- 1Department of Plastic Surgery, The Second Affiliated Hospital, School of Medicine, Zhejiang University, Hangzhou, China
- 2Wenzhou Medical University, Wenzhou, China
- 3Starbody plastic surgery Clinic, Hangzhou, China
- 4Department of Breast Surgery, The First Affiliated Hospital of Wenzhou Medical University, Wenzhou, China
Aging is associated with multiple degenerative diseases, including atherosclerosis, osteoporosis, and Alzheimer’s disease. As the most intuitive manifestation of aging, skin aging has received the most significant attention. Skin aging results from various intrinsic and extrinsic factors. Aged skin is characterized by wrinkles, laxity, elastosis, telangiectasia, and aberrant pigmentation. The underlying mechanism is complex and may involve cellular senescence, DNA damage, oxidative stress (OS), inflammation, and genetic mutations, among other factors. Among them, OS plays an important role in skin aging, and multiple antioxidants (e.g., vitamin C, glutathione, and melatonin) are considered to promote skin rejuvenation. In addition, stem cells that exhibit self-replication, multi-directional differentiation, and a strong paracrine function can exert anti-aging effects by inhibiting OS. With the further development of stem cell technology, treatments related to OS mitigation and involving stem cell use may have a promising future in anti-skin aging therapy.
1 Introduction
Aging is currently defined as a progressive disorder of tissue and organ functions over time, which eventually leads to numerous chronic pathologies (Calcinotto et al., 2019). Skin aging can be categorized into intrinsic aging and extrinsic aging (primarily photoaging) (Wu et al., 2019). Intrinsic aging occurs unavoidably as a result of chronological aging, whereas extrinsic aging occurs upon exposure to environmental factors, such as pollutants and ultraviolet light (Kohl et al., 2011). Both intrinsic and extrinsic aging damage the skin structure and cause dysfunction, which leads to wrinkling, hair loss, reduction of elasticity, impairment of the epidermal barrier maintenance, and multiple physical and psychological issues. In addition, aged skin is more susceptible to injury and infection, poor wound healing, and primary skin cancer (Giangreco et al., 2008). Oxidative stress (OS), a phenomenon characterized by an imbalance between reactive oxygen species (ROS) and antioxidants, has long been considered one of the major driving forces of accelerated skin aging and diseases (Velarde et al., 2012). Engendered by various sources (such as mitochondrial respiration, UV light exposure, and environmental pollution), the excessive accumulation of ROS can induce inflammation, cellular senescence, and aging in the skin (Sies et al., 2017). Skin rejuvenation, as an attempt to reverse the visible signs of aging, involves protection from OS (Yang et al., 2020).
Overall, the skin contains approximately 20 different types of cells (e.g., keratinocytes, melanocytes, Langerhans cells, fibroblasts, and others) which are constantly replenished by various stem cells (Blanpain and Fuchs, 2006). Skin stem cells can be further categorized as epidermal stem cells, hair follicle stem cells, dermal stem cells, and sebaceous gland stem cells (Zouboulis et al., 2008). Despite the tiny proportion they constitute (1%–10% in the basal layer of the epidermis and 0.3% of dermal foreskin fibroblasts), stem cells are highly valued for their self-renewal potential (Niemann and Watt, 2002). Findings from studies have shown the depletion of stem cells during aging (Youn et al., 2004; Aleemardani et al., 2021).
Well known for their beneficial effects on wound healing and skin rejuvenation, skin stem cell-based therapy is being investigated extensively (Dahl, 2012). For example, adipose-derived stem cells (ADSCs) are frequently used in regenerative medicine owing to their extensive paracrine activity, role in angiogenesis and immune modulation, and anti-oxidative potential (Kusuma et al., 2017). In this paper, we review the relationship between OS exposure-related skin aging and the role of stem cells, with an aim to provide novel strategies.
2 Mechanisms underlying skin aging
2.1 Skin aging
As the largest and one of the most complex organs, the skin primarily comprises three parts: epidermis, dermis, and subcutaneous tissue (Campbell et al., 2004). The epidermal layer is further divided into cornified, granular, spinous, and basal layers and contains keratinocytes (accounting for approximately 95% of all cells), melanocytes, and Langerhans cells (Eckhart and Zeeuwen, 2018). Connected by the dermal-epidermal junction, the dermis is mostly composed of fibroblasts and their secretory protein, the extracellular matrix (ECM). According to a single-cell analysis led by Zou et al., the inactivation of HES1 in fibroblasts and KLF6 in keratinocytes can cause cellular senescence (Zou et al., 2021). The reduction of physical interaction between fibroblasts and keratinocytes is suggested to be associated with the impairment of epidermal stem cell maintenance in aged skin (Gruber et al., 2020). Adipocytes are localized to the subcutaneous tissue (Author Anonyms, 2017). Both intrinsic and extrinsic aging leads to changes in all three layers (especially the former two).
Intrinsic aging is a normal physiological process regulated by various genetic factors. It is characterized by thinning, dryness, laxity, fine wrinkles, decreased elasticity, increased brittleness, and susceptibility to several skin disorders such as fibroma mole and seborrheic keratosis (Lavker et al., 1986; Gilchrest, 1989; Gu et al., 2020). Histologically, keratinocytes proliferate at a slow pace and become less active, and the number of epidermal stem cells reduces (Gu et al., 2020). Meanwhile, cytoheterogeneity is more frequent in the basal region with lost polarity (Brégégère et al., 2003). The interface between the epidermis and dermis flattens, making the skin less tolerant to shearing forces (Lavker et al., 1987). Underneath, the decreased activity of fibroblasts is observed with the reduced production of collagen and elastin.
Long-term exposure to noxious pollutants (such as ozone, particulate matter, and cigarette smoke), ultraviolet radiation (UVR), and an unhealthy lifestyle accelerate skin aging (Burke, 2018; Pecorelli et al., 2019). Among them, UVR is the most common cause of photoaging and the primary reason for extrinsic aging (Cavinato and Jansen-Dürr, 2017). Unlike intrinsic aging, photoaging is characterized by pachulosis, thickness, laxity, deep and thick wrinkle formation, hyperpigmentation, telangiectasis, and a higher risk of malignancy (Gu et al., 2020). Histologically, one of the most prominent characteristics is the accumulation of amorphous elastic fibers and disordered collagen formation (Varani et al., 2004). Other typical changes include the flattening of endothelial cells, dilation of remaining skin vessels, and the redistribution of skin adipose (Hughes et al., 2004).
2.2 Involvement of OS in skin aging
The mechanisms underlying skin aging involve cellular, molecular, and genetic changes. Among them, OS is usually considered as the core influencing factor, exerting a key role in both intrinsic aging and photoaging (during which the effect is strengthened) through multiple signaling pathways and subsequent structural remodeling (Figure 1) (Bocheva et al., 2019; Prasanth et al., 2019).
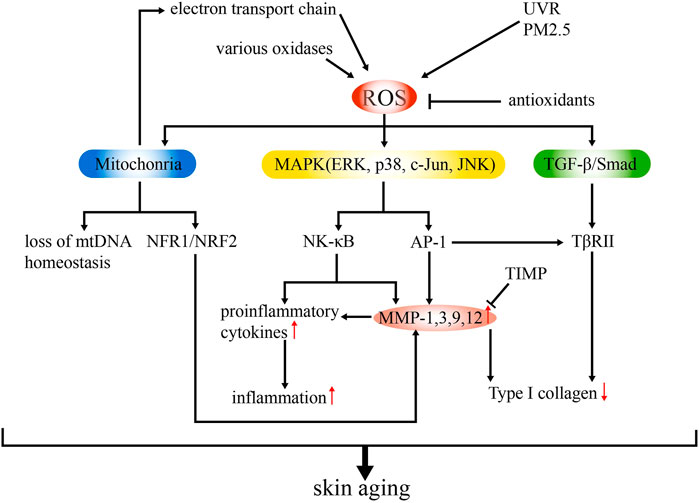
FIGURE 1. Schematic model of the mechanisms of ROS-related skin aging. ROS are generated from various intrinsic (e g., electron transport chain, various oxidases) and extrinsic sources (e.g., UVR, PM2.5). Overproduction of ROS can lead to upregulated MMP expression and inflammation and suppressed collagen synthesis via mitochondrial dysfunction and activation of MAPK and TGF-β signaling pathways. Antioxidants and TIMP can ameliorate ROS production, thus delaying skin aging.
