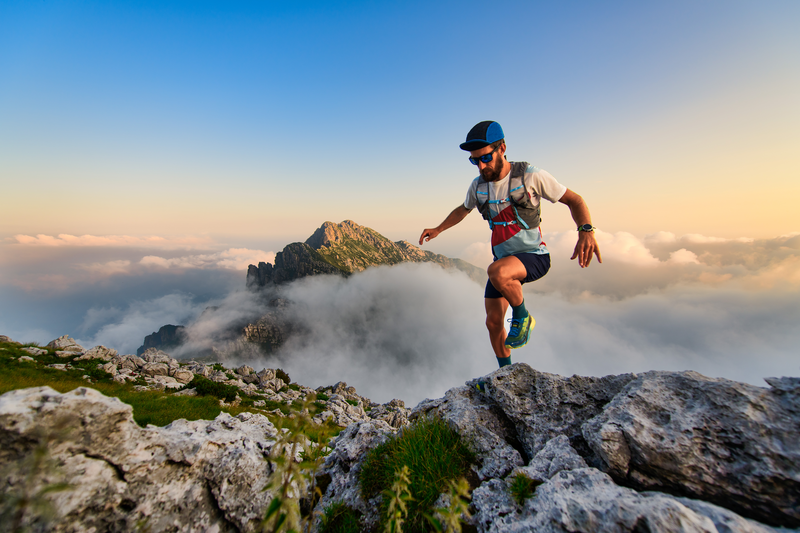
94% of researchers rate our articles as excellent or good
Learn more about the work of our research integrity team to safeguard the quality of each article we publish.
Find out more
REVIEW article
Front. Bioeng. Biotechnol. , 13 October 2022
Sec. Biomaterials
Volume 10 - 2022 | https://doi.org/10.3389/fbioe.2022.1017613
This article is part of the Research Topic Clinic-oriented Multifunctional Biomaterials: From Rational Design to Applications View all 12 articles
The destruction of periodontal tissue is a crucial problem faced by oral diseases, such as periodontitis and tooth avulsion. However, regenerating periodontal tissue is a huge clinical challenge because of the structural complexity and the poor self-healing capability of periodontal tissue. Tissue engineering has led to advances in periodontal regeneration, however, the source of exogenous seed cells is still a major obstacle. With the improvement of in situ tissue engineering and the exploration of stem cell niches, the homing of endogenous stem cells may bring promising treatment strategies in the future. In recent years, the applications of endogenous cell homing have been widely reported in clinical tissue repair, periodontal regeneration, and cell therapy prospects. Stimulating strategies have also been widely studied, such as the combination of cytokines and chemokines, and the implantation of tissue-engineered scaffolds. In the future, more research needs to be done to improve the efficiency of endogenous cell homing and expand the range of clinical applications.
Periodontal tissue is a kind of complex tissue composed of both soft tissue like periodontal ligament (PDL) and gingiva, as well as hard tissue such as alveolar bone and cementum. It plays a crucial role in supporting teeth, bearing occlusal forces, and maintaining oral mucosa integrity. When faced with the destruction of periodontal tissue, negative effects such as tooth loss, and physical and mental health damage could happen (Darveau, 2010; Kinane et al., 2017). The existing clinical treatment methods, such as guided tissue/bone regeneration (GTR/GBR) can improve the clinical efficacy of various tissue defects (Bottino et al., 2012; Xu et al., 2019). However, the effect on periodontal regeneration is not as satisfying, especially in the recovery of physiological structure and function. The ideal result of periodontal therapy is to regenerate the cementum-periodontium-bone system while achieving this goal remains a huge challenge. However, the insufficiency of stem/progenitor cells seems to be the primary limitation for periodontal membrane and alveolar bone reconstruction.
With the development and improvement of cell therapy, researchers have found various stem cells for periodontal regeneration. Mesenchymal stem cells (MSCs) and periodontal ligament stem cells (PDLSCs) are most studied, together with other stem cells such as dental pulp stem cells (DPSCs) and induced pluripotent stem cells (iPSC) (Huang et al., 2009b; Kwon et al., 2018). Those stem cells are routinely isolated, cultured, and amplified in vitro and relatedly applied. However, exogenous stem cell transplantation still has various disadvantages, for example, the complicated technology of in vitro operation, high-cost ex vivo cell culture, and even the potential ethical and safety risks (Lee et al., 2016; Pacelli et al., 2017; Safina and Embree, 2022).
Stem cell homing is a physiologic process which been studied since the 1970s. The fact that intravenously injected HSPCs can find their way home to the marrow was first found in clinical transplant settings. To address the shortcomings of cell transplantation, researchers turned to the homing of endogenous stem cells, attempting to heal wounds by activating the self-repairing capacity by recruiting endogenous stem cells to the defect area (Chen et al., 2010; Ahmed et al., 2021). Stem-cell-homing involves a series of physiological processes including cell recognition, migration, proliferation, and differentiation, and ultimately achieves tissue regeneration, which plays a huge role under certain conditions and has achieved remarkable therapeutic effects (Liesveld et al., 2020). In this method, biomaterials were utilized for bioactive factors delivery as well as the host’s inherent regenerative potential activating (Safina and Embree, 2022; Xin et al., 2022; Yao and Lv, 2022; Zhao et al., 2022). By mobilizing appropriate stem/progenitor cells to specific spaces for tissue repair through cell-material interactions at the defect site, the endogenous regeneration process can be mimicked (Andreas et al., 2014; Mao et al., 2022). The possible advantages of this strategy in promoting periodontal regeneration are as follows: firstly, it provides a solution to some of the limitations of stem cell transplantation, and transforms periodontal regeneration treatment methods into a clinically valid way; secondly, it gives full play to the potential of host self-repair and regeneration, making periodontal tissue regeneration safer; moreover, compared with the introduction of exogenous stem cells, it is simpler and less expensive to treat periodontal diseases and other diseases (Abdulghani and Mitchell, 2019; Safina and Embree, 2022). To make better use of the stem cell homing technique to restore the periodontal defect, this paper reviews the research status of the stem cell homing technique.
Stem cell homing was originally defined as the process of endothelialization through blood vessels and migration of hematopoietic stem cells (HSCs) after transplantation, and finally, HSCs colonize in the bone marrow and restart hematopoiesis, in which many cytokines and chemokines are involved (Ji et al., 2015; Zhu et al., 2015). Blood lineages are considered to be risen from hematopoietic stem and progenitor cells (HSPCs), which then migrate to the bone marrow niche through homing for further proliferation and differentiation (Li et al., 2018a; Blokzijl-Franke et al., 2021; Ranzoni et al., 2021). The homing mechanism of HSPCs is relevant to stem cell transplantation therapy and has been investigated by many researchers (Morrison and Scadden, 2014; Li et al., 2018a; Liesveld et al., 2020), though the specific mechanism of HSPCs homing was not elucidated. While researchers considered that specific cells or cytokines may mediate this process, thus enabling the homing of HSCs (Li et al., 2018a).
Recently, the tissue engineering technique has expanded the meaning of stem cell homing as the process in which endogenous stem cells mainly migrate directionally and across the vascular endothelium to target tissues, and then colonize and survive (Ji et al., 2015; Zhu et al., 2015). In vivo, stem cells are located in various stem cell niches and are exposed to a large number of complex and manageable biomaterials, including chemokines, cytokines, growth factors, the extracellular matrix (ECM), and so on (Chen et al., 2011; Yang et al., 2020) (Figure 1).
FIGURE 1. In situ tissue engineering uses biological molecular and scaffolds to recruit the stem cells from the niches while promoting proliferation and differentiation and achieving tissue regeneration in the end.
Tissue regeneration, also known as in situ tissue engineering, has emerged over the past 20 years because of its remarkable advantages. This technique mimics the human wound healing process and aims to repair or regenerate tissue by recruiting endogenous stem cells to the defections via targeted specific bionic scaffolds and/or bioactive cues that stimulate the host’s biological substance and repairing capacity (Ji et al., 2015; Safina and Embree, 2022). To make the endogenous stem cells migrate toward a specific tissue, these endogenous stem cells must recognize the biomolecules that mediate homing first and manipulate their activity to catalyze the homing process (Chen et al., 2011). The possible mechanism might rely on the development of the scaffold system which is implanted into the damaged area (Lee et al., 2016; Abdulghani and Mitchell, 2019). The proper microenvironment created by the implanted scaffold will then promote the host stem cells to recruit and move through the vascular network or tissue interstitial space to the damaged tissue or organ, where the stem cells then proliferate, differentiate, and form tissue within the scaffold which eventually degrades, leaving only regenerated tissue (Zheng et al., 2019; Chu et al., 2022).
In regenerative medicine, there is growing evidence proving that cell recruitment could stimulate self-repair ability in hosts and harness the born regenerative capacity of tissues. It was regarded as a promising cell-based therapy and has been used to regenerate heart tissue, cartilage tissue, and bone tissue in situ already (Chen et al., 2011; Cai et al., 2018). In terms of articular cartilage repair, it has been demonstrated that intra-articular and peri-articular MSCs are involved in the cartilage repair process, but due to their limited number, they are unable to achieve the desired repair effect and require stem cells from other sites to recruit to the injured site for repair and to facilitate this process, chemotactic agents are required (Yang et al., 2020; Zhang et al., 2020). Lee et al. (2010)utilized poly-ε-caprolactone and hydroxyapatite to create an anatomically correct TGF-β3-containing biological scaffold. The results suggest that for such complex tissues, the regeneration may happen via endogenous cell homing instead of cell transplantation. In addition, endogenous stem cell homing technology has shown great potential in the cardiovascular field, aiming at repairing heart-damaged tissues in myocardial infarction (MI) and ischemic heart disease, and is considered one of the most promising therapeutic strategies (Pacelli et al., 2017; Li et al., 2018b). Shafiq et al. (2018) developed specific cardiac patches, which can promote the mobilization and recruitment of endogenous MSCs to the defected area in acute MI models. The experimental results showed that this new cardiac patch together with suitable scaffold materials is a good choice for promoting in situ vascular regeneration and deserves to be promoted.
