- 1Institute of Bio-inspired Structure and Surface Engineering, College of Mechanical and Electrical Engineering, Nanjing University of Aeronautics and Astronautics, Nanjing, China
- 2Department of Functional Morphology and Biomechanics, Zoological Institute, Kiel University, Kiel, Germany
- 3Embodied Artificial Intelligence and Neurorobotics Laboratory, SDU Biorobotics, The Mærsk Mc-Kinney Møller Institute, University of Southern Denmark, Odense M, Denmark
Developing climbing robots for smooth vertical surfaces (e.g., glass) is one of the most challenging problems in robotics. Here, the adequate functioning of an adhesive foot is an essential factor for successful locomotion performance. Among the various technologies (such as dry adhesion, wet adhesion, magnetic adhesion, and pneumatic adhesion), bio-inspired dry adhesion has been actively studied and successfully applied to climbing robots. Thus, this review focuses on the characteristics of two different types of foot microstructures, namely spatula-shaped and mushroom-shaped, capable of generating such adhesion. These are the most used types of foot microstructures in climbing robots for smooth vertical surfaces. Moreover, this review shows that the spatula-shaped feet are particularly suitable for massive and one-directional climbing robots, whereas mushroom-shaped feet are primarily suitable for light and all-directional climbing robots. Consequently, this study can guide roboticists in selecting the right adhesive foot to achieve the best climbing ability for future robot developments.
1 Introduction
Bio-inspired climbing robots have been widely studied over the past 10 years. [Daltorio et al. (2005); Unver et al. (2005); Daltorio et al. (2006); Daltorio et al. (2007a); Daltorio et al. (2007b); Santos et al. (2008); Sameoto et al. (2008); Menon et al. (2008); Daltorio et al. (2008); Wile et al. (2008b); Wile et al. (2008a); Daltorio et al. (2009); Li et al. (2012); Seitz et al. (2014); Tavakoli and Viegas (2015); Elbadawi et al. (2018); Schiller et al. (2019); Srisuchinnawong et al. (2019)]. An essential factor for climbing robots is adhesion [Silva et al. (2008)], a fundamental phenomenon in nature. Some animals can walk or climb vertical terrains and ceilings using adhesive feet, such as ladybugs, flies, spiders, and geckos. Biological adhesion can be classified into two types: wet and dry. Insects produce a liquid secretion from their feet to adhere to a substrate [Peisker and Gorb (2012); Kovalev et al. (2013); Peisker et al. (2014); Gilet et al. (2018)]. Gastropods adhere to a surface by generating a thin layer of pedal mucus on surfaces [Denny (1980a); Denny (1980b); Denny (1981)]. Harvestmen use viscoelastic fluids to capture small arthropods such as springtails [Wolff et al. (2014); Wolff et al. (2016)]. In particular, the viscoelastic fluids provide wet adhesion. By contrast, the dry adhesion in spiders and geckos is achieved by deformable setae with substrates, which generates an intermolecular adhesion force between the setae and surfaces [Arzt et al. (2003); Tian et al. (2006); Bhushan (2008); Autumn et al. (2014)]. In addition to deformable setae, Autumn et al. (2002) demonstrated that van der Waals forces are also responsible for the dry adhesion of gecko setae. The pads of beetles and flies are divided into setae with flat tips that resemble mushroom shapes or spatulate shapes. The pads of geckos and spiders consist of lamellae, subdivided into setae branches. Furthermore, the terminal element branches are widened and flattened at the tip, making them look like spatula shapes [Peressadko and Gorb (2004); Wolff and Gorb (2012a); Wolff and Gorb (2012b); Wolff and Gorb (2012c); Autumn et al. (2014); Wohlfart et al. (2014); Wolff and Gorb (2015); Frost et al. (2018)]. As the body of the animal increases, the terminal elements of the hairy attachment pads increase in number and density [Arzt et al. (2003); Figure 1]. This allows a more significant number of setae to touch the surface and create a substantial real area of contact. In other words, the real contact area and adhesion strength increase when the dimensions of the terminal elements decrease and their density increase.
