- 1School of Agriculture, Forestry and Food Engineering, Yibin University, Yibin, China
- 2Government College University, Faisalabad, Pakistan
- 3PMAS Arid Agriculture University, Rawalpindi, Pakistan
- 4State Key Laboratory of Tree Genetics and Breeding, Chinese Academy of Forestry, Beijing, China
- 5Beijing Advanced Innovation Center for Tree Breeding by Molecular Design, National Engineering Laboratory for Tree Breeding, College of Biological Sciences and Technology, Beijing Forestry University, Beijing, China
- 6National Institute for Biotechnology and Genetic Engineering College, Pakistan Institute of Engineering and Applied Sciences (NIBGE-C, PIEAS), Faisalabad, Pakistan
- 7Faculty of Veterinary and Animal Sciences, MNS University of Agriculture, Multan, Pakistan
- 8Biotechnology Research Institute, Chinese Academy of Agricultural Sciences, Beijing, China
- 9Beijing Advanced Innovation Center for Tree Breeding by Molecular Design, National Engineering Laboratory for Tree Breeding, Key Laboratory of Genetics and Breeding in Forest Trees and Ornamental Plants, Ministry of Education, College of Biological Sciences and Biotechnology, Beijing Forestry University, Beijing, China
- 10College of Biological Sciences and Biotechnology, Beijing Forestry University, Beijing, China
- 11College of Agriculture, BZU, Bahadur Sub-Campus Layyah, Layyah, Pakistan
- 12Department of Genetics, Faculty of Agriculture, Zagazig University, Zagazig, Egypt
Nanomaterials (NMs) are the leading edge as an amazing class of materials that consists of at least one dimension in the range of 1–100 nm. NMs can be made with exceptional magnetic, electrical, and catalytic properties different from their bulk counterparts. We summarized unique features of NMs, their synthesis, and advances in agri-nanotechnology and cutting-edge nanobionics. The review describes advances in NMs including their applications, dosimetry to ensure biosafety, remote sensing of agro-forestry fields, nanofertilizers, and nanopesticides, and avoid post-harvest losses, gene delivery, and nanobionics. Tree nanobionics has enabled the synthesis and delivery of nanosensors, which enhance the rate of photosynthesis, detection of pathogens, and poisonous residues to ensure biosafety and biomass accumulation. Finally, we conclude by discussing challenges, future perspectives, and agro-ecological risks of using NMs.
Introduction
The term “nano” is used to describe one-billionth or 10–9. Nanoparticles (NPs) possess three external nanoscale dimensions. Bio-nanotechnology is one of the most advanced technologies dealing with particles in the nanometer (nm) range, which has numerous lucrative properties like small size, large surface area, and high penetration capacity, crossing different cellular barriers, and apart from that, their biocompatible nature makes them more suitable for a number of applications like bioimaging, biosensing, targeted drug delivery, and immunomodulation (Table 1). Living organisms due to their cellular composition are somewhat bigger, of almost 10 µm, and their organelles are in the nanometer range. The smaller proteins of just 5 nm in size are even bigger than the smallest novel NPs. This size comparison urges us to think of nanoparticles’ importance in their working inside the cells without any hindrance.
There are different types of nanostructured materials: 1) carbon-based nanomaterials: composed of carbon and present in the form of carbon black, spheres, nanofibers, or ellipsoids, that is, carbon nanofibers, graphene, fullerenes (C60), carbon nanotubes, and carbon black (Kumar and Kumbhat, 2016; Baig et al., 2021); 2) organic/inorganic-based nanomaterials: made of organic matter called organic-based, while those without carbon are called inorganic-based NMs, and non-covalent (weak) interactions are mostly used for the preparation of organic NMs into required structures like liposomes, polymer, micelles, and dendrimers; 3) composite-based nanomaterials: these are the multiphase NPs and NSMs with one phase on the nanoscale dimension, which is either the combination of NPs with other NPs or NPs with larger or metal–organic frameworks or with bulk-type materials. The composites are formed by the combination of metal-based, organic-based, or carbon-based NMs with any form of ceramic, polymer bulk materials, or metal. Morphologies of the NMs synthesized by the different processes depend upon their applications. Dimensions of the nanomaterials classify under different categories (Table 1) (Jeevanandam et al., 2018).
The terms nanoplate or nanorod are in use instead of nanoparticles (NPs) when the nano-objects are with the shortest and longest axis lengths. Two groups of NPs are being synthesized: organic and inorganic. Magnetic NPs belong to inorganic NPs, which can easily be modified in a magnetic field (Natarajan et al., 2019). Materials after conversion to nanoscale exhibit properties like superparamagnetic behavior, chemical reactivity, extraordinary strength, and electrical conductivity (Table 1). Due to unique physiochemical properties and large surface area, gold nanoparticles (Au-NPs) can be transformed into highly efficient nanosensors. Recently, a Au-NP-plated highly efficient James Webb telescope was launched in 2021 harboring a three-time big mirror but half in weight as compared to its predecessor—the Hubble telescope (Figure 1). Au-NPs are less toxic and biologically compatible to be used as nanosensors in plant research (Youssef and El-Sayed, 2018). Similarly, SiO2 and Ag-NPs are being used at a large scale for filtration and sterile food packaging (Wang et al., 2021).
Phyto-nanotechnology or agri-nanotechnology deals with the use of smallest particles, especially at the atomic level in plant science research. This is because nanotechnology for agricultural applications will have to address the large-scale inherent imperfections and complexities of farm production systems (extremely low input use efficiency) that might require nanomaterials with flexible dimensions, which nevertheless perform tasks efficiently in agricultural production systems. Agri-nanotechnology is being used for targeted gene delivery, drug delivery, fertilizer delivery, biosensing, and bioimaging purposes.
Synthesis of Nanoparticles
The leaves of different plants are being used for the biosynthesis of NPs such as leaves of guava, bitter, moringa, and scent. NPs are also being synthesized using processes like alloys of bimetal, metal oxides, and noble metals. Green synthesis is usually preferred due to the easy availability of plants and their non-toxic and eco-friendly nature (Zafar et al., 2021).
Top-Down Approaches
In this approach, bulky materials are divided into smaller materials to produce NPs (Figure 2). In etching or lithography, the electrons or beam of light is used for developing nanoarchitectures, which are further divided into masked and maskless lithography. In masked lithography, templates or masks are used to transfer the nanopatterns, which further includes soft lithography (Yin et al., 2000), photolithography (Szabó et al., 2013), and nanoimprint lithography (Kuo et al., 2003). In maskless lithography, nanopattern writing is performed without any mask, which further consists of electron beam lithography, ion beam lithography, and scanning probe lithography. Ion implantation can be used to perform micro-nano-fabrication with a focused ion beam along with chemical etching. Electro-explosion is the simplest method for the synthesis of nanostructured materials, which is being commonly used for the synthesis of nanofibers like polymers. The advanced method of electrospinning is coaxial electrospinning, in which two capillaries are used as a spinneret. Two viscous liquids or non-viscous liquids are used as core and viscous as shell to synthesize core–shell nanoarchitectures in an electric field. This technique is used to synthesize ultrathin fibers (Baig et al., 2021).
Mechanical milling is used to produce nanocomposites (Figure 3). Nanocomposites produced from this method are Ni, Cu, Al, or Mg-based nanoalloys, carbide-strengthened aluminum alloys, oxide-strengthened aluminum alloys, wear-resistant spray coating, and many other nanocomposites (Yadav et al., 2012). In sputtering, high-energy gas or plasma particles are bombarded onto a solid surface to produce nanomaterials. The method is used to produce thin nanofilms. Various ways are available for the sputtering process to carry on like DC diode sputtering, magnetron, and radio-frequency diode. Magnetron sputtering is used to produce carbon paper substrates and WSe2-layered. The NPs made using this technique had the same composition as the targeted material with little impurities, and it is also cost-effective (Nie et al., 2009; Nam et al., 2020). In laser ablation, NPs are manufactured by the bombardment of a laser beam at the targeted material to produce vapors. This technique is also known as green synthesis as it does not require any stabilizing agent (Figure 3). Nanomaterials produced by this technique are carbon nanomaterials, ceramics, oxide composites, and metal nanoparticles (Baig et al., 2021).