For periodontal regeneration, though the injured periodontium possesses a weak ability for self-healing, it can be significantly promoted when proper treatment and guidance are added (Xu et al., 2019). Increasing evidence demonstrates that directing endogenous stem cells to defected areas contributes to the regenerative and immunomodulating function since the resident MSCs play a key role in periodontal regeneration. Except for MSCs, PDL also contains PDLSCs and osteogenic progenitor cells which can regenerate cementum, bone, and the PDL tissue itself together with MSCs (Cai et al., 2018; Yamamoto et al., 2018; Xu et al., 2019). Additionally, a variety of factor-loaded scaffolds for periodontal regeneration have been fabricated. Yu et al. (2022) constructed a biphasic scaffold combining intrafibrillarly mineralized collagen (IMC) and concentrated growth factor (CGF) to synergist regeneration of periodontal tissues. In animal experiments, PDLSCs, BMSCs, and induced pluripotent stem cells showed the potential to stimulate the formation of new periodontal tissues (Ji et al., 2015). Consequently, the application of cell homing could eliminate clinical constraints associated with periodontal wounds (Cai et al., 2018). Wang et al. (2016) constructed a cell-free stromal cell-derived factor-1α (SDF-1α)-scaffold-parathyroid hormone system which can stimulate the proliferation of CD90+CD34−stromal cells and promote the regeneration of damaged tissues in a rat periodontal defect model. Liang et al. (2021) also used rats to apply SDF-1 topically and exendin-4 (EX-4) systemically. The experimental results showed that combined SDF-1/EX-4 treatment could promote the recruitment of MSCs in vivo, induce early osteoclastogenesis, and promote the expression of osteogenic proteins, which results in both the quantity and quality improvement of regenerated bone. Although the cell homing for periodontal regeneration is still at the experimental stage, it has great potential to facilitate bone-ligament-cementum regeneration in the treatment of periodontal diseases, such as periodontitis, providing a safe, effective and cost-effective alternative therapy (Liu et al., 2019a; Xu et al., 2019).
In periodontal tissue regeneration and repair, endogenous stem cells mainly used include BMSCs and PDLSCs, which can migrate to the defect site and stimulate their proliferation and differentiation by adopting appropriate strategies, such as chemokines, and using tissue-engineered scaffolds (Figure 2; Table 1).
FIGURE 2. Stimulation strategies, such as chemokines, cytokines, scaffolds, and immune cells, for stem cell homing and periodontal tissue regeneration.
To repair periodontal tissues, reparative cells can be acquired from stem niches (Kimura et al., 2014; Liu et al., 2015; Yin et al., 2017). Mainly BMSCs and PDLSCs were studied. PDLSCs are the first choice for studying cell delivery for periodontal regenerative purposes, while bone marrow-derived cells also contribute to it because of the insufficiency of the stem cells in PDL tissue (Yin et al., 2017).
Multipotent MSCs can be proliferated both in vitro and in vivo, which then differentiate into mesodermal tissues of different functions, including bone, cartilage, tendons, fat, and nerves (Pittenger et al., 1999). As a consequence, MSCs have been proposed as potentially useful tissue-engineering seeding cells. Several studies have found that BMSCs can be the origin of cementoblasts, osteoblasts, and periodontal fibroblasts, in addition to secreting extracellular matrix from PDLs, cementum, and alveolar bone (Kawaguchi et al., 2004; Hasegawa et al., 2006).
Immunohistochemistry was used to quantify the engraftment of MSCs in the defect by Liu et al. (2015). A sequential section approach was used to identify MSCs (CD29+/CD45−), which showed that the number of MSC transplanted with SDF-1 significantly increased.
He et al. (2019) selected rat BMSCs to investigate Mφ polarization and endogenous stem cell recruitment which enhance periodontal regeneration. It has been shown that the homing of BMSCs was significantly improved both in vitro and in vivo.
Recently, subsequent attempts were made to harvest MSCs from odontogenic tissues, such as periodontal ligaments (Seo et al., 2004), gingiva (Zhang et al., 2009), the dental follicle (Morsczeck et al., 2005), the dental pulp (Gronthos et al., 2000), apical papilla (Sonoyama et al., 2008), and human exfoliated tissue deciduous teeth (Miura et al., 2003; Hynes et al., 2012). From human-impacted third molars, Seo et al. (2004) successfully isolated PDLSC, which can differentiate into multiple periodontal tissues. (Liu et al., 2008; Huang et al., 2009a; Park et al., 2011).
PDLSCs, located around the blood vessels of periodontal tissues and having the characteristics of mesenchymal stem cells, are one of the most practically applied multipotency stem cells in the field of periodontal tissue repair and regeneration. Liang et al. (2021) tested PDLSCs and confirmed that the proliferation and migration of PDLSCs. In other research, the effects of growth factors on controlling the fate of PDLSCs are studied (Ding et al., 2020).
CD90+CD34− stromal cells have also been studied for recruitment. CD90, also referred to as Thy-1, represents stem and progenitor cells on the cell surface, which makes CD90+CD34− stromal cells to be considered non-hematopoietic stromal cells. A previous study also showed that MSCs, including PDLSCs, could express CD73, CD90, and CD105 while not CD14, CD34, and CD45 (Dominici et al., 2006). Therefore, according to previous studies, CD90+CD34−stromal cells were considered as MSCs (Wang et al., 2016).
Several studies have shown that adult stem cells with CD90 expression have high osteogenic differentiation potential (Chung et al., 2013). In the study by Wang et al. (2016), stromal cells with CD90+CD34− staining were analyzed quantitatively using immunofluorescence double staining. The results showed that host-derived CD90+CD34−stromal cells were recruited and transplanted into the defect. Ding et al. (2020) also used the framework to recruit CD90+CD34− stromal cells. The in vivo research results showed significant promotion of the recruitment of host-derived CD90+CD34− stromal cells at the early stage of wound healing, favoring tissue repair and regeneration.
Some researchers studied the recruiting and regulating fate of PDLSCs and BMSCs together (Ji et al., 2015; Yu et al., 2022). According to Ji et al. (2015), PDLSCs and BMSCs were tested in vitro with canine platelet-rich fibrin (PRF) and treated dentin matrix (TDM). An orthotopic transplantation model using Beagle dogs was developed to regenerate roots with a tooth-PDL-alveolar interface by cell homing in a canine orthotopic model, combined with the use of PRF membranes and TDM. In vitro, PRF significantly stimulated the recruitment and proliferation of PDLSCs and BMSCs. The in vivo results similarly illustrated that roots connected to the alveolar bone by cementum-PDL complexes can be regenerated by implanting PRF and TDM in the alveolar microenvironment, possibly through the homing of BMSCs and PDLSCs.
Currently, the main chemotactic strategies used include: 1) the use of chemotactic agents, such as sdf-1, and the combination of the two; 2) the use of scaffold materials loaded with biological factors; 3) regulatory assistance through macrophages. They are described as follows:
SDF-1, also known as C–X–C motif ligand 12 (CXCL12), is a promising candidate for in situ tissue engineering among various cytokines and chemokines. Previous studies have shown that during the healing process of impaired tissues [brain (Ardelt et al., 2013), heart (Wang et al., 2012; Hajjar and Hulot, 2013; Cavalera and Frangogiannis, 2014), muscle (Brzoska et al., 2012), skin (Yang et al., 2013), and bone (Ji et al., 2013)], elevated levels of SDF-1 at the site of injury can recruit stem/precursor cells from the cardiovascular system and local tissues, and promote their proliferation and differentiation at the site of damage, leading to organ repair and regeneration (Brzoska et al., 2012; Wang et al., 2012; Hajjar and Hulot, 2013; Yang et al., 2013).
In the study of Liu et al. (2015), it has been verified that SDF-1 promoted the proliferation, migration, and differentiation of PDLSCs in vitro. After that, they further confirmed the effect of local application of SDF-1 on stem/progenitor cell homing and periodontal bone regeneration in vivo through a rat mandibular defect model. Host-derived CD29+/CD45− MSCs were confirmed to be triggered to home and graft into periodontal bone defects by loading SDF-1 into collagen membrane scaffolds. As a result of SDF-1 treatment, MSCs were significantly increased and CD45 + HSCs were engrafted.
The same results were obtained by Adelina S. (Cai et al., 2018) using an SDF-1α loaded gelatin sponge (Spongostan®) for rats with mandibular bone defects. At the same time, a possible explanation has been proposed regarding the enhanced periodontal regeneration caused by SDF-1α stimulation: first, bone marrow-derived osteogenic progenitor cells present in the flowing blood may be recruited to the defected area by the SDF-1α/CXCR4 axis in response to the local release of SDF-1α (Otsuru et al., 2008; Ji et al., 2013). The recruited cells play an important role in periodontal regeneration by expressing their multilineage differentiation capacity, as well as secreting promoting cytokines and growth factors (Bryan et al., 2005). Furthermore, SDF-1α may also induce other progenitor cells in the bone marrow such as HSCs and endothelial progenitor cells to migrate to the defect sites via the SDF-1α/CXCR4 axis, creating a proangiogenic environment (Hattori et al., 2003; Petit et al., 2007).
