- 1Department of Orthopedics, The Second Affiliated Hospital of Nanchang University, Nanchang, China
- 2Institute of Orthopedics of Jiangxi Province, Nanchang, China
- 3Institute of Minimally Invasive Orthopedics, Nanchang University, Nanchang, China
- 4Second Clinical College, Nanchang University, Nanchang, China
- 5Jiujiang University, Key Laboratory of Medical Transformation of Jiujiang, Jiujiang, China
Purpose: Extracellular Vesicles (EVs) derived from hMSCs, have the potential to alleviate cartilage damage and inflammation. We aimed to explore the effects of EVs derived from lncRNA malat‐1-overexpressing human mesenchymal stem cells (hMSCs) on chondrocytes.
Material and Methods: hMSCs-derived Extracellular Vesicles (hMSCs-EVs) were identified by transmission electron microscopy and western blot. We used a Sprague-Dawley (SD) rat model of CollagenaseⅡ-induced osteoarthritis (OA) as well as IL-1β-induced OA chondrocytes. Lentiviral vectors were used to overexpress lncRNA malat‐1 in hMSCs. Chondrocyte proliferation, inflammation, extracellular matrix degradation, and cell migration were measured by Edu staining, ELISA, western blot analysis, and transwell assay. Chondrocyte apoptosis was evaluated by flow cytometry, Hoechst 33342/PI Staining, and western blot. Safranine O-fast green (S-O) staining and HE staining were used to assess morphologic alterations of the rat knee joint.
Results: hMSCsmalat−1-EVs decreased MMP-13, IL-6, and Caspase-3 expression in IL-1β-induced OA chondrocytes. Moreover, hMSCsmalat−1-EVs promoted chondrocyte proliferation and migration, suppressed apoptosis, and attenuated IL-1β-induced chondrocyte injury. Our animal experiments suggested that hMSCsmalat−1-EVs were sufficient to prevent cartilage degeneration.
Conclusion: Our findings show that lncRNA malat-1from hMSCs‐delivered EVs can promote chondrocyte proliferation, alleviate chondrocyte inflammation and cartilage degeneration, and enhance chondrocyte repair. Overall, hMSCsmalat−1-EVs might be a new potential therapeutic option for patients with OA.
Introduction
Osteoarthritis (OA) is one of the most common age-related degenerative joint diseases and public health problems worldwide, and can cause pain, disability, social and economic loss (Glyn-Jones et al., 2015). OA is characterized by the destruction of articular cartilage, synovial inflammation, osteophyte formation, and extracellular matrix (ECM) loss (Guilak et al., 2018). The molecular mechanisms underlying the occurrence and progression of OA remain unclear, and currently there are no interventions that can successfully restore the degenerated cartilage and slow down disease progression. Therefore, it is a clinical priority to seek new treatments for OA. Targeting cartilage cell regeneration, apoptosis and of extracellular matrix loss might represent new therapeutic avenues for the management and treatment of OA.
Mesenchymal stem cells (MSCs) are a type of pluripotent stem cells with self-renewal ability, have anti-inflammatory and immune roles, and have the ability of tissue repair. Bone marrow mesenchymal stem cells are used for fracture healing, cartilage repair, kidney injury, articular cartilage injury, myocardial injury and regenerative medicine due to their superior repair ability (Zhang et al., 2015; Chen et al., 2016; Li et al., 2018a; Wang et al., 2018; Yang et al., 2018; Fu et al., 2019). Existing animal experiments and clinical studies have confirmed that intra-articular injection of MSCs can effectively delay the degeneration of articular cartilage, relieve pain and improve joint function (Desando et al., 2013; Matas et al., 2019). At present, MSCs treatment still has certain limitations and side effects, such as thrombosis (Furlani et al., 2009) and abnormal ossification (Breitbach et al., 2007). Therefore, its therapeutic effect needs further research.
In recent years, EVs have gradually become the focus and entered people’s field of vision. Evs carry proteins, mRNA, and non-coding RNA (including miRNA, lncRNA, circRNA) (Tao et al., 2017; Foers et al., 2018). Many current studies have shown that EVs have a huge potential for the MSCs to treating OA (Batrakova and Kim, 2015). The research results of Lei He et show bone marrow mesenchymal stem cell-derived EVs played an important role at protecting cartilage damage and relieving knee osteoarthritis pain in a rat model of osteoarthritis (He et al., 2020). It can be seen that EVs have great potential in the treatment of OA.
LncRNA is a new non-coding RNA widely expressed in the human genome, with a well-conserved sequence and high tissue specificity (Washietl et al., 2014). Metastasis-associated lung adenocarcinoma transcript 1 (malat-1) is a novel transcript of over 8000 nucleotides which has been shown to regulate inflammation (Puthanveetil et al., 2015), promotes chondrocyte proliferation (Yang et al., 2017). LncRNA malat-1 has been extensively studied in human systemic lupus erythematosus (SLE) (Li et al., 2020a), myasthenia gravis (MG) (Felson et al., 2000), cardiovascular disease (Zhu et al., 2019), osteoporosis (Yang et al., 2019), and cancer (Fu et al., 2020). Our previous studies have also found that lncRNA malat-1 in chondrocytes promotes chondrocyte proliferation, suppresses chondrocyte apoptosis, and reduces extracellular matrix degradation (Kong et al., 2019). But to the best of our knowledge no studies have confirmed whether malat-1 in the EVs can protect chondrocytes in OA. Therefore, the specific role of lncRNA malat-1 needs further study. We hypothesized that EVs expressing lncRNA malat-1 can delay chondrocyte degeneration, promote chondrocyte repair, and have a therapeutic potential in OA, and might be a reliable therapeutic approach for OA.
Materials and Methods
Cell Culture and Co-Culture of Chondrocytes and EVs
Human MSCs (hMSCs) and human chondrocyte C28/I2 cells were acquired from ATCC (Manassas, United States) and BeNa Culture Collection (Beijing, China), respectively. Cells were cultured in DMED/F12 (Gibco, United States), containing 10% FBS (Gibco, United States) in a humidified incubator at 37°C and 5% CO2. Cells were passaged every 2–3 days.
To establish an OA cell model, C28/I2 cells at 60–70% confluency were treated with or without 10 ng/ml human IL-1β recombinant protein (Sigma, United States) for 24 h. Following establishment of the vitro OA model, chondrocytes were treated with normal medium, hMSCs-EVs (10 μg/ml), and hMSCsmalat−1-EVs (EVs derived from lncRNA malat-1-overexpressing-hMSCs) for 24 h to investigate the effect of EVs, derived from lncRNA malat-1-overexpressing-hMSCs, on IL-1β-induced chondrocyte damage.
Isolation and Identification of EVs
HMSCs were grown at 80–90% confluency, at which time EVs -free medium (UR51101, Umibio, Shanghai, China) was added. Following 48 h of culture, supernatant was collected. Cell culture media was centrifuged to remove cell debris and then mixed with EVs isolate kit (UR52121, Umibio, Shanghai, China). According to the manufacturer’s instructions, an initial spin was performed at 3000 ×g, at 4°C for 10 min to remove cells and cell debris. Then, the corresponding amount of reagents were added proportionally to the starting sample volume, mixed and incubated at 4°C for 2–4 h, followed by another centrifugation at 10,000 ×g for 60 min to obtain EVs pellets. Pellets were then resuspended with PBS, and purified with an EVs purification filter at 3,000 ×g for 10 min. EVs pellets were resuspended in 400 μl for 40 ml according to the manufacturer’s instructions. EVs were stored at −80°C immediately after isolation until further analysis.
BCA protein assay kit (Beyotime, China) was used to assess EVs concentration. EVs morphological appearance was observed by transmission electron microscopy (TEM, H-7700, Hitachi, Japan), and EVs surface markers were analyzed by western blot (WB).
