- Department of Biomedical Engineering, Shantou University, Shantou, China
Light is an easy acquired, effective and non-invasive external stimulus with great flexibility and focusability. Thus, light responsive hydrogels are of particular interests to researchers in developing accurate and controlled drug delivery systems. Light responsive hydrogels are obtained by incorporating photosensitive moieties into their polymeric structures. Drug release can be realized through three major mechanisms: photoisomerization, photochemical reaction and photothermal reaction. Recent advances in material science have resulted in great development of photosensitizers, such as rare metal nanostructures and black phosphorus nanoparticles, in order to respond to a variety of light sources. Hydrogels incorporated with photosensitizers are crucial for clinical applications, and the use of ultraviolet and near-infrared light as well as up-conversion nanoparticles has greatly increased the therapeutic effects. Existing light responsive drug delivery systems have been utilized in delivering drugs, proteins and genes for chemotherapy, immunotherapy, photodynamic therapy, gene therapy, wound healing and other applications. Principles associated with site-specific targeting, metabolism, and toxicity are used to optimize efficacy and safety, and to improve patient compliance and convenience. In view of the importance of this field, we review current development, challenges and future perspectives of light responsive hydrogels for controlled drug delivery.
1 Introduction
Hydrogels are three-dimensional crosslinked polymeric networks that can absorb large amounts of water or biological fluids. Their structures are formed through chemical or physical crosslinking of different polymer chains, between which either covalent bonds or physical interactions exist to maintain their structural stability (Ebara et al., 2014). Hydrogels are usually characterized with pH-neutral, colorless, odorless, non-toxic, high absorption ability, and excellent stability and constancy in storage with controllable biodegradability (Ullah et al., 2015). Given their technical features, hydrogels have been utilized in designing drug delivery systems for years. Hydrogels can protect the drug from surrounding environments, and their tunable properties plus the ability to retain large fraction of solvents make them ideal carriers for drug delivery systems. By changing hydrogel properties, drug release rate can be accurately controlled. Additionally, hydrogels usually have low affinity with drugs, thus achieve a high fraction of drug release.
Hydrogels can be classified into two groups according to their responses to external stimuli: one is conventional hydrogels, which have no particular sensation to changes of their environment, and the other is stimuli-responsive hydrogels, also known as smart hydrogels that are capable of responding to physical, chemical or biochemical stimuli (Ji et al.eng, 2020). In response to external stimuli, these hydrogels undergo a series of changes in their growth actions, network structure, mechanical strength and permeability (Zhang et al., 2021; Gil and Hudson, 2004/12). Stimuli-responsive hydrogels contain specific components as “on-off” sensors able to detect stimulation signals and subsequently control their changes. For example, ultrasound amplitude and time duration are associated FITC-BSA release rate in chitosan hydrogels with reversible Diels-Alder linkers (Arrizabalaga et al.eng, 2022); and thermosensitive mPEG-PA-PLL hydrogel was used for controlled oral delivery of calcitonin (Cheng et al., 2022). Combining two or more stimuli responsive mechanisms in one hydrogel system, multi-responsive hydrogels can be formed to respond to more than two external stimuli. PF127/TMC/PEG-HA can react to both pH and temperature, and is an example of a dual responsive hydrogel used for in textile-based transdermal therapy (Chatterjee et al., 2019).
2 Fundamentals of light responsive drug delivery system
Light responsive hydrogels are able to respond to light irradiation and subsequently give rise to structural and conformational changes (Jiang and Wen, 2015; Bustamante-Torres et al., 2021). Light is an easy acquired, effective and non-invasive external stimulus that can be highly focused and regulated by manipulating its parameters, including intensity, wavelength, exposure time and beam diameter. Consequently, light offers accurate and spatiotemporal control of drug delivery, and thus received great attention in the past decade (Li et al., 2018a; Rizzo et al., 2021). Light responsive hydrogels are obtained by incorporating photosensitive moieties into the polymer structure. Based on the photosensitizer (PS) used, the response can be reversible or irreversible (Bustamante-Torres et al., 2021). Light can cause cleavage, isomerization, or dimerization of photosensitive groups in hydrogels, and leads to partial or complete decrosslinking, degradation, swelling and/or shrinkage of the hydrogel structure.
The light-controlled drug delivery systems can be classified into three broad categories: photoisomerization-based, photochemical-based, and photothermal-based drug release platforms. Photoisomerization typically involves conformational changes of the hydrogels from trans to cis under light irradiation. During this process, hydrogels open pore sizes and allow drugs to diffuse out of their matrixes (Figure 1A). It does not break chemical bonds of hydrogels, and the process is usually reversible and repeatable (Tomatsu et al., 2011). Photochemical reactions can lead to network structure and configuration changes of the hydrogel, and subsequently induce drug release (Iwaso et al., 2016). Among all photochemical reactions, photocleavage is the one of most commonly used ones for controlled drug delivery. This method is realized by incorporating photocleavable linkers into the hydrogel structure to create nanoparticles that can be cut off by light (Figure 1B) (Miranda and Lovell, 2016; Liese and Hampp, 2011/04). In this case, drugs are tethered to the hydrogel network at designed or selected sites covalently through photocleavable linkers, and can maintain their efficacy and avoid unwanted release to a large extent (Shadish and DeForest, 2020; Wang et al., 2020). The photothermal reaction is utilizing materials able to convert light energy into the heat energy, which in turn induces a disruption of a thermally sensitive drug carrier (Figure 1C) (Qiu et al., 2018; Bordbar-Khiabani and Gasik, 2022). The reaction requires two components - a photosensitizer to convert light into heat, and a material sensitive to temperature changes for drug release.
