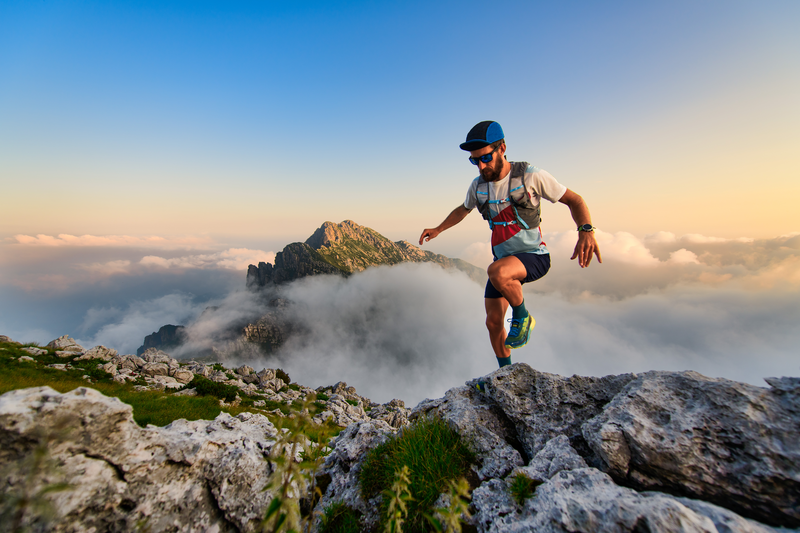
94% of researchers rate our articles as excellent or good
Learn more about the work of our research integrity team to safeguard the quality of each article we publish.
Find out more
ORIGINAL RESEARCH article
Front. Bioeng. Biotechnol. , 28 January 2022
Sec. Bioprocess Engineering
Volume 9 - 2021 | https://doi.org/10.3389/fbioe.2021.812403
This article is part of the Research Topic Biotransformation of Carbohydrates View all 5 articles
Magnetic α-Fe2O3/Fe3O4 heterostructure nanosheets were fabricated via hydrothermal calcination. The activity of penicillin G acylase (PGA), which was covalently immobilized onto silica-decorated heterostructure nanosheets, achieved the highest activity of 387.03 IU/g after 18 h of incubation with 0.1 ml of PGA. In contrast, the activity of free PGA reached the highest level when the temperature was 45°C with a pH of 8.0. However, the activity of free PGA changed more dramatically than immobilized PGA as the relative conditions changed. Moreover, the Michaelis–Menten constant (Km) and reusability of immobilized PGA were also explored. The results showed that free PGA Km and maximum rate (Vmax) were 0.0274 M and 1.167 μl/min, respectively. Km and Vmax values of immobilized PGA were 0.1082 M and 1.294 μl/min, respectively. After 12 cycles of repetitive use, immobilized PGA remained approximately 66% of its initial activity, indicating that the PGA immobilized onto the heterostructure nanosheets showed better stability and reusability than free PGA.
Currently, magnetic materials are attracting great attention from researchers due to their promising applications in various fields, such as biotechnology (Alizadeh and Salimi, 2021), catalysis (Liu et al., 2021a), electrochemistry (Wang et al., 2021), and biosensors (Dalkıran et al., 2019). Iron (II, III) oxide (Fe3O4) nanocomposites have many applications in the enzymatic immobilization field (Li et al., 2019), as they contain the Fe3+ (Liu et al., 2016) and Fe2+ antispinel structures. Moreover, Fe3O4 nanocomposites have structural stability (Pakapongpan and Poo-arporn, 2017), light resistance, good biocompatibility, and good magnetic responsiveness. However, the magnetism of Fe3O4 produces diffusion restrictions (Liu et al., 2021b). Thus, magnetic α-iron (III) oxide (Fe2O3)/Fe3O4 heterogeneous nanomaterials (Zhuang et al., 2015) have been developed to overcome this drawback. The magnetic α-Fe2O3/Fe3O4 heterogeneous nanomaterials immobilized with enzymes (Ansari and Husain, 2012) can be separated by applying an external magnetic field, with the advantages of low toxicity, biocompatibility, simple operation, biodegradation, and effectively reduced capital investment. However, they are also accompanied by the disadvantages of low chemical stability, high concentration tendency, and low immobilized enzyme volume (Angelakeris, 2017). Hence, it is of great significance to combine inorganic or organic materials into magnetic nanocomposites (Pakapongpan and Poo-arporn, 2017) to improve material stability, delay oxidation, and enable better application of immobilized enzymes (Senapati et al., 2018). In this study, the stability of magnetic nanocomposites in an acidic environment was improved by coating them with silicon dioxide (SiO2). The specific surface area of the nanomaterials was significantly increased, and the abundant surface groups were suitable for the modification and transformation of nanomaterials, which was of great significance for enzymatic immobilization.
