- 1Department of Plastic and Reconstructive Surgery, Shanghai Ninth People’s Hospital, Shanghai Jiao Tong University School of Medicine, Shanghai, China
- 2Department of Plastic Surgery, The Third Xiangya Hospital, Central South University, Changsha, China
- 3Department of Orthopaedics, Shanghai Ninth People’s Hospital, Shanghai Jiao Tong University School of Medicine, Shanghai, China
- 4Collage of Biological Science and Medical Engineering, Donghua University, Shanghai, China
Postoperative wound edema, infection, and pain burden the patient’s life. Therefore, the purpose of this study is to develop an effective antibacterial, multifunctional application to prevent postoperative edema and relieve postoperative pain by making full use of the dehydrating and analgesic effects of magnesium sulfate (MgSO4), magnesium oxide (MgO), sodium alginate (SA), and sodium carboxymethyl cellulose (Na-CMC) to make a composite hydrogel, which can promote postoperative detumescence. MgSO4//MgO/SA/Na-CMC composite hydrogel dressings have outstanding mechanical properties, high water absorption, and good biocompatibility. MgO endows the hydrogel dressing with excellent antibacterial properties and better antibacterial activity against common bacteria and multidrug-resistant bacteria. In addition, MgSO4/MgO/SA/Na-CMC hydrogel dressing shows superior dehydration and analgesic properties in the postoperative nude mice model. This study shows that the multifunctional MgSO4/MgO/SA/Na-CMC composite hydrogel dressing developed as a surgical incision dressing has broad prospects in the prevention of incision infection, postoperative edema, and analgesia.
Introduction
A postoperative incision is usually accompanied by complications such as edema and infection, which are significant problems for patients and medical staff (Maheshwer et al., 2021). Especially in plastic surgery, patients have obvious facial edema after the operation, which significantly affects their life and work. Effective incision management after the operation can not only reduce complications and human and material costs but also achieve rapid recovery and reduce unnecessary troubles to life and work caused by surgical complications (El Hosary et al., 2020).
Because of their soft texture and viscosity, hydrogels have been very popular with researchers in recent years (Singh et al., 2013; Bayer, 2021; Cheng et al., 2021; Guimaraes et al., 2021). Hydrogels are ideal dressings with biocompatibility. At the same time, it can be used and replaced according to the needs of patients. This not only reduces the inconvenience of repeated medical treatment after an operation but also reduces the workload of doctors. Because hydrogels are more commonly used as postoperative dressings, at present, many studies reported that hydrogel dressings can achieve bacteriostatic effects or provide the best pH value to promote wound healing (Cheng et al., 2021; Fan et al., 2021; Liang et al., 2021). At present, the clinical hydrogel material used for postoperative incision edema is “cold compress.” The principle of cold compress is only to promote vasoconstriction and reduce postoperative swelling. This solution has obvious limitations: first, only relying on physical cooling to reduce swelling has a limited effect; and second, the postoperative incision is still at risk of infection. Few dressings focus on the needs of plastic surgery for detumescence, anti-infection, and pain. To avoid postoperative edema and infection, the hydrogel dressings we studied should have good water absorption and antibacterial ability.
It is well known that oral magnesium sulfate (MgSO4) forms a hypertonic environment in the intestine and has the function of catharsis (Krenzelok et al., 1985). Magnesium oxide (MgO) also has an antibacterial effect (Muniz Diaz et al., 2021; Wang et al., 2021). Although some researchers have added antibiotics to the dressing to achieve antibacterial effect, it will lead to bacterial resistance (Fischbach and Walsh, 2009). We may solve this issue by directly including MgO, a material with antibacterial activity, into the dressing. At present, hydrogel technology based on sodium alginate (SA) and sodium carboxymethyl cellulose (Na-CMC) has been very mature, which can carry drugs for the treatment of related diseases (Sheng et al., 2021). Therefore, it is feasible for us to add MgSO4/MgO into hydrogels. In this dressing, we used MgSO4 with water absorption and MgO with antibacterial effect. According to the already mature SA/Na-CMC hydrogel preparation technology, combined with MgSO4 and MgO, a new dressing was prepared. This dressing had both absorptive and antibacterial hydrogel dressing and could accelerate wound detumescence after operation. It is very valuable in clinical application.
To this end, we mixed MgSO4, MgO, SA, and Na-CMC in a certain proportion to prepare hydrogels with high water absorption and antibacterial effects. We evaluated the appearance of MgSO4/MgO/SA/Na-CMC composite hydrogel dressings by gross characterization. The internal structure of the hydrogels was examined by scanning electron microscopy. The swelling ratio of the hydrogels was used to assess the water absorbency of hydrogel dressings. After HaCaT cells were co-cultured with the hydrogel extract for a period of time, the biocompatibility of the hydrogel dressing was evaluated by live/dead staining. The antibacterial activity of hydrogel dressings was tested by bacteriostasis experiments of Escherichia coli and Staphylococcus aureus. The surgical incision edema model was used to detect the swelling effect of hydrogels in vivo.