Bottom-Up Approaches
A bottom-up approach is the accumulation of a substance from the bottom, that is, atom-by-atom, molecule-by-molecule, or cluster-by-cluster (Benelmekki, 2015). Chemical vapor deposition (CVD) is used to produce low cost, good stability, free of impurities, long shelf-life, and non-hazardous carbon nanotubes. In this method, a gas containing carbon is used as a precursor, which upon decomposition produces carbon atoms (Machac et al., 2020). Similarly, the hydrothermal method is performed in heterogeneous reactions in an aqueous medium, while the solvothermal method is performed in a non-aqueous medium at high temperature and pressure in a sealed vessel (Chen and Holt-Hindle, 2010). These methods are used to produce nanorods, nanospheres, nanowires, and nanosheets (Chai et al., 2018; Dong et al., 2020).
Soft and hard templating methods are used to produce nanoporous materials. In the soft templating method, the NPs are produced using many soft templates like flexible organic molecules, cationic, non-ionic, and anionic surfactants, and block copolymers. The soft templating technique is used to produce 3D ordered mesoporous materials such as cubic (MCM-48), hexagonal (MCM-41), ordered mesoporous silicas, and lamellar (MCM-50) (Figure 4). Hard template/nano-casting is used to synthesize silica, colloidal crystals, carbon black, particles, wood shells, and carbon nanotubes (Baig et al., 2021). In reverse micelles, water-in-oil emulsions are used for the synthesis of NPs. The size of the nanoparticles is based on the size of these nanoreactors and the concentration of the water. If water is in large amount, the size will be greater, and vice versa (Malik et al., 2012; Nguyen, 2013). In a sol-gel method, the precursor used is a sol such as metal alkoxide, which is readily transformed into a network structure called gel (Baig et al., 2021). This method is widely used to produce composites, powder, and film nanostructures (Parashar et al., 2020).
Characterization of Nanomaterials
Several techniques are used to characterize the NMs. Atomic-scale images of the crystallographic structure of the nanoparticles are obtained with the help of a transmission electron microscope (TEM) (Frenkel et al., 2001). Similarly, high-resolution transmission electron microscopy (HRTEM) is employed for the determination of arrangements of atoms and their local structures, such as lattice vacancies, screw axes, lattice fringe, defects, glide plane, and the arrangement of the surface atomic structures (Benelmekki, 2015). A 2D image with spatial variations can be obtained with the help of scanning electron microscopy (SEM), in which the data for the selected areas of the surface of the NMs can be collected (Zafar et al., 2021). Atomic force microscopy (AFM) is used to capture 3D pictures for quantitative measurement of NMs both in liquid and gas phases to measure volume, width, height, and length, along with the surface texture and morphology at the submicron level with inbuilt image processor software (Figure 5) (Vahabi et al., 2013).
The nanoparticles ranging from 2 to 100 nm are characterized by using ultraviolet–visible spectroscopy (UV-VIS) (Zafar et al., 2018). Dynamic light scattering (DLS) spectroscopy is used for surface charge measurement and the size of the Brownian NPs in a colloidal solution (Tomaszewska et al., 2013). The bonding characteristics of the various nanoparticles, the structure of the elements present in the magnetic nanoparticles, and the mechanism of the reactions occurring at the surface of the nanoparticles can be determined by X-ray diffraction (XRD) (Benelmekki, 2015). Thermogravimetric analysis (TGA) is used to explore the binding efficiency and composition of the coatings of the nanoparticles such as polymers or surfactants. Raman spectroscopy (RS) is used to determine the vibrational signals of the chemical species which are adsorbed at the surface of the NPs while synthesizing. In addition, it can be used to identify the molecular attachment of a single molecule on the surface of silver NPs (Ali et al., 2021). Fourier transform infrared spectroscopy (FTIR) is used to identify the functional groups such as hydroxyl (OH−) and carbonyl (C=O) moieties that are adsorbed on the surface of the products in nanoparticle synthesis (Figure 5) (Mourdikoudis et al., 2018).
Applications of Nanotechnology in Agriculture
Nano-Sensors
Nanosensors act as nano-carriers envisaged to replace the agrochemicals due to their biological compatibility, less soil contamination, efficiency, and precise release of active substances, increased solubilization, and penetration into plant and target tissues (Prasanna et al., 2021). Nanosensors are chosen over the conventional sensors due to nanoscale size, highest sensitivity, efficient signal transduction mechanism, turn off/on mechanism, and durability (Scognamiglio, 2013). On insect bite, biosynthesis of detectable volatile compounds is stimulated in host plants which are detected by wireless nanosensors (Afsharinejad et al., 2015). Floating bio-nanosensors in photosystem II of the chloroplast detect organo-phosphates, neonicotinoids, carbamates, atrazine pesticides, and transmit signals (Přibyl et al., 2006). Similarly, normal glutathione-regulated AuNP-based bio-nanosensor and ceramic-coated nano-biosensors integrated with Ag-Pd electrodes are being widely employed in the detection of Cd2+ toxicity (Fang et al., 2010).
Electrochemical and electromagnetic signals are precisely detected by electrical nanosensors. These kinds of nanosensors are used to detect the pesticide residues in food and environment, quality assurance, remote sensing of cultivated crops, and climatic conditions. For example, Ag/Au-plated carbon electrodes integrated nanosensors are being used in the detection of triazophos, methyl parathion, and organophosphorus insecticidal residues in the post-harvest vegetables (Zhang et al., 2013). Optical sensors are being employed in the detection of heavy metals in freshwater bodies and soil. Nanosensor-based global positioning system (GPS) has enabled remote monitoring of plant growth and auto-irrigation. 1D nanofibers such as potassium niobate (KNbO3) are being used to measure the level of humidity (Ganeshkumar et al., 2016). The major constraint in the acceptability of nanosensors is their cost due to high-tech machinery and inputs.
Nano-Fertilizers
Crop yield can be increased by 35–40% through adequate fertilizer management, irrigation, and use of quality seeds (Shekhawat et al., 2012). Overdosing of conventional fertilizers is contaminating soil, freshwater bodies, and their nutrient use efficiencies are also very low, that is, N ∼35%, P ∼20%, and K ∼40% (Rosell and Sanz, 2012). Nanofertilizers remain fixed in soil for a long time for the complete absorption of nutrients by plants. Nanofertilizers are slow release, control loss, and magnetic in nature which may contain Zn, SiO2, Fe, TiO2, ZnS, and Mn/ZnSe. Metal oxides such as CeO2, ZnO, TiO2, Al2O3, FeO, and ZnO are also being used as nanofertilizers (Sabir et al., 2014). Nano-ZnO- and nano-sulfur-coated monoammonium phosphate and urea displayed slow release of Zn and S to enhance their uptake efficiency (Wilson et al., 2008; Milani et al., 2012). Similarly, nitrogen release was twice that of conventional urea fertilizer when hydroxyapatite-NPs were used to coat urea and encapsulate it into softwood cavities (Kottegoda et al., 2011). Nanofertilizers are easily absorbed by roots and leaves and have numerous advantages over the traditional fertilizers (Figure 6).
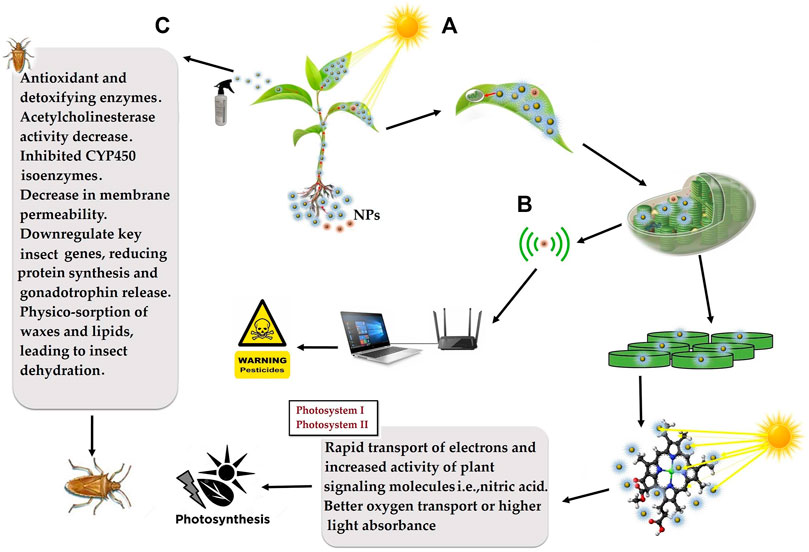
FIGURE 6. Schematic diagram of nanoparticles application for; (A) enhanced light harvesting to accelerate the rate of photosynthesis for increased biomass accumulation and crop yield, (B) nanobionics application in early detection of pathogenic infection and pesticidal poisonous residues for improved biosafety, and (C) slow-release nanopesticides application for precise and durable pest management.
