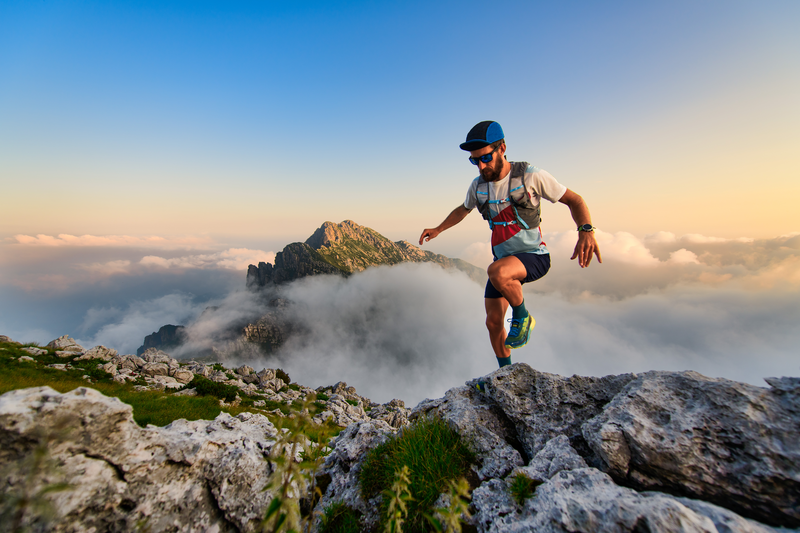
94% of researchers rate our articles as excellent or good
Learn more about the work of our research integrity team to safeguard the quality of each article we publish.
Find out more
ORIGINAL RESEARCH article
Front. Bioeng. Biotechnol. , 17 November 2022
Sec. Biomaterials
Volume 10 - 2022 | https://doi.org/10.3389/fbioe.2022.1050495
This article is part of the Research Topic Recent Advances in Bioceramics for Health View all 8 articles
Background: Iliac crest bone graft (ICBG) is considered the gold standard for spine surgical procedures to achieve a successful fusion due to its known osteoinductive and osteoconductive properties. However, complications related to harvesting procedure and donor site morbidity have been largely reported in the literature, favoring the development of a wide range of alternative products to be used as bone graft extenders or substitutes for spine fusion. Among all, ceramic-based biomaterials have been widely studied and employed in the last years as bone graft substitutes.
Methods: We report here the results of a prospective pilot study aimed to evaluating the grade of ossification obtained by the use of an Mg-doped hydroxyapatite (HA) product to achieve postero-lateral fusion in degenerative spine diseases.
Results: Results show a successful degree of fusion of about 62% at the 12-month follow-up and an improvement of quality of life and health status following surgery, as evaluated by clinical scores (ODI, VAS, and EQ-5L). No adverse events related to the material were reported.
Conclusion: The present pilot study shows the effectiveness and the safety profile of an Mg-doped HA bone graft substitute used to achieve postero-lateral fusion in the treatment of degenerative spine diseases, laying down the basis for further larger clinical investigations.
During the past few decades, spinal fusion procedures have significantly increased to treat a wide range of spinal disorders of degenerative, traumatic, and oncological origin (Reisener et al., 2020). Autologous bone graft from the iliac crest (ICBG) has been classically used to provide spinal fusion and immediate structural support and is still considered the “gold standard” because of the osteoconductive and osteoinductive properties, which allow excellent fusion rates (Banwart et al., 1995; Arrington et al., 1996; Ahlmann et al., 2002; Dimar et al., 2009; Kim et al., 2009). Nevertheless, autologous bone harvesting has shown limitations and significant drawbacks, including superficial infection, wound complications, sensory abnormalities, persistent pain, and hematomas, as well as need for reoperation, scarring, and graft site fracture (Dimar et al., 2009; Kim et al., 2009; Miyazaki et al., 2009; Gruskay et al., 2014).
To overcome all these limitations, different alternatives have been developed, clinically tested, and currently available on the market, including allograft (Park et al., 2013; Gupta et al., 2015), bone morphogenetic protein, demineralized bone matrix (DBM) (Abdullah et al., 2011; Hsu et al., 2012; Fischer et al., 2013), BMPs (Carragee et al., 2011; Kannan et al., 2015), mesenchymal stem cells (MSCs), and bioceramics (Fischer et al., 2013).
Although extensively studied, clinical data available for all these materials are often heterogeneous in quality, type of study and evaluations performed, and conclusions reached (Miyazaki et al., 2009; Abdullah et al., 2011; Alsaleh et al., 2012; Hsu et al., 2012).