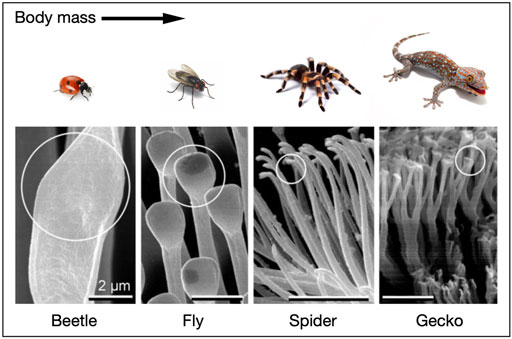
FIGURE 1. Terminal elements of the hairy attachment pads of a beetle, fly, spider, and gecko. The heavier animals show finer spatula structures [courtesy of Nate Abbott for the image of the gecko and modified from Arzt et al. (2003) for images fo the spatula-like terminal elements].
Recently, several types of artificial adhesive feet have been developed based on bio-inspiration studies, such as mechanical adhesion (gripping), pneumatic adhesion (suction cups), magnetic adhesion (permanent magnet), and dry adhesion (elastomer adhesive) [Daltorio et al. (2006); Kim et al. (2008); Hu et al. (2009); Seitz et al. (2014); Hawkes et al. (2015); Xu et al. (2016); Jiang and Xu (2018)]; Elbadawi et al. (2018); Chattopadhyay and Ghoshal (2018). In particular, bio-inspired dry adhesion has been actively studied and applied to climbing robots on smooth vertical surfaces. Therefore, in this review, we focus on bio-inspired adhesive feet for such surfaces. In this regard, two different widely used types of adhesive feet exist spatula-shaped feet (Kim et al. (2008)) and mushroom-shaped feet [Peressadko and Gorb (2004); Daltorio et al. (2006)], both approaches are synthetic reversible adhesive tapes. Each method has advantages and disadvantages depending on the robot’s mass, climbing direction, attachment, detachment, and reusability. A review of current approaches of these two types of adhesives could guide future improvement of robots climbing up smooth vertical surfaces.
2 Bio-Inspired Adhesive Feet on Climbing Robots
Synthetic adhesive feet inspired by animals are suitable for climbing robots. Using adhesive feet, robots can walk on steep slopes and vertical surfaces (Figure 2). These surfaces determine the adhesion technologies for the feet, such as magnetic adhesion on ferromagnetic surfaces, mechanical gripping on trees/pipes, and suction/dry adhesion on glass [Chattopadhyay and Ghoshal (2018)]. Spatula-shaped and mushroom-shaped feet are most commonly used in climbing robots, e.g., StickyBot [Santos et al. (2008)], StickyBot I; [Kim et al. (2008)], StickyBot III [Hawkes et al. (2011)], Geckobot [Unver et al. (2005)], Gecko-Inspired Soft Robot [Schiller et al. (2019)], Abigaille I [Menon et al. (2008)], Abigaille II [Li et al. (2012)], Tailless Gecko Robot [Srisuchinnawong et al. (2019)], 9 g climber [Hawkes et al. (2015)], Mini-WhegsTM7 [Daltorio et al. (2006)], Waalbot II [Murphy et al. (2011)], Wall and Ceiling Climbing Quadruped Robot [Ko et al. (2017)], and Gecko-Inspired Climbing Robot [Shao et al. (2020)]. Thus, this review mainly focuses on bio-inspired adhesive feet for smooth vertical surfaces such as glass.
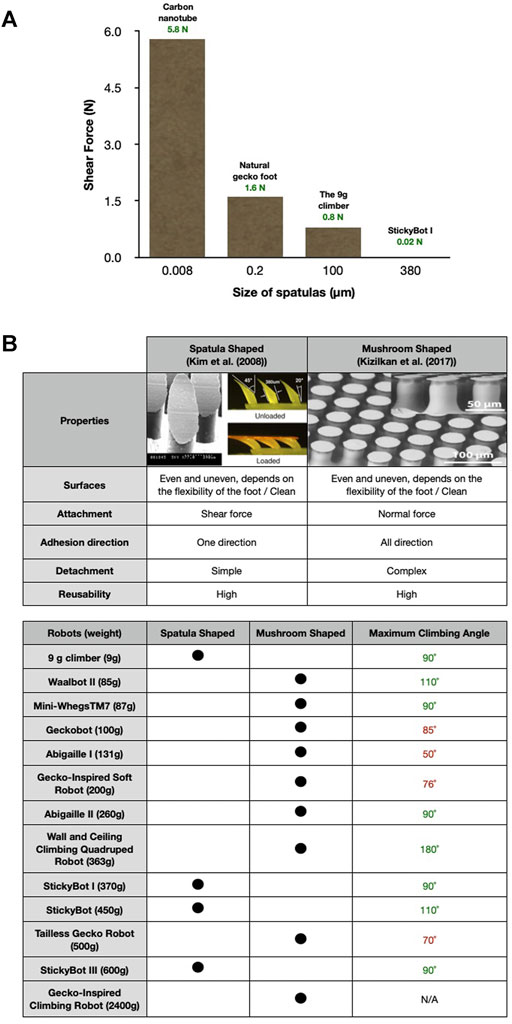
FIGURE 2. (A) The comparison of shear forces between each spatula size of the carbon-nanotube-based synthetic gecko tapes, natural gecko foot, StickyBot I, and the 9 g climber in a 0.16 cm2 area [Ge et al. (2007); Santos et al. (2008); Hawkes et al. (2015)] (B) Characteristics of adhesive materials with spatula-shaped and mushroom-shaped microstructures.