Nanofertilizers are designed by encapsulation of cationic nutrients such as Ca2+, K+, NH4+, and Mg2+ or anionic nutrients such as SO4−, PO4−, and NO3− in nanoparticles, nanoporous materials, or coated on thin films. Nanofertilizers such as NH3-zeolites and K-graphene oxide enhanced P and K use efficiency by avoiding leaching and the precise release of nutrients in the required amount. Similarly, the application of nano-calcite (CaCO3) along with Fe2O3, SiO2 and MgO nanoparticles enhanced the uptake of Ca, Fe, Mg, P, Zn, and Mn (Sabir et al., 2014). The application of ZnO nanofertilizer to barley crop resulted in enhanced photosynthesis rate, root length, shoot length, and 60% higher yield as compared to the traditional ZnSO4 fertilizer (Kale and Kane, 2017). The application of carbon dots together with traditional fertilizers resulted in an increased yield of soybean by 16.74%, rice by 10.29%, maize by 10.93%, and wheat by 28.81% (Iqbal and Umar, 2019). Long time or overuse of nanofertilizers can contaminate the soil and may have some serious health issues in animals and human beings.
Nano-Pesticides
Conventional pesticides are being widely sprayed to control insect pests but only 0.1% of active ingredients reach the target pest (Carriger et al., 2006). Biopesticides are cheap and eco-friendly alternatives of synthetic pesticides, but their mode of action is comparatively slow. Nanopesticides are robust due to the slow and controlled release of poisons to provide durable insect control (Kah et al., 2019). Nanopesticides in low concentration are more effective such as 9.86 mg/L of 50% SDS/Ag/TiO2-IMI can control 100% Martianus dermestoides as compared to 13.45 mg/L of 95% conventional IMI (Guan et al., 2008). Nano-permethrin, nano-acephate, and nano-carbofuran efficiently reach target pests as compared to conventional permethrin, acephate, and carbofuran (Pradhan et al., 2013). SiO2–chlorfenapyr controlled cotton bollworms two times higher than chlorfenapyr microparticles (Wu et al., 2017). Nowadays, nanopesticides are being commercially produced by some multinational companies such as Primo Maxx®, Subdue Maxx®, Banner Maxx® II, Karate Zeon, Penncap-M®, Syngenta, and BASF (Gouin et al., 2008). The application of Ag-SiO2 myco-nanoparticles controlled the following pathogenic fungi Rhizoctonia solani, Colletotrichum gloeosporioides, and Magnaporthe grisea, and Raffaelea species in oak trees (Alghuthaymi et al., 2015). A mixture of trifloxystrobin 25% and tebuconazole 50% nano-fungicides controlled the soil-borne fungus Macrophomina phaseolina (Singh et al., 2017). Nanopesticides are costly and not in reach of common farmers.
Nano-Herbicides
The conventional herbicides are expensive, contaminate the environment, and are hazardous to health, and seize crop growth. Nano-herbicides are a good choice to mitigate the disadvantages of conventional herbicides by precise and targeted delivery and easy to degrade. Nano-atrazine, nano-ametryn, nano-simazine, and nano-paraquat were synthesized via encapsulation in poly (epsilon caprolactone) to enhance their activity, durability, avoid leaching, and toxicity (Anderson et al., 2018). Effectiveness of nano-atrazine against Amaranthus viridis and Bidens pilosa is many folds high as compared to conventional atrazine (De Souza et al., 2021). Nano-emulsion of glyphosate revealed its enhanced efficacy as compared to the commercial formulation (Lim et al., 2013). Conventional herbicides also have serious consequences over beneficial insects such as earthworms exposed to conventional atrazine displayed vacuolization, pyknotic cells, and dismantled epithelial tissues, and chloragogenous layer (Oluah et al., 2010). On the other hand, nano-herbicides resulted in lower toxicity to Pseudokirchneriella subcapitata and Prochilodus lineatus (Andrade et al., 2019).
Nano-Fungicide
Pathogenic fungi such as Alternaria alternata, Fusarium oxysporum, Phoma destructiva, and Cochliobolus lunata cause diseases in plants and resulted in severe yield loss. NMs are being employed in unraveling plant–microbe interaction networks. Nano-fungicides precisely and efficiently mitigate pathogenicity caused by fungal pathogens as compared to conventional fungicides. For example, application of nano-Ag causes severe halt in hyphae, conidiophores, and conidia development of powdery mildew which causes serious damages to cucurbits family (Lamsal et al., 2011). Similarly, the application of TiO2 and ZnO NPs seize the growth of a broad range of plant fungal pathogens such as Penicillium expansum, Botrytis cinerea, and fungal pathogens of litchi (Lu et al., 2006). The biggest challenge in use of nano-fungicides is the unavailability of established dosimetry and high cost.
Nanomaterials Application at Post-harvest Level
Green-synthesized Au/Ag-NPs along with edible coating are being employed in maintaining the long-lasting shelf life of fruits and vegetables to refrain from potential post-harvest loss causing phytopathogens (Nxumalo and Olaniyi, 2022). Post-harvest losses are predominantly caused by the following two insect groups: Coleoptera and Lepidoptera. The application of nano-Ca on fruits and vegetables has proved very effective to control oriental fruit fly and red scale insects (Kitherian, 2017). Similarly, CaCO3-NPs were used as fertilizers to improve the nutritional value of plants and insecticides. Spraying of SiO3-NPs effectively controlled larvae of Asian armyworm (Kitherian, 2017). The modification of Ag-electrodes with Cu-NPs in nanosensors help in early detection of karnal bunt disease in wheat and real-time measurement of salicylic acid (SA) contents in oil extracted from seeds (Kashyap et al., 2017). Spreading of a handful of nano-Al2O3 on wheat grains thoroughly killed the following two insect pests: Stiophilus oryzae and Rhyzopertha dominica (Stadler et al., 2010). To avoid harmful effects of conventional poisonous pesticides over human health, essential oil extract from anise (Pimpinella anisum) in the form of nano-emulsion is being used to control flour beetle (Tribolium castaneum) and nano-SiO2 to control insect pests during grain storage (Gamal, 2018; Rangasamy et al., 2018). Although, the NP application in the field of post-harvest loss has gained notable attention, the major limitations in their commercialization are production cost and potential health risks.
Nanomaterials for Farmland Restoration
The cultivable and fertile farmlands are going to be barren due to over-cultivation, water scarcity, and global warming. NMs have potential in the restoration of farmlands such as hydrogel (potassium polyacrylate), nano-clays, and nano-zeolites can enhance the water holding capacity of soil by 50–70% and reduce soil compactness by 8–10% (Sekhon, 2014). Water-insoluble polymers such as hydrogel application in rainfed land enhanced water use efficiency by avoiding leaching, improved soil texture, evaporation, microbial activity, and resulted in increased crop yield. NMs are eco-friendly, non-toxic to plants, and rapidly degraded into nitrogen, water, and CO2. Similarly, heat-repellant fertilizers such as nano-zeolites increase water holding capacity, nutrient use efficiency, soil aeration, microbial growth, and soil decontamination by absorbing heavy metals (Saponaro et al., 2016).
Nanomaterials as Drought-Tolerant Agents
Drought stress can lead to the production of oxygen radicals that result in increased lipid peroxidation and oxidative stress in the plants. Visible effects include stunted growth, narrow leaves, damaged foliar matrix, and decreased biomass contents. Hydrogel can reduce the drought impact on plants leading to reduced stress and oxygen radical formation. This in turn provides scope for better growth and yield even in unfavorable climatic conditions.