Uptake of PKH26‐Labelled hMSCs‐EVs
For up-take experiments, hMSCs-EVs were labeled with a red fluorescent color PKH26 kit (Sigma-Aldrich, United States), according to the manufacturer’s instructions. Briefly, hMSCs‐EVs were resuspended in 500 µl Diluent C, 2 µl PKH26 dye was then added to 500 µl Diluent C and incubated for 5 min at room temperature (RT). Then, 1 ml of 0.5% EVs‐depleted FBS was added and incubated for 5 min to allow binding to excess dye. hMSCs‐EVs were then collected by centrifugation at 100,000 ×g, at 4°C for 1 h. Next, hMSCs‐EVs were resuspended in PBS and co-cultured with chondrocyte cells. Twenty-four hours later, cells samples were fixed in 4% paraformaldehyde, stained by 4′, 6‐diamidine‐2′2phenylindole dihydrochloride (DAPI) (Beyotime, Shanghai, China) for 10 min at RT, and observed under a fluorescence microscope (Novel, China) at a magnification of ×400.
Cell Transduction and Transfection
Lentiviral vectors targeting malat-1 were purchased from Focus Bioscience (Nanchang, China). Briefly, lentiviral vectors were transfected into competent cell 293T by using Lipofectamine 2000 (Thermo Fisher Scientific, United States). Culture medium was collected and co-cultured with hMSCs. HMSCs were infected lentivirus medium for 16 h, following which medium was replaced with fresh medium for another 48 h. Then, expression of lncRNA malat-1 was assessed by qRT-PCR. Infected hMSCs cells were co-cultured with puromycin (1 µg/ml) until all cells in the control group were dead. hMSCs overexpressing lncRNA malat-1 were filtrated and used for the next experiments.
Cell Viability Assay
CCK-8 was used to detect the effect of EVs on IL-1β-induced-chondrocyte injury. Chondrocyte cells (2 × 103) were cultured in a 96-well plate overnight, and co-cultured with different EVs concentrations (0, 1, 5, 10, 25, and 50 μg/ml) for 24 h. A control group was only co-cultured with normal culture media. According to the manufacturer’s protocol, cells were incubated with 10 μl of CCK-8 solution (TransGen, Beijing, China) in each well for 4 h at 37°C. Finally, the optical density (OD) value at 450 nm of each well was measured by using a microplate reader (Perlong, Beijing, China).
Edu Proliferation Assay
The effects of EVs on chondrocytes were assessed with the Edu Cell Proliferation Assay Kit (Beyotime, China). Cells were seeded in 12-well plates and treated with IL-1β or EVs for 24 h. Following the manufacturer’s manual, 10 μM of Edu solution was added to the plates in an incubator at 37°C for 2 h before fixation and permeabilization. Then, cells were incubated with Click Additive Solution at RT and kept away from light for 30 min. Next, chondrocytes cell nuclei were stained with Hoechst 33342 according to the manufacturer’s instructions. The proportion of cells incorporating Edu was assessed under a fluorescence microscope.
Transwell Assay
Transwell cell culture chamber assay was used to assess cell migration. After treatment with IL-1β or different EVs for 24 h, chondrocytes were digested and resuspended in 2 ml serum-free medium. Then count the cells to ensure that the cell concentration is 7.5 × 104/ml. Next, 400 μl of cell suspension was added to the upper chamber (Corning, United States), and 700 μl DMEM containing 10% FBS was added to each bottom chamber of the 24-well plate. Following co-cultured for 24 h chondrocytes were washed twice with PBS, and fixed 4% paraformaldehyde for 20 min at RT. Then, chondrocytes were stained with 0.5% crystal violet dye for 30 min. Finally, the number of migrating cells was counted under a microscope.
Annexin V-FITC/Propidium Iodide Flow Cytometry Assay
Chondrocytes were cultured in 6-well plates and treated with IL-1β and EVs. After 24 h incubation, cells were resuspended with PBS, cells were counted, then resuspended in binding buffer, followed by incubation with Annexin V-FITC and PI (Beyotime, China) for 20 min at RT in a dark place. The rate of apoptosis was measured by flow cytometry (BD, San Jose, CA, United States).
Hoechst 33342/PI Assay
Hoechst 33342/PI staining kit was acquired from Solarbio (Beijing, China). According to the manufacturer’s instructions, dye and cell staining buffers were added to each well incubated at 4°C for 30 min in a dark place after cell treatment with IL-1β, hMSCs-EVs, or hMSCsmalat−1-EVs. Finally, cells were observed with a fluorescence microscope.
IL-6 ELISA Assay
Following culture and treatment of chondrocyte cells with IL-1β or EVs for 24 h, the supernatants of each group were collected. The concentration of IL-6 was measured with a specific ELISA kit (Neobioscience, Shenzhen, China) following the manufacturer’s instructions. In brief, 100 μl of the control, standard, or sample was added to each well and incubated for 90 min at 37°C. Then, wells were washed five times and incubated with 100 μl of human IL-6 conjugate antibody for 1 h at 37°C and washed again five times. 100 μl of the enzyme combination solution was added into each well and incubated for 30 min at 37°C away from light, followed by another step of washes. 100 μl of substrate solution was then added to each well and incubated for 15 min at 37°C away from the light. Finally, 100 μl of Stop Solution was added, and the OD values were measured at 450 nm by using a microplate reader.
Quantitative Real-Time Polymerase Chain Reaction
Total RNA was isolated from cells using Trizol reagent (Takara, Japan). Reverse transcription was carried out with the PrimeScrip RT reagent Kit with gDNA Eraser (Takara, Japan). TB Green Premix Ex Taq kit (Takara, Japan) was used to perform real-time PCR, and we used the CFX96 Real-Time PCR Detection System (Bio-Rad, United States) with the following primers: HMSC GAPDH: forward, 5′-GGTGGTCTCCTCTGACTTCAACA-3′ and reverse, 5′-TTGCTGTAGCCAAATTCGTTGT-3′; HMSC malat-1:forward, 5′- TCAGGATAATCAGACCACCACAG-3′ and reverse, 5′- GTAACTACCAGCCATTTCTCCAA-3′; Internal control was GAPDH.
Western Blot
Cells were washed three times with PBS and lysed in RIPA buffer with a protease inhibitor cocktail at the ice temperature for 20 min. After centrifugation at 10,000 ×g and 4°C for 10 min, the concentration of total protein levels was measured using the BCA kit. Protein extracts were separated by polyacrylamide gel electrophoresis (12–15%) and transferred to polyvinylidene difluoride (PVDF) membranes. Then, blots were incubated overnight at 4°C with antibodies specific for GAPDH (1:5000,Abcam,United States), CD63 (1:1000, Proteintech, China), CD81 (1:1000,Abcam, United States), TSG101 (1:1000,Abcam, United States), caspase-3 (1:5000,Abcam, United States), IL-6 (1:3000,Proteintech, China),MMP-13 (1:500, Proteintech, China). After washing with TBST 3 times, membranes were incubated with a goat anti-rabbit IgG–horseradish peroxidase antibody. Proteins were visualized using the ECL Chemiluminescent Kit (UE, Suzhou, China), and the chemical luminescence reaction was detected by the TECAN luminescent imaging system.
Animal Studies
All animal experiments were approved by the Institutional Animal Care and Use Committee of Nanchang University (China) and followed by the Guide for the Care and Use of Laboratory Animals by the National Institutes of Health (NIH). 10-week-old male Sprague-Dawley (SD rats) were obtained from the Experimental Animal Center of Nanchang University (Nanchang, China). Animals were kept in an environment with a dark/light cycle at 20–25°C, at ad libitum access to water and food. Animals were randomly divided into four groups randomly (n = 4 per group): Normal; OA + PBS; OA + hMSCs-EVs; OA + hMSCsmalat−1-EVs. To establish the OA model, collagenase Ⅱ was injected into the knee joint cavity of SD rats under anesthesia. Three weeks after the injection, hMSCs-derived EVs were injected into the articular cavity of rats once a week at a concentration of 40 μg/100 μl. Six weeks later, histologic analysis was performed on the knee joint specimens.
Haematoxylin and Eosin and Safranine O-Fast Green Staining
Six weeks after treatment with EVs, all animals were sacrificed and articular cartilage samples were collected, fixed in paraformaldehyde for 24 h and subject to calcium removal for 21 days in 10% EDTA (pH 7.4). Then, knee-joint tissues were embedded in paraffin and sectioned into 5-μm-thick sections. Serial sections were obtained from the medial and lateral compartments at 200-μm intervals. Selected sections were deparaffinized in xylene, rehydrated through a graded series of ethanol washes, and stained by hematoxylin and eosin (H&E) and Safranin O/Fast Green staining (Solarbio, China). The degree of articulatio genus cartilage degeneration on the medial and lateral tibial plateau joint was evaluated according to the Osteoarthritis Research Society International (OARSI) score and the modified Mankin’s score.
