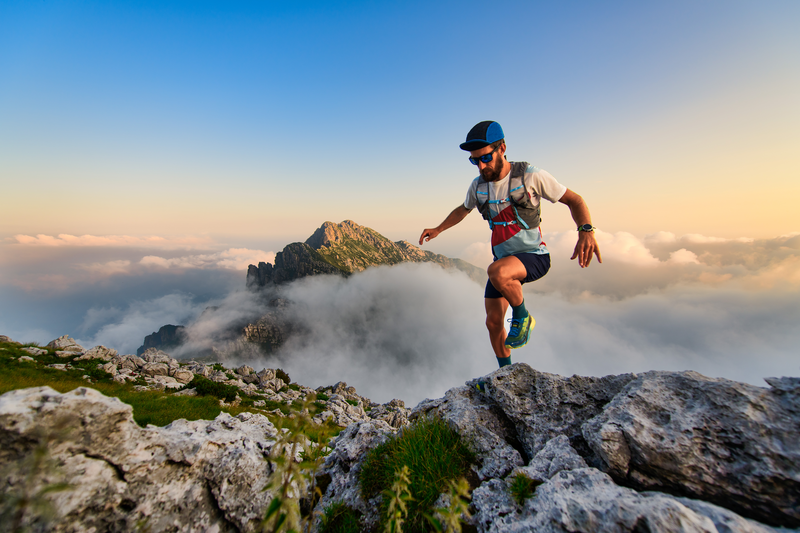
94% of researchers rate our articles as excellent or good
Learn more about the work of our research integrity team to safeguard the quality of each article we publish.
Find out more
GENERAL COMMENTARY article
Front. Bioeng. Biotechnol. , 14 October 2022
Sec. Synthetic Biology
Volume 10 - 2022 | https://doi.org/10.3389/fbioe.2022.1037809
This article is part of the Research Topic Cell-Free Synthetic Biology, Volume II View all 16 articles
This article is a commentary on:
Rapid and Facile Preparation of Giant Vesicles by the Droplet Transfer Method for Artificial Cell Construction
A Commentary on
Rapid and facile preparation of giant vesicles by the droplet transfer method for artificial cell construction
When considered within the well-established field of liposome technology, the droplet transfer method (DTm) might appear as a rather niche technique, but it has literally revolutionized experimental approaches to bottom-up Artificial Cells (ACs) (Stano, 2019). The DTm was firstly used by Vincent Noireaux and Albert Libchaber in their renowned 2004 “bioreactor” paper (Noireaux and Libchaber, 2004) and since then it has been widely applied to the construction of several types of ACs. This method is at the basis of several successful studies, which are not commented here for space limitations. A general discussion on the method can be found in Walde et al. (2010) and in Chapter 1 of “The Giant Vesicle Book” (Dimova et al., 2020).
The timely investigation presented in Frontiers on Bioengineering and Biotechnology by Shimane and Kuruma (2022), to which this commentary is dedicated, proves the importance and the utility of the DTm in order to prepare ACs based on giant liposomes (giant lipid vesicles, GVs). In particular, the Authors have illustrated in a quite detailed and practical way the typical operations required to encapsulate complex macromolecular mixtures–such as the PURE system (Shimizu et al., 2001; Shimizu et al., 2005)—inside GVs in physiological and bioactive conditions, and in high yields. The resulting ACs are then competent for gene expression (a key AC functionality) thanks to the presence of about 100 different macromolecules and dozens of small MW compounds co-captured in the GV lumen. Proteins will be produced in cell-like fashion, i.e., from within.
This commentary is an opportunity to briefly add some considerations on the DTm. We will highlight its major historical developments, the pros and cons, and the still missing mechanistic details.
Demetrios Papahadjopoulos (1934–1998), one of the fathers of liposome research, reported that attempts to prepare liposomes from water-in-oil emulsion droplets date back to the 1970s (Träuble and Grell, 1971; Szoka and Papahadjopoulos, 1980). The aim was the production of conventional small or large unilamellar vesicles (SUVs or LUVs) with an asymmetric membrane. This is in principle possible when the two lipid leaflets of a vesicle membrane are assembled sequentially. Membrane asymmetry was also the goal of more recent studies, due to the Weitz group (Pautot et al., 2003a; Pautot et al., 2003b) and to less cited, but prior, investigators (Zhang et al., 1997; Xiao et al., 1998a; Xiao et al., 1998b).
