- 1Department of Biomedical Engineering, University of Memphis, Memphis, TN, United States
- 2Department of Biomedical Engineering, University of Massachusetts Amherst, Amherst, MA, United States
- 3Department of Small Animal Clinical Sciences, Michigan State University, East Lansing, MI, United States
- 4Orthopaedic Biomechanics Laboratories, College of Osteopathic Medicine, Michigan State University, East Lansing, MI, United States
Post-traumatic osteoarthritis (PTOA) is a debilitating disease that is a result of a breakdown of knee joint tissues following traumatic impact. The interplay of how these tissues influence each other has received little attention because of complex interactions. This study was designed to correlate the degeneration of the menisci, cartilage and subchondral bone following an acute traumatic event that resulted in anterior cruciate ligament (ACL) and medial meniscus tears. We used a well-defined impact injury animal model that ruptures the ACL and tears the menisci. Subsequently, the knee joints underwent ACL reconstruction and morphological analyses were performed on the menisci, cartilage and subchondral bone at 1-, 3- and 6-months following injury. The results showed that the morphological scores of the medial and lateral menisci worsened with time, as did the tibial plateau and femoral condyle articular cartilage scores. The medial meniscus was significantly correlated to the medial tibial subchondral bone at 1 month (p = 0.01), and to the medial tibial cartilage at 3 months (p = 0.04). There was only one significant correlation in the lateral hemijoint, i.e., the lateral tibial cartilage to the lateral tibial subchondral bone at 6 months (p = 0.05). These data may suggest that, following trauma, the observed medial meniscal damage should be treated acutely by means other than a full or partial meniscectomy, since that procedure may have been the primary cause of degenerative changes in the underlying cartilage and subchondral bone. In addition to potentially treating meniscal damage differently, improvements could be made in optimizing treatment of acute knee trauma.
Introduction
Menisci are fibrocartilaginous tissues in the knee joint that aid in load distribution and act to protect the underlying articular cartilage from excessive joint loads. Menisci are frequently torn either in isolated injuries or in combination with damage to the anterior cruciate ligament (ACL). It is well documented that following traumatic damage to the ACL and meniscus, 78–83% of patients will develop post-traumatic osteoarthritis (PTOA) 10–14 years post-injury, regardless of treatment modality (surgical reconstruction or conservative interventions) (Fink et al., 2001; von Porat et al., 2004). More recently, clinical studies have indicated that PTOA can develop as early as 3 years post-injury (Whittaker et al., 2015; Wang et al., 2020).
Knee PTOA is a disease that affects all tissues within the joint including menisci, articular cartilage, subchondral bone, synovium, fat pad and ligaments. The interplay of these tissues and how they affect each other is difficult to study and hence has received limited attention to date. In the Framingham OA study of 913 knees, meniscal damage in a given location was associated with higher regional tibial subchondral bone mineral density (BMD) (Lo et al., 2008). Human clinical studies have shown that there is a strong association between meniscal damage and cartilage loss (Hunter et al., 2006; Sharma et al., 2008), with less meniscal coverage and lower meniscal height increasing the risk of cartilage loss. Few studies, however, have analyzed all three major tissues in the joint, i.e., menisci, cartilage and subchondral bone, and investigated how the degree of degradation correlates with the development of osteoarthritis in the joint.
We have developed a closed-joint impact injury animal model that mimics the clinical development of PTOA following a traumatic insult. This model has been used to document the natural history of PTOA following closed-joint trauma (Fischenich et al., 2015; Pauly et al., 2015; Fischenich et al., 2017a). This model demonstrates degradative changes in the material properties of the menisci, articular cartilage and bone. These changes in our model include increased cartilage fissuring, decreased glycosaminoglycan content in both the cartilage and meniscus, as well as decreases in bone volume (BV) and BMD. However, the previous studies using our model did not attempt to correlate the degradation of the menisci, cartilage and subchondral bone to each other. More recently, our closed-joint impact injury model was combined with surgical reconstruction of the ACL (Narez et al., 2021a; Wei et al., 2021; Wei et al., 2022). Despite reconstruction of the ACL, decreases in the material properties of the menisci and the articular cartilage were still present, but minimal changes were documented in subchondral bone quality and morphometry. Again, there was no attempt to correlate the changes in the subchondral bone to those in the menisci or articular cartilage.
The goals of the current study were to document the degree of damage to menisci, cartilage and subchondral bone in a closed-joint impact injury animal model with ACL surgical reconstruction and determine if there was a potential correlation between the degree of damage in these joint tissues. It was hypothesized there would be a correlation between the level of meniscal degeneration with both the underlying cartilage and the subchondral bone within a given hemijoint.