Statistical Analysis
All experiments were performed in triplicate. Mean ± standard deviation (SD) was used to present the data. Graphics and statistical analyses were conducted by GraphPad Prism 8 software (GraphPad Inc., La Jolla, CA, United States). Student’s t-test for two groups and one-way ANOVA with Tukey post hoc test for three or more groups were used for group comparisons. A p value of less than 0.05 was considered statistically significant.
Results
Identification of EVs and Isolated From Human BMSCs
After 48 h in culture, bone marrow mesenchymal stem cells (hMSCs) showed a uniform spindle morphology (Figure 1A). When the cell confluency reached 80%, EVs were extracted and identified by TEM, NTA, and WB. TEM results showed that EVs exhibited a classic approximate circular cup-shaped structure (Figure 1B). NTA showed a main peak of the particle size of approximately 144 nm (Figure 1C). Furthermore, WB showed that surface molecular markers CD63, CD81, TSG101 of the EVs were highly expressed compared to those of the negative group PBS (Figure 1D, Supplementary Data Sheet S2). These results show that EVs were successfully separated from the hMSCs.
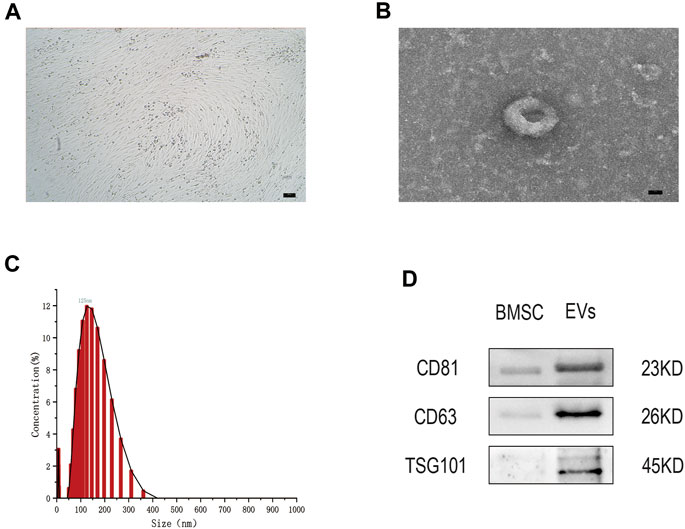
FIGURE 1. (A) The hMSCs were observed by optical microscope. Scale bar = 100 μm. (B) The cup-shaped EVs under TEM. Scale bar = 25 nm (C) Size and concentration of EVs analyzed by NTA. (D) The indicated protein (CD9, CD63, and TSG101) in EVs were detected via western blotting. n = 3.
Establishment of OA Model of Chondrocytes and EVs Uptake Experiment
According to previous literature, IL-1β (10 ng/ml) is appropriate for establishing an inflammatory injury model of chondrocytes to simulate the microenvironment of OA. ELISA experiments have shown that, after IL-1β treatment, the expression of the inflammatory cytokine IL-6 in chondrocytes was significantly higher than in the control group (p < 0.05) (Figure 2A). The expression of this inflammatory factors was also detected by western blot, with the results showing that IL-6 protein expression in the OA model group was increased by 3.89 times (p < 0.05) (Figures 2B,C, Supplementary Data Sheet S1). All data were statistically significant. These results indicate that the inflammatory injury model of chondrocytes has been successfully established.
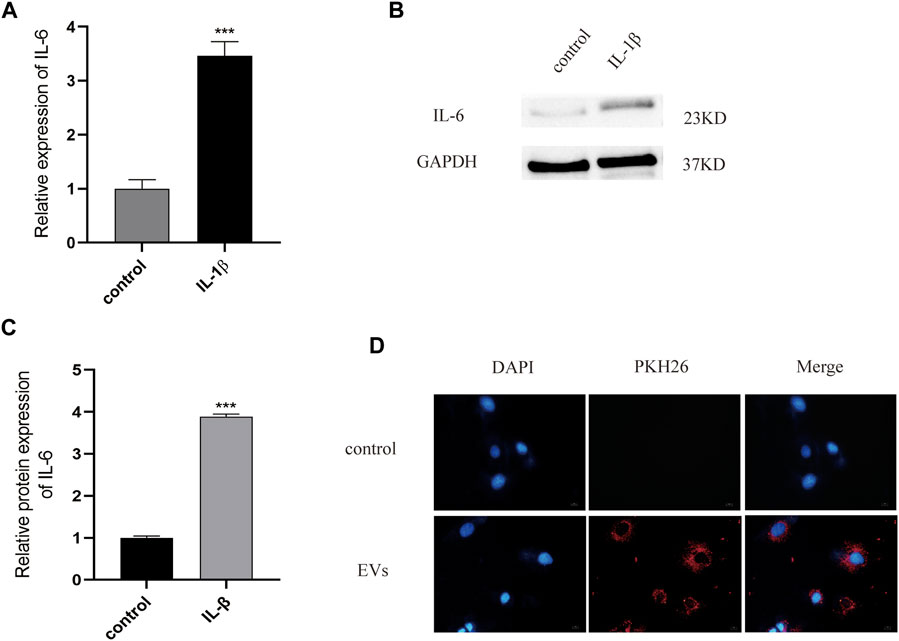
FIGURE 2. (A) The expression of inflammatory factor IL-6 analyzed by Elisa. (B) and (C) The protein of IL-6 measured by western blot. (D) PKH26-labeled were uptaked by chondrocyte. Scale bar = 10 μm, n = 3, ***p < 0.001, t-test for all.
To test the potential of EVs in the treatment of OA, we used PKH26 labeled EVs (red fluorescence), which were then co-cultured with chondrocytes. After 24 h of co-culture, EVs labeled with PKH26 were successfully observed in the cytoplasm of chondrocytes by fluorescence microscopy, suggesting that the EVs had been taken up by chondrocytes (Figure 2D).
LncRNA Malat-1 Overexpression in the EVs Reduces IL-1β Induced Inhibition of Chondrocyte Proliferation
To obtain lncRNA malat-1-rich EVs, lentiviral vectors were used to overexpress malat-1 in hMSCs. qRT-PCR data showed that malat-1 expression increased by approximately 2.6 times compared to the control group (p < 0.05) (Figure 3A). EVs were then extracted from the hMSCs cell serum, and lncRNA malat-1 expression in hMSCsmalat-1-EVs, detected by qRT-PCR, was about 4.03 times higher than in hMSCs-EVs (p < 0.05) (Figure 3B), indicating that lncRNA malat-1 was successfully expressed in EVs. To investigate the role of EVs in OA, we investigated the effects of different concentrations of EVs on chondrocyte vitality after IL-1β treatment. We found chondrocyte vitality was significantly decreased after IL-1β treatment (p < 0.05). There was no significant difference in the 10 µg/ml groups compared to 25 µg/ml and 50 µg/ml concentrations (p > 0.05) (Figure 3C). Therefore, the concentration of 10 µg/ml was selected for subsequent experiments.