Nanomaterials as Growth Enhancers
Irrigation technology has major constraints in the fields of application of fertilizers, herbicides, and germicides. Studies suggest that the use of synthetic fertilizers can be greatly reduced when hydrogel agriculture is practiced without hindering crop yield and nutritional value. It would indeed be more appropriate practice for sustainable agriculture in arid and semi-arid conditions and regions with similar ecological constraints. Moreover, potassium polyacrylate is safe and non-toxic, thus preventing pollution of agro-ecosystems.
Nanomaterials Induced Biomass Accumulation
Carbon dots have a size range between 1–10 nm along with having fluorescent properties. Carbon dots absorb a range of UV light, get excited, and express usually bluish in color at neutral pH. By taking into account the biomass production of any crop or plant can be increased through the application of carbon dots which express blue color. Applied carbon dots are first absorbed by plant roots, later transported to aerial parts and enter cellular organelles, especially chloroplast. In the thylakoid membrane, the carbon dots absorb blue light similar to other pigments present in the photosystem I or II and transfer the obtained energy to the main reaction. The sunlight absorbed by these carbon dots enhances photosynthesis, which ultimately leads to high biomass production (Figure 6). For example, the application of single-walled carbon dots enhance the rate of near-infrared fluorescence light-harvesting, rate of electron transfer by 49%, and downregulate ROS in chloroplast resulting in increased photosynthetic efficiency and crop yield (Giraldo et al., 2014). The application of nano-TiO2 resulted in an increased rate of photosynthesis of spinach by 3.13 times (Zheng et al., 2005). At present, the development of nano-robots and their delivery is challenging which further needs improvement.
Nanoparticles Mediated Cell Culture and Gene Delivery
In order to get the desired aesthetic traits, biotic, and abiotic stress resistance, higher crop yield, and increased biomass for the paper and pulp industry, genetic transformation is inevitable. The exposure of plant cells to NPs perturbs genetic expression, alters biological pathways, and affect plant metabolism (Nair, 2012). The delivery of RNAi constructs coated NPs, including chitosan, cationic dendrimers, and liposomes in insects to target lethal genes for insect control is a promising tool in sustainable crop yield (Yan et al., 2021). SiO2-NPs are being widely employed in gene transformation in animal cells, but it is not successful in plants cells due to the hard cell wall. Recently, gold encapsulated mesoporous SiO2-NPs have been developed which can pass through the cell wall of plants (Torney et al., 2007). Similarly, a cell culture of tobacco can be increased 55–64% by supplementing with multi-walled carbon nanotubes (Khodakovskaya et al., 2012).
Agro-Ecological Risks
Soil Contamination
Soil, a primary recipient of nanofertilizers, is subjected to contamination and side effects caused by chemical processes by NPs and have serious consequences over soil microflora, microfauna, injuries to earthworms digestive tract, and consequently soil fertility (Dubey and Mailapalli, 2016). The properties of nanoparticles may change the structure of the soil and default to detect contamination due to nanoparticles in soil and environment (Lacave et al., 2020). The size of NPs determines their ecotoxic effects such as TiO2 with 7-nm surface area and CeO2 with 15-nm surface area are highly toxic to nematode (Caenorhabditis elegans) as compared to comparatively larger size TiO2 with 20 nm and CeO2 with 45-nm surface area, respectively (Roh et al., 2010). If doses are increased from a certain amount, ZnO nanoparticle becomes toxic to the soil. Similarly, the application of a higher concentration of ZnO such as 5 g/kg of soil resulted in over-accumulation of ZnO in the body of earthworm (Eisenia fetida) and resulted in DNA damage (Hu et al., 2010).
Phyto-Toxicity
In plants, toxic nanoparticles cause severe halt in respiration and photosynthesis (Navarro et al., 2008). The toxicity caused by TiO2 nanoparticles is more severe on algae than daphnids (Hund-Rinke and Simon, 2006). Nanoparticles of TiO2 retain in the soil for a long time, stick with the cell wall of the wheat plant, and resulted in halt in biomass (Du et al., 2019). The corn, lettuce, and rye grass seedlings exposed to Al2O3 nanoparticles displayed stunted roots, while radish and rapeseed displayed elongated roots (Lin and Xing, 2007). Rice seedlings exposed to AgNPs displayed damaged cell walls due to the transport of nanoparticles (Mazumdar and Ahmed, 2011). Notably, ZnO-based nanoparticles remain fixed in the soil for a longer time and are toxic to plants. For example, rice seedlings exposed to ZnO nanoparticles displayed stunted root growth and severe halt in biomass contents (Boonyanitipong et al., 2011). Uncoated Al2O3-NPs application on corn, soybean, cucumber, carrot, and cabbage resulted in stunted roots (Yang and Watts, 2005).
Water Contamination
The leaching of toxic nanoparticles such as Ag, Cu, Al, Ni, TiO2, and Co in freshwater bodies and air is very toxic to humans, animals, and aquatic life. Notably, toxicity caused by nanoparticles in freshwater bodies and air is irreversible. Metal-based nanoparticles are less toxic as compared to their oxides such as TiO2 which causes severe halt in the rate of photosynthesis in algal cells (Sharma et al., 2009). Nanoparticles made of oxides of Ag, Fe, and Cu enhance the rate of mortality, premature hatching, and heart diseases in zebra fish (Zhu et al., 2012).
Human Health Risks
The exposure to nanoparticles through inhalation of air, ingestion through the gastrointestinal tract, and dermal contact may cause serious health risks. Poor ventilation system during manufacturing is a serious cause of inhalation of nanoparticles (Gaffet, 2006). Inhaled nanoparticles of size ≥50 nm pass through the gastrointestinal tract and are transported to the spleen, liver, blood, and finally the bone marrow (Hagens et al., 2007). Originally, the skin is a barrier against pests and contaminants but the injured skin due to dermatitis, eczema, and irritation let nanoparticles penetrate the body which may cause edema, erythema and, eschar formation.
Dosimetry of Nanomaterials to Ensure Biosafety
Indeed, it is mandatory to determine the optimum level of concentration of any new material which must be safer to use. Therefore, the required dose of NMs was determined by lethal dose 50 (LD50). Through LD50, the level of cellular toxicity is determined that at what concentration of NPs cause 50% cell mortality and 50% cell viability. The poisoning potential of NPs can be measured through a variety of assays such as 3-(4,5-dimethylthiazol-2-yl)-2,5-diphenyltetrazolium bromide (MTT) (Raja et al., 2019), terminal deoxynucleotidyl transferase dUTP nick end labeling (TUNEL) apoptosis assay (Jin et al., 2021), Alamar blue assay (Usman et al., 2020), sulforhodamine B (SRB) (Massard et al., 2018), and cell counting kit-8 (CCK-8; Dojindo Molecular Technologies) (Jin et al., 2021) can be used to determine the cellular toxicity (mortality or viability percentage). In vivo application of NPs in agriculture can cause genotoxicity to both terrestrial and aquatic animals which can be measured using sodium dodecyl polyacrylamide gel electrophoresis (SDS-PAGE) assay (El-Sappah et al., 2022). Immunotoxicity caused by NPs can be determined by pro-inflammatory and anti-inflammatory cytokines (Engin and Hayes, 2018). NPs can be employed in the electrochemical detection of heavy metals in agriculture and seafood to ensure biosafety (Aragay and Merkoçi, 2012).
Conclusion and Future Perspectives
Agri-nanotechnology has revolutionized the designing and application of nanoparticles of size 1–100 nm in agriculture and forestry. Nanofertilizers such as SiO2-N remain fixed in the soil for a long time to enhance fertilizer use efficiency and avoid soil and water contamination to ensure biosafety. Nanobionics has enabled the synthesis of nano-robots which can pass through the cell membrane, translocate among organelles, capture photos, and transmit via wireless devices. Nanosensors are being employed in the detection of pesticide residues in fruits, vegetables, and seed oil at the post-harvest level to avoid health risks. A thin lining of green-synthesized edible NPs inside metal packaging improves shelf life, maintains original taste and nutrition, avoids corrosion of metal particles, and ensures health benefits. Nanosensors are being employed in unraveling plant microbe interaction and mitigation of pathogenicity to ensure the biosafety. Hydrogel polymers and nano-zeolites have water holding, heat resistance, enhanced soil aeration, and microbial growth capacity to tolerate drought and heat stress as well as farmland restoration. The prime cause of failure of genetic transformation is landing of construct in junk DNA, which is 70% of the total. Gold capped nano-SiO2 particles have potential in chromosome and site-specific gene delivery. GPS-based nano-devices have enabled remote sensing of agro-forestry cultivation, auto-irrigation, and safety. Among forestry products, cellulose is of high importance because of its use as feedstock in the paper and pulp industry. In photosystems I and II, bionic nanosensors potentially enhance light harvesting and biomass accumulation. Today, the commercial application of NPs in agriculture and forestry is not common due to expensive raw materials and the requirement of high-tech machinery. With the improvement in technology, NP-based agrochemicals will be readily available to farmers, which will contribute much to the improvement of yield, nutritional value, shelf life, and biosafety.