Immobilization techniques for modifying various enzymes are affected by many factors, including the carrier used for the immobilization, properties of the enzyme, solvent type, immobilization process conditions, and reaction medium (Sirisha et al., 2016). Therefore, the enzymatic immobilization approaches should be appropriately selected, according to the immobilized object characteristics and the application direction of the immobilization technology (Zhou and Hartmann, 2013). With the rapid development of science and technology, various methods of enzymatic immobilization have been developed, such as the adsorption approach (Fernandez-Lorente et al., 2020), embedding process (Nadar et al., 2020), binding process (Farid et al., 2020), and crosslinking approach (Tanabe et al., 2004). Among these, the crosslinking approach was chosen in the present study owing to the stability between the substrate and the enzyme. Commonly used crosslinking agents include dicarboxylic acid, glutaraldehyde (GA) (Rajesh et al., 2018), dibutyltin dilaurate, and dimethyl adipic imide.
Penicillin G acylase (PGA) is a critical catalyst in the biological field (Chen et al., 2018). It can hydrolyze benzylpenicillin (penicillin G) to produce side chain-free penicillin, which can be applied to the synthesis of β-lactam antibiotics in the industry (Xue et al., 2015; Xue et al., 2016). These antibiotics play a critical role in our daily lives, and they are often used to treat diseases caused by various microorganisms, ranging from viruses to bacteria (Yu et al., 2019). As a hydrolytic enzyme, PGA has mild conditions of reaction, high sensitivity, high activity, and good substrate selectivity (Liu et al., 2018). However, when free PGA is used directly, it is easily affected by pH, temperature, and other environmental conditions. In addition, it was shown to be challenging to separate free PGA from the reaction system and purify 6-aminopenicillanic acid (6-APA), a compound used for the synthesis of semisynthetic penicillin (Wang et al., 2018), resulting in product contamination and recycling difficulties. The above reasons limit the application of free PGA in the industry. Therefore, PGA immobilization has been developed to solve these problems to a certain extent (Liu et al., 2014; Yang et al., 2014).
In the present study, magnetic α-Fe2O3/Fe3O4 heterogeneous nanosheets were successfully fabricated via hydrothermal calcination. The magnetic nanomaterials were first coated with a silicon layer and then functionalized with glutaraldehyde (GA). PGA was successfully immobilized onto functionalized heterogeneous nanosheets, and the properties of immobilized PGA were evaluated. The immobilization process is shown in Figure 1.
FIGURE 1. The process of penicillin G acylase (PGA) immobilized onto α-Fe2O3/Fe3O4 heterogeneous nanosheets.
Briefly, 0.541 g of FeCl3·6H2O and 0.094 g of NaH2PO4·2H2O were completely dissolved in 80 ml of distilled water by stirring with a magnetic stirrer and hydrothermally heating at 220°C for 24 h. The obtained suspension was rinsed with absolute alcohol and distilled water several times to remove impurities and then centrifuged six times. After removing the supernatant, the obtained intermedium solution was placed in a vacuum drying oven for 12 h to obtain the magnetic α-Fe2O3 nanosheets. Finally, 0.1 g of α-Fe2O3 nanosheets were uniformly mixed with 0.4 g of C6H12O6·H2O at a heating velocity of 3°C/min, and calcined at 600°C for 4 h (Liu et al., 2020). Magnetic α-Fe2O3/Fe3O4 heterogeneous nanosheets were obtained after grinding.
Scanning electron microscopy (SEM) and transmission electron microscopy (TEM) were used to evaluate the morphology of the magnetic α-Fe2O3/Fe3O4 heterogeneous nanosheets. Phase identification of the nanomaterials was performed by x-ray diffraction (XRD), and a vibrating sample magnetometer (VSM) was used to measure the magnetic properties of the products.
First, 1.0 g of α-Fe2O3/Fe3O4 heterogeneous nanosheets mixed with 200 ml of distilled water was transferred into a round bottom flask with a magnetic stirring bar and heated at 80°C for 3 h. Then 10 ml of 1.0 M Na2SiO3 was slowly added to the resulting suspension with rapid stirring. Simultaneously, a small amount of 2.0 M HCl was added to the solution to maintain pH 6.0. The magnetic α-Fe2O3/Fe3O4@SiO2 nanomaterials were successfully obtained after several rounds of washing and centrifugation, and finally drying. Next, 0.1 g of magnetic α-Fe2O3/Fe3O4@SiO2 nanomaterials were placed in a mini centrifuge tube, and 0.2 ml of 25% glutaraldehyde (Sinopharm Chemical Reagent Co., Ltd., Shanghai, China) with 1 ml of 0.05 M phosphate-buffered saline (PBS, pH 7.0) was added to the tube and stirred for 2 h. After this period, the solution was centrifuged, and the supernatant was removed. Magnetic α-Fe2O3/Fe3O4@SiO2-CHO nanocomposites were successfully fabricated after rinsing with 1 ml of 1.0 M NaCl.