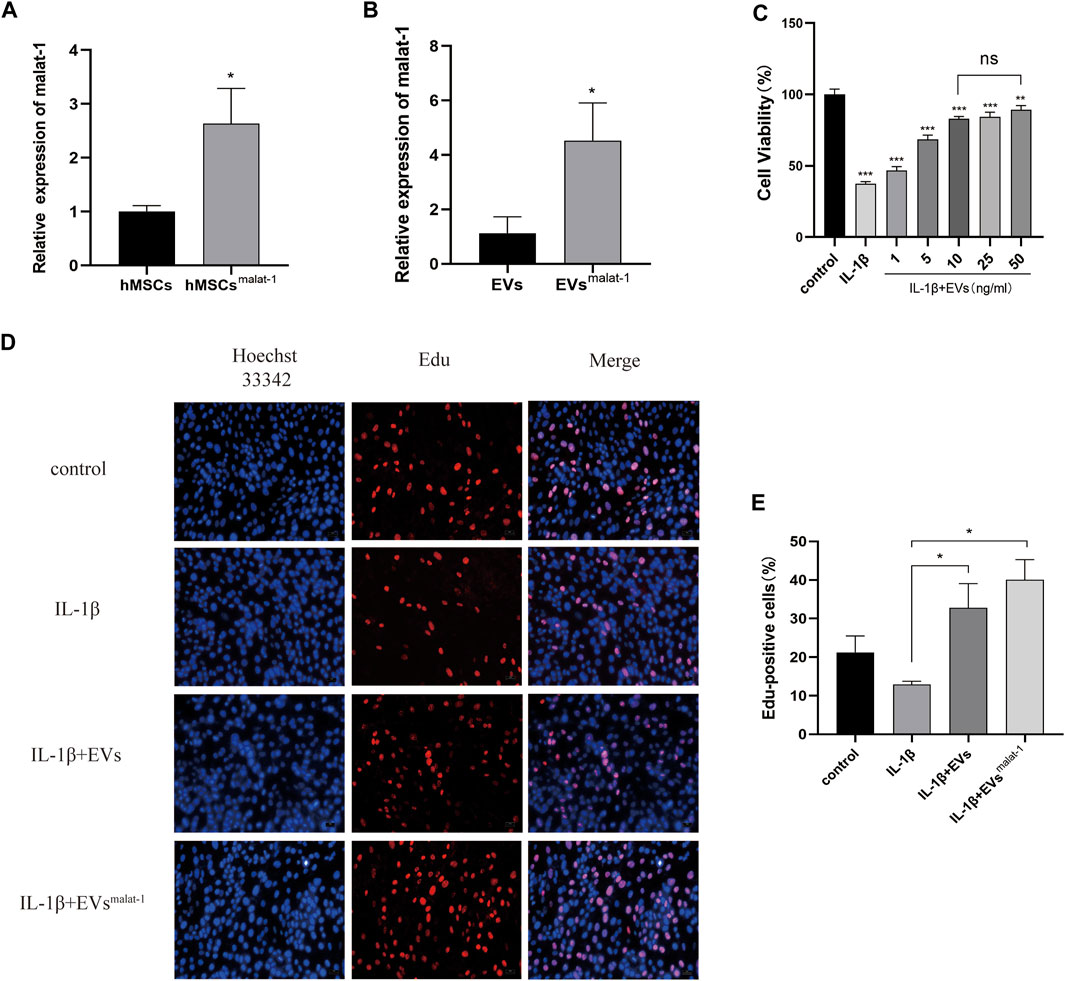
FIGURE 3. (A) The expression of lncRNA malat-1 were analyze between hMSCs and hMSCsover-malat−1 by qRT-PCR, n = 3, *p < 0.05, t-test. (B) The expression of lncRNA malat-1 in two EVs analyzed by qRT-PCR, n = 3, *p < 0.05, t-test. (C) Cell viability of chondrocytes treated with different concentrations of EVs by CCK-8 assay. n = 3, *p < 0.05, one-way ANOVA (D) and (E) Edu assay was performed to detect proliferation of chondrocytes, n = 3, *p < 0.05, one-way ANOVA.
Then, the effects of hMSCs-EVs and hMSCsmalat−1-EVs on chondrocyte proliferation were examined in the different groups. Edu staining confirmed that chondrocytes from the hMSCsmalat−1-EVs group showed better proliferation promotion compared to the hMSCs-EVs group (p < 0.05) (Figures 3D,E).
hMSCsmalat−1-EVs Inhibited Apoptosis and Inflammation of Chondrocytes Induced by IL-1β
We performed additional experiments to further explore the effect of LncRNA malat-1 in EVs on IL -1β induced chondrocyte apoptosis. We measured the apoptosis rate by flow cytometry. As the result show (Figures 4A,B), the apoptosis rate of the IL-1β group (16.62 ± 1.12%, p < 0.05) was significantly higher than that from the normal group (4.25 ± 0.32%, p < 0.05). After treatment with the hMSCs-EVs, the apoptosis rate of chondrocytes (9.67 ± 0.6%, p < 0.05) decreased by a third. At the same time, we found that the apoptosis rate of hMSCsmalat-1-EVs group (7.02 ± 0.42%, p < 0.05) decreased more substantially and was closer to the normal rate. Similarly, Hoechst 33342/PI staining results showed that after IL-1β treatment, the number of apoptotic cells increased significantly (Figures 4C,D). After adding hMSCs-EVs, the number of apoptotic cells decreased, but after adding hMSCsmalat−1-EVs, the number of apoptotic cells decreased even more substantially, suggesting a stronger anti-apoptotic effect of hMSCsmalat−1-EVs. Caspase-3 protein expression was detected by WB, and the results were consistent with those from flow cytometry and Hoechst 33342/PI staining.
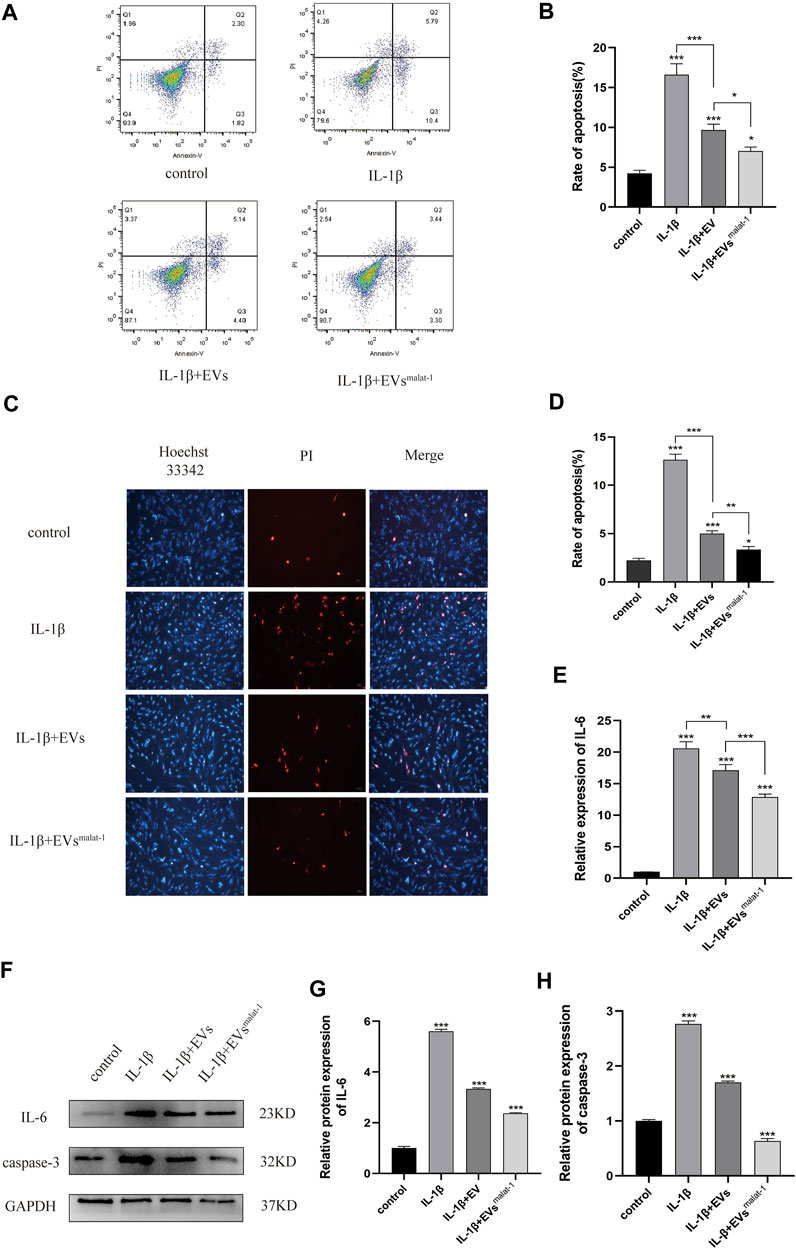
FIGURE 4. (A) and (B) The apoptosis rate of chondrocytes treated with EVs analyzed by flow cytometry assay. (C) and (D) Hoechst 33342/PI assay was used to analyze the apoptosis rate of chondrocytes. (E) Inflammatory factor IL-6 expression was measured using Elisa in chondrocytes after treated with EVs. (F–H) The protein expression of IL-6 and Caspase-3 analyzed by western blot. *p < 0.05, **p < 0.01, ***p < 0.001, vs. control group. n = 3, one-way ANOVA for all.
