- 1Key Laboratory of Carbohydrate Chemistry and Biotechnology, Ministry of Education, School of Biotechnology, Jiangnan University, Wuxi, China
- 2Yancheng Juheng Road Primary School, Yancheng, China
- 3Wuxi School of Medicine, Jiangnan University, Wuxi, China
(R)-p-chlorophenyl-1,2-ethanediol (pCPED) is an important intermediate for the synthesis of (R)-eliprodil that is widely applied in the treatment of ischemic stroke. To prepare (R)-pCPED with high enantiomeric excess (eep) and yield via the enantioconvergent hydrolysis of racemic styrene oxide (rac-pCSO) at high concentration, the bi-enzymatic catalysis was designed and investigated by a pair of epoxide hydrolases, a mutant (PvEH1Z4X4-59) of Phaseolus vulgaris EH1 and a mutant (RpEHF361V) of Rhodotorula paludigena RpEH. Firstly, the maximum allowable concentration of rac-pCSO was confirmed. Subsequently, the addition mode and the weight ratio of two Escherichia coli cells were optimized. Finally, under the optimized reaction conditions—the cell weight ratio 20:1 of E. coli/pveh1z4x4-59 to E. coli/rpehF361V, a simultaneous addition mode, and reaction temperature at 25°C—300 mM rac-pCSO in the 100 ml 4% (v/v) Tween-20/phosphate buffer system (100 mM, pH 7.0) was completely hydrolyzed within 5 h, affording (R)-pCPED with 87.8% eep, 93.4% yield, and 8.63 g/L/h space–time yield (STY). This work would be an efficient technical strategy for the preparation of chiral vicinal diols at industrial scale.
Introduction
Highly value-added chiral chemicals, such as epoxides and/or their corresponding vicinal diols, are versatile chiral building blocks applied in pharmaceuticals, fine chemicals, and agrochemical industries due to the fact that they can perform various chemical reactions with nucleophiles, electrophiles, acids, and bases (Zou et al., 2018). For example, (R)-pCSO is an important intermediate for the synthesis of rimonabant, an antagonist of the cannabinoid CB1 receptor (CB1R), and (R)-p-chlorophenyl-1,2-ethanediol (pCPED) for the synthesis of (R)-eliprodil, a quite promising neuroprotective agent applied in the treatment of ischemic stroke (Di Fabio et al., 1995; Saini and Sareen, 2017). Interestingly, it has been demonstrated that the biological activity of eliprodil in vivo is essentially associated with its (R)-(−)-enantiomer rather than its (S)-(+)-enantiomer and that the pharmacodynamic properties of the two enantiomers are different (Di Fabio et al., 1995). With increasing environment awareness, the biocatalysis using the whole cells or enzymes, an environmental-friendly process with high stereoselectivity and little or no byproducts, has attracted much attention in recent years (Kotik et al., 2013; Patel, 2018). Epoxide hydrolases (EHs, EC 3.3.2.-), which widely exist in microorganisms, plants, invertebrates, and mammals, can stereoselectively catalyze the opening of an active three-membered oxirane ring of racemic epoxides, retaining epoxide enantiomers and/or producing enantiopure vicinal diols (Woo et al., 2015). Based on the catalytic mechanisms of the given EH–epoxide pairs, the asymmetric hydrolysis of rac-epoxides can be divided into two pathways: kinetic resolution and enantioconvergent hydrolysis (Bala and Chimni, 2010). Compared with the kinetic resolution having an intrinsic limitation of 50% maximum yield of epoxide enantiomers, the enantioconvergent hydrolysis can completely convert rac-epoxides into chiral vicinal diols with up to 100% theoretical yield (Wu et al., 2015).
The enantioconvergent hydrolysis of rac-epoxides by mono-enzymatic catalysis is an ideal process for preparing desired chiral diols, whereas few EHs have high and complementary regioselectivities on (S)- and (R)-enantiomers leading to high enantiomeric excess values of chiral diols (eep) (Li et al., 2019a). As numerous EHs with enantio- and/or regio-selectivity have been characterized, bi-enzymatic catalysis has been designed to increase the concentration of epoxides and productivity of chiral diols with high eep by screening pairs of EHs and reaction systems, and optimizing reaction conditions (Hu et al., 2016). For example, using the immobilized StEH with the immobilized AnEH possessing complementary enantio- and regio-selectivity in a sequential addition mode, 4 mM rac-pCSO was completely and convergently converted into (R)-pCPED with 89% eep in 2.65 h (Karboune et al., 2005). However, the low catalytic efficiency and substrate or product inhibition limited the practical application of EHs (Deregnaucourt et al., 2007). Therefore, to break through these bottlenecks, one of the effective strategies is to screen pairs of EHs and to improve their catalytic performance.
In our previous studies, a PvEH1-encoding gene (pveh1, GenBank accession no: KR604729) was cloned and expressed in Escherichia coli BL21(DE3) (Ye et al., 2016). Then, its mutant PvEH1Z4X4-59 towards rac-pCSO was studied. The catalytic performance analysis indicated that PvEH1Z4X4-59, exhibiting high preference towards (S)-pCSO and moderate complementary regioselectivity towards (S)-pCSO (αS = 94.5%) and (R)-pCSO (βR = 80%), can catalyze the hydrolysis of rac-pCSO (10 mM) in an enantioconvergent way, affording (R)-pCPED with 83.3% eep at 100% conversion ratio (c) in 24 h. However, its unsatisfactory regioselectivity coefficient βR (80%) led to low eep of (R)-pCPED, while low substrate concentration led to low catalytic efficiency. In this work, by screening our “in house” available epoxide hydrolases, RpEHF361V, a single site mutant of RpEH from Rhodotorula paludigena, was selected, which was enantio-complementary to PvEH1Z4X4-59. RpEHF361V, preferentially hydrolyzing the (R)-pCSO with a main attack at a β-carbon atom (βR = 93%), can enantioselectively hydrolyze rac-pCSO at high concentration (800 mM), affording (R)-pCPED and retaining (S)-pCSO with over 99% ees and 43.2% yield at 55.5% c in 12 h. Then, the bi-enzymatic catalysis by using the two complementary EHs of PvEH1Z4X4-59 and RpEHF361V was designed for the enantioconvergent hydrolysis of rac-pCSO at high concentration. Additionally, the addition mode and the weight ratio of two recombinant E. coli cells expressing PvEH1Z4X4-59 and RpEHF361V, respectively, were optimized to prepare (R)-pCPED with high eep, yield, and space–time yield (STY).