Then, 4.6 ml of diluted PGA solution, prepared with 0.1 ml penicillin and 4.5 ml PBS (pH 8.0), was added to 0.1 g of magnetic α-Fe2O3/Fe3O4@SiO2-CHO nanocomposites. The solution was uniformly mixed following a shaking time of 18 h. After centrifugation, the content of enzymatic protein in the upper liquid was stained with Coomassie blue G250 (Sinopharm Chemical Reagent Co., Ltd., Shanghai, China) for its quantification.
First, 2 ml of PBS (pH 8.0) or 4 μl of PGA were mixed with 0.1 g of magnetic α-Fe2O3/Fe3O4@SiO2-CHO nanocomposites immobilized with PGA. Five milliliters of 4% penicillin K solution (Aladdin, Shanghai, China) was added to each mixture and incubated for 5 min at room temperature After this time, the mixture was centrifuged, and 1 ml of the supernatant was added to 4 ml of deionized water to compose a 0.5 ml of mixture, and later combined with 3.5 ml of paradimethylaminobenzaldehyde. The UV-vis absorbance of this final solution was measured at 415 nm after an incubation time of 5 min.
For measuring the pH effects, 2 ml of PBS with various pH values (ranging from pH 6.0 to 9.0) was added to 4 μl of free PGA. Simultaneously, 0.1 g of α-Fe2O3/Fe3O4 nanosheets immobilized with PGA, was mixed with 2 ml of PBS at various pH values (6.0–9.0). Enzymatic activity was monitored based on the steps mentioned above.
To evaluate the thermostability of the composites, free and immobilized PGAs were mixed with the PBS solution with the pH value that resulted in higher enzymatic activity, and heated at various temperatures (ranging from 20°C to 60°C) for 5 min. Enzymatic activities were monitored based on the previous experimental steps.
Twenty mini centrifuge tubes were prepared, and 0.5 ml of free PGA and 2 ml of PBS were added to each tube. The tubes were divided into four groups and heated at 30°C, 40°C, 50°C, or 60°C. One sample from each group was heated during five different periods (2–10 h). Subsequently, 5 ml of 4% penicillin K solution at room temperature was added to each one of the 20 tubes. In another set of 20 mini centrifuge tubes, 0.1 g of nanosheets immobilized with PGA was added to 2 ml of PBS only. Enzymatic activity was monitored based on the previously mentioned experimental steps.
To study the enzymatic kinetics of immobilized PGA, various concentrations of penicillin K solution (0.01, 0.0125, 0.017, 0.025, and 0.05 mM) at 37°C were used to determine the initial hydrolysis rates of PGA, using the Lineweaver-Burk plot.
The immobilized PGA property was evaluated by adding 5 ml of 4% penicillin K solution to a mixture of 0.1 g of the nanomaterials immobilized with PGA plus 2 ml of PBS after 5 min. The UV-vis absorbance of the supernatant at 415 nm was measured after centrifugation. The procedures mentioned above were repeated after various rinsing steps with PBS, until the activity of the immobilized PGA was reduced to a certain extent.
The SEM morphology (Figure 2A) and TEM image (Figure 2B) of the prepared heterogeneous nanosheets calcined at 600°C for 4 h showed that the product morphology remained as nanosheets and did not change after calcination. It can be seen that the magnetic α-Fe2O3/Fe3O4 nanosheets exhibited slight aggregation due to the magnetism of the heterogeneous nanosheets. However, they are generally well dispersed. The average diameter and thickness, measured with the Nano Measurer, reached approximately 240 and 40 nm, respectively. The x-ray diffraction (XRD) pattern of the magnetic α-Fe2O3/Fe3O4 heterogeneous nanosheets is depicted in Figure 2C. The characteristic peaks at 24.1°, 33.1°, 35.6°, 40.8°, 49.4°, 54.0°, 62.4°, and 63.9° (Liu et al., 2020) were consistent with those of the standard card of Fe2O3 (JCPDS No. 33-0664), which represent 012, 104, 110, 113, 024, 116, 214, and 300 crystal faces, respectively, indicating the formation of hematite (Mehdizadeh et al., 2020). However, the ratio of the sample peak intensities at 33° and 35.7° was significantly lower than that of the standard Fe2O3 card. This phenomenon could be attributed to the existence of Fe3O4, as the peak at 35.7° exhibited a higher intensity. Based on the standard card of Fe3O4 (JCPDS No. 19-0629), the peak intensity of Fe3O4 at 35.7° was higher than the peak intensity at 33°. In contrast, Fe2O3 exhibited a higher peak intensity at 33° than at 35.7°. Hence, this phenomenon suggests the successful formation of Fe2O3/Fe3O4 heterogeneous nanomaterials. Figure 2D shows that the magnetic saturation strength of the prepared heterogeneous nanosheets was 25.1 emu/g, which was slower than the previously prepared Fe2O3/Fe3O4 nanoparticles and Fe2O3/Fe3O4 nanotubes. However, the magnetic saturation strength of the Fe2O3/Fe3O4 nanosheets was higher than that of the Fe2O3/Fe3O4 nanorods. Therefore, we could apply the external magnetic field method to attract immobilized materials for separation and reduce the aggregation of magnetic nanomaterials.