Bioactive ceramics (i.e., tricalcium phosphate, calcium phosphate, calcium sulfate, hydroxyapatite, and collagen) (Nickoli and Hsu, 2014) are synthetic products which have been developed as osteoconductive scaffolds with chemico-physical properties very similar to the mineral component of human bone (Korovessis et al., 2005; Alsaleh et al., 2012; Gao et al., 2014; Kaiser et al., 2014). These biomaterials are able to stimulate cell proliferation and differentiation and bone tissue regeneration/remodeling while undergoing in the meanwhile slow resorption. Among all, HA is the most similar, for the chemico-physical composition and stoichiometric formula (Ca/P ratio = 1.67), to the mineralized phase of human bone.
To further improve their features, new generation HA-based biomaterials have been developed with superior properties, strongly influenced by the nature of components, the composition, and the morphology. Calcium ions, phosphate ions, and hydroxyl groups can be replaced by other ions, and studies on animal models have demonstrated that HA-substituted ions enable the crystal cell structure of ceramic derivatives to become unstable and more biologically active, thereby promoting rapid cell-mediated material resorption, new bone formation, and remodeling (Nandi et al., 2010).
We previously reported the results of a pre-clinical study performed using an HA-doped bioceramic (SintLife, Finceramica, Faenza, S.p.A., Italy) enriched in magnesium (Mg) to induce spinal fusion in an animal model (Barbanti Bròdano et al., 2014).
In this study, we report the results of a prospective clinical study performed using the same Mg-substituted HA bone graft in a cohort of patients undergoing postero-lateral fusion for degenerative lumbar spine diseases.
The primary aim of the study was the evaluation of the degree of fusion and new bone formation achieved by the use of the bone graft substitute SintLife. The secondary aim was the assessment of the patients’ state of health by the evaluation of clinical scores (Oswestry Disability Index, visual analog scale, and EuroQol (EQ-5D)) in the post-operative period. The third aim was to study the SintLife safety profile in human subjects.
A prospective pilot clinical study was conducted at our center from February 2017 to March 2020, following the approval of the Local Ethics Committee (protocol number 0001112: “Use of the bone substitute SintLife in spinal surgical procedures. A pilot study”). The study was performed in line with the principles of the Declaration of Helsinki. The study design was a pilot study because the bone graft substitute was not used routinely in our center for spinal surgery, and the pilot study allowed evaluating the results obtained in a small scale, providing the basis for larger investigations. Consequently, the sample size was calculated according to the standard clinical activities ordinarily carried out by the center involved in the study and by the consideration reported in the following paragraph, for which the total number of 20 subjects involved was considered sufficient for the assessment of the variables under analysis. This is not a comparative study, and comparison shall be performed only with literature data.
Consecutive patients who had indications of single- or multi-level postero-lateral spinal fusion due to degenerative lumbar spine diseases were screened to be included in the study, after providing written informed consent. The enrolment period was from February 2017 to September 2019, and patients were followed up for 18 months, with the exception of three patients who dropped out because of adverse events occurred after surgery.
Specific inclusion criteria were as follows: skeletally mature subjects, at least 18 years of age at the time of surgery with symptomatic spinal degenerative disc disease requiring postero-lateral fusion at the L1–S1 tract and patients participating in the study who provided informed signed consent. Patient exclusion criteria were applied in the case of local or systemic infections, inflammatory or autoimmune disease, hypercalcemia, coagulation/metabolic disorders, insulin-dependent diabetes, allergic to calcium phosphate salts, drugs, or medical devices, tumor pathologies, alcohol or drug abuse, pregnancy, pharmacologic therapies which might have influenced bone regeneration processes (i.e., chemotherapeutic drugs), and revision surgery.
A conventional posterior approach for lumbar spinal fusion was performed. After the positioning of pedicle screws, decompression of the cauda and nerve roots was achieved with hemilaminectomy and foraminotomy. SintLife was apposed on the hemi-laminae and transverse process on the contralateral side of the hemilaminectomy. On the hemilaminectomy side, foramino-arthrectomy was performed to insert the interbody fusion cage; therefore, on that side, the dura and the nerve roots were exposed, and SintLife could not be put there.
SintLife is a CE-marked, implantable, non-active bone graft substitute, available in the form of a paste, composed of biomimetic hydroxyapatite (HA) enriched in magnesium ions (Mg-HA) in a similar amount as in the one found in human bones. Mg2+ ions are introduced into the HA crystalline cell in the same position and percentage found in the mineral phase of human bone. Previous studies have been demonstrated that the presence of Mg2+ ions deform the structure of the crystalline cell of HA, making it unstable and biologically active, thus favoring new bone formation, bone remodeling, and rapid cell-mediated resorption of the material. Furthermore, Mg-HA actively interacts with water molecules to rapidly capture key proteins involved in osteogenesis. The specific chemical and biomimetic composition, structured geometry, and surface properties allow SintLife to be remodeled and reabsorbed by osteoprogenitor cells in a physiologically adequate period (6–18 months) to promote quick and effective physiological bone regeneration. During the remodeling phase, osteoclast reabsorption activity has been observed around the bone graft material, up to the complete regeneration of new bone (Barbanti Bròdano et al., 2014).
