- 1Health Science Center, Yangtze University, Jingzhou, China
- 2Collaborative Innovation Centre of Regenerative Medicine and Medical BioResource Development and Application Co-constructed by the Province and Ministry, Guangxi Medical University, Nanning, China
- 3Articular Surgery, The Second Nanning People’s Hospital (Third Affiliated Hospital of Guangxi Medical University), Nanning, China
- 4Center for Materials Synthetic Biology, CAS Key Laboratory of Quantitative Engineering Biology, Shenzhen Institute of Synthetic Biology, Shenzhen Institutes of Advanced Technology, Chinese Academy of Sciences, Shenzhen, China
The meniscus is a kind of fibrous cartilage structure that serves as a cushion in the knee joint to alleviate the mechanical load. It is commonly injured, but it cannot heal spontaneously. Traditional meniscectomy is not currently recommended as this treatment tends to cause osteoarthritis. Due to their good biocompatibility and versatile regulation, hydrogels are emerging biomaterials in tissue engineering. Hydrogels are excellent candidates in meniscus rehabilitation and regeneration because they are fine-tunable, easily modified, and capable of delivering exogenous drugs, cells, proteins, and cytokines. Various hydrogels have been reported to work well in meniscus-damaged animals, but few hydrogels are effective in the clinic, indicating that hydrogels possess many overlooked problems. In this review, we summarize the applications and problems of hydrogels in extrinsic substance delivery, meniscus rehabilitation, and meniscus regeneration. This study will provide theoretical guidance for new therapeutic strategies for meniscus repair.
Introduction
The meniscus is a semilunar fibro-cartilaginous tissue located in the knee joint that serves as a cushion at the ends of bones. The main component of the meniscus is the extracellular matrix (ECM), which consists of 72% water, 22% collagen, and 0.8% glycosaminoglycans (GAGs) (Tan and Cooper-White, 2011). The remaining components are mostly proteins, glycoproteins, and fibrochondrocytes interspersed in the meniscus. These cells contain a large number of collagen fibers arranged in bundles, which are aligned with the direction of external force to withstand stress, tension, extrusion, and rotary force (Chen S. et al., 2017). Located between the femoral condyle and the tibia, the meniscus is prone to tear when subjected to longitudinal compression force and transverse shear force at the same time. The greater the degree of knee flexion, the more posterior the tear sites. Meniscus tear is the most common meniscus injury, and its severity varies with the location, type, and shape of the tear. According to the blood supply, the meniscus can be divided into a red zone (lateral area with abundant blood supply), a middle zone (central area with less blood supply), and a white zone (medial area with almost no blood supply). The difficulty of healing increases dramatically from the outside to the inside. Meniscus tears come in many forms: longitudinal, horizontal, and radial. If there is no effective treatment, these three tears can develop into bucket handle tear (longitudinal), flap tear (horizontal), or parrot’s beak tear (radial). Meniscus injury, especially white-on-white meniscus tear, lacks regeneration capacity and frequently leads to arthralgia and osteoarthritis. Repair of the meniscus is important for maintaining knee homeostasis and articular surface integrity. Currently, the common repair methods for meniscus injury include meniscectomy, meniscus rehabilitation, and regeneration. Knee joints are more prone to arthritis because meniscectomy destroys the articular surface and joint stability. With the deepening of related research and the increase in quality of life standards, it has become widely accepted that meniscus injury should be repaired rather than excised (Banovetz et al., 2022).
Combining biology and materials science, tissue engineering offers a new approach to meniscus repair. As prominent biomaterials, hydrogels have received much attention for their good biocompatibility, reduction of shear force, and strong plasticity (Longo et al., 2012). They can be used in biomedicine under reasonable structural and functional design (Abazari et al., 2022; Li et al., 2022d). Modification of hydrogels with different responsive materials is a common technique in tissue engineering to optimize the physical and chemical properties of hydrogels (Zhang L. et al., 2022; Zhao P. et al., 2022; Cao et al., 2022; Zhang Q. et al., 2022; Liu et al., 2022; Shao et al., 2022; Shen et al., 2022; Wu et al., 2022). In the published literature, hydrogels have been adopted as scaffolds to deliver exogenous drugs, cells, and factors to promote meniscus rehabilitation and regeneration (Resmi et al., 2020; An et al., 2021; Li et al., 2022b; Zhao Y. P. et al., 2022; Li et al., 2022c). Meanwhile, hydrogel-based repair materials have been fabricated with bioprinting technology and computer three-dimensional (3D) modeling technology to make full use of their good plasticity and printable features (Shi et al., 2021; Gunes et al., 2022; Janarthanan et al., 2022).
In this review, the applications of hydrogels to extrinsic substance-delivery, meniscus rehabilitation, and meniscus regeneration will be illustrated. A comprehensive search of the English articles was conducted in August 2022 with PubMed, Medline and Embase. The search keywords of this review are “hydrogel, mensicus”. No more than 200 articles were found in each database. These articles conatin a variety of literatures including original atricles, review papers, chapters and comments. Duplicate articles are further screened to eliminate them. After the subjective assessment was included, 181 outcomes were identified as relevant to the topic of our interest. Of these, 149 articles were carefully read and made into this review. During the subsequent overhaul, four newly published articles (Baysan G et al., 2022; Herrera Millar et al., 2022; Kim and Bonassar, 2022; Zihna et al., 2022) were added to this review. Next, the various functionalized hydrogels for meniscus repair in the published literature will be overviewed, and the characteristics and applications of these hydrogels will be briefly introduced. This review may illuminate a new direction for the development of hydrogels for meniscus repair in the clinic.
Extrinsic substance-delivery hydrogels
Drug-delivery hydrogels
Conventional drug administration methods, including oral and intravenous infusion, frequently result in large fluctuations in blood drug concentrations. Once the concentration falls below the effective concentration, the drug has no effect. On the contrary, if the concentration exceeds the tolerance value, cells and tissues may be damaged. Frequent small dose administration can avoid excessive fluctuation of blood concentration, but it is unlikely to be accepted due to inconvenience. Because hydrogels contain numerous voids and exhibit slow degradation, they are suitable candidates for drug delivery systems (Narayanaswamy and Torchilin, 2019). Good biocompatibility, easily controlled administration, and sustained drug release have enabled hydrogels to play important roles in cancer therapy (Norouzi et al., 2016; Sonker et al., 2021; Xiao et al., 2021). To date, hydrogels have been adopted as drug delivery systems in wound healing (Abazari et al., 2022; Zhao P. et al., 2022; Liu et al., 2022), arthritis therapy (Zhang M. et al., 2022; Zhao Y. P. et al., 2022), ophthalmic disease (Lin et al., 2019; Lynch et al., 2020; Akulo et al., 2022; Das et al., 2022), skin disease (Jiang T. et al., 2018; Yuan et al., 2020), vaginal infections (Perinelli et al., 2018; Dos Santos et al., 2020), and other applications (Jacob et al., 2019). Unfortunately, there have been relatively few studies on the effect of drugs on meniscus repair. Petersen et al. found that the healing effect on non-traumatic meniscus lesions seemed to be equal among surgical and non-surgical treatments (Petersen et al., 2015), but a study by Krych et al. demonstrated that non-operative treatment of medial meniscus posterior horn root tears led to worse clinical outcomes (Krych et al., 2017). Research by Lim et al. revealed that non-operative treatments including non-steroidal anti-inflammatory drugs provided symptomatic relief and functional improvements in most patients with degenerative posterior root tear of the medial meniscus (Lim et al., 2010), and Heo et al. showed that a riboflavin-loaded hydrogel reduced scaffold contraction, increased mechanical properties, and delayed enzyme-triggered degradation of collagen scaffolds (Heo et al., 2016). Zhang et al. reported that simvastatin-conjugated gelatin hydrogel promotes the regeneration of an avascular meniscus in the rabbit model of a meniscal defect (Zhang et al., 2016). Tanaka et al., revealed that intra-articular administration of simvastatin-conjugated gelatin hydrogel attenuates of osteoarthritis progression in mice with down-regulation of autophagic marker and inflammatory factors (Tanaka et al., 2019). Tsubosaka et al., compared the effect of eaicosapentanoic acid (EPA) alone and EPA-incorporating gelatin hydrogels on osteoarthritis (OA) progression with animal investigation in C57BL/6J mice, and found that EPA-incorporating gelatin hydrogels prevent OA progression in vivo more effectively than EPA injection alone (Tsubosaka et al., 2020). Wang et al. Reported that Dexamethasone-loaded thermo-sensitive hydrogel exhibits pain-relieving effect in destabilization of medial meniscus (DMM)-induced osteoarthritis of mice models in vivo (Wang et al., 2021). Based on the above studies, we preliminarily believe that drugs used in combination with hydrogels can partially improve joint function and alleviate the discomfort symptoms of meniscus injury by down-regulating the inflammatory environment of the joint cavity, but the repair effect of drugs alone on the organic lesions of the meniscus is relatively weak.
Cell-delivery hydrogels
Hydrogels provide cells with a scaffold that promotes cell proliferation and differentiation while preventing cell loss. Cells can be easily loaded onto hydrogels, resulting in numerous interactions that reproduce the natural interactions of cells and the ECM in tissues. Research by Vernerey et al. suggested that at least five mechanisms, namely communication, mechanosensing, migration, growth, and tissue deposition and elaboration, are involved in cell-hydrogel interactions (Vernerey et al., 2021), which indicates that cells and hydrogels influence each other in tissue engineering applications (Madl and Heilshorn, 2018; Ma and Huang, 2020; Mills et al., 2020). Stem cells are often used for treating human disease because of their multidirectional differentiation potential (Hoang et al., 2022). Cell-loaded hydrogels have been reported to be widely used in tissue engineering. Li et al. suggested that a hydrogel coated with stem cells has a significant effect on the repair of spinal cord injury (Li et al., 2022d). Cell-loaded hydrogels fabricated into either lamellar or 3D forms have been used in treatments for bone and cartilage defects (Liu et al., 2021; Nadine et al., 2022). Due to their high capacity for moisture and good moisturizing effect, porous hydrogels coated with stem cells have been reported to be effective in treating skin wounds (Shafei et al., 2020), limbal stem cell deficiency (Yazdani et al., 2019), damaged kidneys (Jansen et al., 2017), injured cardiac tissue (Waters et al., 2018), and other conditions (Zarrintaj et al., 2021). Meniscal fibrochondrocytes are the main cellular components of the meniscus. Multiple studies have shown that meniscal fibrochondrocytes can form meniscus-like tissue in vitro and promote meniscus regeneration in vivo (Simson et al., 2013; Chen et al., 2019; Lan et al., 2021). Simson et al. encapsulated bovine meniscal fibrochondrocytes in chondroitin sulfate (CS)-bone marrow (BM) hydrogel (CSBM) hydrogels, and found that meniscal fibrochondrocytes were able to survive, proliferate, and produce meniscus ECM in vitro, and meniscus explants adhered by C30B70 fused together after 12 weeks’ implantation in a subcutaneous model of athymic rats (Simson et al., 2013). Baek et al. fabricated a biodegradable and biomimetic nanofibrous scaffold with electrospinning with a biomimetic gel, and demonstrated that cells from avascular and vascular regions of human menisci survived, attached, and infiltrated the scaffold, and secreted the major proteins found in meniscal matrix (Baek et al., 2015). Heo et al. encapsulated fibrochondrocytes isolated from New Zealand white rabbit with Photo-crosslinked collagen-HA hydrogel, and found that gene expression of collagen II and aggrecan was obviously up-regulated (Heo et al., 2016). Chen et al. conducted in vitro study and meniscus defect implantation with meniscal fibrochondrocytes (MFCs) and poly (ε-caprolactone) (PCL)-meniscus extracellular matrix (MECM) hydrogel, and revealed that 2% of meniscus extracellular matrix (MECM)-based hydrogel strongly enhanced chondrogenic marker mRNA expression and cell proliferation, and the regenerated menisci in the PCL-hydrogel-MFCs group had similar histological structures, biochemical contents and biomechanical properties as the native menisci in the sham operation group (Chen et al., 2019). Bahcecioglu et al. cultured fibrochondrocytes in agorose, methacrylated gelatin (GelMA), methacrylated hyaluronic acid (MeHA) and GelMA-MeHA blend hydrogels, and found that these hydrogels are more supportive for in vitro meniscus regeneration (Bahcecioglu et al., 2019b). Lan et al. mixed human meniscus fibrochondrocytes (hMFC) with 3D bioprinted TEMPO (2,2,6,6-tetramethylpiperidine-1-oxyl)-oxidized cellulose nanofiber-alginate hydrogel and then conducted 6 weeks in vitro chondrogenesis’ culture, and found that COL2A1 was highly expressed and more inner meniscus-like phenotype expressed in the TCNF/ALG and collagen-based construct (Lan et al., 2021). Meniscus injury is common, but the number of meniscus cells (MCs) that can be recycled is limited. Kremer et al. compared primary equine mesenchymal stem cells (MSCs) and MCs on three different scaffolds and found that the phenotype of MSCs and MCs co-cultured on a scaffold composed of Col I gel on SIS-muc exhibited the greatest similarity to native meniscus tissue (Kremer et al., 2017). Hagmeijer determined that 20% MCs and 80% MSCs were the most appropriate ratio for a type I collagen hydrogel for meniscus regeneration, and the stimulatory effect of MSCs towards meniscus cells was demonstrated by cellular communication through gap junctions (Hagmeijer et al., 2019). Co-culture of stem cells with MCs is beneficial for stem cells to differentiate into fibrochondrocytes. If MCs could be replaced with stem cells, it would facilitate the continued development of meniscus tissue engineering. Koh et al. encapsulated conditioned medium (CM)-expanded human tonsil-derived mesenchymal stem cells (T-MSCs) in riboflavin-induced photocrosslinked collagen-hyaluronic acid (COL-RF-HA) hydrogels, and cultured in chondrogenic medium containing TGF-β3 in vitro and implanted subcutaneously in female nude Balb-c mice in vivo, and he found that CM-expanded cells support highest cell proliferation, GAG accumulation, and collagen deposition and CM treatment induced complete regeneration when implanted into meniscus defect model (Koh et al., 2017). Yuan et al. firstly buried mECM hydrogel encapsulated with human mesenchymal stem cells (hMSCs) under the skin and implanted subcutaneously for an additional 4 weeks, and then the in situ model of meniscal injury was conducted in orthotopic model of meniscal injury in nude rat. After these procedures, he revealed that decellularized meniscus ECM hydrogel retained tissue-specific proteoglycans and collagens, and significantly upregulated expression of fibrochondrogenic markers, and the meniscus ECM hydrogel in turn supported delivery of hMSCs for integrative repair of a full-thickness defect model in meniscal explants after in vitro culture and in vivo subcutaneous implantation (Yuan et al., 2017). A research of Romanazzo et al. suggested that inner meniscus ECM promoted chondrogenesis of fat pad-derived stem cells with exogenous growth factors, and inner ECM-functionalised hydrogels supported the highest levels of Sox-9 and type II collagen gene expression and sulfated glycosaminoglycans (sGAG) deposition when supplemented with TGFβ3. Whereas, outer meniscus ECM promoted a more elongated cell morphology and the development of a more fibroblastic phenotype In the absence of exogenously supplied growth factors, and a more fibrogenic phenotype was observed in outer ECM-functionalised hydrogels supplemented with connective tissue growth factor (Romanazzo et al., 2018). Chen et al. encapsulated bone mesenchymal stromal cells into A thermosensitive, injectable, in situ crosslinked hydrogel, and found that hydrogel was biocompatible and could stimulate strong fibrochondrogenic differentiation of BMSCs after the incorporation of TGF-β1, and local administration of the BMSC-laden, TGF-β1-incorporated hydrogel could promote the healing of rabbit meniscal injury (Chen et al., 2020). Zhong et al. investigated the effect of mECM on encapsulated MSCs and integrative meniscus repair by in vivo rat subcutaneous implantation and orthotopic meniscus injury model, and revealed that BMSCs-laden mECM hydrogels promote meniscus regeneration and improve joint function (Zhong et al., 2020). These studies suggest that cell-loaded hydrogels can be a promising strategy for meniscus repair. Although both MCs and stem cells have been reported to be useful in meniscus repair, more research is needed to compare their repair effects and underlying mechanisms.
