Zhu, X., Lin, X., Song, C. Y., et al. (2016). Vaspin Regulates the Osteogenic Differentiation of MC3T3-E1 through the PI3K-Akt/miR-34c Loop. Sci. Rep. 6, 1–15. doi:10.1038/srep25578
Long, H., Zeng, X., Liu, Q., Wang, H., Vos, T., Hou, Y., et al. (2020). Burden of Osteoarthritis in China, 1990–2017: Findings from the Global Burden of Disease Study 2017. Lancet Rheumatol. 2, e164–e172. doi:10.1016/S2665-9913(19)30145-6
Lovren, F., Pan, Y., Quan, A., Szmitko, P. E., Singh, K. K., Shukla, P. C., et al. (2010). Adiponectin Primes Human Monocytes into Alternative Anti-inflammatory M2 Macrophages. Am. J. Physiol. - Hear. Circ. Physiol. 299, 656–663. doi:10.1152/ajpheart.00115.2010
Luo, X. H., Guo, L. J., Yuan, L. Q., Xie, H., Zhou, H. D., Wu, X. P., et al. (2005). Adiponectin Stimulates Human Osteoblasts Proliferation and Differentiation via the MAPK Signaling Pathway. Exp. Cell Res. 309, 99–109. doi:10.1016/j.yexcr.2005.05.021
Ma, J., Niu, D. S., Wan, N. J., Qin, Y., and Guo, C. J. (2015). Elevated Chemerin Levels in Synovial Fluid and Synovial Membrane from Patients with Knee Osteoarthritis. Int. J. Clin. Exp. Pathol. 8, 13393–13398.
MacDonald, I. J., Liu, S. C., Su, C. M., Wang, Y. H., Tsai, C. H., and Tang, C. H. (2018). Implications of Angiogenesis Involvement in Arthritis. Int. J. Mol. Sci. 19. doi:10.3390/ijms19072012
Manning, B. D., and Toker, A. (2017). AKT/PKB Signaling: Navigating the Network Brendan. Cell 169, 381–405. doi:10.1016/j.cell.2017.04.001.AKT/PKB
Matsushita, T., Sasaki, H., Takayama, K., Ishida, K., Matsumoto, T., Kubo, S., et al. (2013). The Overexpression of SIRT1 Inhibited Osteoarthritic Gene Expression Changes Induced by Interleukin-1β in Human Chondrocytes. J. Orthop. Res. 31, 531–537. doi:10.1002/jor.22268
Matsuzaki, T., Matsushita, T., Takayama, K., Matsumoto, T., Nishida, K., Kuroda, R., et al. (2014). Disruption of Sirt1 in Chondrocytes Causes Accelerated Progression of Osteoarthritis under Mechanical Stress and during Ageing in Mice. Ann. Rheum. Dis. 73, 1397–1404. doi:10.1136/annrheumdis-2012-202620
Michan, S., and Sinclair, D. (2007). Sirtuins in Mammals: Insights into Their Biological Function. Biochem. J. 404, 1–13. doi:10.1042/BJ20070140
Mobasheri, A., Rayman, M. P., Gualillo, O., Sellam, J., Van Der Kraan, P., and Fearon, U. (2017). The Role of Metabolism in the Pathogenesis of Osteoarthritis. Nat. Rev. Rheumatol. 13, 302–311. doi:10.1038/nrrheum.2017.50
Moilanen, E., Vuolteenaho, K., Koskinen, A., Kukkonen, M., Nieminen, R., Pivrinta, U., et al. (2009). Leptin Enhances Synthesis of Proinflammatory Mediators in Human Osteoarthritic Cartilage-Mediator Role of NO in Leptin-Induced PGE 2, IL-6, and IL-8 Production. Mediators Inflamm. 2009, 345838. doi:10.1155/2009/345838
Monemdjou, R., Vasheghani, F., Fahmi, H., Perez, G., Blati, M., Taniguchi, N., et al. (2012). Association of Cartilage-specific Deletion of Peroxisome Proliferator-Activated Receptor γ with Abnormal Endochondral Ossification and Impaired Cartilage Growth and Development in a Murine Model. Arthritis Rheum. 64, 1551–1561. doi:10.1002/art.33490.Association