Materials and Methods
Materials
Sodium alginate (SA), magnesium sulfate (MgSO4), magnesium oxide (MgO), sodium carboxymethyl cellulose (Na-CMC), and glycerol were purchased from Shanghai Aladdin Biochemical Technology Co., Ltd.
Hydrogel Preparation
We used the dosage of materials listed in Table 1 and added each material to prepare the composite hydrogels. At room temperature, 1 ml glycerol was added to 9 ml double-distilled H2O (dd H2O). At 25°C, MgSO4 and MgO (MgSO4: MgO = 99:1 w/w) powders of different weights were separately added to the aforementioned solution and stirred for 30 min. After adding SA and Na-CMC successively, the solution was stirred in a 65°C constant temperature water bath for 6 h. The thicker liquid was added to the abrasives and placed in the 4°C refrigerator for preservation in subsequent experiments.
Morphological Assay
The appearance of these five groups of hydrogels was photographed with digital cameras. The prepared MgSO4/MgO/SA/Na-CMC hydrogel dressings were freeze-dried for 48 h at −80°C. The samples were cut with a small blade and placed on the scaffold. The internal morphology of the hydrogels was observed by scanning electron microscopy (SEM).
Mechanical Properties
The mechanical properties of these five groups of hydrogels were tested using the HY-940FS universal testing machine (Shanghai, China) at room temperature. The prepared MgSO4/MgO/SA/Na-CMC hydrogel dressings were compressed to 50% deformation under wet conditions. The strain–stress curve was obtained and the compression modulus was calculated.
Swelling Rate Determination
The purpose of the swelling test is to evaluate the ability of materials to absorb solvents. We used ddH2O as the experimental solvent. We calculated the swelling rate in the dry and wet states. First, the prepared hydrogel block (d = 1 cm) was freeze-dried at −80°C for 48 h. The weight of the freeze-dried sample (W0) was measured. Then, the gel was soaked in 25°C ddH2O and weighed once every 5 min. Before weighing, the excess water was removed from the sample surface with a filter paper, and the recorded value was Wt. This was continued until the weight remained constant. Second, the swelling capacity of wet hydrogels is measured. The back skin of mice was intercepted. The prepared hydrogels with a diameter of 1 cm were weighed and recorded as W0. Mice skin was placed on the cell strainer (biosharp, BS-100-CS), infiltrated in ddH2O, and five groups of hydrogels were placed on the top of each group, with three repeated experiments in each group. The weight Wt was recorded every 2 h until the weight was constant.
The swelling rate (SR) was calculated by the following formula:
Among them, (Wt) is the weight of the swelling hydrogel at the time (T) and (W0) is the initial weight of the sample. For each group of three samples, the average value and mean square error are calculated to minimize the error.
Biocompatibility
The synthesized epidermal cells (HaCaT cells) were cultivated in the hydrogel extract, and the state of the cells was determined using live/dead staining assay to assess the hydrogel’s biocompatibility. The HaCaT cells were inoculated in 24-well plates and incubated in a humidified incubator at 37°C and 5% CO2 for three days. The sterilized hydrogels were soaked in sterile PBS for 48 h. Then, 100 ul of the net extract soaked for 48 h was absorbed and co-cultured with 900 ul medium for 24 h. Then, 100 ul of the net extract soaked for 48 h was absorbed, and the cells were co-cultured with 900 ul medium for 48 h. The stained cells were observed under a fluorescence microscope. Living cells absorb calcein AM and emit green fluorescence, while PI enters dead cells and emits red fluorescence after binding with DNA.
Antibacterial Activity Evaluation
We used common Escherichia coli (ATCC 8739) and Staphylococcus aureus (ATCC 6538) to carry out the antibacterial activity on the surface of the hydrogel dressing. The hydrogel dressings were placed on an agar plate (petri dish) and incubated for 72 h at 37°C The diameter of the bacteriostasis circle on the agar plate (petri dish) was calculated. Each cycle was repeated three times and the average value was calculated.
Animals
Male BALB/c nude mice aged 6–8 weeks were purchased from Shanghai Jie SiJieLaboratory Animal Co., Ltd. All animals involved were treated according to the protocol evaluated and approved by the Ethics Committee of the Ninth People’s Hospital, affiliated to Shanghai Jiao Tong University School of Medicine. The mice were randomly divided into five groups with three mice in each group. The experiment was repeated with an intraperitoneal injection of anesthesia. Normal saline containing DAPI fluorescence staining was injected into the subcutaneous soft tissue. After soft tissue swelling of the extremities, the prepared hydrogel was clung to the skin surface. Hydrogels were extracted 4–6 h later. A fluorescent microscope was used to observe whether there was fluorescence in the hydrogel. By observing the swelling of the extremities in nude mice, we could see if the hydrogel was effective.
