- 1Institute of Orthopaedic Research and Biomechanics, Trauma Research Centre, Ulm University, Ulm, Germany
- 2Instituto de Investigação e Inovação Em Saúde (i3S), Universidade Do Porto, Porto, Portugal
- 3Instituto de Engenharia Biomédica (INEB), Universidade Do Porto, Porto, Portugal
- 4Instituto de Ciências Biomédicas Abel Salazar (ICBAS), Universidade do Porto, Porto, Portugal
Mesenchymal stem/stromal cell (MSC)–based therapies for low back pain and intervertebral disc (IVD) degeneration have been emerging, despite the poor knowledge of their full mechanism of action. As failure of the annulus fibrosus (AF) is often associated with IVD herniation and inflammation, the objective of the present study was to investigate the impact of the MSC secretome on human AF cells exposed to mechanical loading and a pro-inflammatory environment. Human AF cells isolated from IVD biopsies from patients with adolescent idiopathic scoliosis (AIS) or disc degeneration (DD) were exposed to physiological cyclic tensile strain (CTS) for 72 h in a custom-made device, with or without interleukin (IL)-1β medium supplementation. AF cells stimulated with CTS + IL-1β were then treated with secretome from IL-1β–preconditioned MSCs for 48 h. AF cell metabolic activity, gene expression, protein secretion, matrix metalloproteinase (MMP) activity, and tissue inhibitor of MMPs (TIMP) concentration were evaluated. Expanded AF cells from AIS and DD patients revealed similar metabolic activity and gene expression profiles. CTS stimulation upregulated collagen type I (COL1A1) expression, while IL-1β significantly stimulated IL-6, IL-8, MMP-1, and MMP-3 gene expression and prostaglandin E2 production by AF cells but downregulated COL1A1. The combination of CTS + IL-1β had a similar outcome as IL-1β alone, accompanied by a significant upregulation of elastin. The MSC secretome did not show any immunomodulatory effect on CTS + IL-1β–stimulated AF cells but significantly decreased MMP-1, MMP-2, MMP-3, and MMP-9, while increasing the production of TIMP-1. The obtained results demonstrate a stronger impact of the inflammatory milieu on human AF cells than upper physiologic mechanical stress. In addition, a new MSC mechanism of action in degenerated IVD consisting of the modulation of AF MMP activity was also evidenced, contributing to the advancement of knowledge in AF tissue metabolism.
Introduction
Low back pain (LBP) is one of the top 10 disorders in the number of years lived with disability for adolescents and young adults (from 10 to 49 years old), affecting 70–85% of the world population and compromising healthy aging (Vos et al., 2020). LBP can be considered a multifactorial disorder but has been strongly associated with degeneration of the intervertebral disc (IVD). Current therapies include physical therapy or anti-inflammatory drugs, and if LBP does not improve, invasive surgery such as discectomy, spinal fusion, or IVD prosthesis can be used (Maher et al., 2017). Such treatments may alleviate LBP symptoms but reduce patients’ mobility, might be temporary, and, overall, do not target the underlying problem.
The outer ring of the IVD, the annulus fibrosus (AF), consists of concentric collagen fibers interconnected by a network of elastin and fibrillin, interrupted at distinct locations by a zone that links the different lamellae, known as the translamellar bridging network (TBLN). AF failure is often associated with structural weakness in restraining the hydrated inner structure, the nucleus pulposus (NP). Factors that induce AF failure include mechanical stress, which has been previously shown to increase pro-inflammatory gene expression in human AF cells (Pratsinis et al., 2016). Mechanical loading appears to act synergistically with a pro-inflammatory environment, weakening the AF through TBLN deregulation (Saggese et al., 2019). But moderate mechanical stress has also shown to rescue pro-inflammatory gene expression of AF cells exposed to a pro-inflammatory stimulus (Zhang et al., 2021). Overall, the pathomechanism of AF mechanical weakening and consequent disc herniation remains to be fully understood.
IVD herniation involves alterations in the AF but also in the NP, including loss of proteoglycans and water content, upregulation of matrix metalloproteinases (MMP-1, -2, -3, -9, among others) and inflammatory mediators (tissue necrosis factor (TNF)-α, interleukin (IL)-1β, etc) that contribute to the weakening and mechanical failure of the disc tissue (Risbud and Shapiro, 2014). More recently, the complement activation product terminal complement complex (TCC), an inflammatory trigger, has also been suggested to be involved in the disease progression (Teixeira et al., 2021a). Unbalanced production of MMPs weakens the IVD extracellular matrix (ECM), which can lead to NP extrusion through the AF, ultimately leading to lumbar disc herniation (LDH). LDH accounts only for 5–15% of LBP cases but can induce sciatica symptoms and loss of bladder/bowel control and is the main cause of spine surgery (Weinstein et al., 2008). Moreover, about 18% of patients present signs of IVD re-herniation after surgery (Shin et al., 2019). Early treatment to reinforce AF in LDH would be an important strategy to prevent disease progression.
Cell-based therapies are emerging to treat LBP and IVD degeneration. In particular, mesenchymal stem/stromal cell (MSC)–based therapies have been shown to promote ECM synthesis in NP and increase disc height in small animal models (Sakai et al., 2006). MSC intravenous administration has also shown to reduce IVD herniation in a rat model of punctured IVD (Cunha et al., 2017). In the clinical trials conducted so far, MSC transplantation appears to be safe and patients reported less pain, but no significant alterations in the IVD were observed (Orozco et al., 2011; Noriega et al., 2017). This discrepancy between animals and humans might be due to the fact that the former lacks the biped position of the human spine and retain a large population of notochordal cells, thus a higher regenerative capacity. In a pro-inflammatory environment characteristic of the degenerated IVD, we have demonstrated that MSCs have been shown to have immunomodulatory features, and we hypothesize that this may occur via a paracrine mechanism (Teixeira et al., 2018). Recently, we have shown that MSC secretome, obtained upon IL-1β preconditioning, also reduces pro-inflammatory markers in both NP and AF organ cultures (Ferreira et al., 2021; Neidlinger-Wilke et al., 2021). Nevertheless, as these studies were conducted in a bovine model, the direct clinical translation may have some restraints. In fact, MSCs and their secretome can be highly influenced by the cells’ microenvironment, as reviewed by Ferreira et al. (2018). The degenerated IVD is a harsh biochemical and biomechanical condition, with acidic pH, high concentration of pro-inflammatory factors, and matrix-degrading enzymes, under high and complex mechanical loading. This environment is a challenge for the successful translation of cell therapies, including those with MSCs. Additionally, more complex in vitro studies are needed which take the multifaceted IVD environment and the interaction of the different influencing factors into account. In this study, we hypothesized that 1) a synergistic action between mechanical loading and a pro-inflammatory microenvironment can deregulate human AF cell phenotypes, and 2) the MSC secretome has an immunomodulatory impact on human AF cell metabolism.
Materials and Methods
MSC Expansion and Secretome Production
Human MSCs (Lonza, n = 3, donor information is summarized in Supplementary Table S1) were seeded (3,000 cells/cm2) and routinely expanded in MSC medium composed of low-glucose Dulbecco’s modified Eagle medium (DMEM, Gibco) supplemented with 10% fetal bovine serum (FBS, HyClone), 1% penicillin/streptomycin (10,000 U/mL—10 mg/ml, Gibco), and 0.5% amphotericin B (250 μg/ml, Gibco), at 37°C, 21% oxygen and humidified atmosphere. The medium was exchanged twice a week, and cells were trypsinized when reaching 70% confluency. For secretome production, 1x106 MSCs (passage 5–9) were seeded in 6-well plates (Corning) and incubated in 5 ml of MSC medium supplemented with recombinant human IL-1β (10 ng/ml, R&D Systems), at 37°C in a humidified atmosphere with 6% O2 and 8.5% CO2 for 48 h, as previously optimized for MSC preconditioning (Ferreira et al., 2021). MSC secretome (MSCsec) was collected by centrifugation at 1800 g for 5 min, at 4°C, to remove cell debris and then stored at −80°C until further use.
