- Department of Orthopaedics, Alpert Medical School of Brown University/Rhode Island Hospital, Providence, RI, United States
Surgical repair of meniscus injury is intended to help alleviate pain, prevent further exacerbation of the injury, restore normal knee function, and inhibit the accelerated development of post-traumatic osteoarthritis (PTOA). Meniscus injuries that are treated poorly or left untreated are reported to significantly increase the risk of PTOA in patients. Current surgical approaches for the treatment of meniscus injuries do not eliminate the risk of accelerated PTOA development. Through recent efforts by scientists to develop innovative and more effective meniscus repair strategies, the use of biologics, allografts, and scaffolds have come into the forefront in pre-clinical investigations. However, gauging the extent to which these (and other) approaches inhibit the development of PTOA in the knee joint is often overlooked, yet an important consideration for determining the overall efficacy of potential treatments. In this review, we catalog recent advancements in pre-clinical therapies for meniscus injuries and discuss the assessment methodologies that are used for gauging the success of these treatments based on their effect on PTOA severity. Methodologies include histopathological evaluation of cartilage, radiographic evaluation of the knee, analysis of knee function, and quantification of OA predictive biomarkers. Lastly, we analyze the prevalence of these methodologies using a systemic PubMed® search for original scientific journal articles published in the last 3-years. We indexed 37 meniscus repair/replacement studies conducted in live animal models. Overall, our findings show that approximately 75% of these studies have performed at least one assessment for PTOA following meniscus injury repair. Out of this, 84% studies have reported an improvement in PTOA resulting from treatment.
Introduction
The meniscus in the knee joint is a fibrocartilaginous tissue positioned between the femoral condyle and the tibial plateau. The meniscus performs vital functions of shock absorption and provides mechanical stability to the knee joint. Unlike articular cartilage, which lines the tibial plateau and femoral condyle of the knee joint, the menisci are soft tissues that are kept in place by ligamentous attachments, and hence they can slide and contort during knee flexion/extension. The medial and lateral menisci are key structures in the knee joint that help to dissipate forces, provide stability, and lubricate and protect the articular cartilage from injury (McDermott and Amis, 2006). With increases in physically active and aged populations, incidents of meniscus injury have spiked at a significant rate. For instance, in the United States alone, meniscus injuries are encountered by 6–8% of active young adults annually and this number is increased in the elderly population (Gee et al., 2020; Kennedy et al., 2020). Initially, the meniscus was considered to be vestigial tissue, which can simply be removed surgically upon major injury, as a means of alleviating pain and restoring function to the knee joint (Chivers and Howitt, 2009; McNulty and Guilak, 2015). However, it is now known that the absence of, or injury to, meniscal tissue significantly increases the risk of post-traumatic osteoarthritis (PTOA) (Badlani et al., 2013; Chang and Brophy, 2020; Rai et al., 2020; Adams et al., 2021; Bedrin et al., 2021). Indeed, according to a recent 2020 epidemiological study of young adults, approximately 13% of patients with meniscus injuries develop OA by 18 years post-injury, whereas only about 3% of patients with uninjured knees developed the disease (Snoeker et al., 2020). While there is a strong emphasis on improving meniscus healing in emerging biomedical research, it is equally important to assess how new strategies for treating these injuries affect the development of OA in the knee. Hence, the efficacy of treatment should not rely solely on the assessment of the extent of meniscus repair, but it should also include a comprehensive evaluation of secondary/indirect outcome measures of therapeutic efficacy, such as its ability to preserve cartilage health, prevent the expression of established PTOA serum and blood biomarkers, and restore knee joint mechanics/function as closely as possible to the pre-injury state. In this review, we have cataloged recent pre-clinical therapeutic strategies that are being developed to promote meniscus healing. We further discuss the methodologies used in these studies to evaluate their respective outcome measures and detail their findings.
Basic Meniscus Structure and Anatomy
Meniscus is divided into three zones from the outer peripheral region to the inner central thinnest region based on the degree of vascularization. The outermost region is thickest, and it is significantly vascularized. It is referred to as the red-red zone (Andrews et al., 2011; Bryceland et al., 2017). Due to the vascularization, the red-red zone is enriched in nutrients and cytokines, enabling the self-healing upon injury in this area. The inner (middle) region is referred as the red-white zone, which contains less vascularization than the red-red zone and has minimal self-healing capacity. The innermost (central) region of the meniscus is completely devoid of vasculature and lacks any ability to self-repair. This white-white zone is tapered in shape with an unattached free edge.
The lateral and medial menisci are smooth, lubricated crescent-shaped fibrocartilage tissues that partially cover and protect the articular cartilage surface from injury. In humans, the lateral and medial menisci vary greatly in their dimensions. The lateral meniscus is approximately 27–29 mm wide and 33–35 mm in length, whereas the medial meniscus ranges from 27–28 mm in width and 41–45 mm in length (Shaffer et al., 2000; McDermott et al., 2004). The lateral meniscus occupies almost one-third of the tibial plateau with the superior concave surface pivoting the femoral condyles, while the ventral surface articulates the tibial condyle (Erdemir, 2016; Zhang et al., 2020). The lateral meniscus has a constant width that occupies a large portion of the total articular surface. The medial meniscus articulates inferiorly with the medial condyle of the tibia and superiorly with the medial condyle of the femur bone. The medial meniscus occupies more than half of the contact portion of the medial compartment. The anterior horn of the medial meniscus attaches near the ACL on the tibia, whereas the posterior horn attaches above the PCL. The medial meniscus is more crescent-shaped and covers a smaller surface of the tibial plateau compared to the lateral meniscus (Makris et al., 2011; Fox et al., 2015). Reduction of meniscal tissue due to surgical resection performed as a treatment for severe meniscus tears can result in increased forces on the articular cartilage during knee loading. Veritably, the amount of meniscus tissue resected is reported to have a positive relationship with detrimental shear stress on the cartilage surfaces that can lead to OA (Vadher et al., 2006).
Clinical Surgical Interventions for Meniscus Injuries
Meniscal injury is one of the most common sports-related knee injuries in the United States (Majewski et al., 2006; Korpershoek et al., 2017). The combined hypovascularity and hypocellularity within the white-white zone of the meniscus significantly hinders its ability to adequately heal (Athanasiou, 2009). A meniscal injury, especially one in a hard-of-healing area such as the white-white zone, becomes a strong predictor for the accelerated development of osteoarthritis (OA). This can be attributed to the undeniable importance of meniscus in maintaining knee joint health as well as the shortcomings of traditional arthroscopic meniscus repair strategies.
Deciding the best clinical course of action for surgically treating a meniscal tear not only depends on the location, nature of the injury, and tear type, but it also relies on the age of the patient and potential co-morbidities (i.e., obesity, concomitant chondral/ligamentous damage that is presented with the meniscus injury). Treatment strategies can range from minor non-surgical means such as physical therapy and the use of non-steroidal anti-inflammatory medications, to surgical means such as meniscus suturing or partial meniscectomy/resection (surgical removal of a portion of the meniscus) (Terry Canale S, 2012; Wells et al., 2021).
Suturing and Resection
Suturing is the most conservative of the surgical approaches for meniscus repair; however, this treatment strategy has the most long-term success rate for repairing isolated tears in younger patients (Hagmeijer et al., 2019). Meniscus tears are commonly sutured according to suture placement orientation, relative to the tear: all inside (Darabos et al., 2012), outside-in (Rodeo, 2000), and inside-out (Steenbrugge et al., 2004) techniques. For more complex tears that present with tissue loss, meniscal resection is used to remove the damaged and unrepairable areas of fibrocartilage in order to reshape the meniscus to its natural semilunar shape, but this removal of tissue has been shown to alter the contact pressures within the joint (Terry Canale S, 2012). Several long-term follow-up studies in athletes and youths who have undergone either partial or complete meniscectomy suggest an increased risk of PTOA (Allen et al., 1984; Jørgensen et al., 1987; Faunø and Nielsen, 1992; Hede et al., 1992; Benedetto and Rangger, 1993; Rangger et al., 1997; Papalia et al., 2011; Longo et al., 2019).
Meniscus Allograft Transplantation
Additionally, meniscal allograft transplantation (MAT) is implemented in cases of severe meniscal injuries which cannot be rectified using suturing or partial resection. MAT has become more frequently used as a state-of-the-art technique utilized in the pursuit of preserving/restoring the function and mechanics of the knee in severe or irreparable meniscus injuries. MAT was first described by Milachowski et al. (Milachowski et al., 1989) in 1989; since then, this strategy has been the subject of refinement in the pre-clinical and clinical settings to restore meniscus (and ultimately joint) function while preventing the development of degenerative changes in the knee (Rodeo, 2001; Lee et al., 2012). While the refined implementation of MAT has shown promising results in patients and athletes (Pereira et al., 2019; Searle et al., 2020), it still has several limitations including extrusion and shrinkage of the graft (Lee et al., 2012). The success rate for the two major scaffolds (i.e., collagen scaffolds, polyurethane scaffolds) varies from 38% to as low as 0% after 4 years of follow-up (Houck et al., 2018). The long-term results of scaffolds and allografts can be unpredictable, and it is documented that some may become non-functional due to shrinkage, extrusion, and fragmentation (Trentacosta et al., 2016; Houck et al., 2018). Taken together, these findings highlight the importance of long-term follow-up studies to inspect not only the health of the meniscus but also the entire knee in order to adequately determine the success of any treatment.