Moon, M. H., Jeong, J. K., Lee, Y. J., Seol, J. W., Jackson, C. J., and Park, S. Y. (2013). SIRT1, a Class III Histone Deacetylase, Regulates TNF-α-Induced Inflammation in Human Chondrocytes. Osteoarthr. Cartil. 21, 470–480. doi:10.1016/j.joca.2012.11.017
Mutabaruka, M.-S., Aissa, M. A., Delalandre, A., Lavigne, M., and Lajeunesse, D. (2010). Research Article Local Leptin Production in Osteoarthritis Subchondral Osteoblasts May Be Responsible for Their Abnormal Phenotypic Expression. Arthritis Res. Ther. 12 (1), R20. doi:10.1186/ar2925
Myers, M. G., Sun, X. J., and White, M. F. (1994). The IRS-1 Signaling System. Trends Biochem. Sci. 19, 289–293. doi:10.1016/0968-0004(94)90007-8
Neumann, E., Junker, S., Schett, G., Frommer, K., and Müller-Ladner, U. (2016). Adipokines in Bone Disease. Nat. Rev. Rheumatol. 12, 296–302. doi:10.1038/nrrheum.2016.49
Otero, M., Lago, R., Gómez, R., Lago, F., Gomez-Reino, J. J., and Gualillo, O. (2007). Phosphatidylinositol 3-kinase, MEK-1 and P38 Mediate Leptin/interferon-Gamma Synergistic NOS Type II Induction in Chondrocytes. Life Sci. 81, 1452–1460. doi:10.1016/j.lfs.2007.09.007
Otero, M., Lago, R., Lago, F., Reino, J. J. G., and Gualillo, O. (2005). Signalling Pathway Involved in Nitric Oxide Synthase Type II Activation in Chondrocytes: Synergistic Effect of Leptin with Interleukin-1. Arthritis Res. Ther. 7, 581–591. doi:10.1186/ar1708
Otero, M., Reino, J. J. G., and Gualillo, O. (2003). Synergistic Induction of Nitric Oxide Synthase Type II: In Vitro Effect of Leptin and Interferon‐γ in Human Chondrocytes and ATDC5 Chondrogenic Cells. Arthritis Rheum. 48, 404–409. doi:10.1002/art.10811
Pallu, S., Francin, P.-J., Guillaume, C., Gegout-Pottie, P., Netter, P., Mainard, D., et al. (2010). Obesity Affects the Chondrocyte Responsiveness to Leptin in Patients with Osteoarthritis. Arthritis Res. Ther. 12 (3), R112. doi:10.1186/ar3048
Patel, J., Donovan, P., and Khosrotehrani, K. (2016). Concise Review : Functional Definition of Endothelial Progenitor Progenitor Cells: A Molecular Perspective. Stem Cell Transl. Med. 5, 1302–1306. doi:10.5966/sctm.2016-0066
Phalitakul, S., Okada, M., Hara, Y., and Yamawaki, H. (2011). Vaspin Prevents TNF-α-Induced Intracellular Adhesion Molecule-1 via Inhibiting Reactive Oxygen Species-dependent NF-Κb and PKCθ Activation in Cultured Rat Vascular Smooth Muscle Cells. Pharmacol. Res. 64, 493–500. doi:10.1016/j.phrs.2011.06.001
Plotz, B., Bomfim, F., Sohail, M. A., and Samuels, J. (2021). Current Epidemiology and Risk Factors for the Development of Hand Osteoarthritis. Curr. Rheumatol. Rep. 23. doi:10.1007/s11926-021-01025-7
Ratneswaran, A., LeBlanc, E. A., Walser, E., Welch, I., Mort, J. S., Borradaile, N., et al. (2015). Peroxisome Proliferator-Activated Receptor δ Promotes the Progression of Posttraumatic Osteoarthritis in a Mouse Model. Arthritis Rheumatol. 67, 454–464. doi:10.1002/art.38915
Rawlings, J. S., Rosler, K. M., and Harrison, D. A. (2004). The JAK/STAT Signaling Pathway. J. Cell Sci. 117, 1281–1283. doi:10.1242/jcs.00963