2.1 Spatula-Shaped
A synthetic dry adhesive inspired by geckoes, directional polymer stalks (DPSs), was designed and manufactured to create a directional adhesive similar to that in gecko feet. DPSs are made from polyurethane (Innovative Polymers, IE-20 AH polyurethane, 20 Shore-A hardness, E ≈ 300 kPa). DPSs comprise an array of micro-spatula-shaped polymer features [Kim et al. (2008); Figure 2B]. The spatula-shaped feet are soft materials that are marginally sticky. Moreover, geometric properties were determined empirically, drawing considering the shapes of gecko setae. Moreover, the DPSs make contact without a high normal preload. The sharp and thin tip shape of the DPS is designed to create a softer sufficient stiffness when pulled parallel to the terrains [Kim et al. (2008)]. The adhesion disappears by pushing or lifting against the shear force. The adhesion force depends on the polymer type, adequate direction, and size of the spatulas. If we consider the spatula size in these robots, Stickybot I have a larger size (380 μm) than the 9 g climber (100 μm) [Kim et al. (2008); Hawkes et al. (2015)]. Stickybot I could not lift over 100% of its body weight [Hawkes et al. (2015)] while, the 9 g climber could climb up a smooth vertical surface while hoisting 1000% of its body weight [Hawkes et al. (2015)]. In contrast to the aforementioned robot spatula-shaped foot, the natural gecko foot has about half a million setae, each of which contains hundreds to thousands of spatulas. The spatulas have an average diameter of 200 nm and an estimated adhesion force of 0.4 μN[(Autumn et al. (2000); Persson and Gorb (2003); Ge et al. (2007)]. Carbon-nanotube-based synthetic gecko tapes consist of thousands of synthetic spatulas with an average diameter of approximately 8 nm which can generate strong adhesion forces. They can adhere more than a natural gecko foot nearly ten times (∼ 100 Ncm−2) [Ge et al. (2007); Qu et al. (2008); Schaber et al. (2015)]. We observe that a smaller size of the spatulas allows for a higher adhesion, as shown in Figure 2A. Furthermore, the feet can be reconditioned by cleaning with soap and water [Kim et al. (2008)]. Therefore, they can be continuously used several times.
2.2 Mushroom-Shaped
The mushroom-shaped adhesive microstructure (MSAMS)—inspired by the attachment systems of beetles from the family Chrysomelidae—was made from polyvinyl siloxane (PVS) with a hexagonal patterning height of approximately 100 μm and a base diameter of 60 μm. The adhesive covers almost half of the contact area of the material [Gorb S. et al. (2007); Figure 2B]. The MSAMS makes contact with the preload along the normal force axis and can be detached by the peeling technique at some angles [Shao et al. (2020)]. The adhesion of MSAMS has approximately twice the pull-off force of surface without MSAMS (flat surface) made from the same material, while both were independent of the preload. The MSAMS has more repeatability than flat specimen in the peel strength [Gorb S. et al. (2007)]. A demonstration of its potential showed that a 20 cm × 20 cm tape supported a weight of approximately 70 kg [Heepe et al. (2012)]. The MSAMS also provides adhesion underwater [Heepe et al. (2012); Heepe and Gorb (2014); Ko et al. (2017)] and has no directional adhesion [Murphy et al. (2011); Seibel et al., 2020)]; therefore, it is one of the most used methods for smooth vertical surface climbing robots. Furthermore, the tape can be reconditioned by cleaning with soap and water [Gorb S. N. et al. (2007)]. Therefore, it can be continuously used multiple times.