Gauging the Success of Emerging Meniscus Repair Strategies in the Pre-Clinical Stage
Clinical assessment of long-term outcomes following meniscus repair/replacement primarily involves looking at reoperation rates for each respective surgical procedure. Other individual assessments include radiographic imaging, surveying the degree of knee pain (as reported by the patient), joint function (i.e., during walking, single hop test), and range of motion (ROM) of the knee in patients using scales such as the Knee injury and Osteoarthritis Outcome Score (KOOS) system, Lysholm, and the Tegner activity scale, to name a few. Some of these outcome measures are understandably difficult if not impractical to implement in animal models that are used in pre-clinical investigations, depending on the species that is used. For this reason, there is a set of assessments that are commonly used in pre-clinical animal studies to gain similar insight into the short and long-term efficacy of treatments.
Developing new and more effective strategies to stimulate meniscus healing is an active area of biomedical research. A number of strategies that are still in the pre-clinical stages of development focus on the use of biologics and biomimetic natural/synthetic materials, which encompass utilizing cells, bioactive factors (both chemical and biological), and injectable/implantable scaffolds, to accelerate meniscus healing (Shimomura et al., 2018). Current primary outcome measures for the success of advanced, pre-clinical meniscal repair treatments include macroscopic and microscopic (histology) assessment of meniscal fibrocartilage using routine/special/immunostaining analysis or magnetic resonance imaging (MRI) of the meniscus, and biomechanical testing of meniscal tissue. Other avenues of assessment include functional analysis of knee kinematics, quantification of secreted inflammation/catabolism associated biomarkers, and macro- and microscopic assessment of the articular surfaces and synovium for PTOA changes.
Histological Assessment of Meniscus Repair Efficacy
Post-mortem histological assessment of meniscal fibrocartilage is currently the gold standard of evaluating the success with which a treatment stimulates healing, especially in pre-clinical animal models. Routine stains such as hematoxylin and eosin (H&E) are useful for the qualitative evaluation of newly formed tissue and its cellularity. Other stains such as Safranin O/Fast Green and Toluidine blue are used primarily to determine molecules of interest that constitute said tissue (i.e., glycosaminoglycans). Further special stains like Picrosirius red allow for the visualization of collagen matrices when imaged under polarized light, providing not only information about the collagen makeup of tissues but also enabling the detection of continuous collagen fiber bundles, which bridge newly formed tissue and pre-existing meniscus tissue at the periphery of the injury site. Furthermore, these stains allow investigators to determine how the orientation of fibers may be changed.
Immunohistochemistry involves the staining of tissue with specific antibodies to detect and visualize matrix molecules (and sometimes even soluble proteins). This technique is used to determine the presence of specific proteins or cells in a given tissue. For instance, López-Franco et al. employed COMP and Ki67 immunohistochemistry to evaluate the differences between healthy human menisci and osteoarthritic human menisci (López-Franco et al., 2016). The use of immunostaining to detect several endogenous markers such as Human Nucleus Antigen (HNA) and cell surface markers CD44 and CD99 have been employed to visualize the endogenous cell localization to the meniscal injury site (Tarafder et al., 2018). Additionally, immunostaining for CD34 and CD146 has been used to distinguish progenitor cell populations in the meniscus, which are proposed to be useful for meniscus repair applications (Osawa et al., 2013).
Longitudinal MRI Imaging to Assess Meniscus Healing Over Time
While histology is arguably the gold standard for evaluating meniscus healing/pathology, it first requires a terminal experimental endpoint where tissue must be extracted for analysis. The benefit of the development and refinement of accurate in vivo imaging techniques for the assessment of meniscus healing is that it allows for longitudinal evaluation in the same subject over time. It has been established that longitudinal Magnetic Resonance Imaging (MRI) using T2 mapping is an informative means of post-injury/repair assessment (Berton et al., 2020; Yamasaki et al., 2020). Additional techniques such as T2Rho and T2* mapping are being adapted and applied to improve the sensitivity of meniscal evaluation (Koff et al., 2013; Zellner et al., 2013; Nakagawa et al., 2016). These techniques have been used to predict tissue degeneration through their ability to detect changes in fiber orientation and tissue biochemistry, including altered water, collagen, and proteoglycan content. For instance, Kondo et al. implanted synovial MSCs (syMSCs) in aged cynomolgus primates and used T1rho MRI mapping to show that this inhibited meniscal degeneration (Kondo et al., 2017). Additionally, Hatsushika et al. reported that repeated intra-articular syMSCs injections were able to stimulate healing of meniscal defects in a porcine in vivo model and used T2 MRI mapping to evaluate their results (Hatsushika et al., 2014). Similarly, Lucidi et al. evaluated the long-term results of medial collagen meniscal implants in human patients approximately 20 years after surgery by performing MRI scans on their knees and analyzing the results using the Yulish scoring system, which takes into account the detection of cartilage and subchondral bone elements (Lucidi et al., 2021). They found that four of the eight patients who underwent surgery developed mild OA symptoms. These studies highlight the importance of long-term longitudinal radiographic evaluation of the knee over the years in patients to evaluate the possible onset and progression of OA.
Assessment of Meniscal Mechanical Properties Following Repair
The measurement of meniscal strength and the characterization of its mechanical compressive properties are used as a means of assessing tissue integrity. Tensile loading (to failure) is a commonly utilized method for testing tensile repair strength (Zellner et al., 2013; Nakagawa et al., 2015; Peloquin et al., 2016; Newberry et al., 2020); indentation testing is used to determine changes in tissue compression (Shen et al., 2013) (Pan et al., 2017), and pushout testing is used to evaluate the extent of shear strength due to integrative repair (Hennerbichler et al., 2007; Wilusz et al., 2008; Newberry et al., 2020). Additionally, detection of changes in the contact forces on tibial femoral articular cartilage can be used as an outcome measure of meniscal injury repair/treatment success. The most common methodologies for achieving this end include the use of MRI and CT mapping (Flanigan et al., 2010). Direct pressure mapping technologies are also commonly used to evaluate the effects of changes in knee forces following non-surgical or surgical treatment to the meniscus (Hennerbichler et al., 2007; Flanigan et al., 2010). In addition, these approaches are crucial to evaluate the effect on the cartilage surface followed by treatment when suturing or partial meniscectomy is performed.
Assessment of Biomarkers
The quantification of protein biomarkers, as a means of detecting specific types of meniscal injuries, has more recently become an area of active investigation with the popularization of sophisticated multiplexing technologies such as Luminex, which allow nanoscale detection of robust sets of soluble protein markers. A meniscus injury is linked to the development of PTOA (Kennedy et al., 2020; Sherman et al., 2020; Tarafder et al., 2020; Adams et al., 2021). As such, the events that occur after traumatic injury or degenerative changes in the meniscus that govern the progression of OA are regulated at the molecular level. For instance, following joint injury, several inflammatory proteins (such as interleukins, TNF-alpha, Prostaglandin E2), collagenases/aggrecanases (such as matrix metalloproteinases, ADAMTS family members), and reactive oxygen species are produced (Roberts et al., 2015; Lepetsos and Papavassiliou, 2016; Roller et al., 2016; Liu et al., 2017; Clair et al., 2019). Collagenases are involved in the metabolism of cartilage and subchondral bones, and they are considered candidate biomarkers for pathological tissue remodeling upon meniscus injury or osteoarthritis (Karsdal et al., 2011; Karsdal et al., 2013). Clair et al., observed a significant upregulation of IL-6, MCP-1, MIP-1β, and MMP-13 in the synovial fluid of the patients with meniscal injury (Clair et al., 2019). These studies reveal that a range of biomarkers is modulated in the synovial fluid as well as in the serum upon injury to the meniscus. A recent study by Brophy et al., has also demonstrated that gene expression analysis of meniscal tissue obtained during arthroscopic meniscal debridement procedures may be used to predict degenerative changes in the cartilage (Brophy et al., 2017). This study reported a differential expression of the adipokines, adiponectin and resistin in meniscal tissue from knees with degenerative changes, suggesting a potential causal relationship. This study highlights the importance of biological signaling in the meniscus for maintaining articular cartilage health.
