- 1Lab for Aging Research, National Clinical Research Center for Geriatrics, West China Hospital, Sichuan University, Chengdu, China
- 2State Key Laboratory of Oral Diseases, National Clinical Research Center for Oral Diseases, Department of Orthodontics, West China Hospital of Stomatology, Sichuan University, Chengdu, China
- 3Department of Hepatobiliary Surgery and Fujian Institute of Hepatobiliary Surgery, Fujian Medical University Union Hospital, Fujian Medical University, Fuzhou, China
Temporomandibular joint osteoarthritis (TMJOA) is a debilitating degenerative disease with high incidence, deteriorating quality of patient life. Currently, due to ambiguous etiology, the traditional clinical strategies of TMJOA emphasize on symptomatic treatments such as pain relief and inflammation alleviation, which are unable to halt or reverse the destruction of cartilage or subchondral bone. A number of studies have suggested the potential application prospect of mesenchymal stem cells (MSCs)-based therapy in TMJOA and other cartilage injury. Worthy of note, exosomes are increasingly being considered the principal efficacious agent of MSC secretions for TMJOA management. The extensive study of exosomes (derived from MSCs, synoviocytes, chondrocytes or adipose tissue et al.) on arthritis recently, has indicated exosomes and their specific miRNA components to be potential therapeutic agents for TMJOA. In this review, we aim to systematically summarize therapeutic properties and underlying mechanisms of MSCs and exosomes from different sources in TMJOA, also analyze and discuss the approaches to optimization, challenges, and prospects of exosome-based therapeutic strategy.
Introduction
Temporomandibular joint osteoarthritis (TMJOA) is a degenerative temporomandibular arthropathy characterized by progressive cartilage degeneration, abnormal subchondral bone remodeling and obvious synovitis (Scrivani et al., 2008; Toller, 1973). Due to the severe concomitant symptoms such as difficulties in chewing, acute or chronic pain, and even maxillofacial deformities, it severely deteriorates the quality of patient life and leads to the large resultant socioeconomic burden. Joint cartilage is composed of chondrocytes and extracellular matrix like collagen fibers, proteoglycans, and hyaluronic acid. Feature of avascular structure of cartilage is detrimental to the exchange of available signaling molecules, migration of progenitor cells, and adequate supply of nutrients and oxygen, resulting in the inability of damaged cartilage tissue to regenerate effectively (Chen et al., 2020). Because of the limited self-healing ability of cartilage, it has become one of the most difficult joint diseases to treat. Compared with other joints in the body, temporomandibular joint has its own characteristics (Macedo et al., 2017; David and Roberts, 2018). Besides, the layer of hyaline cartilage covering generalized joints mainly contained type II collagen, but the cartilage of TMJ is fibrocartilage, which is a kind of cartilage composed of both type I collagen and type II collagen. Because of the structural differences, there will be some differences in treatment strategies when the disease occurs. The cartilage of TMJ has better multidirectional bearing capacity and more dense fibers, which are not easy to degrade and are less affected by aging (Schwartz et al., 2015; Chandrasekaran et al., 2021). However, when it is damaged, the difficulty of restoring normal structure (Kuo et al., 2011). Numerous studies have confirmed that it is a pathological state affected by multiple factors (Figure 1). Excessive mechanical stress is a major factor leading to cartilage rupture in TMJ (Su et al., 2014; Huang et al., 2021; Ootake et al., 2021). Uneven stress distribution in TMJ caused by occlusal disorder was reported to induce the hyperactivity of osteoclasts in subchondral bone. Researchers have demonstrated that inflammation is one of the risk factors of TMJOA (Li et al., 2019a; Li et al., 2019b; Luo et al., 2019; Lei et al., 2022). Liu detected synovial fluid from TMJOA patients and found that the level of inflammatory cytokines was significantly increased.16 Moreover, genetic factors and age-related reduction of host-adaptive capacity are also vital in TMJOA (Xu et al., 2003; Yamaguchi et al., 2014). It is because of the specificality of TMJ structure and the ambiguity of etiological mechanism that the treatment of TMJOA has been set up a huge obstacle.
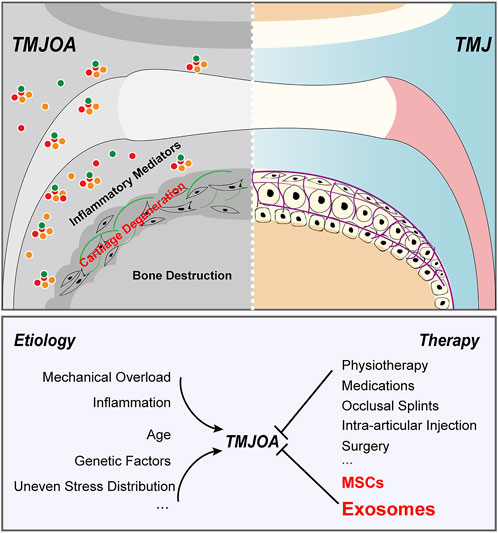
FIGURE 1. Pathogenesis and treatment strategies of TMJOA. TMJOA is a degenerative temporomandibular arthropathy characterized by progressive cartilage degeneration, abnormal subchondral bone remodeling and obvious synovitis. It is a pathological state affected by multiple factors. Traditional clinical strategies of TMJOA emphasize on symptomatic treatments and are unable to halt or reverse the destruction of cartilage or subchondral bone. MSCs and exosomes are highly promising for TMJOA alleviation.
To date, treatment strategies for TMJAO are symptomatic and limited (Figure 1), only to reduce inflammation and relieve pain (Thie et al., 2001). Traditional clinical treatments can stop the progression of the disease to some extent, but they cannot actively restore degraded cartilage or damaged subchondral bone (Derwich et al., 2021; Liu Q. et al., 2022; Matheus et al., 2022). Novel radical therapies for osteoarthritis are urgently required. In recent years, cell-based disease treatment strategies have raised considerable concerns, especially mesenchymal stem cells (MSCs) -based therapies (Matheus et al., 2022). Abundant native MSCs are present in multiple niches in the joint, including subchondral bone, synovial fluid, and adipose tissue. In the last decade, increasing evidence has suggested that MSCs have great potential in the treatment of osteoarthritis. BMSCs have suggested promising therapeutic efficacy for TMJ cartilage repair (Ciocca et al., 2013). Although the role of MSCs in the field of disease treatment cannot be ignored, we still need to comprehensively understand its non-negligible bottlenecks as cell therapy strategies. The host exhibited immunological tolerance toward implanted MSCs and had a potential risk for malignancies, which might also pose a risk to immunological cells for controlling an inflammatory milieu (Lalu et al., 2012). Therefore, it is inevitable to find an alternative approach to solve the dilemma faced by MSC-based therapy. Numerous studies have summarized the bio-effect of MSCs is increasingly attributed to paracrine signaling to transfer its cargo to the body, among which exosomes are a vital carrier for message in many biological and pathological processes. Exosomes provide new perspectives for the development of cell-free and ready-to-use therapy for treatment of cartilage lesions and TMJOA.
Herein, the present review was aimed at discussing the therapeutic potential and corresponding mechanism of MSCs, the biological properties of exosomes derived from diversified cell sources, and advances in our knowledge of their emerging roles in managing TMJOA. We also discussed the detailed exosome-based tissue engineering strategies of TMJOA therapy in the hope of providing inspiration for future investigations. Particularly, we proposed novel perspectives for the development and implementation of exosomes as a cell-free regenerative medicine therapeutic strategy for cartilage repair in TMJOA and discussed future opportunities and challenges in this exciting field.
Mechanisms of MSCs in the treatment of TMJOA
Since MSCs first discovery by Friedenstein (Friedenstein et al., 1982), they are commonly used in the treatment of various diseases, including TMJOA. We conducted a literature review and found that the application of MSCs in osteoarthritis was first reported in 1995, and more and more related research is being carried on, with over 89% of the published in the recent 10 years (Figure 2).
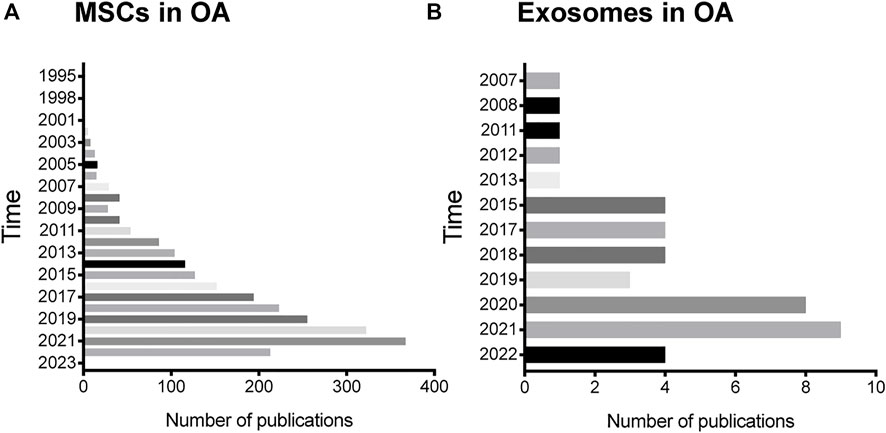
FIGURE 2. Status of MSCs and exosomes research in OA. (A) The annual number of publications related to MSCs research in OA in the past 27 years. (B) The annual number of publications related to exosomes research in OA in the past 15 years.
When TMJOA occurs, the dynamic balance between chondrocyte matrix anabolism and catabolism is disrupted, accelerating the progression of the disease (Weng et al., 2017). Zhang et al. (2017) reported BMSCs reversed the loss of cartilage matrix associated with osteoarthritis and enhanced scavenging activity of the degraded matrix in deep zone chondrocytes. Lu et al. (2015) also found that the implanted GFP-BMSCs differentiated into COL2-positive cells and relieved matrix degradation in TMJOA. It was indicated that human umbilical cord matrix-mesenchymal stem cells (hUCMSC) showed prominent cartilage protective effect and effective cartilage regeneration potential (Kim et al., 2019). Moreover, Maria revealed scaffolds loaded with dental pulp mesenchymal stem cells (DPSCs) effectively supported abundant fibrocartilaginous tissue formation. Besides, other MSCs, such as adipose-derived mesenchymal stem cells (ADMSCs) (Ahtiainen et al., 2013) and synovial fluid derived mesenchymal stem cells (SFDMSCs) (Koyama et al., 2011), have also been indicated to alleviate TMJOA by participating in cartilage matrix metabolism.
Uncoupled remodeling of subchondral bone is another pathological feature contributed to TMJOA (Jiao et al., 2011; Yang et al., 2014; Zheng et al., 2018; Ibrahim et al., 2019). Human exfoliated deciduous teeth stem cells (SHED) markedly improved surface smoothness and bone integrity of the destroyed condylar in TMJOA mice (Chen et al., 2013). Chen K investigated MSC-treated groups demonstrated pronounced micro-architectural changes of the subchondral bone (Tanaka et al., 2008). In addition, it was reported that the migration of BMSCs restored subchondral bone loss in mice with TMJOA (Lu et al., 2015).
Evidence has suggested that sustained inflammation is involved in the onset and progression of TMJOA (Liu W. et al., 2017). When stimulated, immune cells in inflammatory microenvironment release inflammatory factors to affect the matrix metabolism of chondrocytes to deteriorate TMJOA (Tanaka et al., 2008) and are also in close correlation with sensory neuron hyperexcitability to induce the pain of TMJOA (Magnano et al., 2007; Ou et al., 2021). Buul et al. found the decreased expression of IL-1β, MMP-1 and MMP-13 in synovial explants when cultured with MSCs conditioned medium (Van Buul et al., 2012). It was recently shown that BMSCs injection into the bilateral TMJ region significantly reversed high levels of TNF-α and IL-1β in TMJOA (Lu et al., 2015). This is further supported by Hyunjeong Kim’s study (Kim et al., 2019). The self-assembled peptide hydrogels accelerated tissue regeneration by anti-inflammatory modulation (Kim et al., 2016).
Inflammation and immunity go hand in hand (Bartholomew et al., 2002; Koliaraki et al., 2020; Lim et al., 2021; Pham et al., 2021). In the pathogenesis of inflammatory diseases, dysregulation of the host immuno-inflammatory response is one of the important predisposing factors (Theill et al., 2002; Hernández et al., 2011). Similarly, Monasterio proposed cytokines, CCLs and CCRs of the Th1/Th17/Th22 axis were involved in TMJOA pathogenesis (Monasterio et al., 2018). A large number of studies have shown that MSCs regulate innate and acquired immunity in the treatment of OA (Yu et al., 2016). Tang et al. (2021a) reported that hUCMSCs protected cartilage from injury by regulating the macrophages polarization and affecting the joint immune microenvironment, but notably, there was a stronger regulation ability of immune effector process in hUCMSCs-exosomes treatment group.