OS results from redox imbalances, which involve ROS accumulation and antioxidant effect suppression (Birch-Machin and Bowman, 2016). Superoxide anion, hydrogen peroxide, the highly active hydroxyl radical, lipid peroxides, and nitrogen oxides are common ROS present in the body (Mailloux, 2015). They are derived from both intrinsic (e.g., electron transport chain, reactions by oxidases) and extrinsic (e.g., UVR, PM2.5) sources. Excessive ROS can directly damage the cellular structure and function, mediate inflammatory responses, and impair genetic components, leading to skin aging (Kammeyer and Luiten, 2015). As a key regulatory target in ROS-induced skin aging, matrix metalloproteinases (MMPs) mediate the degradation of the different components of the ECM (collagen in particular) (Quan et al., 2009). The synthesis of MMPs is stimulated by ROS through the mitogen-activated protein kinase (MAPK) signaling pathway, in which extracellular signal-regulated kinase (ERK), p38, and c-Jun N-terminal kinase (JNK) are common members. The transcription factor, activator protein 1 (AP-1), is then induced and regulates the transcription of MMP-1, MMP-3, MMP-9, and MMP-12 (Shaulian and Karin, 2002). Nuclear factor-κB (NF-κB), another transcription factor, is also activated and mediates the responses to UVR and photoaging by regulating inflammation and MMP expression (Wang et al., 2019a). Thus, MMPs can be modulated by both AP-1 and NF-κB, which makes it a major effector. Another signaling pathway in which MMP is involved in skin aging is the transforming growth factor beta (TGF-β)/Smad pathway, which is impaired upon the downregulation of TβRII expression (partially induced by AP-1) and leads to the reduced production of type I collagen (Quan et al., 2004; Kim et al., 2012).
In the skin, MMPs are primarily secreted by dermal fibroblasts, whereas epidermal keratinocytes are considered the major source of cytokines (Ansel et al., 1990). The expression of MMPs increases in aged skin owing to OS and relative signaling pathways, and tissue inhibitors of metalloproteinases (TIMPs) decrease the level of MMPs (Nagase et al., 2006). Several factors have been reported to fuel ROS-induced aging through upregulated MMPs (Lee et al., 2021a). For example, MMP-9 expression elevation is linked to increased tumor necrosis factor alpha (TNF-α) expression via NF-kB, AP-1, hypoxia-inducible factor 1 alpha (HIF-1α), and nuclear factor erythroid 2-related factor 2 (Nrf2) (Holvoet et al., 2003). The accumulation of cysteine-rich protein 61 (CCN1), another cytokine, stimulates the production of MMP1 while downregulating TGF-β type-II receptor, thereby hindering ECM homeostasis during aging (Quan et al., 2011). MMP1 expression can also be enhanced by environmental stressors (e.g., tobacco smoke) via the activation of the aryl hydrocarbon receptor (AhR) signaling pathway (Ono et al., 2013).
The over-production of ROS accelerates skin aging. The mitochondria are considered the major source of cellular OS. Endogenous ROS is constantly produced as the byproduct of oxidative phosphorylation in the mitochondrion (Mailloux, 2015). Meanwhile, chronic exposure to UVR also generates ROS, besides increasing nicotinamide adenine dinucleotide phosphate (NADPH) production (Fuller, 2019). During skin aging, ROS also causes damage by inducing mitochondrial dysfunction. Singh et al. revealed that the loss of mtDNA homeostasis is responsible for causing skin wrinkles (Singh et al., 2018). This finding was supported by Umbayev et al. The authors showed a reduction in fibroblast mitochondrial abundance and mtDNA copy number as well as enhanced mtDNA damage in aged skin (Wei et al., 2016; Umbayev et al., 2020). Besides mtDNA, other evidence indicates associations between the mitochondrial redox imbalance and Nrf2 expression. Jinapath et al. confirmed the preventive role of mitochondria-targeted H2S delivery in the mouse skin in vivo through Nrf2 activation (Lohakul et al., 2022). The activation of NRF1/NRF2 transcription was also observed in α-l-hexaguluroic acid hexasodium salt-treated HaCaT cells with improved mitochondrial energy metabolism, and subsequently, the increased expression of MMP and silent information regulator 1 (SIRT1) was also observed (Li et al., 2020a). Similar results were noted in HaCaT cells treated with d-tetramannuronic acid tetrasodium salt (Li et al., 2020b). Moreover, the mitochondrial redox imbalance induces JunB proto-oncogene (JunB) expression, causing fibroblast senescence through p16 upregulation and type 1 insulin-like growth factor (IGF-1) downregulation and eventually leading to skin atrophy via the disruption of stem cell niches (Maity et al., 2021).
2.3 Antioxidants in skin aging
In the past 5 years, several hundred preclinical and clinical studies have shown that the application of antioxidants inhibits skin aging. Herein, we discuss findings from some of the related studies about antioxidants used in treating skin aging to identify potential treatments (Table 1). The anti-aging effect of plant extracts and dietary supplementation has been widely investigated. Additionally, antioxidants extracted from humans and other animals were also shown to be ROS-antagonistic.
First, antioxidants alleviate skin aging by attenuating OS activity and protecting mitochondrial function. For example, citrus sinensis peel extract (CSPE) nanoformulation (Amer et al., 2021), extracts and bioactive compounds derived from seaweed (Pangestuti et al., 2021), and atractyligenin (Xuan et al., 2019) were reported to suppress ROS by activating the MAPK pathway. As such, CSPE nanoformulations (Amer et al., 2021), BlendE, BlendIP (Namkoong et al., 2018), and tannic acid (Daré et al., 2020) are associated with either an increase in antioxidative enzyme expression or a decrease in ROS generation. Second, the regulation of inflammatory responses is beneficial to anti-aging therapy, such as that with a mixture of marigold and rosemary extracts, CSPE nanoformulation (Amer et al., 2021), bamboo leaf flavonoids (BLF) (Gu et al., 2022), and ursolic acid (Samivel et al., 2020). The level of cytokines is reduced, and other signaling pathways, such as TGF-β and hedgehog signaling pathways, are involved. Third, antioxidant systems exert protective effects on DNA (hydroxytyrosol from olive fruit (Avola et al., 2019)), inhibit apoptosis (ursolic acid (Samivel et al., 2020), resveratrol (Cui et al., 2022)), and promote autophagy (caffeine (Li et al., 2018)).
3 Anti-aging effect of stem cells
Stem cells are generally considered powerful candidates in regenerative medicine for combatting skin aging. The disrupted homeostasis and subsequent regulation of ROS activity are considered to be associated with skin aging. Several factors affecting the number, status, and differentiation of stem cells participate in skin aging. Laminins are considered potential anti-aging targets because of their regulatory effects in stem cells. For instance, laminin-332 was confirmed to be responsible for the proper differentiation of interfollicular epidermal stem cells, and the loss of laminin-511 expression results in the reduction of epidermal stem/progenitor cell generation (Yamada et al., 2018; Iriyama et al., 2020). Collagen XVII (COL17A1) showed differential expression in epidermal stem cells in response to genomic or oxidative stress, driving stem cell competition through symmetric cell division. Clones expressing low levels of COL17A1 were eliminated, which promoted skin aging via the depletion of adjacent melanocytes and fibroblasts. Meanwhile, the forced maintenance of COL17A1 showed anti-aging potential (Liu et al., 2019). Besides, the crucial role of nuclear receptor interacting protein 1 (Nrip1) in aging was proposed by Hu et al. Skin aging was indicated to be delayed with the reduced expression of senescence-associated (p21 and p53), inflammation-associated (p65, IL6, and IL-1α), and growth factor-associated (mTOR, Igf1) genes under Nrip1 knockout in ADMSCs (Hu et al., 2021). The disruption of circadian clock activity through BMAL1 depletion is linked to the increased differentiation of interfollicular epidermal stem cells (IFESCs) in arrhythmic, prematurely aging mice (Welz et al., 2019).
The anti-aging effect of stem cells (including its derivatives) is closely related to their anti-ROS potential, with MAPK and TGF-β signaling pathways (which regulate MMP expression and ECM synthesis) as potential junctions. For example, ADSC-CM was found to prevent photoaging and down-regulate ROS activity through the modulation of the MAPKs/AP-1/NF-κB signaling pathway, with the suppressed expression of MMP-1 and IL-6 and elevated level of antioxidant phase II gene heme oxygenase-1 (HO-1) (Li et al., 2019). Meanwhile, TGF-β and Smad expression was recovered after perturbation by UVB (Li et al., 2019). Additionally, the activation of the NF-κB pathway was also observed in another study conducted by Hwang et al. MMP expression and ROS production were ameliorated in the treatment group (with neural stem cell-conditioned medium (NSC-CM) and its secreted factors TIMP-1 and TIMP-2) (Hwang et al., 2019). Moreover, the protective effect of NSC-CM was demonstrated by the activation of the DNA repair enzyme Rad50 and subsequent inhibition of γ-H2AX, a DNA damage marker (Hwang et al., 2019). These results indicate the anti-aging effect of anti-ROS signaling pathways and their promising application of stem cell-conditioned medium.