Protein and cytokine-delivery hydrogels
Because of the interactions between a hydrogel and its loaded cells, the composition and characteristics of the hydrogel directly affect cell fate (Tsou et al., 2016). Delivery of many proteins and cytokines—including bone morphogenetic protein (BMP), transforming growth factor-beta (TGF-β), silk fibroin, and platelet-rich plasma (PRP)—by loading hydrogels with exosomes is reported to be beneficial to the treatment of bone, cartilage, skin, and spinal cord injuries (Qiu et al., 2016; Maisani et al., 2018; Wang L. et al., 2020; Shafei et al., 2020; Cheng et al., 2021; Jiang et al., 2021; Li et al., 2021; Lin et al., 2021; Saygili et al., 2021; Yuan et al., 2021; Zhang et al., 2021; Yan et al., 2022). The ECM is the main extracellular component of the meniscus, and it plays a key role in soft tissue regeneration, providing cells with a dynamic and complex array of biochemical and biomechanical signals that regulate cell adhesion, proliferation, differentiation, and migration (Wu et al., 2021). ECM-functionalized hydrogels are reported to be prominent in enabling meniscus repair when combined with different cells in multiple studies (Baek et al., 2015; Wu et al., 2015; Yuan et al., 2017; Chen et al., 2019; Zhong et al., 2020). Shimomura et al. analyzed the expression of meniscus-associated genes with human bone marrow MSCs (hBMSCs) seeded on inner and outer meniscus-derived ECMs (mECMs) and concluded that ECMs derived from different regions had different effects on the differentiation of stem cells based on the results that inner mECM seeding enhanced the fibrocartilaginous differentiation of hBMSCs, whereas outer mECM seeding promoted a more fibroblastic phenotype (Shimomura et al., 2017). The aforementioned studies indicate that different regions of the meniscus release different factors and substances that stimulate cells to differentiate into cells with different functions, but more research is needed to reveal the underlying mechanisms. Okuno et al. reported that KI24RGDS peptide hydrogel facilitated meniscus repair in a rabbit meniscal defect model (Okuno et al., 2021). In vitro and in vivo studies by Forriol et al. and Ozeki et al. demonstrated that bone morphogenetic protein-7 (BMP-7) is a suitable growth factor to stimulate meniscus regeneration and delay cartilage degeneration (Ozeki et al., 2013; Forriol et al., 2014). As major components of the ECM, collagen types I, II, and III were all revealed to be key regulators in meniscus repair (Mueller et al., 1999; Oda et al., 2015; Wang C. et al., 2020). In addition, Chen et al. and Narita et al. suggested that transforming growth factor β1 and fibroblast growth factor 2 incorporated with hydrogels enhance the healing effect on meniscus injury (Narita et al., 2012; Chen et al., 2020). Pan et al. demonstrated that conditional EGFR deletion in mice and intra-articular injection of a small molecule EGFR inhibitor gefitinib together could promote ECM production (Pan et al., 2017). Meanwhile, Liang et al. found that transforming growth factor beta-3 (TGF-β3) and insulin-like growth factor 1 (IGF-1) are indispensable growth factors by comparing the effects of these factors on the cell differentiation of synovial fluid-derived MSCs (SF-MSCs) toward meniscus fibrochondrocytes (Liang et al., 2018). Ishida et al. proposed that PRP-laden hydrogel may exert a regenerative effect on the meniscus in vitro and in vivo (Ishida et al., 2007). Another protein mainly expressed in red blood cells, erythropoietin, was demonstrated to enhance meniscus repair and prevent osteoarthritis formation (Fu et al., 2020). Hao et al. encapsulated chemokines (platelet-derived growth factor-BB, PDGF-BB) and small chondroinductive molecules (kartogenin, KGN) within biomimetic polycaprolactone/hydrogel, and found that the dual drug-releasing meniscal scaffold possesses the potential to act as an off-the-shelf product for the clinical treatment of meniscal injury and related joint degenerative diseases (Hao et al., 2021). The changes and mechanisms of related regulatory factors during meniscus development and following injury have not been clarified, so more research in this area is required to reveal the roles of related proteins and cytokines at different stages. Figure 1 and Table 1 show the representative reseaches of extrinsic substance-delivery hydrogels in meniscus repair and their outcomes.
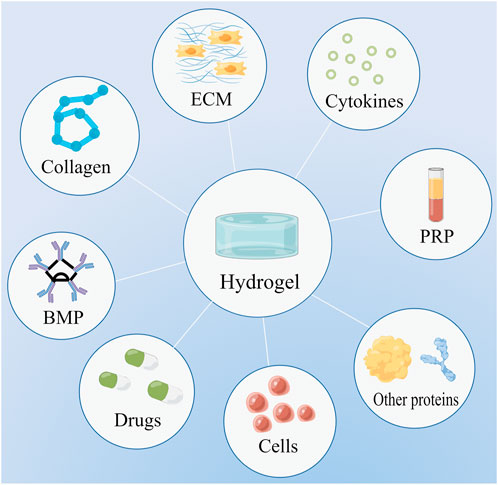
FIGURE 1. Hydrogels can serve as scaffolds to deliver a variety of substances, including drugs, cells, extracellular matrix (ECM), bone morphogenetic protein (BMP), collagen, cytokines, platelet-rich plasma (PRP), and other proteins.
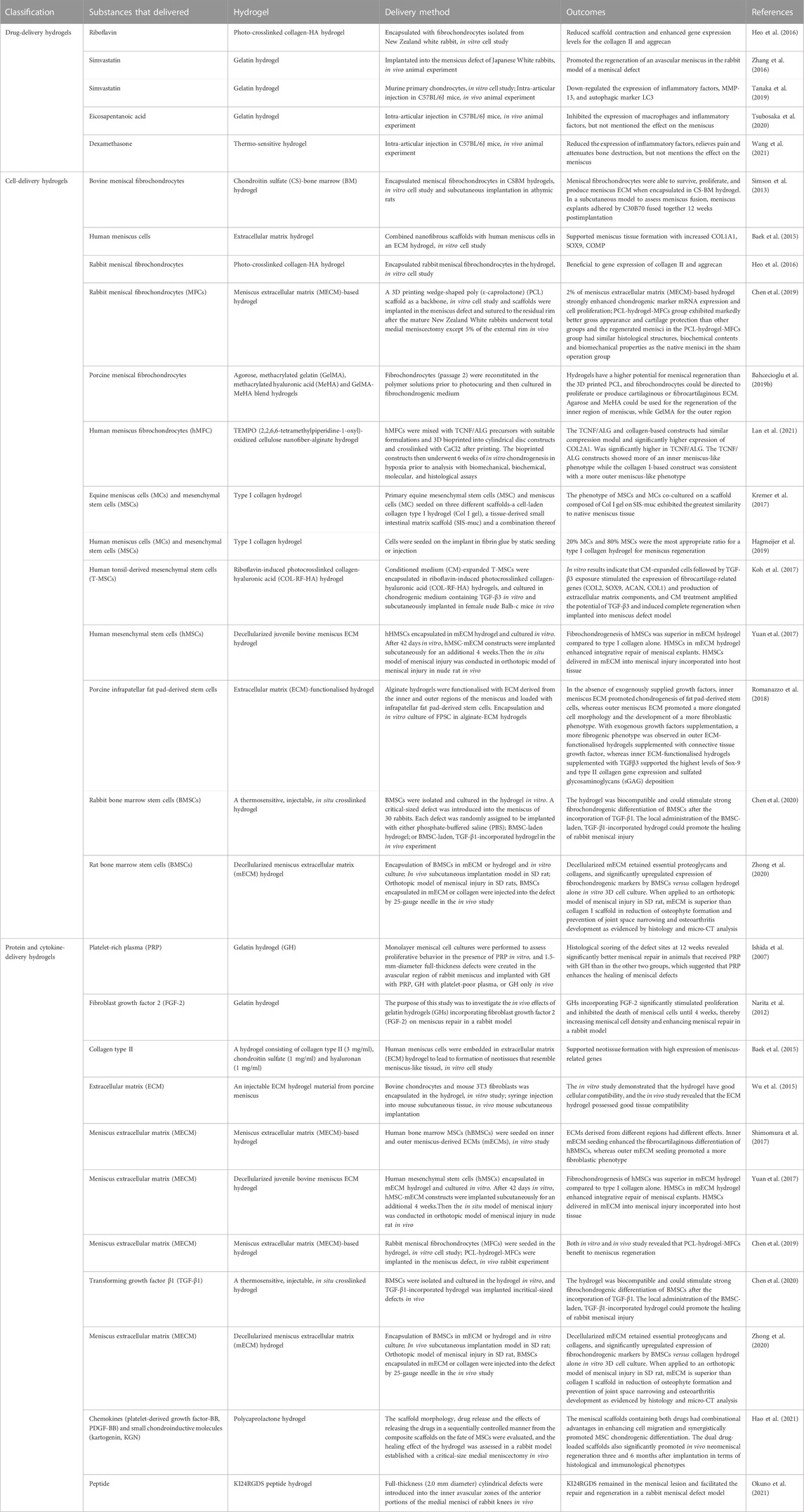
TABLE 1. Summary table of extrinsic substance-delivery hydrogels in meniscus repair and their outcomes.
Meniscus rehabilitation hydrogels
Biological tissue-derived hydrogels
Conservative treatment and meniscus suture are the traditional meniscus repair methods, but their long-term results are not ideal (Chambers and Chambers, 2019; Ulku et al., 2020). Hydrogels may be viable options in meniscus repair since they are lubricating and injectable. A juvenile bovine menisci-derived ECM hydrogel was proposed to exert therapeutic effects on rat meniscus injury (Yuan et al., 2017). Studies by both Zhong et al. and Ruprecht et al. indicated that porcine meniscus-derived matrix (MDM) hydrogels promote the repair of meniscus injury (Ruprecht et al., 2019; Zhong et al., 2020). Visser et al. processed different kinds of hydrogels with equine cartilage, meniscus, and tendon tissue, and found that cell differentiation can be influenced by different hydrogels (Visser et al., 2015). Meanwhile, Scotti et al. demonstrated that cellular fibrin glue has promising potential in enhancing the meniscus bonding effect (Scotti et al., 2009). Recent research suggests that the endostatin in fibrin hydrogel may be the key element to promoting chondrogenic differentiation of swne neonatal meniscal cells (Herrera Millar et al., 2022). In addition, PRP, which contains multiple proteins and growth factors, was reported to be effective in meniscus healing (Braun et al., 2015). Through a 3-month clinical follow-up trial, Popescu et al. found that PRP played a positive role in adolescents with meniscus lesions (Popescu et al., 2020). On the contrary, studies by Dai et al. and Yang et al. reported that a similar effect in functional outcome and pain relief was found in the PRP group and non-PRP group (Dai et al., 2019; Yang et al., 2021). Considering these contradictory results, we speculate that the complex components in PRP may be the cause. More studies are needed to isolate PRP components and examine the effects of various components.