In recent years, although the efficacy of MSCs in treating TMJOA has been widely studied in animal studies and human clinical trials, in fact, the problems encountered in clinical application have been deeply troubling researchers (Table 1). Donor’s age affects the intrinsic activity and functionality of obtained cells (Kim et al., 2020). The lack of standardization for large-scale cell production results in inconsistent cell quality after expansion. Additionally, the senescence and dedifferentiation of cells during the expansion in vitro will also affect potential and increase the risk in the application (Siddappa et al., 2007). More cautiously, there is a potential of tumorigenicity (Le et al., 2012; Waterman et al., 2010). Moreover, the issue of cell storage is also a bottleneck of MSC-based strategy. Whether the biological activity of MSCs will be affected after repeated cryopreservation is a great question to be considered in future. It is noteworthy that there is a paradigm shift that, rather than direct differentiation to cells of the target tissue, the therapeutic efficacy of MSCs in tissue repair and regeneration is predominantly attributed to paracrine signaling, particularly exosomes (Mayourian et al., 2018; Li et al., 2019; Mori et al., 2019; Zhang et al., 2019; Zhou Q.-F. et al., 2020). Therefore, exosome-based therapeutic strategy of TMJOA may be a promising substitute for MSC-based therapy.
Characteristics of exosomes
It was not until 2006 that Ratajczak proposed for the first time that mRNA could be delivered by membrane-derived vesicles (MV) released from the surface of activated eucaryotic cells and exert positive effects on surrounding cells (Ratajczak et al., 2006). Exosome-mediated transfer of RNAs was suggested as a novel mechanism of genetic exchange between cells (Waterman et al., 2010), occurring within the microenvironment or at a distance by traffic of exosomes. Exosomes are the smallest in size ranging from 40 to 160 nm in diameter among the three main subcategories of extracellular vesicles (EVs). Exosomes of different cell origin carry their own various bioactive molecules, containing different types of proteins, DNAs, mRNAs, microRNAs, lipids, metabolites and so on. It is the diversity of contents that illustrates the diversity of exosome functions (Valadi et al., 2007). They are ubiquitously involved in the basic processes of innate and adaptive immunity and immune-mediated disease processes (Garikipati et al., 2018). It was shown that miR-21-5, as a lead cardioactive MSC-exosomal-microRNA, mediated effects on increasing engineered cardiac tissues contractility and was suggested as a specific molecular target for optimizing cardio-therapies (Mayourian et al., 2018). In recent years, increasing studies have been conducted on the application of exosomes in the treatment of neurological diseases (Budden et al., 2021; Xu et al., 2017; Rufino-Ramos et al., 2017). More attention should also be paid to bottlenecks in exosome treatment, including the limitation of increasing exosome production, the difficulty of analyzing the effective components of exosomes and the better improvement of the functions of the active component. Encouragingly, the problems of exosomes faced in the diseases therapy have been gradually handled via various biotechnology modifies. The composition and secretion of exosomes are affected by the environment and signals of donor cells, including hypoxia, heat, and pharmacological intervention (Pegtel et al., 2010; Fan et al., 2020). Therefore, changing the culture conditions of donor cells can meet the clinical needs of exosomes in treating diseases. Because exosomes are excellent carriers, the direct insertion of miRNA mimics or siRNAs into exosomes through electroporation (Ma et al., 2018) and electric pulses (Yang et al., 2020) has attracted the attention of many researchers. By modifying exosomes membrane through genetic manipulation strategy by biotechnology, exosomes can reach the target cells and tissues according to the predetermined route and play a more specific role (Kanki et al., 2011; Wang et al., 2018). The intersection between different cells exosomes and chondrocytes offered a new insight into the pathogenesis and treatment of degenerative joint diseases. Many studies have proposed that exosomes play an irreplaceable role in the treatment of TMJOA. In the following section, we summarized the current studies on the therapeutic effects of exosomes from various cells in TMJOA.
Functional mechanisms and potential therapeutics of exosomes in TMJOA
The different responses of recipient cells to exosomes are mainly due to the heterogeneity of exosomes, including their inconsistent expression of cell surface receptors and different contents. It means that exosomes from different cells have different effects on the same type of cell and the same exosome may also have inconsistent or even contradictory effects on different target cell types or target tissues66 (Table 2). More and more researchers attempt to obtain diversified exosomes and apply them in OA treatment to have a deeper understanding of the occurrence and development of OA and hope to find more novel targets in molecular mechanisms of TMJOA treatment (Figure 3). To date, an increasing amount of literature has indicated that exosomes from different sources (Figure 2), such as MSCs, chondrocytes, and synovial fluid in TMJ cavity, are reportedly important in the treatment of TMJOA. In recent years, researchers have focused on identifying effective constituents in exosomes, such as miRNA, for the treatment of TMJOA, with a view to obtain a more direct, effective, and targeted therapeutic strategy.
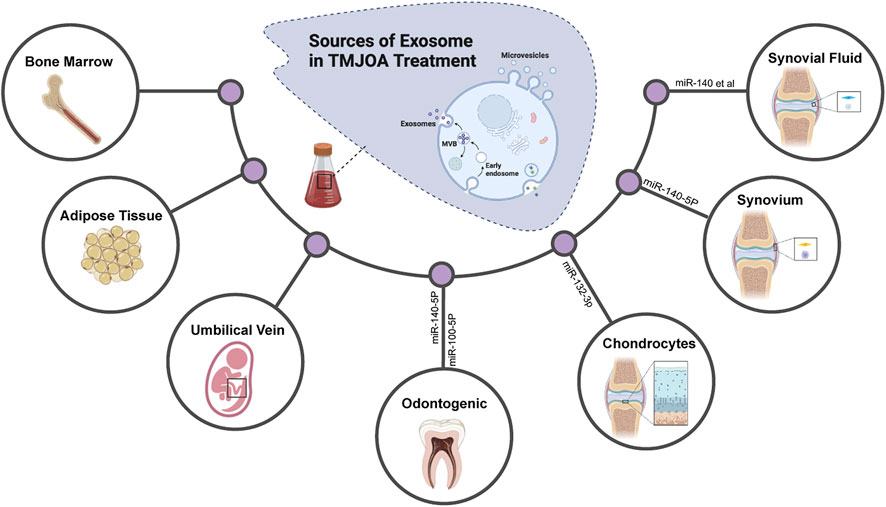
FIGURE 3. Exosomes derived from different tissues and cells are applied for TMJOA treatment. Diversified exosomes are obtained and applied in OA treatment to have a deeper understanding of the occurrence, development of OA, and to find more novel targets in molecular mechanisms of TMJOA treatment.
MSCs-derived exosomes
BMSCs have been used the earliest to treat various diseases because of their outstanding biological characteristics (Li et al., 2012; Li et al., 2020; Dubus et al., 2022). Certainly, BMSCs-derived exosomes have also been demonstrated to be ideal agents for the treatment of osteoarthritis. He Lei investigated BMSCs-exosome stimulation obviously reversed the inhibition effect of IL-1β on the proliferation and migration of chondrocytes, significantly upregulated the expression of COL2A and downregulated MMP13 in vitro and vivo (Armiñán et al., 2010). Although the previous studies were less homogenous due to problems with dose, injection frequency and management timing, one of the therapeutic functionalities of MSC-derived exosome is anti-inflammatory efficacy in promoting functional recovery of matrix metabolism homeostasis. After evaluation of the influences on injections of embryonic stem cell-derived-exosomes in TMJ-OA induced by monosodium iodoacetate (MIA), the underlying molecular mechanisms of exosome-mediated matrix homeostasis in TMJ injury repair and cartilage regeneration were clearly elucidated (Zhang et al., 2019). The obstacle of S-GAG synthesis induced by IL-1β was broken by the exosome treatment. Also, consistent with previous experiments, MSCs exosomes reduced inflammation by suppressing NO and MMP13. Together, MSCs derivative exosomes inhibit cartilage degeneration and TMJOA-induced pain by alleviating inflammation in the early stage, and then promote matrix proliferation and expression as well as the recovery of subchondral bone structure, and finally achieve the repair and regeneration of the overall TMJ.
In addition to alleviating inflammatory events in TMJOA, MSC-exosomes could also inhibit apoptosis of chondrocytes and activation of the immunity (Cosenza et al., 2017; He et al., 2020; Wang Y. et al., 2021). A study by Zhu revealed the exosomes from induced pluripotent stem cells (iPMSCs) or synovial membrane derived MSCs (SMMSCs) accelerated proliferation and migration of chondrocytes (Tang et al., 2021b). Notably, there was a proliferation promotion of chondrocytes in the co-culture studies of chondrocytes and MSCs (Zhu et al., 2017). Zhang found CD163+ cells of the cartilage overlying synovium in exosome-treated defect increased but CD86+ cells decreased, indicating higher M2 macrophages infiltration with a decline in M1 macrophages (Wu et al., 2011). Inflammatory cytokines, such as IL-1β, displayed a concomitant decrease like M1 macrophages. The senescence of chondrocytes appears generally during the progression of TMJOA (Clérigues et al., 2012; Zhang et al., 2018; Varela-Eirín et al., 2022). A wide range of evidence has shown that exosomes from ADMSCs declined the presence of phosphorylated histone H2AX, relieved DNA damage, restored the mitochondrial membrane changes and oxidative stress, and inhibited OA osteoblast senescence (Duarte, 2015). Additionally, numerous studies have indicated that there is a close relationship between autophagy and cartilage biology and the pathology of TMJOA (Almonte-Becerril et al., 2010; Lotz et al., 2011; Jeon and Im, 2017; Tofiño-Vian et al., 2017). It was demonstrated that infrapatellar fat pad (IPFP) MSCs-derived exosomes inhibited apoptosis and balanced the anabolic and catabolic processes of chondrocytes to prevent cartilage from damage by exosomal-miR100-5p-mediatied inhibition of mTOR-autophagy pathway (Ribeiro et al., 2016). The TMJ cavity is a highly complicated environment involving a variety of different cells. Undoubtedly, exosomes secreted by these cells play an important role in the regulation of microenvironment homeostasis.
Chondrocytes-derived exosomes
The chondrocyte is the only cell type of cartilage and is critical in the maintenance of cartilage homeostasis. The effect of primary chondrocytes-derived exosomes on TMJOA has been confirmed (Ni et al., 2019; Wu et al., 2019; Zheng et al., 2019; Liu S.-S. et al., 2022; Nikhil and Kumar, 2022). Liu noticed exosome-like structures in abnormal calcified cartilage together with the decrease of matrix Gla protein and the increase of tissue-nonspecific alkaline phosphatase, CD63 and pyrophosphatase/phosphodiesterase-1 in TMJOA (Ni et al., 2019). After local injection of the exosome inhibitor, the process of calcification was inhibited. They speculated it was a new way in preventing and treating TMJOA to inhibit degenerative chondrocyte-derived exosomes. Chondrocytes-derived exosomes positively affected proliferation of chondrocytes and exhibited significant wound closure promotion due to roles in intercell communication. During the repair of cartilage injury, bilayered cryoge and chondrocytes-derived exosomes had a synergistic effect (Liu Q. et al., 2022). Furthermore, Zheng investigated the proteomics of primary chondrocyte exosomes and found the 2409 proteins of exosomes were involved in mitochondrial damage or dysfunction and immune system process. They attributed the protective effects of chondrocyte-exosomes on osteoarthritis to mitochondrial dysfunction elimination and M1-proinflammatory macrophages infiltration decrease with a concomitant M2- anti-inflammatory macrophage increase in cartilage (Nikhil and Kumar, 2022). It could be inferred from Liu’ results that the exosomes released by articular chondrocytes inhibited catabolism and increased mRNA levels of ACAN and COL2A as a facilitator of cell communication (Zheng et al., 2019). Chondrogenic progenitor cells (CPCs) have MSC characteristics with strong potential of cartilage differentiation and self-renewal ability (Liu et al., 2020). EVs secreted by CPCs from MRL/MpJ superhealer mice (MRL-EVs) had shown superior therapeutic capability in attenuating OA compared with control mice-EVs. MRL-EVs played a vital role in stimulating the proliferation and migration of chondrocytes (Koelling et al., 2009). After miRNA-seq analysis of exosomes, AMPK signaling, regulation of autophagy, and insulin signaling were observed to be associated with differentially expressed miRNAs and miRNA 221-3p was highly enriched in MRL-EVs.