The main effect of SDF-1 is to actively direct endogenous cell homing. However, its promoting effect on the proliferation and differentiation of stem cells is limited. Therefore, combining other active molecules, such as bone morphogenetic protein 7 (BMP-7), parathyroid hormone (PTH), and EX-4, to improve the efficiency of tissue regeneration has been studied.
BMP-7 could modulate osteogenesis and bone cell differentiation potently. A preclinical study assessed its effects on the regeneration of periodontal bone defects and improvement of cementum regeneration (Cook et al., 1995; Giannobile et al., 1998; Ripamonti et al., 2001). Groundbreaking research into the regeneration of PDL tissue via cell homing with SDF1 and BMP-7 was carried out by Kim et al. (2013) in 2010. BMP-7 may be responsible for orthotopic mineralization and formation of the newly formed alveolar bone in rat extraction sockets. The observed putative periodontal ligament also suggests that SDF1 and/or BMP-7 can recruit multiple cell lineages. On this basis, Zhu et al. (2015) further studied the application of SDF1 and BMP-7 in the tooth trauma model frequently. Zhu et al. (2015) hypothesized that SDF1 and BMP-7 may recruit endogenous cells to the root surface to replace the unavailable original PDL cells after avulsed teeth were delayed. During the experiment, avulsed roots coated with SDF1 and BMP-7 were put into the prepared alveolar bone socket. By directing stem cells to the space between replanted roots and the adjacent alveolar bone, SDF1 and BMP-7 were proven to establish the integrated PDL-like structure.
PTH has been used as an optional treatment for bone defects. In periodontal tissue repair, when a large number of endogenous progenitor cells are mobilized directly to the peripheral blood, they will return to the defect site and participate in tissue regeneration, along which PTH is considered a promising periodontal tissue repair agent (Barros et al., 2003; Bashutski et al., 2010; Tokunaga et al., 2011). As a crucial factor for stem cell homing, the chemotactic properties of SDF-1α may be limited by the N-terminal cleavage of the cell surface protein CD26/dipeptidyl peptidase-IV (DPP-IV) at position 2 proline (Christopherson et al., 2002; Christopherson et al., 2004). PTH, known as a DPP-IV inhibitor, has been confirmed recently to enhance the SDF-1α-driven homing of CXCR4 + stem cells in the ischemic heart recently (Huber et al., 2011). In a rat periodontal defect model, Wang et al. (2016) confirmed that PTH/SDF-1α cotherapy was able to induce CD90 + CD34−stromal cell migration and enhance the chemotactic ability of SDF-1α, and accelerate periodontal tissue regeneration.
EX-4, as a full agonist of the glucagon-like peptide-1 receptor (GLP-1R), could promote both migration and proliferation of MSCs (Zhou et al., 2015; Zhang et al., 2016; Sharma et al., 2018; Yap and Misuan, 2019). Recently, studies on EX-4 have proved its capability to inhibit adipogenic stem/precursor cell differentiation while promoting osteogenic differentiation and bone formation. (Feng et al., 2016; Meng et al., 2016; Luciani et al., 2018). Moreover, the quantity of CXCR4+ MSCs was increased by EX-4 via PI3K/AKT-SDF-1/CXCR4 signaling pathway (Zhou et al., 2015). Liang et al. (2021) demonstrated that the combination of SDF-1/EX-4 enhanced the proliferation and migration of PDLSCs in vitro, together with the recruitment of MSCs, inducement of early osteoclastogenesis, expression of osteoblast protein in new bone formation, as long as the formation of new bone in vivo. Therefore, the combination of SDF-1/EX-4 could provide a new strategic option for periodontal bone regeneration in situ.
Scaffold biomaterials should be designed to mimic the natural extracellular environment as much as possible to affect cell behavior and control cell fate in vivo. In the present study, many scaffold materials can not only act as carriers but also play a role in recruiting stem cells and promoting their proliferation and differentiation.
Choukroun et al. (2001) reported in 2001 that PRF could promote the recruitment, proliferation, and differentiation of stem cells by releasing several growth factors, such as transforming growth factor-β(TGF-β), platelet-derived growth factor (PDGF), epidermal growth factor (EGF). TDM, a natural acellular matrix scaffold, could retain essential non-collagenous proteins and growth factors for the regeneration of apical periodontal tissue (Li et al., 2008; Smith et al., 2012). Both PRF and processed TDM are depots of various growth factors that can promote cell homing. Ji et al. (2015) confirmed that PRF can recruit BMSCs and PDLSCs. In addition, the proliferation of PDLSCs and BMSCs was also stimulated in vitro, which is consistent with other studies (Ehrenfest et al., 2010; Chang and Zhao, 2011). The results also demonstrated that TDM was able to direct the differentiation of seed cells (Ma et al., 2008; Wu et al., 2008; Li et al., 2011; Guo et al., 2012; Yang et al., 2012). Treated as one unit, PRF/TDM’s potential to stimulate the differentiation of PDLSCs and BMSCs was confirmed in vitro. In vivo, the experimental results showed that it is essential for endogenous tooth root regeneration by using PRF as bioactive cues, and TDM as an inductive scaffold, together with tooth socket microenvironments.
In addition to scaffold materials of biological origin, synthetic scaffold materials also have similar effects. Ding et al. (2020) developed a super assembly framework (SAF) in which bFGF and BMP-2 were designed to facilitate regeneration of the local cementum-ligament-bone complex in a sequential manner. The in situ tissue engineering framework (iTE-framework) not only showed improved physicochemical properties, but also was shown to promote the proliferation, migration, and osteogenic differentiation of PDLSCs in vitro. A rat periodontal defect model is created for in vivo experiment. The results showed that both the formation of new bone and the regeneration of PDL and cementum tissue could be promoted by the iTE framework significantly.
Microenvironments can influence cell homing by influencing the properties of stem cells. Since there are already many well-established drugs targeting the immune microenvironment in treating periodontitis, it is a promising strategy to combine these existing therapeutic agents and cytokines to enhance the immune microenvironment and promote cell homing and tissue formation, thus achieving higher levels of immune regulation and tissue repair (Yang et al., 2021).
In correlative studies (Abbott et al., 2004), exogenous SDF-1 was found to be insufficient to stimulate stem cell recruitment without injury for periodontal regeneration. A combination of inflammation and SDF-1 may increase stem cell recruitment in the healing process (Chen et al., 2013). In further studies conducted by Li et al. (2018a), it was confirmed that VCAM-1 + macrophage-like cells are important for both homing and retention of HSPCs. In the field of periodontal defect regeneration, He et al. (2019) studied the effect of “macrophage regulation” in periodontal tissue regeneration. It was hypothesized that high-stiffness TG gels modulate Mφ polarization and promote endogenous stem cell recruitment by modulating IL-4 and SDF-1α production. Immunofluorescence staining and histological examination showed that IL-4 could promote the polarization of Mφs into the M2 phenotype, and further promote the osteogenic differentiation of successfully homed BMSCs.
In the future, further studies on the role of immunomodulation in stem cell homing are needed to address these uncertain issues and reach scientific conclusions.
Natural scaffold materials have good biocompatibility and bioactivity, and can also be loaded with a variety of biological factors, effectively promoting the reconstruction of periodontal tissues.
Collagen, as a natural protein, is obtained from the skin, bones, and ligaments of animals and is widely used in tissue engineering scaffolds due to its superior biocompatibility, biodegradability, and weak antigenicity (Reyes and García, 2004; Xiong et al., 2009). In the study of recruiting endogenous stem cells for periodontal tissue regeneration, collagen membrane or collagen solution is often used as a tissue engineering scaffold and plays a role in transporting chemokines and recruiting endogenous stem cells (Liu et al., 2015; Zhu et al., 2015; Wang et al., 2016; Liang et al., 2021). A commercial product, gelatin sponge (Spongostan ®) (Cai et al., 2018), was also used as a carrier for the delivery of SDF-1α.
TDM is a kind of natural matrix scaffold that is decellularized and retains multiple bioactive molecules, including non-collagenous proteins and growth factors (Smith et al., 2012) that are crucial for the formation of periodontal tissue around tooth roots (Li et al., 2008). Several studies have shown that PDLSCs treated with dentin non-collagenous proteins exhibit several signs of differentiation into cementoblasts. In their previous studies, TDM demonstrated a role as an inductive microenvironment and scaffold for tooth root regeneration (Guo et al., 2012; Yang et al., 2012). TDM, compared with nature dentin, has similarities in structure and mechanical characteristics but is more cost-effective. By regulating molecules in a mechanically-suitable environment, it is expected to trigger regenerative processes not only structurally but also physiologically. There was evidence that TDM can direct the differentiation of seeded cells (Ma et al., 2008; Wu et al., 2008; Li et al., 2011; Guo et al., 2012; Yang et al., 2012). The study by Ji et al. (2015) mentioned above demonstrated the effect of TDM in periodontal tissue regeneration.
Another scaffold material of natural origin was prepared by Yu et al. (2022). With biomimetic self-assembly and microstamping techniques, they constructed a parallel hierarchy of mineralized IMC layers and unmineralized collagenized-CGF layers. With special micro-structure, mechanical characteristics, and growth-factor-rich microenvironment, the differential biphasic scaffold simulates the periodontal hard/soft tissue interface perfectly. This solves the obvious disadvantage that monophasic scaffolds cannot be used effectively for regenerating complex multiphasic tissues, such as periodontal tissues (Dan et al., 2014; Cho et al., 2016). This biomimetic IMC scaffold modulates and determines the fate of PDLSC and BMSCs. Even though it cannot secrete growth factors, the osteoid microenvironment can serve as a biofactor-rich microenvironment that facilitates cell osteogenesis (Liu et al., 2019b). CGF is rich in endogenous growth factors, including TGF-β1, VEGF, PDGF-BB, IGF-1, and bFGF. The CGF substrate superstructure with parallel-aligned gaps may also be responsible for the oriented migration of stem cells (Liu et al., 2013). In vitro experiments demonstrated that PDLSCs were successfully differentiated into PDL/osteogenesis using the biphasic scaffold containing CGF/IMC. After implantation in rat periodontal defects, multilineage differentiation could be effectively achieved by recruiting host stem cells into soft and hard periodontal tissues.