Natural biomaterial-derived hydrogels
Raw materials from biological sources are limited and may contain yet undetectable adverse immune effects, so it is necessary to develop hydrogels from natural biomaterials. As a natural polysaccharide, sodium alginate has been widely used in tissue engineering research (Rastogi and Kandasubramanian, 2019). Lan et al. fabricated TEMPO (2,2,6,6-tetramethylpiperidine-1-oxyl)-oxidized cellulose nanofiber/alginate (TCNF/ALG) hydrogel and suggested that human meniscus fibrochondrocytes (hMFC) implanted in the hydrogel exhibited a more inner meniscus-like phenotype, whereas cells cultured in a collagen I-based construct exhibited a more outer meniscus-like phenotype (Lan et al., 2021). As another common hydrogel ingredient, agarose is widely used to prepare gels for separating macromolecular proteins and DNA. Gunja et al. employed agarose molds to detect the effects of agarose mold compliance and surface roughness on self-assembled meniscus-shaped constructs, and they found that 1% agarose exhibited higher potential for preventing construct buckling (Gunja et al., 2009). Hyaluronic acid, a natural moisturizing factor, is mainly used in cosmetology, ophthalmology, and arthrology. Research by Berton et al. and Kim et al. demonstrated that hyaluronic acid hydrogels have a restorative effect in meniscus injury (Kim et al., 2018; Berton et al., 2020). Due to its easy degradation and lack of fixed molecular weight, gelatin is often mixed with other substances (e.g., alginate, agarose, hyaluronic acid, and methacrylate) to fabricate hydrogels (Echave et al., 2017; Salahuddin et al., 2021). A study by Resmi et al. revealed that the injectable alginate dialdehyde-gelatin (15ADA20G) hydrogel shows good integration with the host meniscus tissue and relatively long retention in the close region of meniscus tear (Resmi et al., 2020). Adjusting the PH of gelatin has been reported as a viable method to improve both biochemical and biomechanical properties of hydrogels for tissue-engineered meniscus (Kim and Bonassar, 2022). The main type of collagen in the ECM of the meniscus is type I collagen. An in vitro study by Kremer et al. indicated that type I collagen hydrogel is beneficial to the high expression of meniscus-related proteins in stem cells (Kremer et al., 2017).
Artificial hydrogels
Although natural hydrogels have good biodegradability, artificial hydrogels have better mechanical properties. Acrylamide, a commonly used material for hydrogel preparation, is often used with other natural hydrogel materials or synthetic hydrogel materials. A study by Bahcecioglu et al. stated that gelatin methacrylate (GelMA)-agarose (Ag) hydrogel was suitable for medial meniscus preparation, and GelMA was conducive to lateral meniscus preparation (Bahcecioglu et al., 2019a). Zihna et al. prepared hybrid meniscal constructs using methacrylate gelatin (GelMA) hydrogels and acellular matrices and proved it may be used in meniscus tissue engineering with mechanical tests and in vitro cell experiments (Zihna et al., 2022). Modified polyvinyl alcohol (PVA) hydrogel has better load-bearing capacity and lower cytotoxicity than PVA alone, which is suggested to be beneficial to meniscus repair either alone or combined with gelatin (Hayes et al., 2016; Marrella et al., 2018). Zhang et al. fabricated a biodegradable poly (l-glutamic acid) (PLGA)-g-poly (ε-caprolactone) (PCL) hydrogel and proved that the hydrogel carrying adipose-derived stem cells (ASCs) effectively regenerated meniscus-like tissue in vivo and preserved the corresponding articular cartilage from degeneration over a 16 week period (Zhang K. et al., 2018). In addition, polycaprolactone (PCL) and poly (glycolic acid) (PGA) hydrogels have been demonstrated to be conducive scaffolds for meniscus repair (Aufderheide and Athanasiou, 2015; Chen et al., 2019). Compared with meniscus repair materials, the current research is more inclined to manufacture integrated materials for restoration and regeneration. Recent study of Baysan et al. revealed that a new type of hydrogel composite scaffold made of chitosan, loofah mat, and poly (-3-hydroxybutyrate-co-3-hydroxyvalerate) (PHBV) nanofibers is a promising material for engineering meniscus tissue (Baysan et al., 2022). Figure 2 and Table 2 present the typical investigations of meniscus rehabilitation hydrogels in meniscus repair and their outcomes.
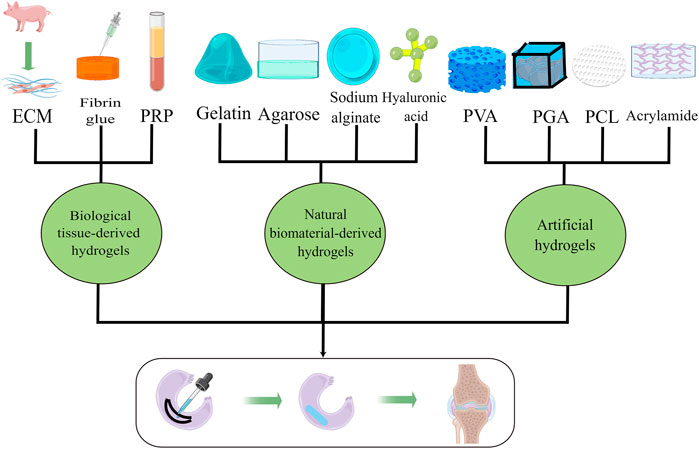
FIGURE 2. Multiple hydrogels used as repair materials for meniscus rehabilitation, including biological tissue-derived hydrogels, natural biomaterial-derived hydrogels, and artificial hydrogels.
Meniscus regeneration hydrogels
Cell-based meniscus regeneration hydrogels
For any foreign scaffold, its fusion with native tissue is essential to its functionality. The key to the fusion of hydrogels is the ability of cells to migrate from the normal tissue to the transplanted tissue, and cell-laden hydrogel may accelerate this fusion process. Autologous cells have been shown to play a good role in meniscus substitution (Kon et al., 2012), but their sources are relatively limited. Two papers by Jiang et al. showed that treated pig xenogeneic meniscus tissue could be incorporated with native tissue in the knee joint, but detectable rabbit-anti-pig antibody in the blood serum revealed that the problem of immune rejection requires further study (Jiang et al., 2012; Jiang D. et al., 2018). Compared with autologous cells and xenogenic cells, stem cells may be a more promising cell source in the field of meniscus regeneration research (Zhou et al., 2022). Multiple studies reported that stem cells are useful in replacing the injured meniscus and restoring its native function when combined with tissue engineering (Jacob et al., 2021; Bian et al., 2022). Basing in vitro and in vivo experiments, Li et al. proved that Apt/GF-scaffolds increased neomeniscal formation in rabbit critical-sized meniscectomies through mesenchymal stem cell (MSC)-specific recruitment (Li H. et al., 2022). Sasaki et al. isolated stem cells from adipose, and demonstrated that adipose-derived stem cells (ASCs)-seeded hydrogels preloaded with TGF-β3 enhanced healing of radial meniscal tears in an in vitro meniscal repair model (Sasaki et al., 2018). Zhang et al. suggested that adipose-derived stem cells (ASCs) encapsulated in a biodegradable poly (l-glutamic acid)/poly (ε-caprolactone) hydrogel effectively regenerated meniscus-like tissue in vivo and preserved the corresponding articular cartilage from degeneration over a 16 week period (Zhang K. et al., 2018). Hagmeijer et al., investigated the feasibility of a one-stage cell-based treatment for meniscus regeneration by augmenting a resorbable collagen-based implant with a combination of recycled meniscus cells and mesenchymal stromal cells (MSCs), and found that the new one-stage cell-based procedure for meniscus regeneration is feasible, and the stimulatory effect of MSCs towards meniscus cells was regulated by communication through gap junctions (Hagmeijer et al., 2019). Baek et al. examined meniscus tissue generation from different human cell sources including meniscus cells derived from vascular and avascular regions, human bone marrow-derived mesenchymal stem cells, synovial cells, and cells from the infrapatellar fat pad (IPFP), and proved that IPFP cells have potential for use in cell-based meniscus regeneration strategies (Baek et al., 2018). Zhong et al. revealed that rat bone marrow stem cells (BMSCs) mixed with decellularized meniscus extracellular matrix (mECM) hydrogel enabled full-thickness meniscus repair in an orthotopic rat model with in vitro 3D cell culture, in vivo rat subcutaneous implantation and orthotopic meniscus injury model (Zhong et al., 2020). Ding et al. reviewed a large number of research studies about meniscal repair and regeneration with mesenchymal stem cells from different sources, including bone marrow, peripheral blood, fat, and articular cavity synovium, and determined that current meniscus repair research is still in its infancy, being mostly confined to in vitro experiments and animal models. Good healing results may be achieved in animals, but not necessarily in humans. The repair ability of animals is different from that of humans, and meniscus injuries in some animals can be completely healed over a proper period of time without any treatment (Ding G. et al., 2022). Chew et al. analyzed four non-duplicate experiments and concluded that it is not evident that stem cells could repair the meniscus with durable neotissue which is comparable to the original meniscus (Chew et al., 2017). Further clinical trials with standard protocols and long-term follow-ups are required to reveal the influence of stem cells on meniscus regeneration.
Scaffold-based meniscus regeneration hydrogels
While the search for optimal cell sources is ongoing, some researchers suggested that scaffold-based meniscus regeneration hydrogels may be more important than cells in meniscus regeneration (Chen Y. et al., 2017). Sun et al. found that 3D-bioprinted TCM meniscus not only restored the anisotropy of native healthy meniscus with PBV infiltration and better shape retention, but better maintained joint function and prevented secondary joint degeneration, which demonstrated that the environment of the joint cavity affects meniscus phenotype (Sun et al., 2021). In the meniscus regeneration system, the composition of hydrogels is undoubtedly an important factor affecting cell phenotype changes for the interaction between cells and hydrogels is constant. The ECM scaffold is a native tissue-based strategy for meniscus repair (Zhang Z. et al., 2018). A variety of ECM hydrogels have been reported to be effective in meniscus regeneration (Wu et al., 2015; Yuan et al., 2017; Chen et al., 2019; Zhong et al., 2020; Guo et al., 2021). As the main component of ECM, type I, II and III collagen were all repported to be associated with cultivation of meniscus cells (Mueller et al., 1999; Oda et al., 2015; Wang C. et al., 2020). Reaearch of Mueller et al. showed that type II matrix is beneficial to meniscus regeneration for its resistance to cell-mediated contracture (Mueller et al., 1999), and study of Wang et al. revealed that type III collagen deficiency inhibits meniscal synthesis through mediating the early stage of type II collagen fibrillogenesis and chondrocyte mechanotransduction (Wang C. et al., 2020), but in vitro and in vivo experiments of Hagmeijer et al. and Oda et al. demonstrated that type I collagen scaffold promotes meniscus regeneration via enhancing cellular communication by gap junctions and suppressing inflammation (Oda et al., 2015; Hagmeijer et al., 2019). Tissue-derived scaffolds include ECM scaffolds and other scaffolds prepared from raw materials extracted from biological tissues, such as animal meniscus, small intestinal submucosa, silk, and PRP (Kwon et al., 2019). Scaffolds derived from the menisci of cattle, pigs, rats, and horses were all proven to be effective in meniscus tissue engineering (Yamasaki et al., 2005; Stapleton et al., 2008; Yamasaki et al., 2008; Stapleton et al., 2011; Chen et al., 2015; Visser et al., 2015; Wu et al., 2015; Yuan et al., 2017; Ruprecht et al., 2019; Zhong et al., 2020). As a collagenous biomaterial, small intestinal submucosa was confirmed by many studies to be a capable scaffold for meniscus regeneration (Cook et al., 2001; Gastel et al., 2001; Welch et al., 2002; Cook et al., 2006; Bradley et al., 2007; Tan et al., 2010). Studies by Wu et al. and Yan et al. reported that silk scaffolds coated with platelet-rich gel or collagen can promote functional meniscus regeneration and prevent osteoarthritis (Wu et al., 2019; Yan et al., 2019). PRP is a biological product with scaffolding properties, and it has been suggested to support meniscus healing (Kemmochi et al., 2018; Liu et al., 2019; Kurnaz and Balta, 2020). Natural hydrogel scaffolds derived from sodium alginate, agarose, hyaluronic acid, and gelatin may be the most intensively studied medical biomaterials in the field of tissue engineering. Numerous studies demonstrate that such scaffolds have very good application prospects in the field of meniscus regeneration (Gunja et al., 2009; Echave et al., 2017; Koh et al., 2017; Kremer et al., 2017; Kim et al., 2018; Rastogi and Kandasubramanian, 2019; Berton et al., 2020; Resmi et al., 2020; Abpeikar et al., 2021; Lan et al., 2021; Salahuddin et al., 2021). A study of Bahcecioglu et al. revealed that hydrogels of agarose, and methacrylated gelatin (GelMA) and hyaluronic acid are more supportive for in vitro meniscus regeneration than three dimensional printed polycaprolactone scaffolds, and agarose and MeHA could be used for the regeneration of the inner region of meniscus while GelMA for the outer region (Bahcecioglu et al., 2019b). Synthetic hydrogel scaffolds are formed by physical and chemical crosslinking of polymer materials, such as polyvinyl alcohol (PVA), polycaprolactone (PCL), poly (glycolic acid) (PGA), poly-L/DL-lactide (PLDLA), polyethylene glycol (PEG), and polyethylene oxide (PEO) (Burgos-Morales et al., 2021). With advances in technology, standards for the biological activity, mechanical properties, and ease of modification are rising for synthetic materials. In the field of meniscus repair, synthetic materials show good performance (Esposito et al., 2013; Aufderheide and Athanasiou, 2015; Hayes et al., 2016; Marrella et al., 2018; Bahcecioglu et al., 2019a; Cojocaru et al., 2020; Li et al., 2020). Holloway et al. reinforced poly (vinyl alcohol) (PVA) hydrogels with ultrahigh molecular weight polyethylene (UHMWPE) and PP fibers, and suggested that the poly (vinyl alcohol)-based fibrous composite was a possible candidate for meniscal tissue replacement after a series of mechanical evaluations (Holloway et al., 2010). Sthijns et al. reviewed numerous published articles and suggested that synthetic materials can regulate ECM secretions by affecting cellular metabolism and the metabolic state (Sthijns, van Blitterswijk, and LaPointe, 2021), which is promising for the field of biomedical materials. However, since most people assume that synthetic materials may cause unexpected side effects, there is still a long way to go before synthetic materials can be used on a large scale in the human body. Besides, among these four hydrogels (ECM scaffolds, Natrual hydrogel scaffolds, Tissur-derived scaffolds, Synthetic hydrogel scaffolds), which one is more suitable for meniscus regeneration needs to be further confirmed by more studies focusing on the interaction between cells and hydrogels.
