- 1Department of Colorectal and Anal Surgery, The Second Hospital of Jilin University, Changchun, China
- 2Department of Education, The Second Hospital of Jilin University, Changchun, China
Acute pancreatitis (AP) is a severe life-threatening inflammatory disease showing primary characteristics of excessive inflammatory response and oxidative stress. Based on the pathophysiology of AP, several anti-inflammatory and anti-oxidative stress agents have been studied. However, the low accumulated concentrations and scattered biodistributions limit the application of these agents. With the development of nanotechnology, functional nanomaterials can improve the bioavailability of drugs and extend their half-life by reducing immunogenicity to achieve targeted drug delivery. The biomaterial-based carriers can mediate the passive or active delivery of drugs to the target site for improved therapeutic effects, such as anti-oxidation and anti-inflammation for AP treatment. Other biomaterials-based nanomedicine may exhibit different functions with/without targeting effects. In this review, we have summarized the targeting and functional effects of biomaterials-based nanoagents specifically for AP treatment.
1 Introduction
Acute pancreatitis (AP) is an unpredictable and potentially fatal inflammatory gastrointestinal disease. The global incidence of AP ranges from 13 to 45 cases per 100,000 people and is increasing annually (Petrov and Yadav, 2019). Studies have shown that alcoholism, smoking, biliary tract abnormalities, and autoimmune diseases usually cause AP, which can be accompanied by epigastric pain, nausea, vomiting, bloating, systemic involvement, and organ failure (Lankisch et al., 2015). According to the clinical severity, AP can be divided into mild, moderate, and severe categories (Ratia Gimenez et al., 2014). Mostly, mild AP can be cured automatically after progressing to a certain extent (Lankisch et al., 2015). Approximately 20% of patients with pancreatitis develop moderate to severe AP, which is difficult to cure, and show symptoms of pancreatic necrosis and/or organ failure accompanied by long-term recurrent episodes in most patients with irreversible pathological changes (Lee and Papachristou, 2019; Mederos et al., 2021). AP can cause exocrine dysfunction of the pancreas and damage acinar cells. Some severe AP cases can progress to systemic inflammatory responses, multiple organ damage, or function failure, with a mortality rate of as high as 20% (Mederos et al., 2021).
Oxygen free radicals (OFRs) and derivatives play an important role in AP progression and pancreatic tissue damage; hydrogen peroxide, superoxide anion, hydroxyl group, and singlet oxygen are the main factors causing cell damage (Padureanu et al., 2022). OFRs can promote the adhesion, activation, and migration of leukocytes, damage the integrity of endothelial cells, and increase the permeability of capillaries, thus leading to the loss of circulating blood volume, microcirculation disorders, and aggravated pancreatic injury (Padureanu et al., 2022). During the AP-mediated inflammatory response process, inflammatory factors and oxidative stress play a synergistic role via triggering a common signaling pathway, mainly activated by MAPK and NF-κB, leading to an amplification of inflammation cascade (Zhang et al., 2022a). Based on the pathophysiology of AP, various anti-oxidative and anti-inflammatory drugs have been explored (Zhang et al., 2022b). However, because of the limited solubility, short half-life, and poor stability, these traditional drugs cannot pass through the blood-pancreas barrier to reach sufficient concentration at the inflammation site (Zhou et al., 2019). Hence, a safe and effective treatment method is yet to be developed to achieve improved anti-oxidation and anti-inflammation effects for AP treatment in the clinic.
In recent years, nanotechnology has been widely applied in the biomedicine field, with rapid progress in the prevention, diagnosis, and treatment of diseases (Gagliardi et al., 2021; Wang et al., 2021). Nanoparticles of different shapes, pore sizes, and structures can be utilized in the development of nanoscale drug delivery systems using polymeric and inorganic materials as carriers (Atiyah et al., 2022). Nanomedicines can improve drug efficacy, safety, physicochemical properties, and pharmacokinetics or pharmacodynamics (Atiyah et al., 2022). Additionally, functional nanomaterials can enhance the bioavailability of oral drugs in vivo and extend their half-life by reducing immunogenicity to achieve targeted drug delivery (Hou et al., 2021; Mallakpour et al., 2022). For AP treatment, biomaterials can act as nanocarriers or nanomedicines for passive or active delivery of drugs to the target site, which is conducive to drug accumulation at the inflammatory pancreatic area, for improved therapeutic effects, including anti-oxidation and anti-inflammation (Zhang et al., 2022b). Other biomaterials-based nanomedicine may possess different functions with/without targeting effects for AP treatment. In this review, we have summarized and discussed the targeting and functional effects of biomaterials-based nanoagents for AP treatment.
2 Role of biomaterials-based nanoagents in AP treatment
Although several important roles of biomaterials as drug carriers and nanomedicine for disease diagnosis and treatment have already been discussed (Festas et al., 2020; Gagliardi et al., 2021), this review mainly outlines the targeting and functional effects of biomaterials-based nanoagents constructed specifically for AP treatment (Figure 1; Table 1).