Data Availability Statement
The datasets presented in this study can be found in online repositories. The names of the repository/repositories and accession number(s) can be found below: GSE192458.
Author Contributions
Conceptualization: MA, KY, JL, SZ, ZH, and AHEl-S; designed the experiments, MA, SZ, ZH, and NI; performed the experiments. MA, SZ, KY, ZH, NA, SSH, MU, MA, MT, SA, JL, SKA, HQ, XZ, and AHEl-S; analyzed the data, MA, SZ, KY, ZH, and AHEl-S; wrote the manuscript, MA, SZ, ZH, and AHEl-S. All authors reviewed the manuscript.
Funding
This work was co-supported by Sichuan Provincial Department of Science and Technology Project (Grant no. 2020JDRC0083) and Scientific Research Project of Yibin University (Grant nos. 2020RC09 and XJ2020007601).
Conflict of Interest
The authors declare that the research was conducted in the absence of any commercial or financial relationships that could be construed as a potential conflict of interest.
Publisher’s Note
All claims expressed in this article are solely those of the authors and do not necessarily represent those of their affiliated organizations, or those of the publisher, the editors, and the reviewers. Any product that may be evaluated in this article, or claim that may be made by its manufacturer, is not guaranteed or endorsed by the publisher.
Acknowledgments
We are very grateful to the kind administration of Yibin University, Yibin 644000, China, and Government College University, Faisalabad, Pakistan, for providing us such a prestigious and well-equipped platform for research and development. We are also grateful to the kind administration of the department of Science and Technology of Sichuan Province, P.R. China.
References
Afsharinejad, A., Davy, A., Jennings, B., and Brennan, C. (2016). Performance Analysis of Plant Monitoring Nanosensor Networks at THz Frequencies. IEEE Internet Things J. 3 (1), 59–69. doi:10.1109/JIOT.2015.2463685
Alghuthaymi, M. A., Almoammar, H., Rai, M., Said-Galiev, E., and Abd-Elsalam, K. A. (2015). Myconanoparticles: Synthesis and Their Role in Phytopathogens Management. Biotechnol. Biotechnological Equipment 29 (2), 221–236. doi:10.1080/13102818.2015.1008194
Ali, A., Shah, T., Ullah, R., Zhou, P., Guo, M., Ovais, M., et al. (2021). Review on Recent Progress in Magnetic Nanoparticles: Synthesis, Characterization, and Diverse Applications. Front. Chem. 9. doi:10.3389/fchem.2021.629054
Anderson, A. A., Yeo, S. K., Brossard, D., Scheufele, D. A., and Xenos, M. A. (2018). Toxic Talk: How Online Incivility Can Undermine Perceptions of Media. Int. J. Public Opin. Res. 30 (1), 156–168. doi:10.1093/ijpor/edw022
Andrade, M., De Marchi, L., Pretti, C., Chiellini, F., Morelli, A., Figueira, E., et al. (2019). The Impacts of Warming on the Toxicity of Carbon Nanotubes in Mussels. Mar. Environ. Res. 145, 11–21. doi:10.1016/j.marenvres.2019.01.013
Aragay, G., and Merkoçi, A. (2012). Nanomaterials Application in Electrochemical Detection of Heavy Metals. Electrochimica Acta 84, 49–61. doi:10.1016/j.electacta.2012.04.044
Arrabito, G., Aleeva, Y., Ferrara, V., Prestopino, G., Chiappara, C., and Pignataro, B. (2020). On the Interaction Between 1D Materials and Living Cells. Jfb 11 (2), 40. doi:10.3390/jfb11020040
Baig, N., Kammakakam, I., and Falath, W. (2021). Nanomaterials: A Review of Synthesis Methods, Properties, Recent Progress, and Challenges. Mater. Adv. 2 (6), 1821–1871. doi:10.1039/D0MA00807A
Benelmekki, M. (2015). “An Introduction to Nanoparticles and Nanotechnology,” in Designing Hybrid Nanoparticles (Morgan & Claypool Publishers).
Boonyanitipong, P., Kositsup, B., Kumar, P., Baruah, S., and Dutta, J. (2011). Toxicity of ZnO and TiO2 Nanoparticles on Germinating Rice Seed Oryza Sativa L. Ijbbb 1 (4), 282–285. doi:10.7763/ijbbb.2011.v1.53
Carriger, J. F., Rand, G. M., Gardinali, P. R., Perry, W. B., Tompkins, M. S., and Fernandez, A. M. (2006). Pesticides of Potential Ecological Concern in Sediment From South Florida Canals: An Ecological Risk Prioritization for Aquatic Arthropods. Soil Sediment. Contamination: Int. J. 15 (1), 21–45. doi:10.1080/15320380500363095
Chai, B., Xu, M., Yan, J., and Ren, Z. (2018). Remarkably Enhanced Photocatalytic Hydrogen Evolution Over MoS 2 Nanosheets Loaded on Uniform CdS Nanospheres. Appl. Surf. Sci. 430, 523–530. doi:10.1016/j.apsusc.2017.07.292
Chen, A., and Holt-Hindle, P. (2010). Platinum-Based Nanostructured Materials: Synthesis, Properties, and Applications. Chem. Rev. 110 (6), 3767–3804. doi:10.1021/cr9003902
de Souza Antônio, R., Guerra, A. C. S., de Andrade, M. B., Nishi, L., Baptista, A. T. A., Bergamasco, R., et al. (2021). Application of Graphene Nanosheet Oxide for Atrazine Adsorption in Aqueous Solution: Synthesis, Material Characterization, and Comprehension of the Adsorption Mechanism. Environ. Sci. Pollut. Res. 28 (5), 5731–5741. doi:10.1007/s11356-020-10693-4
Dong, Y., Du, X.-q., Liang, P., and Man, X.-l. (2020). One-Pot Solvothermal Method to Fabricate 1D-VS4 Nanowires as Anode Materials for Lithium Ion Batteries. Inorg. Chem. Commun. 115, 107883. doi:10.1016/j.inoche.2020.107883
Du, W., Yang, J., Peng, Q., Liang, X., and Mao, H. (2019). Comparison Study of Zinc Nanoparticles and Zinc Sulphate on Wheat Growth: From Toxicity and Zinc Biofortification. Chemosphere 227, 109–116. doi:10.1016/j.chemosphere.2019.03.168
Dubey, A., and Mailapalli, D. R. (2016). “Nanofertilisers, Nanopesticides, Nanosensors of Pest and Nanotoxicity in Agriculture,” in Sustainable Agriculture Reviews (Springer), 307–330. doi:10.1007/978-3-319-26777-7_7
El-Sappah, H. A., Seif, M., Heba, A.-K., Salma, A. S., Sameh, A. A., El-Sappah, H. H., et al. (2022). Genotoxicity and Trace Elements Contents Analysis in Nile Tilapia (Oreochromis niloticus) Indicated the Levels of Aquatic Contamination at Three Egyptian Areas. Front. Vet. Sci. 1, 1. doi:10.3389/fvets.2022.818866
Engin, A. B., and Hayes, A. W. (2018). The Impact of Immunotoxicity in Evaluation of the Nanomaterials Safety. Toxicol. Res. Appl. 2, 239784731875557. doi:10.1177/2397847318755579
Fang, W., Goldberg, M. L., Pohl, N. M., Bi, X., Tong, C., Xiong, B., et al. (2010). Functional and Physical Interaction Between the Selenium-Binding Protein 1 (SBP1) and the Glutathione Peroxidase 1 Selenoprotein. Carcinogenesis 31 (8), 1360–1366. doi:10.1093/carcin/bgq114
Frenkel, A. I., Hills, C. W., and Nuzzo, R. G. (2001). A View From the inside: Complexity in the Atomic Scale Ordering of Supported Metal Nanoparticles. J. Phys. Chem. B. 105 (51), 12689–12703. doi:10.1021/jp012769j
Gaffet, E. (2006). Les nanomatériaux: effets sur la santé de l’homme et sur l’environnement. Afsset, 1–248.