Agent-stimulated meniscus regeneration hydrogels
The human body is an organic whole, but the microenvironment of each local area is unique, differing in parameters such as water content, ion concentration, and pH. Self-assembled polypeptide hydrogels are normally stable and can aggregate spontaneously by forming non-covalent bonds between polypeptide molecules, such as hydrogen bonds, electrostatic interactions, and π-π stacking interactions. Okuno et al. demonstrated that a self-assembling peptide hydrogel scaffold presented a repair and regeneration effect in the meniscus defect of a rabbit model (Okuno et al., 2021).
Non-responsive hydrogels can be classified as traditional hydrogels, and their functions mainly depend on drugs, proteins, and factors loaded on the hydrogels. Research by Zhang et al. suggested that local administration of simvastatin stimulated intrinsic healing of the meniscus (Zhang et al., 2016). Tanaka et al. reported that simvastatin-conjugated gelatin hydrogel could restrain arthritis progression caused by medial meniscectomy (Tanaka et al., 2019). Additionally, PRP-laden hydrogels were shown to exhibit better healing effects than PRP or hydrogel alone in meniscus defects (Ishida et al., 2007; Qi et al., 2021). Other agents loaded on hydrogels also play an important role in regulating the effects of hydrogels. Hydrogels incorporated with transforming growth factor (TGF) were proven to be alternative scaffolds for meniscus healing (Sasaki et al., 2018; Chen et al., 2020). Another growth factor, fibroblast growth factor 2, was indicated to enhance meniscus regeneration with gelatin hydrogel in a rabbit model (Narita et al., 2012).
Compared with non-responsive hydrogels, stimulus-responsive hydrogels can respond to changes in environmental conditions. Sources of stimulation can be divided into internal stimuli (e.g., pH, redox, enzyme, electricity, and glucose) and external stimuli (e.g., heat, light, mechanical force, magnetic field, and ultrasound). Kim et al. utilized enzyme-based approaches to fabricate tyrosinase (TYR)-crosslinked tissue adhesive hydrogels for meniscus repair and found that these approaches exhibited strong biocompatibility and tissue adhesion (Kim et al., 2018). External stimulus-responsive hydrogels, such as photocrosslinking collagen, photo-curable hydrogel, and thermo-sensitive hydrogel, have been reported as prominent gels for meniscus regeneration (Abpeikar et al., 2021; Karami et al., 2021; Wang et al., 2021). A research by Chen and Cheng found that a thermo-responsive chitosan-graft-poly (N-isopropylacrylamide) injectable hydrogel preserves the viability and phenotypic morphology of chondrocytes and meniscus cells (Chen and Cheng, 2006). Due to the lack of knowledge on the changes in internal factors and signaling pathways after meniscus injury, meniscus repair response hydrogels mainly respond to exogenous stimuli at present. More studies are needed to clarify the underlying mechanisms and, thus, facilitate the creation of more efficient meniscus regeneration hydrogels. Figure 3 and Table 3 exhibit the symbolic experiments of meniscus regeneration hydrogels in meniscus repair and their outcomes.
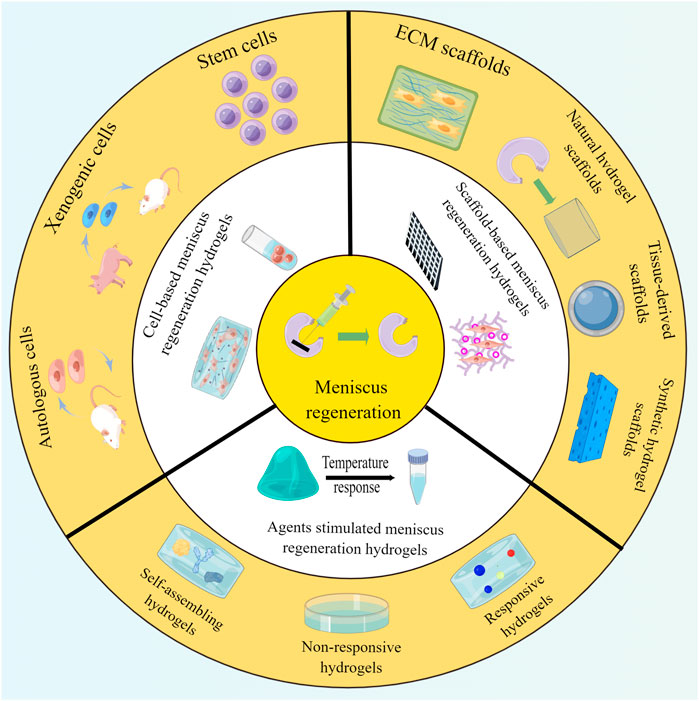
FIGURE 3. Various hydrogels adopted for meniscus regeneration, including cell-based, scaffold-based, and agent-stimulated meniscus regeneration hydrogels.
Conclusions and outlook
In this review, we presented a discussion on the application of hydrogels to the repair of meniscus damage, organized by the different types and functions of hydrogels, and we described each functionalized hydrogel in detail based on previous studies. Early studies focused on hydrogels for meniscus repair, but with the advancement of material technology and biomedicine, functionalized hydrogels are being developed to achieve the goals of being more bionic and promoting regeneration. Currently, the combination of tissue-derived materials, natural materials, composite materials, nanoparticles, organic polymer materials, and hydrogels has opened up a good future for meniscus repair projects. Meniscus repair will also become more accurate with the help of 3D printing technology.
However, the microenvironment of the meniscus is not static, its connection with surrounding tissue cells will change in different periods, and cells will also remodel ECM components to achieve an environment that is optimal for their existence and function. Intuitively, the repair effect of cell-seeded scaffolds should be better than that of cell-free scaffolds. The study of Numpaisal et al. confirmed that fibrin scaffolds loaded with meniscus cells have better repair effects than scaffolds without cells in radial meniscus tear in hind limbs of young cows (Numpaisal et al., 2017). Basic science researchers are more inclined to reckon that cell-seeded scaffolds are superior than cell-free scaffolds in tissue engineering (Pereira et al., 2011; Kon et al., 2012), and unconsciously pay little attention to convenience and operability of practical application. Two cell-free meniscal scaffolds, collagen-based CMI (Ivy Sports Medicine, Lochhamer, Germany) and polyurethane-based ACTIFIT (Ortheq Bioengineering, Londong, United Kingdom), have been reported to be safe for meniscus replacement, but the neonatal tissue was proved to be different from the native meniscus (Pereira et al., 2019). More high-quality studies are needed to prove the influence of cells on cell-free and cell-seeded scaffolds. With their good biocompatibility, capability for extrinsic substance delivery, and suitability for functional modification, hydrogels are a good choice for exogenous agent delivery and repair of tissue defects. The mechanical property of hydrogel is equally a problem that should be concerned. In order to further detect the regionally biochemical differences of hydrogels from different regions, Wu et al. fabricated regionally decellularized meniscal ECM hydrogels with different (outer, middle, and inner) zones of porcine meniscus, and found that the cell-seeded outer meniscus (OM) hydrogel had a nine-fold increase in peak compressive strengths (18.3 ± 3.6 vs. 2.1 ± 0.1 kPa) and a six-fold increase in initial modulus (9.9 ± 2.6 vs. 1.7 ± 0.2 kPa), the cell-seeded middle meniscus (MM) hydrogel experienced a 21-fold increase in peak compressive strengths (27.1 ± 4.6 vs. 1.3 ± 0.1 kPa) and a nine-fold increase in initial modulus (6.7 ± 1.6 vs. 0.8 ± 0.1 kPa), the cell-seeded inner meniscus (IM) hydrogel achieved a 22-fold increase in peak compressive strengths (26.0 ± 3.0 vs. 1.2 ± 0.2 kPa) and a nine-fold increase in initial modulus (9.4 ± 1.8 vs. 0.9 ± 0.2 kPa) over the IM hydrogel only (Wu et al., 2021). Ding et al. compared the scaffold properties of native meniscus, untreated extracellular matris (ECM) and decellularized ECM (dmECM) from porcine meniscus, and found that both dmECM and untreated ECM scaffolds had lower compressive modulus than native meniscus (181 ± 63 kPa, p < 0.001) and the native meniscus was relatively very stiff and showed significantly higher failure compression stresses (2030 ± 250 kPa, p < 0.001) (Ding Y. et al., 2022). From these two studies, it can be seen that although biological hydrogels can improve the mechanical strength by adding cells, they are still difficult to achieve the mechanical strength of normal meniscus. If the initial hydrogel is to achieve the mechanical level of normal meniscus, the biohydrogel must be modified. An et al. developed an injectable hydrogel based on fibrin (Fb) reinforced with Pluronic F127 (F127) and polymethyl methacrylate (PMMA), and proved that the gel was beneficial to meniscus repair with the in vivo segmental defect of the rabbit meniscus model, but the regenerated tissues of Fb/F127 (3.50 ± 0.35 MPa) and Fb/F127/PMMA (3.59 ± 0.89 MPa) was much higher than that of Fb (0.82 ± 0.05 MPa) but inferior to that of healthy tissue (6.63 ± 1.12 MPa) (An et al., 2021). Kobayashi et al. performed mechanical tests for compression and stress-relaxation among human meniscus and polyvinyl alcohol-hydrogel (PVA-H) with different water content, and detected that the human meniscus has unique viscoelastic properties and compressive strength values of approximately 3 MPa by cutting meniscal samples into small cubes for compression (Kobayashi et al., 2003). However, another report by Beaufils and Versonk demonstrated that human meniscus has a compression value of about 0.15 MPa (Beaufils, 2010). From these studies, we found that the mechanical properties of the meniscus may be different in different species, which may be an important issue to be considered in the future animal experimental research. At the same time, whether the addition of synthetic materials to biological materials will have negative effects on their biological-related properties while improving their mechanical properties, which still needs further study. When considering the mechanical strength of the graft, the poor adhesion between the stent and the surrounding tissue also affects the repair effect. Previous study of Karami et al. prestented a composite double-network hydrogel with a dissipative interface and robustly adheres to soft tissues such as cartilage and meniscus (the adhesion strength is up to 130 kPa) (Karami et al., 2018). The research revealed that chemical properties of hydrogels are easier to improve, but the mechanical strength of meniscus varies from region to region and different regions may have different requirements for adhesive strength of repair materials. Hydrogels that can smartly change adhension strength in different regions should be proposed in the future research.
Overall, this review systematically discussed the applications of different functionalized hydrogels in meniscus repair, focusing on extrinsic substance–delivery hydrogels, meniscus rehabilitation hydrogels, and meniscus regeneration hydrogels. Furthermore, the advantages and disadvantages of different functional hydrogels in meniscus repair were analyzed, and several unsolved problems were highlighted to inspire subsequent research. In summary, hydrogels have good prospects in the application of meniscus repair. Although a variety of hydrogels have been shown to exert positive healing effects in meniscus repair with animal models, there are still few reports of hydrogels achieving regenerative repair in humans. The mechanisms of meniscus development and injury need to be further studied, more multifunctional composite hydrogels that can achieve accurate regeneration of different parts of the meniscus in situ and stimulate collagen fibers to grow along the mechanical axis should be proposed, and more conclusive clinical trials should be conducted to screen the effects of these hydrogels.
Author contributions
Conceptualization, PJ and GZ; Literature Review and Writing—Original Draft Preparation, PJ, LL, XC, LC, and WZ; Drawing, XC; Writing—Review and Editing, PJ, LL, and GZ. All authors have read and agreed to the published version of the manuscript.