Materials and Methods
Plasmids, Strains, and Chemicals
Both recombinant plasmids (pET-28a-pveh1z4x4-59 and pET-28a-rpehF361V) and EH-expressing E. coli transformants (E. coli/pveh1z4x4-59 and E. coli/rpehF361V) were constructed and preserved in our lab (Hu et al., 2020b; Xu et al., 2020). The transformants were grown in Luria–Bertani (LB) medium and induced by isopropyl-β-D-thiogalactoside (IPTG). Rac-pCSO, (S)-pCPED, and (R)-pCPED (Energy, Shanghai, China) were used for the assays of activities and regioselectivity coefficients of EHs. All other chemicals were of analytical grade.
EH Expression of E. Coli Transformants and EH Activity Assay
Single colonies of E. coli transformants, such as E. coli/pveh1z4x4-59 and/rpehF361V were separately inoculated into the LB medium containing 100 μg/ml kanamycin and cultured at 37°C for 12–14 h as the seed culture. Then, 1% (v/v) seed culture was inoculated into the fresh LB medium and cultured for 3–4 h until the OD600 value reached 0.6–0.8. After inducing with 0.05 mM IPTG at 20°C for 10 h, the E. coli transformant cells were harvested by centrifugation (8,000 rpm for 5 min, 4°C) and resuspended in 100 mM Na2HPO4-NaH2PO4 buffer (pH 7.0) to a fixed concentration of 100 mg wet cells/ml with nearly 83.3% moisture content of the wet cells, unless stated otherwise. The cell suspension was used as the biocatalyst. Comparatively, E. coli BL21(DE3) transformed with pET-28a, designated E. coli/pET-28a, was used as the negative control.
The catalytic activities of PvEH1Z4X4-59 and RpEHF361V towards rac-pCSO were measured as previously described (Li et al., 2019b), with slight modification. In detail, 100 μl cell suspension was mixed with 25 μl 200 mM rac-pCSO dissolved in methanol (at a final concentration of 10 mM) and 375 μl 100 mM Na2HPO4-NaH2PO4 buffer (pH 7.0), incubated at 25°C for 10 min, and terminated by the addition of 2 ml methanol. The reaction sample was analyzed by high-performance liquid chromatography (HPLC), using a Waters e2695 apparatus (Waters, Milford, MA) equipped with an XBridge BEH C18 column. The mobile phase of methanol/H2O (7:3, v/v) was used at a flow rate of 0.8 ml/min and monitored using a 2489 UV–Vis detector at 220 nm. One activity unit (U) of PvEH1Z4X4-59 or RpEHF361V was defined as the mount of whole cells of E. coli/pveh1z4x4-59 or/rpehF361V hydrolyzing 1 μmol rac-pCSO per minute under the given assay conditions (at pH 7.0 and 25°C for 10 min).
Regioselectivity Coefficients Assay
The EHs regioselectivity coefficients, αS (or βS = 1 − αS) and βR (or αR = 1 − βR), were applied to evaluate the probabilities attacking on Cα (a more hindered benzylic carbon in the oxirane ring) of (S)-enantiomer and on Cβ (a less hindered terminal carbon) of (R)-enantiomer, respectively. In this study, the regioselectivity assay was carried out as follows: 100 μl cell suspension was mixed with 50 μl 200 mM rac-pCSO and 850 μl 100 mM Na2HPO4-NaH2PO4 buffer (pH 7.0) and incubated at 25°C. During the hydrolytic process, aliquots of 50 μl reaction samples were drawn out, extracted with 1 ml ethyl acetate and assayed by HPLC equipped with an AS-H column (Daicel, Osaka, Japan) under the same assay conditions as described above, except for the mobile phase n-hexane/isopropanol (8:2, v/v). The conversion ratio (c value) of rac-pCSO was defined as the percentage of its consumed amount to initial one, while the yield of (R)-pCPED was referred as the percentage of its generated amount to initial amount of rac-pCSO. The ees of (S)-pCSO and eep of (R)-pCPED were calculated according to the following equations: ees = [(Ss − Rs)/(Rs + Ss)] × 100% and eep = [(Rp − Sp)/(Rp + Sp)] × 100%, in which Rs and Ss represent the concentrations of (R)- and (S)-pCSO, respectively, and Rp and Sp the concentrations of (R)- and (S)-pCPED (Li et al., 2019c). Additionally, the “final eep” was defined as eep calculated from the formula above at 100% c value. The αS and βR values of PvEH1Z4X4-59 and RpEHF361V towards (S)- and (R)-pCSO can be obtained by linear regression: eep = (αS + βR − 1) + [(βR − αS) × ees × (1 − c)/c].
Enantioconvergent Hydrolysis of rac-pCSO at Elevated Concentrations by E. Coli/pveh1z4x4-59
The enantioconvergent hydrolytic reactions, in the final volume of 2 ml 4% (v/v) Tween-20/phosphate buffer system (100 mM, pH 7.0), of rac-pCSO at concentrations ranging from 50 to 250 mM, were carried out, respectively, at 25°C using 200 mg/ml wet cells of E. coli/pveh1z4x4-59. Aliquots of 100 μl reaction samples were drawn out at 12 and 24 h and analyzed by HPLC under the same conditions as described above. Using the c and eep values as the criteria, the maximum allowable concentration (MAC) of rac-pCSO was confirmed.
Scale-Up Kinetic Resolution of rac-pCSO by E. Coli/rpehF361V
The enantioselective hydrolytic reactions, in seven aliquots of 2 ml 4% (v/v) Tween-20/phosphate buffer (100 mM, pH 7.0) system consisting of 40 mg/ml wet cells of E. coli/rpehF361V and rac-pCSO at elevated concentrations from 300 to 900 mM, were conducted, respectively, at 25°C for 12 h and analyzed by chiral HPLC. Using the c value of rac-pCSO and ees value of (S)-pCSO as the criteria, the MAC of rac-pCSO was confirmed. The gram-scale kinetic resolution of rac-pCSO at MAC in the 30 ml Tween-20/phosphate buffer system was conducted until the ees of (S)-pCSO reached over 99%.
Enantioconvergent Hydrolysis of rac-pCSO at Elevated Concentrations by E. Coli/pveh1z4x4-59 and E. Coli/rpehF361V
Using the c and eep values as the criteria, enantioconvergent hydrolytic reations of rac-pCSO at concentrations ranging from 100 to 800 mM were carried out in the 2 ml 4% (v/v) Tween-20/phosphate buffer system (100 mM, pH 7.0), using 200 mg/ml wet cells of E. coli/pveh1z4x4-59 and 40 mg/ml wet cells of E. coli/rpehF361V at 25°C for 12 h to conform the MAC of rac-pCSO.