Gamal, M. (2018). Nano-Particles: A Recent Approach for Controlling Stored Grain Insect Pests. Academia J. Agric. Res. 6 (5 Conference Proceedings), 88–94.
Ganeshkumar, R., Sopiha, K. V., Wu, P., Cheah, C. W., and Zhao, R. (2016). Ferroelectric KNbO3 Nanofibers: Synthesis, Characterization and Their Application as a Humidity Nanosensor. Nanotechnology 27 (39), 395607. doi:10.1088/0957-4484/27/39/395607/meta
Giraldo, J. P., Landry, M. P., Faltermeier, S. M., McNicholas, T. P., Iverson, N. M., Boghossian, A. A., et al. (2014). Plant Nanobionics Approach to Augment Photosynthesis and Biochemical Sensing. Nat. Mater. 13 (4), 400–408. doi:10.1038/nmat3890
Gouin, T., Shoeib, M., and Harner, T. (2008). Atmospheric Concentrations of Current-Use Pesticides across South-Central Ontario Using Monthly-Resolved Passive Air Samplers. Atmos. Environ. 42 (34), 8096–8104. doi:10.1016/j.atmosenv.2008.05.070
Guan, H., Chi, D., Yu, J., and Li, X. (2008). A Novel Photodegradable Insecticide: Preparation, Characterization and Properties Evaluation of Nano-Imidacloprid. Pestic. Biochem. Physiol. 92 (2), 83–91. doi:10.1016/j.pestbp.2008.06.008
Hagens, W. I., Oomen, A. G., de Jong, W. H., Cassee, F. R., and Sips, A. J. A. M. (2007). What Do We (Need To) Know About the Kinetic Properties of Nanoparticles in the Body? Regul. Toxicol. Pharmacol. 49 (3), 217–229. doi:10.1016/j.yrtph.2007.07.006
Hu, C. W., Li, M., Cui, Y. B., Li, D. S., Chen, J., and Yang, L. Y. (2010). Toxicological Effects of TiO2 and ZnO Nanoparticles in Soil on Earthworm Eisenia fetida. Soil Biol. Biochem. 42 (4), 586–591. doi:10.1016/j.soilbio.2009.12.007
Huang, D., Li, Z., Zeng, G., Zhou, C., Xue, W., Gong, X., et al. (2019). Megamerger in Photocatalytic Field: 2D G-C3n4 Nanosheets Serve as Support of 0D Nanomaterials for Improving Photocatalytic Performance. Appl. Catal. B: Environ. 240, 153–173. doi:10.1016/j.apcatb.2018.08.071
Hund-Rinke, K., and Simon, M. (2006). Ecotoxic Effect of Photocatalytic Active Nanoparticles (TiO2) on Algae and Daphnids (8 Pp). Env. Sci. Poll Res. Int. 13 (4), 225–232. doi:10.1065/espr2006.06.311
Iqbal, M., Umar, S., and Mahmooduzzafar, fnm. (2019). “Nano-Fertilization to Enhance Nutrient Use Efficiency and Productivity of Crop Plants,” in Nanomaterials and Plant Potential (Springer), 473–505. doi:10.1007/978-3-030-05569-1_19
Jeevanandam, J., Barhoum, A., Chan, Y. S., Dufresne, A., and Danquah, M. K. (2018). Review on Nanoparticles and Nanostructured Materials: History, Sources, Toxicity and Regulations. Beilstein J. Nanotechnol. 9 (1), 1050–1074. doi:10.3762/bjnano.9.98
Jin, M., Li, N., Sheng, W., Ji, X., Liang, X., Kong, B., et al. (2021). Toxicity of Different Zinc Oxide Nanomaterials and Dose-Dependent Onset and Development of Parkinson's Disease-like Symptoms Induced by Zinc Oxide Nanorods. Environ. Int. 146, 106179. doi:10.1016/j.envint.2020.106179
Kah, M., Navarro, D., Kookana, R. S., Kirby, J. K., Santra, S., Ozcan, A., et al. (2019). Impact of (Nano)formulations on the Distribution and Wash-Off of Copper Pesticides and Fertilisers Applied on Citrus Leaves. Environ. Chem. 16 (6), 401–410. doi:10.1071/EN18279
Kale, R. D., and Kane, P. B. (2017). Colour Removal Using Nanoparticles. Text Cloth Sustain. 2 (1), 1–7. doi:10.1186/s40689-016-0015-4
Kashyap, P. L., Kumar, S., and Srivastava, A. K. (2017). Nanodiagnostics for Plant Pathogens. Environ. Chem. Lett. 15 (1), 7–13. doi:10.1007/s10311-016-0580-4
Khodakovskaya, M. V., De Silva, K., Biris, A. S., Dervishi, E., and Villagarcia, H. (2012). Carbon Nanotubes Induce Growth Enhancement of Tobacco Cells. ACS Nano 6 (3), 2128–2135. doi:10.1021/nn204643g
Kitherian, S. (2017). Nano and Bio-Nanoparticles for Insect Control. Res. J. Nanoscience Nanotechnology 7 (1), 1–9. doi:10.3923/rjnn.2017
Kottegoda, N., Munaweera, I., Madusanka, N., and Karunaratne, V. (2011). A Green Slow-Release Fertilizer Composition Based on Urea-Modified Hydroxyapatite Nanoparticles Encapsulated Wood. Curr. Sci. 1, 73–78.
Kuo, C.-W., Shiu, J.-Y., Cho, Y.-H., and Chen, P. (2003). Fabrication of Large-Area Periodic Nanopillar Arrays for Nanoimprint Lithography Using Polymer Colloid Masks. Adv. Mater. 15 (13), 1065–1068. doi:10.1002/adma.200304824
Lacave, J. M., Bilbao, E., Gilliland, D., Mura, F., Dini, L., Cajaraville, M. P., et al. (2020). Bioaccumulation, Cellular and Molecular Effects in Adult Zebrafish After Exposure to Cadmium Sulphide Nanoparticles and to Ionic Cadmium. Chemosphere 238, 124588. doi:10.1016/j.chemosphere.2019.124588
Lamsal, K., Kim, S. W., Jung, J. H., Kim, Y. S., Kim, K. S., and Lee, Y. S. (2011). Application of Silver Nanoparticles for the Control of Colletotrichum Species In Vitro and Pepper Anthracnose Disease in Field. Mycobiology 39 (3), 194–199. doi:10.5941/MYCO.2011.39.3.194
Lim, C. J., Basri, M., Omar, D., Abdul Rahman, M. B., Salleh, A. B., and Raja Abdul Rahman, R. N. Z. (2013). Green Nanoemulsion-Laden Glyphosate Isopropylamine Formulation in Suppressing Creeping Foxglove (A. Gangetica), Slender Button Weed (D. Ocimifolia) and Buffalo Grass (P. Conjugatum). Pest Manag. Sci. 69 (1), 104–111. doi:10.1002/ps.3371
Lin, D., and Xing, B. (2007). Phytotoxicity of Nanoparticles: Inhibition of Seed Germination and Root Growth. Environ. Pollut. 150 (2), 243–250. doi:10.1016/j.envpol.2007.01.016
Lu, J. W., Li, F. B., Guo, T., Lin, L. W., Hou, M. F., and Liu, T. X. (2006). TiO2 Photocatalytic Antifungal Technique for Crops Diseases Control. J. Environ. Sci. 18 (2), 397–401.