Radiographic and clinical data were collected before surgery and at 6- and 12–18-month follow-ups. Radiographic images were used to determine the degree of fusion and bone regeneration. Clinical scores such as the Oswestry Disability Index (ODI), the visual analog scale (VAS), and the EuroQoL-5L (EQ-5L) were used to evaluate the improvement of the patient’s health quality following surgery.
Bone regeneration and the degree of fusion were determined by an independent radiologist on CT scan analysis. Bone regeneration was identified as the presence of a continuous trabecular bone bridge together with the lack of radiolucency as assessed by diagnostic imaging (CT scan), and it was evaluated by Brantigan classification (Table 1), which assesses the spinal fusion from grade A (pseudoarthrosis) to grade E (certain fusion).
Clinical evaluations were collected before surgery and at follow-up visits using the following scores: the Oswestry Disability Index (ODI) for the quantification of patient’s disability for low back pain, the visual analog scale (VAS) for patient’s pain intensity, and EuroQol (EQ-5D) for measurement of the quality of life. The incidence of adverse events, complications, unattended reactions, and incidents was recorded.
No sample size calculation was performed because of the study design (i.e., pilot study). Considering the small number of patients treated and the heterogeneity of the patient population included in the study, no specific statistical analyses have been carried out. Descriptive statistical analysis has been provided for clinical scores (VAS, ODI, and EQ-5D).
Results are presented as the number (n), mean ± standard deviation, and percentage, as appropriate. Changes from the baseline to follow-up scores were analyzed using Student’s t-test. The level of statistical significance was set at p < 0.05. SAS software 9.201 was used. Assessment of fusion and the incidence and type of any adverse event recorded have been reported.
Because of enrolment delays due to the COVID-19 pandemic, the expected sample size of 20 subjects was not reachable, and a final number of 16 subjects were enrolled for the study. The flow chart of the study is represented in Figure 1. In this figure, the number of patients included in the analysis at each time point and the reason for dropout are reported. All the enrolled patients signed the written informed consent for the study and underwent postero-lateral fusion for degenerative lumbar spine diseases.
As reported in Table 2, there were seven males (44%) and nine females (56%), with a mean age of about 54.2 years (age range: 34–80); 37.5% of subjects were aged between 55 and 64 years; 31.3% (5) were aged between 35 and 44 years. The majority of patients were treated for spondylolisthesis (43.9%) and degenerative disc disease (37.5%). An interbody cage was implanted in 13/16 patients through a transforaminal lumbar interbody fusion (TLIF) approach on the side of the hemilaminectomy.
Concerning the length of spinal fusion, nine patients (56%) had one fusion level, three patients (19%) had two fusion levels, and four patients (25%) had three fusion levels (Table 2). Three patients, all of them treated at two levels, were not evaluated at the follow-up because of inflammatory reactions occurring after surgery. Thus, spinal fusion was evaluated by an independent radiologist on CT scans performed at 12-month follow-up in 21 out of 27 treated levels. For each level, the fusion rate was assessed at the side where SintLife was used, and the results obtained according to Brantigan classification are reported in Table 3. The successful degree of fusion was about 62% (considering the levels with C, D, and E grades) (Table 3). The results represented by the Brantigan score were confirmed by quantification of the structural homogeneity of the bone graft area (ROI) (data not shown). Two cases of spinal arthrodesis with SintLife are reported in Figure 2 and Figure 3.
TABLE 3. Evaluation of the fusion status according to the Brantigan score in the presence of SintLife.
FIGURE 2. CT scan performed at 18-month follow-up, showing radiographic fusion at levels L4–L5 and L5–S1 where SintLife was used.
FIGURE 3. CT scan performed at 18-month follow-up, showing the absence of fusion (pseudoarthrosis) at levels L4–L5 and L5–S1 where SintLife was used.
The first case was a 46-year-old female affected by degenerative disc disease L4–L5 and L5–S1 and treated with posterior stabilization L4–S1, PLIF L4–L5, and L5–S1. SintLife was apposed on the left side at L4–L5 and L5–S1 levels. At 18-month follow-up, a complete fusion has been detected at CT scan evaluation (grade E) (Figure 2), associated with significant improvement of clinical outcomes (VAS decreased from score 9 to score 1; ODI decreased from score 66 to score 31; and EQ-5D increased from 20 to 90).