In addition, the effect of LncRNA malat - 1 in EVs on IL - 1β induced chondrocyte inflammatory injury. Based on results from ELISA and WB (Figures 4E,H, Supplementary Data Sheet S1), chondrocyte in the normal group appeared to produce only a small amount of IL - 6 inflammatory-related factors and the OA inflammatory damage was significantly higher (p < 0.05). following EVs treatment, expression levels of IL-6 inflammation-related factors decreased (p < 0.05), but compared with the hMSCs-EVs group, the decrease in the hMSCsmalat−1-EVs group was more substantial (p < 0.05). These results suggest that chondrocyte apoptosis and inflammation induced by IL-1β can be inhibited by lncRNA malat-1 of the EVs, which appears protective for chondrocytes of OA.
hMSCsmalat−1-EVs Reversed IL-1β -Induced Decline of Chondrocyte Migration and Reeducation of MMP-13
We then performed transwell experiments to test the effects of hMSCs-EVs and hMSCsmalat−1-EVs on the ability of chondrocytes to migrate in OA. Our results showed that the number of migrating cells of IL-1β group decreased significantly compared to control groups (p < 0.05) (Figures 5A,B), while no significant differences were observed in the hMSCs-EVs group (p > 0.05). All groups, when treated with hMSCsmalat−1-EVs, exhibited a larger number of migrating cells compared to the IL-1β group and the hMSCs-EVs group (p < 0.05). The results from these experiments showed that IL-1β reduced chondrocyte migration ability, while the EVs reversed these effects via lncRNA malat-1.
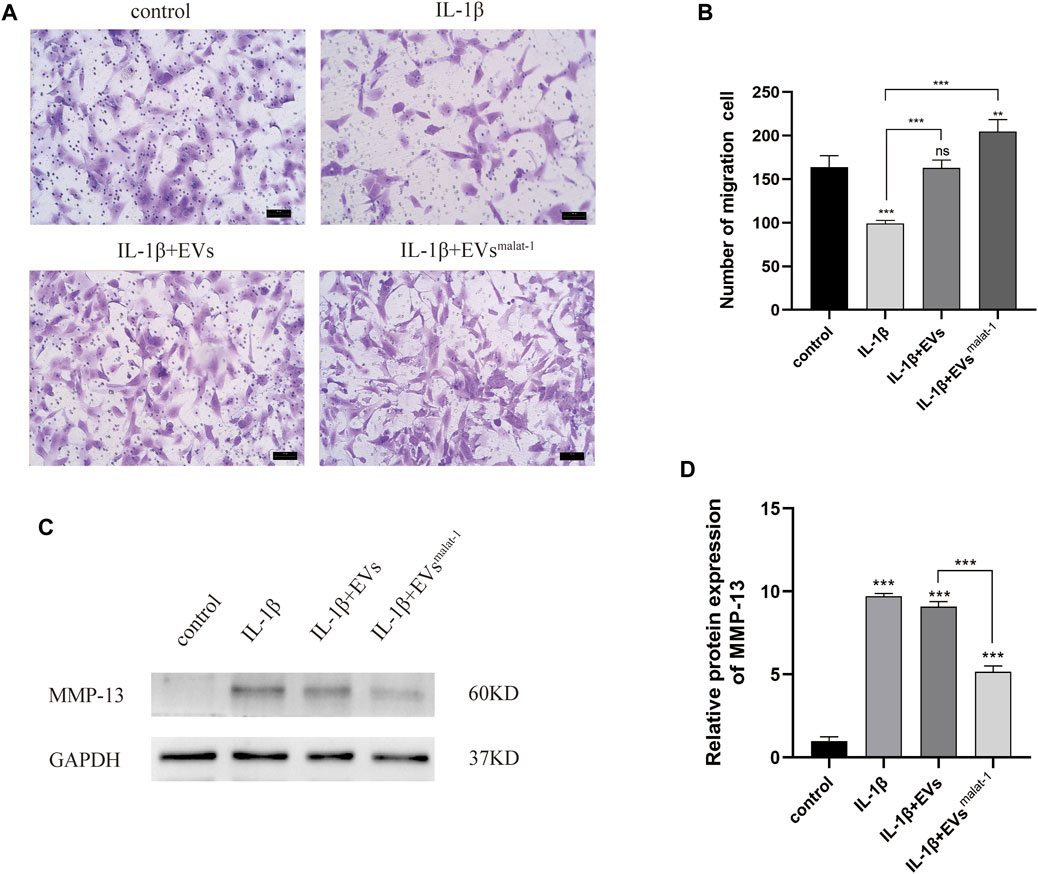
FIGURE 5. (A) and (B) Migration of chondrocytes was detected by Transwell assays. **p < 0.01, ***p < 0.001 vs. IL-1β group. (C) and (D) The protein expression of MMP-13 was detected by western blot, *p < 0.05, **p < 0.01, ***p < 0.001, vs. control group. n = 3, one-way ANOVA for all.
To investigate the effect of EVs by malat-1 on the extracellular matrix (ECM) in OA, matrix metalloproteinase 13 (MMP-13) protein expression was tested. We found that both EVs downregulated the increased MMP-13 protein levels induced by IL-1β (p < 0.05) (Figures 5C,D, Supplementary Data Sheet S1). A more substantial decrease in MMP-13 protein levels was observed in the hMSCsmalat−1-EVs group compared to the hMSCs-EVs group (p < 0.05), suggesting that the EVs delayed IL-1β -induced chondrocyte degeneration through lncRNA malat-1.
hMSCsmalat−1-EVs Alleviated Cartilage Damage in a Rat OA Model
To confirm the protective effect of hMSCsmalat−1-EVs on chondrocytes in vivo, hMSCs-EVs and hMSCsmalat−1-EVs were injected into the articular cavity of OA rats. Six weeks later, OA articular surface changes were observed by HE staining and Safranine O-fast green (S-O) staining. The articular cartilage structure of OA rats was clear and exhibited a smooth surface. In the OA group, the surface was rough and had an irregular shape, the fibers were broken, and the cartilage was substantially damaged (Figures 6A,B). The score of OARSI and Mankin (p < 0.05) increased significantly (Figures 6B,C). After hMSCs-EVs treatment, articular cartilage injury of OA rats was alleviated and OARSI scores and Mankin score both decreases. Compared with hMSCs-EVs, the joint surface of the hMSCsmalat−1-EVs group was smoother, the cartilage damage was substantially alleviated, and the scores decreased (p < 0.05). These results indicate that hMSCs-EVs can alleviate cartilage damage caused by OA via LncRNA malat-1 in a rat model of OA.