Synoviocytes-derived exosomes
Synovial inflammation is observed on magnetic resonance imaging of OA affected joints (Wang et al., 2020). There is increasing evidence that synovial inflammation is positively correlated with TMJOA severity (Roemer et al., 2010), and persistent low-grade synovial inflammation exacerbates cartilage damage (Raghu et al., 2017). Kato analyzed effects of synoviocytes-derived exosomes on chondrocytes and HUVECs (Kato et al., 2014). Compared with resting synoviocytes-derived exosomes, exosomes from IL-1β stimulated synoviocytes significantly promoted matrix catabolism and inhibited anabolism of chondrocytes. Migration and tube formation activity of HUVECs were improved. These findings indicated that SFCs exosomes represented a novel mechanism in the pathogenesis of osteoarthritis, which implied exosomes might be used as a therapeutic strategy for TMJOA. Dysregulated angiogenesis deteriorates the cartilage degradation, bone destruction and synovitis (Kato et al., 2014). Feng Yaping reported HMGB1 increased VEGF and HIF-1α in synovial fibroblasts of TMJOA and conditioned medium obtained from High-mobility group protein 1-treated TMJOA SFCs promoted the migration and tube formation of HUVECs (Chavakis et al., 2007). In addition, it was observed that synoviocytes-derived exosome-mediated cartilage repair was achieved by improvement in cell activity and migration ability as well as reduction of ECM degradation, of which synoviocytes-derived exosomal-lncRNA H19 suppressed the miR-106b-5p/TIMP2 axis (Feng et al., 2021). It was confirmed that the expression of miRNA-126-3p was sharply reduced in synovial fluid exosomes from OA patients. Exosomes derived from miR-126-3p-overexpressing synovial fibroblasts enhanced chondrocytes proliferation and suppressed chondrocytes apoptosis. What’s more, the exosomes significantly constrained the inflammation in chondrocytes by decreasing the IL-1β, IL-6, and TNF-α (Tan et al., 2020).
Synovial tissue maintains the basic composition and volume of synovial fluid. SFCs secrete synovial fluid, which in turn provides a low friction environment and nourishes surrounding tissues. Recently, many studies have focused on analyzing and comparing the synovial fluid derived exosomes differences between osteoarthritis patients and healthy people to find new molecular targets and related mechanisms for the treatment of osteoarthritis (Kolhe et al., 2017; Zhou et al., 2021). It has been proposed that miRNA contents differ between OA patients and healthy people. Moreover, there is a high gender-specific differential expression of miRNA in synovial fluid-derived exosomes in patients with OA (Zhou et al., 2021). Chondrocytes treated with OA-derived EVs had down-regulated expression of anabolic metabolism and elevated expression of catabolic metabolism and inflammatory molecules. Previous studies demonstrated that synovial fluid-derived exosomes of OA patients possessed the characterization of the proinflammatory profile to M1 macrophages. The exosomes upregulated the IL-1β expression and induced the release of chemokines and promoted the production of MMP7 and MMP12 (Kolhe et al., 2017).
Subchondral osteocytes-derived exosomes
Subchondral bone supports the surface cartilage and bears the mechanical load. The crosstalk between the cartilage and subchondral bone is proceeding in an orderly manner, conducted in an exosome-dependent pattern (Domenis et al., 2017). Once the balance of the interaction is disrupted, cartilage breaks down and subchondral bone remodels abnormally, exacerbating the progression of OA (Wu et al., 2022). TMJ is one of the most flexible joints in the body and the subchondral bone of TMJ has an outstanding ability to withstand multidirectional forces. Sun discovered a new mode of osteoclast-osteoblast communication. MiR-214-enriched exosomes secreted by osteoclasts were specifically transferred into osteoblasts via ephrinA2/EphA2 axis and suppressed osteoblast function (Sanchez et al., 2005). Moreover, there was an obvious promotion of bone formation after osteoclast-targeted miR-214-3p inhibition (Sun et al., 2016).One In coculture, researchers found that chondrocytes endocytosed the osteoblast derived exosomes in osteoarthritis sclerotic subchondral bone and upregulated catabolic genes and downregulated chondrocyte-specific genes. Wu demonstrated miR-210-5p suppressed the oxygen consumption of chondrocytes and altered cellular bioenergetics, which could be a potential target for therapeutic intervention in OA (Li et al., 2016). It is suggested that targeting the exosomal-miRNAs-transfer of osteoclasts to chondrocytes is an entirely new treatment strategy. In early-stage osteoarthritis, an upregulation of exosomal-osteoclast-derived microRNAs drove the progression of the disease. However, blockage of osteoclast-originated exosomes retarded osteoarthritis progression, mechanistically, via increasing the resistance of chondrocyte to matrix degeneration, endothelial cell angiogenesis and axon sensory innervation (Xu et al., 2021). To explore the potential osteogenesis of the exosomes from osteoblasts, Ge isolated EVs from MC3T3 and presented osteogenesis-related proteins and pathways through the protein profile. Eukaryotic initiation factor 2 pathways played an important role in osteogenesis and represented a potential therapeutic avenue to tackle OA (Liu et al., 2020). Mineralizing osteoblasts-derived exosomes significantly promoted osteogenesis and influenced miRNA profiles in bone marrow stromal cells, which activated the WNT pathway by increasing β-catenin and dampening Axin1 (Ge et al., 2015).
Adipose tissue-derived exosomes
Adipose tissue-derived exosomes are applicated in the treatment of various diseases (Xu et al., 2019; Zhou Q.-F. et al., 2020; Wei et al., 2020). Intra-articular adipose tissue functions to cushion the shock and acts as one of major sources of cytokines, active mediators as well as regenerative cells in repair. At present, research of adipose-derived exosomes for the treatment of OA mainly focused on adipose tissue-MSCs-derived exosomes (Koh et al., 2012; Ribeiro et al., 2016; Scheja et al., 2019). Sembronio et al. (2021) compared standard OA treatment with hyaluronic acid injections with the new TMJOA therapy of microfragmented adipose tissue injection using the Lipogems technology by a randomized clinical trial. Notably, pain reduction and mouth opening significantly improved in both groups. And the statistical analysis showed that the microfragmented adipose tissue injection group had a statistically significant advantage in the success rate of procedure compared with the hyaluronic acid injections group. Considering the number and secretion capacity of adipocytes, we speculate that their role in osteoarthritis is also critical because they may work as a graft in synovial, secretes exosomes and locally serve as a source of MSCs for a long time. However, a lot of investigations into adipocytes-derived exosomes are still needed to shed light on molecular mechanisms underlying pathogenesis.
Other cell and tissue-derived exosomes
As early as in 2005, microparticles derived from T cells and monocytes were clearly reported to induce the synthesis of matrix metalloproteinases and inflammatory mediators in fibroblasts in a dose-dependent manner (Cui et al., 2016). These results provided evidence for vesicles derived immune cells promoting the destructive activity of SFs. It was reported that Sox9-containing-exosomes of monocytes stimulated with IL-4 or IL-13 upregulated COL2A and ACAN, promoted the differentiation of primary chondrocytes (Distler et al., 2005). Abnormality of the tendon was related to OA progression, which indicated that tendon repair might be another treatment for injury.40 In recent years, some studies have evaluated the role of tendon-derived exosomes in osteoarthritis. In the transwell system, paracrine factors released by tenocytes induced MSCs to the tenogenic differentiation in a TGF-β dependent manner and the inhibition of TGF-β pathway eliminated the effect (Bai et al., 2020).
Noticeably, since the 1970s, many studies have explored the mechanism of platelet rich plasma (PRP) in tissue repair (Toghraie et al., 2011). It contains a variety of cytokines and active substances, promoting tissue regeneration and healing (Xuan et al., 2020). So far, exosomes have been reported to exist in PRP and participate in related physiological and pathological processes (Dohan et al., 2008). Actually, PRP-derived exosomes have been applied to OA treatment via intra articular injection for years in preclinical studies. Zhang incorporated PRP-exosomes into thermosensitive hydrogel (Gel) and assessed its biological activity and the therapeutic effect on OA in vivo (Saumell-Esnaola et al., 2022). It promoted BMSCs proliferation, migration, and chondrogenic differentiation, and inhibited chondrocytes apoptosis and hypertrophy to delay the progression of osteoarthritis. Meanwhile, PRP-derived exosomes inhibited the TNF-α release from chondrocytes and presented a potential in alleviating OA via WNT/β-catenin pathway (Zhang Y. et al., 2022). Alexander found that citrate-anticoagulated platelet-rich plasma-derived exosomes displayed a higher expression of SOX9 protein and a better inhibition effect on proinflammatory cytokine release compared to hyperacute serum-derived exosomes (Liu et al., 2019). Besides, amniotic fluid (AF) is easily to obtain for application in tissue repair and regeneration. Researchers elucidated (Raghu et al., 2017) commonly expressed exosomal-miRNA of AF-derived exosomes, revealed RNA target genes were associated with senescence, fibrosis, and OA pathways, and suggested it as a therapeutic potential strategy for the treatment of osteoarthritis (Otahal et al., 2020).
To date, exosomes derived from different cells and tissues have exhibited effects on the occurrence, development, prevention, and treatment of TMJOA in vitro and in vivo. On the one hand, exosomes play a decisive role in controlling cartilage matrix homeostasis by promoting chondrocyte proliferation and migration and inhibiting chondrocyte apoptosis, thus reversing the deterioration of TMJOA, and alleviating the symptoms of TMJOA. On the other hand, the exosome is trigger in promotion of MSCs migration via various chemotactic pathways and can stimulate chondrogenic differentiation, and repairs cartilage defects. Besides, it cannot be ignored that they also have the great potential in regulating bone homeostasis to better support cartilage. A large number of studies have shown that changes of exosomes in the state of inflammation, which suggests that exosome-based disease treatment strategies will be effective. In the exosome based TMJOA therapy, they reduce the production of inflammatory factors and inhibit the differentiation of proinflammatory M1 macrophages and increase the ratio of M2 macrophages. However, it is just the beginning and further research is urgently needed to explore a more in-depth mechanism and perfect treatment strategy in TMJOA.
Optimization in exosome-based bioengineering strategies of TMJOA therapy
Owing to the uniqueness and complexity of the TMJ, it is a great challenge to achieve complete restoration of its anatomical, structural, and functional integrity. The optimization of exosome-based strategy is a necessary step for TMJOA treatment. Bioengineering is constantly developing and provides an optimized solution in regenerative medicine (Table 3). Helgeland made a systematic review to answer the question of whether scaffold based TMJ tissue regeneration have better outcomes in TMJOA treatment. The overall preclinical evidence indicated that biomaterial scaffolds combined with biological components enhanced the potential for cartilage regeneration in TMJOA (Bellio et al., 2020). Additionally, the optimization of bioengineering technology in exosome based TMJOA therapeutic strategy has aroused the hot interests of researchers. There might be several disadvantages with direct administration of exosome-containing suspension in cartilage regeneration, especially, the difficulty of local exosome retention. Liu developed a photoinduced hydrogel exosome scaffold for a better retention of cargo exosome (Helgeland et al., 2018). In the system, they demonstrated that it retained stem cell-derived exosomes and showed an excellent biocompatibility and cartilage-integration by positively regulating both chondrocytes and BMSCs in vitro and promoting cartilage matrix and cell deposition at cartilage injury site. Using a crosslinked network of chondroitin sulfate, alginate-dopamine, and regenerated silk fibroin, an injectable hydrogel with encapsulated exosomes was exploited in superficial cartilage regeneration. Exosomes released by the hydrogels recruited BMSCs into defects via the chemokine pathway (Liu X. et al., 2017). These findings revealed the hydrogel coated with exosomes as a promising approach for accelerating cartilage regeneration in situ and neo-cartilage extracellular matrix remodeling. Chen designed a 3D printed cartilage extracellular matrix-gelatin methacrylate-exosome delivery scaffold (ECM/GelMA/Ex scaffold) with radial channels and superior cell recruitment capacity. The scaffold not only enhanced cartilage regeneration but also facilitated recovery of subchondral bone. Furthermore, they also found that MSCs exosomes enhanced mitochondrial biogenesis and rescued the mitochondrial dysfunction in degenerated cartilage (Zhang et al., 2021). The controlled exosome release platform with histological biological scaffolds solves the problems of insufficient local exosome concentration and short half-life of exosomes after injection to a large extent, optimizing the exosome based TMJOA treatment strategy.