The second case was a 61-year-old female affected by degenerative disc disease L4–L5 and treated with PLIF L4–L5. SintLife was apposed on the left side at the L4–L5 level. At 18-month follow-up, no fusion was detected (grade A) (Figure 3). However, a clinical outcome improvement was recorded (VAS decreased from score 5 to score 2; ODI decreased from score 30 to score 16; and EQ-5D increased from 60 to 80).
Considering all the patients, the VAS score at baseline was 7.2 ± 1.8, and it decreased to 4.7 ± 1.69 at 6-month follow-up, while it remained stable at 12–18-month follow-up (4.8 ± 2.4), with a statistically significant difference between baseline and follow-up scores, starting from 6 months after surgery (p < 0.0004) (Figure 4).
FIGURE 4. Plot of visual analog scale (VAS) scores evaluated pre-operatively, at 6- and 12–18-month follow-ups. The red asterisk depicts a significant difference between post-operative and pre-operative values.
The Oswestry Disability Index at baseline was 48.3 ± 14.5, and it decreased to 31.6 ± 14.7 at 6-month follow-up and remained stable at 12–18-month follow-up (33.3 ± 18.3), with a statistically significant difference between baseline and follow-up scores, starting from 6 months after surgery (p < 0.0006) (Figure 5). The EQ-5L score at baseline was 45 ± 15, and it increased to 62 ± 13 at 6-month follow-up, and it was 64.5 ± 22 at 12–18-month follow-up, with a statistically significant difference between baseline and follow-up scores, starting from 6 months after surgery (p < 0.0003) (Figure 6).
FIGURE 5. Oswestry Disability Index (ODI) scores evaluated pre-operatively, at 6-month and 12–18-month follow-ups. The red asterisk depicts a significant difference between post-operative and pre-operative values.
FIGURE 6. EuroQoL-5D (EQ-5D) scores evaluated pre-operatively, at 6-month and 12–18-month follow-ups. The red asterisk depicts a significant difference between post-operative and pre-operative values.
Differences between ODI, VAS, and EQ-5D scores at 12–18-month follow-up, as compared to 6-month FU values, were not statistically significant, and a sensitive analysis performed by considering only those patients who underwent all three follow-up visits (n = 13) confirmed the trend.
Three adverse events (i.e., inflammatory reactions) were recorded in the follow-up period, with one requiring surgical debridement and the remaining treated with anti-inflammatory agents. According to the surgeon’s surgical notes, all the events were not classified as related or possibly related to the device. Two events were indeed rather related to inflammatory phenomena patients already suffered from. This was confirmed by elevated pre-operative values of C-reactive protein, as revealed by hematological investigations. The third event occurred after contamination of the surgical wound by Enterococcus faecalis, as ascertained by bacteriological tests.
No serious adverse events (i.e., immunological reactions, early/late infections, deep wound infection, implant mobilization, and early bone graft resorption) were recorded.
Ceramic-based bone graft substitutes (CaP, B-TCP, and hydroxyapatite) have been widely utilized since mid-1950s to reduce the need of iliac crest bone harvesting, given the complications associated with this procedure. As a family, ceramics can widely vary according to the different compositions, manufacturing, porosity, and structure.
Hydroxyapatite, a naturally occurring mineral form of calcium apatite with the formula Ca5(PO4)3(OH), has a stoichiometric formula (Ca/P ratio = 1.67) and a chemico-physical composition which are very similar to the mineralized phase of human bone. The porous structure of HA, with its macropore network and the micropore interconnection, induces rapid vascular and mesenchymal invasion, providing a specific cell flow and the optimal environment for cells to attach, proliferate, and finally differentiate into functional osteoblasts. HA shows high biomimetic properties, osteoconductive potential, and excellent biocompatibility. Through the extended surface-hydrated layer, linked to the crystal nanometer size, HA-based materials are able to exchange surface ions with the surrounding fluids, as needed to fulfill primary biological functions. Because of these properties, throughout years, HA-based bone grafts have evolved as “new generation” biomaterials able to “mimic” the osteoregenerative processes typically found in the human bone mineral turnover. This was performed by the addition of specific ionic species (cations or anions) which have been progressively introduced into the formulation of synthetic apatites. Magnesium is certainly one of the most important bivalent ions associated with biological apatite: it is one of the most abundant minerals in the human body, and approximately 50% of Mg2+ is naturally present in the composition of bone tissue. Mg2+ enables the HA crystal cell structure to become unstable and more biologically active, promoting rapid cell-mediated material resorption, promoting new bone formation, and remodeling by cross-talking with progenitor cells at the molecular level (Landi et al., 2008; Kurien et al., 2013; Barbanti Bròdano et al., 2014).