Methods
All animal procedures for this study were approved by the Institutional Animal Care and Use Committee at Michigan State University (IACUC #05/16-073–00 and #PROTO201900255). Eighteen skeletally mature Flemish Giant rabbits (6.34 ± 0.75 kg, aged 6–9 months) were held in individual cages (32 × 32 × 28 in). The animals, a mix of male (n = 10) and female (n = 8), were anesthetized using standard protocols including 2% isoflurane in oxygen. The right hindlimb of each rabbit was shaved to expose the knee joint. The animals were then positioned into a rigid custom fixture in a servo-hydraulic testing system (Instron Corp, Norwood, MA) (Figure 1) (Isaac et al., 2010a; Fischenich et al., 2015; Pauly et al., 2015; Fischenich et al., 2017a; Fischenich et al., 2017b; Narez et al., 2021a; Wei et al., 2021; Wei et al., 2022). Using controlled impact with a 400 N preload, the actuator compressed the tibia downward 3.5 mm. This motion resulted in an anterior drawer of the knee where the tibia moved anteriorly with respect to the femur, causing ACL rupture. An auto-stop program was developed and used so that the test stopped automatically when there was an approximately 330 N load drop within 30 ms, which was indicative of a soft tissue (ACL) failure. ACL failure was confirmed by a board-certified veterinary surgeon (LMD) via a manual anterior drawer test of the joint. Force, time and displacement data were collected at 5 kHz. The left knee served as a contralateral control. We have previously shown that this impact injury animal model more closely mimics the clinical scenario following ACL rupture than the commonly used ACL surgical transection model, exhibiting many characteristics seen in human PTOA patients (Fischenich et al., 2017b). These animals were part of a much larger study with a total of 96 rabbits that investigated the efficacy of a surgical intervention and a pharmaceutical intervention in mitigating post-traumatic osteoarthritis (Narez et al., 2021a; Narez et al., 2021b; Wei et al., 2021; Narez et al., 2022; Wei et al., 2022). An animal model was necessary to recreate a true ACL/meniscus longitudinal injury study. Animals smaller than a rabbit often develop a calcified meniscus at an early age, hence the choice of the lapine model. The minimum number of animals was used such that a reasonable power (80%) was still obtained. No inhumane procedures were applied to the animals and they all experienced regular gait within 3 days after the intervention. Hence, this animal study fits the 3R’s principle (Hubrecht and Carter, 2019).
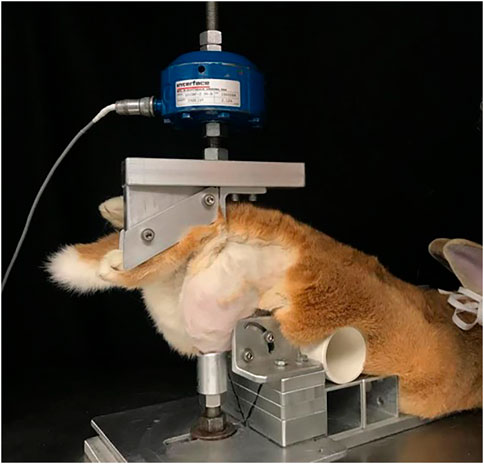
FIGURE 1. Injurious impact of rabbit knee set-up. In prone position (belly down) the rabbit’s hind paw was supported directly below the actuator of a servo-hydraulic machine. The knee was supported to prevent lateral and medial motion (with permission from Narez et al., 2021a).
Approximately 2 weeks post-impact, a board-certified veterinary surgeon (LMD), performed a reconstruction of the torn ACL (Wei et al., 2021). Through a medial parapatellar arthrotomy, the condition of the ACL and meniscus was examined in more detail. If meniscal damage was evident, it was noted and treated with a partial or full meniscectomy. For a partial meniscectomy, only the injured or torn regions were removed. For extensive damage to the meniscus, a full meniscectomy was performed where the entire damaged medial meniscus was removed. Following meniscal debridement, to repair the torn ACL, the musculotendinous junction of the semitendinosus tendon (ST) was transected leaving the tibial attachment intact. At the ACL footprint, a 2.7 mm diameter tibial bone tunnel and a 3.2 mm diameter femoral tunnel were drilled. The ST free end was passed under the medial collateral ligament and through the tunnels via a suture loop. Tension was applied to the ST as it was secured to the femoral bone tunnel with a custom PEEK interference fit screw. To ensure the joint’s stability, post-reconstruction, an anterior drawer test was performed on the reconstructed knee. Postoperatively, the animals were monitored by a licensed veterinary nurse and randomly assigned to one of 3 groups. Rabbits were euthanized at 1-, 3- or 6-months post-trauma, with n = 6 in each time-point group. These timepoints were chosen to correspond to what others have done in the literature studying PTOA (Coleman et al., 2018; Lisee et al., 2021). Based on our previous studies using this animal model, and evaluation of mechanical properties of the cartilage and meniscus, an n = 6 in each group provides 80% power in the study (Narez et al., 2021a; Wei et al., 2022).
Morphology Analysis
Meniscus
Following euthanasia, morphology grading of meniscal tissues was conducted by four separate blinded graders using a scoring system used by previous studies (Matyas et al., 2004; Pauli et al., 2011; Fischenich et al., 2014; Narez et al., 2021a). Each region of the meniscus (anterior, central and posterior) was scored. However, due to the fact that some knees had partial or full meniscectomies, regions were averaged to create one score for the lateral meniscus and one score for the medial meniscus. For both menisci, a score of 0 indicated normal tissue, a score of 1 indicated surface fibrillation, a score of 2 indicated un-displaced tears, a score of 3 indicated displaced tears, and a score of 4 indicated tissue maceration (Figure 2).
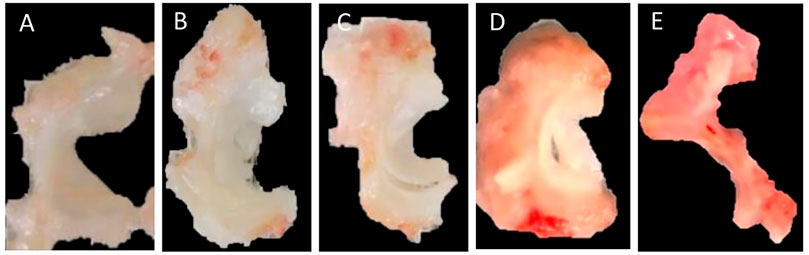
FIGURE 2. Morphological scoring of the meniscus. (A) 0 = normal, (B) 1 = surface fibrillation, (C) 2 = undisplaced tears, (D) 3 = displaced tears, (E) 4 = tissue maceration.