Natural scaffold materials have good biocompatibility and bioactivity, but synthetic scaffold materials usually have more controllable degradation rates and physical and mechanical properties. To overcome the disadvantages of the above materials when used alone, as well as simulate complex periodontal tissues, a new direction in the development of scaffold materials in recent years is to combine two or more materials to obtain good physical and chemical properties while improving their biocompatibility and bioactivity.
Unlike previous approaches that relied primarily on hard materials like collagen, silk, or PLGA, the primary scaffold relies on soft materials (Young et al., 2002; Modino and Sharpe, 2005; Ikeda et al., 2009). The mechanical stiffness of polycaprolactone (PCL) and hydroxyapatite (HA) hybrid has good load-bearing properties (Woodfield et al., 2005). Rapid prototyping methods such as 3D bioprinting may offer additional advantages such as precise control, interconnectivity, as well as anatomical dimensions (Woodfield et al., 2005; Lee et al., 2009). Kim et al. (2010), anatomically shaped human layer scaffold and rat incisor scaffold from a hybrid of PCL and HA via 3D layer-by-bioprinting channels. The collagen solution loaded with SDF1 and BMP-7 was also injected into the scaffold microchannel by a micropipette. Next, the rat model was used for orthotopic/ectopic tooth regeneration without cell transplantation. Quantitatively, the combination of SDF1/BMP-7 significantly improved the cell’s chances of homing into the microchannels of human molar scaffolds compared to scaffolds without growth factors. Besides recruitment of cells into the microchannels, the regeneration of putative PDL and new alveolar bone also supports the claim that cell homing is effective.
Other composite scaffolds, such as a three-dimensional (3D) scaffold of high-stiffness Transglutaminase cross-linked gelatin (TG-gel) constructed by He et al. (2019) and framework (SAF) which can sequentially release bFGF and BMP-2 constructed by Ding et al. (2020), also showed great potential for in situ periodontal tissue regeneration.
Periodontal tissue is a complex tissue composed of PDL, alveolar bone, cementum, and gingiva, which together play an important role in supporting teeth, masticatory forces, and maintaining the mucosa of the oral masticatory. In addition to controlling the inflammation in the periodontal tissues caused by periodontitis (Pihlstrom et al., 2005; Darveau, 2010; Kinane et al., 2017), periodontal therapy aims to regenerate the cementum-ligament-bone complexes (Phillips et al., 2000). The traditional method is stem cell transplantation (Liu et al., 2008), which is unsatisfactory for a complete and stable restoration of periodontal tissue. The use of in situ tissue engineering for periodontal regeneration has recently gained popularity. Specifically, scaffolds are used to deliver chemotactic agents to maximize the host’s intrinsic potential, mobilize appropriate progenitor cells into an area designated for tissue repair, and mimic endogenous regenerative processes (Chen et al., 2015; Martino et al., 2015; Lee et al., 2017). Therefore, the method can regenerate and repair a variety of periodontal tissues, including periodontal ligaments, alveolar bone, cementum, and periodontal ligament fibers (Table 1).
In severe cases of periodontitis, the alveolar bone could be resorbed, resulting in tooth loss. During periodontal therapy, the main goal is to regenerate bone. Tissue engineering techniques have opened up new possibilities for regenerating periodontal bone. Liu et al. (2015) performed in vitro experiments using a Wistar rat model of mandibular buccal bone defects. The effects of SDF-1 on bone formation were assessed. At the early stage of degradation, old bone was resorbed through osteoclastogenesis facilitated by SDF-1, and collagen scaffold formation was accelerated by MMP-9, making space for new bone and other tissue. The anti-inflammatory properties of SDF-1 could reduce the inflammatory response, promote vascularization, recruit MSCs and HSCs to the wound for healing processes, and ultimately enhancing bone regeneration. Even though it is still unclear which mechanism governs bone regeneration, the study confirms that loading SDF-1 into collagen scaffolds shows great potential as in situ tissue engineering strategy in periodontal bone regeneration.
It is insufficient to apply SDF-1 alone for favorable bone regeneration (Cipitria et al., 2017). Hence, other growth factors should be combined to boost periodontal bone regeneration. An in vitro and in vivo study conducted by Liang et al. (2021) showed that SDF-1/EX-4 combination treatment enhanced PDLSC proliferation and migration, as well as in vitro mineral deposition production and early osteoclastogenesis. The osteogenic protein expression in a rat periodontal bone defect model has also been upregulated. This strategy therefore improves the quantity and quality of regenerated bone and provides a new tool for periodontal bone regeneration in situ.
In dental trauma, tooth avulsion is one of the most common but severe cases (Andreasen et al., 1994). After the tooth was avulsed, the fibers of its PDL were torn, which would render immediate but severe injury to the periodontal soft tissue. To survive after avulsed teeth are replanted, regeneration of PDL is essential.
By coating the root surface with SDF-1 and BMP-7, Wenting Zhu et al. (2015) succeeded in generating a PDL-like neo-tissue between the surrounding alveolar bone and the replanted root surface. Since the collagen fibers were inserted deep into the cementum and adjacent bone perpendicularly, neo-tissue displayed periodontium-like characteristics. In essence, it was possible to restore the integrity of the periodontal structure of the teeth. Using this method, it would be possible to determine whether avulsed teeth could be rescued after they had been given up clinically.
The disease of periodontitis involves both periodontal ligaments and alveolar bone, which causes inflammation of gingival tissues, the loss of periodontal attachment, and ultimately leads to tooth loss (Pihlstrom et al., 2005). To limit inflammation within the periodontal tissues and control the progression of periodontitis, the main goal of periodontal therapy is to restore these lost tissues to their original morphology, structure, and function. Due to the complex mineralized/non-mineralized layered structure of periodontal tissues (Woo et al., 2021), regenerative repair is a challenging task for dentists (Bartold et al., 2000) once the loss of hard tissue and soft tissue occurs. While stem cell-based cell delivery therapy shows great promise in periodontal wound healing, culture-expanded stem cells require complex procedures and are expensive to apply. (Chen et al., 2010; Chen et al., 2012b). Endogenous regeneration techniques can stimulate potential self-repair mechanisms in the host by promoting endogenous stem cell recruitment and adaptation to the lesion site (Chen et al., 2011). Compared to complex and expensive ex-vivo manipulation techniques, these options enhanced safety, affordability, and flexibility, making them increasingly popular in periodontal regenerative medicine (Chen et al., 2010).
Using the rat periodontal fenestration defects model, Wang et al. (2016) explored the effects of a cell-free system that combined PTH systemic application to SDF-1α-scaffold on periodontal tissue regeneration in vivo, while co-presentation of IL -4 and SDF-1α in high-stiffness TG-gels on periodontal regeneration was studied by He et al. (2019). Two experiments both led to satisfactory soft tissue and hybrid tissue regeneration.
Due to the difficulty of regenerating complex multiphase tissues by monophasic scaffolds (Dan et al., 2014; Cho et al., 2016), Yu et al. (2022) constructed a biomimetic tissue-specific functional structure to regenerate periodontal tissues (Figure 3). In the hierarchical biphasic architecture, IMC scaffolds could be used for osteogenic differentiation, while CGF fibers exhibited fibroblastic differentiation potential. After implanting the critical-sized intact defect in a rat model, the host stem cells could be recruited effectively, which then achieve multilineage differentiation into periodontal tissues. As the PDL fibers were inserted into the newly formed bone tissue, the CGF/IMC biphasic scaffold successfully reconstructed complete and functional periodontium after 8 weeks of implantation.
FIGURE 3. Potential mechanisms of periodontal hard/soft tissue regeneration by the hierarchical CGF/IMC bilayer architecture. Reprinted from ref (Yu et al. (2022)).
The repair of periodontal defects is a difficult problem of international concern. The existing traditional clinical treatment methods, such as GTR and GBR, fail to restore the physiological structure and function of teeth and periodontal tissues effectively. With the rapid advancements in cell therapy, stem cell transplantation has become the main focus and means of promoting tooth and periodontal regeneration. However, in vitro culture of stem cells requires strict conditions, complex procedures, and faces a huge risk of clinical application. The use of in vivo endogenous stem cell migration to promote tissue regeneration is expected to solve the difficulties of stem cells in vitro and reduce the risk of clinical application, which has a broad research prospect and clinical application space.
Stem cell homing involves a series of physiological processes such as cell adhesion, migration, proliferation, and remodeling, and requires appropriate scaffold materials as well as a certain microenvironment. Current research mainly includes in vitro cell experiments and in vivo animal experiments, while does not involve clinical trials yet. At present, the chemotactic strategies mainly use a variety of chemotactic agents, such as SDF-1, BMP-7, EX-4, PRF, to encourage the migration and proliferation of endogenous stem cells, as well as the differentiation into periodontal tissues. Some scaffold materials are also involved in chemotaxis. Additionally, the application of immune cells (such as macrophages) in cell homing has also been studied.
However, despite the validated role of stem cell homing in periodontal tissue regeneration, there are still significant limitations of the strategy. Firstly, the stem cell sources are hard to define, and the mechanisms by which chemotactic strategies promote stem cell homing have not been fully elucidated. Additionally, the property of endogenous stem cells may be affected under certain physiological conditions such as inflammation and aging, which will limit the effect of stem cell homing strategy. On the other hand, the currently used animal models are comparatively limited and lack in vivo findings in mammals. Meanwhile, due to the maturity of the technology itself, it has not been put into clinical trials.