3 Discussion
Spatula- and mushroom-shaped microstructures can be used on both even and uneven, clean surfaces because of their flexibility [Kim et al. (2008); Gorb S. et al. (2007)]. The attachment system of spatula-shaped feet is very simple. They require only shear force to attach to surfaces [Hawkes et al. (2015)]. By contrast, mushroom-shaped feet require a slight initial normal force to attach to surfaces [Kim et al. (2008); Shao et al. (2020)]. The detachment mechanism of the spatula-shaped microstructure is also straightforward. The adhesion force disappears when the feet are pushed or lifted against the shear force. The system in mushroom-shaped feet is more complicated than the spatula-shaped. The former requires a specific peeling angle for detachment [Shao et al. (2020)].
Furthermore, the climbing direction of the spatula-shaped feet is limited to one-directional adhesion; therefore, robots can climb up to 90 and 110° depending on the foot orientation [Santos et al. (2008)]. In contrast, mushroom-shaped feet provide all-directional adhesion; hence, robots can climb on the ceiling [Murphy et al. (2011); Ko et al. (2017)]. The reusability of the spatula-shaped feet is impressive. Some Stickybot feet with a spatula-shaped structure have been continuously used for over 6 months without significant performance loss [Kim et al. (2008)]. In addition, cleaning the feet with soap and water before use can recondition the adhesion ability [Kim et al. (2008)]. However, mushroom-shaped feet are relatively less reusable. The detachment system damages the structure from time to time [Gorb S. et al. (2007)]. However, cleaning the feet before use could also recondition the adhesion ability (Figure 2B).
4 Conclusion
Both spatula-shaped feet and mushroom-shaped feet have advantages and disadvantages. The substrate surface, robot mass, climbing direction, adhesion force, and reusability are the primary factors to consider when choosing adequate feet. Spatula-shaped feet are suitable for heavy climbing robots as they provide an adhesion force on a shear force axis. In contrast, mushroom-shaped feet provide an adhesion force on the normal force axis. For instance, the 9 g climber could climb while hoisting 1 kg up on a smooth vertical surface [Hawkes et al. (2015)], while the maximum slope climbing angle of the tailless gecko robot (500 g) was 70° [Srisuchinnawong et al. (2019)]. Moreover, the mushroom-shaped feet could climb in all directions because the foot structure provides all-directional adhesion [Murphy et al. (2011); Li et al. (2012)]. However, spatula-shaped feet provide only one direction of adhesion. If robots intend to adhere to overhanging surfaces or ceilings, they should have the ability to change their foot orientation [Santos et al. (2008)]. Finally, reusability is also essential. In this regard, spatula-shaped feet have apparently higher reusability [Gorb S. et al. (2007); Kim et al. (2008)]. However, cleaning the feet before use can restore the adhesion ability of both types of microstructure.
Author Contributions
PB and PM organized and prepared all this manuscript. PB wrote the manuscript. PM and SG reviewed and edited the manuscript. AJ and ZD contributed to the research background. All authors contributed to the article and approved the submitted version.
Funding
This work was supported by NSFC (Grant No. 51861135306) (PM, AJ), German Science Foundation (DFG, GO 995/38-1) (SG), and the National Key R and D Program of China (2020YFB1313504) (PM, ZD).
Conflict of Interest
The authors declare that the research was conducted in the absence of any commercial or financial relationships that could be construed as a potential conflict of interest.
Publisher’s Note
All claims expressed in this article are solely those of the authors and do not necessarily represent those of their affiliated organizations, or those of the publisher, the editors and the reviewers. Any product that may be evaluated in this article, or claim that may be made by its manufacturer, is not guaranteed or endorsed by the publisher.