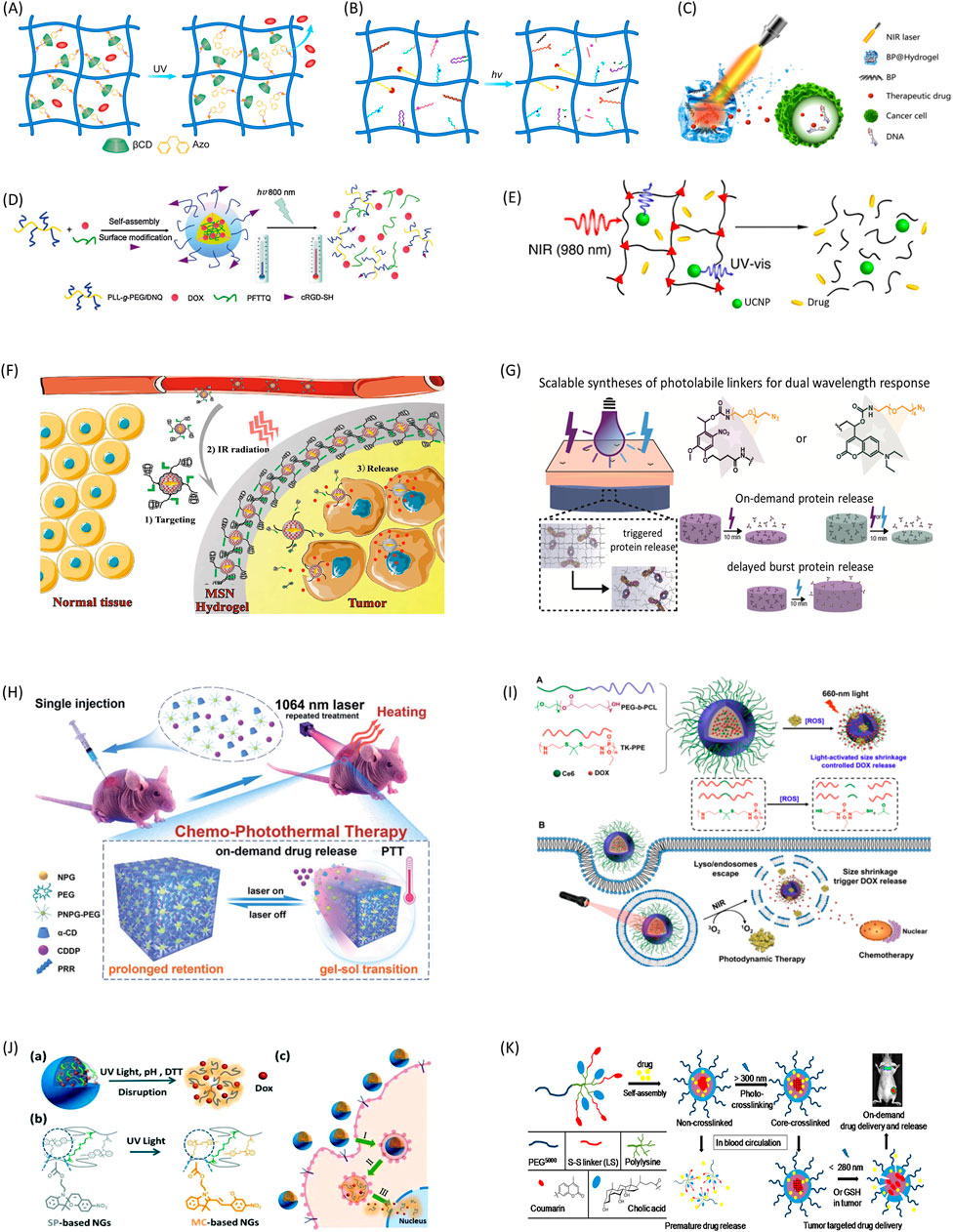
FIGURE 1. Representative mechanisms and applications of light-controlled drug delivery systems. (A) Photoisomerization induced drug release through guest-host interactions. (B) Photocleavage induced drug release through multi mechanisms. (C) Photothermal effect-induced drug release to break DNA chains. Copyright Qiu et al. (2018) NAS. (D) Two-photon light regulated chemo-photothermal therapy with fast drug release. Used with permission of RSC from Yuan et al. (2015). (E) NIR-triggered hydrogel degradation using the UV light generated by UCNPs. Adapted with permission from Yan et al. Copyright 2012 American Chemical Society. (F) Light-induced targeted drug delivery to tumor tissue through photoisomerization. Adapted with permission from Chen et al. Copyright 2016 American Chemical Society. (G) On-demand protein release by dual wavelength lights. Adapted with permission from LeValley et al. Copyright 2020 American Chemical Society. (H) NIR induced repetitive on-demand drug release for chemo-photothermal therapy. Ruan et al. (2019). (I) Combination of photodynamic therapy and chemotherapy through light-induced drug release. Adapted with permission from Cao et al. Copyright 2018 American Chemical Society. (J) DOX release process from light-induced multi stimuli responsive system. Used with permission of RSC from Chen et al., 2017. (K) Light and redox dual responsive coumarin containing micelles as drug nanocarrier for cancer therapy. Shao et al. (2014).
The commonly used light sources include ultraviolet (UV), visible light and near-infrared (NIR). UV once was the most popular light sources because of the availability of a wide range of UV photosensitizers. But it is mainly for in vitro experiments due to its cytotoxicity and low tissue penetration. On the side, NIR is safer for in vivo studies and able to trigger drug release within deep tissues. Thus, NIR is very attractable for clinical applications. Due to recent advance of material science, many new photosensitizers responsive to NIR were developed. Additionally, the use of two-photon excitation (Figure 1D) and up-conversion nanoparticles (UCNPs) (Figure 1E) also greatly expanded situations that NIR can be used (Pokharel and Park, 2022; Yuan et al., 2015; Yan et al., 2012/10). Based on above mechanisms, a variety of drug delivery applications were presented in Table 1 (Tao et al., 2019; Liang et al.eng, 2020; Pourjavadi et al., 2020; Wei et al., 2020; Yu et al., 2020).
3 Photoisomerization-based drug delivery systems
Photoisomerization takes advantage of the conformational changes of certain molecules when stimulated by light irradiation (Pan et al., 2021/10). Azobenzene is the most used molecules in this category. Modifying hydrogels with azobenzene and cyclodextrin (CD) can create light responsive crosslinking between the two components. When in the trans isomer form, azobenzene can have “host-guest” interaction with CD, forming strong crosslinks. Under UV light irradiation, the azobenzene changes to cis isomer form which leads to breaking of crosslinks and drug release.