Cartilage Morphological Assessment
Morphology of the articular cartilage was assessed within 2 h following euthanasia. The surfaces of the tibial plateau and femoral condyles were stained with India ink to highlight surface fissures and other tissue irregularities. A blind morphological assessment (Yoshioka et al., 1996) was conducted by three independent graders with the following numerical grading scale: 1 = intact cartilage with the surface appearing normal, 2 = a few surface lesions that retained ink, 3 = moderate fibrillation retaining intense black patches of ink and 4 = full thickness erosion exposing underlying subchondral bone (Figure 3).
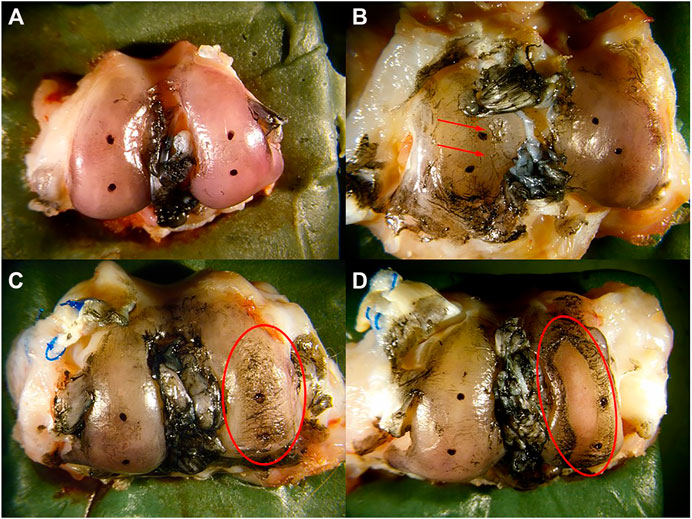
FIGURE 3. Morphological scoring of cartilage. (A) intact cartilage with the surface appearing normal was assigned a score of 1, (B) a few surface lesions (red arrows) that retain ink was assigned a score of 2, (C) moderate fibrillation retaining intense black patches of ink (red circle) was assigned a score of 3, and (D) full thickness erosion exposing underlying bone (red circle) was assigned a score of 4.
Subchondral Bone
Following euthanasia, bones were placed into a 10% neutral-buffered formalin solution. The limbs were decalcified in a 10% formic acid solution and sectioned to isolate regions of interest. The femoral condyles were sectioned along the parasagittal plane, passing through the medial and lateral condyles in a crani-caudal orientation, while the tibia was sectioned in the frontal plane, just anterior to the midline of the tibial plateau. Samples were processed, paraffin-embedded and sectioned at 7 μm. Sections were deparaffinized and stained with Hematoxylin, Saf-O and FG. Slides were imaged with a bright field microscope (Leica Microsystems Inc., Buffalo Grove, IL) and an Olympus DP25 camera (Olympus, Center Valley, PA). Three blind graders evaluated the subchondral bone morphology, using an OARSI score. Scores were reported and averaged before statistical analysis occurred. Scoring: 0 = normal, 1 = small spaces <50% of the length of condyle or plateau, 2 = Moderate spaces/some splits <50% of the length, 3 = moderate spaces/splits >50% of the length, 4 = numerous splits <50% of the length, 5 = numerous splits >50% of the length (Gerwin et al., 2010) (Figure 4).
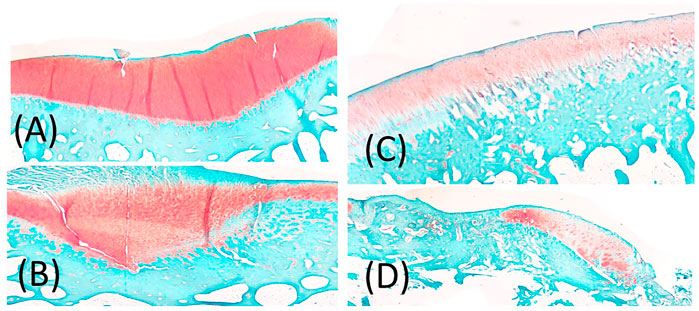
FIGURE 4. (A) representation of Score 1 = small spaces <50% of the length of condyle or plateau, (B) representation of 2 or 3 score depending on 2 = Moderate spaces/some splits <50% of the length, 3 = moderate spaces/splits >50% of the length, (C) 4 = numerous splits <50% of the length, (D) 5 = numerous splits >50% of the length.
Statistical Analysis
Comparisons were only made within a hemijoint, not across hemijoints. Spearman Rank correlations were made between a) medial meniscus and medial cartilage of the tibial plateau, b) medial meniscus and medial cartilage of the femoral condyle, c) medial meniscus and medial tibial plateau subchondral bone, d) medial meniscus and medial femoral condyle subchondral bone, e) medial cartilage of the tibia and the medial tibial plateau subchondral bone, f) medial femoral cartilage and subchondral bone, g) lateral meniscus and lateral cartilage of the tibial plateau, h) lateral meniscus and lateral cartilage of the femoral condyle, i) lateral meniscus and the lateral tibial plateau subchondral bone, j) lateral meniscus and the lateral femoral condyle subchondral bone, k) lateral tibial plateau cartilage and subchondral bone, l) lateral femoral condyle cartilage and subchondral bone, m) lateral tibial cartilage and lateral femoral cartilage, and n) medial tibial cartilage and medial femoral cartilage. These correlations were made at 1, 3 and 6 months for a total of 42 correlations. Statistical significance was set as p-value <0.05.
Results
All animals experienced ACL failure and were reconstructed. Of the 18 rabbits enrolled in the study, three rabbits had a full medial meniscectomy and 7 received a partial medial meniscectomy based on the level of damage observed in the medial meniscus. None of the animals had lateral meniscal damage that was noted during surgical reconstruction of the joint. The average morphological scores for the medial and lateral hemijoints, meniscus, femoral and tibial cartilage as well as tibial plateau and femoral condyle subchondral bone are presented in Table 1. Both the medial and lateral menisci tended to worsen with time, and the medial scores were higher than lateral scores. It is important to note that the lateral menisci had no indication of damage during reconstructive surgery, so the damage that developed to raise the scores over 6 months developed after reconstructive surgery. A similar trend was seen for the medial and lateral, tibial plateau and femoral condyle cartilage. Scores worsened with time, with 6 months scores being the highest. The subchondral bone of the tibial plateau and femoral condyles did not worsen with time and tended to show no consistent trends.