In the last few years, we had experience with an Mg2+-enriched HA biomaterial (SintLife) and tested its potentiality as a bone graft substitute in in vitro experiments and in in vivo animal models. Since 2006, Landi et al. (2006) demonstrated that Mg-substituted hydroxyapatites improved the behavior of mesenchymal stem cells in terms of adhesion, proliferation, and metabolic activation compared to stoichiometric HA.
More recently, in vitro experiments revealed an active interaction between SintLife and human mesenchymal stem cells (hMSCs), with an improvement of cell metabolic activities and bone remodeling (Barbanti Brodano et al., 2012; Manfrini et al., 2013). An in vivo study on sheep treated for postero-lateral fusion with SintLife or autologous bone showed the deposition of new bone tissue provided by SintLife, without qualitative and quantitative differences with respect to new bone formed with autologous bone graft (Barbanti Bròdano et al., 2014). Other studies (Pola et al., 2011; Sartori et al., 2014) demonstrated good osteointegration and deposition of new bone tissue using SintLife, thus confirming our previous findings.
With the present work, we took another step forward and investigated the use of SintLife in human spinal arthrodesis, with the aim to confirm the performance of the bone graft substitute.
The present prospective clinical study showed 63% of solid fusion by the use of an Mg-HA bone graft substitute (SintLife) alone for spinal arthrodesis.
To date, only few studies have investigated the fusion rate of CaP derivative biomaterials (i.e., HA, doped-HA, B-TCP, etc.) used alone, as compared to the gold standard autologous bone. Alimi et al. (2017) showed an overall fusion rate of 80% in degenerative disease patients treated with a silicate-substituted calcium phosphate (Si-CaP) ceramic bone graft in spinal fusion procedures.
At 6-month follow-up, Jenis and Banco (2010) showed 35% of fusion with silicate calcium phosphate, which increased to 76.2% and 76.5% at 12 and 24 months, respectively. Similar results (i.e., 90% of bony fusion after 12 months) were reported by Nagineni et al. (2012) in 2012.
In a review, Buser et al. (2016) showed an overall fusion rate of 70%–90% with the use of different hydroxyapatite-based bone grafts used alone, as compared to autograft. Other relevant literature reports high rates of fusion (of about 70–100%) obtained by the use of HA-based materials combined with autologous bone graft or autograft derivatives (BMA, ICBG, LAG, etc.) (Ransford et al., 1998; Delécrin et al., 2000; Thalgott et al., 2002; McConnell et al., 2003; Korovessis et al., 2005; Nickoli and Hsu, 2014).
Despite the reduced number of subjects enrolled in the study, results from this prospective study allow providing reliable considerations in terms of performance and safety of the device under analysis. SintLife has shown similar fusion rates, as compared to other CaP-derivative bone graft substitutes used alone.
Clinical parameters (ODI, VAS, and EQ-5L) used to measure the patient’s disability, pain, and quality of life assessed at 6 months and then at 12–18 months have shown a significant improvement. The improvement of clinical parameters at long follow-up periods is strictly linked to a solid fusion achieved, thereby confirming the effectiveness of the Mg-HA bone graft SintLife in achieving spinal fusions.
The safety profile of SintLife is confirmed by the lack of adverse events related to the material, as previously demonstrated by a post-marketing surveillance analysis (Barbanti Brodano et al., 2015) and confirmed in the present study. Of the three inflammatory conditions recorded in the previous literature reports, few cases of inflammatory reactions were reported following the use of bone graft substitutes for spinal fusion (Carragee et al., 2011; Kurien et al., 2013). These events are always related to previous patients’ clinical inflammatory conditions or hypersensitivity to some components of the device. In the present study, with the exception of one event related to a contamination by Enterococcus faecalis, two cases of imbalanced patients’ inflammatory conditions were identified following hematological analysis, which suggested no correlation between the event and the device, thereby confirming the safety of SintLife in procedures of spinal arthrodesis.
Limitations to the study are mainly related to the low number of subjects enrolled, moreover, conditioned by the worldwide COVID-19 pandemic which drastically reduced surgical activities during the period of the study conduction.
Taking into consideration that future research studies are needed to further investigate SintLife in spinal arthrodesis procedures, the biomaterial has shown a safe and effective profile.
In conclusion, SintLife, a HA bone graft substitute enriched in magnesium ions, has shown a satisfying biocompatibility, a good performance in terms of spinal fusion and improvement of clinical outcomes, and a good safety profile and can represent a good alternative to autologous bone graft.
The raw data supporting the conclusion of this article will be made available by the authors, without undue reservation.
The studies involving human participants were reviewed and approved by the Istituto Ortopedico Rizzoli Ethics Committee. The patients/participants provided their written informed consent to participate in this study.