Statistical Analysis
All experiments had three groups of repeated control, and the mean ± SD data (n = 3) were expressed. The analysis was performed by ANOVA (one-way analysis of variance) test. Student’s t-test was used to compare the two groups. P
Results and Discussion
Characterization of Prepared Hydrogels
The formulations of these five groups of hydrogels (Ctrl, Mg10, Mg20, Mg30, and Mg40) are shown in Table 1. MgSO4/MgO/SA/Na-CMC hydrogels were successfully prepared with different MgSO4 ratios. The visual examination of hydrogels showed an opaque or translucent appearance, while pure hydrogels without Mg were white (Figures 1A–E). The hydrogels were homogenous and soft, with a diameter of 1 cm and a thickness of about 2–3 mm.
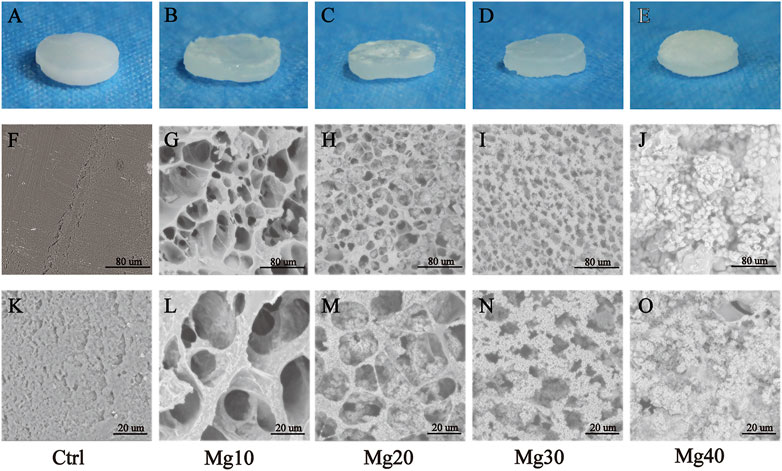
FIGURE 1. (A–E) The appearance of MgSO4/MgO/SA/Na-CMC composite hydrogel dressings. SEM images of the internal structure of hydrogels with low (F–J) and high (K–O) magnification.
Scanning electron microscopy (SEM) was used to examine the morphology of freeze-dried hydrogels. Figures 1F–O show the internal morphology of these five groups of MgSO4/MgO/SA/Na-CMC hydrogels. The results showed that the pure hydrogel material (Ctrl group) was unable to maintain the porous structure because of the shrinkage of the material during the freeze-drying process (Figures 1F,K), while the addition of MgSO4/MgO effectively maintained the porous structure of the hydrogel, which showed an interconnected and homogenous porous structure, and the pore size was about 20–100 μm (Figures 1G,L). However, with the increase of magnesium sulfate content, many magnesium sulfate crystals were evenly dispersed in the pores and the pore size was reduced to 20 μm approximately (Figures 1H,L–N). In Figures 1J, O the pore structures are almost invisible because most of them are filled with magnesium sulfate crystals. In Supplementary Figure S1, it is shown that the average pore size of groups Mg10, Mg20, and Mg30 was 34.30 ± 2.13 um, 21.32 ± 0.83 um, and 13.17 ± 0.39 um, respectively (Mean ± SEM). It indicated that the pore size decreased with the increase of the magnesium compound content of the hydrogel. Generally, a smaller pore size can improve the porosity of the hydrogel, enhancing its absorption. In addition, the mechanical properties of the five groups of hydrogels were tested and shown in Supplementary Figure S2. The compression modulus of the five groups was decreased with more magnesium compounds. This may be due to the increase of magnesium compounds, which improves its porosity but reduces its material density; hence, it reduces its mechanical properties. We found that the mechanical properties of the group Mg40 were the worst, which may limit its application as dressing.
The past ten years have witnessed the rapid development of hydrogels. The good biocompatibility and high expansion of hydrogels have attracted the attention of many researchers (Pooresmaeil et al., 2019; Silva et al., 2019). SA is water-soluble, can effectively absorb wound secretions, and keeps the wound clean and dry, which is conducive to wound healing. In addition, SA is low-cost and easy to obtain (Gao et al., 2020). At room temperature, calcium ions (Ca2+) were crosslinked with SA to form hydrogels to increase mechanical properties (Paudyal et al., 2013). Moreover, Ca2+ after crosslinking could help trigger the coagulation mechanism and promote hemostasis when they were released into the wound (Afjoul et al., 2020).