Of the 42 possible correlations, only 4 were significant (Table 2). The medial meniscus was significantly correlated to the medial tibial subchondral bone at 1 month (p = 0.01) and the medial meniscus was significantly correlated to the medial tibial cartilage at 3 months (p = 0.04). There was only one significant correlation in the lateral hemijoint, the lateral tibial cartilage to the lateral tibial subchondral bone at 6 months (p = 0.05). The medial tibial cartilage significantly correlated to medial femoral cartilage at 6 months.
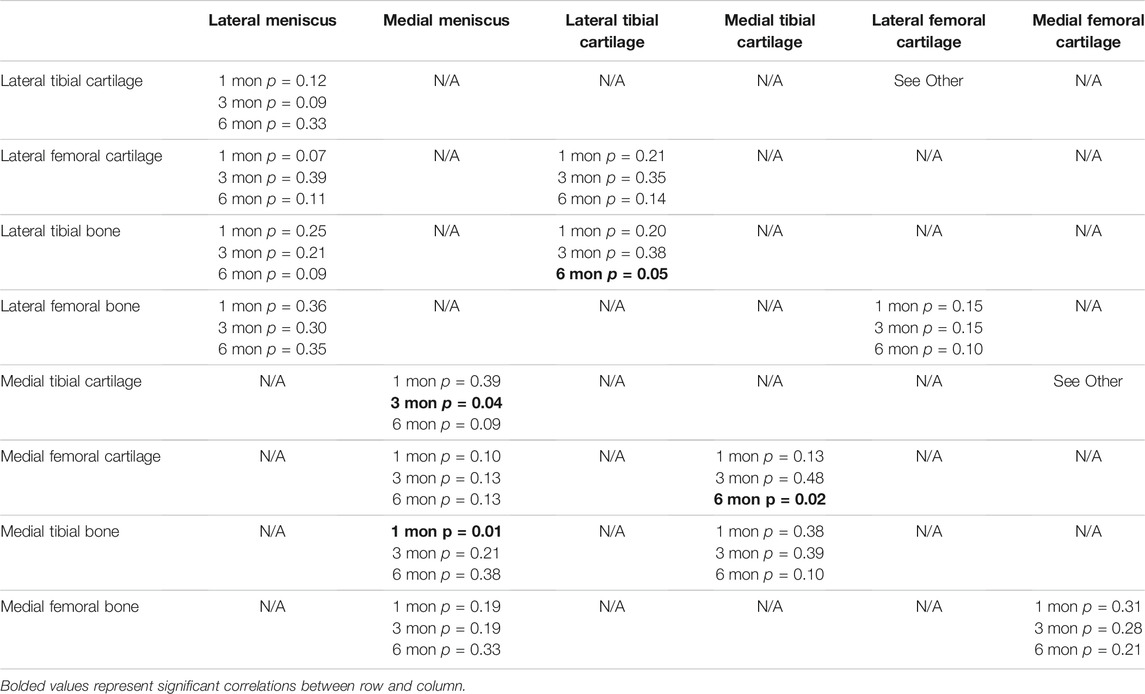
TABLE 2. Statistical values for all correlations between menisci, cartilage and subchondral bone for all comparisons at 1 month (mon), 3 and 6 months.
Discussion
The study showed that significant correlations occurred between 1) the tibial plateau subchondral bone and cartilage in the lateral hemijoint, and 2) between the meniscus and tibial cartilage in the medial hemijoint, 3) between the meniscus and tibial subchondral bone in the medial hemijoint, and 4) between femoral and tibial cartilage in the medial hemijoint. There were no significant correlations between the femoral condyle cartilage and femoral subchondral bone. Hence, in the medial hemijoint, when the meniscus was damaged, the cartilage and subchondral bone were also significantly damaged as early as 1 month. This finding confirms that the menisci likely play a significant role in protecting the cartilage and bone (Fox et al., 2012; Abusara et al., 2018). However, in the lateral compartment, where no meniscal damage was noted during reconstructive surgery, no strong association between the meniscus and either the articular cartilage and underlying subchondral bone damage was noted. Interestingly, at later time points (6 months), the lateral tibial cartilage and tibial plateau bone were correlated. While there was no significant damage acutely to the lateral meniscus, morphological score worsened with time. We hypothesize that perhaps there was either a change in joint kinematics following reconstruction that affected the lateral compartment, or the medial meniscus damage changed the loading in the lateral hemijoint resulting in increased damage. Future studies will need to further investigate possible causes for this result.
Few studies have previously attempted to document correlations between the integrity of the three tissues studied here; meniscus, cartilage and subchondral bone. One previous study, using magnetic resonance imaging did show that meniscal extrusion predicts increases in subchondral bone lesions, expansion of subchondral bone and bone cysts, and is associated with decreases in tibial articular cartilage volume as well as increases in tibial plateau area (Wang et al., 2010). That study, however, did not report the degree of degeneration of the main body of the meniscus, only that it was extruded. Meniscal extrusion has also been associated with the development of osteophytes (Miller et al., 1997; Lerer et al., 2004). While these studies are interesting, they do not report the cause of meniscal extrusion, whether it be due to meniscal attachment laxity or degenerative changes in the main body of the meniscus.
