- University of Colorado Boulder, Boulder, CO, United States
Stents are a widely-used device to treat a variety of cardiovascular diseases. The purpose of this review is to explore the application of regenerative medicine principles into current and future stent designs. This review will cover regeneration-relevant approaches emerging in the current research landscape of stent technology. Regenerative stent technologies include surface engineering of stents with cell secretomes, cell-capture coatings, mimics of endothelial products, surface topography, endothelial growth factors or cell-adhesive peptides, as well as design of bioresorable materials for temporary stent support. These technologies are comparatively analyzed in terms of their regenerative effects, therapeutic effects and challenges faced; their benefits and risks are weighed up for suggestions about future stent developments. This review highlights two unique regenerative features of stent technologies: selective regeneration, which is to selectively grow endothelial cells on a stent but inhibit the proliferation and migration of smooth muscle cells, and stent-assisted regeneration of ischemic tissue injury.
Introduction
Cardiovascular diseases, a group of disorders of the heart or blood vessels, are considered to be the leading cause of mortality worldwide. An estimated 17.9 million people died from cardiovascular diseases in 2019 (32% of all global deaths), with 85% due to heart attack and stroke (WHO, 2021). Coronary artery disease is the most common type of cardiovascular diseases; (Virani et al., 2020) about 18.2 million (6.7%) adults age 20 and older in US have this disease.
Angioplasty, a surgical procedure to open vessel blockages along with placement of a vascular stent, is a common treatment for heart attack and an option for stroke treatment or stroke prevention. More than 2 million people get a stent each year for coronary artery disease alone (Medtronic, 2019). Coronary artery stents account for just over two-thirds of all types of vascular stents. The global market of coronary stents was valued at an estimated $7.7 billion in 2019 and is expected to grow at a compound annual growth rate of 4.7% to reach $11.3 billion in 2027 (GVS, 2020), with some predicting even faster growth (9.8%) (DAIC, 2020). This growth is driven by the rising prevalence of cardiovascular diseases, a growing geriatric population, an increased number of angioplasties and rising preference for the procedure. Though stenting is suggested for severe narrowing of coronary arteries according to current clinical guidelines, a recent report questioned the effectiveness of stenting, compared to medications, in many patients with severe but stable heart disease (Reuters, 2019; NIH, 2020). Additionally, restenosis and/or other disease progression, after 4 years of stenting, were found in 26–35% patients in a study (Taniwaki et al., 2014; Cassese et al., 2015). In patients with peripheral artery stents, 18–40% at 12 months were reported to have in-stent restenosis (Schillinger et al., 2006; Laird et al., 2010), while 5–10% for patients with coronary stents (Shlofmitz et al., 2019; Lawton et al., 2021). To strategize for improved stent performances, there is a re-surging interest in resorbable biomaterials, surface and biomolecule engineering approaches, capitalizing on the concept of vascular healing and regeneration.
Now is an important moment to examine regenerative approaches for this widely-used device in interventional cardiology and assess their benefits and risks for future stent therapies. We will start with a brief overview of the current status of stent uses in clinic, including stent types and uses in treating various cardiovascular diseases. Then, this review focuses on new methods emerging in recent stent research endeavors related to the regeneration concept. Comparative analyses of these methods include the regenerative effects, therapeutic effects and challenges faced. Lastly, thoughts about future perspectives in this subject area are provided in context of recent developments in relevant regenerative medicine.
Current Status of Clinical Use
Vascular stents are a device to treat a partially clogged artery by holding the arterial wall open and preventing it from collapsing. The majority of stents within the coronary stent market are bare metal stent (BMS) and drug eluting stent (DES), with the latter holding a larger share and being accepted as a safer treatment (Jensen and Christiansen, 2019). Nevertheless, the BMS is still widely used due to low cost and low hospitalization rates.
Major clinical issues for stent usage are thrombosis and restenosis. Restenosis, re-narrowing of the stented vessel due to proliferation and migration of smooth muscle cells, is triggered by arterial injury during stenting. Figure 1 illustrates desired and undesired fates of a stented vessel. BMS shows a higher rate of restenosis than DES, while the risk of DES is often a very late (>12-month post-implantation) thrombosis. In general, the pathology associated with stent thrombosis and restenosis starts with the loss of endothelium due to injury, followed by fibrinogen absorption, platelet activation or neointimal hyperplasia (Nakamura et al., 2016). The contributing factors are numerous, including biological, patient-specific, mechanical, and technical factors. The evolution of stenting technologies is accompanied by the gradually improved understanding of stent-related vascular pathologies such as acute arterial injury, inflammation, extracellular matrix deposition, and negative vascular remodeling. It is only through addressing these relevant pathologies that new stent technology can be developed to balance acute, chronic, and long-term requirements for stability, healing and regeneration of stented vessels. The most important element is a complete regeneration of fully functional endothelium over stented vessels.
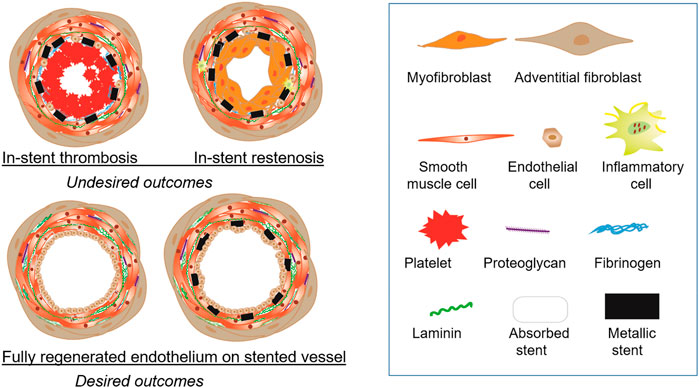
FIGURE 1. Outcomes of endovascular stents. (Top panel) Stents produce undesired outcomes such as in-stent thrombosis and in-stent restenosis. In-stent thrombosis involves accumulation of platelets and presence of fibrinogen. In-stent restenosis causes narrowing of the lumen greater than 50% due to the overgrowth of smooth muscle cells with neointima formation. (Botton panel) The ideal vascular regeneration with stents will have a fully developed endothelial monolayer lining the lumen with either the stent completely bioabsorbed or permanently encapsulated in the intimal layer.
DES
Widely-used DESs have a polymer coating blended with a drug or drug mixture that inhibit vascular smooth muscle cell growth. These anti-proliferative drugs are released over several months to help prevent restenosis. After that period, the stent may trigger platelet adhesion initiating thrombotic response. Controlled release of anti-proliferative agents inhibits neointimal growth but also delays arterial healing, thereby predisposing to a late risk of thrombosis. This high rate of late thrombosis (1–12 months post-implantation), demonstrated by the first generation stents, stimulated DES research for new generation stents in the last decade.
Contemporary second or third generations of stents are superior to the first generation in a number of clinical measures, including late stent thrombosis and strut coverage (Otsuka et al., 2014; Wiemer et al., 2017; Migita et al., 2020), showing a significant reduction in the risk of target lesion revascularization compared to early-generation DESs. Compared to the first generation stents, these stents display thinner stent struts (7–20 μm vs. 75–140 μm) and advancements in coatings including new anti-proliferative drugs and/or new polymers (Bangalore et al., 2018; Zaman et al., 2019). Reduced strut thickness offers greater flexibility and deliverability, while improvements in the drug or polymer realm permit faster vascular healing. Polymer-free stents are also available to decrease inflammatory reactions (McGinty et al., 2015; Worthley et al., 2017). Though newer generation DESs improve the effectiveness and safety profiles of their predecessors, long-term chronic inflammation and very late thrombosis remain serious concerns (Mori et al., 2017). More importantly, the prevention of cell growth by anti-proliferative drugs is counterproductive to vascular healing and regeneration; the coverage of stents with endothelial cells requires growth of these cells.
Covered Stent
Covered stents have a fabric material component that covers a metal stent. The majority of covered stents use polytetrafluoroethylene and have various clinical applications in peripheral arterial disease management. Covered stents offer an effective strategy for the creation of transjugular intrahepatic portosystemic shunts (Geeroms et al., 2017; Cho et al., 2020), as well as for repair endovascular aneurysm (O’Donnell et al., 2019), traumatic arterial lesions, and obstructive vascular disease of the aortoiliac and femoropopliteal sectors. Recently, the application of covered stents have expanded to seal coronary artery perforation (Lee et al., 2016; Wang et al., 2017; Birkemeyer et al., 2021; Wańha et al., 2021) and other perforations in clinic (Rizk et al., 2020). It also has a potential role in the treatment of friable embolization-prone plaques (Dogu Kilic et al., 2016). In the case of transjugular intrahepatic portosystemic shunts, covered stents, compared to BMS, showed higher estimated overall survival in patients (Gupta et al., 2017).
Recently, covered stents have become a platform that offer a minimally invasive, safe, and effective solution for arterial emergencies, such as bleeding, pseudoaneurysm, dissection, or fistula (Geeroms et al., 2017; O’Donnell et al., 2019). During the last few decades, treatment of these potentially life-threatening lesions has shifted from emergency open surgery to a stenting approach. Focusing on iatrogenic arterial injuries, Ruffino et al. gave an insight into the advantages of covered stents (Ruffino et al., 2020). In fact, it was believed that almost all arterial lesions may be treated with covered stents, except for those without anatomic suitability.
Taken together, current stent-assisted therapies—BMS, DES and covered stents—are employed to combat a wide range of vascular diseases and emergencies. Despite low complication rates (approximately 2–3% per year after the first year), stent therapy is still associated with a risk of restenosis and thrombosis, which persists for over 20 years. Recent comparisons of stent therapy and non-stent therapy for treating different clinical conditions such as coronary artery disease and infrainguinal peripheral artery disease showed no difference between stent and non-stent therapies, while the stent procedure is more invasive and has a significantly higher cost (Banerjee et al., 2019; NIH, 2020). Therefore, to demonstrate more competitive advantages over non-stent artery interventions, stent-assisted therapies demand innovations to proactively enhance arterial regeneration and long-term arterial health, in addition to the prevention of disastrous outcomes.
Novel Developments and Challenges in Stents
Similar to the application of regenerative medicine concepts in other tissues or devices, regeneration-relevant approaches emerge in the stent technology in two forms: regenerative surface and biodegradable or bioresorable scaffolding materials. However, due to the presence of diseased artery tissue around a stent, major considerations of all stent technologies must include their therapeutic impact such as the inhibition of arterial recoil and smooth muscle proliferation. To this end, we review the two major types of approaches to stent design by comparing and evaluating their regenerative effects and therapeutic effects.