The dense matrix biological barrier of cartilage makes chondrocyte-targeted drug delivery difficult. Exosomes enter the cell mainly via endocytosis, direct membrane fusion, or pinocytosis, 66 and the released contents could exert biological effects. It indicates exosomes have a great potential as a vehicle for drug delivery. Hence, it is an innovation and optimization in TMJOA treatment to modify surface structures for improving the efficiency of exosomes entering cells and to modulate encapsulated contents for strengthening therapeutic effects by genetic engineering technology or direct physicochemical loading. A study demonstrated that exosomes gained MSC targeting capability after fusing exosomal membrane protein Lamp 2b with MSC-binding peptide E7 (Chen et al., 2019). SFMSCs with E7-exosomes entered the middle zone of the cartilage more easily. Additionally, BMSCs-derived exosomes loading with KGN (a small molecule that can induce MSCs differentiation to chondrocytes) by electroporation efficiently increased COL2 and ACAN and induced higher cartilage differentiation of SFMSCs. In vivo, it showed best cartilage repair. ATF4-overexpressing exosomes were developed by introducing the mRNA of ATF4 into exosomes via electroporation. It showed these exosomes alleviated inflammation and cartilage degeneration in osteoarthritis mice by promoting chondrocytes autophagy and inhibiting apoptosis (Xu et al., 2021). To achieve a more predictable and desirable clinical response, specific therapeutic miRNA enrichment could be performed through the overexpressing genetic technique. It was suggested that miR-140-5p-overexpressing hSDMSCs derived exosomes enhanced the proliferation and migration abilities of chondrocytes (Wang Z. et al., 2021). They observed the exosomes highly expressed miR-140-5p blocked this side-effect in ECM via targeting RalA to enhance SOX9 and ACAN. Exosomes derived from miR-92a-3p-overexpressing MSCs targeted WNT5A to elevate chondrogenesis in MSCs and suppress cartilage degradation in primary chondrocytes (Tao et al., 2017). Besides, hBMSC-derived overexpressing miR-26a-5p exosomes relieved OA and were served as a repressor to retard damage of SFs via PTGS2 downregulation in vitro (Mao et al., 2018). Generally, utilizing a specific exosomal-miRNA mainly involves these proposed mechanisms, the overexpression of miRNA in cells, the isolation of exosomes containing miRNA, then delivery to chondrocytes in inflammatory microenvironment or TMJOA animal models, and finally targeting a pathogenic gene via miRNA. Some studies have shown that the effect of hypoxia-preconditioned exosomes on cartilage repair are superior to that of normoxia-preconditioned exosomes, manifesting in the promotion of chondrocyte proliferation and migration and the inhibition of chondrocyte apoptosis (Jin et al., 2020). TGF-β1-stimulated BMSCs-derived exosomes highly expressed miR-135b and polarized synovial macrophages (SMs) into M2 type to alleviate cartilage destruction. M2 polarization of SMs was significantly reversed by increase of MAPK6 (Zhang B. et al., 2022). Pretreating exosomes with physical or chemical stimulation optimizes exosome-based therapeutic strategies.
Future opportunities and challenges of exosome-based therapeutic strategy in TMJOA
Although exosomes were originally regarded as useless metabolic byproducts, it is well recognized that exosomes, as various carrier of signaling mediators, play a vital role in mediating cell-to-cell communication and in activating immunomodulatory activity. Certainly, numerous studies have shown exosomes are sufficient to treat degenerative diseases, including systemic OA and TMJOA. A correct view of the prospects and existing problems of exosome-based therapeutic strategy is the basis for further research.
Emerging as a trending research area, exosome-based therapeutic strategy in TMJOA has gained much interest because of its unique regulatory ability in TMJ inflammation as well as the low immunogenicity (Wang Y. et al., 2021). Some studies have reported that MSCs show a certain degree of immunogenicity in mediating disease treatment (Qi et al., 2016; Gregory et al., 2005). Compared with MSCs, exosomes have been reported not to express class II human leukocyte antigens and have lower immunogenicity when applicated in vivo (Krampera et al., 2006; Stagg et al., 2006; Sun et al., 2009; Geiger et al., 2015; Qi et al., 2016; Yaghoubi et al., 2019; Kang et al., 2020). TMJ is a complex system, and the immune privilege of exosomes maximally ensures it not to be cleared by immune cells when playing the therapeutic role in TMJOA. In addition, at present, few studies have reported the tumorigenic effect of exosomes in vivo, which might occur in the MSC-based therapy (Wislet-Gendebien et al., 2012; Funes et al., 2014; Li et al., 2022). Because cartilage is a dense biological barrier staggered by collagenous fiber, the transport property of exosomes is advantageous in TMJOA treatment. Being nano-sized and biocompatible, exosomes can be served as nanocarriers, easily reaching to the cartilage to fuse with chondrocytes. Certainly, the side effects associated with cell-based therapy, such as vascular embolism and pulmonary embolism (De Boeck et al., 2013; Jung et al., 2013), are also rare when exosomes are injected systematically. Furthermore, the less strict storage condition also gives exosomes greater possibilities for therapeutic application. Low temperature cold storage or repeated freezing and thawing does not influence exosome sizes and bioactivities, which shows higher clinical application value compared with cell-based therapies. It takes more time to resuscitate frozen cells to normal functional state, and the activity of resuscitated cells cannot be predicted (Mäkelä et al., 2015). Most importantly, many studies have shown that cell-cell interactions are mainly dependent on exosomes. MSCs-derived exosomes share the same or even more powerful biological effects than MSCs (Zhou X. et al., 2020), such as metabolic regulation of cartilage matrix, inhibition of inflammatory factors, relief of TMJOA pain, and homing of cells to the cartilage defect. Moreover, exosomes modified by genetic engineering have higher organotropism and cartilage-targeting capability. It has involved the comparison of exosomal differences in organs or systems between healthy and sick populations. High-throughput sequencing has confirmed that exosomes in the joint system differ between normal state and TMJOA, which indicates exosomes can be used as biomarkers for the early diagnosis of TMJOA. MiRNA is one of the important cargoes of exosomes. Through literature review, we noticed some exosome-derived miRNAs related with TMJOA treatment. RNAhybrid and miRanda databases were used to predict the target genes of the miRNAs, and Gene ontology (GO) and Kyoto Encyclopedia of Genes and Genomes (KEGG) enrichment analysis was performed for the identified target genes (Figure 4). It showed 22 biological processes enriched in the GO analysis, including endocytosis, actin cytoskeleton organization, protein polymerization, and other processes. And it displayed 20 signaling pathways obtained by the KEGG analysis, including osteoclast differentiation, inflammatory mediator regulation of TRP channels, apoptosis, and other pathways. The information of enrichment analysis suggested the mechanism and related targets of exosomes in the treatment of TMJOA, which will help us further study the pathogenesis of TMJOA and find out more effective TMJOA therapeutic strategy in the future.
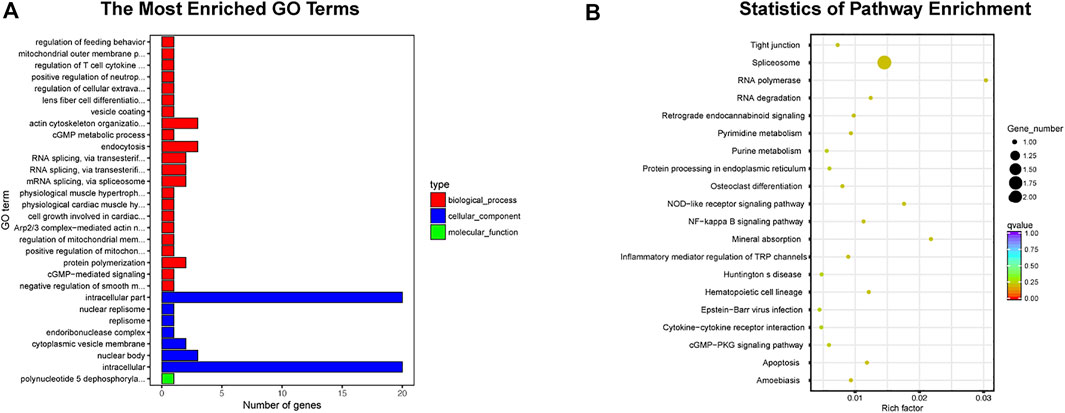
FIGURE 4. Enrichment analysis of exosome-derived miRNAs clearly related to TMJOA. (A) GO analysis, 30 enriched iterms are shown. (B) KEGG analysis, 20 pathways are shown.
Despite the excellent therapeutic effects of exosomes, there are many issues that need to be addressed. Unlike TMJOA cell-therapy, although results from preclinical studies have demonstrated the chondroprotection role of exosomes, explorations into the exosomes efficacy in treatment are still in the start-up stage. Currently, the research on TMJOA treatment mainly focused on small animals. There is almost no large animal studies or human clinical trials to evaluate exosome-based therapeutic strategy in TMJOA. Insufficient evidence from preclinical research and clinical trials significantly hindered the elucidation of mechanisms and the clinical translation applications. Therefore, future studies are recommended to bridge this knowledge gap and validate the safety and efficacy of exosomes therapy. Meanwhile, the difficulties encountered in the acquisition and the preparation of exosomes are inescapable. Various methods of exosomes separation in vitro have been developed, such as ultracentrifugation-based technique, size-based technique, and immuno-affinity action-based technique (Lőrincz et al., 2014). However, the most standardized and optimal operational procedure has not yet been established. The comparability between different studies is poor due to the differences of yield and purity of exosomes. Extracting homotypic exosomes with consistent contents is crucial in precisive therapy and in reduction of adverse effects caused by unintended unknown by-products (Ding et al., 2021). It is urgent to develop an optimal isolation procedure, which maximizes yield and purity of exosomes and minimizes changes of contents and sizes during extraction. In addition, due to the quick turnover of synovial fluid in TMJ cavity, more studies are needed to determine the effective dose and frequency of exosomes injection. Like cell-based therapy, exosome-based therapeutic strategy is also limited by rapid clearance in vivo and short effective period in direct injection. Therefore, it is particularly critical to optimize the therapeutic strategy of exosomes in TMJOA via tissue engineering approaches. Notably, the cartilage of TMJ is fibrocartilage, which is different from the hyaline cartilage of the most joints of the body. It is made up of various proportions of both cartilaginous tissue and fibrous and has a more complex tissue structure and tensile and compressive strength. Although there are many studies on the treatment of OA, it is still questionable whether these treatment measures are also effective for TMJOA. We should explore the effectiveness of these treatments on TMJOA in a more scientific and rigorous manner. ‘A one size fits all’ therapeutic scaffold may not achieve the best treatment effects in TMJOA (Embree et al., 2016; Jiang et al., 2021; Jiang et al., 2021; Fan et al., 2022). According to the characteristics of different fibrocartilage tissue types, layered scaffolds loaded with exosomes exhibit outstanding advantages in the formation of layered tissue structure in cartilage regeneration to simulate the normal fibrocartilage to the maximum extent. Though faced with challenges, exosome-based therapeutic strategy is promising in TMJOA and worthy of further investigations in vivo and in vitro.
Conclusion
In this review, we summarized the roles of MSCs and exosomes in TMJOA, manifesting in the regulation of cartilage matrix metabolism, the balance of subchondral bone homeostasis, the relief of inflammation, and the effects of immune regulation. Currently, MSC-based therapy is facing many challenges, while exosome-based therapeutic strategy can be a promising novel alternative because of its advantages in cell-to-cell communication in TMJ system. Exosomes, as mini vesicles, deliver nucleic acids and proteins to target tissues or cells and exert therapeutic efficacy in TMJOA. The pathogenesis of TMJOA is complicated and multifactorial. The optimization of the existing exosome-based strategies, such as the combination of tissue engineering scaffolds or genetic modification, more effectively reduce the side effects involved in exosomes treatment and improve cartilage repair and regeneration. However, the translation from experimental research to clinical application of exosomes has been hindered due to insufficient evidence of preclinical and clinical trials. Further research is needed to identify effective constituents of TMJO-target exosomes and explore underlying mechanisms, to investigate therapeutic targets, to evaluate the safety of exosomes application, and finally to establish a consensus in the therapeutic potency of exosome-based therapeutic strategy.
Author contributions
Conceptualization, JW, JnL, and WY; writing—original draft preparation WY; writing—review and editing YW, MH, JnL, XZ, YY, JW, and JqL; supervision, project administration, funding acquisition, JW and JnL; created the table, WY and MH; prepared the figures, WY, YW, MH, JqL, XZ, and YY. All authors contributed to the article and approved the submitted version.
Funding
This research was supported by National Natural Science Foundation of China (No. 81970967), Sichuan Health Commission Medical Science and Technology Program (21ZD003) and Research and Develop Program, West China Hospital of Stomatology Sichuan University (RD-03-202101) to JW.
Conflict of interest
The authors declare that the research was conducted in the absence of any commercial or financial relationships that could be construed as a potential conflict of interest.
Publisher’s note
All claims expressed in this article are solely those of the authors and do not necessarily represent those of their affiliated organizations, or those of the publisher, the editors and the reviewers. Any product that may be evaluated in this article, or claim that may be made by its manufacturer, is not guaranteed or endorsed by the publisher.