Swelling Ratio
The higher the SR value, the stronger the water absorbability of the hydrogels will be. This means that the hydrogen absorbs the exudate from the wound more strongly, thus achieving our goal of promoting incision detumescence. Figure 2A shows the percentage of swelling in the dry state containing different concentrations of Mg. The SR was sorted as Mg40 > Mg20 > Mg30 > Mg10 > Ctrl. All hydrogels showed swelling ability, and Mg40 or Mg20 exhibited a higher SR. As shown in Figure 2B, the percentage of swelling in the wet state containing different concentrations of Mg can be observed. The SR was sorted as Mg40 > Mg30 > Mg10 > Mg20 > Ctrl. The SR of the wet hydrogel was weaker than that of the dry state, but the swelling rate of Mg40 and Mg30 was still high. This was mainly due to the hypertonic effect of MgSO4, which was consistent with our expectations.
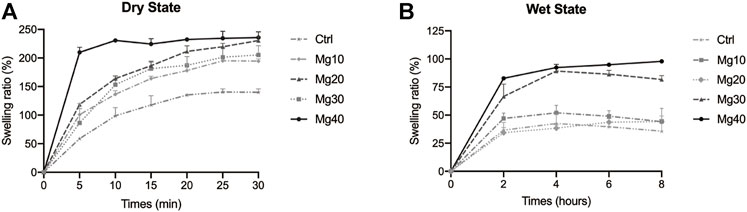
FIGURE 2. The swelling ratio of different MgSO4/MgO/SA/Na-CMC composite hydrogel dresssings in the dry state (A) and in the wet state (B).
MgSO4 had high water absorption and is often used for catharsis (Snyder et al., 2014). We used the hypertonic effect of magnesium sulfate to solve the problem of postoperative incision edema. To select the appropriate concentration of MgSO4, we set different MgSO4 concentration gradients. Based on a comparison of water absorption in different states, we concluded that the higher the concentration, the better the water absorption effect, which corresponded to the characteristics of MgSO4’s hyperosmotic action (Holland et al., 1960).
Biocompatibility
Biocompatibility can help us identify the safety of hydrogels. Five groups of hydrogel extracts were used to culture HaCaT cells for 24 and 48 h to observe the biocompatibility of the hydrogels. After 24 h, the live/dead staining assay showed that there were many living cells (Figure 3). After 48 h of culture, the live/dead staining assay showed that the Mg40 hydrogel contained more than 76% dead cells than other groups. The result of fluorescence staining showed that the hydrogel had good biocompatibility and could be used for biomedical purposes.
Because the hydrogel was closely related to the epidermis, we needed to detect whether it had good biocompatibility using live/death staining detection. Mg2+ is often used with biopharmaceuticals because of its good biocompatibility (Gao et al., 2021). As Mg2+ is a metal ion, it can inhibit cell proliferation (Blangero and Teissie, 1985; Zhen et al., 2015). With prolonged contact time, the contact inhibition effect of hydrogels gradually showed. According to the results of live/dead staining, we chose Mg30, Mg20, Mg10, and Ctrl groups.
Antibacterial Activity of the Hydrogels
Bacterial infection can seriously affect wound healing. The hydrogels prepared by us require anti-infection and antibacterial properties. We used two representative strains, Escherichia coli and Staphylococcus aureus, to test the antibacterial activity of hydrogels. The five groups of hydrogels were co-cultured with Escherichia coli and Staphylococcus aureus in a 37°C incubator. After 2 days of cultivation, the diameter of the inhibition zone was measured as follows: Mg40 > Mg30 > Mg20 > Mg10 > Ctrl (Figure 4). All of these proved that these hydrogels had good antibacterial activity, especially the Mg40 and Mg30 groups, showing great potential for antibacterial and anti-infection properties.
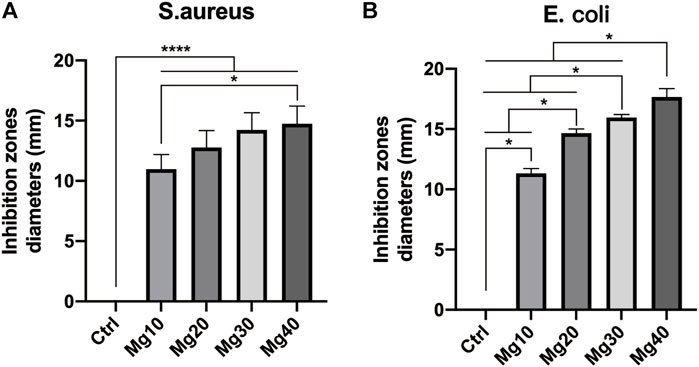
FIGURE 4. The antibacterial activity of MgSO4/MgO/SA/Na-CMC composite hydrogel dressings on Escherichia coli (ATCC 8739) and Staphylococcus aureus (ATCC 6538). P ≤ 0.05 was considered statistically significant. P ≤ 0.05 (*) P ≤ 0.0001(****).