Optimization of the Addition Mode and the Weight Ratio of Two E. Coli Cells
One addition mode of PvEH1z4x4-59 and RpEHF361V, in a sequential way, was used for the enantioconvergent hydrolysis of rac-pCSO (300 mM). In brief, 200 mg wet cells of E. coli/pveh1z4x4-59 or 40 mg wet cells of E. coli/rpehF361V was suspended in 1 ml 4% (v/v) Tween-20/phosphate buffer system (100 mM, pH 7.0) described above, while the initial concentration of rac-pCSO was 300 mM, and the system was incubated at 25°C until (S)-pCSO or (R)-pCSO was completely hydrolyzed, then 40 mg wet cells of E. coli/pveh1Z4X4-59 or 200 mg wet cells of E. coli/pveh1z4x4-59 was added. In a simultaneous way, 200 mg wet cells of E. coli/pveh1z4x4-59 and 40 mg wet cells of E. coli/rpehF361V were added at the beginning stage.
Based on the optimized addition mode, the weight ratios of E. coli/pveh1z4x4-59 to/rpehF361V from 5:1 to 200:1 were analyzed for the enantioconvergent hydrolysis of rac-pCSO. In a nutshell, the wet cells weight of E. coli/pveh1z4x4-59 was 200 mg, and that of E. coli/rpehF361V was ranging from 40 to 1 mg. The c value, eep, yield, and the STY (g/L/h) were used as evaluation criteria to get the optimal reaction conditions. STY and the average turnover frequency (aTOF) were calculated by using the following equations: STY(g/L/h) = Cp/t, aTOF(g/g/h) = Cp/(t × Ce), in which Cp was the concentration of (R)-pCPED (g/L), t was the reaction time, and Ce was the cell concentration (g/L).
Gram-Scale Enantioconvergent Hydrolysis of rac-pCSO Under the Optimal Reaction Conditions
Using the c and eep values as the criteria, the optimal reaction conditions were first confirmed. Subsequently, the scale-up enantioconvergent hydrolysis of rac-pCSO, in the 100 ml 4% (v/v) Tween-20/phosphate buffer system (100 mM, pH 7.0) containing 200 mg wet cells of E. coli/pveh1z4x4-59, 40 mg wet cells of E. coli/pveh1Z4X4-59, and 300 mM rac-pCSO, was carried out at 25°C. During the hydrolytic process, aliquots of 100 μl samples were drawn out periodically and then analyzed by chiral HPLC until rac-pCSO was almost completely hydrolyzed (c > 99%). In addition, eep, yield, and STY were calculated to evaluate its production efficiency. Finally, the aqueous phase was extracted with 20 ml ethyl acetate thrice. The pooled ethyl acetate fractions were washed by saturated NaCl thrice, dried over anhydrous sodium sulfate, and purified by silica gel column chromatography, followed by concentrating under reduced pressure.
Results and Discussion
Regioselectivity Assays of PvEH1Z4X4-59 and RpEHF361V
The regioselectivity coefficients (αS and βR), quantitatively representing its regioselectivities for (S)-and (R)-pCSO, were used to elucidate the eep of (R)-pCPED produced from the enantioconvergent hydrolysis of rac-pCSO (Zhang et al., 2020). In our previous study, the kinetic resolution of rac-pCSO (800 mM) in the Tween-20/phosphate system buffer by PvEH1Z4X4-59 was investigated. The result indicated that PvEH1Z4X4-59 enantiopreferentially hydrolyzed the (S)-pCSO and retained (R)-pCSO. Herein, to develop the industrial application of PvEH1Z4X4-59 in the enantioconvergent hydrolysis of rac-pCSO, the regioselectivity coefficients (αS and βR) for rac-pCSO were tested. As to a given EH, its regioselectivity coefficients were mainly dependent on the catalyzed rac-epoxide, that is, which carbon atom (Cα or Cβ) may mainly be subjected to nucleophilic attack by Asp in EH's catalytic triad (Hu et al., 2020a). As shown in Figure 1, the regioselectivity assay of PvEH1Z4X4-59 exhibited the hydrolytic reaction of 10 mM (S)- and (R)-pCSO using 200 mg/ml wet cells of E. coli/pveh1z4x4-59, possessed with 94.5% αS and 5.5% βS for (S)-pCSO and 20% αR and 80% βR for (R)-pCSO. The reason why PvEH1Z4X4-59 can enantiomatically hydrolyze rac-pCSO is that it has complementary regionselectivity for (R)- and (S)-pCSO. However, the low βR led to the lower final eep of (R)-pCPED. Contrary to PvEH1Z4X4-59, RpEHF361V enantiopreferentially hydrolyzed the (R)-pCSO, and the enantioselectivity assay exhibited the hydrolytic reaction of 10 mM (S)- and (R)-pCSO using 40 mg/ml wet cells of E. coli/rpehF361V, respectively, possessed with 94% αS and 6% βS for (S)-pCSO and 7% αR and 93% βR for (R)-pCSO. The source of higher enantioconvergence was that it must have high and complementary regioselectivity together with low enantioselectivity. However, there were few wild-type (WT) EHs matching the above conditions simultaneously. Therefore, PvEH1Z4X4-59 combined with RpEHF361V may be an ideal solution to hydrolyze rac-pCSO for (R)-pCPED.
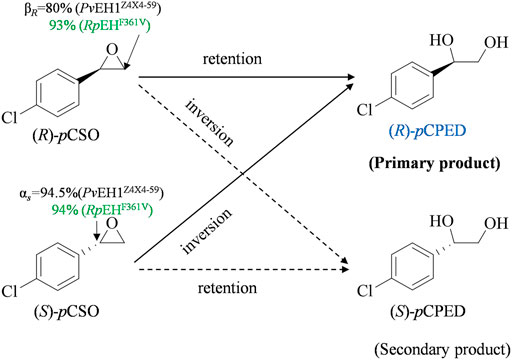
FIGURE 1. The enantioconvergent hydrolysis of rac-pCSO into (R)-pCPED catalyzed by E. coli/pveh1Z4X4-59 and E. coli/rpehF361V, respectively. E. coli/pveh1Z4X4-59 attacks at the Cβ of (R)-pCSO with βR of 80% mainly affording (R)-pCPED and at the Cα of (S)-pCSO with αS of 94.5% also mainly affording (R)-pCPED. E. coli/pveh1Z4X4-59 attacks at the Cβ of (R)-pCSO with βR of 93% mainly affording (R)-pCPED and at the Cα of (S)-pCSO with αS of 94% also mainly affording (R)-pCPED.