Funding
This research was funded by the National Natural Science Foundation of China (81860386), Guangxi Natural Science Foundation (2020GXNSFBA238016), Yangtze University Medical Innovation Fund (2022MIF03), Innovation and Entrepreneurship Training program for university students of Yangtze University (Yz2022303, ydc202201), China College Students' Innovation and Entrepreneurship Training Program (202210489020), and Jingzhou Science and Technology Plan Project (2022HC36).
Acknowledgments
We thank LetPub (www.letpub.com) for its linguistic assistance during the preparation of this manuscript. We also thank Figdraw (https://www.figdraw.com/static/index.html#/) for providing image drawing.
Conflict of interest
The authors declare that the research was conducted in the absence of any commercial or financial relationships that could be construed as a potential conflict of interest.
Publisher’s note
All claims expressed in this article are solely those of the authors and do not necessarily represent those of their affiliated organizations, or those of the publisher, the editors and the reviewers. Any product that may be evaluated in this article, or claim that may be made by its manufacturer, is not guaranteed or endorsed by the publisher.
References
Abazari, M., Akbari, T., Hasani, M., Sharifikolouei, E., Raoufi, M., Foroumadi, A., et al. (2022). Polysaccharide-based hydrogels containing herbal extracts for wound healing applications. Carbohydr. Polym. 294, 119808. doi:10.1016/j.carbpol.2022.119808
Abpeikar, Z., Javdani, M., Mirzaei, S. A., Alizadeh, A., Moradi, L., Soleimannejad, M., et al. (2021). Macroporous scaffold surface modified with biological macromolecules and piroxicam-loaded gelatin nanofibers toward meniscus cartilage repair. Int. J. Biol. Macromol. 183, 1327–1345. doi:10.1016/j.ijbiomac.2021.04.151
Akulo, K. A., Adali, T., Moyo, M. T. G., and Bodamyali, T. (2022). Intravitreal injectable hydrogels for sustained drug delivery in glaucoma treatment and therapy. Polym. (Basel) 14, 2359. doi:10.3390/polym14122359
An, Y. H., Kim, J. A., Yim, H. G., Han, W. J., Park, Y. B., Jin, P. H., et al. (2021). Meniscus regeneration with injectable Pluronic/PMMA-reinforced fibrin hydrogels in a rabbit segmental meniscectomy model. J. Tissue Eng. 12, 204173142110501. doi:10.1177/20417314211050141
Aufderheide, A. C., and Athanasiou, K. A. (2015). Comparison of scaffolds and culture conditions for tissue engineering of the knee meniscus. Tissue Eng. 11, 1095–1104. doi:10.1089/ten.2005.11.1095
Baek, J., Chen, X., Sovani, S., Jin, S., Grogan, S. P., and D'Lima, D. D. (2015). Meniscus tissue engineering using a novel combination of electrospun scaffolds and human meniscus cells embedded within an extracellular matrix hydrogel. J. Orthop. Res. 33, 572–583. doi:10.1002/jor.22802
Baek, J., Sovani, S., Choi, W., Jin, S., Grogan, S. P., and Lima, D. D. (2018). Meniscal tissue engineering using aligned collagen fibrous scaffolds: Comparison of different human cell sources. Tissue Eng. Part A 24, 81–93. doi:10.1089/ten.TEA.2016.0205
Bahcecioglu, G., Bilgen, B., Hasirci, N., and Hasirci, V. (2019a). Anatomical meniscus construct with zone specific biochemical composition and structural organization. Biomaterials 218, 119361. doi:10.1016/j.biomaterials.2019.119361
Bahcecioglu, G., Hasirci, N., Bilgen, B., and Hasirci, V. (2019b). Hydrogels of agarose, and methacrylated gelatin and hyaluronic acid are more supportive for in vitro meniscus regeneration than three dimensional printed polycaprolactone scaffolds. Int. J. Biol. Macromol. 122, 1152–1162. doi:10.1016/j.ijbiomac.2018.09.065
Banovetz, M. T., Roethke, L. C., Rodriguez, A. N., and LaPrade, R. F. (2022). Meniscal root tears: A decade of research on their relevant anatomy, biomechanics, diagnosis, and treatment. Arch. Bone Jt. Surg. 10, 366–380. doi:10.22038/abjs.2021.60054.2958
Baysan, G., Colpankan Gunes, O., Akokay, P., Husemoglu, R. B., Ertugruloglu, P., Ziylan Albayrak, A., et al. (2022). Loofah-chitosan and poly (-3-hydroxybutyrate-co-3-hydroxyvalerate) (PHBV) based hydrogel scaffolds for meniscus tissue engineering applications. Int. J. Biol. Macromol. 221, 1171–1183. doi:10.1016/j.ijbiomac.2022.09.031
Berton, A., Longo, U. G., Candela, V., Greco, F., Martina, F. M., Quattrocchi, C. C., et al. (2020). Quantitative evaluation of meniscal healing process of degenerative meniscus lesions treated with hyaluronic acid: A clinical and mri study. J. Clin. Med. 9, 2280. doi:10.3390/jcm9072280
Bian, Y., Wang, H., Zhao, X., and Weng, X. (2022). Meniscus repair: Up-to-date advances in stem cell-based therapy. Stem Cell Res. Ther. 13, 207. doi:10.1186/s13287-022-02863-7
Bradley, M. P., Fadale, P. D., Hulstyn, M. J., Muirhead, W. R., and Lifrak, J. T. (2007). Porcine small intestine submucosa for repair of goat meniscal defects. Orthopedics 30, 650–656. doi:10.3928/01477447-20070801-15
Braun, H. J., Wasterlain, A. S., and Dragoo, J. L. (2015). The use of PRP in ligament and meniscal healing. Sports Med. Arthrosc. Rev. 21, 206–212. doi:10.1097/jsa.0000000000000005
Burgos-Morales, O., Gueye, M., Lacombe, L., Nowak, C., Schmachtenberg, R., Hörner, M., et al. (2021). Synthetic biology as driver for the biologization of materials sciences. Mat. Today Bio 11, 100115. doi:10.1016/j.mtbio.2021.100115
Cao, Z., Wang, H., Chen, J., Zhang, Y., Mo, Q., Zhang, P., et al. (2022). Silk-based hydrogel incorporated with metal-organic framework nanozymes for enhanced osteochondral regeneration. Bioact. Mat. 20, 221–242. doi:10.1016/j.bioactmat.2022.05.025
Chambers, H. G., and Chambers, R. C. (2019). The natural history of meniscus tears. J. Pediatr. Orthop. 39, S53–S55. doi:10.1097/bpo.0000000000001386
Chen, C., Song, J., Qiu, J., and Zhao, J. (2020). Repair of a meniscal defect in a rabbit model through use of a thermosensitive, injectable, in situ crosslinked hydrogel with encapsulated bone mesenchymal stromal cells and transforming growth factor β1. Am. J. Sports Med. 48, 884–894. doi:10.1177/0363546519898519
Chen, J. P., and Cheng, T. H. (2006). Thermo-responsive chitosan-graft-poly(N-isopropylacrylamide) injectable hydrogel for cultivation of chondrocytes and meniscus cells. Macromol. Biosci. 6, 1026–1039. doi:10.1002/mabi.200600142
Chen, M., Feng, Z., Guo, W., Yang, D., Gao, S., Li, Y., et al. (2019). PCL-MECM-Based hydrogel hybrid scaffolds and meniscal fibrochondrocytes promote whole meniscus regeneration in a rabbit meniscectomy model. ACS Appl. Mat. Interfaces 11, 41626–41639. doi:10.1021/acsami.9b13611
Chen, S., Fu, P., Wu, H., and Pei, M. (2017a). Meniscus, articular cartilage and nucleus pulposus: A comparative review of cartilage-like tissues in anatomy, development and function. Cell Tissue Res. 370, 53–70. doi:10.1007/s00441-017-2613-0
Chen, Y. C., Chen, R. N., Jhan, H. J., Liu, D. Z., Ho, H. O., Mao, Y., et al. (2015). Development and characterization of acellular extracellular matrix scaffolds from porcine menisci for use in cartilage tissue engineering. Tissue Eng. Part C. Methods 21, 971–986. doi:10.1089/ten.tec.2015.0036
Chen, Y., Chen, J., Zhang, Z., Lou, K., Zhang, Q., Wang, S., et al. (2017b). Current advances in the development of natural meniscus scaffolds: Innovative approaches to decellularization and recellularization. Cell Tissue Res. 370, 41–52. doi:10.1007/s00441-017-2605-0
Cheng, J., Chen, Z., Liu, C., Zhong, M., Wang, S., Sun, Y., et al. (2021). Bone mesenchymal stem cell-derived exosome-loaded injectable hydrogel for minimally invasive treatment of spinal cord injury. Nanomedicine (Lond) 16, 1567–1579. doi:10.2217/nnm-2021-0025
Chew, E., Prakash, R., and Khan, W. (2017). Mesenchymal stem cells in human meniscal regeneration: A systematic review. Ann. Med. Surg. (Lond). 24, 3–7. doi:10.1016/j.amsu.2017.09.018
Cojocaru, D. G., Hondke, S., Krüger, J. P., Bosch, C., Croicu, C., Florescu, S., et al. (2020). Meniscus-shaped cell-free polyglycolic acid scaffold for meniscal repair in a sheep model. J. Biomed. Mat. Res. 108, 809–818. doi:10.1002/jbm.b.34435
Cook, J. L., Fox, D. B., Malaviya, P., Tomlinson, J. L., Farr, J., Kuroki, K., et al. (2006). Evaluation of small intestinal submucosa grafts for meniscal regeneration in a clinically relevant posterior meniscectomy model in dogs. J. Knee Surg. 19, 159–167. doi:10.1055/s-0030-1248100
Cook, J. L., Tomlinson, J. L., Arnoczky, S. P., Fox, D. B., Reeves Cook, C., and Kreeger, J. M. (2001). Kinetic study of the replacement of porcine small intestinal submucosa grafts and the regeneration of meniscal-like tissue in large avascular meniscal defects in dogs. Tissue Eng. 7, 321–334. doi:10.1089/10763270152044189
Dai, W. L., Zhang, H., Lin, Z. M., Shi, Z. J., and Wang, J. (2019). Efficacy of platelet-rich plasma in arthroscopic repair for discoid lateral meniscus tears. BMC Musculoskelet. Disord. 20, 113. doi:10.1186/s12891-019-2500-9
Das, S., Saha, D., Majumdar, S., and Giri, L. (2022). Imaging methods for the assessment of a complex hydrogel as an ocular drug delivery system for glaucoma treatment: Opportunities and challenges in preclinical evaluation. Mol. Pharm. 19, 733–748. doi:10.1021/acs.molpharmaceut.1c00831
Ding, G., Du, J., Hu, X., and Ao, Y. (2022a). Mesenchymal stem cells from different sources in meniscus repair and regeneration. Front. Bioeng. Biotechnol. 10, 796367. doi:10.3389/fbioe.2022.796367
Ding, Y., Zhang, W., Sun, B., Mo, X., and Wu, J. (2022b). Cyclic freeze-thaw grinding to decellularize meniscus for fabricating porous, elastic scaffolds. J. Biomed. Mat. Res. A 110, 1824–1839. doi:10.1002/jbm.a.37435
Dos Santos, A. M., Carvalho, S. G., Araujo, V. H. S., Carvalho, G. C., Gremião, M. P. D., and Chorilli, M. (2020). Recent advances in hydrogels as strategy for drug delivery intended to vaginal infections. Int. J. Pharm. X. 590, 119867. doi:10.1016/j.ijpharm.2020.119867
Echave, M. C., Saenz del Burgo, L., Pedraz, J. L., and Orive, G. (2017). Gelatin as biomaterial for tissue engineering. Curr. Pharm. Des. 23, 3567–3584. doi:10.2174/0929867324666170511123101
Esposito, A. R., Moda, M., Cattani, S. M., de Santana, G. M., Barbieri, J. A., Munhoz, M. M., et al. (2013). PLDLA/PCL-T scaffold for meniscus tissue engineering. Biores. Open Access 2, 138–147. doi:10.1089/biores.2012.0293
Forriol, F., Ripalda, P., Duart, J., Esparza, R., and Gortazar, A. R. (2014). Meniscal repair possibilities using bone morphogenetic protein-7. Injury 45 (4), S15–S21. doi:10.1016/S0020-1383(14)70005-1
Fu, X. N., Li, H. W., Du, N., Liang, X., He, S. H., Guo, K. J., et al. (2020). Erythropoietin enhances meniscal regeneration and prevents osteoarthritis formation in mice. Am. J. Transl. Res. 12, 6464–6477.