All authors contributed to the study conception and design. Material preparation, data collection, and analysis were performed by CG, GB, AZ, AN, VC, and GT. The first draft of the manuscript was written by CG and VC, and all authors commented on previous versions of the manuscript. GT revised the manuscript and improved all the sections of the first draft; thus, he was considered the first author along with CG. GT contributed to the final revision of the manuscript. All authors read and approved the final manuscript.
The research was supported by 5x1000- 2019 grant from Italian Ministry of Health.
VC and AN were empolyed by Finceramica Faenza S.p.A.
The remaining authors declare that the research was conducted in the absence of any commercial or financial relationships that could be construed as a potential conflict of interest.
All claims expressed in this article are solely those of the authors and do not necessarily represent those of their affiliated organizations, or those of the publisher, the editors, and the reviewers. Any product that may be evaluated in this article, or claim that may be made by its manufacturer, is not guaranteed or endorsed by the publisher.
BMA, bone marrow aspirate; BMPs, bone morphogenetic proteins; CaP, calcium phosphate; DBM, demineralized bone matrix; HA, hydroxyapatite; hMSCs, human mesenchymal stem cells; ICBG, iliac crest bone graft; LAG, local autograft bone; Mg, magnesium.
Abdullah, K. G., Steinmetz, M. P., Benzel, E. C., and Mroz, T. E. (2011). The state of lumbar fusion extenders. Spine 36 (20), E1328–E1334. doi:10.1097/brs.0b013e318209952b
Ahlmann, E., Patzakis, M., Roidis, N., Shepherd, L., and Holtom, P. (2002). Comparison of anterior and posterior iliac crest bone grafts in terms of harvest-site morbidity and functional outcomes. J. Bone Jt. Surgery-American Volume 84 (5), 716–720. doi:10.2106/00004623-200205000-00003
Alimi, M., Navarro-Ramirez, R., Parikh, K., Njoku, I., Hofstetter, C. P., Tsiouris, A. J., et al. (2017). Radiographic and clinical outcome of silicate-substituted calcium phosphate (Si-CaP) ceramic bone graft in spinal fusion procedures. Clin. Spine Surg. A Spine Publ. 30 (6), E845–E852. doi:10.1097/bsd.0000000000000432
Alsaleh, K. A. M., Tougas, C. A., Roffey, D. M., and Wai, E. K. (2012). Osteoconductive bone graft extenders in posterolateral thoracolumbar spinal fusion: A systematic review. Spine 37 (16), E993–E1000. doi:10.1097/brs.0b013e3182518859
Arrington, E. D., Smith, W. J., Chambers, H. G., Bucknell, A. L., and Davino, N. A. (1996). Complications of iliac crest bone graft harvesting. Clin. Orthop. Relat. Res. 329, 300–309. doi:10.1097/00003086-199608000-00037
Banwart, J. C., Asher, M. A., and Hassanein, R. S. (1995). Iliac crest bone graft harvest donor site morbidity. A statistical evaluation. Spine 20 (9), 1055–1060. doi:10.1097/00007632-199505000-00012
Barbanti Bròdano, G., Giavaresi, G., Lolli, F., Salamanna, F., Parrilli, A., Martini, L., et al. (2014). Hydroxyapatite-based biomaterials versus autologous bone graft in spinal fusion: An in vivo animal study. Spine 39 (11), E661–E668. doi:10.1097/brs.0000000000000311
Barbanti Brodano, G., Mazzoni, E., Tognon, M., Griffoni, C., and Manfrini, M. (2012). Human mesenchymal stem cells and biomaterials interaction: A promising synergy to improve spine fusion. Eur. Spine J. 21 (S1), 3–9. doi:10.1007/s00586-012-2233-z
Barbanti Brodano, G., Griffoni, C., Zanotti, B., Gasbarrini, A., Bandiera, S., Ghermandi, R., et al. (2015). A post-market surveillance analysis of the safety of hydroxyapatite-derived products as bone graft extenders or substitutes for spine fusion. Eur. Rev. Med. Pharmacol. Sci. 19 (19), 3548–3555.