Enantioconvergent of Hydrolysis of rac-pCSO by E. Coli/pveh1z4x4-59 in the Tween-20/Phosphate Buffer System
The EH activity of E. coli/pveh1z4x4-59 towards rac-pCSO was measured to be 53.2 U/g wet cell, which was 4.2-fold than that of E. coli/pveh1 (12.7 U/g wet cell), which was constructed and persevered in our laboratory. No EH activity was detected in the whole cells of E. coli/pET-28a (the negative control). Reportedly, the reason for using whole cells instead of crude or purified enzymes as the biocatalyst was the fact that the former was more easily available and usually had higher stability or tolerability, especially at a high substrate and/or product concentration, than the latter during the hydrolytic reaction (Yoo et al., 2008). To investigate the potential for the production of (R)-pCPED, 200 mg/ml wet cells of E. coli/pveh1z4x4-59 was used to catalyze the enantioconvergent hydrolysis of rac-pCSO at different concentrations of 50, 100, 150, 200, and 250 mM in the 4% (v/v) Tween-20/phosphate buffer system (100 mM, pH 7.0). As shown in Figure 2A, 100 mM rac-pCSO could not be completely hydrolyzed (92.3% c) at 25°C for 12 h, producing (R)-pCPED with about 83.3% eep. When the concentration of rac-pCSO was under 100 mM, it was almost completely hydrolyzed at a prolonged hydrolytic time to 24 h (more than 99% c). However, when the concentration was elevated to 150 mM, its c value was 98% even though the hydrolytic time was prolonged to 24 h (Figure 2B). Consequently, the MAC of rac-pCSO was confirmed as 100 mM. This phenomenon was also observed in the enantioconvergent hydrolysis and kinetic resolution of rac-epoxides by some other EHs, such as ArEH and VrEH3 (Zou et al., 2013; Hu et al., 2017). This can be explained by a high enantioselectivity (E value) towards rac-pCSO in the Tween-20/phosphate buffer kinetic resolution for (S)-pCSO by E. coli/rpehF361V, which cannot catalyze the hydrolysis of rac-pCSO at a higher concentration in an enantioconvergent way.
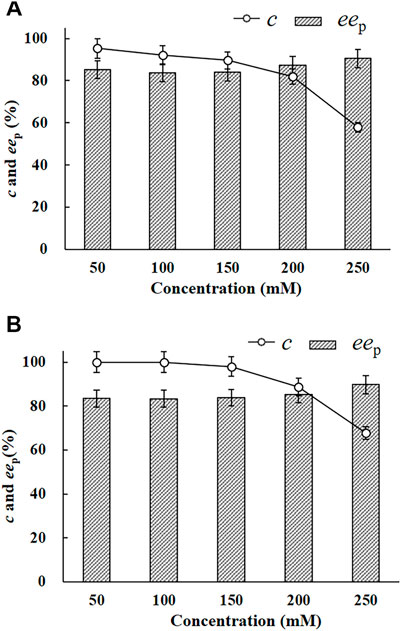
FIGURE 2. The enantioconvergent hydrolysis of rac-pCSO in the 4% (v/v) Tween-20/phosphate buffer system (100 mM, pH 7.0) using 200 mg/ml wet cells of E. coli/pveh1z4x4-59 at 25°C for 12 h (A) and 24 h (B). The hydrolytic reactions of rac-pCSO at 50–250 mM.
Kinetic Resolution for (S)-pCSO by E. Coli/rpehF361V
Analogously, the enantioselective hydrolytic reactions of rac-pCSO at concentrations of 300, 400, 500, 600, 700, 800, and 900 mM were carried out, respectively, at 25°C for 12 h by E. coli/rpehF361V wet cells. As shown in Figure 3A, the MAC of rac-pCSO was confirmed to be 800 mM, which was higher than all those of EHs previously reported, such as 400 mM of SlEH2 and 200 mM of SpEH (Wu et al., 2013; Wen et al., 2021). As the concentration of rac-pCSO was elevated to 900 mM, its c value and the ees of (S)-pCSO were merely 40.0% and 72.1% until 12 h. The scale-up kinetic resolution of 800 mM (123.67 g/L) rac-pCSO using E. coli/rpehF361V wet cells was performed in the 30 ml Tween-20/phosphate buffer system and monitored by chiral HPLC at the given intervals (Figure 3B). After incubation for 4 h, (R)-pCSO was almost completely hydrolyzed at the c value of 54.6%, retaining (S)-pCSO with over 97.2% ees and 45.2% yield. As the hydrolysis of rac-pCSO was continued for 10 h, the yield and ees of (S)-pCSO had no obvious improvement. Consequently, the MAC of rac-pCSO was confirmed as 800 mM.
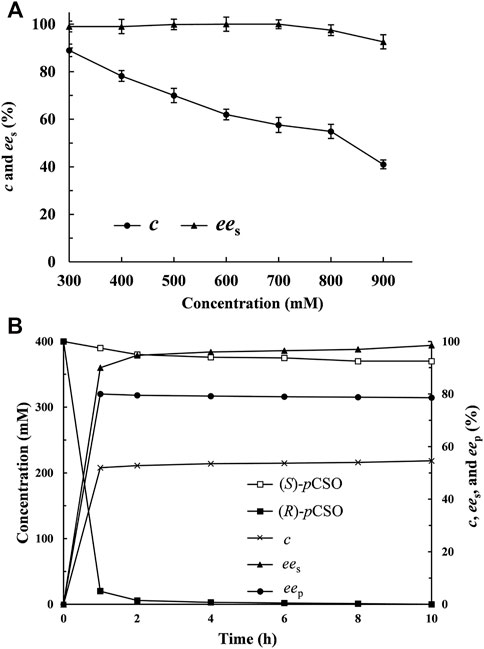
FIGURE 3. The kinetic resolution of rac-pCSO by E. coli/rpehF361V. (A) the hydrolytic reactions of rac-pCSO at 300–900 mM in the 4% (v/v) Tween-20/phosphate buffer system (100 mM, pH 7.0) using 40 mg/ml wet cells of E. coli/rpehF361V at 25°C. (B) process curves of various parameters in the scale-up kinetic resolution of 800 mM rac-pCSO at 25°C within 10 h.