Reducing the risk of infection or antibacterial effect is one of the important conditions for evaluating wound dressings. Metal ions (such as silver ions) were often used in various skin dressings to inhibit the growth of bacteria (Tao et al., 2021). However, the role of silver ions was limited and could not alleviate the problem of surgical incision edema. Therefore, we chose Mg2+. As mentioned earlier, MgSO4 had good water absorption. Moreover, some researchers have reported that Mg2+ has antibacterial properties (Zhou et al., 2021). MgO is easily hydrated and forms a layer of magnesium hydroxide on the surface. A high concentration of reactive oxygen ions can exist on the surface of magnesium oxide. Reactive oxygen species ions are characterized by strong oxidation, which can destroy the peptide bond structure of bacterial cell membrane walls and quickly kill bacteria (Ikram et al., 2021). Therefore, the hydrogels prepared by us also showed antibacterial effects through antibacterial experiments. It is the best choice of dressing raw materials for postoperative surgical incision.
Detumescence Effect of Hydrogels in an Edema Model
After constructing the edema model in nude mice, the detumescence effect of the hydrogel was detected by in vivo tests. A visual examination showed that the extremity of edema in nude mice was obvious, and the hydrogels adhered to the incision of nude mice and were removed after 6–8 h. Figure 5 shows the contrast between the incision before and after the use of hydrogels. Visual examination between the groups showed that the detumescence effect of the four treatment groups was satisfactory, and Mg40 and Mg30 groups were significantly better than the other groups. Our hydrogels had an obvious effect on detumescence. During the experiment, neither infection nor rejection was observed, demonstrating that the hydrogels were biocompatible. The hydrogel was cut longitudinally and observed under a fluorescence microscope. It can be seen that the fluorescent bands of Mg40 and Mg30 were the widest. The order of the fluorescence bands from wide to narrow is Mg40 > Mg30 > Mg20 > Mg10 > Ctrl (Figure 6). This displayed that most water absorption was observed in the Mg40 and Mg30 groups. This signified that these two groups in the hydrogels had a better detumescence effect.
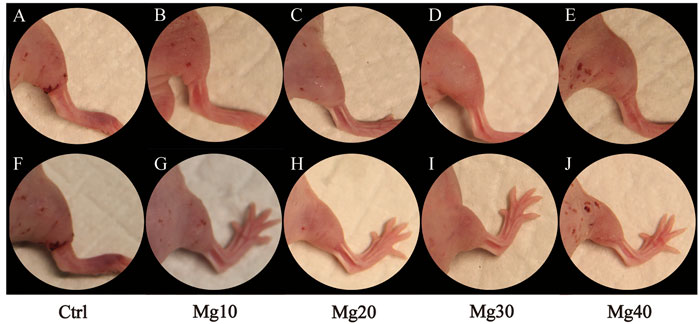
FIGURE 5. Hindlimb edema model in nude mice (A–E), and after treatment of MgSO4/MgO/SA/Na-CMC composite hydrogel dressings (F–J).
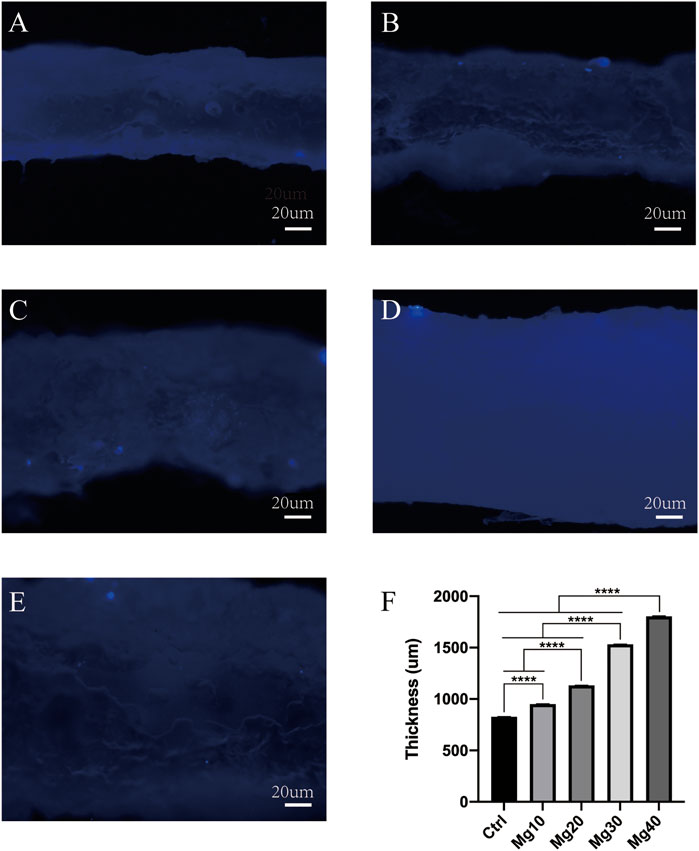
FIGURE 6. The water absorption of different groups (Ctrl, Mg10, Mg20, Mg30, Mg40) of MgSO4/MgO/SA/Na-CMC composite hydrogels under fluorescence microscope (A–E). P ≤ 0.0001 was considered statistically significant (****).