Light responsive azobenzene-CD guest-host chemistry has been widely used for controlled release of drugs from hydrogels under light exposure (Rosales et al., 2018). In one study, the authors developed a light responsive hydrogel by permeating diazobenzene-modified poly(ethylene glycol) (PEG) into βCD grafted alginate. Exposure of UV light leads to controlled cargo release from the hydrogel which was used in wound healing and other applications (Chiang and Chu, 2015). In another study, a model drug was released from a light responsive hydrogel made from azobenzene and PEG. The system showed reversible photoisomerization between its cis and trans isomers under UV-light irradiation allowing model drug release from the hydrogel network. The authors observed clear volume changes during the photoisomerization process and characterized the peak effects at 330 nm and 435 nm respectively. Thus, the drug release can be controlled by the wavelength and intensity of light irradiation (Rastogi et al.eng, 2018). Additionally, Nehls et al. showed similar results with more accurately controlled release rate of an entrapped model drug based on azobenzene-CD chemistry in PEG hydrogels (Nehls et al., 2016). Furthermore, PAA-based hydrogel modified with methoxy-substituted azobenzene and βCD supramolecular complexes showed a gel to sol transition in response to red light irradiation, and subsequently resulted in a dose dependent manner of loaded BSA release. With higher wavelength than UV, red light can be used for deep tissue drug delivery with less energy-induced damage (Wang et al., 2015). Chen et al. designed a DOX-loaded delivery system targeted tumor tissues using muli-responsive formation and degradation of the hydrogel (Figure 1F) (Chen et al.eng, 2016).
Spiropyran is another well-known photosensitizer that can be incorporated into hydrogel networks. It was used to deliver a variety of drugs such as doxorubicin and paclitaxel based on photoisomerization of hydrophobic spiropyran to hydrophilic merocyanine after UV irradiation. During this process, the swelling of hydrogels cause water soluble drugs to diffuse out of the hydrogel networks (Li et al., 2020/05). Tong et al. reported a nanoparticulate drug delivery system comprising spiropyran and PEGylated lipid that allows repetitive drug delivery at given time and location. The light-sensitive switch enables particles to fluoresce and release drugs inside cells when illuminated with UV light providing a spatiotemporal control of drug delivery with and enhanced tissue penetration (Tong et al., 2012). Spiropyrans within hydrogels have also been used as on and off switch triggers for controlled drug release in a number of other studies due to its reversible properties (Ghani et al., 2021/01; Xiao et al.eng, 2016).
4 Photochemical reaction-based drug delivery systems
Photochemical reactions include photooxidation, photocleavage and photopolymerization. For photocleavage induced drug release, o-nitrobenzyl is the most popular photocleavable linkers. It can be incorporated into many hydrogels to give them light responsive properties. The cleavage of o-nitrobenzyls happens at C-O bond in its ester group after exposure to UV light or high energy visual light. In one study, o-nitrobenzyl moieties were added into gelatin methacryloyl(-acetyl) hydrogels with a biotin-functionalized photocleavable macromer, and then controlled release under UV-irradiation is studied. The authors found that liquid chromatography coupled to mass spectrometry analysis of aqueous linker solutions allows the identification of the main cleavage products and the cleavage kinetics (Claaßen et al., 2018). O-nitrobenzyls were also used to cause a macro physical change in the overall structure for drug delivery (Linsley et al., 2017). In o-nitrobenzyl linked PEG and PAM hydrogels, cleavage of o-nitrobenzyls with UV resulted volume shrinking of their polymeric structure, and caused controlled release of drugs entrapped within its matrix (Yan et al., 2012/10). In a similar study for PEG and dextran hydrogel with o-nitrobenzyl linkers, 60 min exposure to UV leads to fifty percent of model drug release due to hydrogel structure dissociation (Peng et al., 2011). In another study, authors showed a novel strategy enabling the use of upconversion luminescence converting NIR light into UV light, which are received by o-nitrobenzyl groups in PEG hydrogels. Subsequently, the photocleavage reaction leads to tethered drugs (Yan et al., 2011/12).
A ruthenium-based photocleavable linker was developed to form hydrogel with entrapped model drugs, which cannot be released until exposure to light. By varying the coordinated ligands, Ru-cross-linkers have 1-photon absorption maxima that are tunable across the visible spectrum and into the near-infrared, which enables photoactivation at multiple, different wavelengths (Rapp and Dmochowski, 2019). In a more recent study, Yerneni et al. tethered exosomes to poly(ethylene oxide)-based hydrogels using atom transfer radical polymerization. The method allowed controlled release over a period of 1 month and the release profile can be programmed through crosslinking density and light stimuli conditions (Yerneni et al.eng, 2022). Yang et al. developed a photocleavable prodrug loaded injectable glycol chitosan (GC) hydrogel for NIR-triggered repetitive drug release. The hydrogel shows good stability, minimum leakage and efficient light responsibility both in vitro and in vivo (Yang et al., 2022).
When photocleavable linkers attach model drugs to polymer matrix covalently, it may cause great reduction to drug activities as well as unspecified tethers. To overcome these disadvantages, some researchers used recombinant protein techniques to modify proteins with a handle in order to attach a protein of interest to the hydrogel matrix. In one study, a PEG-based hydrogel was developed via a click reaction without impact on entrapped enzyme activity under 60°C thermal stress for weeks. The study showed a general method to preserve drug activities at certain conditions and enable controlled drug release when needed (Sridhar et al., 2018/03). In another study, the authors developed a technique to use light-sensing proteins as light-activated reversible binding sites within synthetic poly(ethylene glycol) (PEG) hydrogels. It has reversible changes between “light” and “dark” conformations in response to different lights to control a recombinant protein release from PEG hydrogels spatiotemporally (Hammer et al., 2020/07). In a recent study, both nitrobenzyl and coumarin were used for photolabile crosslinks, and subsequently, on-demand and tunable dual wavelength release of antibody was achieved (Figure 1G) (LeValley et al.eng, 2020).