Human AF Cell Isolation and Culture
Human AF cells were isolated from a total of 12 patients undergoing surgery in the lumbar region: six samples from patients with adolescent idiopathic scoliosis (AIS, age: 16 ± 2 years old, 4 males/2 females), and six samples from patients with disc degeneration (DD, age: 65 ± 11 years old, 5 males/1 female, Pfirrmann grades IV-V). Detailed patient information is summarized in Supplementary Table S2. Open surgery was performed to remove the disc tissue. The AF tissue was macroscopically separated from the cartilaginous endplate and NP, as previously described (Teixeira et al., 2021a; Teixeira et al., 2021b). Visibly fibrous tissue with a clearly identifiable lamellar structure was selected for AF cell isolation. AF cells were obtained upon enzymatic digestion of the tissue for 3–5 h in 0.8 mg/ml collagenase type II (Sigma-Aldrich) in IVD medium without FBS: low-glucose DMEM (Gibco) supplemented with 1% non-essential amino acids (Biochrom), 1% penicillin/streptomycin, 5% amphotericin B, and 1.5% 5M NaCl/0.4 M KCl solution (to adjust osmolarity to 400 mOsm), under agitation, reduced oxygen supply (6% O2 and 8.5% CO2), 37°C, and saturated humidity. Cells were then expanded under sterile conditions in IVD medium containing 5% FBS Superior (Biochrom), as previously described (Teixeira et al., 2016).
Stimulation of AF Cells With CTS and IL-1β and Treatment With MSC Secretome
Human AF cells (passages 3 to 4) were seeded in silicone dishes (300,000 cells/dish) coated with fibronectin (10 μg/ml) and placed in a custom-made cyclic tensile strain (CTS) device (Neidlinger-Wilke et al., 1994), previously modified as described (Pratsinis et al., 2016). After 3 days, the medium was exchanged, and the AF cells were stimulated for 48 h as follows: 1) 2% CTS at 1 Hz was applied 3 h/day to the silicone dishes, similar to what was previously optimized (Wuertz et al., 2007); 2) IL-1β (10 ng/ml), as previously seen to induce a pro-inflammatory response in bovine IVD organ culture (Teixeira et al., 2016); and 3) CTS (1 Hz, 3 h/day) + IL-1β (10 ng/ml). Unstimulated AF cells were kept as a control group. The experimental timeline is depicted in Figure 1A.
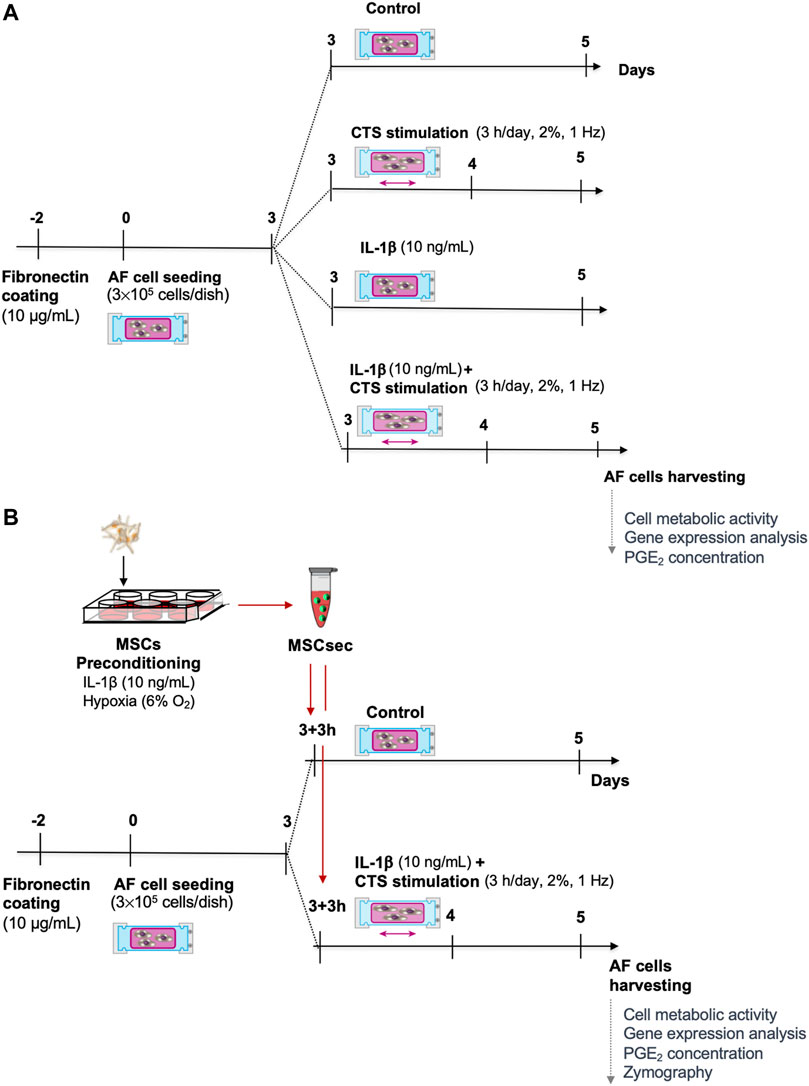
FIGURE 1. Experimental timeline. (A) Human annulus fibrosus (AF) cells were cultured in a custom-made electromechanical device for the application of cyclic tensile strain (CTS) to deformable silicone dishes, with IL-1β (10 ng/ml) or with CTS + IL-1β. (B) The CTS + IL-1β–stimulated AF cells were treated with secretome from human bone marrow–derived mesenchymal stem/stromal cells (MSC) upon preconditioning with IL-1β for 48 h.
In a subsequent set of experiments, the MSC secretome was added to AF cells stimulated with CTS + IL-1β, 3 h after stretching, in a 1:1 ratio with IVD medium. The MSC secretome addition to unstimulated AF cells was used as a control. The experimental timeline is represented in Figure 1B.
After 48 h, AF cells and conditioned medium were collected for different analyses. Conditioned medium was removed and frozen at −80°C for posterior protein analysis by ELISAs and zymography. AF cell metabolic viability was evaluated by resazurin assay, after which cells were immediately shock frozen in RNAlater ICE (Invitrogen) and liquid nitrogen and stored at −80°C for RNA isolation.
Metabolic Activity of AF Cells
The metabolic activity of AF cells was assessed by resazurin reduction assay. AF cells were incubated with 0.02 mg/ml resazurin sodium salt (Sigma-Aldrich) solution in IVD medium for 3 h at 37°C. Fluorescence intensity was measured in a spectrophotometer microplate reader (Tecan), with 530-nm excitation and 590-nm emission filters.
Gene Expression Analysis of AF Cells
AF cells frozen in RNAlater ICE were thawed, the RNAlater was removed, and RNA was isolated using an RNeasy Mini kit (Qiagen). RNA concentration and quality were determined by spectrophotometry (Spark, Tecan). For cDNA synthesis with integrated removal of DNA contamination, 1 µg of RNA was treated with a QuantiTect Reverse Transcription kit (ThermoFisher Scientific). Gene expression analysis was performed with primers for the reference gene human glyceraldehyde 3-phosphate dehydrogenase (GAPDH), as well as for the target genes in Table 1. The transcribed cDNA was mixed with custom-designed primers and the Platinum SYBR Green qPCR SuperMix-UDG (Invitrogen) and ROX Reference Dye (Invitrogen). The runs were performed in a QuantStudio 3 real-time PCR system (Applied Biosystems). The melt curves were analyzed to confirm the specificity of the reaction and a quantification cycle (Cq) 35 cutoff was used. Relative expression levels were calculated by using the Livak method (using the 2−ΔΔCt), where ΔΔCt = ΔCt(sample of interest)—ΔCt(control sample) and ΔCt = Ct(gene of interest)—Ct(GAPDH) (Livak and Schmittgen, 2001).