Besides, other processes, such as the regulation of superoxide dismutase (SOD) expression and fibroblast senescence phenotype by various types of stem cells and their derivatives, are involved. A SOD-dependent mechanism and reversed aging phenotype was suggested by Adam et al., who observed the inhibition of advanced glycation end product and malondialdehyde (MDA, a major product generated by oxygen free radicals) generation and increased SOD expression in their animal model (Zhang et al., 2014; Surowiecka and Strużyna, 2022). Similarly, the injection of ADSC-derived exosomes (ADSC-EVs) observably decreased skin wrinkling, promoted type I collagen synthesis, and suppressed MMP-3 expression (Xu et al., 2020). Evidence from in vivo and in vitro experiments revealed that ROS production was counteracted with the increased expression of the antioxidant enzymes SOD-1 and CAT (Xu et al., 2020). In another experiment led by Deng et al., hucMSC-derived extracellular vesicles (hucMSC-EVs) suppressed photoaging by inhibiting ROS generation, promoting fibroblast proliferation, and preventing cell cycle arrest, presented with the upregulation of glutathione peroxidase one and Col-1 and downregulation of MMP-1 (Deng et al., 2020). In contrast, the combined use of ADSCs and fractional CO2 laser was shown to ameliorate skin aging with a decrease in the MDA content through a SOD-independent pathway (Xu et al., 2014). Xu et al. also observed the improvement of cell cycle arrest and increased expression of Wnt3a and β-catenin, which is positively correlated with TGF-β2 and COLI expression (Xu et al., 2014).
Moreover, an adipose tissue extracellular fraction isolated by Barbara et al. improved skin aging by reducing OS-induced damage and preventing fibroblast senescence (Bellei et al., 2018). In this process, a slight induction of SESN1 (a p53-responsive protein encoding antioxidant modulators of peroxiredoxins) was observed, indicating the attenuation of ROS (Kopnin et al., 2007; Bellei et al., 2018). Real-time RT-PCR and ELISA analyses conducted by Choi et al. revealed the significant suppression of MMP-1, -2, -3, and -9 as well as the enhanced level of collagen and elastin production under the treatment of human adipose-derived stem cell extracellular vesicles (Choi et al., 2019). This was supported by the PCR results reported by Liang et al., which confirmed the increased mRNA expression of type I collagen and decreased expression of type III collagen, MMP-1, and MMP-3 under ADSC-derived exosome treatment (Liang et al., 2020). Simultaneously, TIMP-1 and TGF-β1 were upregulated, which aided the recovery of photo-damaged dermal fibroblasts (Choi et al., 2019).
4 Stem cell-based anti-aging treatments
4.1 Summary of findings from current clinical trials
Stem cells have shown great potential in skin rejuvenation. Associated treatments include stem cell transplantation and the use of derivatives such as conditioned medium and extracellular vesicles. As a common type of stem cell, mesenchymal stem cells (MSCs) are an essential source for skin rejuvenation and can be categorized into several subpopulations, such as amniotic membrane stem cells (AMSCs), ADSCs, human umbilical cord MSCs (hucMSCs), bone marrow stem cells, and human induced pluripotent stem cells (iPSCs) (Charles-de-Sá et al., 2020). Several clinical trials have been conducted or are underway to address the safety, feasibility, and efficacy of stem cell-based therapeutics to improve skin aging in humans (Table 2). Although some study reports have not yet been published, various clinical trials have evidenced the safety and efficacy of MSCs in this regard.
AMSCs are easily obtainable and exhibit low immunogenicity. Also, they secrete several growth factors and cytokines, which can improve collagen synthesis and the proliferation and migration of fibroblasts and keratinocytes (Harrell et al., 2019). Recently, a study on 48 women aged between 41 and 60 years revealed that AMSC-conditioned medium (AMSC-CM) yields significantly lower scores in pore, wrinkle, spot polarized, and spot UV compared to the control group (Prakoeswa et al., 2019). Microneedling was used to enhance the penetration of AMSC-CM. Likewise, the administration of AF-MSC-CM combined with skin needling considerably improved the skin texture, increased collagen and elastic fiber production, and supported the management of facial aging (El-Domyati et al., 2020). According to single-cell profiles, compared with bone marrow stem cells, ADSCs are less heterogenous and less dependent on mitochondrial respiration for energy production, indicating better stemness maintenance and resistance against apoptosis (Zhou et al., 2019).
Evaluation of the potential therapeutic merits of protein extracts (such as IL-6, IL-8, and TGF-β) from ADSCs in 30 Chinese female volunteers showed that, compared with the control group, the intervention group exhibited a considerable improvement in skin roughness, a decreased melanin index, increased skin brightness and elasticity, and reduced wrinkle formation (Wang et al., 2018). Also, an analytical clinical trial of 20 healthy participants (16 women and four men) confirmed the safety and efficacy of autologous ADSC injection in the 3-to-4-month follow-up, as shown by the complete regeneration of solar elastosis (Charles-de-Sá et al., 2020). Further, the remodeling of elaunin and oxytalan fibers in the upper papillary dermis, concomitant with the degradation of elastotic abnormal elastin deposits in the deeper dermis, was observed in the harvested skin samples. Interestingly, an increased level of cathepsin K and MMP-12 and expanded M2 macrophage infiltration were also observed in the post-treatment skin tissues, indicating elastinolysis and the potential anti-inflammatory effects (Charles-de-Sá et al., 2020). Additionally, the application of ADSC-CM (which contains multiple anti-inflammatory cytokines, growth factors, and ECM-regulating molecules) in combination with niacinamide resulted in a statistically more drastic improvement of wrinkles and skin pigmentation in patients post-laser therapy (Lee et al., 2021b).
Moreover, the results of the in vitro analysis suggested the upregulation of type I collagen and decreased pro-inflammatory cytokine, MMP-1, and MMP-2 expression in a dose-dependent manner (Lee et al., 2021b). To compare the regenerative effects of platelet-rich plasma (PRP) injection and ADSCs, 13 patients were injected with stromal vascular fraction (SVF)-enriched fat, expanded adipose-derived stem cells, or fat plus PRP (Rigotti et al., 2016). In addition to findings from their previous study, Gino et al. showed the revearsal of aging in collagen and elastin morphology (Charles-de-Sá et al., 2015; Cohen, 2016). However, the addition of PRP led to no significant advantages but increased inflammatory infiltration and vascular reactivity (Rigotti et al., 2016).
Red deer mesenchymal stem cell extract, another source of stem cells, also showed the ability to rejuvenate aging facial skin with acceptable safety and feasibility (Alhaddad et al., 2019). Besides, some forms of skin rejuvenation occur along with an increase in the level of stem cells. For example, O. rapum extract was demonstrated to reactivate skin renewal via the protection of skin stem cells and maintenance of skin microbiota balance (Meunier et al., 2019). While the level of peripheral CD34+ and CD133+ stem cells failed to increase under the treatment of NutraStem® in combination with an exercise stimulus, a relative restorative potential and anti-oxidative effect was implied. In addition, no severe side effects were observed among the treated groups, which indicated the safety and feasibility of stem cell-based therapy. The common limitations of such studies include the small sample size and lack of long-term follow-up.
4.2 Promising applications of nano-materials: Nanofat and stem cell-derived extracellular vesicles
As mentioned above, skin aging is an extremely complex process that is primarily induced by OS and other intrinsic and environmental factors. In this process, stem cell homeostasis exhibits significant anti-ROS potential in delaying the aging process. The primary goal of skin rejuvenation is to help prevent serious diseases related to skin aging (such as skin cancers) as well as to meet the psychological and aesthetic needs of patients.
Classic options include plastic surgeries, infrared therapy, topical medication (i.e., lotions, injections, and fillers), and dietary supplements (Tsai and Hamblin, 2017; Wang et al., 2020c; Geahchan et al., 2022). Although some of them have been confirmed to be effective, many patients undergoing these treatments complain of limited improvement and lengthy therapeutic cycles. Thus, the development of novel technologies is required. Nanosized ingredients offer enormous advantages, such as better permeation across skin layers, to mediate the intended anti-aging effects (Bhatia et al., 2022). Here, we will discuss the development of combined nanotechnology with stem cells over the last decade, with an aim of providing a useful framework of stem cell-related nano-products.
Generally, nanofat and stem cell (especially ADSC)-derived extracellular vesicles (EVs) (primarily exosomes) are the two core components used. With respect to nanofat, single grafting as well as integrated use with other techniques such as microneedling were invented. When the dimensions are 400–600 μm or less, nanofats include micro-fragmented adipose tissue-containing matrix, stromal vascular cells, and free fatty acids. This can be easily obtained through emulsification and injected through a 27-gauge needle or smaller needle, which is considered an in vivo tissue-engineering treatment (Cohen et al., 2017). As adipocytes cannot survive the isolation process, enhanced stem cell activity is probably a major effect.