5 Photothermal reaction-based drug delivery systems
Photothermal therapy-based drug delivery system includes a photothermal agent, which is able to generate heat through light irradiation. The heat energy is then used to trigger reversible structure changes of thermal-responsive hydrogels, subsequently cause drug release from the system (Pan et al., 2021/10). Some commonly used photothermal agents include both inorganic nanoparticles and organic compounds, such as rare metal nanostructures and black phosphorus (BP) nanoparticles, which have little phototoxicity. These photothermal agents have minimized damage to cells and good penetration for high efficiency, and thus can provide safe thermal effects for controlled drug release (Zhang et al., 2015; Zhu et al., 2015; Wang et al., 2016; Yang et al., 2018). Commonly used thermal-responsive hydrogels include poly(N-isopropylacrylamide) (PNIPAAm), thermosensitive PEG analogs and thermosensitive elastin peptides (ELPs) (Ward and Georgiou, 2011). Because high temperature can lead to cell necrosis, the parameters of applied light irradiation should be carefully selected to avoid thermal damage to surrounding areas of targeted cells or tissues (Yang et al.eng, 2017). For this reason, NIR light is widely used to initiate photothermal effects because of its low energy and deep penetration properties. The drug release parameters can be accurately controlled by light duration and intensity, concentration of photothermal agents, and hydrogel composition (Merino et al., 2015).
BP nanoparticles are one of the most popular photothermal agents. Qiu et al. developed a low–melting-point agarose drug delivery nanostructure containing BP. After injected into cancer tissue, the hydrogel experiences a phase transition to gel states at body temperature. Under NIR irradiation, the hydrogel entered in a melting state which caused drug release from its matrix. Additionally, the release rate is able to be accurately controlled (Qiu et al., 2018). The system demonstrated a high therapeutic efficacy for cancers, and it is harmless and degradable in vivo. In another study, an injectable, NIR-II light-modulated and thermosensitive hydrogel is synthesized through supramolecular self-assembly of a conjugated polymer and alpha-cyclodextrin. This hydrogel intrinsically features NIR responsive characteristics and thermo-responsive properties (Figure 1H) (Ruan et al., 2019).
Rare metals are also widely used as photothermal agents. In one study, platinum nanoparticles were integrated into a NIR light-responsive hydrogel consisting of αCD and PEG-modified dendrimer. Under NIR irradiation, this hydrogel underwent a disruption to release the encapsulated drugs in a controlled manner via the irradiation time (Wang et al., 2016). Platinum nanoparticle has a very good photothermal conversion efficiency and biocompatibility; thus, it is frequently used with hydrogels for drug delivery. In another similar study, aldehyde-modified dextran hydrogel containing dendrimer-encapsulated platinum nanoparticles were developed via imine bond formation. The hydrogel exhibited excellent biocompatibility, photothermal effect and biodegradable properties. It can stay in tumors for days to allow repeated drug release, resulting in tumor regression (Li et al., 2018b).
For a study using organic compounds as the photothermal agent, one type of PNIPAM hydrogel has been synthesized with protoporphyrin IX or pheophorbide as photothermal agents, which are covalently conjugated to the polymer chains. The hydrogels showed great biocompatibility with more than 90% cell viability even at very high photosensitizer concentration suggesting the hydrogels can be applied for photothermal therapy (Belali et al.eng, 2018). In another study, the authors designed a novel type of dynamic-covalent hydrogel (GelPV-DOX-DBNP) for combined chemical and photothermal therapy of cancers. Anticancer drug DOX and photosensitizer perylene diimide zwitterionic polymer (PDS) as well as reductant ascorbic acid (Vc) were encapsulated. Under 600 nm light irradiation, PDS and VC can turn oxygen to hydrogen and cause degradation of hydrogel, subsequently lead to DOX and DBNP release from hydrogel. DBNPs are able to generate heat under NIR irradiation, making the system a useful drug delivery platform (Sun et al.eng, 2020). Cao et al. developed a chemo-photodynamic therapy system using ROS sensitive structure and successfully used for cancer treatment (Figure 1I) (Cao et al., 2018).
6 Light-induced multi-stimuli responsive drug delivery systems
Multi-stimuli responsive hydrogels have been used in a variety of physiological or pathological conditions. Besides responding to light, hydrogels can also be designed to respond to pH, magnetic field and reductant etc (Sharifzadeh and Hosseinkhani, 2017; Pham et al., 2020). In one study, hydrogels that can respond to both pH and NIR is designed to release adamantane-modified doxorubicin (DOX) prodrug using N-isopropylacrylamide (NIPAm) and βCD-based hydrogel. The pH-responsive release of DOX from the nanocomposite hydrogel was observed owing to the cleavage of acid-labile hydrazone bond between DOX and the adamantyl group in acidic environment. NIR irradiation led to accelerated release of DOX from hydrogels because of photothermal effects. The hydrogel can respond to both pH and NIR light and speed up drug release rate in a controlled manner (Xu et al.eng, 2017). In another study combining light and magnetic field, the authors designed a temperature-responsive PNIPAm hydrogel microfibers with controlled shapes and sizes. Then they fabricated light-responsive materials by incorporating photothermal magnetic nanoparticles within the PNIPAm microfibers. The magnetic nanoparticles were incorporated into the PNIPAm microfibers and created heat when subjected to visible light exposure. Volume changes of the PNIPAm hydrogel can be induced by both light irradiation and temperature, suggesting its potential use for drug delivery (Lim et al., 2015). Shao et al. developed a PEG based nanocarrier with light and redox dual responsive properties for cancer therapy. The system possesses good drug loading capacity and stability, and showed preferred tumor accumulation and the prolonged tumor residency by in vivo and ex vivo experiments (Shao et al., 2014). In another study, photo, pH and redox multi-responsive nanogels were developed for drug delivery and fluorescence cell imaging (Chen et al., 2017) (Figures 1J, K). Multi-stimuli responsive hydrogels are multi-functional, and suitable for multi-step drug delivery systems especially for complicated in vivo studies.