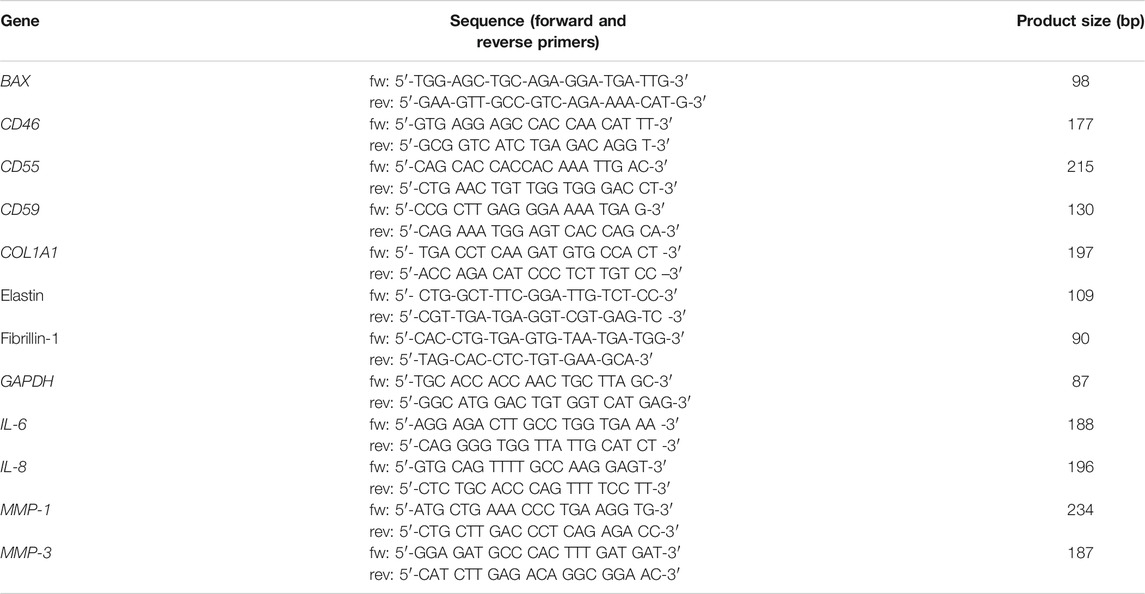
TABLE 1. Human oligonucleotide primers used for qRT-PCR. Primers with shown sequence were custom designed. fw: forward; rev: reverse.
Quantification of PGE2 and TIMPs in AF Culture Supernatants
The concentration of prostaglandin E2 (PGE2, Arbor Assays), human TIMP-1, TIMP-2, TIMP-3, and TIMP-4 (RayBiotech) was measured by using enzyme-linked immunosorbent assay (ELISA) kits according to the manufacturers’ instructions in AF supernatants, after 5 days of culture.
Zymography
The MMP activity of AF cells was investigated by analyzing the conditioned medium through gelatin zymography, as previously described (Cardoso et al., 2014). Briefly, the total protein content in the conditioned medium was determined by bicinchoninic acid assay (Bio-Rad), and 15–20 mg of protein was mixed with sample buffer (10% sodium dodecyl sulfate, 4% sucrose, and 0.03% bromophenol blue in 0.5 M Tris–HCl, pH 6.8) and separated on 10% polyacrylamide gels containing 0.1% gelatin (Sigma-Aldrich) as substrate. After electrophoresis, gels were washed twice with 2% Triton X-100. Gelatin gels were subsequently incubated for 16 h at 37°C in 50 mM Tris–HCl, pH 7.5, and 10 mM CaCl2. Gels were stained with 0.1% Coomassie Brilliant Blue R-250 (Sigma-Aldrich), 50% methanol, and 10% acetic acid (Merck). The molecular weight and activity of the MMPs were estimated by densitometric analysis.
Statistical Analysis
Results are presented as box plots with the median ± interquartile range. Statistical analysis was performed using GraphPad Prism 9 software (GraphPad Software, Inc, La Jolla, CA, United States). Normal distribution data were tested using the D’Agostino–Pearson omnibus normality test. A non-parametric unpaired Mann–Whitney test was used to compare AIS and DD samples. A non-parametric paired Friedman test, followed by Dunn’s multiple comparison test, was used to compare AF cell stimuli and the effect of the MSC secretome. Significance was set at p < 0.05.
Results
Viability and Inflammatory Profile of Human AF Cells Exposed to Mechanical and Inflammatory Stimuli
To evaluate the effect of mechanical loading and inflammatory stimuli on human AF cells’ inflammatory profile, they were cultured in silicone dishes and stimulated with 1) CTS (2%, 1 Hz, 3 h/day), 2) IL-1β (10 ng/ml), or 3) CTS + IL-1β. Unstimulated AF cells were used as controls (Figure 1A). AF cells from both AIS and DD patients were used indiscriminately. We first screened AF cells from different patients for metabolic activity and gene expression profiles regarding apoptosis (BAX), cellular complement regulators (CD46, CD55, CD59), inflammatory markers (IL-6, IL-8), matrix-degrading enzymes (MMP-1, MMP-3), and ECM proteins (COL1A1, elastin, fibrillin-1). No differences were obtained between the different genes in AF cells from the distinct patient groups (Supplementary Figure S1).
To investigate whether AF cell viability was affected by the CTS or IL-1β, mitochondrial metabolic activity and gene expression of BAX, an apoptosis regulator member of the BCL-2 gene family, were analyzed (Figures 2A,B). No differences were observed between the mitochondrial metabolic activity of the different groups. Moreover, no significant differences were observed in the gene expression of BAX when comparing CTS, IL-1β and CTS-IL-1β to the unstimulated control group. However, when comparing CTS + IL-1β with the single stimuli CTS and IL-1β, a significant downregulation was found in comparison to CTS (*p < 0.05).
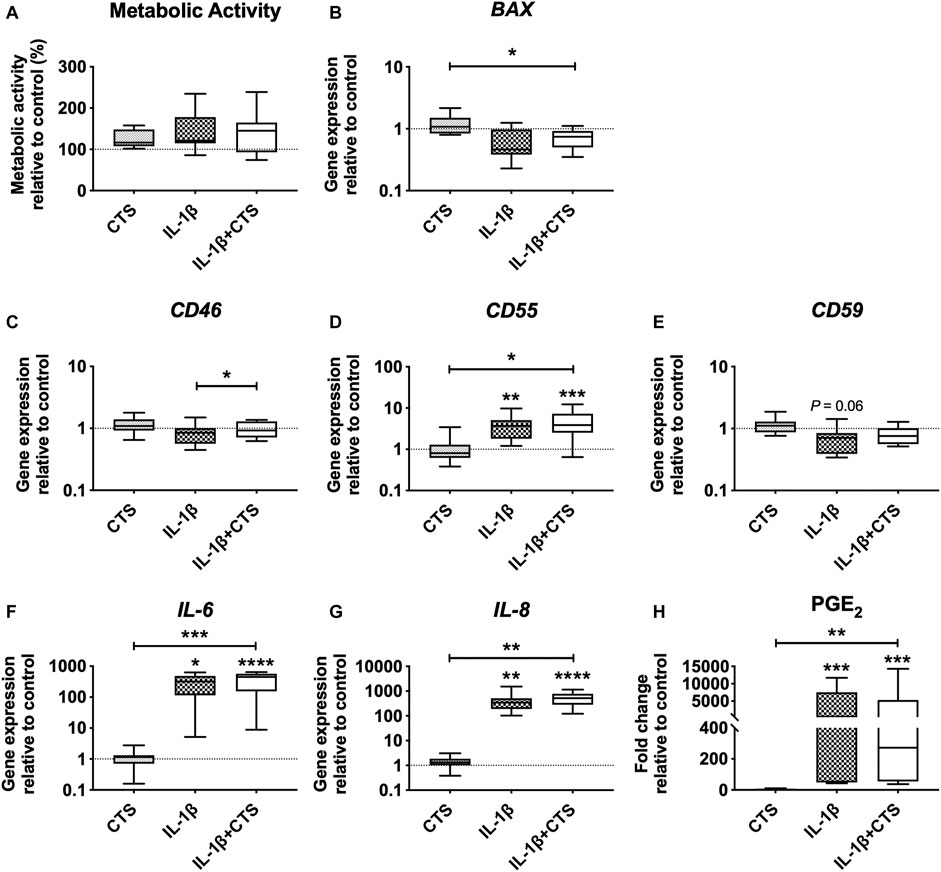
FIGURE 2. Human annulus fibrosus (AF) cells metabolic activity and gene expression of cell apoptosis marker, complement factors, and pro-inflammatory cytokines, in response to cyclic tensile strain (CTS) and the pro-inflammatory cytokine IL-1β (10 ng/ml) after 48 h. (A) AF cells mitochondrial metabolic activity (compared to unstimulated cells). Relative mRNA expression of (B) cell apoptosis marker BAX, (C) complement factors CD46, (D) CD55, and (E) CD59; pro-inflammatory markers (F) IL-6 and (G) IL-8. Results were normalized to expression level of GAPDH and unstimulated cells. (H) Concentration of pro-inflammatory marker PGE2 in (fold change to unstimulated cells). n = 10, *p < 0.05, **p < 0.01.