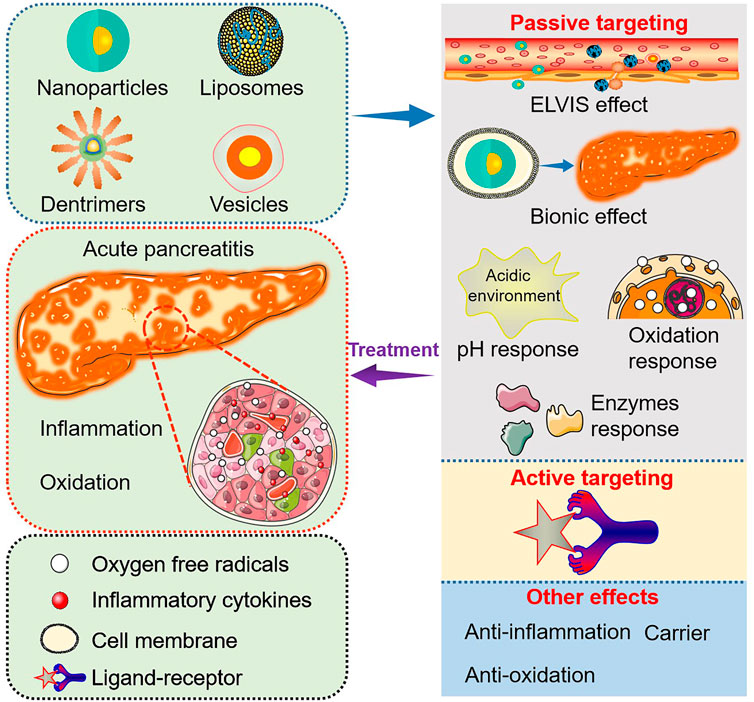
FIGURE 1. Targeting and functional effects of biomaterials-based nanoagents constructed specifically for AP treatment.
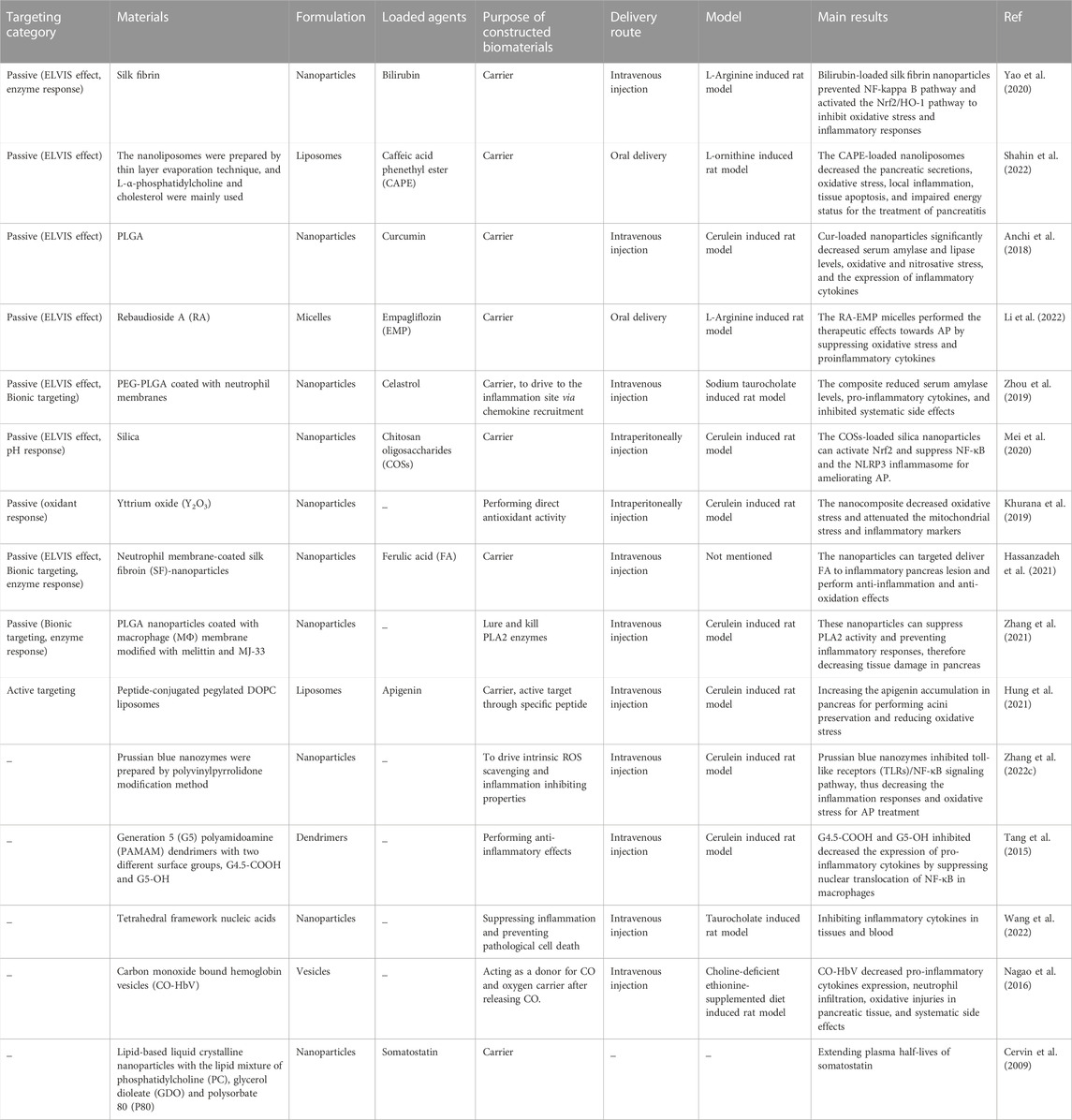
TABLE 1. The targeting and functional effects of biomaterials constructed for nanoagents for AP treatment.
2.1 Targeting effects
Targeting effect is crucial for the treatment of various diseases and can be divided into three aspects, i.e., passive, active, and physical targeting (Russ and Wagner, 2007; Ivey et al., 2016; Kanamala et al., 2016). Accumulation of nanoagents at the target sites for enhanced therapeutic efficacy while reducing systematic side effects can be achieved through targeting effect (Steichen et al., 2013). According to the existing literature, biomaterials-based targeting effects for AP treatment are mainly passive and active (Zhang et al., 2022b).