7 Discussion
Light is a powerful trigger for controlled drug delivery systems. Intensity, spatial and temporal control of light allows excellent manipulation of therapeutic agents in comparison with other physical, chemical, and biological stimuli (Chen et al., 2020). So far, much progress has been made in developing of innovative light responsive hydrogel drug delivery systems with both breadth and depth. But translating these studies into clinical applications still poses a significant challenge. Major issues need to be addressed includes: 1) most tumors are deep within the body, and it is difficult to deliver drugs to these locations. 2) the in vivo biological conditions of human are complex, therapeutic effects of drugs are low comparing in vitro or animal studies. 3) biomaterials of the drug delivery system, including hydrogel, photosensitizer, drug carrier and other components, may accumulate within human body after long-term administration. 4) most light induced drug delivery systems are irreversible, which made drug release inconsistent during the process. 5) non-specific photoreactions in normal tissues need to be considered. 6) photosensitizers are still active in dark conditions, and thus stability of loaded drugs cannot be maintained especially for long-term use.
Considering above issues, potential future developments may include: 1) designing photosensitizers responsive to low energy light source with fast response, high efficacy and deep tissue penetration (Li et al., 2019). Low energy lights, such as NIR, also cause less non-specific phototoxicity in normal tissues. 2) characterizing in vivo biological conditions, such as pH, temperature, redox and enzymatic reactions. These stimuli should be used together with light for drug release. 3) developing highly biocompatible materials for drug delivery systems. Natural biomaterials that mimic human tissues may be promising. 4) combing photoisomerization with other reactions to design reversible systems. Light-responsive proteins may have wider applications for this purpose (Tao et al., 2020). 5) besides using low energy light, monitoring real time drug distribution within tissue is another way minimize non-specific photoreactions. 6) developing stable photosensitizers for long-term stable drug release. Additionally, combining two or more independent photo-induced reactions into one drug delivery system is appealing for clinical use (LeValley et al.eng, 2020).
Author contributions
YX wrote the manuscript. BZ and WY provided supports.
Funding
The research was supported grant #11672078 from the National Natural Science Foundation of China (NSFC) and grant #NTF21014 from Shantou University to YX.
Conflict of interest
The authors declare that the research was conducted in the absence of any commercial or financial relationships that could be construed as a potential conflict of interest.
Publisher’s note
All claims expressed in this article are solely those of the authors and do not necessarily represent those of their affiliated organizations, or those of the publisher, the editors and the reviewers. Any product that may be evaluated in this article, or claim that may be made by its manufacturer, is not guaranteed or endorsed by the publisher.
References
Arrizabalaga, J. H., Smallcomb, M., Abu-Laban, M., Liu, Y., Yeingst, T. J., Dhawan, A., et al. (2022). “Ultrasound-responsive hydrogels for on-demand protein release,”. Editor eng, 5, 3212–3218.ACS Appl. bio Mater. 7. doi:10.1021/acsabm.2c00192
Belali, S., Savoie, H., O’Brien, J. M., Cafolla, A. A., O’Connell, B., Karimi, A. R., et al. (2018). “Synthesis and characterization of temperature-sensitive and chemically cross-linked poly( N-isopropylacrylamide)/Photosensitizer hydrogels for applications in photodynamic therapy,”. Editor eng, 19, 1592–1601.Biomacromolecules5. doi:10.1021/acs.biomac.8b00293
Bordbar-Khiabani, A., and Gasik, M. (2022). Smart hydrogels for advanced drug delivery systems. Int. J. Mol. Sci. 23 (7), 3665. in eng. doi:10.3390/ijms23073665
Bustamante-Torres, M., Romero-Fierro, D., Arcentales-Vera, B., Palomino, K., Magaña, H., Bucio, E., et al. (2021). Hydrogels classification according to the physical or chemical interactions and as stimuli-sensitive materials. Gels 7 (4), 182. doi:10.3390/gels7040182
Cao, Z. Y., Sun, C., Lu, Z., Yao, Z., Wang, J., et al. (2018). ROS-sensitive polymeric nanocarriers with red light-activated size shrinkage for remotely controlled drug release. Chem. Mat. 30 (2), 517–525. doi:10.1021/acs.chemmater.7b04751
Chatterjee, S., Hui, P. C., Kan, C. W., and Wang, W. (2019). Dual-responsive (pH/temperature) Pluronic F-127 hydrogel drug delivery system for textile-based transdermal therapy. Sci. Rep. 9 (1), 11658. (in eng). doi:10.1038/s41598-019-48254-6
Chen, D., Chen, C., Huang, C., Chen, T., and Liu, Z. (2020). Injectable hydrogel for NIR-II photo-thermal tumor therapy and dihydroartemisinin-mediated chemodynamic therapy. Front. Chem. 8, 251. eng. doi:10.3389/fchem.2020.00251
Chen, S., Bian, Q., Wang, P., Zheng, X., Lv, L., Dang, Z., et al. (2017). Photo, pH and redox multi-responsive nanogels for drug delivery and fluorescence cell imaging. Polym. Chem. 8 (39), 6150–6157. doi:10.1039/c7py01424d
Chen, X., et al. (2016). “Light-induced hydrogel based on tumor-targeting mesoporous silica nanoparticles as a theranostic platform for sustained cancer treatment,”. Editor eng, 8, 15857–15863.ACS Appl. Mater. interfaces25
Cheng, Y. E., Wu, I. E., Chen, Y. C., and Chu, I. M. (2022). Thermo-sensitive mPEG-PA-PLL hydrogel for drug release of calcitonin. Gels 8 (5), 282. (in eng). doi:10.3390/gels8050282
Chiang, C. Y., and Chu, C. C. (2015). Synthesis of photoresponsive hybrid alginate hydrogel with photo-controlled release behavior. Carbohydr. Polym. 119, 18–25. (in eng). doi:10.1016/j.carbpol.2014.11.043
Claaßen, C., Claaßen, M. H., Gohl, F., Tovar, G. E. M., Borchers, K., and Southan, A. (2018). “Photoinduced cleavage and hydrolysis of o-nitrobenzyl linker and covalent linker immobilization in gelatin methacryloyl hydrogels,”. Editor eng, 18, e1800104.Macromol. Biosci. 9
Ebara, M., Kotsuchibashi, Y., Uto, K., Aoyagi, T., Kim, Y. J., Narain, R., et al. (2014). “Smart hydrogels,” in Smart biomaterials (Tokyo, Japan: Springer Japan), 9–65.