In the past decade, many studies were focused on the preparation of hydrogel dressing for wound healing and had achieved remarkable results (Cheng et al., 2021). These hydrogel dressings not only had antibacterial properties but also had many functions such as self-healing, hemostasis, and so on (Li et al., 2021). However, few researchers have noticed the impact of surgical incision edema on patients. We took full advantage of the hyperosmolar effect of MgSO4 and added it to the hydrogel scaffold to prepare MgSO4/MgO/SA/Na-CMC composite hydrogels. The model of postoperative incision edema in nude mice could show an obvious detumescence effect.
Conclusion
MgSO4, MgO, SA, and Na-CMC were chosen as the main components of the composite hydrogel dressings. These materials were mixed according to the proportion of each group listed in Table 1, and a group was selected with good biocompatibility, antibacterial activity, and detumescence effect. According to the SR, we screened Mg40 and Mg30 groups. Biocompatibility suggested that the cell mortality of Mg40 was higher than that of the other four groups, and mechanical test results indicated that Mg40 has a significantly worse compression modulus than the other four groups, so Mg40 was excluded. In the antibacterial activity, it could be seen that Mg40 and Mg30 groups had greater inhibition on Escherichia coli and Staphylococcus aureus. In animal experiments, except for the Ctrl group, Mg10, Mg20, Mg30, and Mg40 all had strong detumescence ability. In conclusion, the Mg30 group not only showed excellent antibacterial activity and detumescence effect but also had good biocompatibility. We successfully prepared MgSO4/MgO/SA/Na-CMC composite hydrogel dressings with high water absorbency and antibacterial properties.
Data Availability Statement
The data that support the findings of this study are available on request from the corresponding author.
Ethics Statement
The animal study was reviewed and approved by the Ethics Committee of the Ninth People’s Hospital affiliated to Shanghai Jiao Tong University School of Medicine. Written informed consent was obtained from the owners for the participation of their animals in this study.
Author Contributions
YF and BS performed the research. SW and BS designed the research study. JZ, YX, and SL contributed essential reagents or tools. XL, JC, and HL helped to analyze the data. YF and BS wrote the article. SW and SL revised the article. All authors read and approved the final manuscript.
Funding
This work was supported by a grant from the Natural Science Foundation of China (No. 82002065) and Postdoctoral Science Foundation (No. 2019T120347).
Conflict of Interest
The authors declare that the research was conducted in the absence of any commercial or financial relationships that could be construed as a potential conflict of interest.
Publisher’s Note
All claims expressed in this article are solely those of the authors and do not necessarily represent those of their affiliated organizations, or those of the publisher, the editors, and the reviewers. Any product that may be evaluated in this article, or claim that may be made by its manufacturer, is not guaranteed or endorsed by the publisher.
Acknowledgments
We thank all members of the Department of Plastic and Reconstructive Surgery and Department of Orthopaedics of Shanghai Ninth People’s Hospital, Shanghai Jiao Tong University School of Medicine.