Moreover, CTS + IL-1β stimulation of AF cells significantly increased the expression of the inhibitory complement receptor CD46, compared with the IL-1β–stimulated group (*p < 0.05), and of the inhibitor CD55, compared with the CTS group (*p < 0.05) and unstimulated cells (***p < 0.001; Figures 2C,D). Interestingly, IL-1β by itself also promoted a significant upregulation of CD55 (**, p < 0.01) and a decrease in CD59, the major TCC inhibitor (p = 0.06), compared with control cells (Figures 2D,E).
Regarding the expression of inflammatory markers, as expected, IL-1β stimulation induced the upregulation of IL-6 and IL-8 gene expression and PGE2 secretion by AF cells (*p < 0.05, **p < 0.01 and ***p < 0.001, respectively) in comparison to unstimulated cells (Figures 2F–H). In contrast, CTS stimulation by itself was not shown to affect the production of these markers. The synergic action of mechanical and inflammatory stimuli (CTS + IL-1β) significantly upregulated IL-6 and IL-8 expression, compared with both unstimulated (****p < 0.0001) and CTS-stimulated cells (***p < 0.001 for IL-6 and **p < 0.01 for IL-8), and increased PGE2 production compared with control (***p < 0.001) and with CTS-stimulated cells (**p < 0.01).
Matrix Remodeling Capacity of Human AF Cells Exposed to Mechanical and Inflammatory Stimuli
The effect of mechanical loading and inflammatory stimuli on the production of matrix-degrading enzymes and ECM proteins by human AF cells was also evaluated. The results showed that CTS by itself does not interfere with the matrix remodeling capacity of AF cells. However, when CTS was combined with IL-1β, it significantly increased MMP-1 and MMP-3 expression compared with CTS-treatment (***p < 0.001 and **p < 0.01, respectively) and with unstimulated cells (**p < 0.01 and ***p < 0.001, respectively; Figures 3A,B). A significant upregulation of MMP-3 expression was also observed by the IL-1β-stimulation in comparison to control. In addition, the CTS + IL-1β treatment significantly downregulated COL1A1 by AF cells (*p < 0.05) and upregulated elastin (**p < 0.01), a key AF protein involved in tissue elasticity, compared to CTS-treated cells (Figures 3C,D). This trend was also observed in the presence of IL-1β only, with COL1A1 significantly downregulated (*p < 0.05), in comparison with unstimulated cells. In contrast, neither CTS nor IL-1β seemed to affect fibrillin-1, another glycoprotein involved in AF elasticity (Figure 3E).
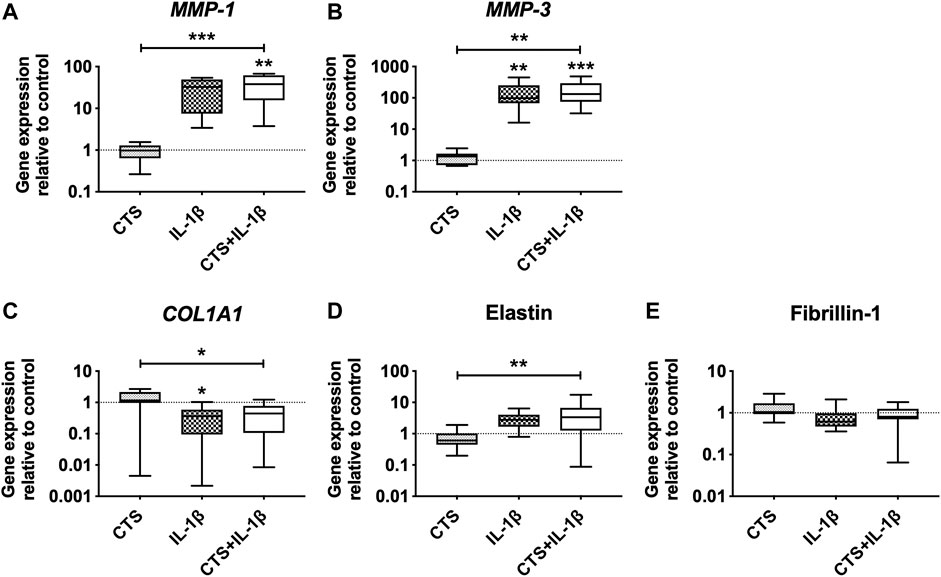
FIGURE 3. Matrix metalloproteinases (MMPs) and extracellular matrix (ECM) gene expression of human AF cells in response to cyclic tensile strain (CTS) and to pro-inflammatory cytokine IL-1β (10 ng/ml) after 48 h. Relative mRNA expression of matrix-degrading enzymes (A) MMP-1, (B) MMP-3, and ECM proteins (C) COL1A1, (D) elastin, and (E) fibrillin-1. Results were normalized to expression level of GAPDH and unstimulated cells. n = 10, *p < 0.05, **p < 0.01.
MSC Secretome Treatment of Human AF Cells Under Mechanical and Inflammatory Stimuli
Since a synergistic action between mechanical stimuli and a pro-inflammatory microenvironment in human AF cells was observed, we went further and investigated the regulatory effect of the MSC secretome in AF cells in the presence of CTS + IL-1β stimulus. Unstimulated AF cell cultures supplemented with MSC secretome served as controls.
The MSC secretome treatment of human AF cells did not affect the gene expression of CD46 and CD59 but significantly upregulated CD55 (*p < 0.05), IL-6, and IL-8 (**p < 0.01) and increased the production of PGE2 (***p < 0.001) compared to unstimulated AF cells (Figure 4B, D–F). Regarding the matrix remodeling capacity of human AF cells, the secretome downregulated the gene expression of MMP-1 (*p < 0.01) and MMP-3 (p = 0.07) (Figures 5A,B) compared to CTS + IL-1β stimulation alone and did not alter COL1A1, elastin, or fibrillin-1 expression (Figures 5C–E). In accordance with the increase in pro-inflammatory cytokines, the MSC secretome significantly upregulated MMP-1 (*p < 0.05) and MMP-3 (**p < 0.05) gene expression in control AF cells. Furthermore, MMP activity was evaluated by gelatin zymography in AF culture supernatants. The pro- and active forms of the gelatinases MMP-2 (between 72 and 63 kDa) and MMP-9 (between 82 and 92 kDa) (Cardoso et al., 2014), particularly the latter, were identified (Figure 5F). The MMP bands were quantified, and a band of MSC secretome as a control was subtracted from the other conditions tested. The results show that the MSC secretome was able to reduce the activity of gelatin-degrading enzymes produced by human AF cells, namely, the active form of MMP-9 (p = 0.06; Figure 5G).
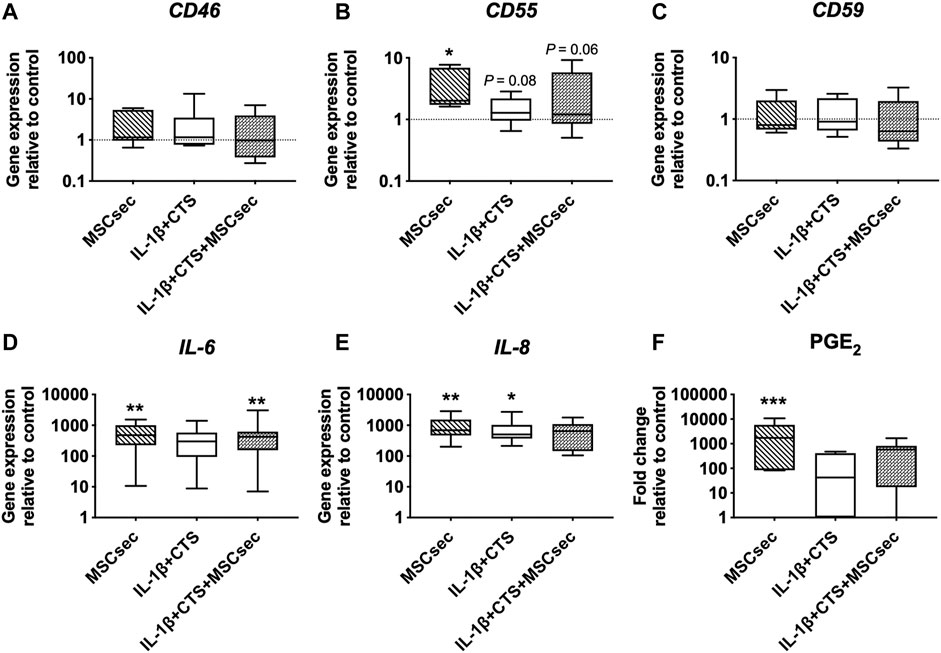
FIGURE 4. Effect of MSC secretome on human AF cells stimulated with cyclic tensile strain (CTS) and IL-1β (10 ng/ml) in the expression of complement markers and pro-inflammatory cytokines after 48 h. Relative mRNA expression of complement regulatory factors (A) CD46, (B) CD55, and (C) CD59; and pro-inflammatory markers (D) IL-6 and (E) IL-8. Results were normalized to expression level of GAPDH and to unstimulated cells. (F) Concentration of pro-inflammatory marker PGE2 (fold change to unstimulated cells). n = 7, *p < 0.05, **p < 0.01.