Previous studies using the same animals as in this current study documented changes in the histological and biomechanical behavior of the cartilage, meniscus and bone (Narez et al., 2021a; Wei et al., 2022). Compared to their contralateral controls, reconstructed limbs showed osteoarthritic changes to both the lateral and the medial menisci, articular cartilage and subchondral bone as early as 1-month post-trauma. The degeneration progressed in all tissues over time up to 6-months. Overall, the medial compartments had more tissue damage than their corresponding lateral counterparts. The damage that was present in these rabbits mimics clinical observations of patients suffering ACL injury and undergoing reconstruction (Felson, 2004; Goldring and Goldring, 2016). There were overall decreases in the cartilage fiber modulus and matrix modulus, and an increase in the cartilage tissue permeability when comparing the reconstructed to the control limbs. Decreases in both the instantaneous and equilibrium modulus were documented in both menisci. Minimal changes were found in bone quality and morphometry; however, bones from the reconstructed limbs showed large volumes of osteophyte formations, with an increase in volume over time. (Narez et al., 2021a; Wei et al., 2022). The reconstructed limbs demonstrated increases in cartilage fissuring and subchondral bone spaces or splits, as well as decreases in cartilage glycosaminoglycan (GAG) staining and tidemark integrity, therefore generally exhibiting more tissue damage than their contralateral control limbs. The analyses of the subchondral bone thickness (SCBT) showed a general trend of thickening of the underlying bone following ACL injury and reconstruction at all three time-points (except for the lateral compartments of the femur and tibia at 6-months), with a significant increase of the SCBT in the medial compartment of the tibia at 6-months post-trauma. GAG coverage analyses found a significant decrease in coverage of the medial meniscus at the 1- month time point. In the lateral meniscus, there was a documented decrease in coverage at both 3 and 6-months. Cortical and trabecular microstructure worsened in the reconstructed limbs with time compared to contralateral limbs. These data indicate that reconstruction alone does not prevent osteoarthritic changes. Taken together with the data from this study correlating the cartilage, menisci and bone morphology, it is likely that there is a complex interplay amongst joint tissues and future work should investigate all joint tissues in a PTOA study and attempt to elude the starting point of damage to explore targeting early interventions to slow or prevent the onset and progression of PTOA.
The importance of subchondral bone in the development of OA has been well characterized in a review by (Li et al., 2013). This review documents the commonly seen subchondral bone sclerosis as a hallmark of OA in addition to microdamage, bone marrow edema-like lesions and bone cysts. Lacourt et al., 2012 studied the relationship between cartilage and subchondral bone in a racehorse model that is a natural model of repetitive trauma-induced osteoarthritis (Lacourt et al., 2012). While that study showed that cartilage damage was associated with bone degeneration, the meniscus was not investigated. In a guinea pig model of spontaneous OA, Zamli et al., 2014 showed that subchondral bone thickening preceded chondrocyte apoptosis and cartilage degradation (Zamli et al., 2014). Wang et al., 2013 showed that subchondral bone changes (i.e., increased thickness, bone mineral density and a decrease in porosity) occurred prior to cartilage degeneration (Wang et al., 2013). While these studies suggest that subchondral bone changes precede cartilage changes in spontaneous OA (Wang et al., 2013; Zamli et al., 2014), which tissue is first affected and potentially induces pathological changes in other joint structures after acute trauma has yet to be elucidated. If the order or sequence of degeneration were known, better therapeutic interventions could be investigated, improving the outcome of ACL replacement surgery or joint treatment following a traumatic impact event.
The current study strongly suggested that 1) early meniscal damage in the medial hemijoint resulted in subsequent damage to the medial cartilage and underlying tibial subchondral bone and 2) early medial meniscal damage likely affected the lateral hemijoint. Our model delivered a single injurious impact to the knee joint that resulted in ACL failure and either no or partial medial meniscal damage. Based on observable structural damage, partial or full medial meniscectomy was performed at the time of ACL reconstructive surgery. Degenerative changes in the medial meniscus, femoral and tibial articular cartilage and subchondral bone were correlated in a first attempt to help understand potential relationships between these three joint tissues after a single blunt joint impact. The study was limited by the fact that not all animals had meniscal damage requiring a meniscectomy and by the relatively small sample size (n = 6) in each group. Another limitation is that we did not apply a fixed pre-tension to the ACL reconstruction in the current study. However, clinically a fixed pre-tension is not used, and further, the pre-tension of the native tissue is not known, so what pre-tension to use remains unclear. Despite these limitations, these data suggested that, following trauma, the observed medial meniscal damage should be treated acutely by means other than a full or partial meniscectomy, since that procedure may have been the primary cause of degenerative changes in the underlying cartilage and subchondral bone. In fact, a clinical study confirms that repairs of meniscal damage are more favorable than meniscectomies (Lutz et al., 2015). Meniscal repair and substitution are becoming more commonplace and future research should continue to optimize these techniques (Paxton et al., 2011; Zaffagnini et al., 2011). Additionally, perhaps other improvements that aim to optimize acute knee trauma should be explored, such as treatment with Poloxamer 188 (P188) which acts to reseal cell membranes following traumatic rupture (Serbest et al., 2006; Natoli and Athanasiou, 2008; PascualGarrido et al., 2009; Bajaj et al., 2010; Isaac et al., 2010b; Coatney et al., 2015; Narez et al., 2021b). And yet, the current study also showed degenerative changes in the lateral cartilage and underlying bone without early observed damage to the overlying lateral meniscus post-trauma. Thus, while PTOA in the joint was likely induced by structural tissue changes in the medial hemijoint, there were likely other pathological processes at play.
Data Availability Statement
The original contributions presented in the study are included in the article/Supplementary Material, further inquiries can be directed to the corresponding author.
Ethics Statement
The animal study was reviewed and approved by the Institutional Animal Care and Use Committee at Michigan State University (IACUC #05/16-073-00 and #PROTO201900255).