For example, the enrichment of ADSCs in nanofat samples was observed by Patrick et al. The subsequent 67 cases treated with nanofat grafting reported improved skin quality with reduced rhytides and pigmentation (Tonnard et al., 2013). Similarly, the combined use of microfat and nanofat grafting yielded satisfactory results in lower eyelid dark circles, indicating the stem cell-like activity of nanofat as potential therapy for skin rejuvenation (Oh et al., 2014). The nanofat group exhibited significantly improved facial soft tissue depression and skin texture as well as an overall satisfaction rate above 90% (Wei et al., 2017). Nanofat-derived stem cells, which show enhanced proliferation and adipogenic differentiation induced by platelet-rich fibrin, functioned similarly to MSCs and shared many of the biological characteristics, such as high levels of CD29, CD44, CD49days, CD54, CD90, and CD105 expression and low levels of CD34, CD45, and CD106 expression (Wei et al., 2017). While microneedling alone requires repeated treatment, its incorporation with nanofat yielded a more lasting anti-aging effect (Verpaele et al., 2019).
The second strategy used for stem cell-derived EV treatment refers to delivery and regulation through nanosized vesicles. Common sources include human umbilical cord mesenchymal stem cells, ADSCs, and iPSCs. EVs are lipid-bilayer carriers (such as exosomes, microvesicles, and apoptotic bodies) containing proteins, lipids, RNAs, and DNAs, responsible for intercellular communication (Théry et al., 2018). As a major subtype of EVs, exosomes are nanoparticles with a diameter of 40–150 nm, known for their ability to deliver not only numerous proteins (e.g., enzymes, cytokines, and transcription factors) but also nucleic acids (especially miRNAs) (Cha et al., 2020). Therefore, stem cell-derived exosomes have shown great potential in skin aging treatment by enhancing fibroblast proliferation and bioactivity, reducing ROS and inflammation, upregulating collagen expression, and downregulating MMP expression. For example, the treatment of exosomes derived from hucMSCs (hucMSC-ex) promoted H2O2 detoxification, repressed DNA damage, and inhibited apoptosis, leading to the attenuation of skin redness, scaling, and inflammatory cell infiltration. Additionally, the cytoprotective effects of hucMSC-ex-derived 14-3-3ζ protein might be associated with the modulation of the antioxidant SIRT1-dependent pathway (Wu et al., 2021). Besides, the anti-aging effect of hucMSC-ex can be strengthened through combination with the marine sponge Haliclona sp. Spicules (SHSs) (Zhang et al., 2020), which resulted in the promotion of HDF proliferation, reduction in the proportion of senescent cells, and rebuilding of the dermal ECM (Zhang et al., 2020). Similarities with remodeled ECM are visible in corneal stromal cells treated with ADSC-derived exosomes (Shen et al., 2018). Besides, Oh et al. reported the suppression of SA-β-Gal and MMP-1/3 and restored type I collagen expression in senescent HDFs treated with exosomes derived from human iPSCs (iPSCs-Exo) (Oh et al., 2018).
Recently, a novel bioinspired approach, cell-engineered nanovesicles (CENVs), with diameters less than 150 nm, was proposed for treating issues like the inhibited production of exosomes while maintaining similar characteristics. In addition to the above changes, iPSC-CENV also inhibited the elevation of p53 and p21 expression, indicating the potential modulation of cell cycle arrest, apoptosis, and cellular senescence (Lee et al., 2020).
5 Discussion
As the human life span extends, the need of people for anti-aging treatment increases. As the direct manifestation of aging, skin aging is a major concern for health-related and aesthetic reasons. It is caused by a joint attack by genetic and environmental factors and can lead to consequences such as elevated risks of injuries, infection, impaired wound healing, and cancer. Thus, it is important to identify methods to delay skin aging.
Chronological and extrinsic skin aging exhibit distinct differences in clinical indicators but involve similar regulatory pathways. In the past few years, numerous studies have been conducted in the field of skin rejuvenation, and some have achieved promising results. OS is considered to initiate skin aging. The overproduction of ROS can induce mitochondrial dysfunction, inflammation, DNA damage and ECM alteration via the activation of MAPK and TGF-β signaling pathways, leading to the upregulation of MMP expression and suppression of collagen synthesis. Antioxidants, including dietary supplements and extracts from plants and animals, ameliorate ROS production, and their therapeutic effects have been confirmed in animal models. Owing to their convenience, easy accessibility and portability, and relatively lower prices, antioxidants are considered as potential treatments. This also led us to determine whether the use of antioxidants from an early age can reduce or prevent further harm. The ingredients need to be used for extended periods to ensure visible improvement and may require lifetime intake/application. Meanwhile, their safety and anti-aging effects need further confirmation.
Stem cell-based treatment has shown great potential in skin rejuvenation and regenerative medicine, as evidenced by findings from several clinical trials. Stem cells such as ADSCs protect cells from oxidative damage by secreting growth factors (such as HGF and VEGF), cytokines such as IL-6 (through the promotion of STAT3 and Nrf2 expression), and antioxidant enzymes (eg. GPx, SOD, and catalase). Protective factors such as laminins, COL17A1, Nrip1, and BAML1 participate in skin aging by promoting stem cell homeostasis. Therefore, it is likely that these factors can serve as anti-aging targets. In addition, the recent progressive application of stem cell-based nanotechnologies has provided insights on this issue. Physical and histological therapeutic effects were observed in nanofat grafting and exosomes derived from stem cells, some of these therapeutic effects were observed in human. Skin rejuvenation yields more significant effects in a shorter time span. However, many of the procedures are invasive and can only be performed at certain qualified facilities. Besides, challenges involving healthcare regulatory issues, poor survival of administered cells, and the risk of biological contamination are present. Furthermore, a cell-free system, such as the sustained release of adipose collagen fragments, has been suggested to overcome the disadvantages of stem cell treatment. Based on the minor complications reported, the safety and feasibility of stem cell-based therapy need to be confirmed in a larger population.
Author contributions
HQ and YS wrote the manuscript. LW and HQ conceived and supervised this work. DL, MZ, CW, and RG revised the manuscript. All authors approved the final version.
Funding
This study was funded by the Zhejiang Provincial Natural Science Foundation of China (LGD21H150001).
Conflict of interest
The authors declare that the research was conducted in the absence of any commercial or financial relationships that could be construed as a potential conflict of interest.
Publisher’s note
All claims expressed in this article are solely those of the authors and do not necessarily represent those of their affiliated organizations, or those of the publisher, the editors and the reviewers. Any product that may be evaluated in this article, or claim that may be made by its manufacturer, is not guaranteed or endorsed by the publisher.
References
Aleemardani, M., Trikić, M. Z., Green, N. H., and Claeyssens, F. (2021). The importance of mimicking dermal-epidermal junction for skin tissue engineering: A review. Bioeng. (Basel) 8, 148. doi:10.3390/bioengineering8110148
Alhaddad, M., Boen, M., Wu, D. C., and Goldman, M. P. (2019). Red deer umbilical cord lining mesenchymal stem cell extract cream for rejuvenation of the face. J. Drugs Dermatology JDD 18, 363–366.
Amer, R. I., Ezzat, S. M., Aborehab, N. M., Ragab, M. F., Mohamed, D., Hashad, A., et al. (2021). Downregulation of MMP1 expression mediates the anti-aging activity of Citrus sinensis peel extract nanoformulation in UV induced photoaging in mice. Biomed. Pharmacother. 138, 111537. doi:10.1016/j.biopha.2021.111537
Ansel, J., Perry, P., Brown, J., Damm, D., Phan, T., Hart, C., et al. (1990). Cytokine modulation of keratinocyte cytokines. J. Investigative Dermatology 94, s101–s107. doi:10.1111/1523-1747.ep12876053
Auh, J. H., and Madhavan, J. (2021). Protective effect of a mixture of marigold and rosemary extracts on UV-induced photoaging in mice. Biomed. Pharmacother. 135, 111178. doi:10.1016/j.biopha.2020.111178
Avola, R., Graziano, A. C. E., Pannuzzo, G., Bonina, F., and Cardile, V. (2019). Hydroxytyrosol from olive fruits prevents blue-light-induced damage in human keratinocytes and fibroblasts. J. Cell Physiol. 234, 9065–9076. doi:10.1002/jcp.27584
Bellei, B., Migliano, E., Tedesco, M., Caputo, S., Papaccio, F., Lopez, G., et al. (2018). Adipose tissue-derived extracellular fraction characterization: Biological and clinical considerations in regenerative medicine. Stem Cell Res. Ther. 9, 207. doi:10.1186/s13287-018-0956-4
Bhatia, E., Kumari, D., Sharma, S., Ahamad, N., and Banerjee, R. (2022). Nanoparticle platforms for dermal antiaging technologies: Insights in cellular and molecular mechanisms. Nanomedicine Nanobiotechnology 14, e1746. doi:10.1002/wnan.1746
Birch-Machin, M. A., and Bowman, A. (2016). Oxidative stress and ageing. Br. J. Dermatology 175, 26–29. doi:10.1111/bjd.14906
Blanpain, C., and Fuchs, E. (2006). Epidermal stem cells of the skin. Annu. Rev. Cell Dev. Biol. 22, 339–373. doi:10.1146/annurev.cellbio.22.010305.104357
Bocheva, G., Slominski, R. M., and Slominski, A. T. (2019). Neuroendocrine aspects of skin aging. Int. J. Mol. Sci. 20, 2798. doi:10.3390/ijms20112798
Brégégère, F., Soroka, Y., Bismuth, J., Friguet, B., and Milner, Y. (2003). Cellular senescence in human keratinocytes: Unchanged proteolytic capacity and increased protein load. Exp. Gerontol. 38, 619–629. doi:10.1016/s0531-5565(03)00059-7
Bryan, F. (2019). Role of PGE-2 and other inflammatory mediators in skin aging and their inhibition by topical natural anti-inflammatories. Cosmetics 6, 1. doi:10.3390/cosmetics6010006
Burke, K. E. (2018). Mechanisms of aging and development-A new understanding of environmental damage to the skin and prevention with topical antioxidants. Mech. Ageing Dev. 172, 123–130. doi:10.1016/j.mad.2017.12.003
Calcinotto, A., Kohli, J., Zagato, E., Pellegrini, L., Demaria, M., and Alimonti, A. (2019). Cellular senescence: Aging, cancer, and injury. Physiol. Rev. 99, 1047–1078. doi:10.1152/physrev.00020.2018
Campbell, K. L., and Lichtensteiger, C. A. (2004). “1 - structure and function of the SKIN,” in Small animal dermatology secrets. Editor K. L. Campbell (Philadelphia: Hanley & Belfus), 1–9.