Overall, the quantification of PTOA biomarkers in the blood and synovial fluid provide a fundamental advantage over more invasive approaches, such as histopathology analysis, while also providing an additional advantage of enabling frequent analysis at multiple time points in the same subject. Analyzing synovial fluid (SF) biomarker profiles can also have advantages since SF is drawn directly from the injury micro-environment. Additionally, due to the small volume of synovial fluid, biomarkers are less diluted as compared to those dispersed into the peripheral blood.
Functional Assessment of the Knee
Lastly, the implication on meniscus repair/replacement on longitudinal knee function is used to gauge overall efficacy in pre-clinical animal models, including both small animals such as rodents and guinea pigs and large animal models like pigs, dogs, and horses. Accurate assessment of knee function is also crucial to make informed treatment decisions, as well as to monitor debilitating effects associated with traumatic joint injury (Ericsson et al., 2006; Bremander et al., 2007; Katz et al., 2013). Gait analysis is one of most commonly used methods to evaluate knee function in the patients with meniscus injury (Bulgheroni et al., 2007; Hyodo et al., 2020; Liu et al., 2020). Similarly, unilateral and bilateral gait analysis is a mainstay in pre-clinical studies investigating the functional implication of meniscus/ACL injury and repair (Jacobs et al., 2014; Karamchedu et al., 2021). Asymmetric gait patterns are typically indicative of potential functional impairment due to the change in the normal range of motion.
Assessment of Knee Histopathology for Signs of OA
Pre-clinical meniscus repair/replacement studies have used knee histopathology as a secondary outcome measure of success (Table 1). However, the effect of meniscus repair/replacement on the pathology of the entire knee is a crucial metric and should arguably be considered a primary outcome measure when assessing the success of treatments. Assessment of the degree of articular cartilage degradation, synovial hyperplasia, mast cell infiltration, and changes in subchondral bone are all features that can be qualitatively and/or semi-quantitatively detected by histopathology analysis of tissue sections using the same staining techniques described previously (i.e., routine/special/immunostaining) for assessing meniscus histopathology.
Emerging Pre-Clinical Meniscal Repair/Replacement Strategies and Their Impact on Knee Function and Pathology
Partial meniscal resection can be an effective short-term treatment for symptomatic complex meniscus tears; however, sizeable evidence shows that this approach leads to the accelerated development of OA. Similarly, MAT has its own set of challenges which were discussed above. Therefore, novel therapeutic approaches are being developed in the pre-clinical models to find effective alternative therapies. These approaches largely include the use of synthetic/biological scaffolds and/or autologous/allogeneic cells. In order to conduct a comprehensive evaluation of the efficacy of such emerging treatments, it is necessary to assess not only the repaired/replaced meniscus itself but also other important metrics that have been discussed in this article that ultimately help to assess knee functionality and/or pathology as fundamental indirect/secondary criteria. These secondary measures can help define the level of success achieved by said treatments.
In 2015, Nakagawa et al. (Nakagawa et al., 2015) performed a study in a porcine model in which they combined suture repair with allogenic MSCs enriched from synovial fluid. The study reported positive outcomes in meniscal histology, collagen deposition and tensile strength in the animal groups that received the combination treatment as compared to those that received only suture repairs (Nakagawa et al., 2015). Hatsushika et al. (Hatsushika et al., 2014), performed similar studies with larger meniscus tears and involved multiple injections of the synovium-derived MSCs. Their results supported the previous finding by noting that multiple injections is advantageous compared to a single injection (Hatsushika et al., 2014). Both studies involved acute injections immediately after the injury. Further studies in which the animals were treated after 3–4 weeks of injury demonstrated that the animal groups that are acutely treated show significantly better repair compared to those that are treated 3–4 weeks post-injury (Ruiz-Ibán et al., 2011). These studies suggest that both the chronology of the injury and the treatment is vital for meniscus injury repair. Inspection of cartilage health and/or knee function would have been most informative regarding the efficacy of this cell-based biologic treatment approach.
There are, however, many recent studies conducted in multiple animal models that have evaluated outcome measures of PTOA as important parameters of success (Table 1). For instance, Wei et al. (Wei et al., 2021) identified a novel progenitor cell population located in the horns of the meniscus that not only helps meniscus repair but also prevents cartilage degradation. The study identified Hedgehog (Hh) signaling as a crucial player in progenitor cell migration to the injury site and the inhibition of cartilage degradation. Further, they used microCT analysis to reveal that the use of Hh agonist does not induce calcification. In another study, Ding et al. (Ding, 2021) demonstrated that the intra-articular injection of BM-MSCs pretreated with c-Jun N-terminal kinase (JNK) inhibitor SP600125 in C57BL/6 mice significantly induced meniscus repair and inhibited cartilage degradation in comparison to the animals that received BM-MSCs without SP600125 pretreatment. Immunohistochemical analysis revealed that the pretreatment of SP600125 inhibits the inflammation on the articular cartilage surface suggesting SP600125 as a potential therapeutic candidate to inhibit the cartilage degradation and OA progression (Ding, 2021). Ozeki et al. (Ozeki et al., 2021) developed a meniscus injury model in micro minipigs and investigated the reparative effects of synovial MSCs on meniscus injury repair. They used cartilage surface degradation as an outcome measure. Histological evaluation and MRI analysis indicated that synovial MSCs improved meniscus healing and also protected the articular cartilage surface from degradation as compared to the controls (Ozeki et al., 2021).
Further, in a recent study by Shimomura et al. (Shimomura et al., 2019), the investigators used an MSC monolayer sheet wrapped around an electrospun nanofiber scaffold to treat a radial meniscal injury in rabbits. In addition to evaluating the meniscus, they conducted a histological evaluation of the articular cartilage and showed that there was significantly less degradation in the cell scaffold treated animals, compared to the control animals. Zhong et al. (Zhong et al., 2020) utilized a rat model of meniscus injury-induced osteoarthritis to investigate the efficacy of an injectable extracellular matrix (ECM) hydrogel on meniscus repair and OA development. Radiographic evaluation using micro-CT scans revealed that the ECM hampers osteophyte formation, prevents narrowing of the joint space, and inhibits OA development. Table 1 is a comprehensive list of meniscus repair/replacement studies in live animal models that were published and referenced on PubMed in the past 3-years. Table 1 summarizes the outcomes and assessment techniques used to evaluate the meniscus itself, knee function, and knee health. Figure 1 illustrates the distribution of these recent studies based on the implemented therapeutic techniques and their efficacy in the assessment of PTOA outcomes.
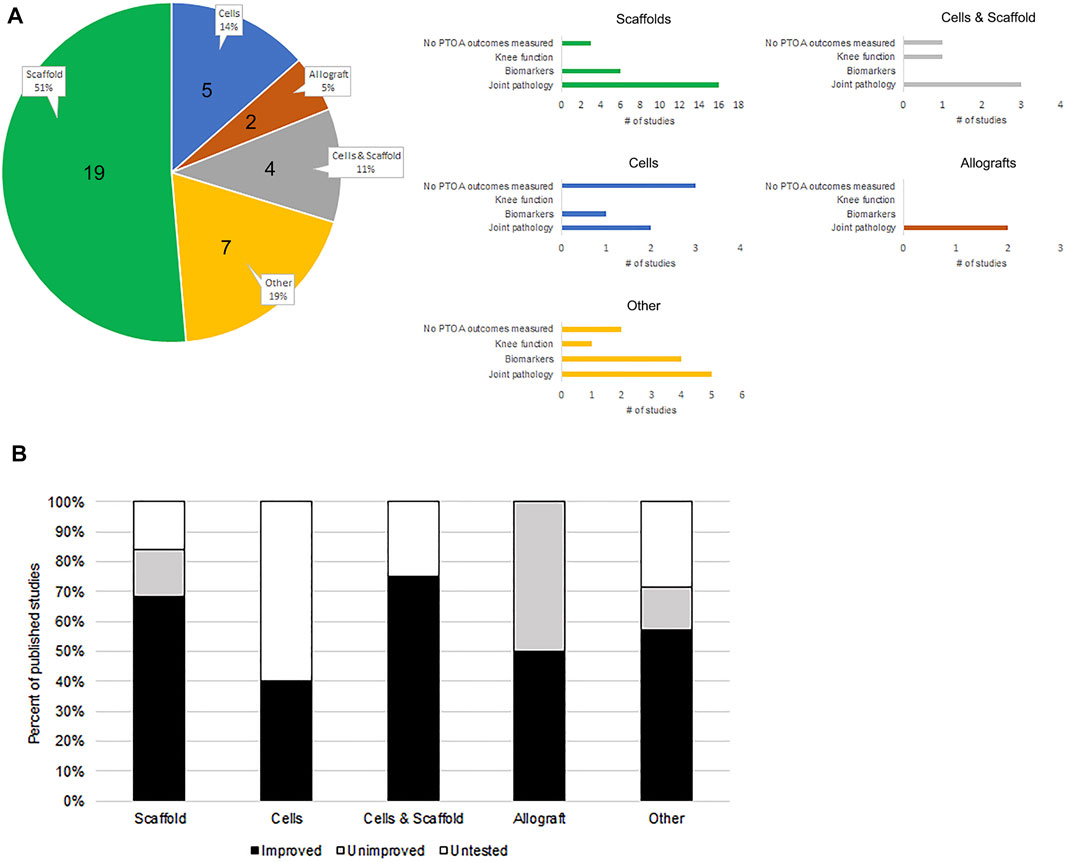
FIGURE 1. Recent pre-clinical meniscus treatment studies in animal models and their PTOA attenuation success rate. (A) The 37 studies indexed in Table 1 were used to extrapolate the distribution of published investigations that utilize different strategies for meniscus injury treatment. Studies have been categorized based on their use of cells, scaffolds, cell and scaffold combination, allografts, or altogether different (other) strategies, by a percentage of all indexed studies. The number of studies is indicated in the pie chart. These categories are further broken down (see respective bar graphs) based on secondary outcome measures used to evaluate therapeutic success, including knee function, mRNA/protein biomarker analysis, and joint pathology detection. Note that some studies have performed more than one type of analysis. (B) Therapy categories were further broken down based on percent distribution of studies in each that reported knee PTOA outcomes.