Ghani, M., Heiskanen, A., Kajtez, J., Rezaei, B., Larsen, N. B., Thomsen, P., et al. (2021/01/27 2021). On-demand reversible UV-triggered interpenetrating polymer network-based drug delivery system using the spiropyran–merocyanine hydrophobicity switch. ACS Appl. Mat. Interfaces 13 (3), 3591–3604. doi:10.1021/acsami.0c19081
Gil, E. S., and Hudson, S. M. (2004). Stimuli-reponsive polymers and their bioconjugates. Prog. Polym. Sci. 29 (12), 1173–1222. doi:10.1016/j.progpolymsci.2004.08.003
Hammer, J. A., Ruta, A., and West, J. L. (2020). Using tools from optogenetics to create light-responsive biomaterials: LOVTRAP-PEG hydrogels for dynamic peptide immobilization. Ann. Biomed. Eng. 48 (7), 1885–1894. doi:10.1007/s10439-019-02407-w
Iwaso, K., Takashima, Y., and Harada, A. (2016). Fast response dry-type artificial molecular muscles with [c2]daisy chains. Nat. Chem. 8 (6), 625–632. in eng. doi:10.1038/nchem.2513
Ji, W., Wu, Q., Han, X., Zhang, W., Wei, W., Chen, L., et al. (2020). “Photosensitive hydrogels: From structure, mechanisms, design to bioapplications,”. Editor eng, 63, 1813–1828.Sci. China. Life Sci. 12 doi:10.1007/s11427-019-1710-8
Jiang, L., and Wen, L. (2015). “2 - photonic sensitive switchable materials,” in Biophotonics for medical applications, I. Meglinski (Sawston, UK: Woodhead Publishing), 25–51. doi:10.1016/B978-0-85709-662-3.00002-6
LeValley, P. J., Sutherland, B. P., Jaje, J., Gibbs, S., Jones, R. M., Gala, R. P., et al. (2020). “On-demand and tunable dual wavelength release of antibody using light-responsive hydrogels,”. Editor eng, 3, 6944–6958.ACS Appl. bio Mater. 10. doi:10.1021/acsabm.0c00823
Li, C., Iscen, A., Palmer, L. C., Schatz, G. C., and Stupp, S. I. (2020). Light-driven expansion of spiropyran hydrogels. J. Am. Chem. Soc. 142 (18), 8447–8453. doi:10.1021/jacs.0c02201
Li, L., Scheiger, J. M., and Levkin, P. A. (2019). Design and applications of photoresponsive hydrogels. Adv. Mat. 31 (26), 1807333. doi:10.1002/adma.201807333
Li, L., Wang, C., Huang, Q., Xiao, J., Zhang, Q., and Cheng, Y. (2018). A degradable hydrogel formed by dendrimer-encapsulated platinum nanoparticles and oxidized dextran for repeated photothermal cancer therapy. J. Mat. Chem. B 6 (16), 2474–2480. doi:10.1039/c8tb00091c
Li, X., and Su, X. (2018). “Multifunctional smart hydrogels: Potential in tissue engineering and cancer therapy,”. Editor eng, 6, 4714–4730.J. Mater. Chem. B 29, doi:10.1039/C8TB01078A
Liang, J., Liu, J., Jin, X., Yao, S., Chen, B., Huang, Q., et al. (2020). “Versatile nanoplatform loaded with doxorubicin and graphene quantum dots/methylene blue for drug delivery and chemophotothermal/photodynamic synergetic cancer therapy,”. Editor eng, 3, 7122–7132.ACS Appl. bio Mater.10. doi:10.1021/acsabm.0c00942
Liese, J., and Hampp, N. A. (2011). Synthesis and photocleavage of a new polymerizable [2+2] hetero dimer for phototriggered drug delivery. J. Photochem. Photobiol. A Chem. 219 (2), 228–234. doi:10.1016/j.jphotochem.2011.02.021
Lim, D., Lee, E., Kim, H., Park, S., Baek, S., and Yoon, J. (2015). Multi stimuli-responsive hydrogel microfibers containing magnetite nanoparticles prepared using microcapillary devices. Soft matter 11 (8), 1606–1613. doi:10.1039/C4SM02564D
Linsley, C. S., and Wu, B. M. (2017). “Recent advances in light-responsive on-demand drug-delivery systems,”. Editor eng, 8, 89–107.Ther. Deliv. 2, doi:10.4155/tde-2016-0060
Merino, S., Martín, C., Kostarelos, K., Prato, M., and Vázquez, E. (2015). Nanocomposite hydrogels: 3D polymer-nanoparticle synergies for on-demand drug delivery. ACS Nano 9 (5), 4686–4697. eng. doi:10.1021/acsnano.5b01433
Miranda, D., and Lovell, J. F. (2016). Mechanisms of light-induced liposome permeabilization. Bioeng. Transl. Med. 1 (3), 267–276. (in eng). doi:10.1002/btm2.10032
Nehls, E. M., Rosales, A. M., and Anseth, K. S. (2016). Enhanced user-control of small molecule drug release from a poly(ethylene glycol) hydrogel via azobenzene/cyclodextrin complex tethers. J. Mat. Chem. B 4 (6), 1035–1039. eng. doi:10.1039/c5tb02004b)
Pan, P., Svirskis, D., Rees, S. W. P., Barker, D., Waterhouse, G. I. N., and Wu, Z. (2021). Photosensitive drug delivery systems for cancer therapy: Mechanisms and applications. J. Control. Release 338, 446–461. doi:10.1016/j.jconrel.2021.08.053
Peng, K., Tomatsu, I., van den Broek, B., Cui, C., Korobko, A. V., van Noort, J., et al. (2011). Dextran based photodegradable hydrogels formed via a Michael addition. Soft matter 7 (10), 4881–4887. doi:10.1039/c1sm05291h
Pham, S. H., Choi, Y., and Choi, J. (2020). Stimuli-responsive nanomaterials for application in antitumor therapy and drug delivery. Pharmaceutics 12 (7), 630. doi:10.3390/pharmaceutics12070630