Gastel, J. A., Muirhead, W. R., Lifrak, J. T., Fadale, P. D., Hulstyn, M. J., and Labrador, D. P. (2001). Meniscal tissue regeneration using a collagenous biomaterial derived from porcine small intestine submucosa. Arthrosc. J. Arthrosc. Relat. Surg. 17, 151–159. doi:10.1053/jars.2001.20959
Gunes, O. C., Kara, A., Baysan, G., Bugra, H. R., Akokay, P., Ziylan, A. A., et al. (2022). Fabrication of 3D Printed poly(lactic acid) strut and wet-electrospun cellulose nano fiber reinforced chitosan-collagen hydrogel composite scaffolds for meniscus tissue engineering. J. Biomater. Appl. 885, 683–697. doi:10.1177/08853282221109339
Gunja, N. J., Huey, D. J., James, R. A., and Athanasiou, K. A. (2009). Effects of agarose mould compliance and surface roughness on self-assembled meniscus-shaped constructs. J. Tissue Eng. Regen. Med. 3, 521–530. doi:10.1002/term.191
Guo, W., Chen, M., Wang, Z., Tian, Y., Zheng, J., Gao, S., et al. (2021). 3D-printed cell-free PCL-MECM scaffold with biomimetic micro-structure and micro-environment to enhance in situ meniscus regeneration. Bioact. Mat. 6, 3620–3633. doi:10.1016/j.bioactmat.2021.02.019
Hagmeijer, M. H., Vonk, L. A., Fenu, M., van Keep, Y. W., Krych, A. J., and Saris, D. B. (2019). Meniscus regeneration combining meniscus and mesenchymal stromal cells in a degradable meniscus implant: An in vitro study. Eur. Cell. Mat. 38, 51–62. doi:10.22203/ecm.v038a05
Hao, L., Tianyuan, Z., Zhen, Y., Fuyang, C., Jiang, W., Zineng, Y., et al. (2021). Biofabrication of cell-free dual drug-releasing biomimetic scaffolds for meniscal regeneration. Biofabrication 14 (1), 015001. doi:10.1088/1758-5090/ac2cd7
Hayes, J. C., Curley, C., Tierney, P., and Kennedy, J. E. (2016). Biomechanical analysis of a salt-modified polyvinyl alcohol hydrogel for knee meniscus applications, including comparison with human donor samples. J. Mech. Behav. Biomed. Mat. 56, 156–164. doi:10.1016/j.jmbbm.2015.11.011
Heo, J., Koh, R. H., Shim, W., Kim, H. D., Yim, H. G., and Hwang, N. S. (2016). Riboflavin-induced photo-crosslinking of collagen hydrogel and its application in meniscus tissue engineering. Drug Deliv. Transl. Res. 6, 148–158. doi:10.1007/s13346-015-0224-4
Herrera Millar, V. R., Canciani, B., Mangiavini, L., Filipe, J. F. S., Aidos, L., Pallaoro, M., et al. (2022). Endostatin in 3D fibrin hydrogel scaffolds promotes chondrogenic differentiation in swine neonatal meniscal cells. Biomedicines 10, 2415. doi:10.3390/biomedicines10102415
Hoang, D. M., Pham, P. T., Bach, T. Q., Ngo, A. T. L., Nguyen, Q. T., Phan, T. T. K., et al. (2022). Stem cell-based therapy for human diseases. Sig. Transduct. Target. Ther. 7, 272. doi:10.1038/s41392-022-01134-4
Holloway, J. L., Lowman, A. M., and Palmese, G. R. (2010). Mechanical evaluation of poly(vinyl alcohol)-based fibrous composites as biomaterials for meniscal tissue replacement. Acta Biomater. 6, 4716–4724. doi:10.1016/j.actbio.2010.06.025
Ishida, K., Kuroda, R., Miwa, M., Tabata, Y., Hokugo, A., Kawamoto, T., et al. (2007). The regenerative effects of platelet-rich plasma on meniscal cells in vitro and its in vivo application with biodegradable gelatin hydrogel. Tissue Eng. 13, 1103–1112. doi:10.1089/ten.2006.0193
Jacob, G., Shimomura, K., Krych, A. J., and Nakamura, N. (2019). The meniscus tear: A review of stem cell therapies. Cells 9, 92. doi:10.3390/cells9010092
Jacob, S., Nair, A. B., Shah, J., Sreeharsha, N., Gupta, S., and Shinu, P. (2021). Emerging role of hydrogels in drug delivery systems, tissue engineering and wound management. Pharmaceutics 13, 357. doi:10.3390/pharmaceutics13030357
Janarthanan, G., Kim, J. H., Kim, I., Lee, C., Chung, E. J., and Noh, I. (2022). Manufacturing of self-standing multi-layered 3D-bioprinted alginate-hyaluronate constructs by controlling the cross-linking mechanisms for tissue engineering applications. Biofabrication 14, 035013. doi:10.1088/1758-5090/ac6c4c
Jansen, K., Schuurmans, C. C. L., Jansen, J., Masereeuw, R., and Vermonden, T. (2017). Hydrogel-based cell therapies for kidney regeneration: Current trends in biofabrication and in vivo repair. Curr. Pharm. Des. 23, 3845–3857. doi:10.2174/1381612823666170710155726
Jiang, D., Zhang, Z. Z., Zhao, F., Wang, S. J., Qi, Y. S., Zhao, L. H., et al. (2018a). The radiated deep-frozen xenogenic meniscal tissue regenerated the total meniscus with chondroprotection. Sci. Rep. 8, 9041. doi:10.1038/s41598-018-27016-w
Jiang, D., Zhao, L. H., Tian, M., Zhang, J. Y., and Yu, J. K. (2012). Meniscus transplantation using treated xenogeneic meniscal tissue: Viability and chondroprotection study in rabbits. Arthrosc. J. Arthrosc. Relat. Surg. 28, 1147–1159. doi:10.1016/j.arthro.2012.01.001
Jiang, G., Li, S., Yu, K., He, B., Hong, J., Xu, T., et al. (2021). A 3D-printed PRP-GelMA hydrogel promotes osteochondral regeneration through M2 macrophage polarization in a rabbit model. Acta Biomater. 128, 150–162. doi:10.1016/j.actbio.2021.04.010
Jiang, T., Wang, T., Li, T., Ma, Y., Shen, S., He, B., et al. (2018b). Enhanced transdermal drug delivery by transfersome-embedded oligopeptide hydrogel for topical chemotherapy of melanoma. ACS Nano 12, 9693–9701. doi:10.1021/acsnano.8b03800
Karami, P., Nasrollahzadeh, N., Wyss, C., O'Sullivan, A., Broome, M., Procter, P., et al. (2021). An intrinsically-adhesive family of injectable and photo-curable hydrogels with functional physicochemical performance for regenerative medicine. Macromol. Rapid Commun. 42, e2000660. doi:10.1002/marc.202000660
Karami, P., Wyss, C. S., Khoushabi, A., Schmocker, A., Broome, M., Moser, C., et al. (2018). Composite double-network hydrogels to improve adhesion on biological surfaces. ACS Appl. Mat. Interfaces 10, 38692–38699. doi:10.1021/acsami.8b10735
Kemmochi, M., Sasaki, S., Takahashi, M., Nishimura, T., Aizawa, C., and Kikuchi, J. (2018). The use of platelet-rich fibrin with platelet-rich plasma support meniscal repair surgery. J. Orthop. 15, 711–720. doi:10.1016/j.jor.2018.05.006
Kim, J., and Bonassar, L. J. (2022). Controlling collagen gelation pH to enhance biochemical, structural, and biomechanical properties of tissue-engineered menisci. J. Biomed. Mat. Res. A 22, 778. doi:10.1002/jbm.a.37464
Kim, S. H., An, Y. H., Kim, H. D., Kim, K., Lee, S. H., Yim, H. G., et al. (2018). Enzyme-mediated tissue adhesive hydrogels for meniscus repair. Int. J. Biol. Macromol. 110, 479–487. doi:10.1016/j.ijbiomac.2017.12.053
Kobayashi, M., Toguchida, J., and Oka, M. (2003). Development of an artificial meniscus using polyvinyl alcohol-hydrogel for early return to, and continuance of, athletic life in sportspersons with severe meniscus injury. I: Mechanical evaluation. Knee 10, 47–51. doi:10.1016/s0968-0160(02)00152-7
Koh, R. H., Jin, Y., Kang, B. J., and Hwang, N. S. (2017). Chondrogenically primed tonsil-derived mesenchymal stem cells encapsulated in riboflavin-induced photocrosslinking collagen-hyaluronic acid hydrogel for meniscus tissue repairs. Acta Biomater. 53, 318–328. doi:10.1016/j.actbio.2017.01.081
Kon, E., Filardo, G., Tschon, M., Fini, M., Giavaresi, G., Marchesini Reggiani, L., et al. (2012). Tissue engineering for total meniscal substitution: Animal study in sheep model-results at 12 months. Tissue Eng. Part A 18, 1573–1582. doi:10.1089/ten.tea.2011.0572
Kremer, A., Ribitsch, I., Reboredo, J., Dürr, J., Egerbacher, M., Jenner, F., et al. (2017). Three-dimensional coculture of meniscal cells and mesenchymal stem cells in collagen type I hydrogel on a small intestinal matrix-A pilot study toward equine meniscus tissue engineering. Tissue Eng. Part A 23, 390–402. doi:10.1089/ten.tea.2016.0317
Krych, A. J., Reardon, P. J., Johnson, N. R., Mohan, R., Peter, L., Levy, B. A., et al. (2017). Non-operative management of medial meniscus posterior horn root tears is associated with worsening arthritis and poor clinical outcome at 5-year follow-up. Knee Surg. Sports Traumatol. Arthrosc. 25, 383–389. doi:10.1007/s00167-016-4359-8
Kurnaz, R., and Balta, O. (2020). Effect of platelet-rich plasma and platelet-rich fibrin matrix on healing of vertical meniscal tears in a rabbit model. Acta Orthop. Traumatol. Turc. 54, 186–195. doi:10.5152/j.aott.2020.02.20
Kwon, H., Brown, W. E., Lee, C. A., Wang, D., Paschos, N., Hu, J. C., et al. (2019). Surgical and tissue engineering strategies for articular cartilage and meniscus repair. Nat. Rev. Rheumatol. 15, 550–570. doi:10.1038/s41584-019-0255-1
Lan, X., Ma, Z., Szojka, A. R. A., Kunze, M., Mulet-Sierra, A., Vyhlidal, M. J., et al. (2021). TEMPO-oxidized cellulose nanofiber-alginate hydrogel as a bioink for human meniscus tissue engineering. Front. Bioeng. Biotechnol. 9, 766399. doi:10.3389/fbioe.2021.766399
Li, H., Zhao, T., Cao, F., Deng, H., He, S., Li, J., et al. (2022a). Integrated bioactive scaffold with aptamer-targeted stem cell recruitment and growth factor-induced pro-differentiation effects for anisotropic meniscal regeneration. Bioeng. Transl. Med. 7, e10302. doi:10.1002/btm2.10302
Li, S., Dong, Q., Peng, X., Chen, Y., Yang, H., Xu, W., et al. (2022b2022). Self-healing hyaluronic acid nanocomposite hydrogels with platelet-rich plasma impregnated for skin regeneration. ACS Nano 16, 11346–11359. doi:10.1021/acsnano.2c05069
Li, Y., Liu, Y., and Guo, Q. (2021). Silk fibroin hydrogel scaffolds incorporated with chitosan nanoparticles repair articular cartilage defects by regulating TGF-β1 and BMP-2. Arthritis Res. Ther. 23, 50. doi:10.1186/s13075-020-02382-x
Li, Z., Chen, Z., Chen, H., Chen, K., Tao, W., Ouyang, X. K., et al. (2022c). Polyphenol-based hydrogels: Pyramid evolution from crosslinked structures to biomedical applications and the reverse design. Bioact. Mat. 17, 49–70. doi:10.1016/j.bioactmat.2022.01.038
Li, Z., Wu, N., Cheng, J., Sun, M., Yang, P., Zhao, F., et al. (2020). Biomechanically, structurally and functionally meticulously tailored polycaprolactone/silk fibroin scaffold for meniscus regeneration. Theranostics 10, 5090–5106. doi:10.7150/thno.44270
Li, Z., Zhao, T., Ding, J., Gu, H., Wang, Q., Wang, Y., et al. (2022d). A reactive oxygen species-responsive hydrogel encapsulated with bone marrow derived stem cells promotes repair and regeneration of spinal cord injury. Bioact. Mat. 19, 550–568. doi:10.1016/j.bioactmat.2022.04.029
Liang, Y., Idrees, E., Szojka, A. R. A., Andrews, S. H. J., Kunze, M., Mulet-Sierra, A., et al. (2018). Chondrogenic differentiation of synovial fluid mesenchymal stem cells on human meniscus-derived decellularized matrix requires exogenous growth factors. Acta Biomater. 80, 131–143. doi:10.1016/j.actbio.2018.09.038
Lim, H. C., Bae, J. H., Wang, J. H., Seok, C. W., and Kim, M. K. (2010). Non-operative treatment of degenerative posterior root tear of the medial meniscus. Knee Surg. Sports Traumatol. Arthrosc. 18, 535–539. doi:10.1007/s00167-009-0891-0
Lin, D., Lei, L., Shi, S., and Li, X. (2019). Stimulus-responsive hydrogel for ophthalmic drug delivery. Macromol. Biosci. 19, e1900001. doi:10.1002/mabi.201900001
Lin, J., Wang, L., Lin, J., and Liu, Q. (2021). Dual delivery of TGF-β3 and ghrelin in microsphere/hydrogel systems for cartilage regeneration. Molecules 26, 5732. doi:10.3390/molecules26195732