Supplementary Material
The Supplementary Material for this article can be found online at: https://www.frontiersin.org/articles/10.3389/fbioe.2022.845345/full#supplementary-material
References
Afjoul, H., Shamloo, A., and Kamali, A. (2020). Freeze-gelled Alginate/gelatin Scaffolds for Wound Healing Applications: An In Vitro, In Vivo Study. Mater. Sci. Eng. C 113, 110957. doi:10.1016/j.msec.2020.110957
Bayer, I. S. (2021). A Review of Sustained Drug Release Studies from Nanofiber Hydrogels. Biomedicines 9 (11), 1612. doi:10.3390/biomedicines9111612
Blangero, C., and Teissié, J. (1985). Ionic Modulation of Electrically Induced Fusion of Mammalian Cells. J. Membrain Biol. 86 (3), 247–253. doi:10.1007/BF01870604
Cheng, H., Shi, Z., Yue, K., Huang, X., Xu, Y., and Gao, C. (2021). Sprayable Hydrogel Dressing Accelerates Wound Healing with Combined Reactive Oxygen Species-Scavenging and Antibacterial Abilities. Acta Biomater. 124, 219–232. doi:10.1016/j.actbio.2021.02.002
El Hosary, R., El-Mancy, S. M. S., El Deeb, K. S., Eid, H. H., El Tantawy, M. E., Shams, M. M., et al. (2020). Efficient Wound Healing Composite Hydrogel Using Egyptian Avena Sativa L. Polysaccharide Containing Beta-Glucan. Int. J. Biol. Macromol 149, 1331–1338. doi:10.1016/j.ijbiomac.2019.11.046
Fan, L., He, Z., Peng, X., Xie, J., Su, F., Wei, D. X., et al. (2021). Injectable, Intrinsically Antibacterial Conductive Hydrogels with Self-Healing and pH Stimulus Responsiveness for Epidermal Sensors and Wound Healing. ACS Appl. Mater. Inter. 13 (45), 53541–53552. doi:10.1021/acsami.1c14216
Fischbach, M. A., and Walsh, C. T. (2009). Antibiotics for Emerging Pathogens. Science 325 (5944), 1089–1093. doi:10.1126/science.1176667
Gao, J., Su, Y., and Qin, Y. X. (2021). Calcium Phosphate Coatings Enhance Biocompatibility and Degradation Resistance of Magnesium alloy: Correlating In Vitro and In Vivo Studies. Bioact Mater. 6 (5), 1223–1229. doi:10.1016/j.bioactmat.2020.10.024
Gao, X., Guo, C., Hao, J., Zhao, Z., Long, H., and Li, M. (2020). Adsorption of Heavy Metal Ions by Sodium Alginate Based Adsorbent-A Review and New Perspectives. Int. J. Biol. Macromol 164, 4423–4434. doi:10.1016/j.ijbiomac.2020.09.046
Guimaraes, C. F., Ahmed, R., Marques, A. P., Reis, R. L., and Demirci, U. (2021). Engineering Hydrogel-Based Biomedical Photonics: Design, Fabrication, and Applications. Adv. Mater. 33 (23), e2006582. doi:10.1002/adma.202006582
Holland, J. J., Hoyer, B. H., Mc, L. L., and Syverton, J. T. (1960). Enteroviral Ribonucleic Acid. I. Recovery from Virus and Assimilation by Cells. J. Exp. Med. 112, 821–839. doi:10.1084/jem.112.5.821
Ikram, M., Inayat, T., Haider, A., Ul-Hamid, A., Haider, J., Nabgan, W., et al. (2021). Graphene Oxide-Doped MgO Nanostructures for Highly Efficient Dye Degradation and Bactericidal Action. Nanoscale Res. Lett. 16 (1), 56. doi:10.1186/s11671-021-03516-z
Krenzelok, E. P., Keller, R., and Stewart, R. D. (1985). Gastrointestinal Transit Times of Cathartics Combined with Charcoal. Ann. Emerg. Med. 14 (12), 1152–1155. doi:10.1016/s0196-0644(85)81019-2
Li, H., Cheng, F., Wei, X., Yi, X., Tang, S., Wang, Z., et al. (2021). Injectable, Self-Healing, Antibacterial, and Hemostatic N,O-carboxymethyl Chitosan/oxidized Chondroitin Sulfate Composite Hydrogel for Wound Dressing. Mater. Sci. Eng. C Mater. Biol. Appl. 118, 111324. doi:10.1016/j.msec.2020.111324
Liang, Y., Li, Z., Huang, Y., Yu, R., and Guo, B. (2021). Dual-Dynamic-Bond Cross-Linked Antibacterial Adhesive Hydrogel Sealants with On-Demand Removability for Post-Wound-Closure and Infected Wound Healing. ACS Nano 15 (4), 7078–7093. doi:10.1021/acsnano.1c00204
Maheshwer, B., Drager, J., John, N. S., Williams, B. T., LaPrade, R. F., and Chahla, J. (2021). Incidence of Intraoperative and Postoperative Complications after Posterolateral Corner Reconstruction or Repair: A Systematic Review of the Current Literature. Am. J. Sports Med. 49 (12), 3443–3452. doi:10.1177/0363546520981697
Muniz Diaz, R., Cardoso-Avila, P. E., Perez Tavares, J. A., Patakfalvi, R., Villa Cruz, V., Perez Ladron de Guevara, H., et al. (2021). Two-Step Triethylamine-Based Synthesis of MgO Nanoparticles and Their Antibacterial Effect against Pathogenic Bacteria. Nanomaterials (Basel) 11 (2). doi:10.3390/nano11020410