Regenerative Stent Surface
Continued efforts towards stent surface modifications are seen in the 1990s and 2000s as well as more recently. Earlier efforts focused on therapeutic effects such as reduced restenosis, using 1) inorganic coatings, including diamond-like carbon (Airoldi et al., 2004), pyrolytic carbon (Danzi et al., 2004), titanium nitride oxide (Windecker et al., 2005), and carbide (Unverdorben et al., 2003); 2) gene-eluting coatings, incorporating mRNA, siRNA, miRNA, or plasmid DNA of therapeutic genes in the coating; (Feldman et al., 2000; Sharif et al., 2012; Wang et al., 2021) 3) cell-seeded stent, using endothelial cells or progenitor cells (Zhu et al., 2008; Shi et al., 2010; Jantzen et al., 2011; Raina et al., 2014). The inorganic coatings provided ineffective or inconclusive performance (e.g., restenosis rate), compared to uncoated BMS (Kim et al., 2005; Meireles et al., 2007; O’Brien and Carroll, 2009). The gene-eluting coatings, like DES, incorporate genes for therapeutic moiety, but offer a longer term efficacy and a wider variety of therapeutic strategies beyond suppressing cell proliferation for restenosis inhibition (Yang et al., 2013; Adeel and Sharif, 2016; Fishbein et al., 2017; Hytönen et al., 2018). Nevertheless, the clinical translation of such coatings is still extremely challenging due to significant technical hurdles, demanding improvements over release kinetics, cell-specific transfection, reduced toxicity, and inflammation. Similarly, great difficulty in the clinical translation is faced by cell-seeded stents (Jantzen et al., 2016; Tsukada et al., 2021).
The root cause of stent pathology, as revealed by numerous studies on BMS and DES, (Cornelissen and Vogt, 2019; Bedair et al., 2017; Kobo et al., 2020; Scafa Udriște et al., 2021), is endothelial dysfunction and/or impaired growth of endothelial cells in the stented artery. Healthy endothelial cells naturally prevent the blood from clotting, and produce molecules such as nitric oxide (NO) and prostacyclin for smooth muscle relaxation and growth inhibition. Thus, modifications of the stent surface in the last decade involve approaches to promote in situ re-endothelialization or to integrate critical endothelial functions. This is achieved through cell secretomes, cell-capture coatings, mimics of endothelial products (e.g., NO), surface topography, endothelial growth factor, cell-adhesive peptides and other biologics. Table 1 provides a brief summary of these functionalization approaches. Details of each approach are described in following paragraphs. As reviewed by Bedair et al. (2017), the immobilization of molecules onto the stent surface involves physical adsorption, encapsulation, ionic bonding or covalent conjugation through various chemical reactions such as carbodiimide chemistry. The covalent conjugation may begin with plasma treatment or a layer of polydopamine for abundant free hydroxyl groups, and then attach a functional polymer such as bioclickable polymer.
The regenerative aspects of a stent are indeed unique. Two prominent features are noted. First, stent-related regeneration is “selective regeneration”, which is to selectively grow endothelial cells on the stent surface but inhibit the proliferation and migration of smooth muscle cells. Therefore, multifunctional coatings in recent developments exhibit both regenerative effect on the endothelium and therapeutic effects on the smooth muscle. Second, stent-assisted regeneration of ischemic tissue injury is needed in certain applications such as myocardial infarction and renal ischemia, where narrowing of an artery is accompanied by the loss of distal vasculature and thus tissue injury. Stenting provides an opportunity to regenerate the tissues with biologics that regenerate stented artery and distal vasculature (d’Souza et al., 2017). Regenerative biologics can also alleviate the impaired vasorelaxation in nonstented proximal and distal segments of stented arteries, in particular those with DES (Nakamura et al., 2011; van den Heuvel et al., 2010; Lim et al., 2012).
Stent Incorporated With Cell Secretome
Cardiovascular stents incorporated with secretomes from stem cells represents a novel direction for regenerative stents. Hu et al. recently reported exosome-eluting stents for vascular healing and tissue regeneration after ischemic injury (Hu et al., 2021). These stents were coated with exosomes derived from mesenchymal stem cells. The bioactive stents promoted vascular healing and repair of ischemic condition, which usually need additional procedures for regenerative therapy in a patient. Exosomes are nanoscale membranous sacs secreted by most cell types; those from stem cells show great potentials of delivering regenerative and therapeutic benefits (Burke et al., 2016; Eisenstein, 2020; Nikfarjam et al., 2020; Wei et al., 2021). A major regenerative benefit of exosomes is to rejuvenate endothelial cells (Baruah and Wary, 2020), which can not only help to cover the stent with a healthy endothelium but also promote distal revascularization for tissue repair. A prominent therapeutic benefit of exosomes is immune-modulation or immune-suppression (Domenis et al., 2018; Suh et al., 2021). The exosome coating on stents may help camouflage the stent, blocking adverse immune reactions. Another novel aspect of the stent design by Hu et al. is the controlled release of exosomes from the stent coating in the presence of reactive oxygen species, a hallmark of inflammation (Hu et al., 2021). In the long run, stents incorporating cell products like exosomes or other forms of cell secretomes might offer a safer and simpler alternative to cell-seeded stents.
Cell-Capture Stent
Another alternative to cell-seeded stents is to utilize biomolecule coatings to capture circulating cells in the blood for endothelial regeneration (Sethi and Lee, 2012; Pang et al., 2015). To that end, various antibodies recognizing endothelial progenitor cell (EPC), like anti- CD34, VE-cad, or CD133, were loaded to the stent surface (Lim et al., 2011; Leopold, 2013; Wawrzyńska et al., 2019). These antibody-coated stents were compared in terms of their capability of capturing circulating EPCs. All types of antibody-coated stents were completely covered with a cell layer in earlier stage (e.g., one-week post-implantation) than BMS in animals. Animal studies showed that stents coated with anti-CD133 or VE-cad, compared with those with anti-CD34, accelerated re-endothelialization and reduced in-stent restenosis (Lee et al., 2012; Wu et al., 2015).
Preclinical or clinical results using cell-capture stents, however, are not always positive. Those with anti-CD34 were found to improve early endothelialization in swine but not affecting neointimal thickness (van Beusekom et al., 2012). Sedaghat et al., using a porcine model, found no difference in re-endothelialization or neointima formation with the use of CD133-stents compared with BMS (Sedaghat et al., 2013). This is supported by studies showing circulating EPCs or bone marrow-derived cells failed to significantly contribute directly to endothelial regeneration; cell-capture stent might increase restenosis rate in clinic (Blessing et al., 2020; Evans et al., 2021). Such inconsistency among preclinical outcomes of cell-capture stents can be attributed to the low number and the high heterogeneity of circulating EPCs (Medina et al., 2017; Keighron et al., 2018). Antibodies on stents may also increase unspecific binding of mononuclear cells, likely causing complications (Sedaghat et al., 2013).
For all regenerative purposes and translational intents, current and future endeavors utilizing cell-capture mechanism may continue in three directions: 1) combining cell-capture molecules with other prohealing/proregenerative molecules; (Chen et al., 2015; Zhang et al., 2015; Wang et al., 2020a; Yang et al., 2020a) 2) using more selective or specific molecules to recognize a subset of EPCs; (Hirase, 2016; Tang et al., 2016) 3) exploiting EPC-derived secretomes such as those in the form of exosomes (Zeng et al., 2021).
NO-Producing Coatings
NO is one of the most potent molecules produced by healthy endothelial cells that play multiple essential roles in cardiovascular physiology (Napoli et al., 2006; Strijdom et al., 2009). Vascular injury, just like in the case of stent deployment, reduced NO production of endothelium. Incorporating a NO-producing mechanism into stent coatings has a range of benefits, including the prevention of thrombosis through inhibiting platelet aggregation, anti-inflammation through reducing monocyte adhesion, inhibition of SMC migration and proliferation through SMC relaxation, as well as stimulation of endothelial proliferation for stent endothelialization (Rao et al., 2020). A recent review by Rao et al. detailed this topic, in particular the therapeutic effect of NO-producing coatings for the prevention of thrombosis and restenosis (Rao et al., 2020).
There are two types of NO-producing coatings, NO-releasing and NO-generating coatings. NO-releasing coatings involves exogenous NO donors such as N-diazeniumdiolates (i.e., NONOates), S-nitroso-N-acetylpenicillamine, and peptide amphiphiles (Kushwaha et al., 2010; Zhu et al., 2020), immobilized by polymer or liposome (Elnaggar et al., 2016). These donors release NO in limited periods of time. To extend the release time, recent developments involves the attachment of NO donors to polymers (Hopkins et al., 2018) or enzyme-sensitive linkers (Winther et al., 2018; Midgley et al., 2020). NO-generating coatings utilize eNOS gene (Sharif et al., 2012), or immobilize catalysts such as copper and selenium to convert endogenous NO donors such as S-nitrosothiols into NO (Yang et al., 2018; Fan et al., 2019; Zhang et al., 2019; Yang et al., 2020b). All the studies using stents with either NO-releasing or NO-generating coating show promising results in vitro and in vivo. This is consistent with the results from recent graft study which employed NO-producing vascular graft on rodents for 12 weeks and found rapid endothelialization and hampered SMC proliferation (Enayati et al., 2021). Despite promising outcomes, long-term preclinical evaluations on NO-generating stents are yet to be performed.
VEGF-Induced Regeneration
VEGF is a most potent, endothelial-specific growth factor to regenerate endothelial cells. Early attempts to incorporate it into stent coatings were made as early as 1990s (Van Belle et al., 1997). However, inconsistent results regarding re-endothelialization and inhibition of restenosis were found in animal studies, which might be derived from different methods of preparing VEGF-eluting stents. Opposite to the finding from an earlier study using VEGF-eluting stent (Van Belle et al., 1997), Swanson et al. found VEGF-eluting stents did not accelerate re-endothelialization or inhibit restenosis, but reduced stent thrombosis (Swanson et al., 2004). Both studies evaluated stents with iliac artery model on New Zealand rabbits. Recent VEGF-bound stents have found more success in the preclinical evaluations. Using a porcine coronary model, Takabatake et al. used VEGF on poly-(ethylene-co-vinyl alcohol) coated stents, and found VEGF-bound stents, compared with anti-CD34 antibody-bound stents, provided highly selective capture of EPCs, followed by a rapid formation of intact endothelium at an early period of stenting (Takabatake et al., 2014). Yang et al. showed DES with VEGF gene completed re-endothelialization and significantly suppressed in-stent restenosis after 1 month compared to commercial DES (Yang et al., 2013). Wang et al. spatially bound VEGF and rapamycin to the base and top of hierarchical capillary coating, respectively, and showed the competitive growth of endothelial cells over smooth muscle cells on the stent surface as well as a high level of re-endothelialization and a very low level of in-stent restenosis using a minipig model (Wang et al., 2020b).
In conclusion, VEGF-bound stents hold great promise in endothelial regeneration but the outcome using this regenerative molecule depends on: 1) co-grafting condition, such as drugs or other molecules coexisting on the stent and collaborating into action at the stent-artery interface; 2) the form of VEGF (e.g., gene, protein, and peptide), and 3) the conjugation method of VEGF, for example eluting vs. polymer-bound.