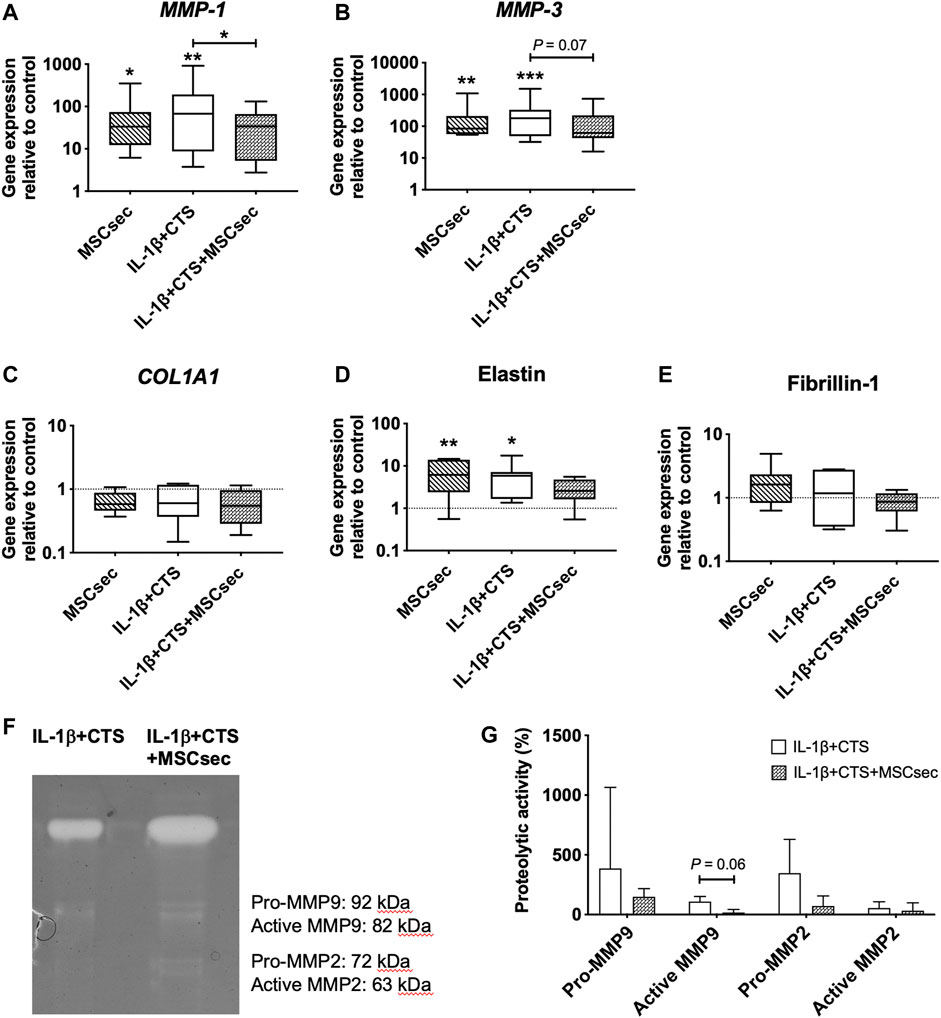
FIGURE 5. Effect of MSC secretome on human AF cells stimulated with cyclic tensile strain (CTS) and IL-1β (10 ng/ml) in MMPs and ECM proteins after 48 h. Relative mRNA expression of the matrix degrading enzymes (A) MMP-1 and (B) MMP-3, and of ECM proteins (C) COL1A1, (D) elastin, and (E) fibrillin-1. Results were normalized to the expression level of GAPDH and unstimulated cells. n = 7, *p < 0.05, **p < 0.01. (F) MMP activity in AF cultures media, determined by gelatin zymography (G) Quantification of MMP-2 and MMP-9 activity by densitometry (n = 3).
In addition, the concentration of different tissue inhibitors of MMPs–TIMP-1, TIMP-2, TIMP-3, and TIMP-4—was quantified in all the conditions (Figure 6), with the concentrations found in the MSC secretome being subtracted from the AF culture supernatants. The MSC secretome significantly increased the production of TIMP-1 by CTS + IL-1β–treated AF cells (*p < 0.05) but did not affect TIMP-2, TIMP-3, or TIMP-4. An increase in TIMP-1 and TIMP-2 was observed in unstimulated cells upon treatment with MSC secretome (**p < 0.01 and *p < 0.05).
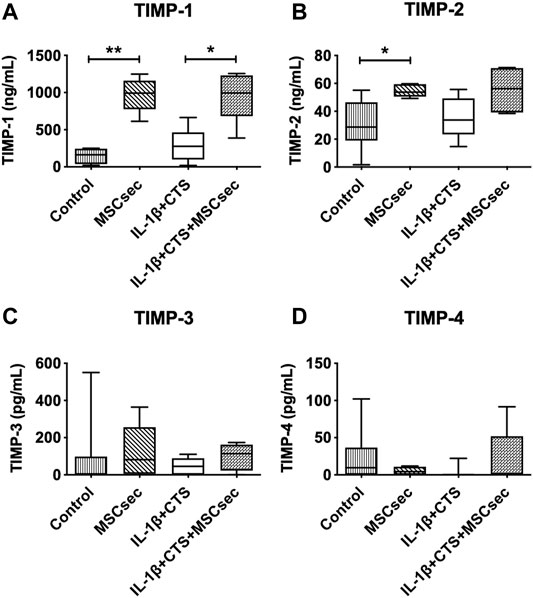
FIGURE 6. Concentration of tissue inhibitors of metalloproteinases (TIMPs) in the supernatant of AF cultures stimulated with cyclic tensile strain (CTS) and IL-1β (10 ng/ml) and treated with MSC secretome after 48 h, determined by ELISA. (A) TIMP-1, (B) TIMP-2, (C) TIMP-3, and (D) TIMP-4. n = 5–9, *p < 0.05, **p < 0.01.
Discussion
In the present study, a pro-inflammatory/catabolic IVD microenvironment was simulated in human AF cell cultures, based on previous ex vivo models of bovine NP (Teixeira et al., 2016) and AF (Saggese et al., 2019) organ cultures, which were maintained in low-glucose, iso-osmotic (400 mOsm), and pro-inflammatory conditions with low oxygen supply. In the AF organ cultures, a synergistic action of cyclic tensile strain (CTS) and IL-1β was shown to increase the synthesis of pro-inflammatory mediators and MMP-3 in the AF and to decrease the strength of the tissue (Saggese et al., 2019). In the present study, we evaluated the effects of these stimuli (CTS, IL-1β, and CTS + IL-1β) on the metabolism of human AF cells, as well as possible alterations induced by the treatment with secretome produced by IL-1β–preconditioned MSCs.