Buser, Z., Brodke, D. S., Youssef, J. A., Meisel, H-J., Myhre, S. L., Hashimoto, R., et al. (2016). Synthetic bone graft versus autograft or allograft for spinal fusion: A systematic review. J. Neurosurg. Spine 25 (4), 509–516. doi:10.3171/2016.1.spine151005
Carragee, E. J., Hurwitz, E. L., and Weiner, B. K. (2011). A critical review of recombinant human bone morphogenetic protein-2 trials in spinal surgery: Emerging safety concerns and lessons learned. Spine J. 11 (6), 471–491. doi:10.1016/j.spinee.2011.04.023
Delécrin, J., Takahashi, S., Gouin, F., and Passuti, N. (2000). A synthetic porous ceramic as a bone graft substitute in the surgical management of scoliosis: A prospective, randomized study. Spine 25 (5), 563–569. doi:10.1097/00007632-200003010-00006
Dimar, J. R., Glassman, S. D., Burkus, J. K., Pryor, P. W., Hardacker, J. W., and Carreon, L. Y. (2009). Two-year fusion and clinical outcomes in 224 patients treated with a single-level instrumented posterolateral fusion with iliac crest bone graft. Spine J. 9 (11), 880–885. doi:10.1016/j.spinee.2009.03.013
Fischer, C. R., Cassilly, R., Cantor, W., Edusei, E., Hammouri, Q., and Errico, T. (2013). A systematic review of comparative studies on bone graft alternatives for common spine fusion procedures. Eur. Spine J. 22 (6), 1423–1435. doi:10.1007/s00586-013-2718-4
Gao, C., Deng, Y., Feng, P., Mao, Z., Li, P., Yang, B., et al. (2014). Current progress in bioactive ceramic scaffolds for bone repair and regeneration. Int. J. Mol. Sci. 15 (3), 4714–4732. doi:10.3390/ijms15034714
Gruskay, J. A., Basques, B. A., Bohl, D. D., Webb, M. L., and Grauer, J. N. (2014). Short-term adverse events, length of stay, and readmission after iliac crest bone graft for spinal fusion. Spine 39 (20), 1718–1724. doi:10.1097/brs.0000000000000476
Gupta, A., Kukkar, N., Sharif, K., Main, B. J., Albers, C. E., and El-Amin, S. F. (2015). Bone graft substitutes for spine fusion: A brief review. World J. Orthop. 6 (6), 449–456. doi:10.5312/wjo.v6.i6.449
Hsu, W., Nickoli, M., Wang, J., Lieberman, J., An, H., Yoon, S., et al. (2012). Improving the clinical evidence of bone graft substitute technology in lumbar spine surgery. Glob. Spine J. 2 (4), 239–248. doi:10.1055/s-0032-1315454
Jenis, L. G., and Banco, R. J. (2010). Efficacy of silicate-substituted calcium phosphate ceramic in posterolateral instrumented lumbar fusion. Spine 35 (20), E1058–E1063. doi:10.1097/brs.0b013e3181df196f
Kaiser, M. G., Groff, M. W., Watters, W. C., Ghogawala, Z., Mummaneni, P. V., Dailey, A. T., et al. (2014). Guideline update for the performance of fusion procedures for degenerative disease of the lumbar spine. Part 16: Bone graft extenders and substitutes as an adjunct for lumbar fusion. J. Neurosurg. Spine 21 (1), 106–132. doi:10.3171/2014.4.spine14325
Kannan, A., Dodwad, S-N. M., and Hsu, W. K. (2015). Biologics in spine arthrodesis. J. Spinal Disord. Tech. 28 (5), 163–170. doi:10.1097/bsd.0000000000000281
Kim, D. H., Rhim, R., Li, L., Martha, J., Swaim, B. H., Banco, R. J., et al. (2009). Prospective study of iliac crest bone graft harvest site pain and morbidity. Spine J. 9 (11), 886–892. doi:10.1016/j.spinee.2009.05.006
Korovessis, P., Koureas, G., Zacharatos, S., Papazisis, Z., and Lambiris, E. (2005). Correlative radiological, self-assessment and clinical analysis of evolution in instrumented dorsal and lateral fusion for degenerative lumbar spine disease. Autograft versus coralline hydroxyapatite. Eur. Spine J. 14 (7), 630–638. doi:10.1007/s00586-004-0855-5
Kurien, T., Pearson, R. G., and Scammell, B. E. (2013). Bone graft substitutes currently available in orthopaedic practice: The evidence for their use. Bone Jt. J. 95-B (5), 583–597. doi:10.1302/0301-620x.95b5.30286
Landi, E., Logroscino, G., Proietti, L., Tampieri, A., Sandri, M., and Sprio, S. (2008). Biomimetic Mg-substituted hydroxyapatite: From synthesis to in vivo behaviour. J. Mat. Sci. Mat. Med. 19 (1), 239–247. doi:10.1007/s10856-006-0032-y
Landi, E., Tampieri, A., Mattioli-Belmonte, M., Celotti, G., Sandri, M., Gigante, A., et al. (2006). Biomimetic Mg- and MgCO3-substituted hydroxyapatites: Synthesis characterization and in vitro behaviour. J. Eur. Ceram. Soc. 26, 2593–2601. doi:10.1016/j.jeurceramsoc.2005.06.040
Manfrini, M., Di Bona, C., Canella, A., Lucarelli, E., Pellati, A., D’Agostino, A., et al. (2013). Mesenchymal stem cells from patients to assay bone graft substitutes. J. Cell. Physiol. 228 (6), 1229–1237. doi:10.1002/jcp.24276
McConnell, J. R., Freeman, B. J. C., Debnath, U. K., Grevitt, M. P., Prince, H. G., and Webb, J. K. (2003). A prospective randomized comparison of coralline hydroxyapatite with autograft in cervical interbody fusion. Spine 28 (4), 317–323. doi:10.1097/01.brs.0000048503.51956.e1
Miyazaki, M., Tsumura, H., Wang, J. C., and Alanay, A. (2009). An update on bone substitutes for spinal fusion. Eur. Spine J. 18 (6), 783–799. doi:10.1007/s00586-009-0924-x
Nagineni, V. V., James, A. R., Alimi, M., Hofstetter, C., Shin, B. J., Njoku, I., et al. (2012). Silicate-substituted calcium phosphate ceramic bone graft replacement for spinal fusion procedures. Spine 37 (20), E1264–E1272. doi:10.1097/brs.0b013e318265e22e
Nandi, S. K., Roy, S., Mukherjee, P., Kundu, B., De, D. K., and Basu, D. (2010). Orthopaedic applications of bone graft & graft substitutes: A review. Indian J. Med. Res. 132, 15–30.