References
Arzt, E., Gorb, S., and Spolenak, R. (2003). From Micro to Nano Contacts in Biological Attachment Devices. Proc. Natl. Acad. Sci. 100, 10603–10606. doi:10.1073/pnas.1534701100
Autumn, K., Liang, Y. A., Hsieh, S. T., Zesch, W., Chan, W. P., Kenny, T. W., et al. (2000). Adhesive Force of a Single Gecko Foot-Hair. Nature 405, 681–685. doi:10.1038/35015073
Autumn, K., Niewiarowski, P. H., and Puthoff, J. B. (2014). Gecko Adhesion as a Model System for Integrative Biology, Interdisciplinary Science, and Bioinspired Engineering. Annu. Rev. Ecol. Evol. Syst. 45, 445–470. doi:10.1146/annurev-ecolsys-120213-091839
Autumn, K., Sitti, M., Liang, Y. A., Peattie, A. M., Hansen, W. R., Sponberg, S., et al. (2002). Evidence for van der waals adhesion in gecko setae. Proc. Natl. Acad. Sci. 99, 12252–12256. doi:10.1073/pnas.192252799
Bhushan, B. (2008).Gecko Feet: Natural Hairy Attachment Systems for Smart Adhesion–Mechanism, Modeling and Development of Bio-Inspired Materials. In Nanotribology and Nanomechanics. Springer, 1073–1134.
Chattopadhyay, P., and Ghoshal, S. K. (2018). Adhesion Technologies of Bio-Inspired Climbing Robots: a Survey. Int. J. Robotics Automation 33. doi:10.2316/journal.206.2018.6.206-5193
Daltorio, K. A., Gorb, S., Peressadko, A., Horchler, A. D., Ritzmann, R. E., and Quinn, R. D. (2006).A Robot that Climbs walls Using Micro-structured Polymer Feet. In Climbing and walking robots. Springer, 131–138. doi:10.1007/3-540-26415-9_15
Daltorio, K. A., Gorb, S., Peressadko, A., Horchler, A. D., Wei, T. E., Ritzmann, R. E., et al. (2007a). Microstructured Polymer Adhesive Feet for Climbing Robots. MRS Bull. 32, 504–508. doi:10.1557/mrs2007.85
Daltorio, K. A., Horchler, A. D., Gorb, S., Ritzmann, R. E., and Quinn, R. D. (2005).A Small wall-walking Robot with Compliant, Adhesive Feet. In 2005 IEEE/RSJ International Conference on Intelligent Robots and Systems. IEEE, 3648–3653. doi:10.1109/iros.2005.1545596
Daltorio, K. A., Wei, T. E., Gorb, S. N., Ritzmann, R. E., and Quinn, R. D. (2007b).Passive Foot Design and Contact Area Analysis for Climbing Mini-Whegs. In Proceedings 2007 IEEE International Conference on Robotics and Automation. IEEE, 1274–1279. doi:10.1109/robot.2007.363160
Daltorio, K. A., Wei, T. E., Horchler, A. D., Southard, L., Wile, G. D., Quinn, R. D., et al. (2009). Mini-whegs Tm Climbs Steep Surfaces Using Insect-Inspired Attachment Mechanisms. Int. J. Robotics Res. 28, 285–302. doi:10.1177/0278364908095334
Daltorio, K. A., Witushynsky, T. C., Wile, G. D., Palmer, L. R., Ab Malek, A., Ahmad, M. R., et al. (2008).A Body Joint Improves Vertical to Horizontal Transitions of a wall-climbing Robot. In 2008 IEEE International Conference on Robotics and Automation. IEEE, 3046–3051. doi:10.1109/robot.2008.4543673
Denny, M. (1980a). Locomotion: the Cost of Gastropod Crawling. Science 208, 1288–1290. doi:10.1126/science.208.4449.1288
Denny, M. (1980b). The Role of Gastropod Pedal Mucus in Locomotion. Nature 285, 160–161. doi:10.1038/285160a0
Denny, M. W. (1981). A Quantitative Model for the Adhesive Locomotion of the Terrestrial Slug, Ariolimax Columbianus. J. Exp. Biol. 91, 195–217. doi:10.1242/jeb.91.1.195
Elbadawi, M., Andrikopoulos, G., Nikolakopoulos, G., and Gustafsson, T. (2018).Bio-inspired Climbing Robots in Wet Environments: Recent Trends in Adhesion Methods and Materials. In 2018 IEEE International Conference on Robotics and Biomimetics (ROBIO). IEEE, 2347–2353. doi:10.1109/robio.2018.8665184
Frost, K. F., Gorb, S. N., and Wolff, J. O. (2018). Adhesion and Friction in Hunting Spiders: The Effect of Contact Splitting on Their Attachment Ability. Zoologischer Anzeiger 273, 231–239. doi:10.1016/j.jcz.2018.01.003
Ge, L., Sethi, S., Ci, L., Ajayan, P. M., and Dhinojwala, A. (2007). Carbon Nanotube-Based Synthetic Gecko tapes. Proc. Natl. Acad. Sci. 104, 10792–10795. doi:10.1073/pnas.0703505104
Gilet, T., Heepe, L., Lambert, P., Compère, P., and Gorb, S. N. (2018). Liquid Secretion and Setal Compliance: the Beetle's Winning Combination for a Robust and Reversible Adhesion. Curr. Opin. Insect Sci. 30, 19–25. doi:10.1016/j.cois.2018.08.002
Gorb, S. N., Sinha, M., Peressadko, A., Daltorio, K. A., and Quinn, R. D. (2007b). Insects Did it First: a Micropatterned Adhesive Tape for Robotic Applications. Bioinspir. Biomim. 2, S117–S125. doi:10.1088/1748-3182/2/4/s01
Gorb, S., Varenberg, M., Peressadko, A., and Tuma, J. (2007a). Biomimetic Mushroom-Shaped Fibrillar Adhesive Microstructure. J. R. Soc. Interf. 4, 271–275. doi:10.1098/rsif.2006.0164
Hawkes, E. W., Christensen, D. L., and Cutkosky, M. R. (2015).Vertical Dry Adhesive Climbing with a 100× Bodyweight Payload. In 2015 IEEE International Conference on Robotics and Automation (ICRA). IEEE, 3762–3769.