Besides cell/scaffold-based pre-clinical investigations of meniscus injury treatment, other less common approaches have also been explored. Some studies that use these approaches also include the additional evaluation of outcome measures that do not directly address the health of the meniscus, but rather the health of the surrounding tissue. For example, Shen et al., recently identified a circulating RNA, circPDE4B, as a potential target for the prevention of cartilage degradation and OA progression upon meniscus injury in mice. Histological evaluation of the mouse knee suggested that adeno-associated virus mediated delivery of circPDE4B inhibits the cartilage matrix breakdown, which was otherwise observed in the control mice knees. Fu et al. (Fu et al., 2020) investigated the actions of Erythropoietin (EPO) in meniscus organ culture and reported the mechanistic details of its effects on meniscus regeneration. Their histological analysis using Alcian Blue and Orange G staining revealed that the EPO treatment significantly reduced the degradation of the cartilage surface and ultimately attenuated OA development. Another recent study by Duan et al. (Duan et al., 2021) underscored the crucial role of extracellular vesicles (EVs) in OA progression upon meniscus injury. Through extensive in vitro and in vivo characterization, the authors revealed that EVs secreted by lipopolysaccharide enriched synovial MSCs inhibit aggrecan and collagen 2 reduction and attenuate the upregulation of IL-1β responsive ADAMTS5. They used histological analysis to reveal that the EVs significantly prevented joint degeneration in a mouse model of OA.
Conclusion
The detection of PTOA development in response to meniscus injury can be achieved using metrics designed to evaluate knee function, its inflammatory microenvironment (through biomarker detection), and the histopathology of tissues in the joint. Our analysis of recent scientific literature shows that 84% of studies involving the use of meniscus scaffolds and 100% of studies using allografts for meniscus injury treatment have examined at least a single outcome measure that reveals the effect on overall knee function/health to help gauge treatment success. On the other hand, only 40% of studies that use cells, and 71% of studies involving non-conventional (other) treatment approaches, have done the same. Altogether, we have found that 75% of all recent studies catalogued in our search have examined at least a single outcome assessment that is relevant to PTOA, as part of the analysis. Approximately 84% of these studies have reported an improved outcome due to treatment.
Author Contributions
JT: Composed most of the manuscript, assembled table and figures, reviewed and revised manuscript. DB: Composed components of the manuscript, reviewed and revised manuscript. SD: Composed components of the manuscript, reviewed and revised manuscript. CJ: Conceptualized review, composed components of the manuscript, reviewed and revised manuscript.
Conflict of Interest
The authors declare that the research was conducted in the absence of any commercial or financial relationships that could be construed as a potential conflict of interest.
Publisher’s Note
All claims expressed in this article are solely those of the authors and do not necessarily represent those of their affiliated organizations, or those of the publisher, the editors and the reviewers. Any product that may be evaluated in this article, or claim that may be made by its manufacturer, is not guaranteed or endorsed by the publisher.
References
Abpeikar, Z., Javdani, M., Mirzaei, S. A., Alizadeh, A., Moradi, L., Soleimannejad, M., et al. (2021). Macroporous Scaffold Surface Modified with Biological Macromolecules and Piroxicam-Loaded Gelatin Nanofibers toward Meniscus Cartilage Repair. Int. J. Biol. Macromolecules 183, 1327–1345. doi:10.1016/j.ijbiomac.2021.04.151
Adams, B. G., Houston, M. N., and Cameron, K. L. (2021). The Epidemiology of Meniscus Injury. Sports Med. Arthrosc. Rev. 29 (3), e24–e33. doi:10.1097/jsa.0000000000000329
Allen, P., Denham, R., and Swan, A. (1984). Late Degenerative Changes after Meniscectomy. Factors Affecting the Knee after Operation. The J. Bone Jt. Surg. Br. volume 66-B (5), 666–671. doi:10.1302/0301-620x.66b5.6548755
Andrews, S., Shrive, N., and Ronsky, J. (2011). The Shocking Truth about Meniscus. J. Biomech. 44 (16), 2737–2740. doi:10.1016/j.jbiomech.2011.08.026
Athanasiou, K. A., and Sanchez-Adams, J. (2009). Engineering The Knee Meniscus. Synthesis Lectures Tissue Eng 1, 1–97. doi:10.2200/S00186ED1V01Y200903TIS001
Badlani, J. T., Borrero, C., Golla, S., Harner, C. D., and Irrgang, J. J. (2013). The Effects of Meniscus Injury on the Development of Knee Osteoarthritis. Am. J. Sports Med. 41 (6), 1238–1244. doi:10.1177/0363546513490276
Bedrin, M. D., Kartalias, K., Yow, B. G., and Dickens, J. F. (2021). Degenerative Joint Disease after Meniscectomy. Sports Med. Arthrosc. Rev. 29 (3), e44–e50. doi:10.1097/jsa.0000000000000301
Benedetto, K. P., and Rangger, C. (1993). Arthroscopic Partial Meniscectomy: 5-year Follow-Up. Knee Surg. Sports Traumatol. Arthrosc. 1 (3-4), 235–238. doi:10.1007/BF01560216
Berton, A., Longo, U. G., Candela, V., Greco, F., Martina, F. M., Quattrocchi, C. C., et al. (2020). Quantitative Evaluation of Meniscal Healing Process of Degenerative Meniscus Lesions Treated with Hyaluronic Acid: A Clinical and MRI Study. J. Clin. Med. 9 (7). doi:10.3390/jcm9072280
Bremander, A. B., Dahl, L. L., and Roos, E. M. (2007). Validity and Reliability of Functional Performance Tests in Meniscectomized Patients with or without Knee Osteoarthritis. Scand. J. Med. Sci. Sports 17 (2), 120–127. doi:10.1111/j.1600-0838.2006.00544.x
Brophy, R. H., Sandell, L. J., Cheverud, J. M., and Rai, M. F. (2017). Gene Expression in Human Meniscal Tears Has Limited Association with Early Degenerative Changes in Knee Articular Cartilage. Connect. Tissue Res. 58 (3-4), 295–304. doi:10.1080/03008207.2016.1211114
Bryceland, J. K., Powell, A. J., and Nunn, T. (2017). Knee Menisci. Cartilage 8 (2), 99–104. doi:10.1177/1947603516654945
Bulgheroni, P., Bulgheroni, M. V., Ronga, M., and Manelli, A. (2007). Gait Analysis of Pre- and post-meniscectomy Knee: a Prospective Study. The Knee 14 (6), 472–477. doi:10.1016/j.knee.2007.08.003
Chang, P. S., and Brophy, R. H. (2020). As Goes the Meniscus Goes the Knee. Clin. Sports Med. 39 (1), 29–36. doi:10.1016/j.csm.2019.08.001
Chen, C., Song, J., Qiu, J., and Zhao, J. (2020). Repair of a Meniscal Defect in a Rabbit Model through Use of a Thermosensitive, Injectable, In Situ Crosslinked Hydrogel with Encapsulated Bone Mesenchymal Stromal Cells and Transforming Growth Factor β1. Am. J. Sports Med. 48 (4), 884–894. doi:10.1177/0363546519898519
Chen, M., Feng, Z., Guo, W., Yang, D., Gao, S., Li, Y., et al. (2019). PCL-MECM-Based Hydrogel Hybrid Scaffolds and Meniscal Fibrochondrocytes Promote Whole Meniscus Regeneration in a Rabbit Meniscectomy Model. ACS Appl. Mater. Inter. 11 (44), 41626–41639. doi:10.1021/acsami.9b13611
Chivers, M. D., and Howitt, S. D. (2009). Anatomy and Physical Examination of the Knee Menisci: a Narrative Review of the Orthopedic Literature. J. Can. Chiropr Assoc. 53 (4), 319–333.