In the future, the regulation of endogenous stem cell fate by different chemotactic agents and the combination of chemotactic agents and scaffold materials will remain the key point of research. Further understanding of homing mechanisms and development of methods to enhance this phenomenon may have further clinical benefits. At the same time, more advanced detection techniques are required to identify the source of recruited stem cells. In addition, improving the animal experimental model of periodontal tissue engineering is also the focus of future research. Only by clarifying the mechanism and obtaining reliable results on a suitable animal model can the transformation from basic experiments to clinical applications be achieved as soon as possible.
Conceived and designed the topic: HX and LM. Wrote the original draft: LM, YW, and HX. Analyzed the data: LM, YW, and YL. Prepared the figures LM, YW, YL, and QH. HX, YW, YL, QH, and LM edited the manuscript. All authors read and approved the final manuscript.
This work was supported by Medical Science and Technology Project of Sichuan Provincial Health Commission (No. 21PJ059), and Science and Technology Application Fundamental Project of Sichuan Province (No. 2022YFS0124).
The authors declare that the research was conducted in the absence of any commercial or financial relationships that could be construed as a potential conflict of interest.
The reviewer, XC, declared a shared affiliation with the authors LM, YW, YL, and HX, to the handling editor at the time of review.
All claims expressed in this article are solely those of the authors and do not necessarily represent those of their affiliated organizations, or those of the publisher, the editors and the reviewers. Any product that may be evaluated in this article, or claim that may be made by its manufacturer, is not guaranteed or endorsed by the publisher.
Abbott, J. D., Huang, Y., Liu, D., Hickey, R., Krause, D. S., and Giordano, F. J. (2004). Stromal cell-derived factor-1 alpha plays a critical role in stem cell recruitment to the heart after myocardial infarction but is not sufficient to induce homing in the absence of injury. Circulation 110, 3300–3305. doi:10.1161/01.Cir.0000147780.30124.Cf
Abdulghani, S., and Mitchell, G. R. (2019). Biomaterials for in situ tissue regeneration: A review. Biomolecules 9, 750. doi:10.3390/biom9110750
Ahmed, G. M., Abouauf, E. A., AbuBakr, N., Fouad, A. M., Dörfer, C. E., and Fawzy El-Sayed, K. M. (2021). Cell-based transplantation versus cell homing approaches for pulp-dentin complex regeneration. Stem Cells Int. 2021, 1–23. doi:10.1155/2021/8483668
Andreas, K., Sittinger, M., and Ringe, J. (2014). Toward in situ tissue engineering: Chemokine-guided stem cell recruitment. Trends Biotechnol. 32, 483–492. doi:10.1016/j.tibtech.2014.06.008
Andreasen, J. O., Andreasen, F. M., and Andersson, L. (1994). Textbook and color atlas of traumatic injuries to the teeth. Hoboken, Wiley-Blackwell: John Wiley & Sons.
Ardelt, A. A., Bhattacharyya, B. J., Belmadani, A., Ren, D. G., and Miller, R. J. (2013). Stromal derived growth factor-1 (CXCL12) modulates synaptic transmission to immature neurons during post-ischemic cerebral repair. Exp. Neurol. 248, 246–253. doi:10.1016/j.expneurol.2013.06.017
Barros, S. P., Silva, M. A. D., Somerman, M. J., and Nociti, F. H. (2003). Parathyroid hormone protects against periodontitis-associated bone loss. J. Dent. Res. 82, 791–795. doi:10.1177/154405910308201006
Bartold, P. M., McCulloch, C. A. G., Narayanan, A. S., and Pitaru, S. (2000). Tissue engineering: A new paradigm for periodontal regeneration based on molecular and cell biology. Periodontol. 2000 24, 253–269. doi:10.1034/j.1600-0757.2000.2240113.x
Bashutski, J. D., Eber, R. M., Kinney, J. S., Benavides, E., Maitra, S., Braun, T. M., et al. (2010). Teriparatide and osseous regeneration in the oral cavity. N. Engl. J. Med. Overseas. Ed. 363, 2396–2405. doi:10.1056/NEJMoa1005361
Blokzijl-Franke, S., Ponsioen, B., Schulte-Merker, S., Herbomel, P., Kissa, K., Choorapoikayil, S., et al. (2021). Phosphatidylinositol-3 kinase signaling controls survival and stemness of hematopoietic stem and progenitor cells. Oncogene 40, 2741–2755. doi:10.1038/s41388-021-01733-5
Bottino, M. C., Thomas, V., Schmidt, G., Vohra, Y. K., Chu, T.-M. G., Kowolik, M. J., et al. (2012). Recent advances in the development of GTR/GBR membranes for periodontal regeneration--a materials perspective. Dent. Mat. 28, 703–721. doi:10.1016/j.dental.2012.04.022
Bryan, D., Walker, K. B., Ferguson, M., and Thorpe, R. (2005). Cytokine gene expression in a murine wound healing model. Cytokine 31, 429–438. doi:10.1016/j.cyto.2005.06.015
Brzoska, E., Kowalewska, M., Markowska-Zagrajek, A., Kowalski, K., Archacka, K., Zimowska, M., et al. (2012). Sdf-1 (CXCL12) improves skeletal muscle regeneration via the mobilisation of Cxcr4 and CD34 expressing cells. Biol. Cell. 104, 722–737. doi:10.1111/boc.201200022
Cai, X., Yang, F., Walboomers, X. F., Wang, Y., Jansen, J. A., van den Beucken, J., Plachokova, A. S., et al. (2018). Periodontal regeneration via chemoattractive constructs. J. Clin. Periodontol. 45, 851–860. doi:10.1111/jcpe.12928
Cavalera, M., and Frangogiannis, N. G. (2014). Targeting the chemokines in cardiac repair. Curr. Pharm. Des. 20, 1971–1979. doi:10.2174/13816128113199990449
Chang, Y. C., and Zhao, J. H. (2011). Effects of platelet-rich fibrin on human periodontal ligament fibroblasts and application for periodontal infrabony defects. Aust. Dent. J. 56, 365–371. doi:10.1111/j.1834-7819.2011.01362.x
Chen, F. M., Lu, H., Wu, L. A., Gao, L. N., An, Y., and Zhang, J. (2013). Surface-engineering of glycidyl methacrylated dextran/gelatin microcapsules with thermo-responsive poly(N-isopropylacrylamide) gates for controlled delivery of stromal cell-derived factor-1α. Biomaterials 34, 6515–6527. doi:10.1016/j.biomaterials.2013.05.014
Chen, F. M., Wu, L. A., Zhang, M., Zhang, R., and Sun, H. H. (2011). Homing of endogenous stem/progenitor cells for in situ tissue regeneration: Promises, strategies, and translational perspectives. Biomaterials 32, 3189–3209. doi:10.1016/j.biomaterials.2010.12.032
Chen, F. M., Zhang, J., Zhang, M., An, Y., Chen, F., and Wu, Z. F. (2010). A review on endogenous regenerative technology in periodontal regenerative medicine. Biomaterials 31, 7892–7927. doi:10.1016/j.biomaterials.2010.07.019
Chen, F. M., Zhao, Y. M., Jin, Y., and Shi, S. T. (2012b). Prospects for translational regenerative medicine. Biotechnol. Adv. 30, 658–672. doi:10.1016/j.biotechadv.2011.11.005
Chen, J. D., Zhang, Y. J., Pan, P. P., Fan, T. T., Chen, M. M., and Zhang, Q. Q. (2015). In situ strategy for bone repair by facilitated endogenous tissue engineering. Colloids Surfaces B Biointerfaces 135, 581–587. doi:10.1016/j.colsurfb.2015.08.019
Cho, H., Tarafder, S., Fogge, M., Kao, K., and Lee, C. H. (2016). Periodontal ligament stem/progenitor cells with protein-releasing scaffolds for cementum formation and integration on dentin surface. Connect. Tissue Res. 57, 488–495. doi:10.1080/03008207.2016.1191478
Choukroun, J., Adda, F., Schoeffler, C., and Vervelle, A. (2001). Une opportunite´ en paro-implantologie: Le PRF. Implantodontie 42, 55–62.
Christopherson, K. W., Hangoc, G., and Broxmeyer, H. E. (2002). Cell surface peptidase CD26/dipeptidylpeptidase IV regulates CXCL12/stromal cell-derived factor-1 alpha-mediated chemotaxis of human cord blood CD34(+) progenitor cells. J. Immunol. 169, 7000–7008. doi:10.4049/jimmunol.169.12.7000
Christopherson, K. W., Hangoc, G., Mantel, C. R., and Broxmeyer, H. E. (2004). Modulation of hematopoietic stem cell homing and engraftment by CD26. Science 305, 1000–1003. doi:10.1126/science.1097071
Chu, G., Zhang, W., Han, F., Li, K., Liu, C., Wei, Q., et al. (2022). The role of microenvironment in stem cell-based regeneration of intervertebral disc. Front. Bioeng. Biotechnol. 10, 968862. doi:10.3389/fbioe.2022.968862
Chung, M. T., Liu, C. J., Hyun, J. S., Lo, D. D., Montoro, D. T., Hasegawa, M., et al. (2013). CD90 (Thy-1)-Positive selection enhances osteogenic capacity of human adipose-derived stromal cells. Tissue Eng. Part A 19, 989–997. doi:10.1089/ten.tea.2012.0370
Cipitria, A., Boettcher, K., Schoenhals, S., Garske, D. S., Schmidt-Bleek, K., Ellinghaus, A., et al. (2017). In-situ tissue regeneration through SDF-1 alpha driven cell recruitment and stiffness-mediated bone regeneration in a critical-sized segmental femoral defect. Acta Biomater. 60, 50–63. doi:10.1016/j.actbio.2017.07.032
Cook, S. D., Salkeld, S. L., and Rueger, D. C. (1995). Evaluation of recombinant human osteogenic protein-1 (rhOP-1) placed with dental implants in fresh extraction sites. J. Oral Implantol. 21, 281–289.