For that, AF cells were isolated from patients diagnosed with either AIS or DD and expanded up to passage 3–4, in order to obtain enough cells to culture under different stimuli. Although organ culture models could better mimic the degenerative 3D environment of the IVD, these are difficult to conduct under standardized and reproducible conditions using limited human IVD biopsies, which are small in size and often very fragmented. Therefore, they were used for cell isolation and 2D cultures of AF cells. Despite the differences observed in IVD tissues from disc degeneration compared to AIS patients, namely, increased TCC deposition and expression of CD59 (a direct TCC inhibitor) (Teixeira et al., 2021a), the expanded AF cells from both patient groups revealed similar behavior. Primary cells always show a certain variability; however, we expected to see differences between the gene expression profiles of cells from AIS and DD due to the lower age of AIS versus DD patients (an average of 16 ± 2 versus 65 ± 11 years old, respectively) and less degeneration in AIS tissues. However, CTS and/or IL-1β did not induce a significantly different response between the two groups for cells in passages 3 to 4. After expansion in 2D cultures, we hypothesize that the cells from DD patients lose their disease phenotype, behaving similarly to AF cells from AIS patients. This suggests that in vitro expansion of AF cells might attenuate cellular changes associated with patients’ age and specific disease, in line with recent findings showing similar expression of complement-related markers by AF cells from AIS and DD patients after challenging with IL-1β and cathepsin-D (Teixeira et al., 2021b).
CTS + IL-1β stimulation of human AF cells induced an upregulation of pro-inflammatory cytokines (IL-6, IL-8, and PGE2) and MMPs (MMP-1, MMP-3), which was observed to a lesser extent in the presence of IL-1β, but not after CTS stimulation by itself. In the present work, 2% strain alone did not seem to be a strong enough stimulus to induce changes in the AF cell gene expression profile. This might be due to the 2D cell cultures, in contrast to previous investigations of human AF cells cultured in 3D collagen gels, in which differences were observed (Neidlinger-Wilke et al., 2005). CTS stimulation of AF cells has been shown to increase COL2A1 and aggrecan expression and decrease MMP-3 expression in human AF cells cultured in 3D collagen gels, independently of the strain magnitude (1–8% strain, 1 Hz) (Neidlinger-Wilke et al., 2005). CTS was also shown to promote upregulation of pro-inflammatory genes, like COX-2, IL-6, and IL-8 in AF cells (8% strain, 1 Hz) (Pratsinis et al., 2016). Under mild pro-inflammatory stimulation (1 ng/ml IL-1β) and 6% CTS (0.05 Hz), CTS was suggested to have a protective effect on rat AF cells (Sowa and Agarwal, 2008). Since a role for complement-associated factors has recently been suggested in a degenerative/pro-inflammatory environment, we investigated the gene expression of the cellular complement regulators CD46, CD55, and CD59 by human AF cells. Here, CD55, but not the other complement factors, was upregulated by CTS + IL-1β stimulation of AF cells. In bovine AF organ cultures, CTS + IL-1β simulation induced upregulation of CD46, but not of CD55 nor CD59 (Neidlinger-Wilke et al., 2021). IL-1β stimulation of human articular chondrocytes upregulated CD45, CD55 and CD59 (Hyc et al., 2003). This indicates that the complement cascade is activated by the CTS and IL-1β stimuli; however, different molecules may be regulated at different timepoints.
Regarding matrix metabolism, CTS + IL-1β stimulation of human AF cells did not alter COL1A1 and fibrillin-1 production but seemed to enhance elastin synthesis. The latter was also promoted by CTS and IL-1β individually. Fibrillin-1 production was previously shown to be increased within the translamellar bridging-network of bovine AF organ cultures in the presence of IL-1β but not in the presence of CTS + IL-1β (Saggese et al., 2019). Besides the differences between 2D and 3D (organ culture), this study also used lower IL-1β concentration (1 ng/ml), which could explain the different results of the present study. Concerning elastin, TNF-α stimulation of human tenocytes was shown to reduce COL1 deposition while increased elastin gene expression (John et al., 2010), in agreement to what was observed with AF cells in our study. Moreover, it has been described that elastin increases with IVD degeneration and aging, particularly in the AF, consisting in a response to restore the lamellar structure under radial loads that potentially cause delamination (Cloyd and Elliott, 2007).
Previously, we have demonstrated that MSCs cultured with bovine NP in the presence of IL-1β downregulated the pro-inflammatory markers bIL-6, bIL-8, and bTNF-α by NP cells but did not promote ECM synthesis (Teixeira et al., 2018). MSCs secrete numerous soluble factors in response to microenvironmental cues, which include pro-inflammatory stimulation, 3D environment, or hypoxia. Therefore, the MSC secretome has been explored as a cell-based/cell-free therapy for several disorders (Ferreira et al., 2018). MSC IL-1β preconditioning has been shown to upregulate chemokines (CCL5, CCL20, CXCL1, CXCL3, CXCL5, CXCL6, CXCL10, CXCL11, and CX (3)CL1), interleukins (IL-6, IL-8, IL-23A, IL-32), toll-like receptors (TLR2, TLR4, CLDN1), MMPs (MMP-1, MMP-3), growth factors (CSF2, TNF-α), and adhesion molecules (ICAM1, ICAM4), compared to unstimulated MSCs (Carrero et al., 2012). Moreover, although the cell passage can affect the cellular response, it has been reported that the composition of the MSC secretome does not vary significantly between passages 3 and 12 (Serra et al., 2018).
In the IVD, the MSC secretome has been suggested to stimulate the cellular repair capacity (Brisby et al., 2013). For instance, the secretome of human umbilical cord–derived MSCs was shown to restore the stemness of NP progenitor cells, thus rejuvenating the degenerated NP (Zeng et al., 2020). Moreover, the secretome of IL-1β–preconditioned MSCs, enriched in IL-6, RANTES, MCP-1, IL-1β, TIMP-2, IL-8, GRO α/β/γ, leptin, MIP-3α, and CXCL1, downregulated the expression/production of inflammatory markers by bovine NP, while promoting aggrecan deposition (Ferreira et al., 2021). It was also shown to downregulate the expression/production of inflammatory markers, complement system regulators, MMPs, TIMPs, and collagen by bovine AF cells in an ex vivo model (Neidlinger-Wilke et al., 2021). In contrast to the previous findings, in the present study, the MSC secretome did not downregulate the expression of cellular complement regulators and pro-inflammatory cytokines of CTS + IL-1β–stimulated human AF cells but strongly downregulated MMP-1 and MMP-3 gene expression. Furthermore, in this study, it was demonstrated to have reduced MMP-2 and MMP-9 activity of human AF cells in presence of the MSC secretome. MMP-1 and MMP-3 are strongly expressed in the IVD of young adults but not in those of children and adolescents, while MMP-2 and MMP-9 are present in older adults, but in fewer cells (Weiler et al., 2002). Moreover, static compression and annular puncture of rat caudal discs have been shown to stimulate MMP-2 activity with simultaneous AF lamellar disorganization, linking MMP-2 to local matrix degradation and collagen remodeling (Rastogi et al., 2013). Therefore, the MSC secretome could be an interesting approach to modulate matrix remodeling in the AF, although further studies need to be conducted in human IVD to better understand its impact on the tissue biomechanics.
In addition, besides being enriched in TIMP-2 (Ferreira et al., 2021), the MSC secretome also stimulated the production of TIMP-1 and TIMP-2, but not TIMP-3 and TIMP-4, by human AF cells, which could be directly correlated with MMP inhibition. This is in agreement with what was previously observed by Le Maitre et al. (2004), who described an increase in TIMP-1 and TIMP-2, but not TIMP-3, in degenerated human IVD biopsies. Vo et al. (2013) also described sthe upregulation of TIMP-1 with IVD degeneration, as well as a downregulation of TIMP-3. Overall, mechanical and inflammatory stimuli play a crucial role in the modulation of the expression levels of MMPs, ADAMTs, and TIMPs. Inhibition of MMPs and ADAMTSs has demonstrated efficacy and therapeutic potential in slowing osteoarthritis progression (Fosang and Little, 2008), which suggests that therapies proposing the inhibition of matrix-degrading enzymes or stimulation of TIMPs are promising for IVD, although they should be tissue-specific and carefully localized.
Our study shows a therapeutic effect of the MSC secretome on the AF cells challenged with CTS + IL-1β. To induce a pro-inflammatory microenvironment, 10 ng/ml IL-1β was used, but other inflammatory cytokines are known to be widely expressed in IVD degeneration/herniation, such as TNF-α or IL-6, and should be also addressed in future studies. More recently, Wangler et al. (2021) have shown that conditioned medium from traumatically injured versus degenerated IVD has different proteome contents, so different inflammatory cytokine cocktails should be explored in the future to deepen the knowledge of the therapeutic effect of MSC secretome on IVD. In vivo experiments that fully recreate the degenerative IVD microenvironment will prove the regulatory effect of the secretome of IL-1β–preconditioned MSCs on the modulation of the immune system response and IVD degeneration.