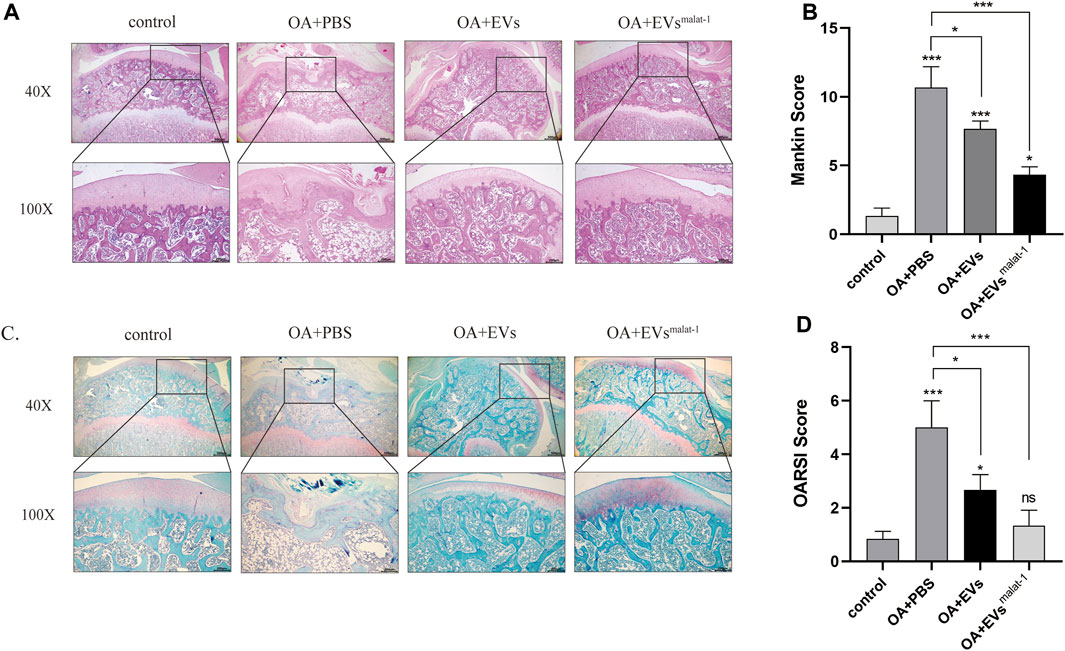
FIGURE 6. (A) Haematoxylin and Eosin staining of SD rat knee joints after injecting EVs for 6 weeks. Scale bar: 200 μm (40X) and 500 μm (100X). (B) The modified Mankin’s score of the SD rat knee joints. (C) Safranin O/Fast Green staining of SD rat knee joints after injecting EVs for 6 weeks. Scale bar: 200 μm (40X) and 500 μm (100X). (D) OARSI scores of the SD rat knee joints. *p < 0.05, **p < 0.01, ***p < 0.001, vs. control group. n = 3, one-way ANOVA for all.
Discussion
The causes of OA are complex and multifactorial, and involve age, gender, degree of obesity, aging, genetic factors, joint damage, and other factors (Felson et al., 2000). In this study, we investigated a new potential treatment for OA. We successfully isolated EVs from hMSCs and used them to treat chondrocytes induced by IL-1β. After that, we harvested lncRNA malat-1-rich EVs through transfecting hMSCs to stably express lncRNA malat-1, which were used in subsequent experiments for evaluating their therapeutic effect on IL-1β induced-cartilage injury. Our results showed that overexpression of lncRNA malat-1 of EVs significantly reduced expression of IL-6, caspase-3, MMP-13 in OA, increased apoptosis, reversed IL-1β induced inhibition of proliferation, and increased the proliferation and migration rates of chondrocytes.
EVs are a type of vesicular structure of 40–160 nm in diameter that is actively secreted by almost all cells (Kalluri and LeBleu, 2020). EVs contain signaling factors such as mRNA, miRNA, and lncRNA, targeting adjacent cells by autocrine and paracrine mechanisms, or distal cells through the circulatory system (Colombo et al., 2014). Compared with stem cell transplantation, EVs became a hot topic given their potential for the treatment of OA due to their characteristics such as immunogenicity, and ease to save. Previous studies have suggested their huge therapeutic potential in hMSCs derived EVs in joint disease (Li et al., 2018b), and the importance of hMSCs paracrine in various diseases (Linero and Chaparro, 2014). In this study, the results of TEM, NTA, and WB indicate that we successfully extracted MSCs-derived EVs Furthermore, we confirmed that cartilage cells successfully uptake EVs by using the PKH26 probe, indicating that EVs can play a role in chondrocytes. EVs have attracted the attention of many scientists as a good carrier for treating the diseases, such as Qing Mao has researched that lncRNA KLF3-AS1 in human mesenchymal stem cell-derived EVs ameliorates pyroptosis of cardiomyocytes and myocardial infarction through miR-138-5p/Sirt1 axis (Mao et al., 2019). Huaxia Yang et also has found that lncRNA malat-1 is a novel inflammatory regulator in human systemic lupus erythematosus (SLE), and has great potential in treating inflammation (Li et al., 2020b). LncRNA malat-1 is a novel transcript of over 8000 nucleotides, and one of the most widely studied lncRNAs. It was first discovered in transcript screening related to non-small cell lung cancer (NSCLC) metastasis and patient survival (Hutchinson et al., 2007). A study of Tony Gutschner shows that lncRNA malat-1 is a critical regulator of the metastasis phenotype of lung cancer cells (Gutschner et al., 2013). In recent years, Jongchan Kim et found that malat-1 can inhibit the metastasis of breast cancer. In other diseases, lncRNA malat-1 is also widely studied, such as Huaxia Yang et has shown stem cell-derived EVs prevent aging-induced cardiac dysfunction through a novel EVs/lncRNA malat-1/NF-κB/TNF-α signaling pathway (Zhu et al., 2019). Furthermore, Xucheng Yang confirmed that lncRNA malat-1 shuttled by EVs derived from bone marrow mesenchymal stem cells-secreted EVs alleviates osteoporosis through mediating microRNA-34c/SATB2 axis (Yang et al., 2019). The exciting thing is that Hongxi Li et found that lncRNA malat-1 can regulate ECM catabolism, inflammation, apoptosis and proliferation in chondrocyte (Li et al., 2020a). Our study show lncRNA malat-1 from EVs reduced expression of IL-6, caspase-3, MMP-13 in OA, decrease apoptosis, promote proliferation and migration. It shows that lncRNA malat-1 from EVs has the ability to delay the degeneration of chondrocytes.
Inflammation plays an important role in the pathogenesis of OA, in which the chondrocytes, synovial membrane, and surrounding tissues produce large amounts of inflammatory factors such as IL-1β, IL-6, TNF-α, that accelerate the progression of osteoarthritis (Eymard et al., 2014; Li et al., 2020a). The up-regulation of inflammatory factors IL-1β, IL-6, TNF-α and other catabolic factors, such as MMP-13, can accelerate apoptosis and matrix degradation of chondrocytes. Inhibition of inflammation is therefore an important part of preventing OA development. In our study, we have detected through both ELISA and WB experiments that malat1 reduces the expression of the inflammatory factor IL-6 in the IL-1β induced OA cell model through EVs. Minqiu et al. found that SFC-miR-126-3p-Exos inhibited apoptosis and inflammation of articular cartilage and chondrocyte degradation in an SD rat model of OA (Zhou et al., 2021). In another study, Shipin Zhang et also researchers and finds that MSC EVs can effectively alleviate temporomandibular joint osteoarthritis by attenuating inflammation and restoring matrix homeostasis. Jiaping Wu et al. found reported lncRNA malat-1 inhibited inflammation of microglia through the miR-154-5p/AQP9 axis (Wu et al., 2020). In this study, we examined the effect of hMSCsmalat−1-EVs on OA inflammation and found that hMSCsmalat−1-EVs can reduce IL-6 levels in the OA model, in agreement with the results of Jiyong Zhang et al. (Zhang et al., 2020) Ruizhang et al. found that lncRNA malat-1 in EVs promoted proliferation and migration of non-small cell lung cancer cells (Zhang et al., 2017). In our study, chondrocyte proliferation decreased significantly, and increased apoptosis and decreased migration capacity were observed, after IL-1β treatment. After treatment with hMSCs-EVs and hMSCsmalat−1-EVs, the negative effect of IL-1β was ameliorated, and the function and vitality of chondrocytes were restored. Moreover, comparison of the two EVs revealed that hMSCsmalat−1-EVs had a better therapeutic effect, which was consistent with our original hypothesis. These results indicated that EVs promoted chondrocyte proliferation, inhibited apoptosis, and enhanced the migration ability of chondrocytes via lncRNA malat-1.
Inflammatory cytokines, such as IL-1β, IL-6, TNF-α, and others, promote the production of MMPs and aggravate the progression of OA (Goldring and Otero, 2011). MMPs are involved in the degradation of various proteins in the ECM. MMP-13 is an important member of the MMPs family, which plays an important role in degrading collagen type II in OA articular cartilage and bones, and is highly expressed in OA patients but barely expressed in healthy people (Burrage et al., 2006). MMP-13 is the main key enzyme of cartilage degeneration and can degrade the ECM. MMP-13 is considered as one of the major contributing factors in the onset of OA (Wan et al., 2020), hence inhibiting MMP-13 expression could represent a new strategy to prevent OA. Xue Chen and others found that EVs secreted by MSCs inhibited the expression of MMP-13 in the traumatic arthritis model by microRNA-136-5p targeting ELF3 (Chen et al., 2011). In the inflammatory injury model of OA generated in this work, we also found that chondrocytes highly expressed MMP-13 after IL-1β treatment, and the effect of hMSCsmalat−1-EVs on MMP-13 was further explored. We found that both hMSCs-EVs and hMSCsmalat−1-EVs downregulated IL-1β-induced MMP-13 increase, but hMSCsmalat−1-EVs appeared to have a stronger protective effect. This result is consistent with our hypothesis, suggesting that hMSCsmalat−1-EVs provide better protection to the ECM and might be a more promising treatment of OA than hMSCs-EVs. Similarly, our animal experiments showed that hMSCsmalat−1-EVs significantly delayed the degeneration of the articular cartilage in SD rats. In this study, we did not investigate the protein changes following hMSCsmalat−1-EVs uptake by chondrocytes, which is a topic that will be investigated in future studies.