FIGURE 2. The scanning electron microscopy (SEM) morphology (A), transmission electron microscopy (TEM) image (B), x-ray diffraction (XRD) pattern (C), hysteresis loops (D) EDS spectrum (E), x-ray photoelectron spectroscopy (XPS) spectrum (F), the chart of pore diameter distributions (G), and N2 adsorption and desorption diagram (H) of magnetic α-Fe2O3/Fe3O4 heterogeneous nanosheets.
The element types and contents of the fabricated nanomaterials are shown in Figure 2E. The proportion of Fe to O was 0.47, according to the data in the figure, meaning that divalent iron and ferrous iron (Liu et al., 2020) might exist. The x-ray photoelectron spectroscopy (XPS) of the Fe2O3/Fe3O4 heterostructure nanosheets is shown in Figure 2F. As investigated in previous studies, the characteristic peaks at around 711 and 724 eV represent the Fe 2p1/2 and Fe 2p3/2 curves, the positions of which were relevant to the ionic states of Fe. The peak at approximately 719 eV corresponds to the satellite peak of Fe3+. With the increase in iron oxidation in the compound, the characteristic peaks all moved toward high binding energy. The Gauss–Lorenz method was adopted to fit the Fe 2p3/2 curve. Peaks of high binding energy at approximately 711 and 713 eV were attributed to Fe3+, while the peak at approximately 709 eV was attributed to Fe2+, which indicated the existence of Fe3O4. The BET spectra of magnetic α-Fe2O3/Fe3O4 heterogeneous nanosheets are displayed in Figures 2G,H. The average pore diameters were focused on 3–4 nm, and the specific surface area of the prepared heterostructure nanosheets was approximately 14.82 m2/g.
The SEM morphology of the magnetic α-Fe2O3/Fe3O4@SiO2 nanocomposites is shown in Figure 3A. The surface of the magnetic α-Fe2O3/Fe3O4@SiO2 nanocomposites was rough, which could be attributed to the SiO2 coating. The magnetic α-Fe2O3/Fe3O4@SiO2 nanocomposites also showed slight aggregation, proving the existence of magnetism in the prepared nanocomposites. The EDS spectrum of Fe2O3/Fe3O4@SiO2 is shown in Figure 3B, revealing that the elemental composition of the prepared products included Si, O, and Fe. As observed in Figures 3C,D, the specific surface area of the silica-decorated heterostructure nanosheets was approximately 12.65 m2/g. The size of the specific surface area was mostly related to the pore structure of the material. The pore diameter of the nanocomposites was approximately 3 nm. The specific surface area and mesoporous structure increased the possibility of enzymatic immobilization onto magnetic nanomaterials.
FIGURE 3. SEM morphology (A), EDS spectrum (B), the chart of pore diameter distributions (C), and N2 adsorption and desorption diagram (D) of α-Fe2O3/Fe3O4@SiO2 nanocomposites.
The automatic docking enzyme program (Zhang et al., 2020) was implemented to generate the docking between PGA and penicillin K, and the results are shown in Figure 4. The location of the active sites might influence the activity of the enzyme. This property enhanced the binding affinity of PGA to the substrate. The presence of Fe2O3 weakened the diffusion limitation caused by Fe3O4 (Esmaeili et al., 2011). However, these processes could not be performed using bare Fe2O3/Fe3O4 heterogeneous nanosheets. Therefore, it was necessary to create an appropriate interface for the active site. SiO2 can provide a large amount of silica hydroxyl groups, which can be used as a coating on the surface of heterogeneous nanosheets and immobilize the carrier of PGA (Pan et al., 2019; Chen et al., 2020). In addition, a suitable immobilization method was used. Glutaraldehyde (GA) is a powerful crosslinking agent that reacts with the free amino group in PGA and does not damage PGA after crosslinking with its active site (Liu et al., 2020). The kinetics model was established by molecular docking to study the mechanism of enzyme immobilization and find the best binding pattern between the two molecules, which was of great value for investigating the immobilization conditions.
FIGURE 4. Orientation confirmation of PGA (A), and molecular docking mode of penicillin K and PGA (B).