Cavinato, M., and Jansen-Dürr, P. (2017). Molecular mechanisms of UVB-induced senescence of dermal fibroblasts and its relevance for photoaging of the human skin. Exp. Gerontol. 94, 78–82. doi:10.1016/j.exger.2017.01.009
Cha, H., Hong, S., Park, J. H., and Park, H. H. (2020). Stem cell-derived exosomes and nanovesicles: Promotion of cell proliferation, migration, and anti-senescence for treatment of wound damage and skin ageing. Pharmaceutics 12, 1135. doi:10.3390/pharmaceutics12121135
Charles-de-Sá, L., Gontijo-de-Amorim, N. F., Maeda Takiya, C., Borojevic, R., Benati, D., Bernardi, P., et al. (2015). Antiaging treatment of the facial skin by fat graft and adipose-derived stem cells. Plastic Reconstr. Surg. 135, 999–1009. doi:10.1097/prs.0000000000001123
Charles-de-Sá, L., Gontijo-de-Amorim, N. F., Rigotti, G., Sbarbati, A., Bernardi, P., Benati, D., et al. (2020). Photoaged skin therapy with adipose-derived stem cells. Plast. Reconstr. Surg. 145, 1037e–1049e. doi:10.1097/prs.0000000000006867
Chen, H., Li, Y., Xie, X., Chen, M., Xue, L., Wang, J., et al. (2022). Exploration of the molecular mechanisms underlying the anti-photoaging effect of limosilactobacillus fermentum XJC60. Front. Cell Infect. Microbiol. 12, 838060. doi:10.3389/fcimb.2022.838060
Choi, J. S., Cho, W. L., Choi, Y. J., Kim, J. D., Park, H.-A., Kim, S. Y., et al. (2019). Functional recovery in photo-damaged human dermal fibroblasts by human adipose-derived stem cell extracellular vesicles. J. Extracell. Vesicles 8, 1565885. doi:10.1080/20013078.2019.1565885
Choi, S.-I., Han, H.-S., Kim, J.-M., Park, G., Jang, Y.-P., Shin, Y.-K., et al. (2021). Eisenia bicyclis extract repairs UVB-induced skin photoaging in vitro and in vivo: Photoprotective effects. Mar. Drugs 19, 693. doi:10.3390/md19120693
Cocetta, V., Cadau, J., Saponaro, M., Giacomini, I., Dall'Acqua, S., Sut, S., et al. (2021). Further assessment of Salvia haenkei as an innovative strategy to counteract skin photo-aging and restore the barrier integrity. Aging 13, 89–103. doi:10.18632/aging.202464
Cohen, S. R. (2016). Commentary on: Expanded stem cells, stromal-vascular fraction, and platelet-rich plasma enriched fat: Comparing results of different facial rejuvenation approaches in a clinical trial. Aesthet. Surg. J. 36, 271–274. doi:10.1093/asj/sjv239
Cohen, S. R., Hewett, S., Ross, L., Delaunay, F., Goodacre, A., Ramos, C., et al. (2017). Regenerative cells for facial surgery: Biofilling and biocontouring. Aesthetic Surg. J. 37, S16–S32. doi:10.1093/asj/sjx078
Cui, B., Wang, Y., Jin, J., Yang, Z., Guo, R., Li, X., et al. (2022). Resveratrol treats UVB-induced photoaging by anti-MMP expression, through anti-inflammatory, antioxidant, and antiapoptotic properties, and treats photoaging by upregulating VEGF-B expression. Oxidative Med. Cell. Longev. 2022, 1–19. doi:10.1155/2022/6037303
Dahl, M. V. (2012). Stem cells and the skin. J. Cosmet. Dermatology 11, 297–306. doi:10.1111/jocd.12008
Daré, R. G., Nakamura, C. V., Ximenes, V. F., and Lautenschlager, S. O. S. (2020). Tannic acid, a promising anti-photoaging agent: Evidences of its antioxidant and anti-wrinkle potentials, and its ability to prevent photodamage and MMP-1 expression in L929 fibroblasts exposed to UVB. Free Radic. Biol. Med. 160, 342–355. doi:10.1016/j.freeradbiomed.2020.08.019
Deng, M., Yu, T. Z., Li, D., Wang, X., Zhou, G., Liu, W., et al. (2020). Human umbilical cord mesenchymal stem cell-derived and dermal fibroblast-derived extracellular vesicles protect dermal fibroblasts from ultraviolet radiation-induced photoaging in vitro. Photochem Photobiol. Sci. 19, 406–414. doi:10.1039/c9pp00421a
Eckhart, L., and Zeeuwen, P. L. J. M. (2018). The skin barrier: Epidermis vs environment. Exp. Dermatol 27, 805–806. doi:10.1111/exd.13731
El-Domyati, M., Moftah, N. H., Nasif, G. A., Ameen, S. W., Ibrahim, M. R., and Ragaie, M. H. (2020). Facial rejuvenation using stem cell conditioned media combined with skin needling: A split-face comparative study. J. Cosmet. Dermatology 19, 2404–2410. doi:10.1111/jocd.13594
Fernando, I. P. S., Heo, S. J., Dias, M., Madusanka, D. M. D., Han, E. J., Kim, M. J., et al. (2021). (-)-Loliolide isolated from sargassum horneri abate UVB-induced oxidative damage in human dermal fibroblasts and subside ECM degradation. Mar. Drugs 19, 435. doi:10.3390/md19080435
Geahchan, S., Baharlouei, P., and Rahman, A. (2022). Marine collagen: A promising biomaterial for wound healing, skin anti-aging, and bone regeneration. Mar. Drugs 20, 61. doi:10.3390/md20010061
Giangreco, A., Qin, M., Pintar, J. E., and Watt, F. M. (2008). Epidermal stem cells are retained in vivo throughout skin aging. Aging Cell 7, 250–259. doi:10.1111/j.1474-9726.2008.00372.x
Gilchrest, B. A. (1989). Skin aging and photoaging: An overview. J. Am. Acad. Dermatology 21, 610–613. doi:10.1016/s0190-9622(89)70227-9
Gruber, F., Kremslehner, C., Eckhart, L., and Tschachler, E. (2020). Cell aging and cellular senescence in skin aging - recent advances in fibroblast and keratinocyte biology. Exp. Gerontol. 130, 110780. doi:10.1016/j.exger.2019.110780
Gu, Y., Han, J., Jiang, C., and Zhang, Y. (2020). Biomarkers, oxidative stress and autophagy in skin aging. Ageing Res. Rev. 59, 101036. doi:10.1016/j.arr.2020.101036
Gu, Y., Xue, F., Xiao, H., Chen, L., and Zhang, Y. (2022). Bamboo leaf flavonoids suppress oxidative stress-induced senescence of HaCaT cells and UVB-induced photoaging of mice through p38 MAPK and autophagy signaling. Nutrients 14, 793. doi:10.3390/nu14040793
Han, H. S., Shin, J. S., Myung, D. B., Ahn, H. S., Lee, S. H., Kim, H. J., et al. (2019). Hydrangea serrata (thunb.) ser. Extract attenuate UVB-induced photoaging through MAPK/AP-1 inactivation in human skin fibroblasts and hairless mice. Nutrients 11, 533. doi:10.3390/nu11030533
Harrell, C. R., Gazdic, M., Fellabaum, C., Jovicic, N., Djonov, V., Arsenijevic, N., et al. (2019). Therapeutic potential of amniotic fluid derived mesenchymal stem cells based on their differentiation capacity and immunomodulatory properties. Curr. Stem Cell Res. Ther. 14, 327–336. doi:10.2174/1574888x14666190222201749
Holvoet, S., Vincent, C., Schmitt, D., and Serres, M. (2003). The inhibition of MAPK pathway is correlated with down-regulation of MMP-9 secretion induced by TNF-alpha in human keratinocytes. Exp. Cell Res. 290, 108–119. doi:10.1016/s0014-4827(03)00293-3
Hseu, Y. C., Vudhya Gowrisankar, Y., Chen, X. Z., Yang, Y. C., and Yang, H. L. (2020). The antiaging activity of ergothioneine in UVA-irradiated human dermal fibroblasts via the inhibition of the AP-1 pathway and the activation of nrf2-mediated antioxidant genes. Oxid. Med. Cell Longev. 2020, 1–13. doi:10.1155/2020/2576823
Hu, Y., Zhu, Y., Gerber, S. D., Osland, J. M., Chen, M., Rao, K. A., et al. (2021). Deletion of Nrip1 delays skin aging by reducing adipose-derived mesenchymal stem cells (ADMSCs) senescence, and maintaining ADMSCs quiescence. Geroscience 43, 1815–1833. doi:10.1007/s11357-021-00344-y
Hughes, V. A., Roubenoff, R., Wood, M., Frontera, W. R., Evans, W. J., and Fiatarone Singh, M. A. (2004). Anthropometric assessment of 10-y changes in body composition in the elderly. Am. J. Clin. Nutr. 80, 475–482. doi:10.1093/ajcn/80.2.475
Hwang, E., Ngo, H. T. T., Seo, S. A., Park, B., Zhang, M., and Yi, T. H. (2018). Protective effect of dietary Alchemilla mollis on UVB-irradiated premature skin aging through regulation of transcription factor NFATc1 and Nrf2/ARE pathways. Phytomedicine 39, 125–136. doi:10.1016/j.phymed.2017.12.025
Hwang, I., Choi, K.-A., Kim, M., and Hong, S. (2019). Neural stem cells and the secreted proteins TIMPs ameliorate UVB-induced skin photodamage. Biochem. Biophysical Res. Commun. 518, 388–395. doi:10.1016/j.bbrc.2019.08.068
Hyun, Y. J., Piao, M. J., Kang, K. A., Zhen, A. X., Madushan Fernando, P. D. S., Kang, H. K., et al. (2019). Effect of fermented fish oil on fine particulate matter-induced skin aging. Mar. Drugs 17.