References
Ahtiainen, K., Mauno, J., Ellä, V., Hagström, J., Lindqvist, C., Miettinen, S., et al. (2013). Autologous adipose stem cells and polylactide discs in the replacement of the rabbit temporomandibular joint disc. J. R. Soc. Interface 10 (85), 20130287. doi:10.1098/rsif.2013.0287
Almonte-Becerril, M., Navarro-Garcia, F., Gonzalez-Robles, A., Vega-Lopez, M. A., Lavalle, C., and Kouri, J. B. (2010). Cell death of chondrocytes is a combination between apoptosis and autophagy during the pathogenesis of Osteoarthritis within an experimental model. Apoptosis 15 (5), 631–638. doi:10.1007/s10495-010-0458-z
Armiñán, A., Gandía, C., García-Verdugo, J. M., Lledó, E., Trigueros, C., Ruiz-Saurí, A., et al. (2010). Mesenchymal stem cells provide better results than hematopoietic precursors for the treatment of myocardial infarction. J. Am. Coll. Cardiol. 55 (20), 2244–2253. doi:10.1016/j.jacc.2009.08.092
Bai, J., Zhang, Y., Zheng, X., Huang, M., Cheng, W., Shan, H., et al. (2020). LncRNA MM2P-induced, exosome-mediated transfer of Sox9 from monocyte-derived cells modulates primary chondrocytes. Cell Death Dis. 11 (9), 763. doi:10.1038/s41419-020-02945-5
Bartholomew, A., Sturgeon, C., Siatskas, M., Ferrer, K., McIntosh, K., Patil, S., et al. (2002). Mesenchymal stem cells suppress lymphocyte proliferation in vitro and prolong skin graft survival in vivo. Exp. Hematol. 30 (1), 42–48. doi:10.1016/s0301-472x(01)00769-x
Bellio, M. A., Young, K. C., Milberg, J., Santos, I., Abdullah, Z., Stewart, D., et al. (2021). Amniotic fluid-derived extracellular vesicles: characterization and therapeutic efficacy in an experimental model of bronchopulmonary dysplasia. Cytother. 23 (12), 1097–1107. doi:10.1016/j.jcyt.2021.07.011
Budden, C. F., Gearing, L. J., Kaiser, R., Standke, L., Hertzog, P. J., and Latz, E. (2021). Inflammasome-induced extracellular vesicles harbour distinct RNA signatures and alter bystander macrophage responses. J. Extracell. Vesicles 10 (10), e12127. doi:10.1002/jev2.12127
Chandrasekaran, P., Kwok, B., Han, B., Adams, S. M., Wang, C., Chery, D. R., et al. (2021). Type V collagen regulates the structure and biomechanics of TMJ condylar cartilage: A fibrous-hyaline hybrid. Matrix Biol. 102, 1–19. doi:10.1016/j.matbio.2021.07.002
Chavakis, E., Hain, A., Vinci, M., Carmona, G., Bianchi, M. E., Vajkoczy, P., et al. (2007). High-mobility group box 1 activates integrin-dependent homing of endothelial progenitor cells. Circulation Res. 100 (2), 204–212. doi:10.1161/01.RES.0000257774.55970.f4
Chen, D., Wu, J. Y., Kennedy, K. M., Yeager, K., Bernhard, J. C., Ng, J. J., et al. (2020). Tissue engineered autologous cartilage-bone grafts for temporomandibular joint regeneration. Sci. Transl. Med. 12 (565), eabb6683. doi:10.1126/scitranslmed.abb6683
Chen, K., Man, C., Zhang, B., Hu, J., and Zhu, S. S. (2013). Effect of in vitro chondrogenic differentiation of autologous mesenchymal stem cells on cartilage and subchondral cancellous bone repair in osteoarthritis of temporomandibular joint. Int. J. Oral Maxillofac. Surg. 42 (2), 240–248. doi:10.1016/j.ijom.2012.05.030
Chen, P., Zheng, L., Wang, Y., Tao, M., Xie, Z., Xia, C., et al. (2019). Desktop-stereolithography 3D printing of a radially oriented extracellular matrix/mesenchymal stem cell exosome bioink for osteochondral defect regeneration. Theranostics 9 (9), 2439–2459. doi:10.7150/thno.31017
Ciocca, L., Donati, D., Ragazzini, S., Dozza, B., Rossi, F., Fantini, M., et al. (2013). Mesenchymal stem cells and platelet gel improve bone deposition within CAD-CAM custom-made ceramic HA scaffolds for condyle substitution. BioMed Res. Int. 2013, 1–10. doi:10.1155/2013/549762
Clérigues, V., Guillén, M. I., Castejón, M. A., Gomar, F., Mirabet, V., and Alcaraz, M. J. (2012). Heme oxygenase-1 mediates protective effects on inflammatory, catabolic and senescence responses induced by interleukin-1β in osteoarthritic osteoblasts. Biochem. Pharmacol. 83 (3), 395–405. doi:10.1016/j.bcp.2011.11.024
Cosenza, S., Ruiz, M., Toupet, K., Jorgensen, C., and Noël, D. (2017). Mesenchymal stem cells derived exosomes and microparticles protect cartilage and bone from degradation in osteoarthritis. Sci. Rep. 7 (1), 16214. doi:10.1038/s41598-017-15376-8
Cui, Y., Luan, J., Li, H., Zhou, X., and Han, J. (2016). Exosomes derived from mineralizing osteoblasts promote ST2 cell osteogenic differentiation by alteration of microRNA expression. FEBS Lett. 590 (1), 185–192. doi:10.1002/1873-3468.12024
De Boeck, A., Pauwels, P., Hensen, K., Rummens, J.-L., Westbroek, W., Hendrix, A., et al. (2013). Bone marrow-derived mesenchymal stem cells promote colorectal cancer progression through paracrine neuregulin 1/HER3 signalling. Gut 62 (4), 550–560. doi:10.1136/gutjnl-2011-301393
Derwich, M., Mitus-Kenig, M., and Pawlowska, E. (2021). Mechanisms of action and efficacy of hyaluronic acid, corticosteroids and platelet-rich plasma in the treatment of temporomandibular joint osteoarthritis-A systematic review. Int. J. Mol. Sci. 22 (14), 7405. doi:10.3390/ijms22147405
Ding, L., Yang, X., Gao, Z., Effah, C. Y., Zhang, X., Wu, Y., et al. (2021). A holistic review of the state-of-the-art microfluidics for exosome separation: An overview of the current status, existing obstacles, and future outlook. Small (Weinheim Der Bergstrasse, Ger. 17 (29), e2007174. doi:10.1002/smll.202007174
Distler, J. H. W., Jüngel, A., Huber, L. C., Seemayer, C. A., Reich, C. F., Gay, R. E., et al. (2005). The induction of matrix metalloproteinase and cytokine expression in synovial fibroblasts stimulated with immune cell microparticles. Proc. Natl. Acad. Sci. U. S. A. 102 (8), 2892–2897. doi:10.1073/pnas.0409781102
Dohan Ehrenfest, D. M., Rasmusson, L., and Albrektsson, T. (2009). Classification of platelet concentrates: From pure platelet-rich plasma (P-PRP) to leucocyte- and platelet-rich fibrin (L-PRF). Trends Biotechnol. 27 (3), 158–167. doi:10.1016/j.tibtech.2008.11.009
Domenis, R., Zanutel, R., Caponnetto, F., Toffoletto, B., Cifù, A., Pistis, C., et al. (2017). Characterization of the proinflammatory profile of synovial fluid-derived exosomes of patients with osteoarthritis. Mediat. Inflamm. 2017, 1–11. doi:10.1155/2017/4814987
Duarte, J. H. (2015). Osteoarthritis: SIRT6 prevents chondrocyte senescence and DNA damage. Nat. Rev. Rheumatol. 11 (5), 260. doi:10.1038/nrrheum.2015.52
Dubus, M., Varin, J., Papa, S., Chevrier, J., Quilès, F., Francius, G., et al. (2022). Bone marrow mesenchymal stem cells offer an immune-privileged niche to Cutibacterium acnes in case of implant-associated osteomyelitis. Acta Biomater. 137, 305–315. doi:10.1016/j.actbio.2021.10.026
Embree, M. C., Chen, M., Pylawka, S., Kong, D., Iwaoka, G. M., Kalajzic, I., et al. (2016). Exploiting endogenous fibrocartilage stem cells to regenerate cartilage and repair joint injury. Nat. Commun. 7, 13073. doi:10.1038/ncomms13073
Fan, S. J., Kroeger, B., Marie, P. P., Bridges, E. M., Mason, J. D., McCormick, K., et al. (2020). Glutamine deprivation alters the origin and function of cancer cell exosomes. EMBO J. 39, e103009. doi:10.15252/embj.2019103009
Fan, Y., Li, Z., and He, Y. (2022). Exosomes in the pathogenesis, progression, and treatment of osteoarthritis. Bioeng. (Basel, Switz. 9 (3), 99. doi:10.3390/bioengineering9030099
Feng, Y., Hu, S., Liu, L., Ke, J., and Long, X. (2021). HMGB1 contributes to osteoarthritis of temporomandibular joint by inducing synovial angiogenesis. J. Oral Rehabil. 48 (5), 551–559. doi:10.1111/joor.13129
Friedenstein, A. J., Latzinik, N. W., Grosheva, A. G., and Gorskaya, U. F. (1982). Marrow microenvironment transfer by heterotopic transplantation of freshly isolated and cultured cells in porous sponges. Exp. Hematol. 10 (2), 217–227.
Funes, J. M., Henderson, S., Kaufman, R., Flanagan, J. M., Robson, M., Pedley, B., et al. (2014). Oncogenic transformation of mesenchymal stem cells decreases Nrf2 expression favoring in vivo tumor growth and poorer survival. Mol. Cancer 13, 20. doi:10.1186/1476-4598-13-20
Garikipati, V. N. S., Shoja-Taheri, F., Davis, M. E., and Kishore, R. (2018). Extracellular vesicles and the application of system biology and computational modeling in cardiac repair. Circ. Res. 123 (2), 188–204. doi:10.1161/CIRCRESAHA.117.311215
Ge, M., Ke, R., Cai, T., Yang, J., and Mu, X. (2015). Identification and proteomic analysis of osteoblast-derived exosomes. Biochem. Biophysical Res. Commun. 467 (1), 27–32. doi:10.1016/j.bbrc.2015.09.135
Geiger, A., Walker, A., and Nissen, E. (2015). Human fibrocyte-derived exosomes accelerate wound healing in genetically diabetic mice. Biochem. Biophysical Res. Commun. 467 (2), 303–309. doi:10.1016/j.bbrc.2015.09.166
Gregory, C. A., Ylostalo, J., and Prockop, D. J. (2005). Adult bone marrow stem/progenitor cells (MSCs) are preconditioned by microenvironmental “niches” in culture: A two-stage hypothesis for regulation of MSC fate. Sci. STKE. 2005 (294), pe37. doi:10.1126/stke.2942005pe37
He, L., He, T., Xing, J., Zhou, Q., Fan, L., Liu, C., et al. (2020). Bone marrow mesenchymal stem cell-derived exosomes protect cartilage damage and relieve knee osteoarthritis pain in a rat model of osteoarthritis. Stem Cell Res. Ther. 11 (1), 276. doi:10.1186/s13287-020-01781-w
Helgeland, E., Shanbhag, S., Pedersen, T. O., Mustafa, K., and Rosén, A. (2018). Scaffold-based temporomandibular joint tissue regeneration in experimental animal models: A systematic ReviewAn abstract of this article was presented as a poster, at the bergen stem cell consortium (BSCC), annual meeting, bergen, Norway, september 3–4, 2017. Tissue Eng. Part B Rev. 24 (4), 300–316. doi:10.1089/ten.TEB.2017.0429
Hernández, M., Dutzan, N., García-Sesnich, J., Abusleme, L., Dezerega, A., Silva, N., et al. (2011). Host-pathogen interactions in progressive chronic periodontitis. J. Dent. Res. 90 (10), 1164–1170. doi:10.1177/0022034511401405
Huang, Z., Yang, R., Zhang, L., Zhu, M., Zhang, C., Wen, J., et al. (2021). BRD4 inhibition alleviates mechanical stress-induced TMJ OA-like pathological changes and attenuates TREM1-mediated inflammatory response. Clin. Epigenetics 13 (1), 10. doi:10.1186/s13148-021-01008-6