Dan, H. X., Vaquette, C., Fisher, A. G., Hamlet, S. M., Xiao, Y., Hutmacher, D. W., et al. (2014). The influence of cellular source on periodontal regeneration using calcium phosphate coated polycaprolactone scaffold supported cell sheets. Biomaterials 35, 113–122. doi:10.1016/j.biomaterials.2013.09.074
Darveau, R. P. (2010). Periodontitis: A polymicrobial disruption of host homeostasis. Nat. Rev. Microbiol. 8, 481–490. doi:10.1038/nrmicro2337
Ding, T., Li, J., Zhang, X., Du, L., Li, Y., Li, D., et al. (2020). Super-assembled core/shell fibrous frameworks with dual growth factors for in situ cementum-ligament-bone complex regeneration. Biomater. Sci. 8, 2459–2471. doi:10.1039/d0bm00102c
Dominici, M., Le Blanc, K., Mueller, I., Slaper-Cortenbach, I., Marini, F. C., Krause, D. S., et al. (2006). Minimal criteria for defining multipotent mesenchymal stromal cells. The International Society for Cellular Therapy position statement. Cytotherapy 8, 315–317. doi:10.1080/14653240600855905
Ehrenfest, D. M. D., Doglioli, P., de Peppo, G. M., Del Corso, M., and Charrier, J. B. (2010). Choukroun's platelet-rich fibrin (PRF) stimulates in vitro proliferation and differentiation of human oral bone mesenchymal stem cell in a dose-dependent way. Archives Oral Biol. 55, 185–194. doi:10.1016/j.archoralbio.2010.01.004
Feng, Y. Y., Su, L., Zhong, X., Wei, G. H., Xiao, H. P., Li, Y. B., et al. (2016). Exendin-4 promotes proliferation and differentiation of MC3T3-E1 osteoblasts by MAPKs activation. J. Mol. Endocrinol. 56, 189–199. doi:10.1530/jme-15-0264
Giannobile, W. V., Ryan, S., Shih, M. S., Su, D. L., Kaplan, P. L., and Chan, T. C. K. (1998). Recombinant human osteogenic protein-1 (OP-1) stimulates periodontal wound healing in class III furcation defects. J. Periodontology 69, 129–137. doi:10.1902/jop.1998.69.2.129
Gronthos, S., Mankani, M., Brahim, J., Robey, P. G., and Shi, S. (2000). Postnatal human dental pulp stem cells (DPSCs) in vitro and in vivo. Proc. Natl. Acad. Sci. U. S. A. 97, 13625–13630. doi:10.1073/pnas.240309797
Guo, W. H., Gong, K., Shi, H. G., Zhu, G. X., He, Y., Ding, B. F., et al. (2012). Dental follicle cells and treated dentin matrix scaffold for tissue engineering the tooth root. Biomaterials 33, 1291–1302. doi:10.1016/j.biomaterials.2011.09.068
Hajjar, R. J., and Hulot, J. S. (2013). Myocardial delivery of stromal cell-derived factor 1 in patients with ischemic heart disease safe and promising. Circ. Res. 112, 746–747. doi:10.1161/circresaha.113.300902
Hasegawa, N., Kawaguchi, H., Hirachi, A., Takeda, K., Mizuno, N., Nishimura, M., et al. (2006). Behavior of transplanted bone marrow-derived mesenchymal stem cells in periodontal defects. J. Periodontology 77, 1003–1007. doi:10.1902/jop.2006.050341
Hattori, K., Heissig, B., and Rafii, S. (2003). The regulation of hematopoietic stem cell and progenitor mobilization by chemokine SDF-1. Leukemia Lymphoma 44, 575–582. doi:10.1080/1042819021000037985
He, X. T., Li, X., Xia, Y., Yin, Y., Wu, R. X., Sun, H. H., et al. (2019). Building capacity for macrophage modulation and stem cell recruitment in high-stiffness hydrogels for complex periodontal regeneration: Experimental studies in vitro and in rats. Acta Biomater. 88, 162–180. doi:10.1016/j.actbio.2019.02.004
Huang, C. Y. C., Pelaez, D., Bendala, J. D., Garcia-Godoy, F., and Cheung, H. S. (2009a). Plasticity of stem cells derived from adult periodontal ligament. Regen. Med. 4, 809–821. doi:10.2217/RME.09.55
Huang, G. T. J., Gronthos, S., and Shi, S. (2009b). Mesenchymal stem cells derived from dental tissues vs. Those from other sources: Their biology and role in regenerative medicine. J. Dent. Res. 88, 792–806. doi:10.1177/0022034509340867
Huber, B. C., Brunner, S., Segeth, A., Nathan, P., Fischer, R., Zaruba, M. M., et al. (2011). Parathyroid hormone is a DPP-IV inhibitor and increases SDF-1-driven homing of CXCR4(+) stem cells into the ischaemic heart. Cardiovasc. Res. 90, 529–537. doi:10.1093/cvr/cvr014
Hynes, K., Menicanin, D., Gronthos, S., and Bartold, P. M. (2012). Clinical utility of stem cells for periodontal regeneration. Periodontol. 2000 59, 203–227. doi:10.1111/j.1600-0757.2012.00443.x
Ikeda, E., Morita, R., Nakao, K., Ishida, K., Nakamura, T., Takano-Yamamoto, T., et al. (2009). Fully functional bioengineered tooth replacement as an organ replacement therapy. Proc. Natl. Acad. Sci. U. S. A. 106, 13475–13480. doi:10.1073/pnas.0902944106
Ji, B., Sheng, L., Chen, G., Guo, S., Xie, L., Yang, B., et al. (2015). The combination use of platelet-rich fibrin and treated dentin matrix for tooth root regeneration by cell homing. Tissue Eng. Part A 21, 26–34. doi:10.1089/ten.tea.2014.0043
Ji, W., Yang, F., Ma, J. L., Bouma, M. J., Boerman, O. C., Chen, Z., et al. (2013). Incorporation of stromal cell-derived factor-1 alpha in PCL/gelatin electrospun membranes for guided bone regeneration. Biomaterials 34, 735–745. doi:10.1016/j.biomaterials.2012.10.016
Kawaguchi, H., Hirachi, A., Hasegawa, N., Iwata, T., Hamaguchi, H., Shiba, H., et al. (2004). Enhancement of periodontal tissue regeneration by transplantation of bone marrow mesenchymal stem cells. J. Periodontology 75, 1281–1287. doi:10.1902/jop.2004.75.9.1281
Kim, K., Lee, C. H., Kim, B. K., and Mao, J. J. (2010). Anatomically shaped tooth and periodontal regeneration by cell homing. J. Dent. Res. 89, 842–847. doi:10.1177/0022034510370803
Kim, K. S., Kim, H. S., Park, J. M., Kim, H. W., Park, M. K., Lee, H. S., et al. (2013). Long-term immunomodulatory effect of amniotic stem cells in an Alzheimer's disease model. Neurobiol. Aging 34, 2408–2420. doi:10.1016/j.neurobiolaging.2013.03.029
Kimura, Y., Komaki, M., Iwasaki, K., Sata, M., Izumi, Y., and Morita, I. (2014). Recruitment of bone marrow-derived cells to periodontal tissue defects. Front. Cell. Dev. Biol. 2, 19. doi:10.3389/fcell.2014.00019
Kinane, D. F., Stathopoulou, P. G., and Papapanou, P. N. (2017). Periodontal diseases. Nat. Rev. Dis. Prim. 3, 17038. doi:10.1038/nrdp.2017.38
Kwon, S. G., Kwon, Y. W., Lee, T. W., Park, G. T., and Kim, J. H. (2018). Recent advances in stem cell therapeutics and tissue engineering strategies. Biomater. Res. 22, 36. doi:10.1186/s40824-018-0148-4
Lee, C. H., Cook, J. L., Mendelson, A., Moioli, E. K., Yao, H., and Mao, J. J. (2010). Regeneration of the articular surface of the rabbit synovial joint by cell homing: A proof of concept study. Lancet 376, 440–448. doi:10.1016/s0140-6736(10)60668-x
Lee, C. H., Marion, N. W., Hollister, S., and Mao, J. J. (2009). tissue formation and vascularization in anatomically shaped human joint condyle ectopically in vivo. TISSUE Eng. PART A 15, 3923–3930. doi:10.1089/ten.tea.2008.0653
Lee, J. S., Jin, Y., Park, H. J., Yang, K., Lee, M. S., Yang, H. S., et al. (2017). In situ bone tissue engineering with an endogenous stem cell mobilizer and osteoinductive nanofibrous polymeric scaffolds. Biotechnol. J. 12, 1700062. doi:10.1002/biot.201700062
Lee, S. J., Yoo, J. J., and Atala, A. (2016). Chapter 1 - fundamentals of in situ tissue regeneration. situ tissue Regen. 2016, 3–17. doi:10.1016/B978-0-12-802225-2.00001-5
Li, D., Xue, W., Li, M., Dong, M., Wang, J., Wang, X., et al. (2018a). VCAM-1(+) macrophages guide the homing of HSPCs to a vascular niche. Nature 564, 119–124. doi:10.1038/s41586-018-0709-7
Li, R., Guo, W. H., Yang, B., Guo, L. J., Sheng, L., Chen, G., et al. (2011). Human treated dentin matrix as a natural scaffold for complete human dentin tissue regeneration. Biomaterials 32, 4525–4538. doi:10.1016/j.biomaterials.2011.03.008
Li, Y. C., Jin, F., Du, Y., Ma, Z. W., Li, F., Wu, G., et al. (2008). Cementum and periodontal ligament-like tissue formation induced using bioengineered dentin. Tissue Eng. Part A 14, 1731–1742. doi:10.1089/ten.tea.2007.0268