Iriyama, S., Yasuda, M., Nishikawa, S., Takai, E., Hosoi, J., and Amano, S. (2020). Decrease of laminin-511 in the basement membrane due to photoaging reduces epidermal stem/progenitor cells. Sci. Rep. 10, 12592. doi:10.1038/s41598-020-69558-y
Jin, Y. J., Ji, Y., Jang, Y. P., and Choung, S. Y. (2021). Acer tataricum subsp. ginnala inhibits skin photoaging via regulating MAPK/AP-1, NF-κB, and tgfβ/smad signaling in UVB-irradiated human dermal fibroblasts. Molecules 26, 662. doi:10.3390/molecules26030662
Kammeyer, A., and Luiten, R. M. (2015). Oxidation events and skin aging. Ageing Res. Rev. 21, 16–29. doi:10.1016/j.arr.2015.01.001
Kim, J.-A., Ahn, B.-N., Kong, C.-S., and Kim, S.-K. (2012). Chitooligomers inhibit UV-A-induced photoaging of skin by regulating TGF-β/Smad signaling cascade. Carbohydr. Polym. 88, 490–495. doi:10.1016/j.carbpol.2011.12.032
Kim, Y. I., Oh, W. S., Song, P. H., Yun, S., Kwon, Y. S., Lee, Y. J., et al. (2018). Anti-photoaging effects of low molecular-weight fucoidan on ultraviolet B-irradiated mice. Mar. Drugs 16.
Kohl, E., Steinbauer, J., Landthaler, M., and Szeimies, R. M. (2011). Skin ageing. J. Eur. Acad. Dermatology Venereol. JEADV 25, 873–884. doi:10.1111/j.1468-3083.2010.03963.x
Kopnin, P. B., Agapova, L. S., Kopnin, B. P., and Chumakov, P. M. (2007). Repression of sestrin family genes contributes to oncogenic Ras-induced reactive oxygen species up-regulation and genetic instability. Cancer Res. 67, 4671–4678. doi:10.1158/0008-5472.can-06-2466
Kusuma, G. D., Carthew, J., Lim, R., and Frith, J. E. (2017). Effect of the microenvironment on mesenchymal stem cell paracrine signaling: Opportunities to engineer the therapeutic effect. Stem Cells Dev. 26, 617–631. doi:10.1089/scd.2016.0349
Lavker, R. M., Zheng, P., and Dong, G. (1987). Aged skin: A study by light, transmission electron, and scanning electron microscopy. J. Investigative Dermatology 88, 44–51. doi:10.1038/jid.1987.9
Lavker, R. M., Zheng, P. S., and Dong, G. (1986). Morphology of aged skin. Dermatol Clin. 4, 379–389. doi:10.1016/s0733-8635(18)30801-5
Lee, H., Cha, H., and Park, J. H. (2020). Derivation of cell-engineered nanovesicles from human induced pluripotent stem cells and their protective effect on the senescence of dermal fibroblasts. Int. J. Mol. Sci. 21, 343. doi:10.3390/ijms21010343
Lee, H., Hong, Y., and Kim, M. (2021). Structural and functional changes and possible molecular mechanisms in aged skin. Int. J. Mol. Sci. 22, 12489. doi:10.3390/ijms222212489
Lee, Y. I., Kim, S., Kim, J., Kim, J., Chung, K. B., and Lee, J. H. (2021). Randomized controlled study for the anti-aging effect of human adipocyte-derived mesenchymal stem cell media combined with niacinamide after laser therapy. J. Cosmet. Dermatol 20, 1774–1781. doi:10.1111/jocd.13767
Lee, Y. I., Lee, S. G., Jung, I., Suk, J., Lee, M. H., Kim, D. U., et al. (2022). Effect of a topical collagen tripeptide on antiaging and inhibition of glycation of the skin: A pilot study. Int. J. Mol. Sci. 23, 1101. doi:10.3390/ijms23031101
Li, D., Shao, R., Wang, N., Zhou, N., Du, K., Shi, J., et al. (2021). Sulforaphane Activates a lysosome-dependent transcriptional program to mitigate oxidative stress. Autophagy 17, 872–887. doi:10.1080/15548627.2020.1739442
Li, L., Ngo, H. T. T., Hwang, E., Wei, X., Liu, Y., Liu, J., et al. (2019). Conditioned medium from human adipose-derived mesenchymal stem cell culture prevents UVB-induced skin aging in human keratinocytes and dermal fibroblasts. Int. J. Mol. Sci. 21, 49. doi:10.3390/ijms21010049
Li, Q., Bai, D., Qin, L., Shao, M., Liu, X., Zhang, S., et al. (2020). Protective effect of L-hexaguluroic acid hexasodium salt on UVA-induced photo-aging in HaCaT cells. Int. J. Mol. Sci. 21, 1201. doi:10.3390/ijms21041201
Li, Q., Bai, D., Qin, L., Shao, M., Zhang, S., Yan, C., et al. (2020). Protective effect of d-tetramannuronic acid tetrasodium salt on UVA-induced photo-aging in HaCaT cells. Biomed. Pharmacother. 126, 110094. doi:10.1016/j.biopha.2020.110094
Li, T., Lu, H., Zhou, L., Jia, M., Zhang, L., Wu, H., et al. (2022). Growth factors-based platelet lysate rejuvenates skin against ageing through NF-κB signalling pathway: In vitro and in vivo mechanistic and clinical studies. Cell Prolif. 55, e13212. doi:10.1111/cpr.13212
Li, Y.-F., Ouyang, S.-H., Tu, L.-F., Wang, X., Yuan, W.-L., Wang, G.-E., et al. (2018). Caffeine protects skin from oxidative stress-induced senescence through the activation of autophagy. Theranostics 8, 5713–5730. doi:10.7150/thno.28778
Liang, J.-X., Liao, X., Li, S.-H., Jiang, X., Li, Z.-H., Wu, Y.-D., et al. (2020). Antiaging properties of exosomes from adipose-derived mesenchymal stem cells in photoaged rat skin. BioMed Res. Int. 2020, 1–13. doi:10.1155/2020/6406395
Liu, N., Matsumura, H., Kato, T., Ichinose, S., Takada, A., Namiki, T., et al. (2019). Stem cell competition orchestrates skin homeostasis and ageing. Nature 568, 344–350. doi:10.1038/s41586-019-1085-7
Lohakul, J., Jeayeng, S., Chaiprasongsuk, A., Torregrossa, R., Wood, M. E., Saelim, M., et al. (2022). Mitochondria-targeted hydrogen sulfide delivery molecules protect against UVA-induced photoaging in human dermal fibroblasts, and in mouse skin in vivo. Antioxid. Redox Signal 36, 1268–1288. doi:10.1089/ars.2020.8255
Mailloux, R. J. (2015). Teaching the fundamentals of electron transfer reactions in mitochondria and the production and detection of reactive oxygen species. Redox Biol. 4, 381–398. doi:10.1016/j.redox.2015.02.001
Maity, P., Singh, K., Krug, L., Koroma, A., Hainzl, A., Bloch, W., et al. (2021). Persistent JunB activation in fibroblasts disrupts stem cell niche interactions enforcing skin aging. Cell Rep. 36, 109634. doi:10.1016/j.celrep.2021.109634
Meunier, M., Scandolera, A., Chapuis, E., Lambert, C., Jarrin, C., Robe, P., et al. (2019). From stem cells protection to skin microbiota balance: Orobanche rapum extract, a new natural strategy. J. Cosmet. Dermatol 18, 1140–1154. doi:10.1111/jocd.12804
Moon, N. R., Kang, S., and Park, S. (2018). Consumption of ellagic acid and dihydromyricetin synergistically protects against UV-B induced photoaging, possibly by activating both TGF-β1 and wnt signaling pathways. J. Photochem Photobiol. B 178, 92–100. doi:10.1016/j.jphotobiol.2017.11.004
Nagase, H., Visse, R., and Murphy, G. (2006). Structure and function of matrix metalloproteinases and TIMPs. Cardiovasc. Res. 69, 562–573. doi:10.1016/j.cardiores.2005.12.002
Namkoong, J., Kern, D., and Knaggs, H. E. (2018). Assessment of human skin gene expression by different blends of plant extracts with implications to periorbital skin aging. Int. J. Mol. Sci. 19, 3349. doi:10.3390/ijms19113349
Niemann, C., and Watt, F. M. (2002). Designer skin: Lineage commitment in postnatal epidermis. Trends Cell Biol. 12, 185–192. doi:10.1016/s0962-8924(02)02263-8
Oh, D. S., Kim, D. H., Roh, T. S., Yun, I. S., and Kim, Y. S. (2014). Correction of dark coloration of the lower eyelid skin with nanofat grafting. Arch. Aesthetic Plast. Surg. 20, 92–96. doi:10.14730/aaps.2014.20.2.92