Ibrahim, M., Kartus, J.-T., Steigen, S. E., Olsen, R., and Meknas, K. (2019). More tendon degeneration in patients with shoulder osteoarthritis. Knee Surg. Sports Traumatol. Arthrosc. 27 (1), 267–275. doi:10.1007/s00167-018-5186-x
Jeon, H., and Im, G.-I. (2017). Autophagy in osteoarthritis. Connect. Tissue Res. 58 (6), 497–508. doi:10.1080/03008207.2016.1240790
Jiang, S., Tian, G., Yang, Z., Gao, X., Wang, F., Li, J., et al. (2021). Enhancement of acellular cartilage matrix scaffold by Wharton's jelly mesenchymal stem cell-derived exosomes to promote osteochondral regeneration. Bioact. Mater. 6 (9), 2711–2728. doi:10.1016/j.bioactmat.2021.01.031
Jiao, K., Niu, L.-N., Wang, M.-Q., Dai, J., Yu, S.-B., Liu, X.-D., et al. (2011). Subchondral bone loss following orthodontically induced cartilage degradation in the mandibular condyles of rats. Bone 48 (2), 362–371. doi:10.1016/j.bone.2010.09.010
Jin, Z., Ren, J., and Qi, S. (2020). Human bone mesenchymal stem cells-derived exosomes overexpressing microRNA-26a-5p alleviate osteoarthritis via down-regulation of PTGS2. Int. Immunopharmacol. 78, 105946. doi:10.1016/j.intimp.2019.105946
Jung, J. W., Kwon, M., Choi, J. C., Shin, J. W., Park, I. W., Choi, B. W., et al. (2013). Familial occurrence of pulmonary embolism after intravenous, adipose tissue-derived stem cell therapy. Yonsei Med. J. 54 (5), 1293–1296. doi:10.3349/ymj.2013.54.5.1293
Kang, M., Choi, J. K., Jittayasothorn, Y., and Egwuagu, C. E. (2020). Interleukin 35-producing exosomes suppress neuroinflammation and autoimmune uveitis. Front. Immunol. 11, 1051. doi:10.3389/fimmu.2020.01051
Kanki, S., Jaalouk, D. E., Lee, S., Yu, A. Y., Gannon, J., and Lee, R. T. (2011). Identification of targeting peptides for ischemic myocardium by in vivo phage display. J. Mol. Cell. Cardiol. 50, 841–848. doi:10.1016/j.yjmcc.2011.02.003
Kato, T., Miyaki, S., Ishitobi, H., Nakamura, Y., Nakasa, T., Lotz, M. K., et al. (2014). Exosomes from IL-1β stimulated synovial fibroblasts induce osteoarthritic changes in articular chondrocytes. Arthritis Res. Ther. 16 (4), R163. doi:10.1186/ar4679
Kim, H., Yang, G., Park, J., Choi, J., Kang, E., and Lee, B.-K. (2019). Therapeutic effect of mesenchymal stem cells derived from human umbilical cord in rabbit temporomandibular joint model of osteoarthritis. Sci. Rep. 9 (1), 13854. doi:10.1038/s41598-019-50435-2
Kim, S. J., Kim, J. E., Kim, S. H., Kim, S. J., Jeon, S. J., Kim, S. H., et al. (2016). Therapeutic effects of neuropeptide substance P coupled with self-assembled peptide nanofibers on the progression of osteoarthritis in a rat model. Biomaterials 74, 119–130. doi:10.1016/j.biomaterials.2015.09.040
Kim, Y. G., Choi, J., and Kim, K. (2020). Mesenchymal stem cell-derived exosomes for effective cartilage tissue repair and treatment of osteoarthritis. Biotechnol. J. 15 (12), e2000082. doi:10.1002/biot.202000082
Koelling, S., Kruegel, J., Irmer, M., Path, J. R., Sadowski, B., Miro, X., et al. (2009). Migratory chondrogenic progenitor cells from repair tissue during the later stages of human osteoarthritis. Cell Stem Cell 4 (4), 324–335. doi:10.1016/j.stem.2009.01.015
Koh, Y.-G., and Choi, Y.-J. (2012). Infrapatellar fat pad-derived mesenchymal stem cell therapy for knee osteoarthritis. Knee 19 (6), 902–907. doi:10.1016/j.knee.2012.04.001
Kolhe, R., Hunter, M., Liu, S., Jadeja, R. N., Pundkar, C., Mondal, A. K., et al. (2017). Gender-specific differential expression of exosomal miRNA in synovial fluid of patients with osteoarthritis. Sci. Rep. 7 (1), 2029. doi:10.1038/s41598-017-01905-y
Koliaraki, V., Prados, A., Armaka, M., and Kollias, G. (2020). The mesenchymal context in inflammation, immunity and cancer. Nat. Immunol. 21 (9), 974–982. doi:10.1038/s41590-020-0741-2
Koyama, N., Okubo, Y., Nakao, K., Osawa, K., Fujimura, K., and Bessho, K. (2011). Pluripotency of mesenchymal cells derived from synovial fluid in patients with temporomandibular joint disorder. Life Sci. 89 (19-20), 741–747. doi:10.1016/j.lfs.2011.09.005
Krampera, M., Cosmi, L., Angeli, R., Pasini, A., Liotta, F., Andreini, A., et al. (2006). Role for interferon-gamma in the immunomodulatory activity of human bone marrow mesenchymal stem cells. Stem Cells Dayt. Ohio) 24 (2), 386–398. doi:10.1634/stemcells.2005-0008
Kuo, J., Wright, G. J., Bach, D. E., Slate, E. H., and Yao, H. (2011). Effect of mechanical loading on electrical conductivity in porcine TMJ discs. J. Dent. Res. 90 (10), 1216–1220. doi:10.1177/0022034511415275
Lalu, M. M., McIntyre, L., Pugliese, C., Fergusson, D., Winston, B. W., Marshall, J. C., et al. (2012). Safe ty of cell therapy with mesenchymal stromal cells (safecell): A systematic review and meta-analysis of clinical trials. PLoS ONE 7, e47559. doi:10.1371/journal.pone.0047559
Lei, J., Chen, S., Jing, J., Guo, T., Feng, J., Ho, T. V., et al. (2022). Inhibiting hh signaling in Gli1+ osteogenic progenitors alleviates TMJOA. J. Dent. Res. 101 (6), 664–674. doi:10.1177/00220345211059079
Li, B., Luan, S., Chen, J., Zhou, Y., Wang, T., Li, Z., et al. (2020). The MSC-derived exosomal lncRNA H19 promotes wound healing in diabetic foot ulcers by upregulating PTEN via MicroRNA-152-3p. Mol. Ther. - Nucleic Acids 19, 814–826. doi:10.1016/j.omtn.2019.11.034
Li, C., Qin, S., Wen, Y., Zhao, W., Huang, Y., and Liu, J. (2022). Overcoming the blood-brain barrier: Exosomes as theranostic nanocarriers for precision neuroimaging. J. Control. Release 349, 902–916. doi:10.1016/j.jconrel.2022.08.002
Li, D., Liu, J., Guo, B., Liang, C., Dang, L., Lu, C., et al. (2016). Osteoclast-derived exosomal miR-214-3p inhibits osteoblastic bone formation. Nat. Commun. 7, 10872. doi:10.1038/ncomms10872
Li, J., Zhang, L., Xin, J., Jiang, L., Li, J., Zhang, T., et al. (2012). Immediate intraportal transplantation of human bone marrow mesenchymal stem cells prevents death from fulminant hepatic failure in pigs. Hepatology 56 (3), 1044–1052. doi:10.1002/hep.25722
Li, R., Wang, Y., Zhang, X., Feng, M., Ma, J., Li, J., et al. (2019a). Exosome-mediated secretion of LOXL4 promotes hepatocellular carcinoma cell invasion and metastasis. Mol. Cancer 18 (1), 18. doi:10.1186/s12943-019-0948-8
Li, W., Zhao, S., Yang, H., Zhang, C., Kang, Q., Deng, J., et al. (2019b). Potential novel prediction of TMJ-OA: MiR-140-5p regulates inflammation through smad/TGF-β signaling. Front. Pharmacol. 10, 15. doi:10.3389/fphar.2019.00015
Lim, A. I., McFadden, T., Link, V. M., Han, S.-J., Karlsson, R.-M., Stacy, A., et al. (2021). Prenatal maternal infection promotes tissue-specific immunity and inflammation in offspring. Sci. (New York, N.Y.) 373 (6558), eabf3002. doi:10.1126/science.abf3002
Liu, Q., Wang, R., Hou, S., He, F., Ma, Y., Ye, T., et al. (2022a). Chondrocyte-derived exosomes promote cartilage calcification in temporomandibular joint osteoarthritis. Arthritis Res. Ther. 24 (1), 44. doi:10.1186/s13075-022-02738-5
Liu, S.-S., Xu, L.-L., Fan, S., Lu, S.-J., Jin, L., Liu, L.-K., et al. (2022b). Effect of platelet-rich plasma injection combined with individualised comprehensive physical therapy on temporomandibular joint osteoarthritis: A prospective cohort study. J. Oral Rehabil. 49 (2), 150–159. doi:10.1111/joor.13261
Liu, W., Sun, Y., He, Y., Zhang, H., Zheng, Y., Yao, Y., et al. (2017a). IL-1β impedes the chondrogenic differentiation of synovial fluid mesenchymal stem cells in the human temporomandibular joint. Int. J. Mol. Med. 39 (2), 317–326. doi:10.3892/ijmm.2016.2832
Liu, X., Shortt, C., Zhang, F., Bater, M. Q., Cowman, M. K., and Kirsch, T. (2020). Extracellular vesicles released from articular chondrocytes play a major role in cell-cell communication. J. Orthop. Res. 38 (4), 731–739. doi:10.1002/jor.24525
Liu, X., Wang, L., Ma, C., Wang, G., Zhang, Y., and Sun, S. (2019). Exosomes derived from platelet-rich plasma present a novel potential in alleviating knee osteoarthritis by promoting proliferation and inhibiting apoptosis of chondrocyte via Wnt/β-catenin signaling pathway. J. Orthop. Surg. Res. 14 (1), 470. doi:10.1186/s13018-019-1529-7
Liu, X., Yang, Y., Li, Y., Niu, X., Zhao, B., Wang, Y., et al. (2017b). Integration of stem cell-derived exosomes with in situ hydrogel glue as a promising tissue patch for articular cartilage regeneration. Nanoscale 9 (13), 4430–4438. doi:10.1039/c7nr00352h
Lőrincz, Á. M., Timár, C. I., Marosvári, K. A., Veres, D. S., Otrokocsi, L., Kittel, Á., et al. (2014). Effect of storage on physical and functional properties of extracellular vesicles derived from neutrophilic granulocytes. J. Extracell. Vesicles 3, 25465. doi:10.3402/jev.v3.25465
Lotz, M. K., and Caramés, B. (2011). Autophagy and cartilage homeostasis mechanisms in joint health, aging and OA. Nat. Rev. Rheumatol. 7 (10), 579–587. doi:10.1038/nrrheum.2011.109
Lu, L., Zhang, X., Zhang, M., Zhang, H., Liao, L., Yang, T., et al. (2015). RANTES and SDF-1 are keys in cell-based therapy of TMJ osteoarthritis. J. Dent. Res. 94 (11), 1601–1609. doi:10.1177/0022034515604621
Luo, P., Jiang, C., Ji, P., Wang, M., and Xu, J. (2019). Exosomes of stem cells from human exfoliated deciduous teeth as an anti-inflammatory agent in temporomandibular joint chondrocytes via miR-100-5p/mTOR. Stem Cell Res. Ther. 10 (1), 216. doi:10.1186/s13287-019-1341-7
Ma, T., Chen, Y., Chen, Y., Meng, Q., Sun, J., Shao, L., et al. (2018). MicroRNA-132, delivered by mesenchymal stem cell-derived exosomes, promote angiogenesis in myocardial infarction. Stem Cells Int. 2018, 1–11. doi:10.1155/2018/3290372
Macedo, C. G., Jain, A. K., Franz-Montan, M., Napimoga, M. H., Clemente-Napimoga, J. T., and Gill, H. S. (2017). Microneedles enhance topical delivery of 15-deoxy-Δ 12, 14 -prostaglandin J 2 and reduce nociception in temporomandibular joint of rats. J. Control. Release 265, 22–29. doi:10.1016/j.jconrel.2017.06.031
Magnano, M. D., Chakravarty, E. F., Broudy, C., Chung, L., Kelman, A., Hillygus, J., et al. (2007). A pilot study of tumor necrosis factor inhibition in erosive/inflammatory osteoarthritis of the hands. J. Rheumatol. 34 (6), 1323–1327.