2.1.1 Passive targeting
During passive targeting, biomaterials-based nanocarriers generally act via physical and chemical interactions (hydrophobic and electrostatic interactions) as well as physical factors (such as carrier size and mass) to achieve targeted drug delivery (Attia et al., 2019). For pancreatitis, passive targeting can be achieved through increased permeability of tissue space and other microenvironment biochemical properties, including pH, reactive oxygen species (ROS), and digestive enzymes (Chiorean and Coveler, 2015).
Inflammatory responses can increase the permeability of blood vessels, subsequently triggering the extravasation through leaky vasculature and inflammatory cell-mediated sequestration (ELVIS) (Gong et al., 2019), which allows passive targeting of suitable size range nanoparticles for accumulation at the inflammation sites (Ren et al., 2019). Yao et al. (2020) developed silk-fibrin (SF)-based nanoparticles encapsulating bilirubin, the main anti-oxidant component of heme catabolism, for AP treatment. The animal experiment showed that the synthesized nanoparticles could selectively target the inflammatory sites in the pancreas to release bilirubin. The bilirubin-loaded SF nanoparticles prevented the NF-κB pathway and activated the Nrf2/HO-1 pathway to inhibit oxidative stress and inflammatory responses. In another study, L-α-phosphatidylcholine and cholesterol were mainly used to prepare nanoliposomes by thin layer evaporation technique (Shahin et al., 2022), loaded with caffeic acid phenethyl ester (CAPE) for oral administration in a rat model. The CAPE-loaded nanoliposomes decreased pancreatic secretions, oxidative stress, local inflammation, tissue apoptosis, and impaired energy status as therapeutic effects for AP treatment. The in vivo biodistribution study of the nanoliposomes was not performed; thus, the targeting effects could not be supported directly. However, the enhanced treatment efficacy was attributed to the altering tissue biodistribution of smaller-size liposomes and cellular uptake mechanisms (Shahin et al., 2022). Notably, liposomes particle size is their most influential characteristic affecting the circulation time and biodistribution after intravenous injection, which plays a vital role in passing through the leaky vasculature and accumulation at inflammatory sites in vivo (Ren et al., 2019). Anchi et al. (2018) synthesized curcumin-loaded poly (lactic-co-glycolic acid) (PLGA) nanoparticles and evaluated the treatment efficacy in a cerulein induced AP model. The nanoparticles delivered curcumin to the inflammatory pancreatic site mediated by the ELVIS effect, resulting in a significant reduction of serum amylase and lipase levels, oxidative and nitrosative stress, and the expression of inflammatory cytokines. In addition, other previous studies have also reported the passive targeting of biomaterials-based nanocarriers via the ELVIS effect for AP therapy. Li et al. (2022) reported empagliflozin (EMP) loaded rebaudioside A (RA) micelles with a particle size of 5.234 ± 0.311 nm. The small particle size of the synthesized RA-EMP micelles (less than 20 nm) could benefit their cellular uptake and tissue accumulation for therapeutic effects against AP in a rat model by suppressing oxidative stress and proinflammatory cytokines.
Biomaterials-based nanoparticles with small size triggering the ELVIS effect at inflammatory sites have been applied in drug delivery and treatment of various inflammatory diseases. However, traditional nanoparticles are easily recognized by the reticuloendothelial system in vivo and are difficult to accumulate passively at the inflammation area via the ELVIS effect. For example, although the small particle size of RA-EMP micelles aided the passive targeting of EMP at the pancreatic inflammation site, the biodistribution study showed the highest EMP concentrations in kidney and liver tissues with reduced EMP accumulation in the pancreas, which could restrict the therapeutic efficacy (Li et al., 2022). Natural cell-membrane-modified nanoparticles have immense advantages in disease diagnosis and treatment due to the unique proteins, peptides, and enzymes present on the surface of cell membranes (Hwang et al., 2015) and are highly biocompatible to achieve extended circulation ability and/or target effects. Additionally, nanoparticles modified by partial or complete cell membranes can acquire the cell-derived bioactive property and homing effect for targeted drug delivery. Till now, membranes of various cell types, including red blood cells (Hu et al., 2011), platelets (Hu et al., 2015), white blood cells (Parodi et al., 2013), cancer cells (Chen et al., 2016), stem cells (Bose et al., 2018), etc., have been applied to modify nanoparticles for disease diagnosis and treatment. Utilizing the natural homing effect of cell membranes, nanoparticles can target the corresponding lesions to increase the therapeutic efficacy; the targeted homing effect is termed Bionic targeting (Liu et al., 2023). AP is an acute inflammatory disease characterized by the infiltration of a large number of inflammatory cells, including neutrophils and macrophages (Mayerle et al., 2012). Neutrophils are the most abundant type of granulocytes, accounting for 40 to 70 percent of all human white blood cells and the host’s first line of defense against invading pathogens and infections. The inherently phagocytic neutrophils can be activated by cytokines which then arrive at the inflammation sites (Selders et al., 2017; Castanheira and Kubes, 2019). Zhou et al. (2019) studied the treatment efficacy and mechanism of celastrol-loaded poly (ethylene glycol) methyl ether-block-PLGA (PEG-PLGA) nanoparticles coated with neutrophil membrane towards AP. The neutrophil membrane-modified nanoparticles aimed to endorse the Bionic targeting effect at the inflammation site through cytokines recruitment. In addition, the size of the applied nanoparticles (156.8 ± 2.3 nm) attributed to the passive ELVIS targeting effect. Furthermore, the modified celastrol-loaded nanoparticles reduced serum amylase levels and pro-inflammatory cytokines and inhibited systematic side effects in a sodium taurocholate-induced AP rat model.