Clair, A. J., Kingery, M. T., Anil, U., Kenny, L., Kirsch, T., and Strauss, E. J. (2019). Alterations in Synovial Fluid Biomarker Levels in Knees with Meniscal Injury as Compared with Asymptomatic Contralateral Knees. Am. J. Sports Med. 47 (4), 847–856. doi:10.1177/0363546519825498
Cojocaru, D. G., Hondke, S., Krüger, J. P., Bosch, C., Croicu, C., Florescu, S., et al. (2020). Meniscus‐shaped Cell‐free Polyglycolic Acid Scaffold for Meniscal Repair in a Sheep Model. J. Biomed. Mater. Res. 108 (3), 809–818. doi:10.1002/jbm.b.34435
Darabos, N., Dovzak-Bajs, I., Bilić, V., Darabos, A., Popović, I., and Cengić, T. (2012). All-inside Arthroscopic Suturing Technique for Meniscal Ruptures. Acta Clin. Croat. 51 (1), 51–54.
Demirkıran, N. D., Havıtçıoğlu, H., Ziylan, A., Cankurt, Ü., and Hüsemoğlu, B. (2019). Novel Multilayer Meniscal Scaffold Provides Biomechanical and Histological Results Comparable to Polyurethane Scaffolds: An 8 Week Rabbit Study. Acta Orthop. Traumatol. Turc 53 (2), 120–128. doi:10.1016/j.aott.2019.02.004
Ding, N. (2021). SP600125 Restored Tumor Necrosis Factor-α-Induced Impaired Chondrogenesis in Bone Mesenchymal Stem Cells and its Anti-osteoarthritis Effect in Mice. Stem Cell Dev 30, 1028–1036. doi:10.1089/scd.2021.0146
Duan, A., Shen, K., Li, B., Li, C., Zhou, H., Kong, R., et al. (2021). Extracellular Vesicles Derived from LPS-Preconditioned Human Synovial Mesenchymal Stem Cells Inhibit Extracellular Matrix Degradation and Prevent Osteoarthritis of the Knee in a Mouse Model. Stem Cel Res Ther 12 (1), 427. doi:10.1186/s13287-021-02507-2
Erdemir, A. (2016). Open Knee: Open Source Modeling and Simulation in Knee Biomechanics. J. Knee Surg. 29 (2), 107–116. doi:10.1055/s-0035-1564600
Ericsson, Y. B., Roos, E. M., and Dahlberg, L. (2006). Muscle Strength, Functional Performance, and Self-Reported Outcomes Four Years after Arthroscopic Partial Meniscectomy in Middle-Aged Patients. Arthritis Rheum. 55 (6), 946–952. doi:10.1002/art.22346
Fan, C., Xu, Z., Wu, T., Cui, C., Liu, Y., Liu, B., et al. (2021). 3D Printing of Lubricative Stiff Supramolecular Polymer Hydrogels for Meniscus Replacement. Biomater. Sci. 9 (15), 5116–5126. doi:10.1039/d1bm00836f
Faunø, P., and Nielsen, A. B. (1992). Arthroscopic Partial Meniscectomy: a Long-Term Follow-Up. Arthroscopy 8 (3), 345–349.
Flanigan, D. C., Lin, F., Koh, J. L., and Zhang, L. Q. (2010). Articular Contact Pressures of Meniscal Repair Techniques at Various Knee Flexion Angles. Orthopedics 33 (7), 475. doi:10.3928/01477447-20100526-04
Fox, A. J. S., Wanivenhaus, F., Burge, A. J., Warren, R. F., and Rodeo, S. A. (2015). The Human Meniscus: a Review of Anatomy, Function, Injury, and Advances in Treatment. Clin. Anat. 28 (2), 269–287. doi:10.1002/ca.22456
Fu, X. N., Li, H. W., Du, N., Liang, X., He, S. H., Guo, K. J., et al. (2020). Erythropoietin Enhances Meniscal Regeneration and Prevents Osteoarthritis Formation in Mice. Am. J. Transl Res. 12 (10), 6464–6477.
Gee, S. M., Tennent, D. J., Cameron, K. L., and Posner, M. A. (2020). The Burden of Meniscus Injury in Young and Physically Active Populations. Clin. Sports Med. 39 (1), 13–27. doi:10.1016/j.csm.2019.08.008
Ghodbane, S. A., Brzezinski, A., Patel, J. M., Plaff, W. H., Marzano, K. N., Gatt, C. J., et al. (2019). Partial Meniscus Replacement with a Collagen-Hyaluronan Infused Three-Dimensional Printed Polymeric Scaffold. Tissue Eng. Part. A. 25 (5-6), 379–389. doi:10.1089/ten.TEA.2018.0160
Guo, W., Chen, M., Wang, Z., Tian, Y., Zheng, J., Gao, S., et al. (2021). 3D-printed Cell-free PCL-MECM Scaffold with Biomimetic Micro-structure and Micro-environment to Enhance In Situ Meniscus Regeneration. Bioactive Mater. 6 (10), 3620–3633. doi:10.1016/j.bioactmat.2021.02.019
Hagmeijer, M. H., Kennedy, N. I., Tagliero, A. J., Levy, B. A., Stuart, M. J., Saris, D. B. F., et al. (2019). Long-term Results after Repair of Isolated Meniscal Tears Among Patients Aged 18 Years and Younger: An 18-Year Follow-Up Study. Am. J. Sports Med. 47 (4), 799–806. doi:10.1177/0363546519826088
Hatsushika, D., Muneta, T., Nakamura, T., Horie, M., Koga, H., Nakagawa, Y., et al. (2014). Repetitive Allogeneic Intraarticular Injections of Synovial Mesenchymal Stem Cells Promote Meniscus Regeneration in a Porcine Massive Meniscus Defect Model. Osteoarthritis and Cartilage 22 (7), 941–950. doi:10.1016/j.joca.2014.04.028
Hede, A., Larsen, E., and Sandberg, H. (1992). The Long Term Outcome of Open Total and Partial Meniscectomy Related to the Quantity and Site of the Meniscus Removed. Int. Orthopaedics 16 (2), 122–125. doi:10.1007/bf00180200
Hennerbichler, A., Moutos, F. T., Hennerbichler, D., Weinberg, J. B., and Guilak, F. (2007). Interleukin-1 and Tumor Necrosis Factor Alpha Inhibit Repair of the Porcine Meniscus In Vitro. Osteoarthritis and Cartilage 15 (9), 1053–1060. doi:10.1016/j.joca.2007.03.003
Houck, D. A., Kraeutler, M. J., Belk, J. W., McCarty, E. C., and Bravman, J. T. (2018). Similar Clinical Outcomes Following Collagen or Polyurethane Meniscal Scaffold Implantation: a Systematic Review. Knee Surg. Sports Traumatol. Arthrosc. 26 (8), 2259–2269. doi:10.1007/s00167-018-4838-1
Hyodo, K., Kanamori, A., Kadone, H., Takahashi, T., Kajiwara, M., and Yamazaki, M. (2020). Gait Analysis Comparing Kinematic, Kinetic, and Muscle Activation Data of Modern and Conventional Total Knee Arthroplasty. Arthroplasty Today 6 (3), 338–342. doi:10.1016/j.artd.2020.03.011
Jacobs, B. Y., Kloefkorn, H. E., and Allen, K. D. (2014). Gait Analysis Methods for Rodent Models of Osteoarthritis. Curr. Pain Headache Rep. 18 (10), 456. doi:10.1007/s11916-014-0456-x
Jørgensen, U., Sonne-Holm, S., Lauridsen, F., and Rosenklint, A. (1987). Long-term Follow-Up of Meniscectomy in Athletes. A Prospective Longitudinal Study. J. Bone Jt. Surg Br 69 (1), 80–83. doi:10.1302/0301-620X.69B1.3818740