Surface Pattern
Specialized micro- and nano-patterns have been introduced on the stent surface to reduce the risk of blood clotting, expedite healing and improve endothelialization. The design of textures on the thin struts of a stent includes nanotubes, ridges, pores, diamonds, leafy structure, or even a pattern mimicking the shape of smooth muscle cells (Cherian et al., 2021) (Junkar et al., 2020). Importantly, the textures are assessed by preferential growth of endothelial cells over smooth muscle cells (Cherian et al., 2020). However, the reason for this selective cell proliferation remain elusive. Manufacturing methods can involve anodization (Saleh et al., 2017), atomic layer deposition (Yang et al., 2019), lithography or femtosecond laser. Lee and Desai, for example, altered the anodization conditions to achieve nanotubular coatings with 110 and 70 nm nanotube diameters, and found competitive growth of endothelial cells over smooth muscle cells on nanotubular stents (Lee and Desai, 2016). Nanostructure formation is free of polymers or new chemicals, which might speed up the approval process for the clinical translation.
Other Biologics-Based Coatings
Two major groups of other biologics used in stent coatings, often in combination with other approaches, are adhesive peptides and glycosaminoglycan (GAG) molecules such as heparin and hyaluronic acid or GAG-mimics such as chitosan.
Adhesive peptides are instrumental for the attachment of circulating cells like EPCs. Blindt et al. found that DES with cyclic RGD peptide inhibited neointimal hyperplasia by recruiting EPCs in porcine coronary arteries (Blindt et al., 2006). With improved vascular healing in second-generation DESs, recent approaches employed adhesive peptides in conjunction with other functional molecules such as CXCL1 (Simsekyilmaz et al., 2016), or more specific adhesive peptides such as YIGSR (Alexander et al., 2018) and REDV (Wei et al., 2013). Jang et al. coated DES with WKYMVm peptide and hyaluronic acid, and using rabbit iliac model found the WKYMVm coating promoted endothelial healing but did not reduce restenosis rate compared to commercial DES (Jang et al., 2015). Wei et al. immobilized REDV peptide and phosphorylcholine on BMS and showed the competitive ability of endothelial cell growth over smooth muscle growth in vitro and in vivo, and reduced restenosis compared to BMS (Wei et al., 2013). The downside of using adhesive peptides to recruit circulating cells, however, is its possibility of increasing unspecific binding of other circulating cells such as mononuclear cells.
Immobilization of GAGs or GAG-mimics onto the stent surface is a strategy to enhance stent anti-thrombogenicity. Heparin, heparin-like molecules and hyaluronic acid are often utilized in combination with other molecules to coat stent surfaces. Heparin was used with NO-producing, DES, or bioresorbable stents (Bae et al., 2017; Lee et al., 2019; Qiu et al., 2019; Zhu et al., 2020; Zhang et al., 2021). Due to its anti-thrombogenic, anti-fouling characteristics, hyaluronic acid often serves as a base adhesive material on stents (Zhang et al., 2015; Kim et al., 2016).
Besides adhesive peptides and GAGs, new signaling molecules such as CD31-mimic are used to stimulate the proliferation of adjacent endothelial cells to regenerate the endothelium (Diaz-Rodriguez et al., 2021).
Stent Coating With a Combination of Biomolecules
There is an increasing interest in researching multifunctional stent surfaces that contain components promoting therapeutic effect, selective regeneration, and/or critical endothelial functions. In particular, coatings are designed to both counteract endothelial dysfunction and mimic endothelial characteristics. For example, recent studies have combined NO production with cell-adhesive ligands or anti-thrombogenic molecules (Qiu et al., 2019; Yang et al., 2020b; Yang et al., 2020c; Lyu et al., 2020; Zhu et al., 2020; Ma et al., 2021; Zhang et al., 2021). Yang et al. functionalized the stent with NO-generating organoselenium and EPC-targeting peptide through mussel adhesive chemistry and bio-orthogonal conjugation (Yang et al., 2020b). The ratio of the two components on stent the coating was optimized for anti-thrombosis, smooth muscle inhibition and EPC-capture capacity, ultimately leading to rapid re-endothelialization and effective stent restenosis prevention in vivo.
Regenerative Strategies for Covered Stents
Few attempts have been made to functionalize or replace the existing polytetrafluoroethylene-covered stent. Among them is the use of polyurethane-covered stent in recent studies, but its clinical efficacy is yet to be demonstrated with comparisons to polytetrafluoroethylene-covered stent (Hernández-Enríquez et al., 2018; Song et al., 2021). A new trend is to develop covered stents using natural-derived or biodegradable materials with regenerative potentials, as illustrated by endothelial progenitor cells-laden coronary stent covered with ECM (Park et al., 2021) and stent covered with silk and elastin proteins (Putzu et al., 2019). Additionally, to replace inert materials used for covering stents, a wide range of vascular-regenerative polymers available for vascular graft applications can also be excellent candidates (Leal et al., 2021). For example, we demonstrated vascular grafts composed of coaxial fibers with a structure of polycaprolactone core and photoclickable, 4-arm thiolated polyethylene glycol-norbornene sheath, which can be conjugated to biomolecules for vascular regeneration (Iglesias-Echevarria et al., 2021). In the future, the platform of covered stent with regenerative covering may evolve into a new type of stent therapy which utilize bioabsorbable materials with regenerative signals to better treat vascular diseases.
In summary, new developments in the stent surface functionalizations focus on the regeneration of the vascular endothelium or the restoration of major endothelial functions such as anti-thrombogenicity and NO production. The endothelium normally controls smooth muscle activities, and provides an efficient barrier against thrombosis and inflammation. However, the endothelium reestablished after the stenting procedure (e.g., percutaneous coronary intervention) is incompetent in terms of its integrity and function, showing poorly formed cell junctions, reduced expression of anti-thrombotic molecules (e.g., thrombomodulin, prostacyclin), and decreased NO production (Otsuka et al., 2012). Drugs from DES further inhibit endothelial regeneration or function restoration. The missing or incompetent endothelium in existing stent products (e.g., BMS, DES, and covered stent) is a root cause of late or very late in-stent thrombosis and restenosis, as well as impaired function of proximal or distal blood vessels. As shown in Figure 2, re-endothelialization mechanisms include regeneration from adjacent cells and regeneration from blood-borne cells, both of which require regenerative cues on stents to override the cell inhibition from therapeutic agents (i.e., drugs). Improved understanding of regeneration mechanisms for competently functioning endothelium is essential for long-term stent performances.
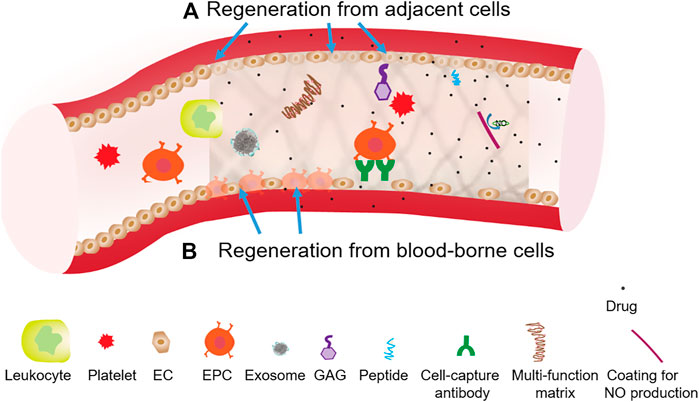
FIGURE 2. Two generation mechanisms using surface functionalizations to override drug inhition effects on stent endothelialization. (A) Regeneration from adjacent cells, which can involve the functionalization of stents with angiogenic peptide or NO producing coating. (B) Regeneration from blood-borne cells, which can involve the functionalization of stents with certain cell-capture antibodies, adhesive peptide or growth factor.
Bioabsorbable Vascular Stent
To mitigate the risk of restenosis and thrombosis in stent, it has long been envisioned that a fully bioresorbable polymer is used in replacement of metal. The first-generation, FDA-approved BVS product was created and launched by Abbott as Absorb BVS stent in September 2012. This type of products was characterized by a degradation rate, ranging from 6 months to 2 years, to avoid undesired long-term effects, but demonstrated worse clinical performances including in-stent thrombosis, restenosis, and target vessel myocardial infarction, when compared to BMS or DES (Capodanno et al., 2015; Lipinski et al., 2016; Serruys et al., 2016). Thus, it was not recommended (Neumann et al., 2019), and pulled off the market in 2017. The first-generation BVS also was found a long-term concern on side branch ostia (Onuma et al., 2017).
Newer BVS technologies and devices mostly loaded with drugs are currently undergoing preclinical and clinical testing (Ellis et al., 2017; Serruys et al., 2017; Omar and Kumbhani, 2019). A promising feature of BVS, shown in a clinical study comparing BVS to DES, is more significant outward remodeling (Serruys et al., 2017). To decrease BVS thrombogenicity, one advancement, like the major improvement made in the second generation of DES, is a progressive reduction of the BVS strut thickness to as thin as 60 μm (Buccheri and Capodanno, 2019). The majority of BVSs on the pre-market consist of polymers such as polylactic acid used in Absorb BVS, but degradable metals such as magnesium (Chen et al., 2019; Hideo-Kajita et al., 2019) or zinc (Fu et al., 2020) are also actively being developed for newer generation of BVS.
To enhance therapeutic and regenerative performances of BVS, bioactive molecules can also coat the BVS surface or be integrated into the bulk polymer. Any regenerative molecules reviewed above (Table 1) may be added into or on top of BVS. Besides these molecules, Yang et al. formed a multilayer coating of collagen type III and hyaluronic acid on a polylactic acid stent via layer-by-layer assembly, which enhanced endothelialization and thromboprotection, and inhibited excessive neointimal hyperplasia using a rabbit abdominal aorta model (Yang et al., 2021). Collagen type III does not present binding sites for platelets while retaining the affinity for endothelial cells.
For a more detailed summary of BVS, please refer to additional reviews on the topic (Omar and Kumbhani, 2019; Cockerill et al., 2021; Yuan et al., 2022). In brief, researchers in academia and industry share the dream of a bioabsorbable scaffold that delivers therapeutic and regenerative agents to the vessel, maintains radial strength for a sufficient period of time (>6 months), and then disappears when therapy and regeneration jobs are done. The BVS materials are resorbed over 6 months to 2 years, having a potential of eliminating chronic inflammation and enabling endothelial regeneration, when compared to permanent metallic stents. However, the clinical performances of existing BVS are inferior to DES or BMS. Improved understanding of the failure mechanisms of existing BVS is essential for the design of new generation BVS with more superior clinical performances.
Challenges and Future Perspectives
With advancements in stent technologies and implantation techniques, the event-free survival rate for stent patients, in particular those with the new generation DES, has kept improving in the last decade. Based on the overall satisfactory results from the new generation DES, it can be extremely challenging for any new stent design or material to demonstrate better effectiveness while being safe and worth enormous investments into innovation and translation. An additional barrier that hampers the translation of the new design or material to clinical applications lies in the lack of sufficient understanding of mechanisms underlying the materials interactions. Further refinement of existing DES has an excellent benefit-risk ratio. Therefore, future developments of permanent metallic stents such as new functional coating or topography strategies may take the advantage of the current DES platform, based off it for design and performance analysis.