Liu, F., Xu, H., and Huang, H. (2019). A novel kartogenin-platelet-rich plasma gel enhances chondrogenesis of bone marrow mesenchymal stem cells in vitro and promotes wounded meniscus healing in vivo. Stem Cell Res. Ther. 10, 201. doi:10.1186/s13287-019-1314-x
Liu, Y., Wang, M., Luo, Y., Liang, Q., Yu, Y., Chen, F., et al. (2021). Enhancing stem cell therapy for cartilage repair in osteoarthritis-A hydrogel focused approach. Gels 7, 263. doi:10.3390/gels7040263
Liu, Z., Tang, W., Liu, J., Han, Y., Yan, Q., Dong, Y., et al. (2022). A novel sprayable thermosensitive hydrogel coupled with zinc modified metformin promotes the healing of skin wound. Bioact. Mat. 20, 610–626. doi:10.1016/j.bioactmat.2022.06.008
Longo, U. G., Loppini, M., Forriol, F., Romeo, G., Maffulli, N., and Denaro, V. (2012). Advances in meniscal tissue engineering. Stem Cells Int. 2012, 1–7. doi:10.1155/2012/420346
Lynch, C. R., Kondiah, P. P. D., Choonara, Y. E., du Toit, L. C., Ally, N., and Pillay, V. (2020). Hydrogel biomaterials for application in ocular drug delivery. Front. Bioeng. Biotechnol. 8, 228. doi:10.3389/fbioe.2020.00228
Ma, J., and Huang, C. (2020). Composition and mechanism of three-dimensional hydrogel system in regulating stem cell fate. Tissue Eng. Part B Rev. 26, 498–518. doi:10.1089/ten.teb.2020.0021
Madl, C. M., and Heilshorn, S. C. (2018). Engineering hydrogel microenvironments to recapitulate the stem cell niche. Annu. Rev. Biomed. Eng. 20, 21–47. doi:10.1146/annurev-bioeng-062117-120954
Maisani, M., Sindhu, K. R., Fenelon, M., Siadous, R., Rey, S., Mantovani, D., et al. (2018). Prolonged delivery of BMP-2 by a non-polymer hydrogel for bone defect regeneration. Drug Deliv. Transl. Res. 8, 178–190. doi:10.1007/s13346-017-0451-y
Marrella, A., Lagazzo, A., Dellacasa, E., Pasquini, C., Finocchio, E., Barberis, F., et al. (2018). 3D porous gelatin/PVA hydrogel as meniscus substitute using alginate micro-particles as porogens. Polym. (Basel) 10, 380. doi:10.3390/polym10040380
Mills, D. K., Luo, Y., Elumalai, A., Esteve, S., Karnik, S., and Yao, S. (2020). Creating structured hydrogel microenvironments for regulating stem cell differentiation. Gels 6, 47. doi:10.3390/gels6040047
Mueller, S. M., Shortkroff, S., Schneider, T. O., Breinan, H. A., Yannas, I. V., and Spector, M. (1999). Meniscus cells seeded in type I and type II collagen-GAG matrices in vitro. Biomaterials 20, 701–709. doi:10.1016/s0142-9612(98)00189-6
Nadine, S., Correia, C. R., and Mano, J. F. (2022). Engineering immunomodulatory hydrogels and cell-laden systems towards bone regeneration. Biomater. Adv. 140, 213058. doi:10.1016/j.bioadv.2022.213058
Narayanaswamy, R., and Torchilin, V. P. (2019). Hydrogels and their applications in targeted drug delivery. Molecules 24, 603. doi:10.3390/molecules24030603
Narita, A., Takahara, M., Sato, D., Ogino, T., Fukushima, S., Kimura, Y., et al. (2012). Biodegradable gelatin hydrogels incorporating fibroblast growth factor 2 promote healing of horizontal tears in rabbit meniscus. Arthrosc. J. Arthrosc. Relat. Surg. 28, 255–263. doi:10.1016/j.arthro.2011.08.294
Norouzi, M., Nazari, B., and Miller, D. W. (2016). Injectable hydrogel-based drug delivery systems for local cancer therapy. Drug Discov. Today 21, 1835–1849. doi:10.1016/j.drudis.2016.07.006
Numpaisal, P. O., Rothrauff, B. B., Gottardi, R., Chien, C. L., and Tuan, R. S. (2017). Rapidly dissociated autologous meniscus tissue enhances meniscus healing: An in vitro study. Connect. Tissue Res. 58, 355–365. doi:10.1080/03008207.2016.1245727
Oda, S., Otsuki, S., Kurokawa, Y., Hoshiyama, Y., Nakajima, M., and Neo, M. (2015). A new method for meniscus repair using type I collagen scaffold and infrapatellar fat pad. J. Biomater. Appl. 29, 1439–1448. doi:10.1177/0885328215568984
Okuno, N., Otsuki, S., Aoyama, J., Nakagawa, K., Murakami, T., Ikeda, K., et al. (2021). Feasibility of a self-assembling peptide hydrogel scaffold for meniscal defect: An in vivo study in a rabbit model. J. Orthop. Res. 39, 165–176. doi:10.1002/jor.24841
Ozeki, N., Muneta, T., Koga, H., Katagiri, H., Otabe, K., Okuno, M., et al. (2013). Transplantation of Achilles tendon treated with bone morphogenetic protein 7 promotes meniscus regeneration in a rat model of massive meniscal defect. Arthritis Rheum. 65, 2876–2886. doi:10.1002/art.38099
Pan, Z., Wu, Y., Zhang, X., Fu, Q., Li, J., Yang, Y., et al. (2017). Delivery of epidermal growth factor receptor inhibitor via a customized collagen scaffold promotes meniscal defect regeneration in a rabbit model. Acta Biomater. 62, 210–221. doi:10.1016/j.actbio.2017.07.008
Pereira, H., Fatih Cengiz, I., Gomes, S., Espregueira-Mendes, J., Ripoll, P. L., Monllau, J. C., et al. (2019). Meniscal allograft transplants and new scaffolding techniques. EFORT Open Rev. 4, 279–295. doi:10.1302/2058-5241.4.180103
Pereira, H., Frias, A. M., Oliveira, J. M., Espregueira-Mendes, J., and Reis, R. L. (2011). Tissue engineering and regenerative medicine strategies in meniscus lesions. Arthrosc. J. Arthrosc. Relat. Surg. 27, 1706–1719. doi:10.1016/j.arthro.2011.08.283
Perinelli, D. R., Campana, R., Skouras, A., Bonacucina, G., Cespi, M., Mastrotto, F., et al. (2018). Chitosan loaded into a hydrogel delivery system as a strategy to treat vaginal Co-infection. Pharmaceutics 10, 23. doi:10.3390/pharmaceutics10010023
Petersen, W., Achtnich, A., Lattermann, C., and Kopf, S. (2015). The treatment of non-traumatic meniscus lesions. Dtsch. Arztebl. Int. 112, 705–713. doi:10.3238/arztebl.2015.0705
Popescu, M. B., Carp, M., Tevanov, I., Nahoi, C. A., Stratila, M. A., Haram, O. M., et al. (2020). Isolated meniscus tears in adolescent patients treated with platelet-rich plasma intra-articular injections: 3-Month clinical outcome. Biomed. Res. Int. 2020, 1–5. doi:10.1155/2020/8282460
Qi, Y., Tang, R., Shi, Z., Feng, G., and Zhang, W. (2021). Wnt5a/Platelet-rich plasma synergistically inhibits IL-1β-induced inflammatory activity through NF-κB signaling pathway and prevents cartilage damage and promotes meniscus regeneration. J. Tissue Eng. Regen. Med. 15, 612–624. doi:10.1002/term.3198
Qiu, M., Chen, D., Shen, C., Shen, J., Zhao, H., and He, Y. (2016). Platelet-rich plasma-loaded poly(d, l-lactide)-Poly(ethylene glycol)-Poly(d, l-lactide) hydrogel dressing promotes full-thickness skin wound healing in a rodent model. Int. J. Mol. Sci. 17, 1001. doi:10.3390/ijms17071001
Rastogi, P., and Kandasubramanian, B. (2019). Review of alginate-based hydrogel bioprinting for application in tissue engineering. Biofabrication 11, 042001. doi:10.1088/1758-5090/ab331e
Resmi, R., Parvathy, J., John, A., and Joseph, R. (2020). Injectable self-crosslinking hydrogels for meniscal repair: A study with oxidized alginate and gelatin. Carbohydr. Polym. 234, 115902. doi:10.1016/j.carbpol.2020.115902
Romanazzo, S., Vedicherla, S., Moran, C., and Kelly, D. J. (2018). Meniscus ECM-functionalised hydrogels containing infrapatellar fat pad-derived stem cells for bioprinting of regionally defined meniscal tissue. J. Tissue Eng. Regen. Med. 12, e1826–e1835. doi:10.1002/term.2602
Ruprecht, J. C., Waanders, T. D., Rowland, C. R., Nishimuta, J. F., Glass, K. A., Stencel, J., et al. (2019). Meniscus-derived matrix scaffolds promote the integrative repair of meniscal defects. Sci. Rep. 9, 8719. doi:10.1038/s41598-019-44855-3
Salahuddin, B., Wang, S., Sangian, D., Aziz, S., and Gu, Q. (2021). Hybrid gelatin hydrogels in nanomedicine applications. ACS Appl. Bio Mat. 4, 2886–2906. doi:10.1021/acsabm.0c01630
Sasaki, H., Rothrauff, B. B., Alexander, P. G., Lin, H., Gottardi, R., Fu, F. H., et al. (2018). In vitro repair of meniscal radial tear with hydrogels seeded with adipose stem cells and TGF-β3. Am. J. Sports Med. 46, 2402–2413. doi:10.1177/0363546518782973
Saygili, E., Kaya, E., Ilhan-Ayisigi, E., Saglam-Metiner, P., Alarcin, E., Kazan, A., et al. (2021). An alginate-poly(acrylamide) hydrogel with TGF-β3 loaded nanoparticles for cartilage repair: Biodegradability, biocompatibility and protein adsorption. Int. J. Biol. Macromol. 172, 381–393. doi:10.1016/j.ijbiomac.2021.01.069
Scotti, C., Pozzi, A., Mangiavini, L., Vitari, F., Boschetti, F., Domeneghini, C., et al. (2009). Healing of meniscal tissue by cellular fibrin glue: An in vivo study. Knee Surg. Sports Traumatol. Arthrosc. 17, 645–651. doi:10.1007/s00167-009-0745-9
Shafei, S., Khanmohammadi, M., Heidari, R., Ghanbari, H., Taghdiri Nooshabadi, V., Farzamfar, S., et al. (2020). Exosome loaded alginate hydrogel promotes tissue regeneration in full-thickness skin wounds: An in vivo study. J. Biomed. Mat. Res. A 108, 545–556. doi:10.1002/jbm.a.36835
Shao, Z., Yin, T., Jiang, J., He, Y., Xiang, T., and Zhou, S. (2022). Wound microenvironment self-adaptive hydrogel with efficient angiogenesis for promoting diabetic wound healing. Bioact. Mat. 20, 561–573. doi:10.1016/j.bioactmat.2022.06.018
Shen, X., Li, S., Zhao, X., Han, J., Chen, J., Rao, Z., et al. (2022). Dual-crosslinked regenerative hydrogel for sutureless long-term repair of corneal defect. Bioact. Mat. 20, 434–448. doi:10.1016/j.bioactmat.2022.06.006
Shi, W., Fang, F., Kong, Y., Greer, S. E., Kuss, M., Liu, B., et al. (2021). Dynamic hyaluronic acid hydrogel with covalent linked gelatin as an anti-oxidative bioink for cartilage tissue engineering. Biofabrication 14, 014107. doi:10.1088/1758-5090/ac42de
Shimomura, K., Rothrauff, B. B., and Tuan, R. S. (2017). Region-specific effect of the decellularized meniscus extracellular matrix on mesenchymal stem cell-based meniscus tissue engineering. Am. J. Sports Med. 45, 604–611. doi:10.1177/0363546516674184
Simson, J. A., Strehin, I. A., Allen, B. W., and Elisseeff, J. H. (2013). Bonding and fusion of meniscus fibrocartilage using a novel chondroitin sulfate bone marrow tissue adhesive. Tissue Eng. Part A 19, 1843–1851. doi:10.1089/ten.TEA.2012.0578
Sonker, M., Bajpai, S., Khan, M. A., Yu, X., Tiwary, S. K., and Shreyash, N. (2021). Review of recent advances and their improvement in the effectiveness of hydrogel-based targeted drug delivery: A hope for treating cancer. ACS Appl. Bio Mat. 4, 8080–8109. doi:10.1021/acsabm.1c00857
Stapleton, T. W., Ingram, J., Fisher, J., and Ingham, E. (2011). Investigation of the regenerative capacity of an acellular porcine medial meniscus for tissue engineering applications. Tissue Eng. Part A 17, 231–242. doi:10.1089/ten.tea.2009.0807
Stapleton, T. W., Ingram, J., Katta, J., Knight, R., Korossis, S., Fisher, J., et al. (2008). Development and characterization of an acellular porcine medial meniscus for use in tissue engineering. Tissue Eng. Part A 14, 505–518. doi:10.1089/tea.2007.0233
Sthijns, M. M. J. P. E., van Blitterswijk, C. A., and LaPointe, V. L. S. (2021). Synthetic materials that affect the extracellular matrix via cellular metabolism and responses to a metabolic state. Front. Bioeng. Biotechnol. 9, 742132. doi:10.3389/fbioe.2021.742132