Hawkes, E. W., Ulmen, J., Esparza, N., and Cutkosky, M. R. (2011).Scaling walls: Applying Dry Adhesives to the Real World. In 2011 IEEE/RSJ International Conference on Intelligent Robots and Systems. IEEE, 5100–5106. doi:10.1109/iros.2011.6095103
Heepe, L., and Gorb, S. N. (2014). Biologically Inspired Mushroom-Shaped Adhesive Microstructures. Annu. Rev. Mater. Res. 44, 173–203. doi:10.1146/annurev-matsci-062910-100458
Heepe, L., Kovalev, A. E., Varenberg, M., Tuma, J., and Gorb, S. N. (2012). First Mushroom-Shaped Adhesive Microstructure: a Review. Theor. Appl. Mech. Lett. 2, 014008. doi:10.1063/2.1201408
Hu, B., Wang, L., Zhao, Y., and Fu, Z. (2009). A Miniature wall Climbing Robot with Biomechanical Suction Cups. Ind. Robot: Int. J. doi:10.1108/01439910910994623
Jiang, Q., and Xu, F. (2018). Grasping Claws of Bionic Climbing Robot for Rough wall Surface: Modeling and Analysis. Appl. Sci. 8, 14.
Ko, H., Yi, H., and Jeong, H. E. (2017). Wall and Ceiling Climbing Quadruped Robot with superior Water Repellency Manufactured Using 3d Printing (Uniclimb). Int. J. Precis. Eng. Manuf.-Green Tech. 4, 273–280. doi:10.1007/s40684-017-0033-y
Kovalev, A. E., Filippov, A. E., and Gorb, S. N. (2013). Insect Wet Steps: Loss of Fluid from Insect Feet Adhering to a Substrate. J. R. Soc. Interf. 10, 20120639. doi:10.1098/rsif.2012.0639
Li, Y., Ahmed, A., Sameoto, D., and Menon, C. (2012). Abigaille Ii: toward the Development of a Spider-Inspired Climbing Robot. Robotica 30, 79–89. doi:10.1017/s0263574711000373
Menon, C., Li, Y., Sameoto, D., and Martens, C. (2008).Abigaille-i: towards the Development of a Spider-Inspired Climbing Robot for Space Use. In 2008 2nd IEEE RAS & EMBS International Conference on Biomedical Robotics and Biomechatronics. IEEE, 384–389. doi:10.1109/biorob.2008.4762903
Murphy, M. P., Kute, C., Mengüç, Y., and Sitti, M. (2011). Waalbot Ii: Adhesion Recovery and Improved Performance of a Climbing Robot Using Fibrillar Adhesives. Int. J. Robotics Res. 30, 118–133. doi:10.1177/0278364910382862
Peisker, H., and Gorb, S. N. (2012). Evaporation Dynamics of Tarsal Liquid Footprints in Flies (Calliphora Vicina)and Beetles (Coccinella septempunctata). J. Exp. Biol. 215, 1266–1271. doi:10.1242/jeb.065722
Peisker, H., Heepe, L., Kovalev, A. E., and Gorb, S. N. (2014). Comparative Study of the Fluid Viscosity in Tarsal Hairy Attachment Systems of Flies and Beetles. J. R. Soc. Interf. 11, 20140752. doi:10.1098/rsif.2014.0752
Peressadko, A., and Gorb, S. N. (2004). When Less Is More: Experimental Evidence for Tenacity Enhancement by Division of Contact Area. J. Adhes. 80, 247–261. doi:10.1080/00218460490430199
Persson, B. N. J., and Gorb, S. (2003). The Effect of Surface Roughness on the Adhesion of Elastic Plates with Application to Biological Systems. J. Chem. Phys. 119, 11437–11444. doi:10.1063/1.1621854
Qu, L., Dai, L., Stone, M., Xia, Z., and Wang, Z. L. (2008). Carbon Nanotube Arrays with strong Shear Binding-On and Easy normal Lifting-Off. Science 322, 238–242. doi:10.1126/science.1159503
Sameoto, D., Li, Y., and Menon, C. (2008). Multi-scale Compliant Foot Designs and Fabrication for Use with a Spider-Inspired Climbing Robot. J. Bionic Eng. 5, 189–196. doi:10.1016/s1672-6529(08)60024-4