To address the long-term concern about permanent metallic stents—an ongoing risk of restenosis or thrombosis arising from the implant site which persists for at least 20 years (Yamaji et al., 2010; Yamaji et al., 2016; Stone et al., 2019) occurring after the first year at a rate of approximately 2–3% per year—BVS composed of biodegradable polymers or metals holds great potentials. Hypothetically, BVS provides a temporary scaffold for selective vascular regeneration—a complete re-endothelialization and significant outward remodeling. Such regeneration ideally may prevent the adverse events, including strut fractures, loss of vessel compliance, vasomotion, maladaptive vascular remodeling, and development of late neoatherosclerosis (Stone et al., 2019). Currently, this dream of BVS is not close to reality. A step further towards the dream is to innovate biodegradable materials engineering and innovate designs for both stent structure and surface, with the goal of balancing short-term and long-term requirements for BVS in terms of mechanics, therapy and regeneration. In parallel, mechanisms underlying BVS-related vascular pathobiology should be defined in a synergistic manner.
Finally, an amazing array of stent functionalization strategies are available in the literatures. However, translation efforts of them into clinical practices remain very limited if not futile. Future efforts in this area may fall into two categories: 1) Stent design according to specific needs of a patient’s cohort (or a specialized preclinical model) for competitive efficacy and superior outcome. For example, the regeneration of distal vasculature is only desired in ischemic conditions. Another example is for cell-capture stents: the EPC quantity, quality and capability can vary greatly among patients, and thus in situ stent cellularization results can vary accordingly. In this case, custom design strategies may be necessary for a specific patient’s cohort. 2) Multiple signaling strategies with defined spatiotemporal regimes of regenerative signals.
Author Contributions
WT drafted the review. PS, MR, and RJ edited it.
Conflict of Interest
The authors declare that the research was conducted in the absence of any commercial or financial relationships that could be construed as a potential conflict of interest.
Publisher’s Note
All claims expressed in this article are solely those of the authors and do not necessarily represent those of their affiliated organizations, or those of the publisher, the editors, and the reviewers. Any product that may be evaluated in this article, or claim that may be made by its manufacturer, is not guaranteed or endorsed by the publisher.
Acknowledgments
The authors would like to acknowledge the funding sources (NHLBI-HL119371 and AHA-19TPA34850168).
References
Adeel, M. Y., and Sharif, F. (2016). Advances in Stent-Mediated Gene Delivery. Expert Opin. Drug Deliv. 13, 465–468. doi:10.1517/17425247.2016.1144589
Airoldi, F., Colombo, A., Tavano, D., Stankovic, G., Klugmann, S., Paolillo, V., et al. (2004). Comparison of diamond-like Carbon-Coated Stents versus Uncoated Stainless Steel Stents in Coronary Artery Disease. Am. J. Cardiol. 93, 474–477. doi:10.1016/j.amjcard.2003.10.048
Alexander, G. C., Hwang, P. T. J., Chen, J., Kim, J., Brott, B. C., Yoon, Y. S., et al. (2018). Nanomatrix Coated Stent Enhances Endothelialization but Reduces Platelet, Smooth Muscle Cell, and Monocyte Adhesion under Physiologic Conditions. ACS Biomater. Sci. Eng. 4, 107–115. doi:10.1021/acsbiomaterials.7b00676
Bae, I.-H., Lim, K. S., Park, D. S., Shim, J.-W., Lee, S.-Y., Jang, E.-J., et al. (2017). Sirolimus Coating on Heparinized Stents Prevents Restenosis and Thrombosis. J. Biomater. Appl. 31, 1337–1345. doi:10.1177/0885328217706222
Banerjee, S., Jeon-Slaughter, H., Armstrong, E. J., Bajzer, C., Abu-Fadel, M., Khalili, H., et al. (2019). Clinical Outcomes and Cost Comparisons of Stent and Non-stent Interventions in Infrainguinal Peripheral Artery Disease: Insights from the Excellence in Peripheral Artery Disease (XLPAD) Registry. J. Invasive Cardiol. 31, 1–9.
Bangalore, S., Toklu, B., Patel, N., Feit, F., and Stone, G. W. (2018). Newer-Generation Ultrathin Strut Drug-Eluting Stents versus Older Second-Generation Thicker Strut Drug-Eluting Stents for Coronary Artery Disease. Circulation 138, 2216–2226. doi:10.1161/CIRCULATIONAHA.118.034456
Baruah, J., and Wary, K. K. (2020). Exosomes in the Regulation of Vascular Endothelial Cell Regeneration. Front. Cel Dev. Biol. 7, 353. doi:10.3389/fcell.2019.00353
Bedair, T. M., ElNaggar, M. A., Joung, Y. K., and Han, D. K. (2017). Recent Advances to Accelerate Re-endothelialization for Vascular Stents. J. Tissue Eng. 8, 204173141773154. doi:10.1177/2041731417731546
Birkemeyer, R., Olivecrona, G. K., Hellig, F., Wöhrle, J., Rottbauer, W., Witkowski, A., et al. (2021). Sealing of Coronary Perforations with a Second-Generation Covered Stent Graft - Results from the PAST-PERF Registry. Cardiovasc. Revascularization Med. 25, 20–26. doi:10.1016/j.carrev.2020.10.012
Blessing, R., Ahoopai, M., Geyer, M., Brandt, M., Zeiher, A. M., Münzel, T., et al. (2020). The Bioengineered Combo Dual-Therapy CD34 Antibody-Covered Sirolimus-Eluting Coronary Stent in Patients with Chronic Total Occlusion Evaluated by Clinical Outcome and Optical Coherence Tomography Imaging Analysis. Jcm 10, 80. doi:10.3390/jcm10010080
Blindt, R., Vogt, F., Astafieva, I., Fach, C., Hristov, M., Krott, N., et al. (2006). A Novel Drug-Eluting Stent Coated with an Integrin-Binding Cyclic Arg-Gly-Asp Peptide Inhibits Neointimal Hyperplasia by Recruiting Endothelial Progenitor Cells. J. Am. Coll. Cardiol. 47, 1786–1795. doi:10.1016/j.jacc.2005.11.081
Buccheri, S., and Capodanno, D. (2019). Bioabsorbable Stents: Only Bad News? Eur. Heart J. Supplements 21, B28–B30. doi:10.1093/eurheartj/suz009
Burke, J., Kolhe, R., Hunter, M., Isales, C., Hamrick, M., and Fulzele, S. (2016). Stem Cell-Derived Exosomes: A Potential Alternative Therapeutic Agent in Orthopaedics. Stem Cell Int. 2016, 1–6. doi:10.1155/2016/5802529
Capodanno, D., Gori, T., Nef, H., Latib, A., Mehilli, J., Lesiak, M., et al. (2015). Percutaneous Coronary Intervention with Everolimus-Eluting Bioresorbable Vascular Scaffolds in Routine Clinical Practice: Early and Midterm Outcomes from the European Multicentre GHOST-EU Registry. EuroIntervention 10, 1144–1153. doi:10.4244/EIJY14M07_11
Cassese, S., Byrne, R. A., Schulz, S., Hoppman, P., Kreutzer, J., Feuchtenberger, A., et al. (2015). Prognostic Role of Restenosis in 10 004 Patients Undergoing Routine Control Angiography after Coronary Stenting. Eur. Heart J. 36, 94–99. doi:10.1093/eurheartj/ehu383
Chen, C., Chen, J., Wu, W., Shi, Y., Jin, L., Petrini, L., et al. (2019). In Vivo and In Vitro Evaluation of a Biodegradable Magnesium Vascular Stent Designed by Shape Optimization Strategy. Biomaterials 221, 119414. doi:10.1016/j.biomaterials.2019.119414
Chen, F., Yang, F., Zhao, Q., Feng, S., Li, W., Bi, Y., et al. (2015). Long-Term Effects of Novel Combination Coating Anti-CD34 Antibody Applied on Sirolimus-Eluting Stents. J. Interv. Cardiol. 28, 257–263. doi:10.1111/joic.12208
Cherian, A. M., Joseph, J., Nair, M. B., Nair, S. V., Maniyal, V., and Menon, D. (2020). Successful Reduction of Neointimal Hyperplasia on Stainless Steel Coronary Stents by Titania Nanotexturing. ACS Omega 5, 17582–17591. doi:10.1021/acsomega.0c02045
Cherian, A. M., Nair, S. V., Maniyal, V., and Menon, D. (2021). Surface Engineering at the Nanoscale: A Way Forward to Improve Coronary Stent Efficacy. APL Bioeng. 5, 021508. doi:10.1063/5.0037298
Cho, J. K., Serna, J., Clark, T. W. I., Dagli, M., Mondschein, J. I., Shlansky-Goldberg, R. D., et al. (2020). Impact of PTFE-Covered Stent Position and Extension on TIPS Patency. Abdom. Radiol. 45, 3915–3922. doi:10.1007/s00261-020-02566-8
Cockerill, I., See, C. W., Young, M. L., Wang, Y., and Zhu, D. (2021). Designing Better Cardiovascular Stent Materials: A Learning Curve. Adv. Funct. Mater. 31, 2005361. doi:10.1002/adfm.202005361
Cornelissen, A., and Vogt, F. J. (2019). The Effects of Stenting on Coronary Endothelium from a Molecular Biological View: Time for Improvement? J. Cel Mol Med 23, 39–46. doi:10.1111/jcmm.13936
d'Souza, J., Giri, J., and Kobayashi, T. (2017). Stent-based Revascularization for Complex Lesions in PAD. J. Cardiovasc. Surg. 58, 715–721. doi:10.23736/s0021-9509.17.09994-3
Daic, (2020). Coronary and Peripheral Stent Market Expected to Double through 2025. DAIC. Available at: http://www.dicardiology.com/content/coronary-and-peripheral-stent-market-expected-double-through-2025.(accessed November 4, 2021).
Danzi, G. B., Capuano, C., Sesana, M., Baglini, R., Bartorelli, A. L., Trabattoni, D., et al. (2004). Six-month Clinical and Angiographic Outcomes of the Tecnic Carbostent Coronary System: the Phantom IV Study. J. Invasive Cardiol. 16, 641–644.