Mäkelä, T., Takalo, R., Arvola, O., Haapanen, H., Yannopoulos, F., Blanco, R., et al. (2015). Safety and biodistribution study of bone marrow-derived mesenchymal stromal cells and mononuclear cells and the impact of the administration route in an intact porcine model. Cytotherapy 17 (4), 392–402. doi:10.1016/j.jcyt.2014.12.004
Mao, G., Zhang, Z., Hu, S., Zhang, Z., Chang, Z., Huang, Z., et al. (2018). Exosomes derived from miR-92a-3p-overexpressing human mesenchymal stem cells enhance chondrogenesis and suppress cartilage degradation via targeting WNT5A. Stem Cell Res. Ther. 9 (1), 247. doi:10.1186/s13287-018-1004-0
Matheus, H. R., Özdemir, Ş. D., and Guastaldi, F. P. S. (2022). Stem cell-based therapies for temporomandibular joint osteoarthritis and regeneration of cartilage/osteochondral defects: A systematic review of preclinical experiments. Osteoarthr. Cartil. 30 (9), 1174–1185. doi:10.1016/j.joca.2022.05.006
Mayourian, J., Ceholski, D. K., Gorski, P. A., Mathiyalagan, P., Murphy, J. F., Salazar, S. I., et al. (2018). Exosomal microRNA-21-5p mediates mesenchymal stem cell paracrine effects on human cardiac tissue contractility. Circ. Res. 122 (7), 933–944. doi:10.1161/CIRCRESAHA.118.312420
Monasterio, G., Castillo, F., Rojas, L., Cafferata, E. A., Alvarez, C., Carvajal, P., et al. (2018). Th1/Th17/Th22 immune response and their association with joint pain, imagenological bone loss, rankl expression and osteoclast activity in temporomandibular joint osteoarthritis: A preliminary report. J. Oral Rehabil. 45 (8), 589–597. doi:10.1111/joor.12649
Mori, M. A., Ludwig, R. G., Garcia-Martin, R., Brandão, B. B., and Kahn, C. R. (2019). Extracellular miRNAs: From biomarkers to mediators of physiology and disease. Cell Metab. 30 (4), 656–673. doi:10.1016/j.cmet.2019.07.011
Ni, Z., Kuang, L., Chen, H., Xie, Y., Zhang, B., Ouyang, J., et al. (2019). The exosome-like vesicles from osteoarthritic chondrocyte enhanced mature IL-1β production of macrophages and aggravated synovitis in osteoarthritis. Cell Death Dis. 10 (7), 522. doi:10.1038/s41419-019-1739-2
Nikhil, A., and Kumar, A. (2022). Evaluating potential of tissue-engineered cryogels and chondrocyte derived exosomes in articular cartilage repair. Biotechnol. Bioeng. 119 (2), 605–625. doi:10.1002/bit.27982
Ootake, T., Ishii, T., Sueishi, K., Watanabe, A., Ishizuka, Y., Amano, K., et al. (2021). Effects of mechanical stress and deficiency of dihydrotestosterone or 17β-estradiol on Temporomandibular Joint Osteoarthritis in mice. Osteoarthr. Cartil. 29 (11), 1575–1589. doi:10.1016/j.joca.2021.08.005
Otahal, A., Kramer, K., Kuten-Pella, O., Weiss, R., Stotter, C., Lacza, Z., et al. (2020). Characterization and chondroprotective effects of extracellular vesicles from plasma- and serum-based autologous blood-derived products for osteoarthritis therapy. Front. Bioeng. Biotechnol. 8, 584050. doi:10.3389/fbioe.2020.584050
Ou, F., Huang, Y., Sun, J., Su, K., He, Y., Zeng, R., et al. (2021). Yohimbine ameliorates temporomandibular joint chondrocyte inflammation with suppression of NF-κB pathway. Inflammation 44 (1), 80–90. doi:10.1007/s10753-020-01310-0
Pegtel, D. M., Cosmopoulos, K., Thorley-Lawson, D. A., van Eijndhoven, M. A., Hopmans, E. S., Lindenberg, J. L., et al. (2010). Functional delivery of viral miRNAs via exosomes. Proc. Natl. Acad. Sci. U. S. A. 107 (14), 6328–6333. doi:10.1073/pnas.0914843107
Pham, P. T., Fukuda, D., Nishimoto, S., Kim-Kaneyama, J.-R., Lei, X.-F., Takahashi, Y., et al. (2021). STING, a cytosolic DNA sensor, plays a critical role in atherogenesis: A link between innate immunity and chronic inflammation caused by lifestyle-related diseases. Eur. Heart J. 42 (42), 4336–4348. doi:10.1093/eurheartj/ehab249
Qi, X., Zhang, J., Yuan, H., Xu, Z., Li, Q., Niu, X., et al. (2016). Exosomes secreted by human-induced pluripotent stem cell-derived mesenchymal stem cells repair critical-sized bone defects through enhanced angiogenesis and osteogenesis in osteoporotic rats. Int. J. Biol. Sci. 12 (7), 836–849. doi:10.7150/ijbs.14809
Raghu, H., Lepus, C. M., Wang, Q., Wong, H. H., Lingampalli, N., Oliviero, F., et al. (2017). CCL2/CCR2, but not CCL5/CCR5, mediates monocyte recruitment, inflammation and cartilage destruction in osteoarthritis. Ann. Rheum. Dis. 76 (5), 914–922. doi:10.1136/annrheumdis-2016-210426
Ratajczak, J., Miekus, K., Kucia, M., Zhang, J., Reca, R., Dvorak, P., et al. (2006). Embryonic stem cell-derived microvesicles reprogram hematopoietic progenitors: Evidence for horizontal transfer of mRNA and protein delivery. Leukemia 20 (5), 847–856. doi:10.1038/sj.leu.2404132
Ribeiro, M., López de Figueroa, P., Blanco, F. J., Mendes, A. F., and Caramés, B. (2016). Insulin decreases autophagy and leads to cartilage degradation. Osteoarthr. Cartil. 24 (4), 731–739. doi:10.1016/j.joca.2015.10.017
Roemer, F. W., Kassim Javaid, M., Guermazi, A., Thomas, M., Kiran, A., Keen, R., et al. (2010). Anatomical distribution of synovitis in knee osteoarthritis and its association with joint effusion assessed on non-enhanced and contrast-enhanced MRI. Osteoarthr. Cartil. 18 (10), 1269–1274. doi:10.1016/j.joca.2010.07.008
Rufino-Ramos, D., Albuquerque, P. R., Carmona, V., Perfeito, R., Kahn, C. R., Nobre, R. J., and Pereira de Almeida, L. (2017). Extracellular vesicles: Novel promising delivery systems for therapy of brain diseases. J. Control. Release 262, 247–258. doi:10.1016/j.jconrel.2017.07.001
Sanchez, C., Deberg, M. A., Piccardi, N., Msika, P., Reginster, J. Y. L., and Henrotin, Y. E. (2005). Subchondral bone osteoblasts induce phenotypic changes in human osteoarthritic chondrocytes. Osteoarthr. Cartil. 13 (11), 988–997. doi:10.1016/j.joca.2005.07.012
Saumell-Esnaola, M., Delgado, D., García Del Caño, G., Beitia, M., Sallés, J., González-Burguera, I., et al. (2022). Isolation of platelet-derived exosomes from human platelet-rich plasma: Biochemical and morphological characterization. Int. J. Mol. Sci. 23 (5), 2861. doi:10.3390/ijms23052861
Scheja, L., and Heeren, J. (2019). The endocrine function of adipose tissues in health and cardiometabolic disease. Nat. Rev. Endocrinol. 15 (9), 507–524. doi:10.1038/s41574-019-0230-6
Schwartz, A. G., Long, F., and Thomopoulos, S. (2015). Enthesis fibrocartilage cells originate from a population of Hedgehog-responsive cells modulated by the loading environment. Development 142 (1), 196–206. doi:10.1242/dev.112714
Scrivani, S. J., Keith, D. A., and Kaban, L. B. (2008). Temporomandibular disorders. N. Engl. J. Med. Overseas. Ed. 359 (25), 2693–2705. doi:10.1056/NEJMra0802472
Sembronio, S., Tel, A., Tremolada, C., Lazzarotto, A., Isola, M., and Robiony, M. (2021). Temporomandibular joint arthrocentesis and microfragmented adipose tissue injection for the treatment of internal derangement and osteoarthritis: A randomized clinical trial. J. Oral Maxillofac. Surg. 79 (7), 1447–1456. doi:10.1016/j.joms.2021.01.038
Siddappa, R., Licht, R., van Blitterswijk, C., and de Boer, J. (2007). Donor variation and loss of multipotency during in vitro expansion of human mesenchymal stem cells for bone tissue engineering. J. Orthop. Res. 25 (8), 1029–1041. doi:10.1002/jor.20402
Stagg, J., Pommey, S., Eliopoulos, N., and Galipeau, J. (2006). Interferon-gamma-stimulated marrow stromal cells: A new type of nonhematopoietic antigen-presenting cell. Blood 107 (6), 2570–2577. doi:10.1182/blood-2005-07-2793
Su, S. C., Tanimoto, K., Tanne, Y., Kunimatsu, R., Hirose, N., Mitsuyoshi, T., et al. (2014). Celecoxib exerts protective effects on extracellular matrix metabolism of mandibular condylar chondrocytes under excessive mechanical stress. Osteoarthr. Cartil. 22 (6), 845–851. doi:10.1016/j.joca.2014.03.011
Sun, L., Akiyama, K., Zhang, H., Yamaza, T., Hou, Y., Zhao, S., et al. (2009). Mesenchymal stem cell transplantation reverses multiorgan dysfunction in systemic lupus erythematosus mice and humans. Stem Cells 27 (6), 1421–1432. doi:10.1002/stem.68
Sun, W., Zhao, C., Li, Y., Wang, L., Nie, G., Peng, J., et al. (2016). Osteoclast-derived microRNA-containing exosomes selectively inhibit osteoblast activity. Cell Discov. 2, 16015. doi:10.1038/celldisc.2016.15
Tan, F., Wang, D., and Yuan, Z. (2020). The fibroblast-like synoviocyte derived exosomal long non-coding RNA H19 alleviates osteoarthritis progression through the miR-106b-5p/TIMP2 Axis. Inflammation 43 (4), 1498–1509. doi:10.1007/s10753-020-01227-8
Tanaka, E., Detamore, M. S., and Mercuri, L. G. (2008). Degenerative disorders of the temporomandibular joint: Etiology, diagnosis, and treatment. J. Dent. Res. 87 (4), 296–307. doi:10.1177/154405910808700406
Tang, S., Chen, P., Zhang, H., Weng, H., Fang, Z., Chen, C., et al. (2021a). Comparison of curative effect of human umbilical cord-derived mesenchymal stem cells and their small extracellular vesicles in treating osteoarthritis. Int. J. Nanomedicine 16, 8185–8202. doi:10.2147/IJN.S336062
Tang, S., Tang, T., Gao, G., Wei, Q., Sun, K., and Huang, W. (2021b). Bone marrow mesenchymal stem cell-derived exosomes inhibit chondrocyte apoptosis and the expression of MMPs by regulating Drp1-mediated mitophagy. Acta Histochem. 123 (8), 151796. doi:10.1016/j.acthis.2021.151796
Tao, S.-C., Yuan, T., Zhang, Y.-L., Yin, W.-J., Guo, S.-C., and Zhang, C.-Q. (2017). Exosomes derived from miR-140-5p-overexpressing human synovial mesenchymal stem cells enhance cartilage tissue regeneration and prevent osteoarthritis of the knee in a rat model. Theranostics 7 (1), 180–195. doi:10.7150/thno.17133
Theill, L. E., Boyle, W. J., and Penninger, J. M. (2002). RANK-L and RANK: T cells, bone loss, and mammalian evolution. Annu. Rev. Immunol. 20, 795–823. doi:10.1146/annurev.immunol.20.100301.064753
Thie, N. M., Prasad, N. G., and Major, P. W. (2001). Evaluation of glucosamine sulfate compared to ibuprofen for the treatment of temporomandibular joint osteoarthritis: A randomized double blind controlled 3 month clinical trial. J. Rheumatol. 28 (6), 1347–1355.