The pH-responsive materials are usually capable of physical or chemical changes within a specific pH range (Lu et al., 2014). The pH-sensitive nanomaterials can achieve responsive release of drugs at the inflammatory site and correspondingly increase the drug accumulation (Kellum et al., 2004). Enhanced cellular metabolic activity leads to anaerobic glycolysis and lactic acid formation at the inflammation site (Zhang et al., 2020), resulting in an acidic microenvironment within the damaged pancreatic tissue. Applying pH-sensitive biomaterials to achieve passive targeting at the pancreatic tissues may further enhance the anti-oxidative and anti-inflammatory therapeutic effects. Silica nanoparticles, possessing porous structures and good biocompatibility, are usually used as carriers that can release drugs mediated by pH control to obtain their high concentrations within targeted tissues (Li et al., 2017; Zhang et al., 2018). Mei et al. (2020) developed silica-based nanoparticles encapsulating chitosan oligosaccharides (COSs) for their (COSs) targeted delivery to the pancreas, exerting anti-oxidation and anti-inflammation effects by activating Nrf2 and suppressing NF-κB and NLRP3 inflammasome for ameliorating AP. Moreover, the small size of the nanoparticles (305 nm) could also contribute to the ELVIS targeting effect.
The normal physiological ROS levels play an essential role in cell differentiation, proliferation, and migration (Hajam et al., 2022). In AP conditions, ROS are excessively produced in activated neutrophil and macrophage organelles such as mitochondria and endoplasmic reticulum. Overproduction of ROS can promote the production of inflammatory cytokines such as tumor necrosis factor alpha (TNFα), interleukin (IL) 1β, and IL 6, accelerating the progression of AP (Carrasco et al., 2014). The nanocomposites sensitive to oxidative response can be utilized in targeted therapy for AP. For example, intraperitoneal administration of yttrium oxide (Y2O3) nanoparticles decreased oxidative stress and attenuated the mitochondrial stress and inflammatory markers in an AP rat model (Khurana et al., 2019). The Y2O3 nanoparticles, like catalase and superoxide dismutase mimetic, possess promising anti-oxidation properties (Heckert et al., 2008).
During the trigger and development of AP, a variety of enzymes can be secreted by pancreatic tissues, such as proteolytic enzymes (Hu et al., 2020) and phospholipase A2 (PLA2) (Friess et al., 2001). Biomaterials-based nanoagents with enzyme response properties can achieve passive targeting effects at inflammatory sites to improve therapeutic mechanisms for AP. As discussed above, the SF protein in bilirubin-loaded SF nanoparticles, which induced the ELVIS passive targeting effects towards inflammatory pancreatic tissues (Yao et al., 2020), can be degraded by proteolytic enzymes, making SF a candidate material for enzyme-responsive nanoagents design (Zhao et al., 2015). As a result, the bilirubin-loaded SF nanoparticles also exhibited enzyme-responsive targeting effects for enhanced inhibition of oxidative stress and inflammatory responses (Yao et al., 2020). In another study, neutrophil membrane-coated SF-nanoparticles encapsulated with ferulic acid (FA) were developed for in vivo targeted delivery of FA to inflammatory pancreas lesions showing anti-inflammation and anti-oxidation effects (Hassanzadeh et al., 2021). The major components of the nanoparticles, SF and the entrapped neutrophil membrane, endowed the nanoagent with enzyme-responsive and Bionic targeting effects, respectively. Additionally, FA is a phenolic compound that has been applied in the treatment of various diseases, especially those accompanied by severe oxidative stress and inflammation responses (Zdunska et al., 2018). Zhang et al. (2021) synthesized macrophage (MΦ) membrane-coated PLGA nanoparticles modified with melittin and MJ-33 for AP treatment. Melittin is a short peptide with a high affinity for PLA2, while MJ-33 serves as a PLA2 inhibitor. The MΦ membrane and melittin in the nanocomposite may benefit the Bionic and enzyme-responsive targeting effects, respectively. In a rat AP model, these nanoparticles suppressed PLA2 activity, thus, preventing inflammatory responses and decreasing pancreatic tissue damage.
2.1.2 Active targeting
Passive targeting based on the ELVIS effect and other mechanisms is insufficient due to the non-specific reaction between cells and nanoagents, leading to a significant reduction in cellular endocytosis of nanoagents. This affects the bioavailability of drugs and their therapeutic effects, resulting in drug leakage and resistance in the organism (Lee et al., 2022). To overcome these limitations, biomaterials-based agents with active targeting properties should be considered; specific ligands or non-serum based-biomarkers can be applied to increase the treatment efficacy of AP. The nanoparticles modified with specific peptides can recognize and bind with corresponding receptors expressed in certain cells in the inflammatory sites, resulting in enhanced drug accumulation (Da Silva-Candal et al., 2019; Lin et al., 2021). Hung et al. (2021) selected five pancreatitis-specific peptides using a computational-guided in vivo phage display approach, demonstrating selectivity to different pancreatic cells. The peptide-conjugated liposomes were encapsulated with apigenin for the targeted delivery of the drug to inflammatory pancreatic tissues for acinar cell preservation and oxidative stress reduction. Despite the advantages, the active targeting property of biomaterials-based nanoagents has not been studied and exploited extensively for AP treatment, highlighting the need for further research in this area.