Diaz-Rodriguez, S., Rasser, C., Mesnier, J., Chevallier, P., Gallet, R., Choqueux, C., et al. (2021). Coronary Stent CD31-Mimetic Coating Favours Endothelialization and Reduces Local Inflammation and Neointimal Development In Vivo. Eur. Heart J. 42, 1760–1769. doi:10.1093/eurheartj/ehab027
Dogu Kilic, I., Fabris, E., Serdoz, R., Caiazzo, G., Foin, N., Abou-Sherif, S., et al. (2016). Coronary Covered Stents. EuroIntervention 12, 1288–1295. doi:10.4244/EIJV12I10A210
Domenis, R., Cifù, A., Quaglia, S., Pistis, C., Moretti, M., Vicario, A., et al. (2018). Pro Inflammatory Stimuli Enhance the Immunosuppressive Functions of Adipose Mesenchymal Stem Cells-Derived Exosomes. Sci. Rep. 8, 13325. doi:10.1038/s41598-018-31707-9
Eisenstein, M. (2020). Inside the Stem-Cell Pharmaceutical Factory. Nature 582, S16–S18. doi:10.1038/d41586-020-01770-2
Ellis, S. G., Steffenino, G., Kereiakes, D. J., Stone, G. W., van Geuns, R. J., Abizaid, A., et al. (2017). Clinical, Angiographic, and Procedural Correlates of Acute, Subacute, and Late Absorb Scaffold Thrombosis. JACC: Cardiovasc. Interventions 10, 1809–1815. doi:10.1016/j.jcin.2017.06.067
Elnaggar, M. A., Seo, S. H., Gobaa, S., Lim, K. S., Bae, I.-H., Jeong, M. H., et al. (2016). Nitric Oxide Releasing Coronary Stent: A New Approach Using Layer-By-Layer Coating and Liposomal Encapsulation. Small 12, 6012–6023. doi:10.1002/smll.201600337
Enayati, M., Schneider, K. H., Almeria, C., Grasl, C., Kaun, C., Messner, B., et al. (2021). S-nitroso Human Serum Albumin as a Nitric Oxide Donor in Drug-Eluting Vascular Grafts: Biofunctionality and Preclinical Evaluation. Acta Biomater. 134, 276–288. doi:10.1016/j.actbio.2021.07.048
Evans, C. E., Iruela-Arispe, M. L., and Zhao, Y.-Y. (2021). Mechanisms of Endothelial Regeneration and Vascular Repair and Their Application to Regenerative Medicine. Am. J. Pathol. 191, 52–65. doi:10.1016/j.ajpath.2020.10.001
Fan, Y., Zhang, Y., Zhao, Q., Xie, Y., Luo, R., Yang, P., et al. (2019). Immobilization of Nano Cu-MOFs with Polydopamine Coating for Adaptable Gasotransmitter Generation and Copper Ion Delivery on Cardiovascular Stents. Biomaterials 204, 36–45. doi:10.1016/j.biomaterials.2019.03.007
Feldman, M. D., Sun, B., Koci, B. J., Wu, C. C., Kneller, J. F., Borovetz, H. S., et al. (2000). Stent-based Gene Therapy. J. Long Term Eff. Med. Implants 10, 47–68. doi:10.1615/jlongtermeffmedimplants.v10.i12.60
Fishbein, I., Guerrero, D. T., Alferiev, I. S., Foster, J. B., Minutolo, N. G., Chorny, M., et al. (2017). Stent-based Delivery of Adeno-Associated Viral Vectors with Sustained Vascular Transduction and iNOS-Mediated Inhibition of In-Stent Restenosis. Gene Ther. 24, 717–726. doi:10.1038/gt.2017.82
Fu, J., Su, Y., Qin, Y.-X., Zheng, Y., Wang, Y., and Zhu, D. (2020). Evolution of Metallic Cardiovascular Stent Materials: A Comparative Study Among Stainless Steel, Magnesium and Zinc. Biomaterials 230, 119641. doi:10.1016/j.biomaterials.2019.119641
Geeroms, B., Laleman, W., Laenen, A., Heye, S., Verslype, C., van der Merwe, S., et al. (2017). Expanded Polytetrafluoroethylene-Covered Stent-Grafts for Transjugular Intrahepatic Portosystemic Shunts in Cirrhotic Patients: Long-Term Patency and Clinical Outcome Results. Eur. Radiol. 27, 1795–1803. doi:10.1007/s00330-016-4570-5
Gupta, A. C., Wang, W., Shah, C., Sands, M. J., Bullen, J., Remer, E. M., et al. (2017). Added Value of Covered Stents in Transjugular Intrahepatic Portosystemic Shunt: A Large Single-Center Experience. Cardiovasc. Intervent Radiol. 40, 1723–1731. doi:10.1007/s00270-017-1694-1
Gvs, (2020). Coronary Stents Market Size & Share Report, 2020-2027. GVS. Available at: https://www.grandviewresearch.com/industry-analysis/coronary-stents-industry (accessed November 4, 2021).
Hernández-Enríquez, M., Lairez, O., Campelo-Parada, F., Lhermusier, T., Bouisset, F., Roncalli, J., et al. (2018). Outcomes after Use of Covered Stents to Treat Coronary Artery Perforations. Comparison of Old and New-Generation Covered Stents. J. Interven Cardiol. 31, 617–623. doi:10.1111/joic.12525
Hideo-Kajita, A., Garcia-Garcia, H. M., Haude, M., Joner, M., Koolen, J., Ince, H., et al. (2019). First Report of Edge Vascular Response at 12 Months of Magmaris, A Second-Generation Drug-Eluting Resorbable Magnesium Scaffold, Assessed by Grayscale Intravascular Ultrasound, Virtual Histology, and Optical Coherence Tomography. A Biosolve-II Trial Sub-study. Cardiovasc. Revascularization Med. 20, 392–398. doi:10.1016/j.carrev.2019.02.019
Hirase, T. (2016). Capturing VE-Cadherin-Positive Endothelial Progenitor Cells for In-Stent Vascular Repair. Jat 23, 46–47. doi:10.5551/jat.ED027
Hopkins, S. P., Pant, J., Goudie, M. J., Schmiedt, C., and Handa, H. (2018). Achieving Long-Term Biocompatible Silicone via Covalently Immobilized S-Nitroso-N-Acetylpenicillamine (SNAP) that Exhibits 4 Months of Sustained Nitric Oxide Release. ACS Appl. Mater. Inter. 10, 27316–27325. doi:10.1021/acsami.8b08647
Hu, S., Li, Z., Shen, D., Zhu, D., Huang, K., Su, T., et al. (2021). Exosome-eluting Stents for Vascular Healing after Ischaemic Injury. Nat. Biomed. Eng. 5, 1174–1188. doi:10.1038/s41551-021-00705-0
Hytönen, J. P., Taavitsainen, J., Laitinen, J. T. T., Partanen, A., Alitalo, K., Leppänen, O., et al. (2018). Local Adventitial Anti-angiogenic Gene Therapy Reduces Growth of Vasa-Vasorum and In-Stent Restenosis in WHHL Rabbits. J. Mol. Cell Cardiol. 121, 145–154. doi:10.1016/j.yjmcc.2018.07.007
Iglesias-Echevarria, M., Johnson, R., Rafuse, M., Ding, Y., and Tan, W. (2021). Vascular Grafts with Tailored Stiffness and a Ligand Environment via Multiarmed Polymer Sheath for Expeditious Regeneration. ACS Appl. Bio Mater. 4, 545–558. doi:10.1021/acsabm.0c01114
Jang, E.-J., Bae, I.-H., Park, D. S., Lee, S.-Y., Lim, K. S., Park, J.-K., et al. (2015). Effect of a Novel Peptide, WKYMVm- and Sirolimus-Coated Stent on Re-endothelialization and Anti-restenosis. J. Mater. Sci. Mater. Med. 26, 251. doi:10.1007/s10856-015-5585-1
Jantzen, A. E., Lane, W. O., Gage, S. M., Jamiolkowski, R. M., Haseltine, J. M., Galinat, L. J., et al. (2011). Use of Autologous Blood-Derived Endothelial Progenitor Cells at point-of-care to Protect against Implant Thrombosis in a Large Animal Model. Biomaterials 32, 8356–8363. doi:10.1016/j.biomaterials.2011.07.066
Jantzen, A. E., Noviani, M., Mills, J. S., Baker, K. M., Lin, F.-H., Truskey, G. A., et al. (2016). Point-of-care Seeding of Nitinol Stents with Blood-Derived Endothelial Cells. J. Biomed. Mater. Res. 104, 1658–1665. doi:10.1002/jbm.b.33510
Jensen, L. O., and Christiansen, E. H. (2019). Are Drug-Eluting Stents Safer Than Bare-Metal Stents? The Lancet 393, 2472–2474. doi:10.1016/S0140-6736(19)31000-1
Junkar, I., Kulkarni, M., Benčina, M., Kovač, J., Mrak-Poljšak, K., Lakota, K., et al. (2020). Titanium Dioxide Nanotube Arrays for Cardiovascular Stent Applications. ACS Omega 5, 7280–7289. doi:10.1021/acsomega.9b04118
Keighron, C., Lyons, C. J., Creane, M., O'Brien, T., and Liew, A. (2018). Recent Advances in Endothelial Progenitor Cells toward Their Use in Clinical Translation. Front. Med. 5, 354. doi:10.3389/fmed.2018.00354
Kim, S. M., Park, K.-S., Lih, E., Hong, Y. J., Kang, J. H., Kim, I. H., et al. (2016). Fabrication and Characteristics of Dual Functionalized Vascular Stent by Spatio-Temporal Coating. Acta Biomater. 38, 143–152. doi:10.1016/j.actbio.2016.04.029
Kim, Y.-H., Lee, C. W., Hong, M.-K., Park, S.-W., Tahk, S.-J., Yang, J.-Y., et al. (2005). Randomized Comparison of Carbon Ion-Implanted Stent versus Bare Metal Stent in Coronary Artery Disease: The Asian Pacific Multicenter Arthos Stent Study (PASS) Trial. Am. Heart J. 149, 336–341. doi:10.1016/j.ahj.2004.02.018
Kobo, O., Saada, M., Meisel, S. R., Hellou, E., Frimerman, A., Abu Fanne, R., et al. (2020). Modern Stents: Where Are We Going? Rambam Maimonides Med. J. 11, e0017. doi:10.5041/RMMJ.10403
Kushwaha, M., Anderson, J. M., Bosworth, C. A., Andukuri, A., Minor, W. P., Lancaster, J. R., et al. (2010). A Nitric Oxide Releasing, Self Assembled Peptide Amphiphile Matrix that Mimics Native Endothelium for Coating Implantable Cardiovascular Devices. Biomaterials 31, 1502–1508. doi:10.1016/j.biomaterials.2009.10.051
Laird, J. R., Katzen, B. T., Scheinert, D., Lammer, J., Carpenter, J., Buchbinder, M., et al. (2010). Nitinol Stent Implantation versus Balloon Angioplasty for Lesions in the Superficial Femoral Artery and Proximal Popliteal Artery. Circ. Cardiovasc. Interventions 3, 267–276. doi:10.1161/CIRCINTERVENTIONS.109.903468
Lawton, J. S., Tamis-Holland, J. E., Bangalore, S., Bates, E. R., Beckie, T. M., Bischoff, J. M., et al. (2021). 2021 ACC/AHA/SCAI Guideline for Coronary Artery Revascularization: A Report of the American College of Cardiology/American Heart Association Joint Committee on Clinical Practice Guidelines. Circulation 145 (3), e18–e114. doi:10.1161/CIR.0000000000001038
Leal, B. B. J., Wakabayashi, N., Oyama, K., Kamiya, H., Braghirolli, D. I., and Pranke, P. (2021). Vascular Tissue Engineering: Polymers and Methodologies for Small Caliber Vascular Grafts. Front. Cardiovasc. Med. 7, 376. doi:10.3389/fcvm.2020.592361