Conclusion
All together, our results based on an IL-1β induced OA model, indicate that EVs derived from hMSCs with lncRNA malat-1 overexpression promote chondrocyte proliferation and migration, suppress inflammation, degradation of the ECM, and appear to have a good protective effect in OA, providing a new potential therapeutic option for the prevention and treatment of OA.
Data Availability Statement
The raw data supporting the conclusions of this article will be made available by the authors, without undue reservation.
Ethics Statement
The animal study was reviewed and approved by Institutional Animal Care and Use Committee of Nanchang University.
Author Contributions
XC, CP, CY, and WH: conception and design. TW: provision of study materials. QC, CP, and BL: collection and assembly of data. CP and WH: data analysis and interpretation. XC, CP, JX, and GY: manuscript writing. All authors final approval of manuscript.
Funding
This research was supported by the Double Thousand Plan Foundation of Jiangxi Province Human Resources And Social Security Department (Grant number: G/Y2577). Key Intervertebral Disc Laboratory of Jiangxi Province (Grant number: 2020ACBL206012).
Conflict of Interest
The authors declare that the research was conducted in the absence of any commercial or financial relationships that could be construed as a potential conflict of interest.
Publisher’s Note
All claims expressed in this article are solely those of the authors and do not necessarily represent those of their affiliated organizations, or those of the publisher, the editors and the reviewers. Any product that may be evaluated in this article, or claim that may be made by its manufacturer, is not guaranteed or endorsed by the publisher.
Supplementary Material
The Supplementary Material for this article can be found online at: https://www.frontiersin.org/articles/10.3389/fbioe.2021.772002/full#supplementary-material
References
Batrakova, E. V., and Kim, M. S. (2015). Using Exosomes, Naturally-Equipped Nanocarriers, for Drug Delivery. J. Control. Release, 396–405. doi:10.1016/j.jconrel.2015.07.030
Breitbach, M., Bostani, T., and Roell, W. (2007). Potential Risks of Bone Marrow Cell Transplantation into Infarcted Hearts. Blood 110, 1362–1369. doi:10.1182/blood-2006-12-063412
Burrage, P. S., and Mix, K. S. (2006). Mix and C E Brinckerhoff, Matrix Metalloproteinases: Role in Arthritis. Front. Biosci. : a J. virtual Libr. 11, 529–543. doi:10.2741/1817
Chen, X., Shi, Y., Xue, P., Zheng, J., Ma, X., Li, J., et al. (2020). Mesenchymal Stem Cell-Derived Exosomal microRNA-136-5p Inhibits Chondrocyte Degeneration in Traumatic Osteoarthritis by Targeting ELF3. Arthritis Res. Ther. 22, 256. doi:10.1186/s13075-020-02325-6
Chen, Y., Chen, Y., Zhang, S., Du, X., and Bai, B. (2016). Parathyroid Hormone-Induced Bone Marrow Mesenchymal Stem Cell Chondrogenic Differentiation and its Repair of Articular Cartilage Injury in Rabbits. Med. Sci. Monit. Basic Res. 22, 132–145. doi:10.12659/msmbr.900242
Colombo, M., Raposo, G., and Thery, C. (2014). Biogenesis, Secretion, and Intercellular Interactions of Exosomes and Other Extracellular Vesicles. Annu. Rev. Cel Dev Biol 30, 255–289. doi:10.1146/annurev-cellbio-101512-122326
Desando, G., Cavallo, C., Sartoni, F., Martini, L., Parrilli, A., Veronesi, F., et al. (2013). Intra-articular Delivery of Adipose Derived Stromal Cells Attenuates Osteoarthritis Progression in an Experimental Rabbit Model. Arthritis Res. Ther. 15, R22. doi:10.1186/ar4156
Eymard, F., Pigenet, A., Citadelle, D., Beneli, C., Chevalier, X., Houard, X., et al. (2014). Induction of an Inflammatory and Prodegradative Phenotype in Autologous Fibroblast-like Synoviocytes by the Infrapatellar Fat Pad from Patients with Knee Osteoarthritis. Arthritis Rheumatol. (Hoboken, N.J). 66, 2165–2174. doi:10.1002/art.38657
Felson, D. T., Lawrence, R. C., Dieppe, P. A., Jorden, J. M., Sharma, L., Zhang, Y., et al. (2000). Osteoarthritis: New Insights. Part 1: the Disease and its Risk Factors. Ann. Intern. Med. 133, 635–646. doi:10.7326/0003-4819-133-8-200010170-00016
Foers, A. D., Chatfield, S., and Dagley, L. F. (2018). Enrichment of Extracellular Vesicles from Human Synovial Fluid Using Size Exclusion Chromatography. J. Extracell Vesicles 7, 1490145. doi:10.1080/20013078.2018.1490145
Fu, S., Wang, Y., Li, H., Chen, L., and Liu, Q. (2020). Regulatory Networks of LncRNA MALAT-1 in Cancer. Cancer Manag. Res. 12, 10181–10198. doi:10.2147/cmar.s276022
Fu, X., Liu, G., Halim, A., Yung, J., Qing, L., and Guanbin, S. (2019). Mesenchymal Stem Cell Migration and Tissue Repair. Cells 8, 784. doi:10.3390/cells8080784
Furlani, D., Ugurlucan, M., and Ong, L. (2009). Is the Intravascular Administration of Mesenchymal Stem Cells Safe? Mesenchymal Stem Cells and Intravital Microscopy. Microvasc. Res. 77, 370–376. doi:10.1016/j.mvr.2009.02.001
Glyn-Jones, S., Palmer, A. J. R., and Agricola, R. (2015). Osteoarthritis. Lancet, 376–387. doi:10.1016/s0140-6736(14)60802-3
Goldring, M. B., and Otero, M. (2011). Inflammation in Osteoarthritis. Curr. Opin. Rheumatol. 23, 471–478. doi:10.1097/BOR.0b013e328349c2b1
Guilak, F., Nims, R. J., Dicks, A., Wu, C. L., and Meulenbelt, I. (2018). Osteoarthritis as a Disease of the Cartilage Pericellular Matrix. Matrix Biol. 71, 40–50. doi:10.1016/j.matbio.2018.05.008
Gutschner, T., Hammerle, M., Eissmann, M., Hsu, J., Kim, Y., Hung, G., et al. (2013). The Noncoding RNA MALAT1 Is a Critical Regulator of the Metastasis Phenotype of Lung Cancer Cells. Cancer Res. 73, 1180–1189. doi:10.1158/0008-5472.can-12-2850
Hutchinson, J. N., Ensminger, A. W., Clemson, C. M., Lynch, C. R., Lawrence, J. B., and Andrew, C. (2007). A Screen for Nuclear Transcripts Identifies Two Linked Noncoding RNAs Associated with SC35 Splicing Domains. BMC Genomics 8, 39. doi:10.1186/1471-2164-8-39
Kalluri, R., and LeBleu, V. S. (2020). The Biology, Function, and Biomedical Applications of Exosomes. Science 367, eaau6977. doi:10.1126/science.aau6977
Kong, X., Wang, J., Cao, Y., Zhang, H., Lu, X., Wang, Y., et al. (2019). The Long Noncoding RNA MALAT-1 Functions as a Competing Endogenous RNA to Regulate MSL2 Expression by Sponging miR-338-3p in Myasthenia Gravis. J. Cell. Biochem. 120, 5542–5550. doi:10.1002/jcb.27838