Li, Z., Shen, D., Hu, S., Su, T., Huang, K., Liu, F., et al. (2018b). Pretargeting and bioorthogonal click chemistry-mediated endogenous stem cell homing for heart repair. ACS Nano 12, 12193–12200. doi:10.1021/acsnano.8b05892
Liang, Q., Du, L., Zhang, R., Kang, W., and Ge, S. (2021). Stromal cell-derived factor-1/Exendin-4 cotherapy facilitates the proliferation, migration and osteogenic differentiation of human periodontal ligament stem cells in vitro and promotes periodontal bone regeneration in vivo. Cell. Prolif. 54, e12997. doi:10.1111/cpr.12997
Liesveld, J. L., Sharma, N., and Aljitawi, O. S. (2020). Stem cell homing: From physiology to therapeutics. Stem Cells 38, 1241–1253. doi:10.1002/stem.3242
Liu, H. R., Li, M. Q., Du, L. Q., Yang, P. S., and Ge, S. H. (2015). Local administration of stromal cell-derived factor-1 promotes stem cell recruitment and bone regeneration in a rat periodontal bone defect model. Mater. Sci. Eng. C 53, 83–94. doi:10.1016/j.msec.2015.04.002
Liu, J., Ruan, J., Weir, M. D., Ren, K., Schneider, A., Wang, P., et al. (2019a). Periodontal bone-ligament-cementum regeneration via scaffolds and stem cells. Cells 8, 537. doi:10.3390/cells8060537
Liu, W. T., Wei, Y., Zhang, X. H., Xu, M. M., Yang, X. P., and Deng, X. L. (2013). Lower extent but similar rhythm of osteogenic behavior in hBMSCs cultured on nanofibrous scaffolds versus induced with osteogenic supplement. Acs Nano 7, 6928–6938. doi:10.1021/nn402118s
Liu, Y., Luo, D., Yu, M., Wang, Y., Jin, S. S., Li, Z. X., et al. (2019b). Thermodynamically controlled self-assembly of hierarchically staggered architecture as an osteoinductive alternative to bone autografts. Adv. Funct. Mat. 29, 1806445. doi:10.1002/adfm.201806445
Liu, Y., Zheng, Y., Ding, G., Fang, D. J., Zhang, C. M., Bartold, P. M., et al. (2008). Periodontal ligament stem cell-mediated treatment for periodontitis in miniature swine. STEM CELLS 26, 1065–1073. doi:10.1634/stemcells.2007-0734
Luciani, P., Fibbi, B., Mazzanti, B., Deledda, C., Ballerini, L., Aldinucci, A., et al. (2018). The effects of Exendin-4 on bone marrow-derived mesenchymal cells. Endocrine 60, 423–434. doi:10.1007/s12020-017-1430-2
Ma, Z. F., Li, S., Song, Y. L., Tang, L., Ma, D. D., Liu, B. L., et al. (2008). The biological effect of dentin noncollagenous proteins (DNCPs) on the human periodontal ligament stem cells (HPDLSCs) in vitro and in vivo. Tissue Eng. Part A 14, 2059–2068. doi:10.1089/ten.tea.2008.0021
Mao, Y., Chen, Y., Li, W., Wang, Y., Qiu, J., Fu, Y., et al. (2022). Physiology-Inspired multilayer nanofibrous membranes modulating endogenous stem cell recruitment and osteo-differentiation for staged bone regeneration. Adv. Healthc. Mat. 2022, e2201457. doi:10.1002/adhm.202201457
Martino, M. M., Briquez, P. S., Maruyama, K., and Hubbell, J. A. (2015). Extracellular matrix-inspired growth factor delivery systems for bone regeneration. Adv. Drug Deliv. Rev. 94, 41–52. doi:10.1016/j.addr.2015.04.007
Meng, J. R., Ma, X., Wang, N., Jia, M., Bi, L., Wang, Y. Y., et al. (2016). Activation of GLP-1 receptor promotes bone marrow stromal cell osteogenic differentiation through beta-catenin. Stem Cell. Rep. 6, 579–591. doi:10.1016/j.stemcr.2016.02.002
Miura, M., Gronthos, S., Zhao, M. R., Lu, B., Fisher, L. W., Robey, P. G., et al. (2003). Shed: Stem cells from human exfoliated deciduous teeth. Proc. Natl. Acad. Sci. U. S. A. 100, 5807–5812. doi:10.1073/pnas.0937635100
Modino, S. A. C., and Sharpe, P. T. (2005). Tissue engineering of teeth using adult stem cells. ARCHIVES ORAL Biol. 50, 255–258. doi:10.1016/j.archoralbio.2005.01.002
Morrison, S. J., and Scadden, D. T. (2014). The bone marrow niche for haematopoietic stem cells. Nature 505, 327–334. doi:10.1038/nature12984
Morsczeck, C., Gotz, W., Schierholz, J., Zellhofer, F., Kuhn, U., Mohl, C., et al. (2005). Isolation of precursor cells (PCs) from human dental follicle of wisdom teeth. MATRIX Biol. 24, 155–165. doi:10.1016/j.matbio.2004.12.004
Otsuru, S., Tamai, K., Yamazaki, T., Yoshikawa, H., and Kaneda, Y. (2008). Circulating bone marrow-derived osteoblast progenitor cells are recruited to the bone-forming site by the CXCR4/stromal cell-derived factor-1 pathway. Stem Cells 26, 223–234. doi:10.1634/stemcells.2007-0515
Pacelli, S., Basu, S., Whitlow, J., Chakravarti, A., Acosta, F., Varshney, A., et al. (2017). Strategies to develop endogenous stem cell-recruiting bioactive materials for tissue repair and regeneration. Adv. Drug Deliv. Rev. 120, 50–70. doi:10.1016/j.addr.2017.07.011
Park, J. Y., Jeon, S. H., and Choung, P. H. (2011). Efficacy of periodontal stem cell transplantation in the treatment of advanced periodontitis. Cell. Transpl. 20, 271–286. doi:10.3727/096368910X519292
Petit, I., Jin, D., and Rafii, S. (2007). The SDF-1-CXCR4 signaling pathway: A molecular hub modulating neo-angiogenesis. Trends Immunol. 28, 299–307. doi:10.1016/j.it.2007.05.007
Phillips, C. L., Bradley, D. A., Schlotzhauer, C. L., Bergfeld, M., Libreros-Minotta, C., Gawenis, L. R., et al. (2000). Oim mice exhibit altered femur and incisor mineral composition and decreased bone mineral density. Bone 27, 219–226. doi:10.1016/s8756-3282(00)00311-2
Pihlstrom, B. L., Michalowicz, B. S., and Johnson, N. W. (2005). Periodontal diseases. Lancet 366, 1809–1820. doi:10.1016/s0140-6736(05)67728-8
Pittenger, M. F., Mackay, A. M., Beck, S. C., Jaiswal, R. K., Douglas, R., Mosca, J. D., et al. (1999). Multilineage potential of adult human mesenchymal stem cells. Science 284, 143–147. doi:10.1126/science.284.5411.143
Ranzoni, A. M., Tangherloni, A., Berest, I., Riva, S. G., Myers, B., Strzelecka, P. M., et al. (2021). Integrative single-cell RNA-seq and ATAC-seq analysis of human developmental hematopoiesis. Cell. Stem Cell. 28, 472–487.e7. e7. doi:10.1016/j.stem.2020.11.015
Reyes, C. D., and García, A. J. (2004). α2β1integrin-specific collagen-mimetic surfaces supporting osteoblastic differentiation. J. Biomed. Mat. Res. A 69, 591–600. doi:10.1002/jbm.a.30034
Ripamonti, U., Crooks, J., Petit, J. C., and Rueger, D. C. (2001). Periodontal tissue regeneration by combined applications of recombinant human osteogenic protein-1 and bone morphogenetic protein-2. A pilot study in Chacma baboons (Papio ursinus). Eur. J. Oral Sci. 109, 241–248. doi:10.1034/j.1600-0722.2001.00041.x
Safina, I., and Embree, M. C. (2022). Biomaterials for recruiting and activating endogenous stem cells in situ tissue regeneration. Acta Biomater. 143, 26–38. doi:10.1016/j.actbio.2022.03.014
Seo, B. M., Miura, M., Gronthos, S., Bartold, P. M., Batouli, S., Brahim, J., et al. (2004). Investigation of multipotent postnatal stem cells from human periodontal ligament. Lancet 364, 149–155. doi:10.1016/S0140-6736(04)16627-0
Shafiq, M., Zhang, Y., Zhu, D., Zhao, Z., Kim, D. H., Kim, S. H., et al. (2018). In situ cardiac regeneration by using neuropeptide substance P and IGF-1C peptide eluting heart patches. Regen. Biomater. 5, 303–316. doi:10.1093/rb/rby021
Sharma, D., Verma, S., Vaidya, S., Kalia, K., and Tiwari, V. (2018). Recent updates on GLP-1 agonists: Current advancements & challenges. Biomed. Pharmacother. 108, 952–962. doi:10.1016/j.biopha.2018.08.088
Smith, A. J., Scheuen, B. A., Takahashi, Y., Ferracane, J. L., Shelton, R. M., and Cooper, P. R. (2012). Dentine as a bioactive extracellular matrix. Archives Oral Biol. 57, 109–121. doi:10.1016/j.archoralbio.2011.07.008
Sonoyama, W., Liu, Y., Yamaza, T., Tuan, R. S., Wang, S., Shi, S., et al. (2008). Characterization of the apical papilla and its residing stem cells from human immature permanent teeth: A pilot study. J. Endod. 34, 166–171. doi:10.1016/j.joen.2007.11.021