Lee, J. M., Choe, W., Kim, B.-K., Seo, W.-W., Lim, W.-H., Kang, C.-K., et al. (2012). Comparison of Endothelialization and Neointimal Formation with Stents Coated with Antibodies against CD34 and Vascular Endothelial-Cadherin. Biomaterials 33, 8917–8927. doi:10.1016/j.biomaterials.2012.08.066
Lee, P. P., and Desai, T. A. (2016). Nitinol-Based Nanotubular Arrays with Controlled Diameters Upregulate Human Vascular Cell ECM Production. ACS Biomater. Sci. Eng. 2, 409–414. doi:10.1021/acsbiomaterials.5b00553
Lee, S. J., Jo, H. H., Lim, K. S., Lim, D., Lee, S., Lee, J. H., et al. (2019). Heparin Coating on 3D Printed Poly (L-lactic Acid) Biodegradable Cardiovascular Stent via Mild Surface Modification Approach for Coronary Artery Implantation. Chem. Eng. J. 378, 122116. doi:10.1016/j.cej.2019.122116
Lee, W.-C., Hsueh, S.-K., Fang, C.-Y., Wu, C.-J., Hang, C.-L., and Fang, H.-Y. (2016). Clinical Outcomes Following Covered Stent for the Treatment of Coronary Artery Perforation. J. Interv. Cardiol. 29, 569–575. doi:10.1111/joic.12347
Leopold, J. A. (2013). Prohealing Endothelial Progenitor Cell Capture Stents. Circ. Cardiovasc. Interventions 6, 494–495. doi:10.1161/CIRCINTERVENTIONS.113.000812
Lim, S.-H., Flammer, A. J., Yoon, M. H., Lennon, R. J., Gulati, R., Mathew, V., et al. (2012). The Long-Term Effect of Coronary Stenting on Epicardial and Microvascular Endothelial Function. Circ. Cardiovasc. Interventions 5, 523–529. doi:10.1161/CIRCINTERVENTIONS.112.970111
Lim, W.-H., Seo, W.-W., Choe, W., Kang, C.-K., Park, J., Cho, H.-J., et al. (2011). Stent Coated with Antibody against Vascular Endothelial-Cadherin Captures Endothelial Progenitor Cells, Accelerates Re-endothelialization, and Reduces Neointimal Formation. Atvb 31, 2798–2805. doi:10.1161/ATVBAHA.111.226134
Lipinski, M. J., Escarcega, R. O., Baker, N. C., Benn, H. A., Gaglia, M. A., Torguson, R., et al. (2016). Scaffold Thrombosis after Percutaneous Coronary Intervention with ABSORB Bioresorbable Vascular Scaffold. JACC: Cardiovasc. Interventions 9, 12–24. doi:10.1016/j.jcin.2015.09.024
Lyu, N., Du, Z., Qiu, H., Gao, P., Yao, Q., Xiong, K., et al. (2020). Mimicking the Nitric Oxide‐Releasing and Glycocalyx Functions of Endothelium on Vascular Stent Surfaces. Adv. Sci. 7, 2002330. doi:10.1002/advs.202002330
Ma, Q., Shi, X., Tan, X., Wang, R., Xiong, K., Maitz, M. F., et al. (2021). Durable Endothelium-Mimicking Coating for Surface Bioengineering Cardiovascular Stents. Bioactive Mater. 6, 4786–4800. doi:10.1016/j.bioactmat.2021.05.009
McGinty, S., Vo, T. T. N., Meere, M., McKee, S., and McCormick, C. (2015). Some Design Considerations for Polymer-free Drug-Eluting Stents: a Mathematical Approach. Acta Biomater. 18, 213–225. doi:10.1016/j.actbio.2015.02.006
Medina, R. J., Barber, C. L., Sabatier, F., Dignat-George, F., Melero-Martin, J. M., Khosrotehrani, K., et al. (2017). Endothelial Progenitors: A Consensus Statement on Nomenclature. STEM CELLS Translational Med. 6, 1316–1320. doi:10.1002/sctm.16-0360
Medtronic (2019). Coronary ACoronary Artery Disease - what Is Balloon Angioplasty | Medtronicrtery Disease (CAD) - Balloon Angioplasty and Stenting. Medtronic. Available at: https://www.medtronic.com/us-en/patients/treatments-therapies/heart-surgery-cad/balloon-angioplasty-stenting-what-is-it.html (accessed November 4, 2021).
Meireles, G. C. X., Abreu, L. M. d., Forte, A. A. d. C., Sumita, M. K., Sumita, J. H., and Aliaga, J. D. C. S. (2007). Estudo comparativo randomizado Do implante de Stent de aço inoxidável recoberto por carbono semelhante ao diamante versus não recoberto em pacientes com doença arterial coronariana. Arq. Bras. Cardiol. 88, 390–395. doi:10.1590/s0066-782x2007000400004
Midgley, A. C., Wei, Y., Li, Z., Kong, D., and Zhao, Q. (2020). Nitric‐Oxide‐Releasing Biomaterial Regulation of the Stem Cell Microenvironment in Regenerative Medicine. Adv. Mater. 32, 1805818. doi:10.1002/adma.201805818
Migita, S., Kitano, D., Li, Y., Yamada, S., Mukaiyama, T., Onishi, A., et al. (2020). Pathology of Coronary Artery after Third-Generation Drug-Eluting Stent Implantation in Low-Density Lipoprotein Receptor Knockout Mini Pigs and Human Autopsy Cases. Atherosclerosis 315, e93. doi:10.1016/j.atherosclerosis.2020.10.287
Mori, H., Atmakuri, D. R., Torii, S., Braumann, R., Smith, S., Jinnouchi, H., et al. (2017). Very Late Pathological Responses to Cobalt-Chromium Everolimus‐Eluting, Stainless Steel Sirolimus‐Eluting, and Cobalt-Chromium Bare Metal Stents in Humans. Jaha 6, e007244. doi:10.1161/JAHA.117.007244
Nakamura, K., Keating, J. H., and Edelman, E. R. (2016). Pathology of Endovascular Stents. Interv. Cardiol. Clin. 5, 391–403. doi:10.1016/j.iccl.2016.02.006
Nakamura, T., Brott, B. C., Brants, I., Panchal, D., Li, J., Chen, J. P., et al. (2011). Vasomotor Function after Paclitaxel-Coated Balloon post-dilation in Porcine Coronary Stent Model. JACC: Cardiovasc. Interventions 4, 247–255. doi:10.1016/j.jcin.2010.08.028
Napoli, C., de Nigris, F., Williams-Ignarro, S., Pignalosa, O., Sica, V., and Ignarro, L. J. (2006). Nitric Oxide and Atherosclerosis: an Update. Nitric Oxide 15, 265–279. doi:10.1016/j.niox.2006.03.011
Neumann, F. J., Sousa-Uva, M., Ahlsson, A., Alfonso, F., Banning, A. P., Benedetto, U., et al. (2019). 2018 ESC/EACTS Guidelines on Myocardial Revascularization. Eur. Heart J. 40, 87–165. doi:10.1093/eurheartj/ehy394
NIH (2020). NIH-funded Studies Show Stents and Surgery No Better than Medication, Lifestyle Changes at Reducing Cardiac Events. (National Institutes of Health). Available at: https://www.nih.gov/news-events/news-releases/nih-funded-studies-show-stents-surgery-no-better-medication-lifestyle-changes-reducing-cardiac-events (accessed November 20, 2021).
Nikfarjam, S., Rezaie, J., Zolbanin, N. M., and Jafari, R. (2020). Mesenchymal Stem Cell Derived-Exosomes: a Modern Approach in Translational Medicine. J. Transl Med. 18, 449. doi:10.1186/s12967-020-02622-3
O'Donnell, T. F. X., Patel, V. I., Deery, S. E., Li, C., Swerdlow, N. J., Liang, P., et al. (2019). The State of Complex Endovascular Abdominal Aortic Aneurysm Repairs in the Vascular Quality Initiative. J. Vasc. Surg. 70, 369–380. doi:10.1016/j.jvs.2018.11.021
O’Brien, B., and Carroll, W. (2009). The Evolution of Cardiovascular Stent Materials and Surfaces in Response to Clinical Drivers: A Review. Acta Biomater. 5, 945–958. doi:10.1016/j.actbio.2008.11.012
Omar, W. A., and Kumbhani, D. J. (2019). The Current Literature on Bioabsorbable Stents: a Review. Curr. Atheroscler. Rep. 21, 54. doi:10.1007/s11883-019-0816-4
Onuma, Y., Grundeken, M. J., Nakatani, S., Asano, T., Sotomi, Y., Foin, N., et al. (2017). Serial 5-Year Evaluation of Side Branches Jailed by Bioresorbable Vascular Scaffolds Using 3-Dimensional Optical Coherence Tomography. Circ. Cardiovasc. Interv. 10, e004393. doi:10.1161/CIRCINTERVENTIONS.116.004393
Otsuka, F., Finn, A. V., Yazdani, S. K., Nakano, M., Kolodgie, F. D., and Virmani, R. (2012). The Importance of the Endothelium in Atherothrombosis and Coronary Stenting. Nat. Rev. Cardiol. 9, 439–453. doi:10.1038/nrcardio.2012.64
Otsuka, F., Vorpahl, M., Nakano, M., Foerst, J., Newell, J. B., Sakakura, K., et al. (2014). Pathology of Second-Generation Everolimus-Eluting Stents versus First-Generation Sirolimus- and Paclitaxel-Eluting Stents in Humans. Circulation 129, 211–223. doi:10.1161/CIRCULATIONAHA.113.001790
Pang, J. H., Farhatnia, Y., Godarzi, F., Tan, A., Rajadas, J., Cousins, B. G., et al. (2015). In Situ Endothelialization: Bioengineering Considerations to Translation. Small 11, 6248–6264. doi:10.1002/smll.201402579
Park, C., Park, K. S., Jeong, M. J., Kim, H. B., Bae, I., Lim, K. S., et al. (2021). A Robustly Supported Extracellular Matrix Improves the Intravascular Delivery Efficacy of Endothelial Progenitor Cells. Adv. Funct. Mater. 31, 2100324. doi:10.1002/adfm.202100324
Putzu, M., Causa, F., Parente, M., González de Torre, I., Rodriguez-Cabello, J. C., and Netti, P. A. (2019). Silk-ELR Co-recombinamer Covered Stents Obtained by Electrospinning. Regenerative Biomater. 6, 21–28. doi:10.1093/rb/rby022
Qiu, H., Qi, P., Liu, J., Yang, Y., Tan, X., Xiao, Y., et al. (2019). Biomimetic Engineering Endothelium-like Coating on Cardiovascular Stent through Heparin and Nitric Oxide-Generating Compound Synergistic Modification Strategy. Biomaterials 207, 10–22. doi:10.1016/j.biomaterials.2019.03.033
Raina, T., Iqbal, J., Arnold, N., Moore, H., Aflatoonian, B., Walsh, J., et al. (2014). Coronary Stents Seeded with Human Trophoblastic Endovascular Progenitor Cells Show Accelerated Strut Coverage without Excessive Neointimal Proliferation in a Porcine Model. EuroIntervention 10, 709–716. doi:10.4244/EIJV10I6A123
Rao, J., Pan Bei, H., Yang, Y., Liu, Y., Lin, H., and Zhao, X. (2020). Nitric Oxide-Producing Cardiovascular Stent Coatings for Prevention of Thrombosis and Restenosis. Front. Bioeng. Biotechnol. 8, 578. doi:10.3389/fbioe.2020.00578
Reuters (2019). Stents No Better than Drugs for many Heart Patients. U.S. study, Reuters. Available at: https://www.reuters.com/article/us-health-heart-stent-idUSKBN1XQ0MR (accessed November 4, 2021).