Overall, Figure 5A shows the Fourier Transform infrared spectroscopy (FTIR) of PGA, Fe2O3/Fe3O4 nanosheets, Fe2O3/Fe3O4@SiO2, α- Fe2O3/Fe3O4@SiO2-CHO, and Fe2O3/Fe3O4@SiO2-CH=N-PGA. Figure 5A (a) shows the absorption peaks of PGA. As illustrated in Figure 5A (b), the stretching vibration of the Fe–O bond from magnetic Fe2O3/Fe3O4 heterogeneous nanosheets was significant to the absorption peak at 550 cm−1. The peaks at around 781 and 836 cm−1 in Figure 5A (c) successfully evidenced the SiO2 coating of Fe2O3/Fe3O4 nanomaterials, due to the stretching vibration of the Si–O bond. Similar to Figure 5A (c), the stronger peak caused by the modification of GA appeared at approximately 596 cm−1 in Figure 5A (d). The enhanced absorption peak at 1,650 cm−1 in Figure 5A (e) corresponds to the characteristic peak of PGA, which is caused by the stretching vibration of the C=N bond. FTIR spectrum analysis showed that PGA was successfully immobilized onto the silica-decorated heterostructure nanosheets. Thenceforth, to obtain the optimum time for PGA immobilization, 2 ml of PBS was shaken with immobilized PGA for various periods (6–24 h) at a frequency of 115 rpm. After each incubation time, 5 ml of the 4% penicillin K was added to the PGA solution, followed by another 10 min of reaction. The enzymatic activity of the centrifuged supernatant is shown in Figure 5B, which shows that the activity trend first increased and then dropped with the extension of immobilization time. The enzymatic activity reached the highest level of 387.03 IU/g at 18 h. The reason for the decreased activity was that the immobilized PGA was inactivated with extended immobilization time. The influence of various concentrations on immobilized PGA is represented in Figure 5C. First, 2 ml of PBS was prepared with different amounts of free PGA (0.05, 0.1, 0.15, and 0.2 ml). The following experimental procedures for free PGA were similar to those of the immobilized PGA mentioned above. The activity initially exhibited an upward trend and then decreased. When the concentration was 0.58 g/L, the enzymatic activity reached the highest level. The increasing trend might be due to the magnetic nanomaterials that were not completely connected with the PGA. As the concentration increased, excess PGA was not covalently bonded with the nanomaterials but was absorbed on the nanomaterials, which resulted in a smaller specific surface area and masking of the active spots. The decreasing trend after 18 h was caused by less contact between the immobilized enzyme and its substrate.
FIGURE 5. The Fourier transform infrared (FTIR) spectra (A) of the PGA immobilization process and the influences of time (B) and concentration (C) on the activity of PGA.
The influence of pH and thermostability was investigated to monitor the highest activity of immobilized and free PGA. The results in Figure 6A show that the trend of the enzyme activity increased first and then dropped with the pH increment, reaching the highest level at pH 8.0. Therefore, the activities of free PGA at 20°C–60°C were determined at pH 8.0 after 10 min of reaction. As shown in Figure 6B, the activity increased first and then dropped dramatically with the increment in the temperature of the reaction, reaching the maximum activity at 45°C. As observed from the graph, free PGA activity varied more dramatically than the immobilized PGA, which suggested that the immobilized PGA exhibited greater thermal stability than free PGA. Above all, the nanomaterials improved the structure of PGA, preventing conformational changes and degeneration in extreme environments. The thermostability was analyzed based on previous experiments, as shown in Figures 6C,D, respectively. The immobilized and free PGAs were cultured in PBS (pH 8.0) at 30°C, 40°C, 50°C, and 60°C for different intervals (2–10 h). When the heating time and the reaction temperature increased, the relative activity of both immobilized and free PGA decreased to a certain degree. As observed clearly in the figure, the activity dropped intensely at 50°C and 60°C. This phenomenon may be attributed to the destruction of the activity center. Immobilized PGA exhibited higher activity than free PGA at 30°C and 40°C. This could be because the immobilized carrier decreased the conformational change of the active center of the PGA. The conclusion drawn from these analyses reveals that PGA immobilized onto magnetic Fe2O3/Fe3O4 heterogeneous nanomaterials may be conducive to the application of enzymes.
FIGURE 6. The pH value (A), temperatures (B), and thermostabilities on free (C) and immobilized PGA (D), the Lineweaver-Burk diagram (E) and the reproductivity of immobilized PGA (F).
Different concentrations of penicillin K acting as the substrate reacted with free and immobilized PGA during different periods. The corresponding initial velocities were measured by linear regression analysis after measuring the amount of 6-aminopenicillanic acid (6-APA).
The Lineweaver-Burk diagram was constructed by linear regression analysis, as shown in Figure 6E. The Km value (0.108 mM) of the immobilized PGA was approximately four times the Km value (0.027 mM) of free PGA. This could be attributed to the formation of a diffusion layer around the immobilized PGA, which had a stereoscopic effect on the substrate. Therefore, this may reduce the binding of the PGA active site with its substrate and lessen the affinity between the immobilized PGA and the substrate.
The regenerative performance of PGA can determine its practical value in industrial applications. The reusability of immobilized PGA is clearly shown in Figure 6F, and the percentage of residual activity was determined using the initial activity as the control (100%). As revealed in the picture, the activity of immobilized PGA onto nanomaterials dropped with its reuse, which was associated with the loss of some parts of the enzyme in the carrier separation. However, the activity remained around 66% after multiple cycles, which suggested that the immobilized PGA exhibited good reusability (Sheldon and Van Pelt, 2013).