Nickoli, M. S., and Hsu, W. K. (2014). Ceramic-based bone grafts as a bone grafts extender for lumbar spine arthrodesis: A systematic review. Glob. Spine J. 4 (3), 211–216. doi:10.1055/s-0034-1378141
Park, J. J., Hershman, S. H., and Kim, Y. H. (2013). Updates in the use of bone grafts in the lumbar spine. Bull. Hosp. Jt. Dis. 71 (1), 39–48.
Pola, E., Nasto, L. A., Tampieri, A., Lattanzi, W., Di Giacomo, G., Colangelo, D., et al. (2011). Bioplasty for vertebral fractures: Preliminary results of a pre-clinical study on goats using autologous modified skin fibroblasts. Int. J. Immunopathol. Pharmacol. 24 (1), 139–142. doi:10.1177/03946320110241s226
Ransford, A. O., Morley, T., Edgar, M. A., Webb, P., Passuti, N., Chopin, D., et al. (1998). Synthetic porous ceramic compared with autograft in scoliosis surgery. J. Bone Jt. Surg. Br. volume 80 (1), 13–18. doi:10.1302/0301-620x.80b1.0800013
Reisener, M-J., Pumberger, M., Shue, J., Girardi, F. P., and Hughes, A. P. (2020). Trends in lumbar spinal fusion-a literature review. J. Spine Surg. 6 (4), 752–761. doi:10.21037/jss-20-492
Sartori, M., Giavaresi, G., Tschon, M., Martini, L., Dolcini, L., Fiorini, M., et al. (2014). Long-term in vivo experimental investigations on magnesium doped hydroxyapatite bone substitutes. J. Mat. Sci. Mat. Med. 25 (6), 1495–1504. doi:10.1007/s10856-014-5177-5
Keywords: lumbar degenerative disc disease, spinal fusion, bone graft substitute, Brantigan score, bioceramics
Citation: Griffoni C, Tedesco G, Canella V, Nataloni A, Zerbi A, Tosini G, Gasbarrini A and Barbanti-Brodano G (2022) Ceramic bone graft substitute (Mg-HA) in spinal fusion: A prospective pilot study. Front. Bioeng. Biotechnol. 10:1050495. doi: 10.3389/fbioe.2022.1050495
Received: 21 September 2022; Accepted: 28 October 2022;
Published: 17 November 2022.
Edited by:
Monica Sandri, National Research Council (CNR), ItalyReviewed by:
Hans Joerg Meisel, Berufsgenossenschaftliche Kliniken Bergmannstrost Halle, GermanyCopyright © 2022 Griffoni, Tedesco, Canella, Nataloni, Zerbi, Tosini, Gasbarrini and Barbanti-Brodano. This is an open-access article distributed under the terms of the Creative Commons Attribution License (CC BY). The use, distribution or reproduction in other forums is permitted, provided the original author(s) and the copyright owner(s) are credited and that the original publication in this journal is cited, in accordance with accepted academic practice. No use, distribution or reproduction is permitted which does not comply with these terms.
*Correspondence: Cristiana Griffoni, Y3Jpc3RpYW5hLmdyaWZmb25pQGlvci5pdA==
†These authors have contributed equally to this work
Disclaimer: All claims expressed in this article are solely those of the authors and do not necessarily represent those of their affiliated organizations, or those of the publisher, the editors and the reviewers. Any product that may be evaluated in this article or claim that may be made by its manufacturer is not guaranteed or endorsed by the publisher.
Research integrity at Frontiers
Learn more about the work of our research integrity team to safeguard the quality of each article we publish.