Sangbae Kim, S., Spenko, M., Trujillo, S., Heyneman, B., Santos, D., and Cutkosky, M. R. (2008). Smooth Vertical Surface Climbing with Directional Adhesion. IEEE Trans. Robot. 24, 65–74. doi:10.1109/tro.2007.909786
Santos, D., Heyneman, B., Kim, S., Esparza, N., and Cutkosky, M. R. (2008).Gecko-inspired Climbing Behaviors on Vertical and Overhanging Surfaces. In 2008 IEEE International Conference on Robotics and Automation. IEEE, 1125–1131. doi:10.1109/robot.2008.4543355
Schaber, C. F., Heinlein, T., Keeley, G., Schneider, J. J., and Gorb, S. N. (2015). Tribological Properties of Vertically Aligned Carbon Nanotube Arrays. Carbon 94, 396–404. doi:10.1016/j.carbon.2015.07.007
Schiller, L., Seibel, A., and Schlattmann, J. (2019). Toward a Gecko-Inspired, Climbing Soft Robot. Front. Neurorobot. 13, 106. doi:10.3389/fnbot.2019.00106
Seibel, A., Yıldız, M., and Zorlubaş, B. (2020). A Gecko-Inspired Soft Passive Gripper. Biomimetics 5, 12. doi:10.3390/biomimetics5020012
Seitz, B. F., Goldberg, B., Doshi, N., Ozcan, O., Christensen, D. L., Hawkes, E. W., et al. (2014).Bio-inspired Mechanisms for Inclined Locomotion in a Legged Insect-Scale Robot. In 2014 IEEE International Conference on Robotics and Biomimetics (ROBIO 2014). IEEE, 791–796. doi:10.1109/robio.2014.7090428
Shao, D., Chen, J., Ji, A., Dai, Z., and Manoonpong, P. (2020) IEEE, 578–585. doi:10.1109/robosoft48309.2020.9116045Hybrid Soft-Rigid Foot with Dry Adhesive Material Designed for a Gecko-Inspired Climbing Robot2020 3rd IEEE International Conference on Soft Robotics (RoboSoft).
Silva, M. F., Machado, J. T., and Tar, J. K. (2008) IEEE, 127–132. doi:10.1109/icccyb.2008.4721392A Survey of Technologies for Climbing Robots Adhesion to Surfaces2008 IEEE International Conference on Computational Cybernetics
Srisuchinnawong, A., Shao, D., Ngamkajornwiwat, P., Teerakittikul, P., Dai, Z., Ji, A., et al. (2019) IEEE, 468–473. doi:10.1109/icar46387.2019.8981580Neural Control for Gait Generation and Adaptation of a Gecko Robot2019 19th International Conference on Advanced Robotics (ICAR)
Tavakoli, M., and Viegas, C. (2015).Bio-inspired Climbing Robots. In Biomimetic Technologies. Elsevier, 301–320. doi:10.1016/b978-0-08-100249-0.00014-8
Tian, Y., Pesika, N., Zeng, H., Rosenberg, K., Zhao, B., McGuiggan, P., et al. (2006). Adhesion and Friction in Gecko Toe Attachment and Detachment. Proc. Natl. Acad. Sci. 103, 19320–19325. doi:10.1073/pnas.0608841103
Unver, O., Murphy, M., and Sitti, M. (2005). Geckobot and Waalbot: Small-Scale wall Climbing Robots. Infotech@ Aerospace, 6940. doi:10.2514/6.2005-6940
Wile, G. D., Daltorio, K. A., Diller, E. D., Palmer, L. R., Gorb, S. N., Ritzmann, R. E., et al. (2008a).Screenbot: Walking Inverted Using Distributed Inward Gripping. In 2008 IEEE/RSJ International Conference on Intelligent Robots and Systems. IEEE, 1513–1518. doi:10.1109/iros.2008.4651045
Wile, G. D., Daltorio, K. A., Palmer, L. R., Witushynsky, T. C., Southard, L., Ahmad, M. R., et al. (2008b).Making Orthogonal Transitions with Climbing Mini-whegsTM. In 2008 IEEE International Conference on Robotics and Automation. IEEE, 1775–1776.