Enantioconvergent Hydrolysis of rac-pCSO by E. Coli/rpehF361V and E. Coli/pveh1z4x4-59
In the above experiment, 100 mM rac-pCSO substrate was too low to achieve the industrial production of (R)-pCPED. To efficiently prepare (R)-pCPED with high eep, yield, and STY, different concentrations of 100–800 mM rac-pCSO were carried out, respectively, at 25°C using 200 mg/ml wet cells of E. coli/pveh1z4x4-59 and 40 mg/ml wet cells of E. coli/rpehF361V. After incubation for 12 h, 300 mM rac-pCSO was almost completely hydrolyzed (c > 99%), producing (R)-pCPED with about 87.5% eep (Figure 4). However, when the concentration of rac-pCSO was increased to 400 mM, its c and the eep values were merely 93.5% and 87.9%, which did not meet the requirement of enantioconvergent hydrolysis. Therefore, the MAC was confirmed as 300 mM. In the previous experiments, 800 mM rac-pCSO could be completely hydrolyzed via the kinetic resolution by E. coli/pveh1z4x4-59 or E. coli/rpehF361V, respectively. Under theoretical conditions, the enantioconvergent hydrolytic maximum concentration of rac-pCSO by double enzymes should be also at 800 mM. The result indicated that high product concentration rather than substrate concentration affected the activities of PvEH1Z4X4-59 and RpEHF361V and that the simultaneous addition of the two enzymes caused a faster reaction speed, producing (R)-pCPED with high concentration in a short time, which inhibited the activities of the enzymes at the later stage of the reaction. Similarly, in many EH-catalyzed hydrolytic reactions, diol products can inhibit the reaction (Lee and Shuler, 2007).
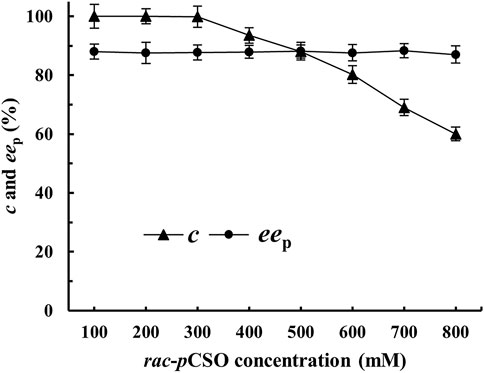
FIGURE 4. The enantioconvergent hydrolysis of rac-pCSO at 100–800 mM in the 4% (v/v) Tween-20/phosphate buffer system (100 mM, pH 7.0) using 200 mg/ml wet cells of E. coli/pveh1z4x4-59 and 40 mg/ml wet cells E. coli/rpehF361V at 25°C for 12 h.
Optimization of Both Addition Mode and Weight Ratio of the Two E. Coli Cells
In addition to the selected Tween-20/phosphate buffer system towards the given pair of EH-substrate, the addition mode may be an important parameter influencing the enzymatic catalytic performance (Wang et al., 2017). In the sequential addition mode, 300 mM rac-pCSO was first hydrolyzed by 200 mg/ml wet cells of E. coli/pveh1z4x4-59 for 6 h until (S)-pCSO was completely hydrolyzed, retaining (R)-pCSO with 59.9% c and 96.8% ees. Then, 40 mg/ml wet cells of E. coli/rpehF361V was added to sequentially hydrolyze the residual (R)-pCSO for 2 h, thereby affording (R)-pCPED with 85.8% eep, 91.5% yield, and 5.32 g/L/h STY at 100% c (Figure 5A). This result indicated that, by adding an extra complementary RpEHF361V, the eep of (R)-pCPED was slightly increased from 83.3% to 85.8%, while the reaction time was significantly shortened from 24 to 8 h and the substrate concentration was increased from 100 to 300 mM. Similarly, 300 mM rac-pCSO was first hydrolyzed by 40 mg/ml wet cells of E. coli/rpehF361V for 4 h, retaining (S)-pCSO with 55.2% c and 96.5% ees. Then, 200 mg/ml wet cells of E. coli/pveh1z4x4-59 was added to sequentially hydrolyze the residual (S)-pCSO for 6 h, thereby affording (R)-pCPED with 86.3% eep, 92.1% yield, and 4.28 g/L/h STY at 100% c (Figure 5B). In another addition mode, 300 mM rac-pCSO was hydrolyzed for 4 h by adding E. coli/pveh1z4x4-59 and E. coli/rpehF361V simultaneously, affording (R)-pCPED with 87.5% eep, 93.2% yield and 10.8 g/L/h STY at 100% c (Figure 5C). Compared with the sequential addition mode, the STY of the simultaneous addition mode was significantly improved, while eep and yield of (R)-pCPED showed no significant difference. Although 800 mM rac-pCSO can be completely hydrolyzed by kinetic resolution, the reaction time could be up to 12 h, and the concentration (360 mM) of the product near the end point of the reaction may inhibit the enzymatic activities. Therefore, the simultaneous addition mode could cause a faster reaction speed and produce (R)-pCPED with a high concentration in a short time, which did not affect the enzymatic activities during the rapid reaction phase. This result was similar to that reported previously, such as using 150 U StEH and 500 U AnEH in 125 ml buffer solution in a sequential addition mode, rac-pCSO can be completely hydrolyzed with about 2 days, affording (R)-pCPED with 96% eep and 93% yield (Manoj et al., 2001). Consequently, the simultaneous addition mode was selected to save time and improve production efficiency.