The shift of interest from LUVs to GVs, and from membrane asymmetry to high encapsulation yield coincided with the above-mentioned Noireaux and Libchaber (2004) paper. This double-leap actually made the difference, and brought the DTm to the attention of the AC community. Indeed the DTm, when the isotonic density gradient strategy is employed1, allows the facile preparation of solute-filled GVs, solving “once for all” (and simultaneously) long standing issues such as efficient macromolecule (co)encapsulation, employment of physiological conditions (concentrated/salty buffers), reduction of the volume of precious solution to be entrapped, reduction of the preparation time, and avoidance of difficult to handle equipment. Moreover, in most cases the resulting GVs appear to be gracefully unilamellar (GUVs) (Chiba et al., 2014).
Subsequent investigations have refined, optimized (Fujii et al., 2014; Moga et al., 2019; Uyeda et al., 2022), scaled-up (Rampioni et al., 2018) and extended the DTm, including the structural analysis of the resulting GVs by flow cytometry (Nishimura et al., 2009), its implementation in microfluidic (Matosevic and Paegel, 2011) or in rotatory capillary devices (Abkarian et al., 2011), but essentially there have been no major noteworthy findings.
The advantages of the DTm have been mentioned above (see also Figure 1A). A further merit consists in the possibility of encapsulating very large particles (e.g., nanoparticles, SUVs/LUVs, organellae, bacteria, etc.) inside GVs. This feature has lead to the easy preparation of nested multi-compartmentalized systems that mimic eukaryotic cells. A pregnant example refers to ACs endowed with natural or artificial energy-producing organellae in their lumen (Berhanu et al., 2019; Altamura et al., 2021). Another valuable feature concerns the reconstitution of membrane proteins from within, in order to obtain otherwise unfeasible physiological-like orientations (Yanagisawa et al., 2011; Altamura et al., 2017).
FIGURE 1. The droplet transfer method (DTm): advantageous features and cues for further investigations. (A) The advent of the DTm in AC research is quite attractive as it allows the formation of solute-filled GVs in a quite easy and inexpensive manner. Additional advantages are the rapidity (few minutes), no need of specific equipment, employment of small volumes (especially important when complex mixtures need to be encapsulated in the vesicle lumen), the possibility of using physiological buffers, and the possibility of entrapping very large particles. (B) Several experimental steps for carrying out the DTm have been recently investigated and optimized, but open questions remain, and they can be the focus of future investigations. For example, it would be important to know more about the lipid dynamics on the oil/water interface, the rate of interface coverage by lipids and the recovery of lipids in the “naked” area after a w/o droplet has been transfer. What factors affect the fraction of w/o droplets that become GVs? Similarly, the very mechanism of w/o droplet formation (via stochastic fragmentation and coagulation) is not well known, as well as the factors determining the droplet size and the solute distribution. Other questions can be: are droplets always covered by a lipid monolayer? What is the amount of residual “oil” in the membrane of resulting GVs? In the case of lipid mixtures, preferential localization of some lipid species on all interfaces (vs those remaining in bulk solution) can occur, and thus, do the obtained GVs have a lipid composition that mirrors the overall lipid composition? Is there any correlation between w/o droplets and GVs size and solute distributions?
The most-often repeated criticism pertains to the possible residual presence of hydrocarbons in the GVs membrane. Researchers interested in studying phenomena strictly dependent on biophysical/biochemical properties of membranes are understandably worried about this issue (e.g., membrane permeability and mechanical properties, dynamics of proteins embedded into the membrane) (e.g., Campillo et al., 2013; Kamiya et al., 2016; Faizi et al., 2022). The “mineral oils” typically used in the DTm actually are cheap heterogeneous mixture of linear, branched, and possibly cyclic hydrocarbons, which can be adsorbed on or absorbed in the membrane (McIntosh et al., 1980; Richens et al., 2015). Pautot et al. (2003b) already recognized this issue, recalling that it could be alleviated by using specific hydrocarbons (e.g., squalene). Fortunately, there are types of investigations that can be safely carried out disregarding the potential presence of residual oil in the GV membrane (clearly, it depends on the aim of the experiment).
A less frequently mentioned aspect is that DTm is not the best choice in studies intended to explore the spontaneous formation of cell-like systems from lipids and solutes. Therefore, when ACs are intended as primitive cell models and the experimental focus is on spontaneous assembly mechanisms in an aqueous environment, other preparation methods should be considered.