Oh, M., Lee, J., Kim, Y. J., Rhee, W. J., and Park, J. H. (2018). Exosomes derived from human induced pluripotent stem cells ameliorate the aging of skin fibroblasts. Int. J. Mol. Sci. 19, 1715. doi:10.3390/ijms19061715
Ono, Y., Torii, K., Fritsche, E., Shintani, Y., Nishida, E., Nakamura, M., et al. (2013). Role of the aryl hydrocarbon receptor in tobacco smoke extract-induced matrix metalloproteinase-1 expression. Exp. Dermatol 22, 349–353. doi:10.1111/exd.12148
Pangestuti, R., Shin, K. H., and Kim, S. K. (2021). Anti-photoaging and potential skin health benefits of seaweeds. Mar. Drugs 19, 172. doi:10.3390/md19030172
Park, E. K., Lee, H. J., Lee, H., Kim, J. H., Hwang, J., Koo, J. I., et al. (2018). The anti-wrinkle mechanism of melatonin in UVB treated HaCaT keratinocytes and hairless mice via inhibition of ROS and sonic hedgehog mediated inflammatory proteins. Int. J. Mol. Sci. 19, 1995. doi:10.3390/ijms19071995
Pecorelli, A., Woodby, B., Prieux, R., and Valacchi, G. (2019). Involvement of 4-hydroxy-2-nonenal in pollution-induced skin damage. Biofactors 45, 536–547. doi:10.1002/biof.1513
Petkovic, M., Leal, E. C., Alves, I., Bose, C., Palade, P. T., Singh, P., et al. (2021). Dietary supplementation with sulforaphane ameliorates skin aging through activation of the Keap1-Nrf2 pathway. J. Nutr. Biochem. 98, 108817. doi:10.1016/j.jnutbio.2021.108817
Prakoeswa, C. R. S., Pratiwi, F. D., Herwanto, N., Citrashanty, I., Indramaya, D. M., Murtiastutik, D., et al. (2019). The effects of amniotic membrane stem cell-conditioned medium on photoaging. J. Dermatol. Treat. 30, 478–482. doi:10.1080/09546634.2018.1530438
Prasanth, M. I., Sivamaruthi, B. S., Chaiyasut, C., and Tencomnao, T. (2019). A review of the role of green tea (camellia sinensis) in antiphotoaging, stress resistance, neuroprotection, and autophagy. Nutrients 11, 474. doi:10.3390/nu11020474
Quan, T., He, T., Kang, S., Voorhees, J. J., and Fisher, G. J. (2004). Solar ultraviolet irradiation reduces collagen in photoaged human skin by blocking transforming growth factor-beta type II receptor/Smad signaling. Am. J. Pathology 165, 741–751. doi:10.1016/s0002-9440(10)63337-8
Quan, T., Qin, Z., Robichaud, P., Voorhees, J. J., and Fisher, G. J. (2011). CCN1 contributes to skin connective tissue aging by inducing age-associated secretory phenotype in human skin dermal fibroblasts. J. Cell Commun. Signal 5, 201–207. doi:10.1007/s12079-011-0144-0
Quan, T., Qin, Z., Xia, W., Shao, Y., Voorhees, J. J., and Fisher, G. J. (2009). Matrix-degrading metalloproteinases in photoaging. J. Investigative Dermatology Symposium Proc. 14, 20–24. doi:10.1038/jidsymp.2009.8
Rigotti, G., Charles-de-Sá, L., Gontijo-de-Amorim, N. F., Takiya, C. M., Amable, P. R., Borojevic, R., et al. (2016). Expanded stem cells, stromal-vascular fraction, and platelet-rich plasma enriched fat: Comparing results of different facial rejuvenation approaches in a clinical trial. Aesthet. Surg. J. 36, 261–270. doi:10.1093/asj/sjv231
Samivel, R., Nagarajan, R. P., Subramanian, U., Khan, A. A., Masmali, A., Almubrad, T., et al. (2020). Inhibitory effect of ursolic acid on ultraviolet B radiation-induced oxidative stress and proinflammatory response-mediated senescence in human skin dermal fibroblasts. Oxidative Med. Cell. Longev. 2020, 1–17. doi:10.1155/2020/1246510
Shaulian, E., and Karin, M. (2002). AP-1 as a regulator of cell life and death. Nat. Cell Biol. 4, E131–E136. doi:10.1038/ncb0502-e131
Shen, T., Zheng, Q.-Q., Shen, J., Li, Q.-S., Song, X.-H., Luo, H.-B., et al. (2018). Effects of adipose-derived mesenchymal stem cell exosomes on corneal stromal fibroblast viability and extracellular matrix synthesis. Chin. Med. J. Engl. 131, 704–712. doi:10.4103/0366-6999.226889
Sies, H., Berndt, C., and Jones, D. P. (2017). Oxidative stress. Annu. Rev. Biochem. 86, 715–748. doi:10.1146/annurev-biochem-061516-045037
Singh, B., Schoeb, T. R., Bajpai, P., Slominski, A., and Singh, K. K. (2018). Reversing wrinkled skin and hair loss in mice by restoring mitochondrial function. Cell Death Dis. 9, 735. doi:10.1038/s41419-018-0765-9
Surowiecka, A., and Strużyna, J. (2022). Adipose-derived stem cells for facial rejuvenation. J. Pers. Med. 12, 117. doi:10.3390/jpm12010117
Théry, C., Witwer, K. W., Aikawa, E., Alcaraz, M. J., Anderson, J. D., Andriantsitohaina, R., et al. (2018). Clinical research using extracellular vesicles: Insights from the international society for extracellular vesicles 2018 annual meeting. J. Extracell. Vesicles 7, 1535744. doi:10.1080/20013078.2018.1535744
Tian, L., Ke, D., Hong, Y., Zhang, C., Tian, D., Chen, L., et al. (2021). Artesunate treatment ameliorates ultraviolet irradiation-driven skin photoaging via increasing β-catenin expression. Aging (Albany NY) 13, 25325–25341. doi:10.18632/aging.203749
Tonnard, P., Verpaele, A., Peeters, G., Hamdi, M., Cornelissen, M., and Declercq, H. (2013). Nanofat grafting: Basic research and clinical applications. Plastic Reconstr. Surg. 132, 1017–1026. doi:10.1097/prs.0b013e31829fe1b0
Tsai, S.-R., and Hamblin, M. R. (2017). Biological effects and medical applications of infrared radiation. J. Photochem. Photobiol. B, Biol. 170, 197–207. doi:10.1016/j.jphotobiol.2017.04.014
Umbayev, B., Askarova, S., Almabayeva, A., Saliev, T., Masoud, A. R., and Bulanin, D. (2020). Galactose-induced skin aging: The role of oxidative stress. Oxid. Med. Cell Longev. 2020, 1–15. doi:10.1155/2020/7145656
Varani, J., Schuger, L., Dame, M. K., Leonard, C., Fligiel, S. E. G., Kang, S., et al. (2004). Reduced fibroblast interaction with intact collagen as a mechanism for depressed collagen synthesis in photodamaged skin. J. Investigative Dermatology 122, 1471–1479. doi:10.1111/j.0022-202x.2004.22614.x
Velarde, M. C., Flynn, J. M., Day, N. U., Melov, S., and Campisi, J. (2012). Mitochondrial oxidative stress caused by Sod2 deficiency promotes cellular senescence and aging phenotypes in the skin. Aging 4, 3–12. doi:10.18632/aging.100423
Verpaele, A., Tonnard, P., Jeganathan, C., Ramaut, L., and Needling, Nanofat (2019). Nanofat needling. Plastic Reconstr. Surg. 143, 1062–1065. doi:10.1097/prs.0000000000005455
Wang, L., Kim, H. S., Oh, J. Y., Je, J. G., Jeon, Y. J., and Ryu, B. (2020). Protective effect of diphlorethohydroxycarmalol isolated from Ishige okamurae against UVB-induced damage in vitro in human dermal fibroblasts and in vivo in zebrafish. Food Chem. Toxicol. 136, 110963. doi:10.1016/j.fct.2019.110963
Wang, L., Lee, W., Cui, Y. R., Ahn, G., and Jeon, Y. J. (2019). Protective effect of green tea catechin against urban fine dust particle-induced skin aging by regulation of NF-κB, AP-1, and MAPKs signaling pathways. Environ. Pollut. 252, 1318–1324. doi:10.1016/j.envpol.2019.06.029
Wang, L., Oh, J. Y., Kim, Y. S., Lee, H. G., Lee, J. S., and Jeon, Y. J. (2020). Anti-photoaging and anti-melanogenesis effects of fucoidan isolated from hizikia fusiforme and its underlying mechanisms. Mar. Drugs 18, 427. doi:10.3390/md18080427
Wang, X., Shu, X., Huo, W., Zou, L., and Li, L. (2018). Efficacy of protein extracts from medium of Adipose-derived stem cells via microneedles on Asian skin. J. Cosmet. Laser Ther. 20, 237–244.