Paudyal, H., Pangeni, B., Inoue, K., Kawakita, H., Ohto, K., Ghimire, K. N., et al. (2013). Preparation of Novel Alginate Based Anion Exchanger from Ulva Japonica and its Application for the Removal of Trace Concentrations of Fluoride from Water. Bioresour. Technol. 148, 221–227. doi:10.1016/j.biortech.2013.08.116
Pooresmaeil, M., Behzadi Nia, S., and Namazi, H. (2019). Green Encapsulation of LDH(Zn/Al)-5-Fu with Carboxymethyl Cellulose Biopolymer; New Nanovehicle for Oral Colorectal Cancer Treatment. Int. J. Biol. Macromol 139, 994–1001. doi:10.1016/j.ijbiomac.2019.08.060
Sheng, Y., Gao, J., Yin, Z. Z., Kang, J., and Kong, Y. (2021). Dual-drug Delivery System Based on the Hydrogels of Alginate and Sodium Carboxymethyl Cellulose for Colorectal Cancer Treatment. Carbohydr. Polym. 269, 118325. doi:10.1016/j.carbpol.2021.118325
Silva, K., de Carvalho, D. E. L., Valente, V. M. M., Campos Rubio, J. C., Faria, P. E., and Silva-Caldeira, P. P. (2019). Concomitant and Controlled Release of Furazolidone and Bismuth(III) Incorporated in a Cross-Linked Sodium Alginate-Carboxymethyl Cellulose Hydrogel. Int. J. Biol. Macromol 126, 359–366. doi:10.1016/j.ijbiomac.2018.12.136
Singh, B., Sharma, S., and Dhiman, A. (2013). Design of Antibiotic Containing Hydrogel Wound Dressings: Biomedical Properties and Histological Study of Wound Healing. Int. J. Pharm. 457 (1), 82–91. doi:10.1016/j.ijpharm.2013.09.028
Snyder, A., Koeller, G., Seiwert, B., Abraham, G., and Schusser, G. F. (2014). Influence of Laxatives on Gastric Emptying in Healthy Warmblood Horses Evaluated with the Acetaminophen Absorption Test. Berl Munch Tierarztl Wochenschr 127 (3-4), 170–175.
Tao, G., Cai, R., Wang, Y., Zuo, H., and He, H. (2021). Fabrication of Antibacterial Sericin Based Hydrogel as an Injectable and Mouldable Wound Dressing. Mater. Sci. Eng. C Mater. Biol. Appl. 119, 111597. doi:10.1016/j.msec.2020.111597
Wang, B., Wu, Z., Wang, S., Wang, S., Niu, Q., Wu, Y., et al. (2021). Mg/Cu-doped TiO2 Nanotube Array: A Novel Dual-Function System with Self-Antibacterial Activity and Excellent Cell Compatibility. Mater. Sci. Eng. C Mater. Biol. Appl. 128, 112322. doi:10.1016/j.msec.2021.112322
Zhen, Z., Liu, X., Huang, T., Xi, T., and Zheng, Y. (2015). Hemolysis and Cytotoxicity Mechanisms of Biodegradable Magnesium and its Alloys. Mater. Sci. Eng. C Mater. Biol. Appl. 46, 202–206. doi:10.1016/j.msec.2014.08.038
Zhou, W., Yan, J., Li, Y., Wang, L., Jing, L., Li, M., et al. (2021). Based on the Synergistic Effect of Mg(2+) and Antibacterial Peptides to Improve the Corrosion Resistance, Antibacterial Ability and Osteogenic Activity of Magnesium-Based Degradable Metals. Biomater. Sci. 9 (3), 807–825. doi:10.1039/d0bm01584a
Keywords: hydrogel, detumescence, antibacterial, MgSO4, MgO
Citation: Fang Y, Li H, Chen J, Xiong Y, Li X, Zhou J, Li S, Wang S and Sun B (2022) Highly Water-Absorptive and Antibacterial Hydrogel Dressings for Rapid Postoperative Detumescence. Front. Bioeng. Biotechnol. 10:845345. doi: 10.3389/fbioe.2022.845345
Received: 29 December 2021; Accepted: 23 March 2022;
Published: 13 May 2022.
Edited by:
Sushila Maharjan, Brigham and Women’s Hospital and Harvard Medical School, United StatesReviewed by:
Mojgan Heydari, Materials and Energy Research Center, IranKyung Min Park, Incheon National University, South Korea
Ilker Bayer, Italian Institute of Technology (IIT), Italy
Copyright © 2022 Fang, Li, Chen, Xiong, Li, Zhou, Li, Wang and Sun. This is an open-access article distributed under the terms of the Creative Commons Attribution License (CC BY). The use, distribution or reproduction in other forums is permitted, provided the original author(s) and the copyright owner(s) are credited and that the original publication in this journal is cited, in accordance with accepted academic practice. No use, distribution or reproduction is permitted which does not comply with these terms.
*Correspondence: Binbin Sun, c3VuYmluYmluMTk5MkAxNjMuY29t; Shoubao Wang, d3hsZHJhZ29uMTk4NDE4QDE2My5jb20=