Reggio, A., Rosina, M., Palma, A., Cerquone Perpetuini, A., Petrilli, L. L., Gargioli, C., et al. (2020). Adipogenesis of Skeletal Muscle Fibro/adipogenic Progenitors Is Affected by the WNT5a/GSK3/β-Catenin axis. Cell Death Differ 27, 2921–2941. doi:10.1038/s41418-020-0551-y
Reggio, A., Spada, F., Rosina, M., Massacci, G., Zuccotti, A., Fuoco, C., et al. (2019). The Immunosuppressant Drug Azathioprine Restrains Adipogenesis of Muscle Fibro/Adipogenic Progenitors from Dystrophic Mice by Affecting AKT Signaling. Sci. Rep. 9. doi:10.1038/s41598-019-39538-y
Rigoglou, S., and Papavassiliou, A. G. (2013). The NF-Κb Signalling Pathway in Osteoarthritis. Int. J. Biochem. Cell Biol. 45, 2580–2584. doi:10.1016/j.biocel.2013.08.018
Roman-Blas, J. A., and Jimenez, S. A. (2006). NF-κB as a Potential Therapeutic Target in Osteoarthritis and Rheumatoid Arthritis. Osteoarthr. Cartil. 14, 839–848. doi:10.1016/j.joca.2006.04.008
Rosa, S. C., Rufino, A. T., Judas, F., Tenreiro, C., Lopes, M. C., and Mendes, A. F. (2011). Expression and Function of the Insulin Receptor in normal and Osteoarthritic Human Chondrocytes: Modulation of Anabolic Gene Expression, Glucose Transport and GLUT-1 Content by Insulin. Osteoarthr. Cartil. 19, 719–727. doi:10.1016/j.joca.2011.02.004
Saito, T., and Tanaka, S. (2017). Molecular Mechanisms Underlying Osteoarthritis Development: Notch and NF-Κb. Arthritis Res. Ther. 19, 1–7. doi:10.1186/s13075-017-1296-y
Saitta, B., Elphingstone, J., Limfat, S., Shkhyan, R., and Evseenko, D. (2019). CaMKII Inhibition in Human Primary and Pluripotent Stem Cell- Derived Chondrocytes Modulates Effects of TGFβ and BMP through SMAD Signaling Biagio. Osteoarthr. Cartil. 27, 158–171. doi:10.1016/j.joca.2018.08.017.CaMKII
Saklatvala, J. (2007). Inflammatory Signaling in Cartilage: MAPK and NF-Κb Pathways in Chondrocytes and the Use of Inhibitors for Research into Pathogenesis and Therapy of Osteoarthritis. Curr. Drug Targets 8, 305–313. doi:10.2174/138945007779940115
Scirpo, R., Fiorotto, R., Villani, A., Amenduni, M., Spirli, C., and Strazzabosco, M. (2015). Stimulation of Nuclear Receptor Peroxisome Proliferator-Activated Receptor-γ Limits NF-κb-dependent Inflammation in Mouse Cystic Fibrosis Biliary Epithelium. Hepatology 62, 1551–1562. doi:10.1002/hep.28000
Sharma, L. (2021). Osteoarthritis of the Knee. N. Engl. J. Med. 384, 51–59. doi:10.1056/NEJMcp1903768
Shi, J., Zhang, C., Yi, Z., and Lan, C. (2016). Explore the Variation of MMP3, JNK, P38 MAPKs, and Autophagy at the Early Stage of Osteoarthritis. IUBMB Life 68, 293–302. doi:10.1002/iub.1482
Song, Y. Z., Guan, J., Wang, H. J., Ma, W., Li, F., Xu, F., et al. (2016). Possible Involvement of Serum and Synovial Fluid Resistin in Knee Osteoarthritis: Cartilage Damage, Clinical, and Radiological Links. J. Clin. Lab. Anal. 30, 437–443. doi:10.1002/jcla.21876
Su, Y. P., Chen, C. N., Chang, H. I., Huang, K. C., Cheng, C. C., Chiu, F. Y., et al. (2017). Low Shear Stress Attenuates COX-2 Expression Induced by Resistin in Human Osteoarthritic Chondrocytes. J. Cel. Physiol. 232, 1448–1457. doi:10.1002/jcp.25644