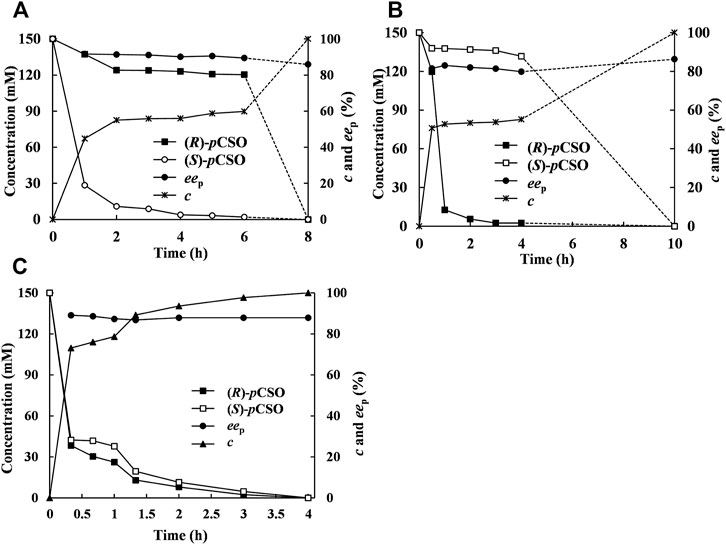
FIGURE 5. The hydrolytic course curve of rac-pCSO catalyzed by E. coli/pveh1z4x4-59 and E. coli/rpehF361V. (A) bi-enzymatic catalysis in a sequential addition mode. About 300 mM rac-pCSO was first hydrolyzed by E. coli/pveh1z4x4-59, and then E. coli/rpehF361V was added to sequentially hydrolyze the residual (R)-pCSO. (B) About 300 mM rac-pCSO was first hydrolyzed by E. coli/rpehF361V, and then E. coli/pveh1z4x4-59 was added to sequentially hydrolyze the residual (S)-pCSO. (C) Bi-enzymatic catalysis in a simultaneous addition mode. About 300 mM rac-pCSO was hydrolyzed by adding both E. coli/rpehF361V and E. coli/pveh1z4x4-59 simultaneously. The enantioconvergent hydrolysis of rac-pCSO was in the 4% (v/v) Tween-20/phosphate buffer system (100 mM, pH 7.0) at 25°C.
The eep of (R)-pCPED was increased from 82.2% to 87.8% as the weight ratios of E. coli/pveh1z4x4-59 and E. coli/rpehF361V range from 200:1 to 20:1 while slightly decreased to 87.5% as the weight ratio increased to 200:40 (Table 1). Consequently, considering the highest c value, eep, and yield, the weight ratio was confirmed as 20:1. The weight ratio of two whole cells or enzymes for the bi-enzymatic catalysis had a distinct effect on the eep of enantioconvergent hydrolysis of racemic epoxides. Enantioconvergent hydrolysis of rac-SO was achieved by using two recombinant epoxide hydrolases of CcEH and McEH, affording (R)-PED with 92% eep at the optimized weight ratio of 0.3:1, which was higher than that (89% eep) at the ratio of 0.1:1 (Kim et al., 2008).
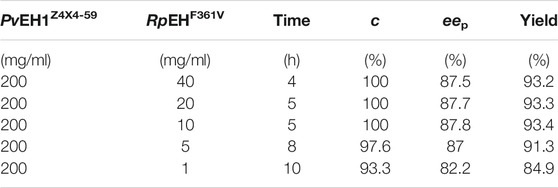
TABLE 1. The enantioconvergent hydrolysis of rac-pCSO at elevated weight ratios in the 4% (v/v) Tween-20/phosphate buffer system.
Gram-Scale Production of (R)-pCPED via Enantioconvergent Hydrolysis of rac-pCSO
Under the above optimized reaction conditions, the enantioconvergent hydrolysis of rac-pCSO was conducted in the 100 ml 4% (v/v) Tween-20/phosphate buffer system (100 mM, pH 7.0) and monitored by HPLC at given time intervals (Figure 6). After incubation for 5 h, 300 mM rac-pCSO was completely hydrolyzed, affording (R)-pCPED with 87.8% eep, 93.4% yield, and 8.63 g/L/h STY. Subsequently, 4.20 g (R)-pCPED was produced in 81.1% isolated yield after being purified by silica gel column chromatography. The enantioconvergent hydrolysis of rac-pCSO by using two E. coli cells expressing PvEH1z4x4-59 and RpEHF361V exhibited the highest substrate concentration and STY (300 mM and 8.63 g/L/h) among all known enantioconvergent hydrolytic reactions, such as those by the combinations of StEH and AnEH (4 mM, 0.24 g/L/h, and 89% eep) (Karboune et al., 2005), an E. coli transformant expressing Caulobacter crescentus EH (109 mM, 4.29 g/L/h and 95% eep) (Hwang et al., 2008), and a double-site mutant E. coli/pveh3G170E/F187X (150 mM, 1.50 g/L/h, and 92.8% eep) (Hu et al., 2018). Although the substrate concentration and STY were higher, the eep value was a little low. In the following work, protein engineering methods will be used to improve eep value.
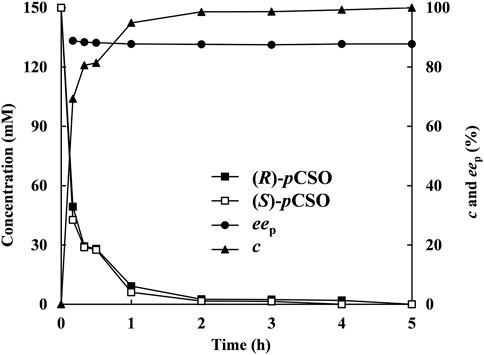
FIGURE 6. The hydrolysis of 300 mM rac-pCSO in the 4% (v/v) Tween-20/phosphate buffer system (100 mM, pH 7.0) by E. coli/rpehF361V and E. coli/pveh1z4x4-59 under the optimized conditions—the time courses of (R)- and (S)-pCSO concentrations, c of rac-pCSO and eep of (R)-PED.
Conclusion
Enantioconvergent hydrolysis of rac-pCSO by PvEH1Z4X4-59 and kinetic resolution for (S)-pCSO by RpEHF361V were investigated at elevated concentrations. Based on their high and complementary regioselectivities, a bi-enzymatic catalysis technique using two recombinant E. coli wet cells was designed and optimized for the enantioconvergent hydrolysis of rac-pCSO at high concentration. Both addition mode and weight ratio of the two E. coli cells were optimized. A simultaneous addition mode of both E. coli/pveh1z4x4-59 and E. coli/rpehF361V was first selected, by which the STY was significantly improved. Then, considering the highest eep and aTOF values, the weight ratio was confirmed as 20:1. Finally, in the 100 ml 4% (v/v) Tween-20/phosphate buffer system (100 mM, pH 7.0) under the optimized weight ratio and the simultaneous addition mode, the enantioconvergent hydrolysis of rac-pCSO at 300 mM was carried out, producing (R)-pCPED with 87.8% eep, 93.4% yield, and 8.63 g/L/h STY. In conclusion, this work would provide an efficient technical strategy for the preparation of chiral vicinal diols at industrial scale.
Data Availability Statement
The original contributions presented in the study are included in the article/Supplementary Material, further inquiries can be directed to the corresponding author.
Author Contributions
MW: Conceptualization, Methodology, Review and editing. DZ and YL: Project administration, Data curation, Original draft preparation, and Visualization. TW, WL, and XC: Formal analysis and Resources.