2.2 Other functional effects
Besides the passive and active targeting effects, the biomaterials used for nanoagents construction may possess several other functional properties for AP treatment. Zhang et al. (2022b) developed polyvinylpyrrolidone (PVP)-modified molybdenum selenide two-dimensional nanosheets (MoSe2@PVP NSs), which could act as artificial enzymes and mimic multi-enzyme activities, including catalase (CAT), superoxide dismutase (SOD), peroxidase (POD), and glutathione peroxidase (GPx) to scavenge ROS and reactive nitrogen species (RNS) in an AP rat model. In another study, Prussian blue nanozyme (PBzyme) prepared by the PVP modification method could drive intrinsic ROS scavenging and inflammation-inhibiting properties in AP therapy (Xie et al., 2021). Tang et al. (2015) synthesized Generation 5 (G5) polyamidoamine (PAMAM) dendrimers with two different surface groups, G4.5-COOH and G5-OH, and studied their therapeutic mechanisms for AP. Both dendrimers reduced pathological injuries and inflammation responses in the pancreas. A similar study was conducted by Wang et al. (2022) in which tetrahedral framework nucleic acids were developed for suppressing inflammation and preventing pathological cell death caused by AP. Based on previous studies that have reported carbon monoxide (CO)-mediated regulation of inflammatory responses and oxidative stress effectively (Otterbein et al., 2000; Zuckerbraun et al., 2007), CO-bound hemoglobin vesicles (CO-HbV) were synthesized for AP treatment (Nagao et al., 2016). CO-HbV could serve as a CO donor for driving inflammation inhibition effect and an oxygen carrier after releasing CO, exerting oxidation prevention effect. In addition, a few biomaterial-based nanoparticles were utilized only as carriers for specific drugs without studying their biodistribution to support any targeting effect (Cervin et al., 2009).
3 Conclusions and perspectives
AP is a life-threatening severe inflammatory disease with no clear pathogenesis. However, it is mainly characterized by inflammation infiltration and oxidative stress. Recent reports on AP treatment have primarily focused on inhibiting inflammatory responses and preventing oxidation in pancreatic tissues. With the development of nanotechnology, the biomaterials-based nanoagents may achieve passive (e.g., ELVIS and Bionic effect, pH, ROS and enzyme-dependent) or active (specific ligand or non-serum based biomarkers dependent) targeting and other functional effects (e.g., multi-enzyme activities, anti-inflammation and anti-oxidation effect) for AP treatment. Future research should be inclined towards the following aspects: (Petrov and Yadav, 2019) Studies on biomaterial-based nanoagents with active targeting to aid the release and accumulation of nanoagents in injured pancreatic tissues (Lankisch et al., 2015); Advantages of nanocarriers should be integrated for precise AP treatment to reduce side effects (Ratia Gimenez et al., 2014); Studies should be carried out in AP animal models with information on pathology and statistical data to obtain convincing results.
Author contributions
YC wrote this study. QC drafted the figure and table. JL and TL designed and revised this study.
Funding
This study was supported by National Natural Science Foundation of China (Grant No. 32000953), Science and Technology Department of Jilin Province (Grant No. YDZJ202201ZYTS004).
Conflict of interest
The authors declare that the research was conducted in the absence of any commercial or financial relationships that could be construed as a potential conflict of interest.
The reviewer XW declared a shared parent affiliation with the authors to the handling editor at the time of review.
Publisher’s note
All claims expressed in this article are solely those of the authors and do not necessarily represent those of their affiliated organizations, or those of the publisher, the editors and the reviewers. Any product that may be evaluated in this article, or claim that may be made by its manufacturer, is not guaranteed or endorsed by the publisher.
References
Anchi, P., Khurana, A., Swain, D., Samanthula, G., and Godugu, C. (2018). Sustained-release curcumin microparticles for effective prophylactic treatment of exocrine dysfunction of pancreas: A preclinical study on cerulein-induced acute pancreatitis. J. Pharm. Sci. 107 (11), 2869–2882. doi:10.1016/j.xphs.2018.07.009
Atiyah, N. A., Albayati, T. M., and Atiya, M. A. (2022). Interaction behavior of curcumin encapsulated onto functionalized sba-15 as an efficient carrier and release in drug delivery. J. Mol. Struct. 1260, 132879. doi:10.1016/j.molstruc.2022.132879
Attia, M. F., Anton, N., Wallyn, J., Omran, Z., and Vandamme, T. F. (2019). An overview of active and passive targeting strategies to improve the nanocarriers efficiency to tumour sites. J. Pharm. Pharmacol. 71 (8), 1185–1198. doi:10.1111/jphp.13098