The ever increasing number of reports that exploit the production of ACs based on the DTm contrasts with the sporadic study about its mechanistic details. Pautot et al. (2003ab) reported several insightful observations, but because their protocol is quite different from the ones commonly used today, more investigations are needed (see Figure 1B). An elegant study made with a 90° tilted microscope (in order to observe the dynamic of droplet transfer) came from the group of Yoshikawa (Ito et al., 2013).
For example, open questions concern the mechanism of formation and the structure of the w/o droplets (always surrounded by a monolayer?) and the distribution of solutes therein. The dynamics of lipids at the interface between the emulsion phase and the aqueous outer phase and the very mechanism of bilayer formation via adhesion of the two lipid leaflets, during the transfer, are key aspects too. Moreover, the case when lipid mixtures are used is not well studied (as well as the usage of non-phospholipid amphiphiles): does the composition of the resulting GV membranes mirrors the overall bulk composition of lipids used in the preparation? (Some lipids could be preferentially included in the membrane).
The author confirms being the sole contributor of this work and has approved it for publication.
The Author is grateful to all students and collaborators that have contributed to the Author’s research on this subject.
The author declares that the research was conducted in the absence of any commercial or financial relationships that could be construed as a potential conflict of interest.
All claims expressed in this article are solely those of the authors and do not necessarily represent those of their affiliated organizations, or those of the publisher, the editors and the reviewers. Any product that may be evaluated in this article, or claim that may be made by its manufacturer, is not guaranteed or endorsed by the publisher.
1The isotonic density gradient was introduced, to the best of our knowledge, by Takagi and collaborators (Hamada et al., 2008; but see also Hadorn and Eggenberger Hotz, 2009), providing a key modification that has definitely led a reliable GV production method. It consists in the usage of isotonic sucrose and glucose solutions (e.g., 200 mM or higher), respectively, as inner and outer aqueous solutions, in order to facilitate the droplet transfer from the top oil phase to the bottom aqueous phase. High concentrations of sugars, however, can affect membrane properties (Andersen et al., 2011). Träuble and Grell (1971) employed instead CsCl gradients, but the resulting vesicles were osmotically sensitive.
Abkarian, M., Loiseau, E., and Massiera, G. (2011). Continuous droplet interface crossing encapsulation (cDICE) for high throughput monodisperse vesicle design. Soft Matter 7, 4610–4614. doi:10.1039/C1SM05239J
Altamura, E., Albanese, P., Marotta, R., Milano, F., Fiore, M., Trotta, M., et al. (2021). Chromatophores efficiently promote light-driven ATP synthesis and DNA transcription inside hybrid multicompartment artificial cells. Proc. Natl. Acad. Sci. U.S.A. 118, e2012170118. doi:10.1073/pnas.2012170118
Altamura, E., Milano, F., Tangorra, R. R., Trotta, M., Omar, O. H., Stano, P., et al. (2017). Highly oriented photosynthetic reaction centers generate a proton gradient in synthetic protocells. Proc. Natl. Acad. Sci. U.S.A. 114, 3837–3842. doi:10.1073/pnas.1617593114
Andersen, H. D., Wang, C., Arleth, L., Peters, G. H., and Westh, P. (2011). Reconciliation of opposing views on membrane-sugar interactions. Proc. Natl. Acad. Sci. U.S.A. 108, 1874–1878. doi:10.1073/pnas.1012516108
Berhanu, S., Ueda, T., and Kuruma, Y. (2019). Artificial photosynthetic cell producing energy for protein synthesis. Nat. Commun. 10, 1325. doi:10.1038/s41467-019-09147-4
Campillo, C., Sens, P., Köster, D., Pontani, L.-L., Lévy, D., Bassereau, P., et al. (2013). Unexpected membrane dynamics unveiled by membrane nanotube extrusion. Biophysical J. 104, 1248–1256. doi:10.1016/j.bpj.2013.01.051
Chiba, M., Miyazaki, M., and Ishiwata, S. (2014). Quantitative analysis of the lamellarity of giant liposomes prepared by the inverted emulsion method. Biophysical J. 107, 346–354. doi:10.1016/j.bpj.2014.05.039
Dimova, R., Stano, P., Marques, C. M., and Walde, P. (2020). “Preparation methods for giant unilamellar vesicles,” in The giant vesicle Book. Editors R. Dimova, and C. M. Marques (Boca Raton, FL: Taylor & Francis Group), 3–20.