Machac, P., Cichon, S., Lapcak, L., and Fekete, L. (2020). Graphene Prepared by Chemical Vapour Deposition Process. Graphene Technol. 5, 9–17. doi:10.1007/s41127-019-00029-6
Malik, M. A., Wani, M. Y., and Hashim, M. A. (2012). Microemulsion Method: A Novel Route to Synthesize Organic and Inorganic Nanomaterials. Arabian J. Chem. 5 (4), 397–417. doi:10.1016/j.arabjc.2010.09.027
Massard, C., Dubois, C., Raspal, V., Daumar, P., Sibaud, Y., Mounetou, E., et al. (2018). Cytotoxicity Study of Gold Nanoparticles on the Basal-like Triple-Negative HCC-1937 Breast Cancer Cell Line. Jbnb 09 (01), 13–25. doi:10.4236/jbnb.2018.91002
Mazumdar, H., and Ahmed, G. (2011). Phytotoxicity Effect of Silver Nanoparticles on Oryza Sativa. Int. J. ChemTech Res. 3 (3), 1494–1500.
Milani, S., Baldelli Bombelli, F., Pitek, A. S., Dawson, K. A., and Rädler, J. (2012). Reversible versus Irreversible Binding of Transferrin to Polystyrene Nanoparticles: Soft and Hard Corona. ACS Nano 6 (3), 2532–2541. doi:10.1021/nn204951s
Mourdikoudis, S., Pallares, R. M., and Thanh, N. T. K. (2018). Characterization Techniques for Nanoparticles: Comparison and Complementarity Upon Studying Nanoparticle Properties. Nanoscale 10 (27), 12871–12934. doi:10.1039/C8NR02278J
Nair, P. K. R. (2012). Carbon Sequestration Studies in Agroforestry Systems: A Reality-Check. Agroforest Syst. 86 (2), 243–253. doi:10.1007/s10457-011-9434-z
Nam, J. H., Jang, M. J., Jang, H. Y., Park, W., Wang, X., Choi, S. M., et al. (2020). Room-Temperature Sputtered Electrocatalyst WSe2 Nanomaterials for Hydrogen Evolution Reaction. J. Energ. Chem. 47, 107–111. doi:10.1016/j.jechem.2019.11.027
Nandwana, V., Huang, W., Li, Y., and Dravid, V. P. (2018). One-Pot Green Synthesis of Fe3O4/MoS2 0D/2D Nanocomposites and Their Application in Noninvasive Point-of-Care Glucose Diagnostics. ACS Appl. Nano Mater. 1 (4), 1949–1958. doi:10.1021/acsanm.8b00429
Natarajan, S., Harini, K., Gajula, G. P., Sarmento, B., Neves-Petersen, M. T., and Thiagarajan, V. (2019). Multifunctional Magnetic Iron Oxide Nanoparticles: Diverse Synthetic Approaches, Surface Modifications, Cytotoxicity Towards Biomedical and Industrial Applications. BMC Mat. 1 (1), 1–22. doi:10.1186/s42833-019-0002-6
Navarro, E., Piccapietra, F., Wagner, B., Marconi, F., Kaegi, R., and Odzak, N. (2008). Toxicity of Silver Nanoparticles to Chlamydomonas reinhardtii. Environ. Sci. Technol. 42, 8959–8964.
Ngô, C., and Van de Voorde, M. H. (2014). “Nanomaterials: Doing More with Less,” in Nanotechnology in a Nutshell (Springer), 55–70. doi:10.2991/978-94-6239-012-6_4
Nguyen, T.-D. (2013). From Formation Mechanisms to Synthetic Methods Toward Shape-Controlled Oxide Nanoparticles. Nanoscale 5 (20), 9455–9482. doi:10.1039/C3NR01810E
Nie, M., Sun, K., and Meng, D. D. (2009). Formation of Metal Nanoparticles by Short-Distance Sputter Deposition in a Reactive Ion Etching Chamber. J. Appl. Phys. 106 (5), 054314. doi:10.1063/1.3211326
Nxumalo, K. A., and Olaniyi, A. (2022). “Green Synthesized Nanomaterials as an Alternative to Restricted Chemicals in Postharvest Preservation of Horticultural Crops: A Review,” in E3S Web of Conferences, 05006.
Oluah, M., Obiezue, R. N. N., Ochulor, A. J., and Onuoha, E. (2010). Toxicity and Histopathological Effect of Atrazine (Herbicide) on the Earthworm Nsukkadrilus Mbae under Laboratory Conditions. Anim. Res. Int. 7 (3), 1287–1293.
Parashar, M., Shukla, V. K., and Singh, R. (2020). Metal Oxides Nanoparticles via Sol-Gel Method: A Review on Synthesis, Characterization and Applications. J. Mater. Sci. Mater. Electron. 31 (5), 3729–3749. doi:10.1007/s10854-020-02994-8
Pradhan, P., Lüdeke, M. K. B., Reusser, D. E., and Kropp, J. P. (2013). Embodied Crop Calories in Animal Products. Environ. Res. Lett. 8 (4), 044044. doi:10.1088/1748-9326/8/4/044044
Prasad Yadav, T., Manohar Yadav, R., and Pratap Singh, D. (2012). Mechanical Milling: A Top Down Approach for the Synthesis of Nanomaterials and Nanocomposites. Nn 2 (3), 22–48. doi:10.5923/j.nn.20120203.01
Prasanna, P., Kumar, P., Kumar, S., Rajana, V. K., Kant, V., Prasad, S. R., et al. (2021). Current Status of Nanoscale Drug Delivery and the Future of Nano-Vaccine Development for Leishmaniasis - A Review. Biomed. Pharmacother. 141, 111920. doi:10.1016/j.biopha.2021.111920
Přibyl, J., Hepel, M., and Skládal, P. (2006). Piezoelectric Immunosensors for Polychlorinated Biphenyls Operating in Aqueous and Organic Phases. Sensors Actuators B: Chem. 113 (2), 900–910. doi:10.1016/j.snb.2005.03.077
Raja, I. S., Song, S.-J., Kang, M. S., Lee, Y. B., Kim, B., Hong, S. W., et al. (2019). Toxicity of Zero- and One-Dimensional Carbon Nanomaterials. Nanomaterials 9 (9), 1214. doi:10.3390/nano9091214
Rangasamy, K., Athiappan, M., Devarajan, N., Samykannu, G., Parray, J. A., Aruljothi, K. N., et al. (2018). Pesticide Degrading Natural Multidrug Resistance Bacterial Flora. Microb. Pathogenesis 114, 304–310. doi:10.1016/j.micpath.2017.12.013
Roh, J.-Y., Park, Y.-K., Park, K., and Choi, J. (2010). Ecotoxicological Investigation of CeO2 and TiO2 Nanoparticles on the Soil Nematode Caenorhabditis elegans Using Gene Expression, Growth, Fertility, and Survival as Endpoints. Environ. Toxicol. Pharmacol. 29 (2), 167–172. doi:10.1016/j.etap.2009.12.003
Rosell, J. R., and Sanz, R. (2012). A Review of Methods and Applications of the Geometric Characterization of Tree Crops in Agricultural Activities. Comput. Electronics Agric. 81, 124–141. doi:10.1016/j.compag.2011.09.007
Sabir, S., Arshad, M., and Chaudhari, S. K. (2014). Zinc Oxide Nanoparticles for Revolutionizing Agriculture: Synthesis and Applications. Scientific World J. 2014, 1–8. doi:10.1155/2014/925494
Saponaro, S., Sezenna, E., and Mastorgio, A. (2016). Pollutants in Groundwater. Filtration Mater. Groundwater: A Guide Good Pract. 1, 1–44.