Author Contributions
GN, FW, and MP oversaw data collection and analysis, and compiled all data. LD carried out all animal surgeries FW, RH and TH designed the study and oversaw all work. TH prepared the manuscript. All authors reviewed and edited the manuscript. All authors have read and approved the final submitted manuscript.
Funding
Research reported in this publication was supported by the U.S. Department of Defense under Grant Numbers W81XWH-16-1-0734 and W81XWH-16-1-0735.
Conflict of Interest
The authors declare that the research was conducted in the absence of any commercial or financial relationships that could be construed as a potential conflict of interest.
Publisher’s Note
All claims expressed in this article are solely those of the authors and do not necessarily represent those of their affiliated organizations, or those of the publisher, the editors, and the reviewers. Any product that may be evaluated in this article, or claim that may be made by its manufacturer, is not guaranteed or endorsed by the publisher.
Acknowledgments
The authors would like to acknowledge Clifford Beckett and Patrick Vaughn for technical support, and Dr. Maria Dolores Porcel Sanchez and Heather DeFore for surgical assistance during this study.
Reference
Abusara, Z., Andrews, S. H. J., von Kossel, M., and Herzog, W. (2018). Menisci Protect Chondrocytes from Load-Induced Injury. Sci. Rep. 8 (1), 1–11. doi:10.1038/s41598-018-32503-1
Bajaj, S., Shoemaker, T., Hakimiyan, A. A., Rappoport, L., Pascual-Garrido, C., Oegema, T. R., et al. (2010). Protective Effect of P188 in the Model of Acute Trauma to Human Ankle Cartilage: The Mechanism of Action. J. Orthop. Trauma 24 (9), 571–576. doi:10.1097/BOT.0b013e3181ec4712
Coatney, G. A., Abraham, A. C., Fischenich, K. M., Button, K. D., Haut, R. C., and Haut Donahue, T. L. (2015). Efficacy of P188 on Lapine Meniscus Preservation Following Blunt Trauma. J. Mech. Behav. Biomed. Mater. 47, 57–64. doi:10.1016/j.jmbbm.2015.03.008
Coleman, M. C., Goetz, J. E., Brouillette, M. J., Seol, D., Willey, M. C., Petersen, E. B., et al. (2018). Targeting Mitochondrial Responses to Intra-Articular Fracture to Prevent Posttraumatic Osteoarthritis. Sci. Transl. Med. 10 (427), eaan5372. doi:10.1126/SCITRANSLMED.AAN5372
Felson, D. T. (2004). An Update on the Pathogenesis and Epidemiology of Osteoarthritis. Radiologic Clin. North America 42 (1), 1–9. doi:10.1016/s0033-8389(03)00161-1
Fink, C., Hoser, C., Hackl, W., Navarro, R. A., and Benedetto, K. P. (2001). Long-Term Outcome of Operative or Nonoperative Treatment of Anterior Cruciate Ligament Rupture -Is Sports Activity a Determining Variable? Int. J. Sports Med. 22 (4), 304–309. doi:10.1055/s-2001-13823
Fischenich, K. M., Button, K. D., Coatney, G. A., Fajardo, R. S., Leikert, K. M., Haut, R. C., et al. (2015). Chronic Changes in the Articular Cartilage and Meniscus Following Traumatic Impact to the Lapine Knee. J. Biomech. 48 (2), 246–253. doi:10.1016/j.jbiomech.2014.11.038
Fischenich, K. M., Button, K. D., DeCamp, C., Haut, R. C., Donahue, T. L. H., and Donahue, H. (2017). Comparison of Two Models of Post-Traumatic Osteoarthritis; Temporal Degradation of Articular Cartilage and Menisci. J. Orthop. Res. 35 (3), 486–495. doi:10.1002/JOR.23275
Fischenich, K. M., Coatney, G. A., Haverkamp, J. H., Button, K. D., DeCamp, C., Haut, R. C., et al. (2014). Evaluation of Meniscal Mechanics and Proteoglycan Content in a Modified Anterior Cruciate Ligament Transection Model. J. biomechanical Eng. 136 (7), 0710011–0710018. doi:10.1115/1.4027468
Fischenich, K. M., Pauly, H. M., Button, K. D., Fajardo, R. S., DeCamp, C. E., Haut, R. C., et al. (2017). A Study of Acute and Chronic Tissue Changes in Surgical and Traumatically-Induced Experimental Models of Knee Joint Injury Using Magnetic Resonance Imaging and Micro-Computed Tomography. Osteoarthritis and Cartilage 25 (4), 561–569. doi:10.1016/j.joca.2016.10.010
Fox, A. J. S., Bedi, A., and Rodeo, S. A. (2012). The Basic Science of Human Knee Menisci. Sports Health 4 (4), 340–351. doi:10.1177/1941738111429419
Gerwin, N., Bendele, A. M., Glasson, S., and Carlson, C. S. (2010). The OARSI Histopathology Initiative - Recommendations for Histological Assessments of Osteoarthritis in the Rat. Osteoarthritis and cartilage 18 (Suppl. 3), S24–S34. doi:10.1016/J.JOCA.2010.05.030
Goldring, S. R., and Goldring, M. B. (2016). Changes in the Osteochondral Unit during Osteoarthritis: Structure, Function and Cartilage-Bone Crosstalk. Nat. Rev. Rheumatol. 12 (11), 632–644. doi:10.1038/NRRHEUM.2016.148