Pokharel, M., and Park, K. (2022). Light mediated drug delivery systems: A review. J. drug Target. 30 (4), 368–380. in eng. doi:10.1080/1061186x.2021.2005610
Pourjavadi, A., Bagherifard, M., and Doroudian, M. (2020). Synthesis of micelles based on chitosan functionalized with gold nanorods as a light sensitive drug delivery vehicle. Int. J. Biol. Macromol. 149, 809–818. in eng. doi:10.1016/j.ijbiomac.2020.01.162
Qiu, M., Wang, D., Liang, W., Liu, L., Zhang, Y., Chen, X., et al. (2018). Novel concept of the smart NIR-light–controlled drug release of black phosphorus nanostructure for cancer therapy. Proc. Natl. Acad. Sci. U. S. A. 115 (3), 501–506. doi:10.1073/pnas.1714421115
Rapp, T. L., and Dmochowski, I. J. (2019). Ruthenium-cross-linked hydrogels for rapid, visible-light protein release. Methods Enzymol. 624, 151–166. (in eng). doi:10.1016/bs.mie.2019.04.017
Rastogi, S. K., Anderson, H. E., Lamas, J., Barret, S., Cantu, T., Zauscher, S., et al. (2018). “Enhanced release of molecules upon ultraviolet (UV) light irradiation from photoresponsive hydrogels prepared from bifunctional azobenzene and four-arm poly(ethylene glycol),”. Editor eng, 10, 30071–30080.ACS Appl. Mater. interfaces36. doi:10.1016/bs.mie.2019.04.017
Rizzo, F., and Kehr, N. S. (2021). “Recent advances in injectable hydrogels for controlled and local drug delivery,”. Editor eng, 10, e2001341.Adv. Healthc. Mater. 1, doi:10.1021/acsami.6b16183
Rosales, A. M., Rodell, C. B., Chen, M. H., Morrow, M. G., Anseth, K. S., and Burdick, J. A. (2018). “Reversible control of network properties in azobenzene-containing hyaluronic acid-based hydrogels,”. Editor eng, 29, 905–913.Bioconjugate Chem.4, doi:10.1021/acs.bioconjchem.7b00802
Ruan, C. P., Liu, C., Hu, H., Guo, X. L., Jiang, B. P., Liang, H., et al. (2019). NIR-II light-modulated thermosensitive hydrogel for light-triggered cisplatin release and repeatable chemo-photothermal therapy. Chem. Sci. 10 (17), 4699–4706. doi:10.1039/c9sc00375d
Shadish, J. A., and DeForest, C. A. (2020). Site-selective protein modification: From functionalized proteins to functional biomaterials. Matter 2 (1), 50–77. doi:10.1016/j.matt.2019.11.011
Shao, Y., Shi, C., Xu, G., Guo, D., and Luo, J. (2014). “Photo and redox dual responsive reversibly cross-linked nanocarrier for efficient tumor-targeted drug delivery,”. Editor eng, 6, 10381–10392.ACS Appl. Mater. interfaces 13. doi:10.1021/am501913m
Sharifzadeh, G., and Hosseinkhani, H. (2017). Biomolecule-responsive hydrogels in medicine. Adv. Healthc. Mat. 6 (24), 1700801. doi:10.1002/adhm.201700801
Sridhar, B. V., Janczy, J. R., Hatlevik, Ø., Wolfson, G., Anseth, K. S., and Tibbitt, M. W. (2018/03/12 2018). Thermal stabilization of biologics with photoresponsive hydrogels. Biomacromolecules 19 (3), 740–747. doi:10.1021/acs.biomac.7b01507
Sun, P., Huang, T., Wang, X., Wang, G., Liu, Z., Chen, G., et al. (2020). “Dynamic-covalent hydrogel with NIR-triggered drug delivery for localized chemo-photothermal combination therapy,”. Editor eng, 21, 556–565.Biomacromolecules 2. doi:10.1021/acs.biomac.9b01290
Tao, B., Lin, C., Deng, Y., Yuan, Z., Shen, X., Chen, M., et al. (2019). Copper-nanoparticle-embedded hydrogel for killing bacteria and promoting wound healing with photothermal therapy. J. Mat. Chem. B 7 (15), 2534–2548. in eng. doi:10.1039/c8tb03272f
Tao, Y., Chan, H. F., Shi, B., Li, M., and Leong, K. W. (2020). “Light: A magical tool for controlled drug delivery,”. Editor eng, 30.Adv. Funct. Mater. 49. doi:10.1002/adfm.202005029
Tomatsu, I., Peng, K., and Kros, A. (2011). Photoresponsive hydrogels for biomedical applications. Adv. drug Deliv. Rev. 63 (14-15), 1257–1266. (in eng). doi:10.1016/j.addr.2011.06.009
Tong, R., Hemmati, H. D., Langer, R., and Kohane, D. S. (2012). “Photoswitchable nanoparticles for triggered tissue penetration and drug delivery,”. Editor eng, 134, 8848–8855.)J. Am. Chem. Soc.21
Ullah, F., Othman, M. B., Javed, F., Ahmad, Z., and Md Akil, H. (2015). Classification, processing and application of hydrogels: A review. Mater. Sci. Eng. C 57, 414–433. (in eng). doi:10.1016/j.msec.2015.07.053
Wang, C., Yang, P., Li, Y., Liu, C., Wei, Q., Yang, L., et al. (2020). S, S-Tetrazine-Based hydrogels with visible light cleavable properties for on-demand anticancer drug delivery. Research 2020, 6563091–6563111. doi:10.34133/2020/6563091
Wang, D., Wagner, M., Butt, H. J., and Wu, S. (2015). Supramolecular hydrogels constructed by red-light-responsive host-guest interactions for photo-controlled protein release in deep tissue. Soft matter 11 (38), 7656–7662. (in eng). doi:10.1039/c5sm01888a