Bose, R. J. C., Kim, B. J., Arai, Y., Han, I-b., Moon, J. J., Paulmurugan, R., et al. (2018). Bioengineered stem cell membrane functionalized nanocarriers for therapeutic targeting of severe hindlimb ischemia. Biomaterials 185, 360–370. doi:10.1016/j.biomaterials.2018.08.018
Carrasco, C., Beatriz Rodriguez, A., and Pariente, J. A. (2014). Effects of melatonin on the oxidative damage and pancreatic antioxidant defenses in cerulein-induced acute pancreatitis in rats. Hepatobiliary Pancreat. Dis. Int. 13 (4), 442–446. doi:10.1016/s1499-3872(14)60271-x
Castanheira, F. V. S., and Kubes, P. (2019). Neutrophils and nets in modulating acute and chronic inflammation. Blood 133 (20), 2178–2185. doi:10.1182/blood-2018-11-844530
Cervin, C., Vandoolaeghe, P., Nistor, C., Tiberg, F., and Johnsson, M. (2009). A combined in vitro and in vivo study on the interactions between somatostatin and lipid-based liquid crystalline drug carriers and bilayers. Eur. J. Pharm. Sci. 36 (4-5), 377–385. doi:10.1016/j.ejps.2008.11.001
Chen, Z., Zhao, P., Luo, Z., Zheng, M., Tian, H., Gong, P., et al. (2016). Cancer cell membrane-biomimetic nanoparticles for homologous-targeting dual-modal imaging and photothermal therapy. Acs Nano 10 (11), 10049–10057. doi:10.1021/acsnano.6b04695
Chiorean, E. G., and Coveler, A. L. (2015). Pancreatic cancer: Optimizing treatment options, new, and emerging targeted therapies. Drug Des. Dev. Ther. 9, 3529–3545. doi:10.2147/dddt.S60328
Da Silva-Candal, A., Brown, T., Krishnan, V., Lopez-Loureiro, I., Avila-Gomez, P., Pusuluri, A., et al. (2019). Shape effect in active targeting of nanoparticles to inflamed cerebral endothelium under static and flow conditions. J. Control. Release 309, 94–105. doi:10.1016/j.jconrel.2019.07.026
Festas, A. J., Ramos, A., and Davim, J. P. (2020). Medical devices biomaterials - a review. Proc. Institution Mech. Eng. Part L-Journal Materials-Design Appl. 234 (1), 218–228. doi:10.1177/1464420719882458
Friess, H., Shrikhande, S., Riesle, E., Kashiwagi, M., Baczako, K., Zimmermann, A., et al. (2001). Phospholipase A2 isoforms in acute pancreatitis. Ann. Surg. 233 (2), 204–212. doi:10.1097/00000658-200102000-00009
Gagliardi, A., Giuliano, E., Venkateswararao, E., Fresta, M., Bulotta, S., Awasthi, V., et al. (2021). Biodegradable polymeric nanoparticles for drug delivery to solid tumors. Front. Pharmacol. 12, 601626. doi:10.3389/fphar.2021.601626
Gong, T., Zhang, P., Deng, C., Xiao, Y., Gong, T., and Zhang, Z. (2019). An effective and safe treatment strategy for rheumatoid arthritis based on human serum albumin and Kolliphor® HS 15. Nanomedicine 14 (16), 2169–2187. doi:10.2217/nnm-2019-0110
Hajam, Y. A., Rani, R., Ganie, S. Y., Sheikh, T. A., Javaid, D., Qadri, S. S., et al. (2022). Oxidative stress in human pathology and aging: Molecular mechanisms and perspectives. Cells 11 (3), 552. doi:10.3390/cells11030552
Hassanzadeh, P., Arbabi, E., and Rostami, F. (2021). Coating of ferulic acid-loaded silk fibroin nanoparticles with neutrophil membranes: A promising strategy against the acute pancreatitis. Life Sci. 270, 119128. doi:10.1016/j.lfs.2021.119128
Heckert, E. G., Karakoti, A. S., Seal, S., and Self, W. T. (2008). The role of cerium redox state in the sod mimetic activity of nanoceria. Biomaterials 29 (18), 2705–2709. doi:10.1016/j.biomaterials.2008.03.014
Hou, X., Zaks, T., Langer, R., and Dong, Y. (2021). Lipid nanoparticles for mrna delivery. Nat. Rev. Mater. 6 (12), 1078–1094. doi:10.1038/s41578-021-00358-0
Hu, C-M. J., Fang, R. H., Wang, K-C., Luk, B. T., Thamphiwatana, S., Dehaini, D., et al. (2015). Nanoparticle biointerfacing by platelet membrane cloaking. Nature 526 (7571), 118–121. doi:10.1038/nature15373
Hu, C-M. J., Zhang, L., Aryal, S., Cheung, C., Fang, R. H., and Zhang, L. (2011). Erythrocyte membrane-camouflaged polymeric nanoparticles as a biomimetic delivery platform. Proc. Natl. Acad. Sci. U. S. A. 108 (27), 10980–10985. doi:10.1073/pnas.1106634108
Hu, Q., Bao, Y., Gan, S., Zhang, Y., Han, D., and Niu, L. (2020). Electrochemically controlled grafting of polymers for ultrasensitive electrochemical assay of trypsin activity. Biosens. Bioelectron. 165, 112358. doi:10.1016/j.bios.2020.112358
Hung, J., Awasthi, R., Klibanov, A. L., and Kelly, K. A. (2021). Identification of novel ligands for targeted antifibrotic therapy of chronic pancreatitis. Int. J. Nanomedicine 16, 5495–5512. doi:10.2147/ijn.S318331
Hwang, J., Jeong, Y., Park, J. M., Lee, K. H., Hong, J. W., and Choi, J. (2015). Biomimetics: Forecasting the future of science, engineering, and medicine. Int. J. Nanomedicine 10, 5701–5713. doi:10.2147/ijn.S83642
Ivey, J. W., Bonakdar, M., Kanitkar, A., Davalos, R. V., and Verbridge, S. S. (2016). Improving cancer therapies by targeting the physical and chemical hallmarks of the tumor microenvironment. Cancer Lett. 380 (1), 330–339. doi:10.1016/j.canlet.2015.12.019
Kanamala, M., Wilson, W. R., Yang, M., Palmer, B. D., and Wu, Z. (2016). Mechanisms and biomaterials in ph-responsive tumour targeted drug delivery: A review. Biomaterials 85, 152–167. doi:10.1016/j.biomaterials.2016.01.061