Sun, Y., Zhang, Y., Wu, Q., Gao, F., Wei, Y., Ma, Y., et al. (2021). 3D-bioprinting ready-to-implant anisotropic menisci recapitulate healthy meniscus phenotype and prevent secondary joint degeneration. Theranostics 11, 5160–5173. doi:10.7150/thno.54864
Tan, G. K., and Cooper-White, J. J. (2011). Interactions of meniscal cells with extracellular matrix molecules: Towards the generation of tissue engineered menisci. Cell adh. Migr. 5, 220–226. doi:10.4161/cam.5.3.14463
Tan, Y., Zhang, Y., and Pei, M. (2010). Meniscus reconstruction through coculturing meniscus cells with synovium-derived stem cells on small intestine submucosa-a pilot study to engineer meniscus tissue constructs. Tissue Eng. Part A 16, 67–79. doi:10.1089/ten.tea.2008.0680
Tanaka, T., Matsushita, T., Nishida, K., Takayama, K., Nagai, K., Araki, D., et al. (2019). Attenuation of osteoarthritis progression in mice following intra-articular administration of simvastatin-conjugated gelatin hydrogel. J. Tissue Eng. Regen. Med. 13, 423–432. doi:10.1002/term.2804
Tsou, Y. H., Khoneisser, J., Huang, P. C., and Xu, X. (2016). Hydrogel as a bioactive material to regulate stem cell fate. Bioact. Mat. 1, 39–55. doi:10.1016/j.bioactmat.2016.05.001
Tsubosaka, M., Kihara, S., Hayashi, S., Nagata, J., Kuwahara, T., Fujita, M., et al. (2020). Gelatin hydrogels with eicosapentaenoic acid can prevent osteoarthritis progression in vivo in a mouse model. J. Orthop. Res. 38, 2157–2169. doi:10.1002/jor.24688
Ulku, T. K., Kaya, A., and Kocaoglu, B. (2020). Suture configuration techniques have no effect on mid-term clinical outcomes of arthroscopic meniscus root repairs. Knee 27, 676–682. doi:10.1016/j.knee.2020.04.017
Vernerey, F. J., Lalitha Sridhar, S., Muralidharan, A., and Bryant, S. J. (2021). Mechanics of 3D cell-hydrogel interactions: Experiments, models, and mechanisms. Chem. Rev. 121, 11085–11148. doi:10.1021/acs.chemrev.1c00046
Visser, J., Levett, P. A., te Moller, N. C., Besems, J., Boere, K. W., van Rijen, M. H., et al. (2015). Crosslinkable hydrogels derived from cartilage, meniscus, and tendon tissue. Tissue Eng. Part A 21, 1195–1206. doi:10.1089/ten.tea.2014.0362
Wang, C., Brisson, B. K., Terajima, M., Li, Q., Hoxha, K., Han, B., et al. (2020a). Type III collagen is a key regulator of the collagen fibrillar structure and biomechanics of articular cartilage and meniscus. Matrix Biol. 85-86, 47–67. doi:10.1016/j.matbio.2019.10.001
Wang, L., Wang, J., Zhou, X., Sun, J., Zhu, B., Duan, C., et al. (2020b). A new self-healing hydrogel containing hucMSC-derived exosomes promotes bone regeneration. Front. Bioeng. Biotechnol. 8, 564731. doi:10.3389/fbioe.2020.564731
Wang, Q. S., Xu, B. X., Fan, K. J., Fan, Y. S., Teng, H., and Wang, T. Y. (2021). Dexamethasone-loaded thermo-sensitive hydrogel attenuates osteoarthritis by protecting cartilage and providing effective pain relief. Ann. Transl. Med. 9, 1120. doi:10.21037/atm-21-684
Waters, R., Alam, P., Pacelli, S., Chakravarti, A. R., Ahmed, R. P. H., and Paul, A. (2018). Stem cell-inspired secretome-rich injectable hydrogel to repair injured cardiac tissue. Acta Biomater. 69, 95–106. doi:10.1016/j.actbio.2017.12.025
Welch, J. A., Montgomery, R. D., Lenz, S. D., Plouhar, P., and Shelton, W. R. (2002). Evaluation of small-intestinal submucosa implants for repair of meniscal defects in dogs. Am. J. Vet. Res. 63, 427–431. doi:10.2460/ajvr.2002.63.427
Wu, J., Ding, Q., Dutta, A., Wang, Y., Huang, Y. H., Weng, H., et al. (2015). An injectable extracellular matrix derived hydrogel for meniscus repair and regeneration. Acta Biomater. 16, 49–59. doi:10.1016/j.actbio.2015.01.027
Wu, J., Xu, J., Huang, Y., Tang, L., and Hong, Y. (2021). Regional-specific meniscal extracellular matrix hydrogels and their effects on cell-matrix interactions of fibrochondrocytes. Biomed. Mat. 17, 014105. doi:10.1088/1748-605X/ac4178
Wu, Y., Hong, J., Jiang, G., Li, S., Chen, S., Chen, W., et al. (2019). Platelet-rich gel-incorporated silk scaffold promotes meniscus regeneration in a rabbit total meniscectomy model. Regen. Med. 14, 753–768. doi:10.2217/rme-2018-0087
Wu, Y., Li, X., Sun, Y., Tan, X., Wang, C., Wang, Z., et al. (2022). Multiscale design of stiffening and ROS scavenging hydrogels for the augmentation of mandibular bone regeneration. Bioact. Mat. 20, 111–125. doi:10.1016/j.bioactmat.2022.05.021
Xiao, Y., Gu, Y., Qin, L., Chen, L., Chen, X., Cui, W., et al. (2021). Injectable thermosensitive hydrogel-based drug delivery system for local cancer therapy. Colloids Surfaces B Biointerfaces 200, 111581. doi:10.1016/j.colsurfb.2021.111581
Yamasaki, T., Deie, M., Shinomiya, R., Izuta, Y., Yasunaga, Y., Yanada, S., et al. (2005). Meniscal regeneration using tissue engineering with a scaffold derived from a rat meniscus and mesenchymal stromal cells derived from rat bone marrow. J. Biomed. Mat. Res. A 75, 23–30. doi:10.1002/jbm.a.30369
Yamasaki, T., Deie, M., Shinomiya, R., Yasunaga, Y., Yanada, S., and Ochi, M. (2008). Transplantation of meniscus regenerated by tissue engineering with a scaffold derived from a rat meniscus and mesenchymal stromal cells derived from rat bone marrow. Artif. Organs 32, 519–524. doi:10.1111/j.1525-1594.2008.00580.x
Yan, R., Chen, Y., Gu, Y., Tang, C., Huang, J., Hu, Y., et al. (2019). A collagen-coated sponge silk scaffold for functional meniscus regeneration. J. Tissue Eng. Regen. Med. 13, 156–173. doi:10.1002/term.2777
Yan, W., Xu, X., Xu, Q., Sun, Z., Jiang, Q., and Shi, D. (2022). Platelet-rich plasma combined with injectable hyaluronic acid hydrogel for porcine cartilage regeneration: A 6-month follow-up. Regen. Biomater. 7, 77–90. doi:10.1093/rb/rbz039
Yang, C. P., Hung, K. T., Weng, C. J., Chen, A. C., Hsu, K. Y., and Chan, Y. S. (2021). Clinical outcomes of meniscus repair with or without multiple intra-articular injections of platelet rich plasma after surgery. J. Clin. Med. 10, 2546. doi:10.3390/jcm10122546
Yazdani, M., Shahdadfar, A., Jackson, C. J., and Utheim, T. P. (2019). Hyaluronan-based hydrogel scaffolds for limbal stem cell transplantation: A review. Cells 8, 245. doi:10.3390/cells8030245
Yuan, L., Pan, M., Lei, M., Zhou, X., Hu, D., Liu, Q., et al. (2020). A novel composite of micelles and hydrogel for improving skin delivery of hydrocortisone and application in atopic dermatitis therapy. Appl. Mat. Today 19, 100593. doi:10.1016/j.apmt.2020.100593
Yuan, T., Li, Z., Zhang, Y., Shen, K., Zhang, X., Xie, R., et al. (2021). Injectable ultrasonication-induced silk fibroin hydrogel for cartilage repair and regeneration. Tissue Eng. Part A 27, 1213–1224. doi:10.1089/ten.tea.2020.0323
Yuan, X., Wei, Y., Villasante, A., Ng, J. J. D., Arkonac, D. E., Chao, P. G., et al. (2017). Stem cell delivery in tissue-specific hydrogel enabled meniscal repair in an orthotopic rat model. Biomaterials 132, 59–71. doi:10.1016/j.biomaterials.2017.04.004
Zarrintaj, P., Khodadadi Yazdi, M., Youssefi Azarfam, M., Zare, M., Ramsey, J. D., Seidi, F., et al. (2021). Injectable cell-laden hydrogels for tissue engineering: Recent advances and future opportunities. Tissue Eng. Part A 27, 821–843. doi:10.1089/ten.tea.2020.0341
Zhang, F. X., Liu, P., Ding, W., Meng, Q. B., Su, D. H., Zhang, Q. C., et al. (2021). Injectable Mussel-Inspired highly adhesive hydrogel with exosomes for endogenous cell recruitment and cartilage defect regeneration. Biomaterials 278, 121169. doi:10.1016/j.biomaterials.2021.121169
Zhang, K., Wu, J., Zhang, W., Yan, S., Ding, J., Chen, X., et al. (2018a). In situ formation of hydrophobic clusters to enhance mechanical performance of biodegradable poly(l-glutamic acid)/poly(ε-caprolactone) hydrogel towards meniscus tissue engineering. J. Mat. Chem. B 6, 7822–7833. doi:10.1039/c8tb01453a
Zhang, L., Li, T., Yu, Y., Shi, K., Bei, Z., Qian, Y., et al. (2022a). An injectable conductive hydrogel restores electrical transmission at myocardial infarct site to preserve cardiac function and enhance repair. Bioact. Mat. 20, 339–354. doi:10.1016/j.bioactmat.2022.06.001
Zhang, M., Hu, W., Cai, C., Wu, Y., Li, J., and Dong, S. (2022b). Advanced application of stimuli-responsive drug delivery system for inflammatory arthritis treatment. Mat. Today Bio 14, 100223. doi:10.1016/j.mtbio.2022.100223
Zhang, Q., Liu, Y., Li, J., Wang, J., and Liu, C. (2022c). Recapitulation of growth factor-enriched microenvironment via BMP receptor activating hydrogel. Bioact. Mat. 20, 638–650. doi:10.1016/j.bioactmat.2022.06.012
Zhang, S., Matsushita, T., Kuroda, R., Nishida, K., Matsuzaki, T., Matsumoto, T., et al. (2016). Local administration of simvastatin stimulates healing of an avascular meniscus in a rabbit model of a meniscal defect. Am. J. Sports Med. 44, 1735–1743. doi:10.1177/0363546516638342
Zhang, Z., Guo, W., Gao, S., Chen, M., Li, X., Zhang, X., et al. (2018b). Native tissue-based strategies for meniscus repair and regeneration. Cell Tissue Res. 373, 337–350. doi:10.1007/s00441-017-2778-6
Zhao, P., Feng, Y., Zhou, Y., Tan, C., and Liu, M. (2022a). Gold@Halloysite nanotubes-chitin composite hydrogel with antibacterial and hemostatic activity for wound healing. Bioact. Mat. 20, 355–367. doi:10.1016/j.bioactmat.2022.05.035
Zhao, Y. P., Han, J. F., Zhang, F. Y., Liao, T. T., Na, R., Yuan, X. F., et al. (2022b). Flexible nano-liposomes-based transdermal hydrogel for targeted delivery of dexamethasone for rheumatoid arthritis therapy. Drug Deliv. (Lond). 29, 2269–2282. doi:10.1080/10717544.2022.2096718
Zhong, G., Yao, J., Huang, X., Luo, Y., Wang, M., Han, J., et al. (2020). Injectable ECM hydrogel for delivery of BMSCs enabled full-thickness meniscus repair in an orthotopic rat model. Bioact. Mat. 5, 871–879. doi:10.1016/j.bioactmat.2020.06.008
Zhou, Y. F., Zhang, D., Yan, W. T., Lian, K., and Zhang, Z. Z. (2022). Meniscus regeneration with multipotent stromal cell therapies. Front. Bioeng. Biotechnol. 10, 796408. doi:10.3389/fbioe.2022.796408
Keywords: hydrogel, meniscus, function, extracellular matrix, cell, tissue engineering
Citation: Jin P, Liu L, Chen X, Cheng L, Zhang W and Zhong G (2022) Applications and prospects of different functional hydrogels in meniscus repair. Front. Bioeng. Biotechnol. 10:1082499. doi: 10.3389/fbioe.2022.1082499
Received: 28 October 2022; Accepted: 28 November 2022;
Published: 08 December 2022.
Edited by:
Andreia Cerqueira, University of Jaume I, SpainReviewed by:
Fengyuan Zhao, Peking University Third Hospital, ChinaGirish Pattappa, University Medical Center Regensburg, Germany
Copyright © 2022 Jin, Liu, Chen, Cheng, Zhang and Zhong. This is an open-access article distributed under the terms of the Creative Commons Attribution License (CC BY). The use, distribution or reproduction in other forums is permitted, provided the original author(s) and the copyright owner(s) are credited and that the original publication in this journal is cited, in accordance with accepted academic practice. No use, distribution or reproduction is permitted which does not comply with these terms.
*Correspondence: Pan Jin, amlucGFubW91bnRhaW5AMTYzLmNvbQ==; Gang Zhong, MTMyNTc3ODg2NjdAMTYzLmNvbQ==
†These authors have contributed equally to this work