Wang, Y., Wang, L., Wen, X., Hao, D., Zhang, N., He, G., et al. (2019). NF-κB signaling in skin aging. Mech. Ageing Dev. 184, 111160. doi:10.1016/j.mad.2019.111160
Wang, Z., Man, M.-Q., Li, T., Elias, P. M., and Mauro, T. M. (2020). Aging-associated alterations in epidermal function and their clinical significance. Aging 12, 5551–5565. doi:10.18632/aging.102946
Wei, H., Gu, S.-X., Liang, Y.-D., Liang, Z.-J., Chen, H., Zhu, M.-G., et al. (2017). Nanofat-derived stem cells with platelet-rich fibrin improve facial contour remodeling and skin rejuvenation after autologous structural fat transplantation. Oncotarget 8, 68542–68556. doi:10.18632/oncotarget.19721
Wei, Y., Jian-Ming, C., Xiao-Jun, T., Chu, L., Dong-Jie, H., Jing, J., et al. (2016). “Mitochondrial impairment mechanism in D-galactose-induced senescence in experimental fibroblast cell model,” in Proceedings of the 2016 International Conference on Biological Sciences and Technology (Dordrecht: Atlantis Press), 113–124.
Welz, P.-S., Zinna, V. M., Symeonidi, A., Koronowski, K. B., Kinouchi, K., Smith, J. G., et al. (2019). BMAL1-Driven tissue clocks respond independently to light to maintain homeostasis. Cell 177, 1029. doi:10.1016/j.cell.2019.07.030
Wu, P.-Y., Lin, T.-Y., Hou, C.-W., Chang, Q.-X., Wen, K.-C., Lin, C.-Y., et al. (2019). 1, 2-bis[(3-Methoxyphenyl)Methyl]Ethane-1, 2-dicarboxylic acid reduces UVB-induced photodamage in vitro and in vivo. Antioxidants (Basel) 8, 452. doi:10.3390/antiox8100452
Wu, P., Zhang, B., Han, X., Sun, Y., Sun, Z., Li, L., et al. (2021). HucMSC exosome-delivered 14-3-3ζ alleviates ultraviolet radiation-induced photodamage via SIRT1 pathway modulation. Aging (Albany NY) 13, 11542–11563. doi:10.18632/aging.202851
Xu, D., Li, C., and Zhao, M. (2022). Attenuation of UV-induced skin photoaging in rats by walnut protein hydrolysates is linked to the modulation of MAPK/AP-1 and TGF-β/Smad signaling pathways. Food & Funct. 13, 609–623. doi:10.1039/d1fo02598h
Xu, P., Xin, Y., Zhang, Z., Zou, X., Xue, K., Zhang, H., et al. (2020). Extracellular vesicles from adipose-derived stem cells ameliorate ultraviolet B-induced skin photoaging by attenuating reactive oxygen species production and inflammation. Stem Cell Res. Ther. 11, 264. doi:10.1186/s13287-020-01777-6
Xu, X., Wang, H.-Y., Zhang, Y., Liu, Y., Li, Y.-Q., Tao, K., et al. (2014). Adipose-derived stem cells cooperate with fractional carbon dioxide laser in antagonizing photoaging: A potential role of wnt and β-catenin signaling. Cell Biosci. 4, 24. doi:10.1186/2045-3701-4-24
Xuan, S. H., Lee, N. H., and Park, S. N. (2019). Atractyligenin, a terpenoid isolated from coffee silverskin, inhibits cutaneous photoaging. J. Photochem Photobiol. B 194, 166–173. doi:10.1016/j.jphotobiol.2019.04.002
Yamada, T., Hasegawa, S., Miyachi, K., Date, Y., Inoue, Y., Yagami, A., et al. (2018). Laminin-332 regulates differentiation of human interfollicular epidermal stem cells. Mech. Ageing Dev. 171, 37–46. doi:10.1016/j.mad.2018.03.007
Yang, R., Wang, J., Chen, X., Shi, Y., and Xie, J. (2020). Epidermal stem cells in wound healing and regeneration. Stem Cells Int. 2020, 1–11. doi:10.1155/2020/9148310
Youn, S. W., Kim, D. S., Cho, H. J., Jeon, S. E., Bae, I. H., Yoon, H. J., et al. (2004). Cellular senescence induced loss of stem cell proportion in the skin in vitro. J. Dermatol Sci. 35, 113–123. doi:10.1016/j.jdermsci.2004.04.002
Zhang, K., Yu, L., Li, F. R., Li, X., Wang, Z., Zou, X., et al. (2020). Topical application of exosomes derived from human umbilical cord mesenchymal stem cells in combination with sponge spicules for treatment of photoaging. Int. J. Nanomedicine 15, 2859–2872. doi:10.2147/ijn.s249751
Zhang, S., Dong, Z., Peng, Z., and Lu, F. (2014). Anti-aging effect of adipose-derived stem cells in a mouse model of skin aging induced by D-galactose. PLoS One 9, e97573. doi:10.1371/journal.pone.0097573
Zhou, W., Lin, J., Zhao, K., Jin, K., He, Q., Hu, Y., et al. (2019). Single-cell profiles and clinically useful properties of human mesenchymal stem cells of adipose and bone marrow origin. Am. J. Sports Med. 47, 1722–1733. doi:10.1177/0363546519848678
Zou, Z., Long, X., Zhao, Q., Zheng, Y., Song, M., Ma, S., et al. (2021). A single-cell transcriptomic atlas of human skin aging. Dev. Cell 56, 383–397.e8. doi:10.1016/j.devcel.2020.11.002
Keywords: skin, aging, stem cell, oxidative stress, materials
Citation: Qian H, Shan Y, Gong R, Lin D, Zhang M, Wang C and Wang L (2023) Mechanism of action and therapeutic effects of oxidative stress and stem cell-based materials in skin aging: Current evidence and future perspectives. Front. Bioeng. Biotechnol. 10:1082403. doi: 10.3389/fbioe.2022.1082403
Received: 28 October 2022; Accepted: 23 December 2022;
Published: 09 January 2023.
Edited by:
Huacheng He, Oujiang Laboratory, ChinaReviewed by:
Lei Hu, Capital Medical University, ChinaHan Liu, The University of Chicago, United States
Copyright © 2023 Qian, Shan, Gong, Lin, Zhang, Wang and Wang. This is an open-access article distributed under the terms of the Creative Commons Attribution License (CC BY). The use, distribution or reproduction in other forums is permitted, provided the original author(s) and the copyright owner(s) are credited and that the original publication in this journal is cited, in accordance with accepted academic practice. No use, distribution or reproduction is permitted which does not comply with these terms.
*Correspondence: Huan Qian, aHVhbnFpYW5Aemp1LmVkdS5jbg==; Lu Wang, d2x1MTA0QGdtYWlsLmNvbQ==