Sugita, S., Hosaka, Y., Okada, K., Mori, D., Yano, F., Kobayashi, H., et al. (2015). Transcription Factor Hes1 Modulates Osteoarthritis Development in Cooperation with Calcium/calmodulin-dependent Protein Kinase 2. Proc. Natl. Acad. Sci. U. S. A. 112, 3080–3085. doi:10.1073/pnas.1419699112
Sun, A. R. J., Udduttula, A., Li, J., Liu, Y., Ren, P. G., and Zhang, P. (2021). Cartilage Tissue Engineering for Obesity-Induced Osteoarthritis: Physiology, Challenges, and Future Prospects. J. Orthop. Transl. 26, 3–15. doi:10.1016/j.jot.2020.07.004
Sun, H. Y., Hu, K. Z., and Yin, Z. S. (2017). Inhibition of the P38-MAPK Signaling Pathway Suppresses the Apoptosis and Expression of Proinflammatory Cytokines in Human Osteoarthritis Chondrocytes. Cytokine 90, 135–143. doi:10.1016/j.cyto.2016.11.002
Sun, K., Luo, J., Guo, J., Yao, X., Jing, X., and Guo, F. (2020). The PI3K/AKT/mTOR Signaling Pathway in Osteoarthritis: a Narrative Review. Osteoarthr. Cartil. 28, 400–409. doi:10.1016/j.joca.2020.02.027
Takayama, K., Ishida, K., Matsushita, T., Fujita, N., Hayashi, S., Sasaki, K., et al. (2009). SIRT1 Regulation of Apoptosis of Human Chondrocytes. Arthritis Rheum. 60, 2731–2740. doi:10.1002/art.24864
Tang, C.-H., Chiu, Y.-C., Tan, T.-W., Yang, R.-S., and Fu, W.-M. (2007). Adiponectin Enhances IL-6 Production in Human Synovial Fibroblast via an AdipoR1 Receptor, AMPK, P38, and NF-Κb Pathway. J. Immunol. 179, 5483–5492. doi:10.4049/jimmunol.179.8.5483
Tang, F., Wang, Y., Hemmings, B. A., Rüegg, C., and Xue, G. (2018). PKB/Akt-dependent Regulation of Inflammation in Cancer. Semin. Cancer Biol. 48, 62–69. doi:10.1016/j.semcancer.2017.04.018
Tarabeih, N., Kalinkovich, A., Shalata, A., and Livshits, G. (2020). Circulating Levels of Visceral Adipose Tissue-Derived Serine Protease Inhibitor (Vaspin) Appear as a Marker of Musculoskeletal Pain Disability. Diagnostics 10. doi:10.3390/diagnostics10100797
Thommesen, L., Stunes, A. K., Monjo, M., Grøsvik, K., Tamburstuen, M. V., Kjøbli, E., et al. (2006). Expression and Regulation of Resistin in Osteoblasts and Osteoclasts Indicate a Role in Bone Metabolism. J. Cel. Biochem. 99, 824–834. doi:10.1002/jcb.20915
Tong, K. M., Chen, C. P., Huang, K. C., Shieh, D. C., Cheng, H. C., Tzeng, C. Y., et al. (2011). Adiponectin Increases MMP-3 Expression in Human Chondrocytes through Adipor1 Signaling Pathway. J. Cel. Biochem. 112, 1431–1440. doi:10.1002/jcb.23059
Tong, K. M., Shieh, D. C., Chen, C. P., Tzeng, C. Y., Wang, S. P., Huang, K. C., et al. (2008). Leptin Induces IL-8 Expression via Leptin Receptor, IRS-1, PI3K, Akt cascade and Promotion of NF-κB/p300 Binding in Human Synovial Fibroblasts. Cell. Signal. 20, 1478–1488. doi:10.1016/j.cellsig.2008.04.003
Tsai, C. H., Liu, S. C., Chung, W. H., Wang, S. W., Wu, M. H., and Tang, C. H. (2020). Visfatin Increases VEGF-dependent Angiogenesis of Endothelial Progenitor Cells during Osteoarthritis Progression. Cells 9, 1–15. doi:10.3390/cells9051315
Tsuchida, A. I., Beekhuizen, M., ’t Hart, M. C., Radstake, T. R. D. J., Dhert, W. J. A., Saris, D. B. F., et al. (2014). Cytokine Profiles in the Joint Depend on Pathology, but Are Different between Synovial Fluid, Cartilage Tissue and Cultured Chondrocytes. Arthritis Res. Ther. 16, 1–15. doi:10.1186/s13075-014-0441-0