Rizk, T., Patel, D., Young, E., Ramakrishnan, V., and Mansour, K. (2020). Multidisciplinary Management of Subclavian Artery Perforation and Complications. Cureus 12, e8009. doi:10.7759/cureus.8009
Ruffino, M. A., Fronda, M., Varello, S., Discalzi, A., Mancini, A., Muratore, P., et al. (2020). Emergency Management of Iatrogenic Arterial Injuries with a Low-Profile Balloon-Expandable Stent-Graft: Preliminary Results. Medicine (Baltimore) 99, e19655. doi:10.1097/MD.0000000000019655
Saleh, Y. E., Gepreel, M. A., and Allam, N. K. (2017). Functional Nanoarchitectures for Enhanced Drug Eluting Stents. Sci. Rep. 7, 40291. doi:10.1038/srep40291
Scafa Udriște, A., Niculescu, A.-G., Grumezescu, A. M., and Bădilă, E. (2021). Cardiovascular Stents: A Review of Past, Current, and Emerging Devices. Materials 14, 2498. doi:10.3390/ma14102498
Schillinger, M., Sabeti, S., Loewe, C., Dick, P., Amighi, J., Mlekusch, W., et al. (2006). Balloon Angioplasty versus Implantation of Nitinol Stents in the Superficial Femoral Artery. N. Engl. J. Med. 354, 1879–1888. doi:10.1056/NEJMoa051303
Sedaghat, A., Sinning, J.-M., Paul, K., Kirfel, G., Nickenig, G., and Werner, N. (2013). First In Vitro and In Vivo Results of an Anti-human CD133-Antibody Coated Coronary Stent in the Porcine Model. Clin. Res. Cardiol. 102, 413–425. doi:10.1007/s00392-013-0547-4
Serruys, P. W., Chevalier, B., Sotomi, Y., Cequier, A., Carrié, D., Piek, J. J., et al. (2016). Comparison of an Everolimus-Eluting Bioresorbable Scaffold with an Everolimus-Eluting Metallic Stent for the Treatment of Coronary Artery Stenosis (ABSORB II): a 3 year, Randomised, Controlled, Single-Blind, Multicentre Clinical Trial. The Lancet 388, 2479–2491. doi:10.1016/S0140-6736(16)32050-5
Serruys, P. W., Katagiri, Y., Sotomi, Y., Zeng, Y., Chevalier, B., van der Schaaf, R. J., et al. (2017). Arterial Remodeling after Bioresorbable Scaffolds and Metallic Stents. J. Am. Coll. Cardiol. 70, 60–74. doi:10.1016/j.jacc.2017.05.028
Sethi, R., and Lee, C.-H. (2012). Endothelial Progenitor Cell Capture Stent: Safety and Effectiveness. J. Interv. Cardiol. 25, 493–500. doi:10.1111/j.1540-8183.2012.00740.x
Sharif, F., Hynes, S. O., McCullagh, K. J. A., Ganley, S., Greiser, U., McHugh, P., et al. (2012). Gene-eluting Stents: Non-viral, Liposome-Based Gene Delivery of eNOS to the Blood Vessel wall In Vivo Results in Enhanced Endothelialization but Does Not Reduce Restenosis in a Hypercholesterolemic Model. Gene Ther. 19, 321–328. doi:10.1038/gt.2011.92
Shi, H.-J., Cao, A.-H., Chen, J., Deng, G., and Teng, G.-J. (2010). Transjugular Intrahepatic Portosystemic Shunt with an Autologous Endothelial Progenitor Cell Seeded Stent:. Acad. Radiol. 17, 358–367. doi:10.1016/j.acra.2009.10.007
Shlofmitz, E., Iantorno, M., and Waksman, R. (2019). Restenosis of Drug-Eluting Stents. Circ. Cardiovasc. Interventions 12, e007023. doi:10.1161/CIRCINTERVENTIONS.118.007023
Simsekyilmaz, S., Liehn, E. A., Weinandy, S., Schreiber, F., Megens, R. T. A., Theelen, W., et al. (2016). Targeting In-Stent-Stenosis with RGD- and CXCL1-Coated Mini-Stents in Mice. PLOS ONE 11, e0155829. doi:10.1371/journal.pone.0155829
Song, X., Qin, Q., Chang, S., Xu, R., Fu, M., Lu, H., et al. (2021). Clinical Outcomes of Self-Made Polyurethane-Covered Stent Implantation for the Treatment of Coronary Artery Perforations. J. Interv. Cardiol. 2021, 1–9. doi:10.1155/2021/6661763
Stone, G. W., Kimura, T., Gao, R., Kereiakes, D. J., Ellis, S. G., Onuma, Y., et al. (2019). Time-Varying Outcomes with the Absorb Bioresorbable Vascular Scaffold during 5-Year Follow-Up. JAMA Cardiol. 4, 1261–1269. doi:10.1001/jamacardio.2019.4101
Strijdom, H., Chamane, N., and Lochner, A. (2009). Nitric Oxide in the Cardiovascular System: a Simple Molecule with Complex Actions. Cardiovasc. J. Afr. 20, 303–310.
Suh, J. H., Joo, H. S., Hong, E. B., Lee, H. J., and Lee, J. M. (2021). Therapeutic Application of Exosomes in Inflammatory Diseases. Ijms 22, 1144. doi:10.3390/ijms22031144
Swanson, N., Hogrefe, K., Javed, Q., Malik, N., and Gershlick, A. H. (2004). Vascular Endothelial Growth Factor (VEGF)-Eluting Stents: In Vivo Effects on Thrombosis, Endothelialization and Intimal Hyperplasia. J. Invasive Cardiol. 15, 688–692.
Takabatake, S., Hayashi, K., Nakanishi, C., Hao, H., Sakata, K., Kawashiri, M.-A., et al. (2014). Vascular Endothelial Growth Factor-Bound Stents: Application of In Situ Capture Technology of Circulating Endothelial Progenitor Cells in Porcine Coronary Model. J. Int. Cardiol. 27, 63–72. doi:10.1111/joic.12087
Tang, H., Wang, Q., Wang, X., Zhou, J., Zhu, M., Qiao, T., et al. (2016). Effect of a Novel Stent on Re-endothelialization, Platelet Adhesion, and Neointimal Formation. Jat 23, 67–80. doi:10.5551/jat.31062
Taniwaki, M., Stefanini, G. G., Silber, S., Richardt, G., Vranckx, P., Serruys, P. W., et al. (2014). 4-Year Clinical Outcomes and Predictors of Repeat Revascularization in Patients Treated with New-Generation Drug-Eluting Stents. J. Am. Coll. Cardiol. 63, 1617–1625. doi:10.1016/j.jacc.2013.12.036
Tsukada, J., Mela, P., Jinzaki, M., Tsukada, H., Schmitz-Rode, T., and Vogt, F. (2021). Development of In Vitro Endothelialised Stents - Review. Stem Cel Rev Rep. 1, 1. doi:10.1007/s12015-021-10238-3
Unverdorben, M., Sippel, B., Degenhardt, R., Sattler, K., Fries, R., Abt, B., et al. (2003). Comparison of a Silicon Carbide-Coated Stent versus a Noncoated Stent in Human Beings: The Tenax versus Nir Stent Study's Long-Term Outcome. Am. Heart J. 145, e17. doi:10.1067/mhj.2003.90
Van Belle, E., Maillard, L., Tio, F. O., and Isner, J. M. (1997). Accelerated Endothelialization by Local Delivery of Recombinant Human Vascular Endothelial Growth Factor Reduces In-Stent Intimal Formation. Biochem. Biophysical Res. Commun. 235, 311–316. doi:10.1006/bbrc.1997.6772
van Beusekom, H. M. M., Ertaş, G., Sorop, O., Serruys, P. W., and van der Giessen, W. J. (2012). The Genous Endothelial Progenitor Cell Capture Stent Accelerates Stent Re-endothelialization but Does Not Affect Intimal Hyperplasia in Porcine Coronary Arteries. Cathet. Cardiovasc. Intervent. 79, 231–242. doi:10.1002/ccd.22928
van den Heuvel, M., Sorop, O., Batenburg, W. W., Bakker, C. L., de Vries, R., Koopmans, S. J., et al. (2010). Specific Coronary Drug-Eluting Stents Interfere with Distal Microvascular Function after Single Stent Implantation in Pigs. JACC: Cardiovasc. Interventions 3, 723–730. doi:10.1016/j.jcin.2010.05.003
Virani, S. S., Alonso, A., Benjamin, E. J., Bittencourt, M. S., Callaway, C. W., Carson, A. P., et al. (2020). Heart Disease and Stroke Statistics-2020 Update: A Report from the American Heart Association. Circulation 141, e139–e596. doi:10.1161/CIR.0000000000000757
Wang, H. J., Lin, J. J., Lo, W. Y., Chang, C. P., Hsu, C. H., Hsieh, L. C., et al. (2017). Clinical Outcomes of Polytetrafluoroethylene-Covered Stents for Coronary Artery Perforation in Elderly Patients Undergoing Percutaneous Coronary Interventions. Acta Cardiol. Sin 33, 605–613. doi:10.6515/ACS20170625A
Wang, J., Qian, H.-L., Chen, S.-Y., Huang, W.-P., Huang, D.-N., Hao, H.-Y., et al. (2021). miR-22 Eluting Cardiovascular Stent Based on a Self-Healable Spongy Coating Inhibits In-Stent Restenosis. Bioactive Mater. 6, 4686–4696. doi:10.1016/j.bioactmat.2021.04.037
Wang, J., Xue, Y., Liu, J., Hu, M., Zhang, H., Ren, K., et al. (2020). Hierarchical Capillary Coating to Biofunctionlize Drug-Eluting Stent for Improving Endothelium Regeneration. Research 2020, 1–14. doi:10.34133/2020/1458090
Wang, Y., Lan, H., Yin, T., Zhang, X., Huang, J., Fu, H., et al. (2020). Covalent Immobilization of Biomolecules on Stent Materials through Mussel Adhesive Protein Coating to Form Biofunctional Films. Mater. Sci. Eng. C. 106, 110187. doi:10.1016/j.msec.2019.110187
Wańha, W., Januszek, R., Kołodziejczak, M., Kuźma, Ł., Tajstra, M., Figatowski, T., et al. (2021). Procedural and 1-year Outcomes Following Large Vessel Coronary Artery Perforation Treated by Covered Stents Implantation: Multicentre CRACK Registry. PLOS ONE 16, e0249698. doi:10.1371/journal.pone.0249698
Wawrzyńska, M., Duda, M., Wysokińska, E., Strządała, L., Biały, D., Ulatowska-Jarża, A., et al. (2019). Functionalized CD133 Antibody Coated Stent Surface Simultaneously Promotes EPCs Adhesion and Inhibits Smooth Muscle Cell Proliferation-A Novel Approach to Prevent In-Stent Restenosis. Colloids Surf. B: Biointerfaces 174, 587–597. doi:10.1016/j.colsurfb.2018.11.061
Wei, W., Ao, Q., Wang, X., Cao, Y., Liu, Y., Zheng, S. G., et al. (2021). Mesenchymal Stem Cell-Derived Exosomes: A Promising Biological Tool in Nanomedicine. Front. Pharmacol. 11, 1954. doi:10.3389/fphar.2020.590470
Wei, Y., Ji, Y., Xiao, L.-L., Lin, Q.-k., Xu, J.-p., Ren, K.-f., et al. (2013). Surface Engineering of Cardiovascular Stent with Endothelial Cell Selectivity for In Vivo Re-endothelialisation. Biomaterials 34, 2588–2599. doi:10.1016/j.biomaterials.2012.12.036
Who, (2021). Cardiovascular Diseases (CVDs). WHO. Available at: https://www.who.int/news-room/fact-sheets/detail/cardiovascular-diseases-(cvds (accessed November 4, 2021).