Faizi, H. A., Tsui, A., Dimova, R., and Vlahovska, P. M. (2022). Bending rigidity, capacitance, and shear viscosity of giant vesicle membranes prepared by spontaneous swelling, electroformation, gel-assisted, and phase transfer methods: A comparative study. Langmuir 38, 10548–10557. doi:10.1021/acs.langmuir.2c01402
Fujii, S., Matsuura, T., Sunami, T., Nishikawa, T., Kazuta, Y., Yomo, T., et al. (2014). Liposome display for in vitro selection and evolution of membrane proteins. Nat. Protoc. 9, 1578–1591. doi:10.1038/nprot.2014.107
Hadorn, M., and Eggenberger Hotz, P. (2009). “Multivesicular assemblies as real-world testbeds for embryogenic evolutionary systems,” in Artificial life: Borrowing from Biology. Lecture notes in computer science. Editors K. Korb, M. Randall, and T. Hendtlass (Berlin, Heidelberg: Springer), 169–178. doi:10.1007/978-3-642-10427-5_17
Hamada, T., Miura, Y., Komatsu, Y., Kishimoto, Y., Vestergaard, M., Takagi, M., et al. (2008). Construction of asymmetric cell-sized lipid vesicles from lipid-coated water-in-oil microdroplets. J. Phys. Chem. B 112, 14678–14681. doi:10.1021/jp807784j
Ito, H., Yamanaka, T., Kato, S., Hamada, T., Takagi, M., Ichikawa, M., et al. (2013). Dynamical formation of lipid bilayer vesicles from lipid-coated droplets across a planar monolayer at an oil/water interface. Soft Matter 9, 9539–9547. doi:10.1039/c3sm51766g
Kamiya, K., Kawano, R., Osaki, T., Akiyoshi, K., and Takeuchi, S. (2016). Cell-sized asymmetric lipid vesicles facilitate the investigation of asymmetric membranes. Nat. Chem. 8, 881–889. doi:10.1038/nchem.2537
Matosevic, S., and Paegel, B. M. (2011). Stepwise synthesis of giant unilamellar vesicles on a microfluidic assembly line. J. Am. Chem. Soc. 133, 2798–2800. doi:10.1021/ja109137s
McIntosh, T. J., Simon, S. A., and MacDonald, R. C. (1980). The organization of n-alkanes in lipid bilayers. Biochimica Biophysica Acta (BBA) - Biomembr. 597, 445–463. doi:10.1016/0005-2736(80)90219-9
Moga, A., Yandrapalli, N., Dimova, R., and Robinson, T. (2019). Optimization of the inverted emulsion method for high‐yield production of biomimetic giant unilamellar vesicles. ChemBioChem 20, 2674–2682. doi:10.1002/cbic.201900529
Nishimura, K., Hosoi, T., Sunami, T., Toyota, T., Fujinami, M., Oguma, K., et al. (2009). Population analysis of structural properties of giant liposomes by flow cytometry. Langmuir 25, 10439–10443. doi:10.1021/la902237y
Noireaux, V., and Libchaber, A. (2004). A vesicle bioreactor as a step toward an artificial cell assembly. Proc. Natl. Acad. Sci. U.S.A. 101, 17669–17674. doi:10.1073/pnas.0408236101
Pautot, S., Frisken, B. J., and Weitz, D. A. (2003a). Engineering asymmetric vesicles. Proc. Natl. Acad. Sci. U.S.A. 100, 10718–10721. doi:10.1073/pnas.1931005100
Pautot, S., Frisken, B. J., and Weitz, D. A. (2003b). Production of unilamellar vesicles using an inverted emulsion. Langmuir 19, 2870–2879. doi:10.1021/la026100v
Rampioni, G., D’Angelo, F., Messina, M., Zennaro, A., Kuruma, Y., Tofani, D., et al. (2018). Synthetic cells produce a quorum sensing chemical signal perceived byPseudomonas aeruginosa. Chem. Commun. 54, 2090–2093. doi:10.1039/C7CC09678J
Richens, J. L., Lane, J. S., Mather, M. L., and O’Shea, P. (2015). The interactions of squalene, alkanes and other mineral oils with model membranes; effects on membrane heterogeneity and function. J. Colloid Interface Sci. 457, 225–231. doi:10.1016/j.jcis.2015.06.052
Shimizu, Y., Inoue, A., Tomari, Y., Suzuki, T., Yokogawa, T., Nishikawa, K., et al. (2001). Cell-free translation reconstituted with purified components. Nat. Biotechnol. 19, 751–755. doi:10.1038/90802