Urban, H., and Little, C. B. (2018). The Role of Fat and Inflammation in the Pathogenesis and Management of Osteoarthritis. Rheumatol. (United Kingdom) 57, iv10–iv21. doi:10.1093/rheumatology/kex399
Ushiyama, T., Chano, T., Inoue, K., and Matsusue, Y. (2003). Cytokine Production in the Infrapatellar Fat Pad: Another Source of Cytokines in Knee Synovial Fluids. Ann. Rheum. Dis. 62, 108–112. doi:10.1136/ard.62.2.108
Vasheghani, F., Monemdjou, R., Fahmi, H., Zhang, Y., Perez, G., Blati, M., et al. (2013). Adult Cartilage-specific Peroxisome Proliferator-Activated Receptor Gamma Knockout Mice Exhibit the Spontaneous Osteoarthritis Phenotype. Am. J. Pathol. 182, 1099–1106. doi:10.1016/j.ajpath.2012.12.012
Vasheghani, F., Zhang, Y., Li, Y. H., Blati, M., Fahmi, H., Lussier, B., et al. (2015). PPARγ Deficiency Results in Severe, Accelerated Osteoarthritis Associated with Aberrant mTOR Signalling in the Articular Cartilage. Ann. Rheum. Dis. 74, 569–578. doi:10.1136/annrheumdis-2014-205743
Wang, J., Chen, Z., and Guan, Z. (2021). Vaspin Deficiency Failed to Promote the Proliferation of BMSCs in Osteoarthritis. Int. J. Rheum. Dis. 24, 90–95. doi:10.1111/1756-185X.14010
Wang, W., Zhang, J., Huo, Y., Zheng, Y., and Gui, Y. (2022). Effects of the Leptin-Mediated MAPK/ERK Signaling Pathway on Collagen II Expression in Knee Cartilage of Newborn Male Mice from Obese Maternal Offspring. Biomolecules 12 (3), 477. doi:10.3390/biom12030477
Wang, Y.-H., Kuo, S.-J., Liu, S.-C., Wang, S.-W., Tsai, C.-H., Fong, Y.-C., et al. (2020). Apelin Affects the Progression of Osteoarthritis by Regulating VEGF-dependent Angiogenesis and miR-150-5p Expression in Human Synovial Fibroblasts. Cells 9, 594. doi:10.3390/cells9030594
Wang, Y., Fan, X., Xing, L., and Tian, F. (2019). Wnt Signaling: A Promising Target for Osteoarthritis Therapy. Cell Commun. Signal. 17, 1–14. doi:10.1186/s12964-019-0411-x
Wang, Y., Kim, J., Chan, A., Whyne, C., and Nam, D. (2018). A Two Phase Regulation of Bone Regeneration: IL-17F Mediates Osteoblastogenesis via C/EBP-β In Vitro. Bone 116, 47–57. doi:10.1016/j.bone.2018.07.007
Wang, Y., Xu, Z., Wang, J., and Xu, S. (2016). DUSP19, a Downstream Effector of Leptin, Inhibits Chondrocyte Apoptosis via Dephosphorylating JNK during Osteoarthritis Pathogenesis. Mol. Biosyst. 12, 721–728. doi:10.1039/c5mb00776c
Wang, Y., Zhang, X., Shao, J., Liu, H., Liu, X., and Luo, E. (2017). Adiponectin Regulates BMSC Osteogenic Differentiation and Osteogenesis through the Wnt/β-Catenin Pathway. Sci. Rep. 7, 1–13. doi:10.1038/s41598-017-03899-z
Wang, Y., Zhao, X., Lotz, M., Terkeltaub, R., and Liu-Bryan, R. (2015). Mitochondrial Biogenesis Is Impaired in Osteoarthritic Chondrocytes but Reversible via Peroxisome Proliferator- Activated Receptor-γ Coactivator 1α. Arthritis Rheumatol. 176, 139–148. doi:10.1002/art.39182.Mitochondrial
Wei, Y., Jin, Z., Zhang, H., Piao, S., Lu, J., and Bai, L. (2018). The Transient Receptor Potential Channel, Vanilloid 5, Induces Chondrocyte Apoptosis via Ca 2+ CaMKII-dependent MAPK and Akt/mTOR Pathways in a Rat Osteoarthritis Model. Cell. Physiol. Biochem. 51, 2309–2323. doi:10.1159/000495874