Karamchedu, N. P., Murray, M. M., Sieker, J. T., Proffen, B. L., Portilla, G., Costa, M. Q., et al. (2021). Bridge-Enhanced Anterior Cruciate Ligament Repair Leads to Greater Limb Asymmetry and Less Cartilage Damage Than Untreated ACL Transection or ACL Reconstruction in the Porcine Model. Am. J. Sports Med. 49 (3), 667–674. doi:10.1177/0363546521989265
Karsdal, M. A., Nielsen, M. J., Sand, J. M., Henriksen, K., Genovese, F., Bay-Jensen, A.-C., et al. (2013). Extracellular Matrix Remodeling: The Common Denominator in Connective Tissue DiseasesPossibilities for Evaluation and Current Understanding of the Matrix as More Than a Passive Architecture, but a Key Player in Tissue Failure. ASSAY Drug Development Tech. 11 (2), 70–92. doi:10.1089/adt.2012.474
Karsdal, M. A., Woodworth, T., Henriksen, K., Maksymowych, W. P., Genant, H., Vergnaud, P., et al. (2011). Biochemical Markers of Ongoing Joint Damage in Rheumatoid Arthritis - Current and Future Applications, Limitations and Opportunities. Arthritis Res. Ther. 13 (2), 215. doi:10.1186/ar3280
Katz, J. N., Brophy, R. H., Chaisson, C. E., de Chaves, L., Cole, B. J., Dahm, D. L., et al. (2013). Surgery versus Physical Therapy for a Meniscal Tear and Osteoarthritis. N. Engl. J. Med. 368 (18), 1675–1684. doi:10.1056/NEJMoa1301408
Kennedy, M. I., Strauss, M., and LaPrade, R. F. (2020). Injury of the Meniscus Root. Clin. Sports Med. 39 (1), 57–68. doi:10.1016/j.csm.2019.08.009
Kim, J.-A., An, Y.-H., Yim, H.-G., Han, W.-J., Park, Y.-B., Park, H. J., et al. (2021). Injectable Fibrin/Polyethylene Oxide Semi-IPN Hydrogel for a Segmental Meniscal Defect Regeneration. Am. J. Sports Med. 49 (6), 1538–1550. doi:10.1177/0363546521998021
Koch, M., Hammer, S., Fuellerer, J., Lang, S., Pfeifer, C. G., Pattappa, G., et al. (2019). Bone Marrow Aspirate Concentrate for the Treatment of Avascular Meniscus Tears in a One-step Procedure-Evaluation of an In Vivo Model. Int. J. Mol. Sci. 20 (5). doi:10.3390/ijms20051120
Koff, M. F., Shah, P., Pownder, S., Romero, B., Williams, R., Gilbert, S., et al. (2013). Correlation of Meniscal T2* with Multiphoton Microscopy, and Change of Articular Cartilage T2 in an Ovine Model of Meniscal Repair. Osteoarthritis and Cartilage 21 (8), 1083–1091. doi:10.1016/j.joca.2013.04.020
Kondo, S., Muneta, T., Nakagawa, Y., Koga, H., Watanabe, T., Tsuji, K., et al. (2017). Transplantation of Autologous Synovial Mesenchymal Stem Cells Promotes Meniscus Regeneration in Aged Primates. J. Orthop. Res. 35 (6), 1274–1282. doi:10.1002/jor.23211
Korpershoek, J. V., de Windt, T. S., Hagmeijer, M. H., Vonk, L. A., and Saris, D. B. (2017). Cell-Based Meniscus Repair and Regeneration: At the Brink of Clinical Translation?: A Systematic Review of Preclinical Studies. Orthop. J. Sports Med. 5 (2), 2325967117690131. doi:10.1177/2325967117690131
Kurnaz, R., Balta, O., and Balta, O. (2020). Effect of Platelet-Rich Plasma and Platelet-Rich Fibrin Matrix on Healing of Vertical Meniscal Tears in a Rabbit Model. Aott 54 (2), 186–195. doi:10.5152/j.aott.2020.02.20
Lee, S. R., Kim, J. G., and Nam, S. W. (2012). The Tips and Pitfalls of Meniscus Allograft Transplantation. Knee Surg. Relat. Res. 24 (3), 137–145. doi:10.5792/ksrr.2012.24.3.137
Lepetsos, P., and Papavassiliou, A. G. (2016). ROS/oxidative Stress Signaling in Osteoarthritis. Biochim. Biophys. Acta (Bba) - Mol. Basis Dis. 1862 (4), 576–591. doi:10.1016/j.bbadis.2016.01.003
Li, Y., Chen, M., Yan, J., Zhou, W., Gao, S., Liu, S., et al. (2021). Tannic acid/Sr2+-Coated Silk/graphene Oxide-Based Meniscus Scaffold with Anti-inflammatory and Anti-ROS Functions for Cartilage protection and Delaying Osteoarthritis. Acta Biomater. 126, 119–131. doi:10.1016/j.actbio.2021.02.046
Li, Y., Chen, M., Zhou, W., Gao, S., Luo, X., Peng, L., et al. (2020). Cell-free 3D Wet-Electrospun PCL/silk fibroin/Sr2+ Scaffold Promotes Successful Total Meniscus Regeneration in a Rabbit Model. Acta Biomater. 113, 196–209. doi:10.1016/j.actbio.2020.06.017
Li, Z., Wu, N., Cheng, J., Sun, M., Yang, P., Zhao, F., et al. (2020). Biomechanically, Structurally and Functionally Meticulously Tailored Polycaprolactone/silk Fibroin Scaffold for Meniscus Regeneration. Theranostics 10 (11), 5090–5106. doi:10.7150/thno.44270
Liu, B., Goode, A. P., Carter, T. E., Utturkar, G. M., Huebner, J. L., Taylor, D. C., et al. (2017). Matrix Metalloproteinase Activity and Prostaglandin E2 Are Elevated in the Synovial Fluid of Meniscus Tear Patients. Connect. Tissue Res. 58 (3-4), 305–316. doi:10.1080/03008207.2016.1256391
Liu, F., Xu, H., and Huang, H. (2019). A Novel Kartogenin-Platelet-Rich Plasma Gel Enhances Chondrogenesis of Bone Marrow Mesenchymal Stem Cells In Vitro and Promotes Wounded Meniscus Healing In Vivo. Stem Cel Res Ther 10 (1), 201. doi:10.1186/s13287-019-1314-x
Liu, X., Huang, H., Ren, S., Rong, Q., and Ao, Y. (2020). Use of the Normalcy index for the Assessment of Abnormal Gait in the Anterior Cruciate Ligament Deficiency Combined with Meniscus Injury. Computer Methods Biomech. Biomed. Eng. 23 (14), 1102–1108. doi:10.1080/10255842.2020.1789119
Longo, U. G., Ciuffreda, M., Candela, V., Rizzello, G., D'Andrea, V., Mannering, N., et al. (2019). Knee Osteoarthritis after Arthroscopic Partial Meniscectomy: Prevalence and Progression of Radiographic Changes after 5 to 12 Years Compared with Contralateral Knee. J. Knee Surg. 32 (5), 407–413. doi:10.1055/s-0038-1646926
López-Franco, M., López-Franco, O., Murciano-Antón, M. A., Cañamero-Vaquero, M., Fernández-Aceñero, M. J., Herrero-Beaumont, G., et al. (2016). Meniscal Degeneration in Human Knee Osteoarthritis: In Situ Hybridization and Immunohistochemistry Study. Arch. Orthopaedic Trauma Surg. 136 (2), 175–183. doi:10.1007/s00402-015-2378-4