Magnetic α-Fe2O3/Fe3O4 heterogeneous nanosheets were fabricated via a hydrothermal calcination process (Liu et al., 2020; Hong et al., 2021). The magnetic saturation strength of the prepared nanosheets reached a maximum of 25.1 emu/g after calcination for 4 h. The average diameter and thickness of the magnetic nanosheets were approximately 240 and 40 nm, respectively. PGA was immobilized onto the modified carrier by covalent crosslinking, which effectively improved the catalytic performance of PGA. In contrast to free PGA, immobilized PGA exhibited better stability and reusability, with catalytic activity remaining at 66% of the initial activity after 12 cycles. The present study suggests that immobilizing PGA onto magnetic α-Fe2O3/Fe3O4 heterogeneous nanosheets can play a critical role in catalytic applications.
The original contributions presented in the study are included in the article/Supplementary Material, further inquiries can be directed to the corresponding authors.
ZL conceptualized the study. DH and RL formulated the methodology. YN curated the data and performed the investigation. ZW was in charge of the software and visualization. SK performed the formal analysis. ZL acquired the funding and supervised the study. YN, ZW, SK, and DH wrote the original draft. RL reviewed and edited the manuscript, performed the validation, and was in charge of project administration and resources.
This work was supported by the Jiangsu Provincial Postgraduate Scientific Practice and Innovation Project (Grant No. SJCX21_1722) and the Science and Technology Innovation Project of CHN Energy (Grant No. GJNY-20-109).
The authors declare that the research was conducted in the absence of any commercial or financial relationships that could be construed as a potential conflict of interest.
All claims expressed in this article are solely those of the authors and do not necessarily represent those of their affiliated organizations, or those of the publisher, the editors, and the reviewers. Any product that may be evaluated in this article, or claim that may be made by its manufacturer, is not guaranteed or endorsed by the publisher.
Alizadeh, N., and Salimi, A. (2021). Multienzymes Activity of Metals and Metal Oxide Nanomaterials: Applications from Biotechnology to Medicine and Environmental Engineering. J. Nanobiotechnol. 19, 26. doi:10.1186/s12951-021-00771-1
Angelakeris, M. (2017). Magnetic Nanoparticles: A Multifunctional Vehicle for Modern Theranostics. Biochim. Biophys. Acta (Bba) - Gen. Subjects 1861, 1642–1651. doi:10.1016/j.bbagen.2017.02.022
Ansari, S. A., and Husain, Q. (2012). Potential Applications of Enzymes Immobilized On/in Nano Materials: A Review. Biotechnol. Adv. 30, 512–523. doi:10.1016/j.biotechadv.2011.09.005
Chen, J., Huang, W., Pan, S., and Liu, R. (2020). A Facile Urea-Hydrolysis Calcination Process for the Preparation of α-Fe2O3 Nanoparticles and α-Fe2O3 Nanorods and Their Fabrication Mechanisms. AIP Adv. 10, 025028. doi:10.1063/1.5143329
Chen, X., Yang, L., Zhan, W., Wang, L., Guo, Y., Wang, Y., et al. (2018). Immobilization of Penicillin G Acylase on Paramagnetic Polymer Microspheres with Epoxy Groups. Chin. J. Catal. 39, 47–53. doi:10.1016/S1872-2067(17)62934-6
Dalkıran, B., Erden, P. E., Kaçar, C., and Kılıç, E. (2019). Disposable Amperometric Biosensor Based on Poly‐L‐lysine and Fe 3 O 4 NPs‐chitosan Composite for the Detection of Tyramine in Cheese. Electroanalysis 31, 1324–1333. doi:10.1002/elan.201900092
Esmaeili, E., Salavati-Niasari, M., Mohandes, F., Davar, F., and Seyghalkar, H. (2011). Modified Single-phase Hematite Nanoparticles via a Facile Approach for Large-Scale Synthesis. Chem. Eng. J. 170, 278–285. doi:10.1016/j.cej.2011.03.010
Farid, S., Zhao, J., and Li, S.-P. (2020). Recent Development in the Application of Immobilized Oxidative Enzymes for Bioremediation of Hazardous Micropollutants - A Review. Chemosphere 239, 124716. doi:10.1016/j.chemosphere.2019.124716
Fernandez-Lorente, G., Rocha-Martín, J., and Guisan, J. M. (2020). Immobilization of Lipases by Adsorption on Hydrophobic Supports: Modulation of Enzyme Properties in Biotransformations in Anhydrous Media. Methods Mol. Biol. 2100, 143–158. doi:10.1007/978-1-0716-0215-7_9