Wu, S. S., Liang, Q. H., Liu, Y., Cui, R. R., Yuan, L. Q., and Liao, E. Y. (2013). Omentin-1 Stimulates Human Osteoblast Proliferation through PI3K/Akt Signal Pathway. Int. J. Endocrinol. 2013, 2–7. doi:10.1155/2013/368970
Xie, C., and Chen, Q. (2019). Adipokines: New Therapeutic Target for Osteoarthritis? Curr. Rheumatol. Rep. 21, 1–9. doi:10.1007/s11926-019-0868-z
Xie, H., Xie, P. L., Luo, X. H., Wu, X. P., Zhou, H. D., Tang, S. Y., et al. (2012). Omentin-1 Exerts Bone-Sparing Effect in Ovariectomized Mice. Osteoporos. Int. 23, 1425–1436. doi:10.1007/s00198-011-1697-8
Xie, H., Xie, P. L., Wu, X. P., Chen, S. M., Zhou, H. D., Yuan, L. Q., et al. (2011). Omentin-1 Attenuates Arterial Calcification and Bone Loss in Osteoprotegerin-Deficient Mice by Inhibition of RANKL Expression. Cardiovasc. Res. 92, 296–306. doi:10.1093/cvr/cvr200
Xin, P., Xu, X., Deng, C., Liu, S., Wang, Y., Zhou, X., et al. (2020). The Role of JAK/STAT Signaling Pathway and its Inhibitors in Diseases. Int. Immunopharmacol. 80, 106210. doi:10.1016/j.intimp.2020.106210
Xu, M., Feng, M., Peng, H., Qian, Z., Zhao, L., and Wu, S. (2020). Epigenetic Regulation of Chondrocyte Hypertrophy and Apoptosis through Sirt1/P53/P21 Pathway in Surgery-Induced Osteoarthritis. Biochem. Biophys. Res. Commun. 528, 179–185. doi:10.1016/j.bbrc.2020.04.097
Xue, J. F., Shi, Z. M., Zou, J., and Li, X. L. (2017). Inhibition of PI3K/AKT/mTOR Signaling Pathway Promotes Autophagy of Articular Chondrocytes and Attenuates Inflammatory Response in Rats with Osteoarthritis. Biomed. Pharmacother. 89, 1252–1261. doi:10.1016/j.biopha.2017.01.130
Yan, M., Zhang, J., Yang, H., and Sun, Y. (2018). The Role of Leptin in Osteoarthritis. Med. (United States 97. doi:10.1097/MD.0000000000010257
Yang, R., Zhang, D., Yu, K., Sun, L., Yang, J., Zhao, C., et al. (2018). Detection of miR-22, miR-140 and Bone Morphogenetic Proteins (BMP)-2 Expression Levels in Synovial Fluid of Osteoarthritis Patients before and after Arthroscopic Debridement. Med. Sci. Monit. 24, 863–868. doi:10.12659/MSM.908110
Yang, S., Ryu, J. H., Oh, H., Jeon, J., Kwak, J. S., Kim, J. H., et al. (2015). NAMPT (Visfatin), a Direct Target of Hypoxiainducible Factor-2α, Is an Essential Catabolic Regulator of Osteoarthritis. Ann. Rheum. Dis. 74, 595–602. doi:10.1136/annrheumdis-2013-204355
Yang, W. H., Liu, S. C., Tsai, C. H., Fong, Y. C., Wang, S. J., ChangSen, Y., et al. (2013). Leptin Induces IL-6 Expression through OBRl Receptor Signaling Pathway in Human Synovial Fibroblasts. PLoS One 8, 2–11. doi:10.1371/journal.pone.0075551
Yaykasli, K. O., Hatipoglu, O. F., Yaykasli, E., Yildirim, K., Kaya, E., Ozsahin, M., et al. (2015). Leptin Induces ADAMTS-4, ADAMTS-5, and ADAMTS-9 Genes Expression by Mitogen-Activated Protein Kinases and NF-Κb Signaling Pathways in Human Chondrocytes. Cell Biol. Int. 39, 104–112. doi:10.1002/cbin.10336
Zanotti, S., and Canalis, E. (2013). Interleukin 6 Mediates Select Effects of Notch in Chondrocytes Stefano. Osteoarthr. Cartil. 21, 1766–1773. doi:10.1016/j.joca.2013.08.010.Interleukin