Funding
This work was financially supported by the China Postdoctoral Science Foundation (No. 2018M630522) and the National Natural Science Foundation of China (No. 21676117).
Conflict of Interest
The authors declare that the research was conducted in the absence of any commercial or financial relationships that could be construed as a potential conflict of interest.
Publisher’s Note
All claims expressed in this article are solely those of the authors and do not necessarily represent those of their affiliated organizations or those of the publisher, the editors, and the reviewers. Any product that may be evaluated in this article, or claim that may be made by its manufacturer, is not guaranteed or endorsed by the publisher.
References
Bala, N., and Chimni, S. S. (2010). Recent Developments in the Asymmetric Hydrolytic Ring Opening of Epoxides Catalysed by Microbial Epoxide Hydrolase. Tetrahedron: Asymmetry 21, 2879–2898. doi:10.1016/j.tetasy.2010.11.013
Deregnaucourt, J., Archelas, A., Barbirato, F., Paris, J.-M., and Furstoss, R. (2007). Enzymatic Transformations 63. High-Concentration Two Liquid-Liquid PhaseAspergillus niger Epoxide Hydrolase-Catalysed Resolution: Application to Trifluoromethyl-Substituted Aromatic Epoxides. Adv. Synth. Catal. 349, 1405–1417. doi:10.1002/adsc.200700085
Di Fabio, R., Pietra, C., Thomas, R. J., and Ziviani, L. (1995). The Asymmetric Synthesis of Both Enantiomers of Eliprodil. Bioorg. Med. Chem. Lett. 5, 551–554. doi:10.1016/0960-894X(95)00071-Z
Hu, B.-C., Hu, D., Li, C., Xu, X.-F., Wen, Z., and Wu, M.-C. (2020a). Near-perfect Kinetic Resolution of Racemic P-Chlorostyrene Oxide by SlEH1, a Novel Epoxide Hydrolase from Solanum lycopersicum with Extremely High Enantioselectivity. Int. J. Biol. Macromolecules 147, 1213–1220. doi:10.1016/j.ijbiomac.2019.10.091
Hu, B.-C., Li, C., Wang, R., Zong, X.-C., Li, J.-P., Li, J.-F., et al. (2018). Improvement in the Activity and Enantioconvergency of PvEH3, an Epoxide Hydrolase from Phaseolus vulgaris, for P-Chlorostyrene Oxide by Site-Saturation Mutagenesis. Catal. Commun. 117, 9–13. doi:10.1016/j.catcom.2018.08.019
Hu, D., Tang, C., Li, C., Kan, T., Shi, X., Feng, L., et al. (2017). Stereoselective Hydrolysis of Epoxides by reVrEH3, a Novel Vigna Radiata Epoxide Hydrolase with High Enantioselectivity or High and Complementary Regioselectivity. J. Agric. Food Chem. 65, 9861–9870. doi:10.1021/acs.jafc.7b03804
Hu, D., Wang, R., Shi, X.-L., Ye, H.-H., Wu, Q., Wu, M.-C., et al. (2016). Kinetic Resolution of Racemic Styrene Oxide at a High Concentration by Recombinant Aspergillus Usamii Epoxide Hydrolase in an N -hexanol/buffer Biphasic System. J. Biotechnol. 236, 152–158. doi:10.1016/j.jbiotec.2016.08.013
Hu, D., Zong, X.-C., Xue, F., Li, C., Hu, B.-C., and Wu, M.-C. (2020b). Manipulating Regioselectivity of an Epoxide Hydrolase for Single Enzymatic Synthesis of (R)-1,2-diols from Racemic Epoxides. Chem. Commun. 56, 2799–2802. doi:10.1039/d0cc00283f
Hwang, S., Choi, C. Y., and Lee, E. Y. (2008). Enantioconvergent Bioconversion of P-Chlorostyrene Oxide to (R)-p-chlorophenyl-1,2-ethandiol by the Bacterial Epoxide Hydrolase of Caulobacter crescentus. Biotechnol. Lett. 30, 1219–1225. doi:10.1007/s10529-008-9668-7
Karboune, S., Archelas, A., Furstoss, R., and Baratti, J. C. (2005). Immobilization of theSolanum Tuberosumepoxide Hydrolase and its Application in an Enantioconvergent Process. Biocatal. Biotransformation 23, 397–405. doi:10.1080/10242420500372328
Kim, H. S., Lee, O. K., Hwang, S., Kim, B. J., and Lee, E. Y. (2007). Biosynthesis of (R)-phenyl-1,2-ethanediol from Racemic Styrene Oxide by Using Bacterial and marine Fish Epoxide Hydrolases. Biotechnol. Lett. 30, 127–133. doi:10.1007/s10529-007-9495-2
Kotik, M., Zhao, W., Iacazio, G., and Archelas, A. (2013). Directed Evolution of Metagenome-Derived Epoxide Hydrolase for Improved Enantioselectivity and Enantioconvergence. J. Mol. Catal. B: Enzymatic 91, 44–51. doi:10.1016/j.molcatb.2013.02.006
Lee, E. Y., and Shuler, M. L. (2007). Molecular Engineering of Epoxide Hydrolase and its Application to Asymmetric and Enantioconvergent Hydrolysis. Biotechnol. Bioeng. 98, 318–327. doi:10.1002/bit.21444
Li, C., Hu, B.-C., Hu, D., Xu, X.-F., Zong, X.-C., Li, J.-P., et al. (2019a). Stereoselective Ring-Opening of Styrene Oxide at Elevated Concentration by Phaseolus vulgaris Epoxide Hydrolase, PvEH2, in the Organic/aqueous Biphasic System. Catal. Commun. 123, 1–5. doi:10.1016/j.catcom.2019.01.024
Li, C., Kan, T.-T., Hu, D., Wang, T.-T., Su, Y.-J., Zhang, C., et al. (2019c). Improving the Activity and Enantioselectivity of PvEH1, a Phaseolus vulgaris Epoxide Hydrolase, for O-Methylphenyl Glycidyl Ether by Multiple Site-Directed Mutagenesis on the Basis of Rational Design. Mol. Catal. 476, 110517. doi:10.1016/j.mcat.2019.110517
Li, C., Zhao, J., Hu, D., Hu, B.-C., Wang, R., Zang, J., et al. (2019b). Multiple Site-Directed Mutagenesis of a Phaseolus vulgaris Epoxide Hydrolase to Improve its Catalytic Performance towards P-Chlorostyrene Oxide Based on the Computer-Aided Re-design. Int. J. Biol. Macromolecules 121, 326–332. doi:10.1016/j.ijbiomac.2018.10.030