Lucidi, G. A., Grassi, A., Al-Zu'bi, B. B. H., Macchiarola, L., Agostinone, P., Marcacci, M., et al. (2021). Satisfactory Clinical Results and Low Failure Rate of Medial Collagen Meniscus Implant (CMI) at a Minimum 20 Years of Follow-Up. Knee Surg. Sports Traumatol. Arthrosc. 29 (12), 4270–4277. doi:10.1007/s00167-021-06556-1
Majewski, M., Susanne, H., and Klaus, S. (2006). Epidemiology of Athletic Knee Injuries: A 10-year Study. The Knee 13 (3), 184–188. doi:10.1016/j.knee.2006.01.005
Makris, E. A., Hadidi, P., and Athanasiou, K. A. (2011). The Knee Meniscus: Structure-Function, Pathophysiology, Current Repair Techniques, and Prospects for Regeneration. Biomaterials 32 (30), 7411–7431. doi:10.1016/j.biomaterials.2011.06.037
McDermott, I. D., and Amis, A. A. (2006). The Consequences of Meniscectomy. J. Bone Jt. Surg. Br. volume 88-B (12), 1549–1556. doi:10.1302/0301-620x.88b12.18140
McDermott, I. D., Sharifi, F., Bull, A. M. J., Gupte, C. M., Thomas, R. W., and Amis, A. A. (2004). An Anatomical Study of Meniscal Allograft Sizing. Knee Surg. Sports Traumatol. Arthrosc. 12 (2), 130–135. doi:10.1007/s00167-003-0366-7
McNulty, A. L., and Guilak, F. (2015). Mechanobiology of the Meniscus. J. Biomech. 48 (8), 1469–1478. doi:10.1016/j.jbiomech.2015.02.008
Milachowski, K. A., Weismeier, K., and Wirth, C. J. (1989). Homologous Meniscus Transplantation. Experimental and Clinical Results. Int. Orthop. 13 (1), 1–11. doi:10.1007/BF00266715
Nakagawa, Y., Fortier, L. A., Mao, J. J., Lee, C. H., Goodale, M. B., Koff, M. F., et al. (2019). Long-term Evaluation of Meniscal Tissue Formation in 3-Dimensional-Printed Scaffolds with Sequential Release of Connective Tissue Growth Factor and TGF-Β3 in an Ovine Model. Am. J. Sports Med. 47 (11), 2596–2607. doi:10.1177/0363546519865513
Nakagawa, Y., Muneta, T., Kondo, S., Mizuno, M., Takakuda, K., Ichinose, S., et al. (2015). Synovial Mesenchymal Stem Cells Promote Healing after Meniscal Repair in Microminipigs. Osteoarthritis and Cartilage 23 (6), 1007–1017. doi:10.1016/j.joca.2015.02.008
Nakagawa, Y., Sekiya, I., Kondo, S., Tabuchi, T., Ichinose, S., Koga, H., et al. (2016). Relationship between MRIT1rho Value and Histological Findings of Intact and Radially Incised Menisci in Microminipigs. J. Magn. Reson. Imaging 43 (2), 434–445. doi:10.1002/jmri.24988
Narez, G. E., Brown, G., Herrick, A., Ek, R. J., Dejardin, L., Wei, F., et al. (2021). Assessment of Changes in the Meniscus and Subchondral Bone in a Novel Closed-Joint Impact and Surgical Reconstruction Lapine Model. J. Biomech. 126, 110630. doi:10.1016/j.jbiomech.2021.110630
Newberry, J., Desai, S., Adler, C., Li, N., Karamchedu, N. P., Fleming, B. C., et al. (2020). SDF-1 Preconditioned HPC Scaffolds Mobilize Cartilage-Derived Progenitors and Stimulate Meniscal Fibrocartilage Repair in Human Explant Tissue Culture. Connect. Tissue Res. 61 (3-4), 338–348. doi:10.1080/03008207.2019.1689966
Nishida, Y., Hashimoto, Y., Orita, K., Nishino, K., Kinoshita, T., and Nakamura, H. (2020). Intra-Articular Injection of Stromal Cell-Derived Factor 1α Promotes Meniscal Healing via Macrophage and Mesenchymal Stem Cell Accumulation in a Rat Meniscal Defect Model. Int. J. Mol. Sci. 21 (15). doi:10.3390/ijms21155454
Okuno, N., Otsuki, S., Aoyama, J., Nakagawa, K., Murakami, T., Ikeda, K., et al. (2021). Feasibility of a Self‐assembling Peptide Hydrogel Scaffold for Meniscal Defect: An In Vivo Study in a Rabbit Model. J. Orthop. Res. 39 (1), 165–176. doi:10.1002/jor.24841
Osawa, A., Harner, C. D., Gharaibeh, B., Matsumoto, T., Mifune, Y., Kopf, S., et al. (2013). The Use of Blood Vessel-Derived Stem Cells for Meniscal Regeneration and Repair. Med. Sci. Sports Exerc. 45 (5), 813–823. doi:10.1249/mss.0b013e31827d1e06
Otsuki, S., Nakagawa, K., Murakami, T., Sezaki, S., Sato, H., Suzuki, M., et al. (2019). Evaluation of Meniscal Regeneration in a Mini Pig Model Treated with a Novel Polyglycolic Acid Meniscal Scaffold. Am. J. Sports Med. 47 (8), 1804–1815. doi:10.1177/0363546519850578
Ozeki, N., Kohno, Y., Kushida, Y., Watanabe, N., Mizuno, M., Katano, H., et al. (2021). Synovial Mesenchymal Stem Cells Promote the Meniscus Repair in a Novel Pig Meniscus Injury Model. J. Orthop. Res. 39 (1), 177–183. doi:10.1002/jor.24846
Pan, Z., Wu, Y., Zhang, X., Fu, Q., Li, J., Yang, Y., et al. (2017). Delivery of Epidermal Growth Factor Receptor Inhibitor via a Customized Collagen Scaffold Promotes Meniscal Defect Regeneration in a Rabbit Model. Acta Biomater. 62, 210–221. doi:10.1016/j.actbio.2017.07.008
Papalia, R., Del Buono, A., Osti, L., Denaro, V., and Maffulli, N. (2011). Meniscectomy as a Risk Factor for Knee Osteoarthritis: a Systematic Review. Br. Med. Bull. 99, 89–106. doi:10.1093/bmb/ldq043
Peloquin, J. M., Santare, M. H., and Elliott, D. M. (2016). Advances in Quantification of Meniscus Tensile Mechanics Including Nonlinearity, Yield, and Failure. J. Biomech. Eng. 138 (2), 021002. doi:10.1115/1.4032354
Pereira, H., Fatih Cengiz, I., Gomes, S., Espregueira-Mendes, J., Ripoll, P. L., Monllau, J. C., et al. (2019). Meniscal Allograft Transplants and New Scaffolding Techniques. EFORT Open Rev. 4 (6), 279–295. doi:10.1302/2058-5241.4.180103
Rai, M. F., Brophy, R. H., and Rosen, V. (2020). Molecular Biology of Meniscus Pathology: Lessons Learned from Translational Studies and Mouse Models. J. Orthop. Res. 38 (9), 1895–1904. doi:10.1002/jor.24630
Rangger, C., Kathrein, A., Klestil, T., and Glötzer, W. (1997). Partial Meniscectomy and Osteoarthritis. Sports Med. 23 (1), 61–68. doi:10.2165/00007256-199723010-00006
Roberts, S., Evans, H., Wright, K., van Niekerk, L., Caterson, B., Richardson, J. B., et al. (2015). ADAMTS-4 Activity in Synovial Fluid as a Biomarker of Inflammation and Effusion. Osteoarthritis and Cartilage 23 (9), 1622–1626. doi:10.1016/j.joca.2015.05.006
Rodeo, S. A. (2000). Arthroscopic Meniscal Repair with Use of the Outside-In Technique. Instr. Course Lect 49, 195–206.