Zhang, C., Chiu, K. Y., Chan, B. P. M., Li, T., Wen, C., Xu, A., et al. (2018). Knocking Out or Pharmaceutical Inhibition of Fatty Acid Binding Protein 4 (FABP4) Alleviates Osteoarthritis Induced by High-Fat Diet in Mice. Osteoarthr. Cartil. 26, 824–833. doi:10.1016/j.joca.2018.03.002
Zhang, Y., Yang, Y., Wang, C., Wan, S., Yao, Z., Zhang, Y., et al. (2020). Identification of Diagnostic Biomarkers of Osteoarthritis Based on Multi-Chip Integrated Analysis and Machine Learning. DNA Cell Biol 39, 2245–2256. doi:10.1089/dna.2020.5552
Zhang, Z. M., Shen, C., Li, H., Fan, Q., Ding, J., Jin, F. C., et al. (2016). Leptin Induces the Apoptosis of Chondrocytes in an In Vitro Model of Osteoarthritis via the JAK2-STAT3 Signaling Pathway. Mol. Med. Rep. 13, 3684–3690. doi:10.3892/mmr.2016.4970
Zhao, C. W., Gao, Y. H., Song, W. X., Liu, B., Ding, L., Dong, N., et al. (2019). An Update on the Emerging Role of Resistin on the Pathogenesis of Osteoarthritis. Mediators Inflamm. 2019, 1532164. doi:10.1155/2019/1532164
Zhao, X., Dong, Y., Zhang, J., Li, D., Hu, G., Yao, J., et al. (2016). Leptin Changes Differentiation Fate and Induces Senescence in Chondrogenic Progenitor Cells. Cell Death Dis 7. doi:10.1038/cddis.2016.68
Zhao, X., Li, Y., Lin, X., Wang, J., Zhao, X., Xie, J., et al. (2018). Ozone Induces Autophagy in Rat Chondrocytes Stimulated with IL-1β through the AMPK/mTOR Signaling Pathway. J. Pain Res. 11, 3003–3017. doi:10.2147/JPR.S183594
Zheng, L., Zhang, Z., Sheng, P., and Mobasheri, A. (2021). The Role of Metabolism in Chondrocyte Dysfunction and the Progression of Osteoarthritis. Ageing Res. Rev. 66, 101249. doi:10.1016/j.arr.2020.101249
Zhou, S., Lu, W., Chen, L., Ge, Q., Chen, D., Xu, Z., et al. (2017). AMPK Deficiency in Chondrocytes Accelerated the Progression of Instability-Induced and Ageing-Associated Osteoarthritis in Adult Mice. Sci. Rep. 7, 1–14. doi:10.1038/srep43245
Keywords: adipokine, osteoarthritis, signaling pathway, cartilage, degeneration, obesity
Citation: Zhang C, Lin Y, Yan CH and Zhang W (2022) Adipokine Signaling Pathways in Osteoarthritis. Front. Bioeng. Biotechnol. 10:865370. doi: 10.3389/fbioe.2022.865370
Received: 29 January 2022; Accepted: 31 March 2022;
Published: 19 April 2022.
Edited by:
Songlin Peng, Southern University of Science and Technology, ChinaReviewed by:
Yong-Can Huang, Peking University Shenzhen Hospital, ChinaGuangyi Li, Shanghai Jiao Tong University Affiliated Sixth People’s Hospital, China
Copyright © 2022 Zhang, Lin, Yan and Zhang. This is an open-access article distributed under the terms of the Creative Commons Attribution License (CC BY). The use, distribution or reproduction in other forums is permitted, provided the original author(s) and the copyright owner(s) are credited and that the original publication in this journal is cited, in accordance with accepted academic practice. No use, distribution or reproduction is permitted which does not comply with these terms.
*Correspondence: Chun Hoi Yan, eWFuY2h1bmhvaUBnbWFpbC5jb20=; Wenming Zhang, emhhbmd3bTA1OTFAZmptdS5lZHUuY24=
†These authors have contributed equally to this work