Wiemer, M., Stoikovic, S., Samol, A., Dimitriadis, Z., Ruiz-Nodar, J. M., Birkemeyer, R., et al. (2017). Third Generation Drug Eluting Stent (DES) with Biodegradable Polymer in Diabetic Patients: 5 Years Follow-Up. Cardiovasc. Diabetol. 16, 23. doi:10.1186/s12933-017-0500-3
Windecker, S., Simon, R., Lins, M., Klauss, V., Eberli, F. R., Roffi, M., et al. (2005). Randomized Comparison of a Titanium-Nitride-Oxide-Coated Stent with a Stainless Steel Stent for Coronary Revascularization. Circulation 111, 2617–2622. doi:10.1161/CIRCULATIONAHA.104.486647
Winther, A. K., Fejerskov, B., ter Meer, M., Jensen, N. B. S., Dillion, R., Schaffer, J. E., et al. (2018). Enzyme Prodrug Therapy Achieves Site-specific, Personalized Physiological Responses to the Locally Produced Nitric Oxide. ACS Appl. Mater. Inter. 10, 10741–10751. doi:10.1021/acsami.8b01658
Worthley, S. G., Abizaid, A., Kirtane, A. J., Simon, D. I., Windecker, S., Brar, S., et al. (2017). First-in-Human Evaluation of a Novel Polymer-free Drug-Filled Stent. JACC: Cardiovasc. Interventions 10, 147–156. doi:10.1016/j.jcin.2016.10.020
Wu, X., Yin, T., Tian, J., Tang, C., Huang, J., Zhao, Y., et al. (2015). Distinctive Effects of CD34- and CD133-specific Antibody-Coated Stents on Re-endothelialization and In-Stent Restenosis at the Early Phase of Vascular Injury. Regenerative Biomater. 2, 87–96. doi:10.1093/rb/rbv007
Yamaji, K., Kimura, T., Morimoto, T., Nakagawa, Y., Inoue, K., Soga, Y., et al. (2010). Very Long-Term (15 to 20 years) Clinical and Angiographic Outcome after Coronary Bare Metal Stent Implantation. Circ. Cardiovasc. Interventions 3, 468–475. doi:10.1161/CIRCINTERVENTIONS.110.958249
Yamaji, K., Räber, L., Zanchin, T., Spitzer, E., Zanchin, C., Pilgrim, T., et al. (2016). Ten-year Clinical Outcomes of First-Generation Drug-Eluting Stents: the Sirolimus-Eluting vs. Paclitaxel-Eluting Stents for Coronary Revascularization (SIRTAX) VERY LATE Trial. Eur. Heart J. 37, 3386–3395. doi:10.1093/eurheartj/ehw343
Yang, D., Yan, W., Qiu, J., Huang, Y., Li, T., Wang, Y., et al. (2020). Mussel Adhesive Protein Fused with VE‐cadherin Extracellular Domain Promotes Endothelial‐cell Tight Junctions and In Vivo Endothelization Recovery of Vascular Stent. J. Biomed. Mater. Res. 108, 94–103. doi:10.1002/jbm.b.34369
Yang, F., Chang, R., and Webster, T. (2019). Atomic Layer Deposition Coating of TiO2 Nano-Thin Films on Magnesium-Zinc Alloys to Enhance Cytocompatibility for Bioresorbable Vascular Stents. Ijn 14, 9955–9970. doi:10.2147/IJN.S199093
Yang, J., Zeng, Y., Zhang, C., Chen, Y.-X., Yang, Z., Li, Y., et al. (2013). The Prevention of Restenosis In Vivo with a VEGF Gene and Paclitaxel Co-eluting Stent. Biomaterials 34, 1635–1643. doi:10.1016/j.biomaterials.2012.11.006
Yang, L., Wu, H., Lu, L., He, Q., Xi, B., Yu, H., et al. (2021). A Tailored Extracellular Matrix (ECM) - Mimetic Coating for Cardiovascular Stents by Stepwise Assembly of Hyaluronic Acid and Recombinant Human Type III Collagen. Biomaterials 276, 121055. doi:10.1016/j.biomaterials.2021.121055
Yang, Y., Gao, P., Wang, J., Tu, Q., Bai, L., Xiong, K., et al. (2020). Endothelium-Mimicking Multifunctional Coating Modified Cardiovascular Stents via a Stepwise Metal-Catechol-(Amine) Surface Engineering Strategy. Research 2020, 1–20. doi:10.34133/2020/9203906
Yang, Z., Yang, Y., Zhang, L., Xiong, K., Li, X., Zhang, F., et al. (2018). Mussel-inspired Catalytic Selenocystamine-Dopamine Coatings for Long-Term Generation of Therapeutic Gas on Cardiovascular Stents. Biomaterials 178, 1–10. doi:10.1016/j.biomaterials.2018.06.008
Yang, Z., Zhao, X., Hao, R., Tu, Q., Tian, X., Xiao, Y., et al. (2020). Bioclickable and Mussel Adhesive Peptide Mimics for Engineering Vascular Stent Surfaces. Proc. Natl. Acad. Sci. USA 117, 16127–16137. doi:10.1073/pnas.2003732117
Yuan, W., Xia, D., Wu, S., Zheng, Y., Guan, Z., and Rau, J. V. (2022). A Review on Current Research Status of the Surface Modification of Zn-Based Biodegradable Metals. Bioactive Mater. 7, 192–216. doi:10.1016/j.bioactmat.2021.05.018
Zaman, A., de Winter, R. J., Kogame, N., Chang, C. C., Modolo, R., Spitzer, E., et al. (2019). Safety and Efficacy of a Sirolimus-Eluting Coronary Stent with Ultra-thin Strut for Treatment of Atherosclerotic Lesions (TALENT): a Prospective Multicentre Randomised Controlled Trial. The Lancet 393, 987–997. doi:10.1016/S0140-6736(18)32467-X
Zeng, C.-Y., Xu, J., Liu, X., and Lu, Y.-Q. (2021). Cardioprotective Roles of Endothelial Progenitor Cell-Derived Exosomes. Front. Cardiovasc. Med. 8, 717536. doi:10.3389/fcvm.2021.717536
Zhang, B., Yao, R., Hu, C., Maitz, M. F., Wu, H., Liu, K., et al. (2021). Epigallocatechin Gallate Mediated sandwich-like Coating for Mimicking Endothelium with Sustained Therapeutic Nitric Oxide Generation and Heparin Release. Biomaterials 269, 120418. doi:10.1016/j.biomaterials.2020.120418
Zhang, F., Zhang, Q., Li, X., Huang, N., Zhao, X., and Yang, Z. (2019). Mussel-inspired Dopamine-CuII Coatings for Sustained In Situ Generation of Nitric Oxide for Prevention of Stent Thrombosis and Restenosis. Biomaterials 194, 117–129. doi:10.1016/j.biomaterials.2018.12.020
Zhang, S., Zhang, F., Feng, B., Fan, Q., Yang, F., Shang, D., et al. (2015). Hematopoietic Stem Cell Capture and Directional Differentiation into Vascular Endothelial Cells for Metal Stent-Coated Chitosan/hyaluronic Acid Loading CD133 Antibody. Tissue Eng. A 21, 1173–1183. doi:10.1089/ten.TEA.2014.0352
Zhu, T., Zhou, M., Gao, W., Fang, D., Liu, Z., Wu, G., et al. (2020). Coronary Stents Decorated by Heparin/NONOate Nanoparticles for Anticoagulant and Endothelialized Effects. Langmuir 36, 2901–2910. doi:10.1021/acs.langmuir.0c00112
Keywords: stent, selective regeneration, reendothelialization, restenosis, bioabsorable
Citation: Selvakumar PP, Rafuse MS, Johnson R and Tan W (2022) Applying Principles of Regenerative Medicine to Vascular Stent Development. Front. Bioeng. Biotechnol. 10:826807. doi: 10.3389/fbioe.2022.826807
Received: 01 December 2021; Accepted: 17 January 2022;
Published: 07 March 2022.
Edited by:
J. Mary Murphy, National University of Ireland Galway, IrelandReviewed by:
Katsuhiro Hosoyama, Iwate Prefectural Central Hospital, JapanMarie-noelle Giraud, Université de Fribourg, Switzerland
Copyright © 2022 Selvakumar, Rafuse, Johnson and Tan. This is an open-access article distributed under the terms of the Creative Commons Attribution License (CC BY). The use, distribution or reproduction in other forums is permitted, provided the original author(s) and the copyright owner(s) are credited and that the original publication in this journal is cited, in accordance with accepted academic practice. No use, distribution or reproduction is permitted which does not comply with these terms.
*Correspondence: Wei Tan, d3RhbkBjb2xvcmFkby5lZHU=