Kellum, J. A., Song, M. C., and Li, J. Y. (2004). Science review: Extracellular acidosis and the immune response: Clinical and physiologic implications. Crit. Care 8 (5), 331–336. doi:10.1186/cc2900
Khurana, A., Anchi, P., Allawadhi, P., Kumar, V., Sayed, N., Packirisamy, G., et al. (2019). Yttrium oxide nanoparticles reduce the severity of acute pancreatitis caused by cerulein hyperstimulation. Nanomedicine-Nanotechnology Biol. Med. 18, 54–65. doi:10.1016/j.nano.2019.02.018
Lankisch, P. G., Apte, M., and Banks, P. A. (2015). Acute pancreatitis. Lancet 386 (9988), 85–96. doi:10.1016/s0140-6736(14)60649-8
Lee, D., Kwon, S., Jang, S-Y., Park, E., Lee, Y., and Koo, H. (2022). Overcoming the obstacles of current photodynamic therapy in tumors using nanoparticles. Bioact. Mater. 8, 20–34. doi:10.1016/j.bioactmat.2021.06.019
Lee, P. J., and Papachristou, G. L. (2019). New insights into acute pancreatitis. Nat. Rev. Gastroenterology Hepatology 16 (8), 479–496. doi:10.1038/s41575-019-0158-2
Li, Q., Cao, Q., Yuan, Z., Wang, M., Chen, P., and Wu, X. (2022). A novel self-nanomicellizing system of empagliflozin for oral treatment of acute pancreatitis: An experimental study. Nanomedicine-Nanotechnology Biol. Med. 42, 102534. doi:10.1016/j.nano.2022.102534
Li, Y., Li, N., Pan, W., Yu, Z., Yang, L., and Tang, B. (2017). Hollow mesoporous silica nanoparticles with tunable structures for controlled drug delivery. Acs Appl. Mater. Interfaces 9 (3), 2123–2129. doi:10.1021/acsami.6b13876
Lin, Z-C., Hwang, T-L., Huang, T-H., Tahara, K., Trousil, J., and Fang, J-Y. (2021). Monovalent antibody-conjugated lipid-polymer nanohybrids for active targeting to desmoglein 3 of keratinocytes to attenuate psoriasiform inflammation. Theranostics 11 (10), 4567–4584. doi:10.7150/thno.56995
Liu, R., He, L., Liu, M., Chen, L., Hou, J., Shi, J., et al. (2023). Erythrocyte membrane encapsulated gambogic acid nanoparticles as a therapeutic for hepatocellular carcinoma. Chin. Chem. Lett. 34 (1), 107575. doi:10.1016/j.cclet.2022.05.089
Lu, Y., Sun, W., and Gu, Z. (2014). Stimuli-responsive nanomaterials for therapeutic protein delivery. J. Control. Release 194, 1–19. doi:10.1016/j.jconrel.2014.08.015
Mallakpour, S., Nikkhoo, E., and Hussain, C. M. (2022). Application of mof materials as drug delivery systems for cancer therapy and dermal treatment. Coord. Chem. Rev. 451, 214262. doi:10.1016/j.ccr.2021.214262
Mayerle, J., Dummer, A., Sendler, M., Malla, S. R., van den Brandt, C., Teller, S., et al. (2012). Differential roles of inflammatory cells in pancreatitis. J. Gastroenterology Hepatology 27, 47–51. doi:10.1111/j.1440-1746.2011.07011.x
Mederos, M. A., Reber, H. A., and Girgis, M. D. (2021). Acute pancreatitis: A review. Jama-Journal Am. Med. Assoc. 325 (4), 382–390. doi:10.1001/jama.2020.20317
Mei, Q., Deng, G., Huang, Z., Yin, Y., Li, C., Hu, J., et al. (2020). Porous cos@SiO(2)Nanocomposites ameliorate severe acute pancreatitis and associated lung injury by regulating the Nrf2 signaling pathway in mice. Front. Chem. 8, 720. doi:10.3389/fchem.2020.00720
Nagao, S., Taguchi, K., Sakai, H., Yamasaki, K., Watanabe, H., Otagiri, M., et al. (2016). Carbon monoxide-bound hemoglobin vesicles ameliorate multiorgan injuries induced by severe acute pancreatitis in mice by their anti-inflammatory and antioxidant properties. Int. J. Nanomedicine 11, 5611–5620. doi:10.2147/ijn.S118185
Otterbein, L. E., Bach, F. H., Alam, J., Soares, M., Lu, H. T., Wysk, M., et al. (2000). Carbon monoxide has anti-inflammatory effects involving the mitogen-activated protein kinase pathway. Nat. Med. 6 (4), 422–428. doi:10.1038/74680
Padureanu, V., Florescu, D. N., Padureanu, R., Ghenea, A. E., Gheonea, D. I., and Oancea, C. N. (2022). Role of antioxidants and oxidative stress in the evolution of acute pancreatitis (review). Exp. Ther. Med. 23 (3), 197. doi:10.3892/etm.2022.11120
Parodi, A., Quattrocchi, N., van de Ven, A. L., Chiappini, C., Evangelopoulos, M., Martinez, J. O., et al. (2013). Synthetic nanoparticles functionalized with biomimetic leukocyte membranes possess cell-like functions. Nat. Nanotechnol. 8 (1), 61–68. doi:10.1038/nnano.2012.212
Petrov, M. S., and Yadav, D. (2019). Global epidemiology and holistic prevention of pancreatitis. Nat. Rev. Gastroenterology Hepatology 16 (3), 175–184. doi:10.1038/s41575-018-0087-5
Ratia Gimenez, T., Gutierrez Calvo, A., and Granell Vicent, J. (2014). Etiology of acute pancreatitis. Central Eur. J. Med. 9 (4), 530–542. doi:10.2478/s11536-013-0279-x
Ren, H., He, Y., Liang, J., Cheng, Z., Zhang, M., Zhu, Y., et al. (2019). Role of liposome size, surface charge, and pegylation on rheumatoid arthritis targeting therapy. Acs Appl. Mater. Interfaces 11 (22), 20304–20315. doi:10.1021/acsami.8b22693
Russ, V., and Wagner, E. (2007). Cell and tissue targeting of nucleic acids for cancer gene therapy. Pharm. Res. 24 (6), 1047–1057. doi:10.1007/s11095-006-9233-9