Scognamiglio, V. (2013). Nanotechnology in Glucose Monitoring: Advances and Challenges in the Last 10 Years. Biosens. Bioelectron. 47, 12–25. doi:10.1016/j.bios.2013.02.043
Sekhon, B. (2014). Nanotechnology in Agri-Food Production: An Overview. Nsa 7, 31. doi:10.2147/NSA.S39406
Sharma, V. K., Yngard, R. A., and Lin, Y. (2009). Silver Nanoparticles: Green Synthesis and Their Antimicrobial Activities. Adv. Colloid Interf. Sci. 145 (1-2), 83–96. doi:10.1016/j.cis.2008.09.002
Shekhawat, K., Rathore, S. S., Premi, O. P., Kandpal, B. K., and Chauhan, J. S. (2012). Advances in Agronomic Management of Indian Mustard (Brassica juncea (L.) Czernj. Cosson): An Overview. Int. J. Agron. 2012, 1–14. doi:10.1155/2012/408284,
Singh, N., Gupta, V. K., Kumar, A., and Sharma, B. (2017). Synergistic Effects of Heavy Metals and Pesticides in Living Systems. Front. Chem. 5, 70. doi:10.3389/fchem.2017.00070
Sinha, S., Kim, H., and Robertson, A. W. (2021). Preparation and Application of 0D-2D Nanomaterial Hybrid Heterostructures for Energy Applications. Mater. Today Adv. 12, 100169. doi:10.1016/j.mtadv.2021.100169
Stadler, T., Buteler, M., and Weaver, D. K. (2010). Novel Use of Nanostructured Alumina as an Insecticide. Pest Manag. Sci. 66 (6), 577–579. doi:10.1002/ps.1915
Szabó, Z., Volk, J., Fülöp, E., Deák, A., and Bársony, I. (2013). Regular ZnO Nanopillar Arrays by Nanosphere Photolithography. Photon. Nanostructures - Fundamentals Appl. 11 (1), 1–7. doi:10.1016/j.photonics.2012.06.009
Tiwari, J. N., Tiwari, R. N., and Kim, K. S. (2012). Zero-Dimensional, One-Dimensional, Two-Dimensional and Three-Dimensional Nanostructured Materials for Advanced Electrochemical Energy Devices. Prog. Mater. Sci. 57 (4), 724–803. doi:10.1016/j.pmatsci.2011.08.003
Tomaszewska, E., Soliwoda, K., Kadziola, K., Tkacz-Szczesna, B., Celichowski, G., Cichomski, M., et al. (2013). Detection Limits of DLS and UV-Vis Spectroscopy in Characterization of Polydisperse Nanoparticles Colloids. J. Nanomater. 2013, 1–10. doi:10.1155/2013/313081
Torney, F., Trewyn, B. G., Lin, V. S.-Y., and Wang, K. (2007). Mesoporous Silica Nanoparticles Deliver DNA and Chemicals into Plants. Nat. Nanotech. 2 (5), 295–300. doi:10.1038/nnano.2007.108
Usman, M., Zaheer, Y., Younis, M. R., Demirdogen, R. E., Hussain, S. Z., Sarwar, Y., et al. (2020). The Effect of Surface Charge on Cellular Uptake and Inflammatory Behavior of Carbon Dots. Colloid Interf. Sci. Commun. 35, 100243. doi:10.1016/j.colcom.2020.100243
Vahabi, S., Nazemi Salman, B., and Javanmard, A. (2013). Atomic Force Microscopy Application in Biological Research: A Review Study. Iran J. Med. Sci. 38 (2), 76–83.
Wang, M., Li, Y., Yang, J., Shi, R., Xiong, L., and Sun, Q. (2021). Effects of Food-Grade Inorganic Nanoparticles on the Probiotic Properties of Lactobacillus Plantarum and Lactobacillus Fermentum. LWT 139, 110540. doi:10.1016/j.lwt.2020.110540
Wang, Z., Hu, T., Liang, R., and Wei, M. (2020). Application of Zero-Dimensional Nanomaterials in Biosensing. Front. Chem. 8, 320. doi:10.3389/fchem.2020.00320
Wilson, M. A., Tran, N. H., Milev, A. S., Kannangara, G. S. K., Volk, H., and Lu, G. Q. M. (2008). Nanomaterials in Soils. Geoderma 146 (1-2), 291–302. doi:10.1016/j.geoderma.2008.06.004
Wu, X., Song, Y., Yan, X., Zhu, C., Ma, Y., Du, D., et al. (2017). Carbon Quantum Dots as Fluorescence Resonance Energy Transfer Sensors for Organophosphate Pesticides Determination. Biosens. Bioelectron. 94, 292–297. doi:10.1016/j.bios.2017.03.010
Yan, S., Ren, B. Y., and Shen, J. (2021). Nanoparticle‐Mediated Double‐Stranded RNA Delivery System: A Promising Approach for Sustainable Pest Management. Insect Sci. 28 (1), 21–34. doi:10.1111/1744-7917.12822
Yang, L., and Watts, D. J. (2005). Particle Surface Characteristics May Play an Important Role in Phytotoxicity of Alumina Nanoparticles. Toxicol. Lett. 158 (2), 122–132. doi:10.1016/j.toxlet.2005.03.003
Yin, Y., Gates, B., and Xia, Y. (2000). A Soft Lithography Approach to the Fabrication of Nanostructures of Single Crystalline Silicon with Well-Defined Dimensions and Shapes. Adv. Mater. 12 (19), 1426–1430. doi:10.1002/1521-4095(200010)12:19<1426::AID-ADMA1426>3.0.CO;2-B
Youssef, A. M., and El-Sayed, S. M. (2018). Bionanocomposites Materials for Food Packaging Applications: Concepts and Future Outlook. Carbohydr. Polym. 193, 19–27. doi:10.1016/j.carbpol.2018.03.088
Zafar, S., Ashraf, A., Ashraf, M. Y., Asad, F., Perveen, S., Zafar, M. A., et al. (2018). Preparation of Eco-Friendly Antibacterial Silver Nanoparticles from Leaf Extract of Ficus Benjamina. Biomed. J. 1 (5), 1.
Zafar, S., Hasnain, Z., Aslam, N., Mumtaz, S., Jaafar, H. Z., Wahab, P. E. M., et al. (2021). Impact of Zn Nanoparticles Synthesized via green and Chemical Approach on Okra (Abelmoschus Esculentus L.) Growth Under Salt Stress. Sustainability 13 (7), 3694. doi:10.3390/su13073694
Zhang, D., Fang, Y., Miao, Z., Ma, M., Du, X., Takahashi, S., et al. (2013). Direct Electrodeposion of Reduced Graphene Oxide and Dendritic Copper Nanoclusters on Glassy Carbon Electrode for Electrochemical Detection of Nitrite. Electrochimica Acta 107, 656–663. doi:10.1016/j.electacta.2013.06.015
Zheng, L., Hong, F., Lu, S., and Liu, C. (2005). Effect of Nano-TiO2 on Strength of Naturally Aged Seeds and Growth of Spinach. Bter 104 (1), 083–092. doi:10.1385/BTER:104:1:083
Zhu, X., Tian, S., and Cai, Z. (2012). Toxicity Assessment of Iron Oxide Nanoparticles in Zebrafish (Danio rerio) Early Life Stages. PLoS ONE 7 (9), e46286. doi:10.1371/journal.pone.0046286
Keywords: nanosensors, nanofertilizer, nanopesticides, nanobionics, biosafety, biomass accumulation
Citation: Abbas M, Yan K, Li J, Zafar S, Hasnain Z, Aslam N, Iqbal N, Hussain SS, Usman M, Abbas M, Tahir M, Abbas S, Abbas SK, Qiulan H, Zhao X and El-Sappah AH (2022) Agri-Nanotechnology and Tree Nanobionics: Augmentation in Crop Yield, Biosafety, and Biomass Accumulation. Front. Bioeng. Biotechnol. 10:853045. doi: 10.3389/fbioe.2022.853045
Received: 12 January 2022; Accepted: 28 February 2022;
Published: 26 April 2022.
Edited by:
Gongke Zhou, Qingdao Agricultural University, ChinaReviewed by:
Madhuri Sharon, Walchand Center for Research in Naynotechnology and Bionanotechnology, IndiaSarfraz Shafiq, Western University, Canada
Copyright © 2022 Abbas, Yan, Li, Zafar, Hasnain, Aslam, Iqbal, Hussain, Usman, Abbas, Tahir, Abbas, Abbas, Qiulan, Zhao and El-Sappah. This is an open-access article distributed under the terms of the Creative Commons Attribution License (CC BY). The use, distribution or reproduction in other forums is permitted, provided the original author(s) and the copyright owner(s) are credited and that the original publication in this journal is cited, in accordance with accepted academic practice. No use, distribution or reproduction is permitted which does not comply with these terms.
*Correspondence: Sara Zafar, sarazafar@gcuf.edu.pk; Zuhair Hasnain, zuhair@uaar.edu.pk; Ahmed H. El-Sappah, ahmed_elsappah2006@yahoo.com
†These authors have contributed equally to this work and share first authorship