Hong, J., Yang, F., and Sun, Z. (2021). Hexagonal Bi-pyramid α-Fe2O3 Microcrystals: Unusual Formation, Characterization and Application for Gas Sensing. J. Alloys Compounds 889, 161515. doi:10.1016/j.jallcom.2021.161515
Li, Y., Liu, J., Fu, Y., Xie, Q., and Li, Y. (2019). Correction to: Magnetic-Core@dual-Functional-Shell Nanocomposites with Peroxidase Mimicking Properties for Use in Colorimetric and Electrochemical Sensing of Hydrogen Peroxide. Microchim. Acta 186, 1–9. doi:10.1007/s00604-019-3366-0
Liu, D.-M., Chen, J., and Shi, Y.-P. (2018). Advances on Methods and Easy Separated Support Materials for Enzymes Immobilization. Trac Trends Anal. Chem. 102, 332–342. doi:10.1016/j.trac.2018.03.011
Liu, H., Lu, J., Zhao, X., and Xu, T. (2021a). Ionic Liquids Immobilized on Nanomaterials: An Efficient Strategy in Catalytic Reactions. Synth. Commun. 51, 2265–2286. doi:10.1080/00397911.2021.1936057
Liu, L., Chen, L., Liang, J., Liu, L., and Han, H. (2016). A Novel Ratiometric Probe Based on Nitrogen-Doped Carbon Dots and Rhodamine B Isothiocyanate for Detection of Fe3+in Aqueous Solution. J. Anal. Methods Chem. 2016, 1–7. doi:10.1155/2016/4939582
Liu, R., Huang, W., Pan, S., Li, Y., Yu, L., and He, D. (2020). Covalent Immobilization and Characterization of Penicillin G Acylase on Magnetic Fe2O3/Fe3O4 Heterostructure Nanoparticles Prepared via a Novel Solution Combustion and Gel Calcination Process. Int. J. Biol. Macromolecules 162, 1587–1596. doi:10.1016/j.ijbiomac.2020.07.283
Liu, W., Luo, J., Zhuang, X., Shen, W., Zhang, Y., Li, S., et al. (2014). Efficient Preparation of Enantiopure L-Tert-Leucine through Immobilized Penicillin G Acylase Catalyzed Kinetic Resolution in Aqueous Medium. Biochem. Eng. J. 83, 116–120. doi:10.1016/j.enzmictec.2014.03.01110.1016/j.bej.2013.12.016
Liu, Z., Cheng, Q., Wang, Y., Li, K., Wang, R., and Zhang, J. (2021b). The Interaction of Nanoparticulate Fe3O4 during the Diffusion-Limited Aggregation Process: A Molecular Dynamics Simulation. Powder Technology 384, 141–147. doi:10.1016/j.powtec.2020.09.075
Mehdizadeh, P., Orooji, Y., Amiri, O., Salavati-Niasari, M., and Moayedi, H. (2020). Green Synthesis Using Cherry and orange Juice and Characterization of TbFeO3 Ceramic Nanostructures and Their Application as Photocatalysts under UV Light for Removal of Organic Dyes in Water. J. Clean. Prod. 252, 119765. doi:10.1016/j.jclepro.2019.119765
Nadar, S. S., Vaidya, L., and Rathod, V. K. (2020). Enzyme Embedded Metal Organic Framework (Enzyme-MOF): De Novo Approaches for Immobilization. Int. J. Biol. Macromolecules 149, 861–876. doi:10.1016/j.ijbiomac.2020.01.240
Pakapongpan, S., and Poo-arporn, R. P. (2017). Self-assembly of Glucose Oxidase on Reduced Graphene Oxide-Magnetic Nanoparticles Nanocomposite-Based Direct Electrochemistry for Reagentless Glucose Biosensor. Mater. Sci. Eng. C 76, 398–405. doi:10.1016/j.msec.2017.03.031
Pan, S., Huang, W., Li, Y., Yu, L., and Liu, R. (2020). A Facile Diethyl-Carbonate-Assisted Combustion Process for the Preparation of the Novel Magnetic α-Fe2O3/Fe3O4 Heterostructure Nanoparticles. Mater. Lett. 262, 127071. doi:10.1016/j.matlet.2019.127071
Rajesh, A., Zimmerman, D., Spivack, K., Abdulmalik, O., and Elmer, J. (2018). Glutaraldehyde Cross-Linking Increases the Stability ofLumbricus Terrestriserythrocruorin. Biotechnol. Prog. 34, 521–528. doi:10.1002/btpr.2593
Senapati, S., Mahanta, A. K., Kumar, S., and Maiti, P. (2018). Controlled Drug Delivery Vehicles for Cancer Treatment and Their Performance. Sig. Transduct. Target. Ther. 3, 7. doi:10.1038/s41392-017-0004-3
Sheldon, R. A., and Van Pelt, S. (2013). Enzyme Immobilisation in Biocatalysis: Why, what and How. Chem. Soc. Rev. 42, 6223–6235. doi:10.1039/c3cs60075k
Sirisha, V. L., Jain, A., and Jain, A. (2016). Enzyme Immobilization. Adv. Nutr. Res. 79, 179–211. doi:10.1016/bs.afnr.2016.07.004
Tanabe, T., Shin, M., and Fujiwara, K. (2004). Immunoelectron Microscopy Study of Polyamines Using a Newly Prepared Monoclonal Antibody against Spermidine: Use of a Mixture of Glutaraldehyde and Paraformaldehyde as a Cross-Linking Agent in the Preparation of the Antigen. J. Biochem. 135, 501–507. doi:10.1093/jb/mvh066