Manoj, K. M., Archelas, A., Baratti, j., and Furstoss, R. (2001). Microbiological Transformations. Part 45: A green Chemistry Preparative Scale Synthesis of Enantiopure Building Blocks of Eliprodil: Elaboration of a High Substrate Concentration Epoxide Hydrolase-Catalyzed Hydrolytic Kinetic Resolution Process. Tetrahedron 57, 695–701. doi:10.1016/S0040-4020(00)01032-2
Patel, R. N. (2018). Biocatalysis for Synthesis of Pharmaceuticals. Bioorg. Med. Chem. 26, 1252–1274. doi:10.1016/j.bmc.2017.05.023
Saini, P., and Sareen, D. (2017). An Overview on the Enhancement of Enantioselectivity and Stability of Microbial Epoxide Hydrolases. Mol. Biotechnol. 59, 98–116. doi:10.1007/s12033-017-9996-8
Wang, R., Hu, D., Zong, X., Li, J., Ding, L., Wu, M., et al. (2017). Enantioconvergent Hydrolysis of Racemic Styrene Oxide at High Concentration by a Pair of Novel Epoxide Hydrolases into (R)-phenyl-1,2-ethanediol. Biotechnol. Lett. 39, 1917–1923. doi:10.1007/s10529-017-2433-z
Wen, Z., Hu, B.-C., Hu, D., Liu, Y.-Y., Zhang, D., Zang, J., et al. (2021). Efficient Kinetic Resolution of Para-Chlorostyrene Oxide at Elevated Concentration by Solanum lycopersicum Epoxide Hydrolase in the Presence of Tween-20. Catal. Commun. 149, 106180. doi:10.1016/j.catcom.2020.106180
Woo, J.-H., Kang, K.-M., Kwon, T.-H., Park, N.-H., and Lee, E. Y. (2015). Isolation, Identification and Characterization of marine Bacteria Exhibiting Complementary Enantioselective Epoxide Hydrolase Activity for Preparing Chiral Chlorinated Styrene Oxide Derivatives. J. Ind. Eng. Chem. 28, 225–228. doi:10.1016/j.jiec.2015.02.018
Wu, S., Li, A., Chin, Y. S., and Li, Z. (2013). Enantioselective Hydrolysis of Racemic and Meso-Epoxides with Recombinant Escherichia coli Expressing Epoxide Hydrolase from Sphingomonas Sp. HXN-200: Preparation of Epoxides and Vicinal Diols in High Ee and High Concentration. ACS Catal. 3, 752–759. doi:10.1021/cs300804v
Wu, Y.-W., Kong, X.-D., Zhu, Q.-Q., Fan, L.-Q., and Xu, J.-H. (2015). Chemoenzymatic Enantioconvergent Hydrolysis of P-Nitrostyrene Oxide into (R)-p-nitrophenyl Glycol by a Newly Cloned Epoxide Hydrolase VrEH2 from Vigna Radiata. Catal. Commun. 58, 16–20. doi:10.1016/j.catcom.2014.08.020
Xu, X.-F., Hu, D., Hu, B.-C., Li, C., Liu, Y.-Y., and Wu, M.-C. (2020). Near-perfect Kinetic Resolution of O-Methylphenyl Glycidyl Ether by RpEH, a Novel Epoxide Hydrolase from Rhodotorula Paludigena JNU001 with High Stereoselectivity. Appl. Microbiol. Biotechnol. 104, 6199–6210. doi:10.1007/s00253-020-10694-w
Ye, H.-H., Hu, D., Shi, X.-L., Wu, M.-C., Deng, C., and Li, J.-F. (2016). Directed Modification of a Novel Epoxide Hydrolase from Phaseolus vulgaris to Improve its Enantioconvergence towards Styrene Epoxides. Catal. Commun. 87, 32–35. doi:10.1016/j.catcom.2016.08.036
Yoo, S. S., Park, S., and Lee, E. Y. (2008). Enantioselective Resolution of Racemic Styrene Oxide at High Concentration Using Recombinant Pichia pastoris Expressing Epoxide Hydrolase of Rhodotorula Glutinis in the Presence of Surfactant and Glycerol. Biotechnol. Lett. 30, 1807–1810. doi:10.1007/s10529-008-9762-x
Zhang, C., Li, C., Zhu, X.-x., Liu, Y.-y., Zhao, J., and Wu, M.-c. (2020). Highly Regio- and Enantio-Selective Hydrolysis of Two Racemic Epoxides by GmEH3, a Novel Epoxide Hydrolase from Glycine max. Int. J. Biol. Macromolecules 164, 2795–2803. doi:10.1016/j.ijbiomac.2020.08.011
Zou, S.-P., Zheng, Y.-G., Wu, Q., Wang, Z.-C., Xue, Y.-P., and Liu, Z.-Q. (2018). Enhanced Catalytic Efficiency and Enantioselectivity of Epoxide Hydrolase from Agrobacterium Radiobacter AD1 by Iterative Saturation Mutagenesis for (R)-epichlorohydrin Synthesis. Appl. Microbiol. Biotechnol. 102, 733–742. doi:10.1007/s00253-017-8634-5
Keywords: bi-enzymatic catalysis, kinetic resolution, enantioconvergent hydrolysis, epoxide hydrolase, high substrate concentration
Citation: Zhang D, Lei Y, Wang T, Lin W, Chen X and Wu M (2022) Gram-Scale Synthesis of (R)-P-Chlorophenyl-1,2-Ethanediol at High Concentration by a Pair of Epoxide Hydrolases. Front. Bioeng. Biotechnol. 10:824300. doi: 10.3389/fbioe.2022.824300
Received: 29 November 2021; Accepted: 06 January 2022;
Published: 28 February 2022.
Edited by:
Ya-Jun Wang, Zhejiang University of Technology, ChinaReviewed by:
Aitao Li, Hubei University, ChinaWu Qiaqing, Tianjin Institute of Industrial Biotechnology (CAS), China
Wen-Yong Lou, South China University of Technology, China
Copyright © 2022 Zhang, Lei, Wang, Lin, Chen and Wu. This is an open-access article distributed under the terms of the Creative Commons Attribution License (CC BY). The use, distribution or reproduction in other forums is permitted, provided the original author(s) and the copyright owner(s) are credited and that the original publication in this journal is cited, in accordance with accepted academic practice. No use, distribution or reproduction is permitted which does not comply with these terms.
*Correspondence: Minchen Wu, biowmc@126.com
†These authors have contributed equally to this work