Conclusion
Overall, this study demonstrates that the inflammatory milieu, in synergy with tensile strain, has an impact on human AF cells, promoting upregulation of inflammatory markers, cellular complement regulators, and elastin; increasing MMP activity and TIMP production; and decreasing COL1A1 expression. Moreover, this study evidences a novel aspect of the mechanism of action of the MSC secretome in degenerated IVD that consists in the modulation of MMP activity and TIMP-1 production by AF cells.
Data Availability Statement
The original contributions presented in the study are included in the article/Supplementary Material, further inquiries can be directed to the corresponding author.
Ethics Statement
The studies involving human participants were reviewed and approved by Ulm University (371/19), Ulm, Germany, and by Heidelberg University (S-051/2016), Heidelberg, Germany. Written informed consent to participate in this study was provided by the participants or by the participants’ legal guardian/next of kin (when underage).
Author Contributions
RG and GT designed the study, analyzed the data, and wrote the manuscript. TS, ZY, and JF contributed to data collection and revised the manuscript. AI, H-JW, and CN-W helped to optimize the research and proofread the manuscript. All authors read and approved the final manuscript.
Funding
The study was supported by Ulm University (L.SBN.0157), German Spine Foundation (Deutsche Wirbelsäulenstiftung) and the German Academic Exchange Service (DAAD). RG was supported by the Alexander von Humboldt Foundation and by Conselho de Reitores das Universidades Portuguesas.
Conflict of Interest
The authors declare that the research was conducted in the absence of any commercial or financial relationships that could be construed as a potential conflict of interest.
Publisher’s Note
All claims expressed in this article are solely those of the authors and do not necessarily represent those of their affiliated organizations, or those of the publisher, the editors, and the reviewers. Any product that may be evaluated in this article, or claim that may be made by its manufacturer, is not guaranteed or endorsed by the publisher.
Acknowledgments
The authors wish to thank Mrs. Iris Baum for technical support.
Supplementary Material
The Supplementary Material for this article can be found online at: https://www.frontiersin.org/articles/10.3389/fbioe.2021.802789/full#supplementary-material
References
Brisby, H., Papadimitriou, N., Brantsing, C., Bergh, P., Lindahl, A., and Barreto Henriksson, H. (2013). The Presence of Local Mesenchymal Progenitor Cells in Human Degenerated Intervertebral Discs and Possibilities to Influence These In Vitro: a Descriptive Study in Humans. Stem Cell Development. 22, 804–814. doi:10.1089/scd.2012.0179
Cardoso, A. P., Pinto, M. L., Pinto, A. T., Oliveira, M. I., Pinto, M. T., Gonçalves, R., et al. (2014). Macrophages Stimulate Gastric and Colorectal Cancer Invasion through EGFR Y1086, C-Src, Erk1/2 and Akt Phosphorylation and smallGTPase Activity. Oncogene. 33, 2123–2133. doi:10.1038/onc.2013.154
Carrero, R., Cerrada, I., Lledó, E., Dopazo, J., García-García, F., Rubio, M.-P., et al. (2012). IL1β Induces Mesenchymal Stem Cells Migration and Leucocyte Chemotaxis Through NF-Κb. Stem Cell Rev Rep. 8, 905–916. doi:10.1007/s12015-012-9364-9
Cloyd, J. M., and Elliott, D. M. (2007). Elastin Content Correlates with Human Disc Degeneration in the Anulus Fibrosus and Nucleus Pulposus. Spine. 32, 1826–1831. doi:10.1097/brs.0b013e3181132a9d
Cunha, C., Almeida, C. R., Almeida, M. I., Silva, A. M., Molinos, M., Lamas, S., et al. (2017). Systemic Delivery of Bone Marrow Mesenchymal Stem Cells for In Situ Intervertebral Disc Regeneration. Stem Cell Transl. Med. 6, 1029–1039. doi:10.5966/sctm.2016-0033
Ferreira, J. R., Teixeira, G. Q., Santos, S. G., Barbosa, M. A., Almeida-Porada, G., and Gonçalves, R. M. (2018). Mesenchymal Stromal Cell Secretome: Influencing Therapeutic Potential by Cellular Pre-conditioning. Front. Immunol. 9, 2837. doi:10.3389/fimmu.2018.02837
Ferreira, J., Teixeira, G. Q., Teixeira, G., Neto, e., Ribeiro-Machado, c., Silva, A., et al. (2021). IL-1β-pre-Conditioned Mesenchymal Stem/Stromal Cells' Secretome Modulates the Inflammatory Response and Aggrecan Deposition in Intervertebral Disc. Eur. Cell Mater. 41, 431–543. doi:10.22203/ecm.v041a28
Fosang, A. J., and Little, C. B. (2008). Drug Insight: Aggrecanases as Therapeutic Targets for Osteoarthritis. Nat. Rev. Rheumatol. 4, 420–427. doi:10.1038/ncprheum0841
Hyc, A., Osiecka-Iwan, A., Strzelczyk, P., and Moskalewski, S. (2003). Effect of IL-1β, TNF-Agr; and IL-4 on Complement Regulatory Protein mRNA Expression in Human Articular Chondrocytes. Int. J. Mol. Med. 11, 91–94. doi:10.3892/ijmm.11.1.91
John, T., Lodka, D., Kohl, B., Ertel, W., Jammrath, J., Conrad, C., et al. (2010). Effect of Pro-Inflammatory and Immunoregulatory Cytokines on Human Tenocytes. J. Orthop. Res. 28, 1071–1077. doi:10.1002/jor.21079
Le Maitre, C. L., Freemont, A. J., and Hoyland, J. A. (2004). Localization of Degradative Enzymes and Their Inhibitors in the Degenerate Human Intervertebral Disc. J. Pathol. 204, 47–54. doi:10.1002/path.1608
Livak, K. J., and Schmittgen, T. D. (2001). Analysis of Relative Gene Expression Data Using Real-Time Quantitative PCR and the 2−ΔΔCT Method. Methods. 25, 402–408. doi:10.1006/meth.2001.1262
Maher, C., Underwood, M., and Buchbinder, R. (2017). Non-Specific Low Back Pain. The Lancet. 389, 736–747. doi:10.1016/s0140-6736(16)30970-9
Neidlinger-Wilke, C., Ekkerlein, A., Goncalves, R., Ferreira, J., Ignatius, A., Wilke, H., et al. (2021). Mesenchymal Stem Cell Secretome Decreases the Inflammatory Response in Annulus Fibrosus Organ Cultures. Eur. Cell Mater. 42, 1–19. doi:10.22203/ecm.v042a01
Neidlinger-Wilke, C., Wilke, H.-J., and Claes, L. (1994). Cyclic Stretching of Human Osteoblasts Affects Proliferation and Metabolism: A New Experimental Method and its Application. J. Orthop. Res. 12, 70–78. doi:10.1002/jor.1100120109
Neidlinger-Wilke, C., Würtz, K., Liedert, A., Schmidt, C., Börm, W., Ignatius, A., et al. (2005). A Three-Dimensional Collagen Matrix as a Suitable Culture System for the Comparison of Cyclic Strain and Hydrostatic Pressure Effects on Intervertebral Disc Cells. J. Neurosurg. Spine. 2, 457–465. doi:10.3171/spi.2005.2.4.0457
Noriega, D. C., Ardura, F., Hernández-Ramajo, R., Martín-Ferrero, M. Á., Sánchez-Lite, I., Toribio, B., et al. (2017). Intervertebral Disc Repair by Allogeneic Mesenchymal Bone Marrow Cells. Transplantation. 101, 1945–1951. doi:10.1097/tp.0000000000001484