Hubrecht, R. C., and Carter, E. (2019). The 3Rs and Humane Experimental Technique: Implementing Change. Animals 9 (10), 754. doi:10.3390/ANI9100754
Hunter, D. J., Zhang, Y. Q., Niu, J. B., Tu, X., Amin, S., Clancy, M., et al. (2006). The Association of Meniscal Pathologic Changes with Cartilage Loss in Symptomatic Knee Osteoarthritis. Arthritis Rheum. 54 (3), 795–801. doi:10.1002/ART.21724
Isaac, D. I., Golenberg, N., and Haut, R. C. (2010). Acute Repair of Chondrocytes in the Rabbit Tibiofemoral Joint Following blunt Impact Using P188 Surfactant and a Preliminary Investigation of its Long-Term Efficacy. J. Orthop. Res. 28 (4), 553–558. doi:10.1002/jor.21022
Isaac, D. I., Meyer, E. G., and Haut, R. C. (2010). Development of a Traumatic Anterior Cruciate Ligament and Meniscal Rupture Model with a Pilot In Vivo Study. J. biomechanical Eng. 132 (6), 064501. doi:10.1115/1.4001111
Lacourt, M., Gao, C., Li, A., Girard, C., Beauchamp, G., Henderson, J. E., et al. (2012). Relationship between Cartilage and Subchondral Bone Lesions in Repetitive Impact Trauma-Induced Equine Osteoarthritis. Osteoarthritis and Cartilage 20, 572–583. doi:10.1016/j.joca.2012.02.004
Lerer, D. B., Umans, H. R., Hu, M. X., and Jones, M. H. (2004). The Role of Meniscal Root Pathology and Radial Meniscal Tear in Medial Meniscal Extrusion. Skeletal Radiol. 33 (10), 569–574. doi:10.1007/s00256-004-0761-2
Li, G., Yin, J., Gao, J., Cheng, T. S., Pavlos, N. J., Zhang, C., et al. (2013). Subchondral Bone in Osteoarthritis: Insight into Risk Factors and Microstructural Changes. Arthritis Res. Ther. 15 (6), 223. Available at: http://arthritis-research.com/content/15/6/223. doi:10.1186/ar4405
Lisee, C., Spang, J. T., Loeser, R., Longobardi, L., Lalush, D., Nissman, D., et al. (2021). Tibiofemoral Articular Cartilage Composition Differs Based on Serum Biochemical Profiles Following Anterior Cruciate Ligament Reconstruction. Osteoarthritis and cartilage 29 (12), 1732–1740. doi:10.1016/J.JOCA.2021.09.005
Lo, G. H., Niu, J., McLennan, C. E., Kiel, D. P., McLean, R. R., Guermazi, A., et al. (2008). Meniscal Damage Associated with Increased Local Subchondral Bone Mineral Density: A Framingham Study. Osteoarthritis and Cartilage 16 (2), 261–267. doi:10.1016/J.JOCA.2007.07.007
Lutz, C., Dalmay, F., Ehkirch, F.-P., Cucurulo, T., Laporte, C., Le Henaff, G., et al. (2015). Meniscectomy Versus Meniscal Repair: 10years Radiological and Clinical Results in Vertical Lesions in Stable Knee. Orthopaedics Traumatol. Surg. Res. 101 (8), S327–S331. doi:10.1016/J.OTSR.2015.09.008
Matyas, J. R., Atley, L., Ionescu, M., Eyre, D. R., and Poole, A. R. (2004). Analysis of Cartilage Biomarkers in the Early Phases of Canine Experimental Osteoarthritis. Arthritis Rheum. 50 (2), 543–552. doi:10.1002/art.20027
Miller, T. T., Staron, R. B., Feldman, F., and Çepel, E. (1997). Meniscal Position on Routine MR Imaging of the Knee. Skeletal Radiol. 26, 424–427. doi:10.1007/s002560050259
Narez, G. E., Brown, G., Herrick, A., Ek, R. J., Dejardin, L., Wei, F., et al. (2021). Assessment of Changes in the Meniscus and Subchondral Bone in a Novel Closed-Joint Impact and Surgical Reconstruction Lapine Model. J. Biomech. 126, 110630. doi:10.1016/J.JBIOMECH.2021.110630
Narez, G. E., Brown, G., Herrick, A., Ek, R. J., Dejardin, L., Wei, F., et al. (2022). Evaluating the Efficacy of Combined P188 Treatment and Surgical Intervention in Preventing Post-Traumatic Osteoarthritis Following a Traumatic Knee Injury. J. Biomech. Eng. 144 (4), 041003. doi:10.1115/1.4052564
Narez, G. E., Wei, F., Dejardin, L., Haut, R. C., and Haut Donahue, T. L. (2021). A Single Dose of P188 Prevents Cell Death in Meniscal Explants Following Impact Injury. J. Mech. Behav. Biomed. Mater. 117, 104406. doi:10.1016/j.jmbbm.2021.104406
Natoli, R. M., and Athanasiou, K. A. (2008). P188 Reduces Cell Death and IGF-I Reduces GAG Release Following Single-Impact Loading of Articular Cartilage. J. Biomech. Eng. 130 (4), 41012. doi:10.1115/1.2939368
Pascual Garrido, C., Hakimiyan, A. A., Rappoport, L., Oegema, T. R., Wimmer, M. A., and Chubinskaya, S. (2009). Anti-Apoptotic Treatments Prevent Cartilage Degradation after Acute Trauma to Human Ankle Cartilage. Osteoarthritis and Cartilage 17 (9), 1244–1251. doi:10.1016/j.joca.2009.03.007
Pauli, C., Grogan, S. P., Patil, S., Otsuki, S., Hasegawa, A., Koziol, J., et al. (2011). Macroscopic and Histopathologic Analysis of Human Knee Menisci in Aging and Osteoarthritis. Osteoarthritis and Cartilage 19 (9), 1132–1141. doi:10.1016/j.joca.2011.05.008