Tofiño-Vian, M., Guillén, M. I., Pérez Del Caz, M. D., Castejón, M. A., and Alcaraz, M. J. (2017). Extracellular vesicles from adipose-derived mesenchymal stem cells downregulate senescence features in osteoarthritic osteoblasts. Oxidative Med. Cell. Longev. 2017, 1–12. doi:10.1155/2017/7197598
Toghraie, F. S., Chenari, N., Gholipour, M. A., Faghih, Z., Torabinejad, S., Dehghani, S., et al. (2011). Treatment of osteoarthritis with infrapatellar fat pad derived mesenchymal stem cells in Rabbit. Knee 18 (2), 71–75. doi:10.1016/j.knee.2010.03.001
Toller, P. A. (1973). Osteoarthrosis of the mandibular condyle. Br. Dent. J. 134 (6), 223–231. doi:10.1038/sj.bdj.4802982
Valadi, H., Ekström, K., Bossios, A., Sjöstrand, M., Lee, J. J., and Lötvall, J. O. (2007). Exosome-mediated transfer of mRNAs and microRNAs is a novel mechanism of genetic exchange between cells. Nat. Cell Biol. 9 (6), 654–659. doi:10.1038/ncb1596
van Buul, G. M., Villafuertes, E., Bos, P. K., Waarsing, J. H., Kops, N., Narcisi, R., et al. (2012). Mesenchymal stem cells secrete factors that inhibit inflammatory processes in short-term osteoarthritic synovium and cartilage explant culture. Osteoarthr. Cartil. 20 (10), 1186–1196. doi:10.1016/j.joca.2012.06.003
Varela-Eirín, M., Carpintero-Fernández, P., Guitián-Caamaño, A., Varela-Vázquez, A., García-Yuste, A., Sánchez-Temprano, A., et al. (2022). Extracellular vesicles enriched in connexin 43 promote a senescent phenotype in bone and synovial cells contributing to osteoarthritis progression. Cell Death Dis. 13 (8), 681. doi:10.1038/s41419-022-05089-w
Wang, R., Jiang, W., Zhang, L., Xie, S., Zhang, S., Yuan, S., et al. (2020). Intra-articular delivery of extracellular vesicles secreted by chondrogenic progenitor cells from MRL/MpJ superhealer mice enhances articular cartilage repair in a mouse injury model. Stem Cell Res. Ther. 11 (1), 93. doi:10.1186/s13287-020-01594-x
Wang, R., and Xu, B. (2021). TGF-β1-modified MSC-derived exosomal miR-135b attenuates cartilage injury via promoting M2 synovial macrophage polarization by targeting MAPK6. Cell Tissue Res. 384 (1), 113–127. doi:10.1007/s00441-020-03319-1
Wang, X., Chen, Y., Zhao, Z., Meng, Q., Yu, Y., Sun, J., et al. (2018). Engineered exosomes with ischemic myocardium-targeting peptide for targeted therapy in myocardial infarction. J. Am. Heart Assoc. 7 (15), e008737. doi:10.1161/JAHA.118.008737
Wang, Y., He, S.-H., Liang, X., Zhang, X.-X., Li, S.-S., and Li, T.-F. (2021a). ATF4-modified serum exosomes derived from osteoarthritic mice inhibit osteoarthritis by inducing autophagy. IUBMB Life 73 (1), 146–158. doi:10.1002/iub.2414
Wang, Z., Yan, K., Ge, G., Zhang, D., Bai, J., Guo, X., et al. (2021b). Exosomes derived from miR-155-5p-overexpressing synovial mesenchymal stem cells prevent osteoarthritis via enhancing proliferation and migration, attenuating apoptosis, and modulating extracellular matrix secretion in chondrocytes. Cell Biol. Toxicol. 37 (1), 85–96. doi:10.1007/s10565-020-09559-9
Waterman, R. S., Tomchuck, S. L., Henkle, S. L., and Betancourt, A. M. (2010). A new mesenchymal stem cell (MSC) paradigm: Polarization into a pro-inflammatory MSC1 or an immunosuppressive MSC2 phenotype. PloS One 5 (4), e10088. doi:10.1371/journal.pone.0010088
Wei, M., Gao, X., Liu, L., Li, Z., Wan, Z., Dong, Y., et al. (2020). Visceral adipose tissue derived exosomes exacerbate colitis severity via pro-inflammatory MiRNAs in high fat diet fed mice. ACS Nano 14 (4), 5099–5110. doi:10.1021/acsnano.0c01860
Weng, Y., Liu, Y., Du, H., Li, L., Jing, B., Zhang, Q., et al. (2017). Glycosylation of DMP1 is essential for chondrogenesis of condylar cartilage. J. Dent. Res. 96 (13), 1535–1545. doi:10.1177/0022034517717485
Wislet-Gendebien, S., Poulet, C., Neirinckx, V., Hennuy, B., Swingland, J. T., Laudet, E., et al. (2012). In vivo tumorigenesis was observed after injection of in vitro expanded neural crest stem cells isolated from adult bone marrow. PloS One 7 (10), e46425. doi:10.1371/journal.pone.0046425
Wu, J., Kuang, L., Chen, C., Yang, J., Zeng, W.-N., Li, T., et al. (2019). miR-100-5p-abundant exosomes derived from infrapatellar fat pad MSCs protect articular cartilage and ameliorate gait abnormalities via inhibition of mTOR in osteoarthritis. Biomaterials 206, 87–100. doi:10.1016/j.biomaterials.2019.03.022
Wu, L., Leijten, J. C. H., Georgi, N., Post, J. N., van Blitterswijk, C. A., and Karperien, M. (2011). Trophic effects of mesenchymal stem cells increase chondrocyte proliferation and matrix formation. Tissue Eng. Part A 17 (9-10), 1425–1436. doi:10.1089/ten.TEA.2010.0517
Wu, X., Crawford, R., Xiao, Y., Mao, X., and Prasadam, I. (2021). Osteoarthritic subchondral bone release exosomes that promote cartilage degeneration. Cells 10 (2), 251. doi:10.3390/cells10020251
Wu, Y., Li, J., Zeng, Y., Pu, W., Mu, X., Sun, K., et al. (2022). Exosomes rewire the cartilage microenvironment in osteoarthritis: From intercellular communication to therapeutic strategies. Int. J. Oral Sci. 14 (1), 40. doi:10.1038/s41368-022-00187-z
Xu, B., Zhang, Y., Du, X.-F., Li, J., Zi, H.-X., Bu, J.-W., et al. (2017). Neurons secrete miR-132-containing exosomes to regulate brain vascular integrity. Cell Res. 27 (7), 882–897. doi:10.1038/cr.2017.62
Xu, L., Flahiff, C. M., Waldman, B. A., Wu, D., Olsen, B. R., Setton, L. A., et al. (2003). Osteoarthritis-like changes and decreased mechanical function of articular cartilage in the joints of mice with the chondrodysplasia gene (cho). Arthritis Rheum. 48 (9), 2509–2518. doi:10.1002/art.11233
Xu, T., Xu, M., Bai, J., Lin, J., Yu, B., Liu, Y., et al. (2019). Tenocyte-derived exosomes induce the tenogenic differentiation of mesenchymal stem cells through TGF-β. Cytotechnology 71 (1), 57–65. doi:10.1007/s10616-018-0264-y
Xu, X., Liang, Y., Li, X., Ouyang, K., Wang, M., Cao, T., et al. (2021). Exosome-mediated delivery of kartogenin for chondrogenesis of synovial fluid-derived mesenchymal stem cells and cartilage regeneration. Biomaterials 269, 120539. doi:10.1016/j.biomaterials.2020.120539
Xuan, Z., Yu, W., Dou, Y., and Wang, T. (2020). Efficacy of platelet-rich plasma for low back pain: A systematic review and meta-analysis. J. Neurol. Surg. A. Cent. Eur. Neurosurg. 81 (6), 529–534. doi:10.1055/s-0040-1709170
Yaghoubi, Y., Movassaghpour, A., Zamani, M., Talebi, M., Mehdizadeh, A., and Yousefi, M. (2019). Human umbilical cord mesenchymal stem cells derived-exosomes in diseases treatment. Life Sci. 233, 116733. doi:10.1016/j.lfs.2019.116733
Yamaguchi, T., Nakaoka, H., Yamamoto, K., Fujikawa, T., Kim, Y. I., Yano, K., et al. (2014). Genome-wide association study of degenerative bony changes of the temporomandibular joint. Oral Dis. 20 (4), 409–415. doi:10.1111/odi.12141
Yang, T., Zhang, J., Cao, Y., Zhang, M., Jing, L., Jiao, K., et al. (2014). Decreased bone marrow stromal cells activity involves in unilateral anterior crossbite-induced early subchondral bone loss of temporomandibular joints. Archives Oral Biol. 59 (9), 962–969. doi:10.1016/j.archoralbio.2014.05.024
Yang, Z., Shi, J., Xie, J., Wang, Y., Sun, J., Liu, T., et al. (2020). Large-scale generation of functional mRNA-encapsulating exosomes via cellular nanoporation. Nat. Biomed. Eng. 4, 69–83. doi:10.1038/s41551-019-0485-1
Yu, B., Sondag, G. R., Malcuit, C., Kim, M., and Safadi, F. F. (2016). Macrophage-associated osteoactivin/GPNMB mediates mesenchymal stem cell survival, proliferation, and migration via a CD44-dependent mechanism. J. Cell. Biochem. 17 (7), 1511–1521. doi:10.1002/jcb.25394
Zhang, B., Tian, X., Qu, Z., Hao, J., and Zhang, W. (2022a). Hypoxia-preconditioned extracellular vesicles from mesenchymal stem cells improve cartilage repair in osteoarthritis. Membranes 12 (2), 225. doi:10.3390/membranes12020225
Zhang, F.-X., Liu, P., Ding, W., Meng, Q.-B., Su, D.-H., Zhang, Q.-C., et al. (2021). Injectable Mussel-Inspired highly adhesive hydrogel with exosomes for endogenous cell recruitment and cartilage defect regeneration. Biomaterials 278, 121169. doi:10.1016/j.biomaterials.2021.121169
Zhang, M., Yang, H., Lu, L., Wan, X., Zhang, J., Zhang, H., et al. (2017). Matrix replenishing by BMSCs is beneficial for osteoarthritic temporomandibular joint cartilage. Osteoarthr. Cartil. 25 (9), 1551–1562. doi:10.1016/j.joca.2017.05.007
Zhang, S., Chuah, S. J., Lai, R. C., Hui, J. H. P., Lim, S. K., and Toh, W. S. (2018). MSC exosomes mediate cartilage repair by enhancing proliferation, attenuating apoptosis and modulating immune reactivity. Biomaterials 156, 16–27. doi:10.1016/j.biomaterials.2017.11.028
Zhang, S., Teo, K. Y. W., Chuah, S. J., Lai, R. C., Lim, S. K., and Toh, W. S. (2019). MSC exosomes alleviate temporomandibular joint osteoarthritis by attenuating inflammation and restoring matrix homeostasis. Biomaterials 200, 35–47. doi:10.1016/j.biomaterials.2019.02.006
Zhang, Y., Wang, X., Chen, J., Qian, D., Gao, P., Qin, T., et al. (2022b). Exosomes derived from platelet-rich plasma administration in site mediate cartilage protection in subtalar osteoarthritis. J. Nanobiotechnology 20 (1), 56. doi:10.1186/s12951-022-01245-8
Zheng, L., Pi, C., Zhang, J., Fan, Y., Cui, C., Zhou, Y., et al. (2018). Aberrant activation of latent transforming growth factor-β initiates the onset of temporomandibular joint osteoarthritis. Bone Res. 6, 26. doi:10.1038/s41413-018-0027-6
Zheng, L., Wang, Y., Qiu, P., Xia, C., Fang, Y., Mei, S., et al. (2019). Primary chondrocyte exosomes mediate osteoarthritis progression by regulating mitochondrion and immune reactivity. Nanomedicine Lond. Engl. 14 (24), 3193–3212. doi:10.2217/nnm-2018-0498
Zhou, Q.-F., Cai, Y.-Z., and Lin, X.-J. (2020a). The dual character of exosomes in osteoarthritis: Antagonists and therapeutic agents. Acta Biomater. 105, 15–25. doi:10.1016/j.actbio.2020.01.040
Zhou, X., Li, Z., Qi, M., Zhao, P., Duan, Y., Yang, G., et al. (2020b). Brown adipose tissue-derived exosomes mitigate the metabolic syndrome in high fat diet mice. Theranostics 10 (18), 8197–8210. doi:10.7150/thno.43968
Zhou, Y., Ming, J., Li, Y., Li, B., Deng, M., Ma, Y., et al. (2021). Exosomes derived from miR-126-3p-overexpressing synovial fibroblasts suppress chondrocyte inflammation and cartilage degradation in a rat model of osteoarthritis. Cell Death Discov. 7 (1), 37. doi:10.1038/s41420-021-00418-y
Zhu, Y., Wang, Y., Zhao, B., Niu, X., Hu, B., Li, Q., et al. (2017). Comparison of exosomes secreted by induced pluripotent stem cell-derived mesenchymal stem cells and synovial membrane-derived mesenchymal stem cells for the treatment of osteoarthritis. Stem Cell Res. Ther. 8 (1), 64. doi:10.1186/s13287-017-0510-9
Keywords: temporomandibular joint osteoarthritis, Exosome, mesenchymal stem cell, therapeutic strategy, optimization in bioengineering
Citation: Yuan W, Wu Y, Huang M, Zhou X, Liu J, Yi Y, Wang J and Liu J (2022) A new frontier in temporomandibular joint osteoarthritis treatment: Exosome-based therapeutic strategy. Front. Bioeng. Biotechnol. 10:1074536. doi: 10.3389/fbioe.2022.1074536
Received: 19 October 2022; Accepted: 14 November 2022;
Published: 25 November 2022.
Edited by:
Feng-Juan Lyu, South China University of Technology, ChinaReviewed by:
Fengyuan Zhao, Peking University Third Hospital, ChinaCarlo Tremolada, Manchester Metropolitan University, United Kingdom
Copyright © 2022 Yuan, Wu, Huang, Zhou, Liu, Yi, Wang and Liu. This is an open-access article distributed under the terms of the Creative Commons Attribution License (CC BY). The use, distribution or reproduction in other forums is permitted, provided the original author(s) and the copyright owner(s) are credited and that the original publication in this journal is cited, in accordance with accepted academic practice. No use, distribution or reproduction is permitted which does not comply with these terms.
*Correspondence: Jin Liu, bGl1amluQHNjdS5lZHUuY24=; Jun Wang, d2FuZ2p1bnZAc2N1LmVkdS5jbg==