Tokunaga, K., Seto, H., Ohba, H., Mihara, C., Hama, H., Horibe, M., et al. (2011). Topical and intermittent application of parathyroid hormone recovers alveolar bone loss in rat experimental periodontitis. J. Periodontal Res. 46, 655–662. doi:10.1111/j.1600-0765.2011.01386.x
Wang, F., Du, L. Q., and Ge, S. H. (2016). PTH/SDF-1 alpha cotherapy induces CD90+CD34-stromal cells migration and promotes tissue regeneration in a rat periodontal defect model. Sci. Rep. 6, 30403. doi:10.1038/srep30403
Wang, K., Zhao, X. H., Kuang, C. Y., Qian, D. H., Wang, H., Jiang, H., et al. (2012). Overexpression of SDF-1 alpha enhanced migration and engraftment of cardiac stem cells and reduced infarcted size via CXCR4/PI3K pathway. Plus One 7, e43922. doi:10.1371/journal.pone.0043922
Woo, H. N., Cho, Y. J., Tarafder, S., and Lee, C. H. (2021). The recent advances in scaffolds for integrated periodontal regeneration. Bioact. Mater. 6, 3328–3342. doi:10.1016/j.bioactmat.2021.03.012
Woodfield, T. B. F., Van Blitterswijk, C. A., De Wijn, J., Sims, T. J., Hollander, A. P., and Riesle, J. (2005). Polymer scaffolds fabricated with pore-size gradients as a model for studying the zonal organization within tissue-engineered cartilage constructs. TISSUE Eng. 11, 1297–1311. doi:10.1089/ten.2005.11.1297
Wu, J., Jin, F., Tang, L., Yu, J., Xu, L., Yang, Z., et al. (2008). Dentin non-collagenous proteins (dNCPs) can stimulate dental follicle cells to differentiate into cementoblast lineages. Biol. Cell. 100, 291–302. doi:10.1042/bc20070092
Xin, L., Zheng, X., Chen, J., Hu, S., Luo, Y., Ge, Q., et al. (2022). An acellular scaffold facilitates endometrial regeneration and fertility restoration via recruiting endogenous mesenchymal stem cells. Adv. Healthc. Mat. 2022, e2201680. doi:10.1002/adhm.202201680
Xiong, X., Ghosh, R., Hiller, E., Drepper, F., Knapp, B., Brunner, H., et al. (2009). A new procedure for rapid, high yield purification of Type I collagen for tissue engineering. Process Biochem. 44, 1200–1212. doi:10.1016/j.procbio.2009.06.010
Xu, X. Y., Li, X., Wang, J., He, X. T., Sun, H. H., and Chen, F. M. (2019). Concise review: Periodontal tissue regeneration using stem cells: Strategies and translational considerations. Stem Cells Transl. Med. 8, 392–403. doi:10.1002/sctm.18-0181
Yamamoto, T., Ugawa, Y., Kawamura, M., Yamashiro, K., Kochi, S., Ideguchi, H., et al. (2018). Modulation of microenvironment for controlling the fate of periodontal ligament cells: The role of rho/ROCK signaling and cytoskeletal dynamics. J. Cell. Commun. Signal. 12, 369–378. doi:10.1007/s12079-017-0425-3
Yang, B., Chen, G., Li, J., Zou, Q., Xie, D., Chen, Y. L., et al. (2012). Tooth root regeneration using dental follicle cell sheets in combination with a dentin matrix - based scaffold. Biomaterials 33, 2449–2461. doi:10.1016/j.biomaterials.2011.11.074
Yang, B., Pang, X., Li, Z., Chen, Z., and Wang, Y. (2021). Immunomodulation in the treatment of periodontitis: Progress and perspectives. Front. Immunol. 12, 781378. doi:10.3389/fimmu.2021.781378
Yang, D. Z., Sun, S. J., Wang, Z. G., Zhu, P. F., Yang, Z. L., and Zhang, B. (2013). Stromal cell-derived factor-1 receptor CXCR4-overexpressing bone marrow mesenchymal stem cells accelerate wound healing by migrating into skin injury areas. Cell. Reprogr. 15, 206–215. doi:10.1089/cell.2012.0046
Yang, Z., Li, H., Yuan, Z., Fu, L., Jiang, S., Gao, C., et al. (2020). Endogenous cell recruitment strategy for articular cartilage regeneration. Acta Biomater. 114, 31–52. doi:10.1016/j.actbio.2020.07.008
Yao, D., and Lv, Y. (2022). A cell-free difunctional demineralized bone matrix scaffold enhances the recruitment and osteogenesis of mesenchymal stem cells by promoting inflammation resolution. Biomater. Adv. 139, 213036. doi:10.1016/j.bioadv.2022.213036
Yap, M. K. K., and Misuan, N. (2019). Exendin-4 from Heloderma suspectum venom: From discovery to its latest application as type II diabetes combatant. Basic Clin. Pharmacol. Toxicol. 124, 513–527. doi:10.1111/bcpt.13169
Yin, Y., Li, X., He, X. T., Wu, R. X., Sun, H. H., and Chen, F. M. (2017). Leveraging stem cell homing for therapeutic regeneration. J. Dent. Res. 96, 601–609. doi:10.1177/0022034517706070
Young, C. S., Terada, S., Vacanti, J. P., Honda, M., Bartlett, J. D., and Yelick, P. C. (2002). Tissue engineering of complex tooth structures on biodegradable polymer scaffolds. J. Dent. Res. 81, 695–700. doi:10.1177/154405910208101008
Yu, M., Luo, D., Qiao, J., Guo, J., He, D., Jin, S., et al. (2022). A hierarchical bilayer architecture for complex tissue regeneration. Bioact. Mat. 10, 93–106. doi:10.1016/j.bioactmat.2021.08.024
Zhang, Q. Z., Shi, S. H., Liu, Y., Uyanne, J., Shi, Y. F., Shi, S. T., et al. (2009). Mesenchymal stem cells derived from human gingiva are capable of immunomodulatory functions and ameliorate inflammation-related tissue destruction in experimental colitis. J. Immunol. 183, 7787–7798. doi:10.4049/jimmunol.0902318
Zhang, S., Hu, B., Liu, W., Wang, P., Lv, X., Chen, S., et al. (2020). Articular cartilage regeneration: The role of endogenous mesenchymal stem/progenitor cell recruitment and migration. Semin. Arthritis Rheum. 50, 198–208. doi:10.1016/j.semarthrit.2019.11.001
Zhang, Y., Chen, S., Liu, B. C., Zhou, H., Hu, S. Y., Zhou, Y., et al. (2016). Exendin-4 promotes proliferation of adipose-derived stem cells through ERK and JNK signaling pathways. Vitro Cell. Dev. Biol. -Animal. 52, 598–606. doi:10.1007/s11626-016-0003-7
Zhao, Y., Zhao, S., Ma, Z., Ding, C., Chen, J., and Li, J. (2022). Chitosan-based scaffolds for facilitated endogenous bone Re-generation. Pharm. (Basel) 15, 1023. doi:10.3390/ph15081023
Zheng, C., Chen, J., Liu, S., and Jin, Y. (2019). Stem cell-based bone and dental regeneration: A view of microenvironmental modulation. Int. J. Oral Sci. 11, 23. doi:10.1038/s41368-019-0060-3
Zhou, H., Li, D. D., Shi, C., Xin, T., Yang, J. J., Zhou, Y., et al. (2015). Effects of Exendin-4 on bone marrow mesenchymal stem cell proliferation, migration, and apoptosis in vitro. Sci. Rep. 5, 12898. doi:10.1038/srep12898
Keywords: periodontal regeneration, endogenous stem cell, cell homing, regenerative medicine, stimulation strategies
Citation: Meng L, Wei Y, Liang Y, Hu Q and Xie H (2022) Stem cell homing in periodontal tissue regeneration. Front. Bioeng. Biotechnol. 10:1017613. doi: 10.3389/fbioe.2022.1017613
Received: 12 August 2022; Accepted: 03 October 2022;
Published: 13 October 2022.
Edited by:
Jianxun Ding, Changchun Institute of Applied Chemistry (CAS), ChinaReviewed by:
Lei Hu, Beijing Stomatological Hospital, Capital Medical University, ChinaCopyright © 2022 Meng, Wei, Liang, Hu and Xie. This is an open-access article distributed under the terms of the Creative Commons Attribution License (CC BY). The use, distribution or reproduction in other forums is permitted, provided the original author(s) and the copyright owner(s) are credited and that the original publication in this journal is cited, in accordance with accepted academic practice. No use, distribution or reproduction is permitted which does not comply with these terms.
*Correspondence: Huixu Xie, YWl0aWFuMDA3QDEyNi5jb20=
Disclaimer: All claims expressed in this article are solely those of the authors and do not necessarily represent those of their affiliated organizations, or those of the publisher, the editors and the reviewers. Any product that may be evaluated in this article or claim that may be made by its manufacturer is not guaranteed or endorsed by the publisher.
Research integrity at Frontiers
Learn more about the work of our research integrity team to safeguard the quality of each article we publish.