Wohlfart, E., Wolff, J. O., Arzt, E., and Gorb, S. N. (2014). The Whole Is More Than the Sum of All its Parts: Collective Effect of Spider Attachment Organs. J. Exp. Biol. 217, 222–224. doi:10.1242/jeb.093468
Wolff, J. O., and Gorb, S. N. (2015). Adhesive Foot Pads: an Adaptation to Climbing? an Ecological Survey in Hunting Spiders. Zoology 118, 1–7. doi:10.1016/j.zool.2014.04.006
Wolff, J. O., and Gorb, S. N. (2012a). Comparative Morphology of Pretarsal Scopulae in Eleven Spider Families. Arthropod Struct. Dev. 41, 419–433. doi:10.1016/j.asd.2012.04.004
Wolff, J. O., and Gorb, S. N. (2012c). Surface Roughness Effects on Attachment Ability of the Spider Philodromus Dispar (Araneae, Philodromidae). J. Exp. Biol. 215, 179–184. doi:10.1242/jeb.061507
Wolff, J. O., and Gorb, S. N. (2012b). The Influence of Humidity on the Attachment Ability of the Spider Philodromus Dispar (Araneae, Philodromidae). Proc. R. Soc. B. 279, 139–143. doi:10.1098/rspb.2011.0505
Wolff, J. O., Schönhofer, A. L., Martens, J., Wijnhoven, H., Taylor, C. K., and Gorb, S. N. (2016). The Evolution of Pedipalps and Glandular Hairs as Predatory Devices in Harvestmen (Arachnida, Opiliones). Zool J. Linn. Soc. 177, 558–601. doi:10.1111/zoj.12375
Wolff, J. O., Schönhofer, A. L., Schaber, C. F., and Gorb, S. N. (2014). Gluing the 'unwettable': Soil-Dwelling Harvestmen Use Viscoelastic Fluids for Capturing Springtails. J. Exp. Biol. 217, 3535–3544. doi:10.1242/jeb.108852
Keywords: bio-inspired climbing robots, smooth vertical surfaces, adhesive foot, spatula-shaped, mushroom-shaped
Citation: Borijindakul P, Ji A, Dai Z, Gorb SN and Manoonpong P (2021) Mini Review: Comparison of Bio-Inspired Adhesive Feet of Climbing Robots on Smooth Vertical Surfaces. Front. Bioeng. Biotechnol. 9:765718. doi: 10.3389/fbioe.2021.765718
Received: 27 August 2021; Accepted: 20 September 2021;
Published: 30 September 2021.
Edited by:
Yi-Chen Ethan Li, Feng Chia University, TaiwanReviewed by:
Jie Ju, Henan University, ChinaElena Pierro, University of Basilicata, Italy
Giuseppe Carbone, Politecnico di Bari, Italy
Copyright © 2021 Borijindakul , Ji , Dai , Gorb and Manoonpong . This is an open-access article distributed under the terms of the Creative Commons Attribution License (CC BY). The use, distribution or reproduction in other forums is permitted, provided the original author(s) and the copyright owner(s) are credited and that the original publication in this journal is cited, in accordance with accepted academic practice. No use, distribution or reproduction is permitted which does not comply with these terms.
*Correspondence: Pongsiri Borijindakul , a29iZWthbmdAbnVhYS5lZHUuY24=; Poramate Manoonpong , cG9tYUBudWFhLmVkdS5jbg==