Selders, G. S., Fetz, A. E., Radic, M. Z., and Bowlin, G. L. (2017). An overview of the role of neutrophils in innate immunity, inflammation and host-biomaterial integration. Regen. Biomater. 4 (1), 55–68. doi:10.1093/rb/rbw041
Shahin, N. N., Shamma, R. N., and Ahmed, I. S. (2022). A nano-liposomal formulation of caffeic acid phenethyl ester modulates Nrf2 and NF-κβ signaling and alleviates experimentally induced acute pancreatitis in a rat model. Antioxidants 11 (8), 1536. doi:10.3390/antiox11081536
Steichen, S. D., Caldorera-Moore, M., and Peppas, N. A. (2013). A review of current nanoparticle and targeting moieties for the delivery of cancer therapeutics. Eur. J. Pharm. Sci. 48 (3), 416–427. doi:10.1016/j.ejps.2012.12.006
Tang, Y., Han, Y., Liu, L., Shen, W., Zhang, H., Wang, Y., et al. (2015). Protective effects and mechanisms of G5 pamam dendrimers against acute pancreatitis induced by caerulein in mice. Biomacromolecules 16 (1), 174–182. doi:10.1021/bm501390d
Wang, B., Kostarelos, K., Nelson, B. J., and Zhang, L. (2021). Trends in micro-/nanorobotics: Materials development, actuation, localization, and system integration for biomedical applications. Adv. Mater. 33 (4), 2002047. doi:10.1002/adma.202002047
Wang, Y., Li, Y., Gao, S., Yu, X., Chen, Y., and Lin, Y. (2022). Tetrahedral framework nucleic acids can alleviate taurocholate-induced severe acute pancreatitis and its subsequent multiorgan injury in mice. Nano Lett. 22 (4), 1759–1768. doi:10.1021/acs.nanolett.1c05003
Xie, X., Zhao, J., Gao, W., Chen, J., Hu, B., Cai, X., et al. (2021). Prussian blue nanozyme-mediated nanoscavenger ameliorates acute pancreatitis via inhibiting tlrs/nf-kappa B signaling pathway. Theranostics 11 (7), 3213–3228. doi:10.7150/thno.52010
Yao, Q., Jiang, X., Zhai, Y-Y., Luo, L-Z., Xu, H-L., Xiao, J., et al. (2020). Protective effects and mechanisms of bilirubin nanomedicine against acute pancreatitis. J. Control. Release 322, 312–325. doi:10.1016/j.jconrel.2020.03.034
Zdunska, K., Dana, A., Kolodziejczak, A., and Rotsztejn, H. (2018). Antioxidant properties of ferulic acid and its possible application. Skin Pharmacol. Physiology 31 (6), 332–336. doi:10.1159/000491755
Zhang, J., Wang, Y., Liu, B., Liu, X., and Xu, Z. (2022). Activity of ligustrum vulgare L extracts against acute pancreatitis in murine models by regulation of P38 mapk and nf-kappa B signaling pathways. Saudi J. Biol. Sci. 29 (1), 273–278. doi:10.1016/j.sjbs.2021.08.087
Zhang, L., Xie, P., Wu, H., Zhao, J., and Wang, S. (2022). 2d Mose2@Pvp nanosheets with multi-enzyme activity alleviate the acute pancreatitis via scavenging the reactive oxygen and nitrogen species. Chem. Eng. J. 446, 136792. doi:10.1016/j.cej.2022.136792
Zhang, Q., Li, S., Yu, Y., Zhu, Y., and Tong, R. (2022). A mini-review of diagnostic and therapeutic nano-tools for pancreatitis. Int. J. Nanomedicine 17, 4367–4381. doi:10.2147/ijn.S385590
Zhang, Q., Zhou, J., Zhou, J., Fang, R. H., Gao, W., and Zhang, L. (2021). Lure-and-Kill macrophage nanoparticles alleviate the severity of experimental acute pancreatitis. Nat. Commun. 12 (1), 4136. doi:10.1038/s41467-021-24447-4
Zhang, R., Liu, R., Liu, C., Pan, L., Qi, Y., Cheng, J., et al. (2020). A ph/ros dual-responsive and targeting nanotherapy for vascular inflammatory diseases. Biomaterials 230, 119605. doi:10.1016/j.biomaterials.2019.119605
Zhang, Y., Cao, X., Xiang, S., and Tong, Z. (2018). Hyaluronic acid coated hollow mesoporous silica nanoparticles for reduction-triggered drug release. Micro & Nano Lett. 13 (11), 1550–1555. doi:10.1049/mnl.2018.5141
Zhao, Z., Li, Y., and Xie, M-B. (2015). Silk fibroin-based nanoparticles for drug delivery. Int. J. Mol. Sci. 16 (3), 4880–4903. doi:10.3390/ijms16034880
Zhou, X., Cao, X., Tu, H., Zhang, Z-R., and Deng, L. (2019). Inflammation-targeted delivery of celastrol via neutrophil membrane-coated nanoparticles in the management of acute pancreatitis. Mol. Pharm. 16 (3), 1397–1405. doi:10.1021/acs.molpharmaceut.8b01342
Keywords: acute pancreatitis, passive targeting, active targeting, nanotechnology, biomaterials
Citation: Cai Y, Cao Q, Li J and Liu T (2023) Targeting and functional effects of biomaterials-based nanoagents for acute pancreatitis treatment. Front. Bioeng. Biotechnol. 10:1122619. doi: 10.3389/fbioe.2022.1122619
Received: 13 December 2022; Accepted: 29 December 2022;
Published: 10 January 2023.
Edited by:
Weiguo Xu, Changchun Institute of Applied Chemistry (CAS), ChinaCopyright © 2023 Cai, Cao, Li and Liu. This is an open-access article distributed under the terms of the Creative Commons Attribution License (CC BY). The use, distribution or reproduction in other forums is permitted, provided the original author(s) and the copyright owner(s) are credited and that the original publication in this journal is cited, in accordance with accepted academic practice. No use, distribution or reproduction is permitted which does not comply with these terms.
*Correspondence: Jiannan Li, am5saUBjaWFjLmFjLmNu; Tongjun Liu, dG9uZ2p1bmxpdUAxNjMuY29t