Wang, X., Chen, Z., Li, K., Wei, X., Chen, Z., Ruso, J. M., et al. (2019). The Study of Titanium Dioxide Modification by Glutaraldehyde and its Application of Immobilized Penicillin Acylase. Colloids Surf. A: Physicochemical Eng. Aspects 560, 298–305. doi:10.1016/j.colsurfa.2018.10.001
Wang, Y., Zhao, Y., Ding, X., and Qiao, L. (2021). Recent Advances in the Electrochemistry of Layered post-transition Metal Chalcogenide Nanomaterials for Hydrogen Evolution Reaction. J. Energ. Chem. 60, 451–479. doi:10.1016/j.jechem.2021.01.0212095-4956/
Xue, P., Gu, Y., Su, W., Shuai, H., and Wang, J. (2016). In Situ one-pot Preparation of Superparamagnetic Hydrophilic Porous Microspheres for Covalently Immobilizing Penicillin G Acylase to Synthesize Amoxicillin. Appl. Surf. Sci. 362, 427–433. doi:10.1016/j.apsusc.2015.11.209
Xue, P., Su, W., Gu, Y., Liu, H., and Wang, J. (2015). Hydrophilic Porous Magnetic poly(GMA-MBAA-NVP) Composite Microspheres Containing Oxirane Groups: An Efficient Carrier for Immobilizing Penicillin G Acylase. J. Magnetism Magn. Mater. 378, 306–312. doi:10.1016/j.jmmm.2014.11.048
Yang, L., Gao, Z., Guo, Y., Zhan, W., Guo, Y., Wang, Y., et al. (2014). Immobilization of Penicillin G Acylase on Paramagnetic Aldehyde-Functionalized Mesostructured Cellular Foams. Enzyme Microb. Technology 60, 32–39. doi:10.1016/j.enzmictec.2014.03.011
Yu, Q., Wang, Z., Zhang, Y., and Liu, R. (2019). Covalent Immobilization and Characterization of Penicillin G Acylase on Amino and GO Functionalized Magnetic Ni0.5Zn0.5Fe2O4@SiO2 Nanocomposite Prepared via a Novel Rapid-Combustion Process. Int. J. Biol. Macromolecules 134, 507–515. doi:10.1016/j.ijbiomac.2019.05.066
Zhang, J., Chen, L., Zhu, Y., and Zhang, Y. (2020). Study on the Molecular Interactions of Hydroxylated Polycyclic Aromatic Hydrocarbons with Catalase Using Multi-Spectral Methods Combined with Molecular Docking. Food Chem. 309, 125743. doi:10.1016/j.foodchem.2019.125743
Zhou, Z., and Hartmann, M. (2013). Progress in Enzyme Immobilization in Ordered Mesoporous Materials and Related Applications. Chem. Soc. Rev. 42, 3894–3912. doi:10.1016/bs.afnr.2016.07.00410.1039/c3cs60059a
Keywords: magnetic α-Fe2O3/Fe3O4 heterogeneous nanosheets, immobilization, penicillin G acylase, hydrothermal calcination process, reusability
Citation: Ni Y, Lv Z, Wang Z, Kang S, He D and Liu R (2022) Immobilization and Evaluation of Penicillin G Acylase on Hydroxy and Aldehyde Functionalized Magnetic α-Fe2O3/Fe3O4 Heterostructure Nanosheets. Front. Bioeng. Biotechnol. 9:812403. doi: 10.3389/fbioe.2021.812403
Received: 10 November 2021; Accepted: 10 December 2021;
Published: 28 January 2022.
Edited by:
Jung-Kul Lee, Konkuk University, South KoreaReviewed by:
Abdelmageed M. Othman, National Research Centre, EgyptCopyright © 2022 Ni, Lv, Wang, Kang, He and Liu. This is an open-access article distributed under the terms of the Creative Commons Attribution License (CC BY). The use, distribution or reproduction in other forums is permitted, provided the original author(s) and the copyright owner(s) are credited and that the original publication in this journal is cited, in accordance with accepted academic practice. No use, distribution or reproduction is permitted which does not comply with these terms.
*Correspondence: Dawei He, aGVAMTYzLmNvbQ==; Ruijiang Liu, bHVja3lzdGFyX2xyakB1anMuZWR1LmNu
†These authors have contributed equally to this work and share first authorship
‡These authors have contributed equally to this work and share last authorship
Disclaimer: All claims expressed in this article are solely those of the authors and do not necessarily represent those of their affiliated organizations, or those of the publisher, the editors and the reviewers. Any product that may be evaluated in this article or claim that may be made by its manufacturer is not guaranteed or endorsed by the publisher.
Research integrity at Frontiers
Learn more about the work of our research integrity team to safeguard the quality of each article we publish.