Shimizu, Y., Kanamori, T., and Ueda, T. (2005). Protein synthesis by pure translation systems. Methods 36, 299–304. doi:10.1016/j.ymeth.2005.04.006
Shimane, Y., and Kuruma, Y. (2022). Rapid and Facile Preparation of Giant Vesicles by the Droplet Transfer Method for Artificial Cell Construction. Front. in Bioeng. Biotechnol. 10, 873854
Stano, P. (2019). Gene expression inside liposomes: From early studies to current protocols. Chem. Eur. J. 25, 7798–7814. doi:10.1002/chem.201806445
Szoka, F., and Papahadjopoulos, D. (1980). Comparative properties and methods of preparation of lipid vesicles (liposomes). Annu. Rev. Biophys. Bioeng. 9, 467–508. doi:10.1146/annurev.bb.09.060180.002343
Träuble, H., and Grell, E. (1971). Carriers and specificity in membranes. IV. Model vesicles and membranes. The formation of asymmetrical spherical lecithin vesicles. Neurosci. Res. Program Bull. 9, 373–380.
Uyeda, A., Reyes, S. G., Kanamori, T., and Matsuura, T. (2022). Identification of conditions for efficient cell-sized liposome preparation using commercially available reconstituted in vitro transcription-translation system. J. Biosci. Bioeng. 133, 181–186. doi:10.1016/j.jbiosc.2021.10.008
Walde, P., Cosentino, K., Engel, H., and Stano, P. (2010). Giant vesicles: Preparations and applications. Chem. Eur. J. Chem. Bio. 11, 848–865. doi:10.1002/cbic.201000010
Xiao, Z., Huang, N., Xu, M., Lu, Z., and Wei, Y. (1998a). Novel preparation of asymmetric liposomes with inner and outer layer of different materials. Chem. Lett. 27, 225–226. doi:10.1246/cl.1998.225
Xiao, Z., Xu, M., Li, M., Lu, Z., and Wei, Y. (1998b). Preparation of asymmetric bilayer-vesicles with inner and outer monolayers composed of different amphiphilic molecules. Supramol. Sci. 5, 619–622. doi:10.1016/S0968-5677(98)00088-1
Yanagisawa, M., Iwamoto, M., Kato, A., Yoshikawa, K., and Oiki, S. (2011). Oriented reconstitution of a membrane protein in a giant unilamellar vesicle: Experimental verification with the potassium channel KcsA. J. Am. Chem. Soc. 133, 11774–11779. doi:10.1021/ja2040859
Keywords: synthetic cells, artificial cells, protocells, lipid vesicles, liposomes, liposome technology, droplet transfer method, inverted emulsion method
Citation: Stano P (2022) Commentary: Rapid and facile preparation of giant vesicles by the droplet transfer method for artificial cell construction. Front. Bioeng. Biotechnol. 10:1037809. doi: 10.3389/fbioe.2022.1037809
Received: 06 September 2022; Accepted: 26 September 2022;
Published: 14 October 2022.
Edited by:
Simon J. Moore, University of Kent, United KingdomReviewed by:
Aleksander Czogalla, University of Wrocław, PolandCopyright © 2022 Stano. This is an open-access article distributed under the terms of the Creative Commons Attribution License (CC BY). The use, distribution or reproduction in other forums is permitted, provided the original author(s) and the copyright owner(s) are credited and that the original publication in this journal is cited, in accordance with accepted academic practice. No use, distribution or reproduction is permitted which does not comply with these terms.
*Correspondence: Pasquale Stano, cGFzcXVhbGUuc3Rhbm9AdW5pc2FsZW50by5pdA==
Disclaimer: All claims expressed in this article are solely those of the authors and do not necessarily represent those of their affiliated organizations, or those of the publisher, the editors and the reviewers. Any product that may be evaluated in this article or claim that may be made by its manufacturer is not guaranteed or endorsed by the publisher.
Research integrity at Frontiers
Learn more about the work of our research integrity team to safeguard the quality of each article we publish.