Li, H., Xie, S., and Li, H. (2020). LncRNA MALAT1 Mediates Proliferation of LPS Treated-Articular Chondrocytes by Targeting the miR-146a-PI3K/Akt/mTOR axis. Life Sci. 254, 116801. doi:10.1016/j.lfs.2019.116801
Li, H., Xie, S., Li, H., Zhang, R., and Zhang, H. (2020). LncRNA MALAT1 Mediates Proliferation of LPS Treated-Articular Chondrocytes by Targeting the miR-146a-PI3K/Akt/mTOR axis. Life Sci. 254, 116801. doi:10.1016/j.lfs.2019.116801
Li, L., Duan, X., and Fan, Z. (2018). Mesenchymal Stem Cells in Combination with Hyaluronic Acid for Articular Cartilage Defects. Scientific Rep. 8, 9900. doi:10.1038/s41598-018-27737-y
Li, Z., Wang, Y., Xiao, K., Weng, X., Xiang, S., and Li, Z. (2018). Emerging Role of Exosomes in the Joint Diseases. Cell Physiol. Biochem. : Int. J. Exp. Cell. Physiol. Biochem. Pharmacol. 47, 2008–2017. doi:10.1159/000491469
Linero, I., and Chaparro, O. (2014). Paracrine Effect of Mesenchymal Stem Cells Derived from Human Adipose Tissue in Bone Regeneration. PloS one 9, e107001. doi:10.1371/journal.pone.0107001
Mao, Q., Liang, X. L., Zhang, C. L., Pang, Y. H., and Lu, Y. X. (2019). LncRNA KLF3-AS1 in Human Mesenchymal Stem Cell-Derived Exosomes Ameliorates Pyroptosis of Cardiomyocytes and Myocardial Infarction through miR-138-5p/Sirt1 axis. Stem Cel. Res. Ther. 10, 393. doi:10.1186/s13287-019-1522-4
Matas, J., Orrego, M., Amenabar, D., Infante, C., Tapia-Limonchi, R., Cadiz, M. I., et al. (2019). Umbilical Cord-Derived Mesenchymal Stromal Cells (MSCs) for Knee Osteoarthritis: Repeated MSC Dosing Is Superior to a Single MSC Dose and to Hyaluronic Acid in a Controlled Randomized Phase I/II Trial. Stem Cell Transl Med 8, 215–224. doi:10.1002/sctm.18-0053
Puthanveetil, P., Chen, S., Feng, B., and Gautam, A. (2015). Long Non-coding RNA MALAT1 Regulates Hyperglycaemia Induced Inflammatory Process in the Endothelial Cells. J. Cell. Mol. Med., 1418–1425. doi:10.1111/jcmm.12576
He, T., He, L., Xing, J., Fan, L., Liu, C., Wu, D., et al. (2020). Bone Marrow Mesenchymal Stem Cell-Derived Exosomes Protect Cartilage Damage and Relieve Knee Osteoarthritis Pain in a Rat Model of Osteoarthritis. Stem Cel. Res. Ther. 11, 276. doi:10.1186/s13287-020-01781-w
Tao, S. C., Yuan, T., and Zhang, Y. L. (2017). Exosomes Derived from miR-140-5p-Overexpressing Human Synovial Mesenchymal Stem Cells Enhance Cartilage Tissue Regeneration and Prevent Osteoarthritis of the Knee in a Rat Model. Theranostics 7, 180–195. doi:10.7150/thno.17133
Wan, Y., Li, W., Liao, Z., Chen, X., Yan, M., Tang, Z., et al. (2020). Selective MMP-13 Inhibitors: Promising Agents for the Therapy of Osteoarthritis. Curr. Med. Chem. 27, 3753–3769. doi:10.2174/0929867326666181217153118
Wang, X., Wang, C., Gou, W., Xu, X., Wang, Y., Wang, A., et al. (2018). The Optimal Time to Inject Bone Mesenchymal Stem Cells for Fracture Healing in a Murine Model. Stem Cel. Res. Ther., 272. doi:10.1186/s13287-018-1034-7
Washietl, S., Kellis, M., and Garber, M. (2014). Evolutionary Dynamics and Tissue Specificity of Human Long Noncoding RNAs in Six Mammals. Genome Res. 24, 616–628. doi:10.1101/gr.165035.113
Wu, J., Wang, C., and Ding, H. (2020). LncRNA MALAT1 Promotes Neuropathic Pain Progression through the miR1545p/AQP9 axis in CCI Rat Models. Mol. Med. Rep. 21, 291–303. doi:10.3892/mmr.2019.10829
Yang, H., Liang, N., Wang, M., Fei, Y., Sun, J., Li, Z., et al. (2017). Long Noncoding RNA MALAT-1 Is a Novel Inflammatory Regulator in Human Systemic Lupus Erythematosus. Oncotarget 8, 77400–77406. doi:10.18632/oncotarget.20490
Yang, R. C., Zhu, X. L., Wang, J., Wan, F., Lin, Y., Zhu, B., et al. (2018). Bone Marrow Mesenchymal Stem Cells Attenuate the Progression of Focal Segmental Glomerulosclerosis in Rat Models. BMC Nephrol. 19, 335. doi:10.1186/s12882-018-1137-5
Yang, X., Yang, J., Lei, P., and Wen, T. (2019). LncRNA MALAT1 Shuttled by Bone Marrow-Derived Mesenchymal Stem Cells-Secreted Exosomes Alleviates Osteoporosis through Mediating microRNA-34c/SATB2 axis. Aging 11, 8777–8791. doi:10.18632/aging.102264
Zhang, J., Rong, Y., Luo, C., and Cui, W. (2020). Bone Marrow Mesenchymal Stem Cell-Derived Exosomes Prevent Osteoarthritis by Regulating Synovial Macrophage Polarization. Aging 12, 25138–25152. doi:10.18632/aging.104110
Zhang, M., Liu, D., Li, S., Zhang, Y., Chang, L., Sun, F., et al. (2015). Bone Marrow Mesenchymal Stem Cell Transplantation Retards the Natural Senescence of Rat Hearts. Stem Cell Transl Med 4, 494–502. doi:10.5966/sctm.2014-0206
Zhang, R., Xia, Y., Wang, Z., Zheng, J., Chen, Y., Li, X., et al. (2017). Serum Long Non Coding RNA MALAT-1 Protected by Exosomes Is Up-Regulated and Promotes Cell Proliferation and Migration in Non-small Cell Lung Cancer. Biochem. Biophys. Res. Commun. 19, 406–414. doi:10.1016/j.bbrc.2017.06.055
Zhou, Y., Ming, J., Li, Y., Li, B., Li, J., Liu, S., et al. (2021). Exosomes Derived from miR-126-3p-Overexpressing Synovial Fibroblasts Suppress Chondrocyte Inflammation and Cartilage Degradation in a Rat Model of Osteoarthritis. Cel Death Discov. 7, 37. doi:10.1038/s41420-021-00418-y
Keywords: mesenchymal stem cells, chondrocyte, osteoarthritis, inflammation, extracellular vesicles
Citation: Pan C, Huang W, Chen Q, Xu J, Yao G, Li B, Wu T, Yin C and Cheng X (2021) LncRNA Malat-1 From MSCs-Derived Extracellular Vesicles Suppresses Inflammation and Cartilage Degradation in Osteoarthritis. Front. Bioeng. Biotechnol. 9:772002. doi: 10.3389/fbioe.2021.772002
Received: 07 September 2021; Accepted: 30 November 2021;
Published: 15 December 2021.
Edited by:
Enrico Ragni, Galeazzi Orthopedic Institute (IRCCS), ItalyReviewed by:
Jehan J. El-Jawhari, Nottingham Trent University, United KingdomSusana G. Santos, Universidade do Porto, Portugal
Copyright © 2021 Pan, Huang, Chen, Xu, Yao, Li, Wu, Yin and Cheng. This is an open-access article distributed under the terms of the Creative Commons Attribution License (CC BY). The use, distribution or reproduction in other forums is permitted, provided the original author(s) and the copyright owner(s) are credited and that the original publication in this journal is cited, in accordance with accepted academic practice. No use, distribution or reproduction is permitted which does not comply with these terms.
*Correspondence: Xigao Cheng, MjI4MjA2ODQ2QHFxLmNvbQ==