Orozco, L., Soler, R., Morera, C., Alberca, M., Sánchez, A., and García-Sancho, J. (2011). Intervertebral Disc Repair by Autologous Mesenchymal Bone Marrow Cells: a Pilot Study. Transplantation. 92, 822–828. doi:10.1097/tp.0b013e3182298a15
Pratsinis, H., Papadopoulou, A., Neidlinger-Wilke, C., Brayda-Bruno, M., Wilke, H.-J., and Kletsas, D. (2016). Cyclic Tensile Stress of Human Annulus Fibrosus Cells Induces MAPK Activation: Involvement in Proinflammatory Gene Expression. Osteoarthritis and Cartilage. 24, 679–687. doi:10.1016/j.joca.2015.11.022
Rastogi, A., Kim, H., Twomey, J. D., and Hsieh, A. H. (2013). MMP-2 Mediates Local Degradation and Remodeling of Collagen by Annulus Fibrosus Cells of the Intervertebral Disc. Arthritis Res. Ther. 15, R57. doi:10.1186/ar4224
Risbud, M. V., and Shapiro, I. M. (2014). Role of Cytokines in Intervertebral Disc Degeneration: Pain and Disc Content. Nat. Rev. Rheumatol. 10, 44–56. doi:10.1038/nrrheum.2013.160
Saggese, T., Teixeira, G. Q., Wade, K., Moll, L., Ignatius, A., Wilke, H.-J., et al. (2019). Georg Schmorl Prize of The German Spine Society (Dwg) 2018: Combined Inflammatory and Mechanical Stress Weakens the Annulus Fibrosus: Evidences from a Loaded Bovine AF Organ Culture. Eur. Spine J. 28, 922–933. doi:10.1007/s00586-019-05901-w
Sakai, D., Mochida, J., Iwashina, T., Hiyama, A., Omi, H., Imai, M., et al. (2006). Regenerative Effects of Transplanting Mesenchymal Stem Cells Embedded in Atelocollagen to the Degenerated Intervertebral Disc. Biomaterials. 27, 335–345. doi:10.1016/j.biomaterials.2005.06.038
Serra, S. C., Costa, J. C., Assunção-Silva, R. C., Teixeira, F. G., Silva, N. A., Anjo, S. I., et al. (2018). Influence of Passage Number on the Impact of the Secretome of Adipose Tissue Stem Cells on Neural Survival, Neurodifferentiation and Axonal Growth. Biochimie. 155, 119–128. doi:10.1016/j.biochi.2018.09.012
Shin, E.-H., Cho, K.-J., Kim, Y.-T., and Park, M.-H. (2019). Risk Factors for Recurrent Lumbar Disc Herniation after Discectomy. Int. Orthopaedics (Sicot). 43, 963–967. doi:10.1007/s00264-018-4201-7
Sowa, G., and Agarwal, S. (2008). Cyclic Tensile Stress Exerts a Protective Effect on Intervertebral Disc Cells. Am. J. Phys. Med. Rehabil. 87, 537–544. doi:10.1097/phm.0b013e31816197ee
Teixeira, G. Q., Boldt, A., Nagl, I., Pereira, C. L., Benz, K., Wilke, H.-J., et al. (2016). A Degenerative/Proinflammatory Intervertebral Disc Organ Culture: an Ex Vivo Model for Anti-inflammatory Drug and Cell Therapy. Tissue Eng. C: Methods. 22, 8–19. doi:10.1089/ten.tec.2015.0195
Teixeira, G. Q., Pereira, C. L., Ferreira, J. R., Maia, A. F., Gomez-Lazaro, M., Barbosa, M. A., et al. (2018). Immunomodulation of Human Mesenchymal Stem/Stromal Cells in Intervertebral Disc Degeneration. Spine (Phila Pa 1976). 43, E673–E682. doi:10.1097/brs.0000000000002494
Teixeira, G. Q., Yong, Z., Goncalves, R. M., Kuhn, A., Riegger, J., Brisby, H., et al. (2021a). Terminal Complement Complex Formation Is Associated with Intervertebral Disc Degeneration. Eur. Spine J. 30, 217–226. doi:10.1007/s00586-020-06592-4
Teixeira, G. Q., Yong, Z., Kuhn, A., Riegger, J., Goncalves, R. M., Ruf, M., et al. (2021b). Interleukin-1β and Cathepsin D Modulate Formation of the Terminal Complement Complex in Cultured Human Disc Tissue. Eur. Spine J. 30, 2247–2256. doi:10.1007/s00586-021-06901-5
Vo, N. V., Hartman, R. A., Yurube, T., Jacobs, L. J., Sowa, G. A., and Kang, J. D. (2013). Expression and Regulation of Metalloproteinases and Their Inhibitors in Intervertebral Disc Aging and Degeneration. Spine J. 13, 331–341. doi:10.1016/j.spinee.2012.02.027
Vos, T., Lim, S. S., Abbafati, C., Abbas, K. M., Abbasi, M., Abbasifard, M., et al. (2020). Global burden of 369 Diseases and Injuries in 204 Countries and Territories, 1990-2019: a Systematic Analysis for the Global Burden of Disease Study 2019. Lancet. 396, 1204–1222. doi:10.1016/S0140-6736(20)30925-9
Wangler, S., Kamali, A., Wapp, C., Wuertz-Kozak, K., Häckel, S., Fortes, C., et al. (2021). Uncovering the Secretome of Mesenchymal Stromal Cells Exposed to Healthy, Traumatic, and Degenerative Intervertebral Discs: a Proteomic Analysis. Stem Cell Res. Ther. 12, 11. doi:10.1186/s13287-020-02062-2
Weiler, C., Nerlich, A., Zipperer, J., Bachmeier, B., and Boos, N. (2002). 2002 SSE Award Competition in Basic Science: Expression of Major Matrix Metalloproteinases Is Associated with Intervertebral Disc Degradation and Resorption. Eur. Spine J. 11, 308–320. doi:10.1007/s00586-002-0472-0
Weinstein, J. N., Lurie, J. D., Tosteson, T. D., Tosteson, A. N. A., Blood, E. A., Abdu, W. A., et al. (2008). Surgical versus Nonoperative Treatment for Lumbar Disc Herniation. Spine. 33, 2789–2800. doi:10.1097/brs.0b013e31818ed8f4
Wuertz, K., Urban, J. P. G., Klasen, J., Ignatius, A., Wilke, H.-J., Claes, L., et al. (2007). Influence of Extracellular Osmolarity and Mechanical Stimulation on Gene Expression of Intervertebral Disc Cells. J. Orthop. Res. 25, 1513–1522. doi:10.1002/jor.20436
Zeng, X., Lin, J., Wu, H., Yu, J., Tu, M., Cheang, L. H., et al. (2020). Effect of Conditioned Medium from Human Umbilical Cord-Derived Mesenchymal Stromal Cells on Rejuvenation of Nucleus Pulposus Derived Stem/Progenitor Cells from Degenerated Intervertebral Disc. Int. J. Stem Cell. 13, 257–267. doi:10.15283/ijsc20027
Keywords: disc degeneration, disc herniation, annulus fibrosus cells, mechanical loading, inflammation, mesenchymal stem cells, secretome, matrix metalloproteinases
Citation: Gonçalves RM, Saggese T, Yong Z, Ferreira JR, Ignatius A, Wilke H-J, Neidlinger-Wilke C and Teixeira GQ (2022) Interleukin-1β More Than Mechanical Loading Induces a Degenerative Phenotype in Human Annulus Fibrosus Cells, Partially Impaired by Anti-Proteolytic Activity of Mesenchymal Stem Cell Secretome. Front. Bioeng. Biotechnol. 9:802789. doi: 10.3389/fbioe.2021.802789
Received: 27 October 2021; Accepted: 27 December 2021;
Published: 28 January 2022.
Edited by:
Rahul Gawri, McGill University, CanadaReviewed by:
Yong-Can Huang, Peking University Shenzhen Hospital, ChinaDiane Gregory, Wilfrid Laurier University, Canada
Copyright © 2022 Gonçalves, Saggese, Yong, Ferreira, Ignatius, Wilke, Neidlinger-Wilke and Teixeira. This is an open-access article distributed under the terms of the Creative Commons Attribution License (CC BY). The use, distribution or reproduction in other forums is permitted, provided the original author(s) and the copyright owner(s) are credited and that the original publication in this journal is cited, in accordance with accepted academic practice. No use, distribution or reproduction is permitted which does not comply with these terms.
*Correspondence: Graciosa Q. Teixeira, Z3JhY2lvc2EudGVpeGVpcmFAdW5pLXVsbS5kZQ==
†These authors have contributed equally to this work and share last authorship