Pauly, H. M., Larson, B. E., Coatney, G. A., Button, K. D., DeCamp, C. E., Fajardo, R. S., et al. (2015). Assessment of Cortical and Trabecular Bone Changes in Two Models of Post-Traumatic Osteoarthritis. J. Orthop. Res. 33 (12), 1835–1845. doi:10.1002/jor.22975
Paxton, E. S., Stock, M. V., and Brophy, R. H. (2011). Meniscal Repair Versus Partial Meniscectomy: A Systematic Review Comparing Reoperation Rates and Clinical Outcomes. Arthrosc. J. Arthroscopic Relat. Surg. 27 (9), 1275–1288. doi:10.1016/J.ARTHRO.2011.03.088
Serbest, G., Horwitz, J., Jost, M., and Barbee, K. A. (2006). Mechanisms of Cell Death and Neuroprotection by Poloxamer 188 after Mechanical Trauma. FASEB j. 20 (2), 308–310. doi:10.1096/fj.05-4024fje
Sharma, L., Eckstein, F., Song, J., Guermazi, A., Prasad, P., Kapoor, D., et al. (2008). Relationship of Meniscal Damage, Meniscal Extrusion, Malalignment, and Joint Laxity to Subsequent Cartilage Loss in Osteoarthritic Knees. Arthritis Rheum. 58 (6), 1716–1726. doi:10.1002/art.23462
von Porat, A., Roos, E. M., and Roos, H. (2004). High Prevalence of Osteoarthritis 14 Years after an Anterior Cruciate Ligament Tear in Male Soccer Players: A Study of Radiographic and Patient Relevant Outcomes. Ann. Rheum. Dis. 63 (3), 269–273. doi:10.1136/ard.2003.008136
Wang, L.-J., Zeng, N., Yan, Z.-P., Li, J.-T., and Ni, G.-X. (2020). Post-Traumatic Osteoarthritis Following ACL Injury. Arthritis Res. Ther. 22, 57. doi:10.1186/s13075-020-02156-5
Wang, T., Wen, C.-Y., Yan, C.-H., Lu, W.-W., and Chiu, K.-Y. (2013). Spatial and Temporal Changes of Subchondral Bone Proceed to Microscopic Articular Cartilage Degeneration in Guinea Pigs with Spontaneous Osteoarthritis. Osteoarthritis and Cartilage 21, 574–581. doi:10.1016/j.joca.2013.01.002
Wang, Y., Wluka, A. E., Pelletier, J. P., Martel-Pelletier, J., Abram, F., Ding, C., et al. (2010). Meniscal Extrusion Predicts Increases in Subchondral Bone Marrow Lesions and Bone Cysts and Expansion of Subchondral Bone in Osteoarthritic Knees. Rheumatology 49 (5), 997–1004. doi:10.1093/rheumatology/keq034
Wei, F., Haut Donahue, T., Haut, R. C., Porcel Sanchez, M. D., and Dejardin, L. M. (2021). Reconstruction of the Cranial Cruciate Ligament Using a Semitendinosus Autograft in a Lapine Model. Vet. Surg. 50 (6), 1326–1337. doi:10.1111/vsu.13643
Wei, F., Powers, M. J. F., Narez, G. E., Dejardin, L. M., Haut Donahue, T., and Haut, R. C. (2022). Post-Traumatic Osteoarthritis in Rabbits Following Traumatic Injury and Surgical Reconstruction of the Knee. Ann. Biomed. Eng. 50, 169–182. doi:10.1007/S10439-022-02903-6
Whittaker, J. L., Woodhouse, L. J., Nettel-Aguirre, A., and Emery, C. A. (2015). Outcomes Associated with Early Post-Traumatic Osteoarthritis and Other Negative Health Consequences 3-10 Years Following Knee Joint Injury in Youth Sport. Osteoarthritis and Cartilage 23 (7), 1122–1129. doi:10.1016/J.JOCA.2015.02.021
Yoshioka, M., Coutts, R. D., Amiel, D., and Hacker, S. A. (1996). Characterization of a Model of Osteoarthritis in the Rabbit Knee. Osteoarthritis and Cartilage 4 (2), 87–98. doi:10.1016/s1063-4584(05)80318-8
Zaffagnini, S., Marcheggiani Muccioli, G. M., Lopomo, N., Bruni, D., Giordano, G., Ravazzolo, G., et al. (2011). Prospective Long-Term Outcomes of the Medial Collagen Meniscus Implant Versus Partial Medial Meniscectomy. Am. J. Sports Med. 39 (5), 977–985. doi:10.1177/0363546510391179
Keywords: impact injury, meniscus, cartilage, subchondral bone, knee
Citation: Haut Donahue TL, Narez GE, Powers M, Dejardin LM, Wei F and Haut RC (2022) A Morphological Study of the Meniscus, Cartilage and Subchondral Bone Following Closed-Joint Traumatic Impact to the Knee. Front. Bioeng. Biotechnol. 10:835730. doi: 10.3389/fbioe.2022.835730
Received: 14 December 2021; Accepted: 22 February 2022;
Published: 21 March 2022.
Edited by:
Nicola Francesco Lopomo, University of Brescia, ItalyReviewed by:
Matteo Berni, Rizzoli Orthopedic Institute (IRCCS), ItalyGregorio Marchiori, Rizzoli Orthopedic Institute (IRCCS), Italy
Copyright © 2022 Haut Donahue, Narez, Powers, Dejardin, Wei and Haut. This is an open-access article distributed under the terms of the Creative Commons Attribution License (CC BY). The use, distribution or reproduction in other forums is permitted, provided the original author(s) and the copyright owner(s) are credited and that the original publication in this journal is cited, in accordance with accepted academic practice. No use, distribution or reproduction is permitted which does not comply with these terms.
*Correspondence: T. L. Haut Donahue, dGhkb25haHVAZ21haWwuY29t