Wang, X., Wang, C., Zhang, Q., and Cheng, Y. (2016). “Near infrared light-responsive and injectable supramolecular hydrogels for on-demand drug delivery,”. Editor eng, 52, 978–981.Chem. Commun. Camb. Engl.5. doi:10.1039/c5cc08391e
Ward, M. A., and Georgiou, T. K. (2011). Thermoresponsive polymers for biomedical applications. Polymers 3 (3), 1215–1242. doi:10.3390/polym3031215
Wei, X., Liu, C., Wang, Z., and Luo, Y. (2020). 3D printed core-shell hydrogel fiber scaffolds with NIR-triggered drug release for localized therapy of breast cancer. Int. J. Pharm. 580, 119219. in eng. doi:10.1016/j.ijpharm.2020.119219
Xiao, X., Hu, J., Wang, X., Huang, L., Chen, Y., Wang, W., et al. (2016). “A dual-functional supramolecular hydrogel based on a spiropyran-galactose conjugate for target-mediated and light-controlled delivery of microRNA into cells,”. Editor eng, 52, 12517–12520.Chem. Commun. Camb. Engl.84. doi:10.1039/c6cc07386g
Xu, X., Huang, Z., Huang, Z., Zhang, X., He, S., Sun, X., et al. (2017). “Injectable, NIR/pH-Responsive nanocomposite hydrogel as long-acting implant for chemophotothermal synergistic cancer therapy,”. Editor eng, 9, 20361–20375.ACS Appl. Mater. interfaces 24. doi:10.1039/c6cc07386g
Yan, B., Boyer, J.-C., Branda, N. R., and Zhao, Y. (2011/12/14 2011). Near-infrared light-triggered dissociation of block copolymer micelles using upconverting nanoparticles. J. Am. Chem. Soc. 133 (49), 19714–19717. doi:10.1021/ja209793b
Yan, B., Boyer, J.-C., Habault, D., Branda, N. R., and Zhao, Y. (2012/10/10 2012). Near infrared light triggered release of biomacromolecules from hydrogels loaded with upconversion nanoparticles. J. Am. Chem. Soc. 134 (40), 16558–16561. doi:10.1021/ja308876j
Yang, G., Wan, X., Gu, Z., Zeng, X., and Tang, J. (2018). Near infrared photothermal-responsive poly(vinyl alcohol)/black phosphorus composite hydrogels with excellent on-demand drug release capacity. J. Mat. Chem. B 106 (11), 1622–1632. doi:10.1039/c7tb03090h
Yang, Y., Zhu, W., Dong, Z., Chao, Y., Xu, L, Chen, M., et al. (2017). “1D coordination polymer nanofibers for low-temperature photothermal therapy,”. Editor eng, 29.Adv. Mater. Deerf. Beach, Fla.)40. doi:10.1002/adma.201703588
Yang, Y., Long, K., Wang, Y., Li, L., Shi, J., Liu, J., et al. (2022). NIR light-triggered quantitative pulsed drug release. Adv. Healthc. Mat. 11 (8), e2102362. (in eng). doi:10.1002/adhm.202102362
Yerneni, S. S., Lathwal, S., Cuthbert, J., Kapil, K., Szczepaniak, G., Jeong, J., et al. (2022). “Controlled release of exosomes using atom transfer radical polymerization-based hydrogels,”. Editor eng, 23, 1713–1722.Biomacromolecules4. doi:10.1021/acs.biomac.1c01636
Yu, T., Tong, L., Ao, Y., Zhang, G., Liu, Y., and Zhang, H. (2020). “NIR triggered PLGA coated Au-TiO(2) core loaded CPT-11 nanoparticles for human papillary thyroid carcinoma therapy,”. Editor eng, 27, 855–863.Drug Deliv.1. doi:10.1080/10717544.2020.1775723
Yuan, Y., Wang, Z., Cai, P., Liu, J., Liao, L. D., Hong, M., et al. (2015). Conjugated polymer and drug co-encapsulated nanoparticles for chemo- and photo-thermal combination therapy with two-photon regulated fast drug release. Nanoscale 7 (7), 3067–3076. in eng. doi:10.1039/c4nr06420h
Zhang, H., Zhu, X., Ji, Y., Jiao, X., Chen, Q., Hou, L., et al. (2015). Near-infrared-triggered in situ hybrid hydrogel system for synergistic cancer therapy. J. Mat. Chem. B 3 (30), 6310–6326. doi:10.1039/C5TB00904A
Zhang, P., Li, M., Xiao, C., and Chen, X. (2021). “Stimuli-responsive polypeptides for controlled drug delivery,”. Editor eng, 57, 9489–9503.Chem. Commun. Camb. Engl.75. doi:10.1039/d1cc04053g
Keywords: hydrogel, light responsive, drug delivery, photosensitizer, programmed release
Citation: Xing Y, Zeng B and Yang W (2022) Light responsive hydrogels for controlled drug delivery. Front. Bioeng. Biotechnol. 10:1075670. doi: 10.3389/fbioe.2022.1075670
Received: 20 October 2022; Accepted: 05 December 2022;
Published: 16 December 2022.
Edited by:
Yasser Zare, Motamed Cancer Institute, IranReviewed by:
Amir Rostami, Persian Gulf University, IranCopyright © 2022 Xing, Zeng and Yang. This is an open-access article distributed under the terms of the Creative Commons Attribution License (CC BY). The use, distribution or reproduction in other forums is permitted, provided the original author(s) and the copyright owner(s) are credited and that the original publication in this journal is cited, in accordance with accepted academic practice. No use, distribution or reproduction is permitted which does not comply with these terms.
*Correspondence: Yanghui Xing, yhxing@stu.edu.cn