Rodeo, S. A. (2001). Meniscal Allografts-Where Do We Stand? Am. J. Sports Med. 29 (2), 246–261. doi:10.1177/03635465010290022401
Roller, B. L., Monibi, F., Stoker, A. M., Bal, B. S., and Cook, J. L. (2016). Identification of Novel Synovial Fluid Biomarkers Associated with Meniscal Pathology. J. Knee Surg. 29 (1), 47–62. doi:10.1055/s-0034-1394165
Ruiz-Ibán, M. Á., Díaz-Heredia, J., García-Gómez, I., Gonzalez-Lizán, F., Elías-Martín, E., Abraira, V., et al. (2011). The Effect of the Addition of Adipose-Derived Mesenchymal Stem Cells to a Meniscal Repair in the Avascular Zone: an Experimental Study in Rabbits. Arthroscopy 27 (12), 1688–1696. doi:10.1016/j.arthro.2011.06.041
Schreiner, A. J., Stannard, J. P., Cook, C. R., Bozynski, C. C., Kuroki, K., Stoker, A. M., et al. (2021). Comparison of Meniscal Allograft Transplantation Techniques Using a Preclinical Canine Model. J. Orthop. Res. 39 (1), 154–164. doi:10.1002/jor.24668
Searle, H., Asopa, V., Coleman, S., and McDermott, I. (2020). The Results of Meniscal Allograft Transplantation Surgery: what Is success? BMC Musculoskelet. Disord. 21 (1), 159. doi:10.1186/s12891-020-3165-0
Shaffer, B., Kennedy, S., Klimkiewicz, J., and Yao, L. (2000). Preoperative Sizing of Meniscal Allografts in Meniscus Transplantation. Am. J. Sports Med. 28 (4), 524–533. doi:10.1177/03635465000280041301
Shen, S., Yang, Y., Shen, P., Ma, J., Fang, B., Wang, Q., et al. (2021). circPDE4B Prevents Articular Cartilage Degeneration and Promotes Repair by Acting as a Scaffold for RIC8A and MID1. Ann. Rheum. Dis. 80 (9), 1209–1219. doi:10.1136/annrheumdis-2021-219969
Shen, W., Chen, J., Zhu, T., Yin, Z., Chen, X., Chen, L., et al. (2013). Osteoarthritis Prevention through Meniscal Regeneration Induced by Intra-articular Injection of Meniscus Stem Cells. Stem Cell Development 22 (14), 2071–2082. doi:10.1089/scd.2012.0563
Sherman, S. L., DiPaolo, Z. J., Ray, T. E., Sachs, B. M., and Oladeji, L. O. (2020). Meniscus Injuries. Clin. Sports Med. 39 (1), 165–183. doi:10.1016/j.csm.2019.08.004
Shimomura, K., Hamamoto, S., Hart, D. A., Yoshikawa, H., and Nakamura, N. (2018). Meniscal Repair and Regeneration: Current Strategies and Future Perspectives. J. Clin. Orthopaedics Trauma 9 (3), 247–253. doi:10.1016/j.jcot.2018.07.008
Shimomura, K., Rothrauff, B. B., Hart, D. A., Hamamoto, S., Kobayashi, M., Yoshikawa, H., et al. (2019). Enhanced Repair of Meniscal Hoop Structure Injuries Using an Aligned Electrospun Nanofibrous Scaffold Combined with a Mesenchymal Stem Cell-Derived Tissue Engineered Construct. Biomaterials 192, 346–354. doi:10.1016/j.biomaterials.2018.11.009
Snoeker, B., Turkiewicz, A., Magnusson, K., Frobell, R., Yu, D., Peat, G., et al. (2020). Risk of Knee Osteoarthritis after Different Types of Knee Injuries in Young Adults: a Population-Based Cohort Study. Br. J. Sports Med. 54 (12), 725–730. doi:10.1136/bjsports-2019-100959
Steenbrugge, F., Verdonk, R., Hrel, C., and Verstraete, K. (2004). Arthroscopic Meniscus Repair: Inside-Out Technique vs. Biofix Meniscus Arrow. Knee Surg. Sports Traumatol. Arthrosc. 12 (1), 43–49. doi:10.1007/s00167-003-0446-8
Stein, S. E. C., von Luebken, F., Warnecke, D., Gentilini, C., Skaer, N., Walker, R., et al. (2019). The challenge of Implant Integration in Partial Meniscal Replacement: an Experimental Study on a Silk Fibroin Scaffold in Sheep. Knee Surg. Sports Traumatol. Arthrosc. 27 (2), 369–380. doi:10.1007/s00167-018-5160-7
Strauss, E., Caborn, D. N. M., Nyland, J., Horng, S., Chagnon, M., and Wilke, D. (2019). Tissue Healing Following Segmental Meniscal Allograft Transplantation: a Pilot Study. Knee Surg. Sports Traumatol. Arthrosc. 27 (6), 1931–1938. doi:10.1007/s00167-019-05355-z
Sun, Y., Zhang, Y., Wu, Q., Gao, F., Wei, Y., Ma, Y., et al. (2021). 3D-bioprinting Ready-To-Implant Anisotropic Menisci Recapitulate Healthy Meniscus Phenotype and Prevent Secondary Joint Degeneration. Theranostics 11 (11), 5160–5173. doi:10.7150/thno.54864
Takata, Y., Nakase, J., Shimozaki, K., Asai, K., and Tsuchiya, H. (2020). Autologous Adipose-Derived Stem Cell Sheet Has Meniscus Regeneration-Promoting Effects in a Rabbit Model. Arthrosc. J. Arthroscopic Relat. Surg. 36 (10), 2698–2707. doi:10.1016/j.arthro.2020.06.004
Tarafder, S., Park, G., and Lee, C. H. (2020). Explant Models for Meniscus Metabolism, Injury, Repair, and Healing. Connect. Tissue Res. 61 (3-4), 292–303. doi:10.1080/03008207.2019.1702031
Tarafder, S., Gulko, J., Sim, K. H., Yang, J., Cook, J. L., and Lee, C. H. (2018). Engineered Healing of Avascular Meniscus Tears by Stem Cell Recruitment. Sci. Rep. 8 (1), 8150. doi:10.1038/s41598-018-26545-8
Trentacosta, N., Graham, W. C., and Gersoff, W. K. (2016). Meniscal Allograft Transplantation: State of the Art. Sports Med. Arthrosc. Rev. 24 (2), e23–e33. doi:10.1097/jsa.0000000000000107
Vadher, S. P., Nayeb-Hashemi, H., Canavan, P. K., and Warner, G. M. (2006). Finite Element Modeling Following Partial Meniscectomy: Effect of Various Size of Resection. Conf. Proc. IEEE Eng. Med. Biol. Soc. 2006, 2098–2101. doi:10.1109/IEMBS.2006.259378
Wei, Y., Sun, H., Gui, Y., Yao, L., Han, L., Yu, W., et al. (2021). The Critical Role of Hedgehog-Responsive Mesenchymal Progenitors in Meniscus Development and Injury Repair. Elife 10. doi:10.7554/elife.62917
Wells, M. E., Scanaliato, J. P., Dunn, J. C., and Garcia, E. S. J. (2021). Meniscal Injuries: Mechanism and Classification. Sports Med. Arthrosc. Rev. 29 (3), 154–157. doi:10.1097/jsa.0000000000000311
Wilusz, R. E., Weinberg, J. B., Guilak, F., and McNulty, A. L. (2008). Inhibition of Integrative Repair of the Meniscus Following Acute Exposure to Interleukin-1 In Vitro. J. Orthop. Res. 26 (4), 504–512. doi:10.1002/jor.20538
Wu, Y., Hong, J., Jiang, G., Li, S., Chen, S., Chen, W., et al. (2019). Platelet-rich Gel-Incorporated Silk Scaffold Promotes Meniscus Regeneration in a Rabbit Total Meniscectomy Model. Regenerative Med. 14 (8), 753–768. doi:10.2217/rme-2018-0087
Yamasaki, S., Hashimoto, Y., Nishida, Y., Teraoka, T., Terai, S., Takigami, J., et al. (2020). Assessment of Meniscal Healing Status by Magnetic Resonance Imaging T2 Mapping after Meniscal Repair. Am. J. Sports Med. 48 (4), 853–860. doi:10.1177/0363546520904680
Yan, R., Chen, Y., Gu, Y., Tang, C., Huang, J., Hu, Y., et al. (2019). A Collagen-Coated Sponge Silk Scaffold for Functional Meniscus Regeneration. J. Tissue Eng. Regen. Med. 13 (2), 156–173. doi:10.1002/term.2777
Zellner, J., Hierl, K., Mueller, M., Pfeifer, C., Berner, A., Dienstknecht, T., et al. (2013). Stem Cell-Based Tissue-Engineering for Treatment of Meniscal Tears in the Avascular Zone. J. Biomed. Mater. Res. 101 (7), 1133–1142. doi:10.1002/jbm.b.32922
Zhang, L., Liu, G., Han, B., Wang, Z., Yan, Y., Ma, J., et al. (2020). Knee Joint Biomechanics in Physiological Conditions and How Pathologies Can Affect it: A Systematic Review. Appl. Bionics Biomech. 2020, 7451683. doi:10.1155/2020/7451683
Zhang, Z.-Z., Zhou, Y.-F., Li, W.-P., Jiang, C., Chen, Z., Luo, H., et al. (2019). Local Administration of Magnesium Promotes Meniscal Healing through Homing of Endogenous Stem Cells: A Proof-Of-Concept Study. Am. J. Sports Med. 47 (4), 954–967. doi:10.1177/0363546518820076
Zhao, W., Zou, T., Cui, H., Lv, Y., Gao, D., Ruan, C., et al. (2020). Parathyroid Hormone (1-34) Promotes the Effects of 3D Printed Scaffold-Seeded Bone Marrow Mesenchymal Stem Cells on Meniscus Regeneration. Stem Cel Res Ther 11 (1), 328. doi:10.1186/s13287-020-01845-x
Keywords: meniscus, repair, replacement, PTOA, osteoarthritis
Citation: Trivedi J, Betensky D, Desai S and Jayasuriya CT (2021) Post-Traumatic Osteoarthritis Assessment in Emerging and Advanced Pre-Clinical Meniscus Repair Strategies: A Review. Front. Bioeng. Biotechnol. 9:787330. doi: 10.3389/fbioe.2021.787330
Received: 30 September 2021; Accepted: 06 December 2021;
Published: 22 December 2021.
Edited by:
Andreas Martin Seitz, Ulm University Medical Center, GermanyReviewed by:
Muhammad Farooq Rai, Washington University School of Medicine in St. Louis, United StatesSonia Bansal, Hospital for Special Surgery, United States
Copyright © 2021 Trivedi, Betensky, Desai and Jayasuriya. This is an open-access article distributed under the terms of the Creative Commons Attribution License (CC BY). The use, distribution or reproduction in other forums is permitted, provided the original author(s) and the copyright owner(s) are credited and that the original publication in this journal is cited, in accordance with accepted academic practice. No use, distribution or reproduction is permitted which does not comply with these terms.
*Correspondence: Chathuraka T. Jayasuriya, Q2hhdGh1cmFrYV9KYXlhc3VyaXlhQEJyb3duLmVkdQ==