- 1Department of Clinical Laboratory, Longhua Hospital, Shanghai University of Traditional Chinese Medicine, Shanghai, China
- 2Eye Institute and Department of Ophthalmology, Eye and ENT Hospital, Fudan University, Shanghai, China
- 3Institutes of Brain Science, State Key Laboratory of Medical Neurobiology and MOE Frontiers Center for Brain Science, Fudan University, Shanghai, China
- 4NHC Key Laboratory of Myopia, Fudan University, Shanghai, China
- 5Key Laboratory of Myopia, Chinese Academy of Medical Sciences, Shanghai, China
- 6Shanghai Key Laboratory of Visual Impairment and Restoration, Shanghai, China
VEGF165, a regulator of angiogenesis, has been widely used as a serum biomarker for a number of human diseases, including cancer, rheumatoid arthritis, bronchial asthma, and diabetic eye disease. The rapid, accurate, and convenient detection of VEGF165 is a crucial step in effective healthcare monitoring, disease diagnosis, and prognosis assessment. In this study, a fluorescent aptasensor based on an assembled G-quadruplex and the signal molecule ThT was developed for VEGF165 detection. First, G-rich DNA fragments were assembled at both ends of the anti-VEGF165 aptamer, and the B-DNA form was converted into a G-quadruplex structure aptamer (G4-Apt). Then, ThT was introduced, and the G-quadruplex significantly enhanced the fluorescence intensity of the bound ThT. When VEGF165 was present, the higher affinity of the aptamer to the target protein allowed the G4-Apt/VEGF165 complex to form and release ThT, which emitted only weak fluorescence in the free state. Therefore, the aptasensor exhibited a good linear detection window from 1.56 to 25 nM VEGF165, with a limit of detection of 0.138 nM. In addition, the aptasensor was applied to detect VEGF165 in clinical serum samples, showing good accuracy, reproducibility, and stability. These results indicate that our developed fluorescent aptasensor can potentially be a reliable, convenient, and cost-effective approach for the sensitive, specific, and rapid detection of the VEGF165 biomarker.
Introduction
Vascular endothelial growth factor (VEGF) is a strong endothelial-cell-specific mitogen that plays central roles in the regulation of physiologic and pathologic angiogenesis (Greenberg and Jin, 2004). It can bind to extracellular receptor tyrosine kinases and activate different intracellular signaling pathways, which will in turn stimulate vascular endothelial cell growth, survival, and proliferation (Olsson et al., 2006). In mammals, the VEGF family consists of five subgroups: VEGF-A, VEGF-B, VEGF-C, VEGF-D, and the placenta growth factor (Goel and Mercurio, 2013; Simons et al., 2016; Rud’ko et al., 2017). VEGF-A is the prototype member, with at least four isoforms: VEGF121, VEGF165, VEGF189, and VEGF206. Among these, VEGF165 is the most potent pro-angiogenic isoform and has been widely used as a biomarker for several human diseases, including cancer, rheumatoid arthritis, bronchial asthma, and diabetic eye disease (Nakahara et al., 2003; Niu and Chen, 2010; Paradowska-Gorycka et al., 2015; Tsai et al., 2015; Wong et al., 2016). Moreover, VEGF165 levels also significantly differ in various stages of human disease. For example, the serum of patients with diabetes, non-proliferative diabetic retinopathy, and proliferative diabetic retinopathy contains higher levels of VEGF165 compared with serum from healthy individuals (Manaviat et al., 2011; Ahuja et al., 2019; Abu-Yaghi et al., 2020; Wang et al., 2020). Therefore, accurate, sensitive, and rapid detection of the disease-specific biomarker VEGF165 in bodily fluids may serve as an effective tool for healthcare monitoring as well as disease diagnosis and prognosis.
Currently, various VEGF165 detection techniques based on immunoassays have been reported, including immunohistochemistry, radioimmunoassays, and enzyme-linked immunosorbent assays (ELISAs) (Anthony et al., 1997; Goncalves et al., 2007; Takahashi et al., 2016). Although these traditional immunological methods provide invaluable insights for the analysis of VEGF165, they are labor-intensive and time-consuming and require cumbersome operating protocols, which limit their application in real-time clinical diagnosis. In recent years, aptamer-based biosensors have gained strong clinical application prospects for the detection of disease-specific biomarkers (Zhang et al., 2018; Gao S. et al., 2020; Chinnappan et al., 2021; Gao et al., 2021; Liu et al., 2021; Xu et al., 2021). An aptamer, defined as a chemical antibody, is a functional single-stranded DNA or RNA oligonucleotide that can bind to a target with high affinity and specificity (Ellington and Szostak, 1990; Tuerk and Gold, 1990). It can be obtained by in vitro SELEX technology and folded into a unique three-dimensional structure, such as G-quadruplex, B-DNA, Z-DNA, and i-motif, to perform its recognition, diagnosis, and treatment functions (Yuan et al., 2017; Gao L. et al., 2020; Hassani et al., 2020; Manibalan et al., 2020; Xu et al., 2020; Li et al., 2021). Compared with antibodies, aptamers have significant advantages as novel recognition molecules (Radom et al., 2013; Vigneshvar et al., 2016; Zhou and Rossi, 2017). For example, they have a wide range of targets, low immunogenicity, and low toxicity. They can also be prepared by chemical synthesis in large batches without the need to immunize animals. Additionally, aptamers are easy to modify and label, inexpensive, and less affected by environmental factors, such as pH and temperature. This allows for easy long-term storage and transportation. Because of these advantages, many aptasensors based on surface plasmon resonance, surface-enhanced Raman scattering, and electrochemical workstations have been reported for VEGF165 detection (Zhao et al., 2015; Xu et al., 2017; Dehghani et al., 2018; Fu et al., 2020; Lee et al., 2020). However, expensive sensing equipment, complicated operation procedures, and high reliance on professional operators are the main hindrances that limit their point-of-care testing in clinical applications.
In this study, a simple, fast, accurate, and cost-effective aptasensor was established for VEGF165 detection using an assembled G-quadruplex and the signal molecule thioflavin T (ThT). First, the anti-VEGF165 aptamer with a B-DNA form was successfully assembled into a G-quadruplex structure aptamer (G4-Apt). Then, the fluorescent dye ThT was introduced, which acts as a signal indicator by specifically binding to the G-quadruplex. The G-quadruplex could significantly enhance the fluorescence intensity of the bound ThT. When VEGF165 was present, the higher affinity of the aptamer to the target protein promoted the transformation of the G4-Apt/ThT to the G4-Apt/VEGF165 complex. The bound ThT was simultaneously released, and it emitted only weak fluorescence in the free state. Therefore, the developed fluorescent aptasensor exhibited a linear detection window, from 1.56 to 25 nM; good sensitivity, with a limit of detection (LOD) of 0.138 nM; and a high target specificity for VEGF165. Furthermore, the aptasensor was applied to detect VEGF165 in spiked serum samples. A recovery percentage of 95.33–109.18% and a coefficient of variation of 3.39–7.69% were obtained, suggesting that the proposed detection system has good accuracy, reproducibility, and stability. Therefore, the developed aptasensor offers a reliable, convenient, and cost-effective approach for the sensitive, specific, and rapid detection of the VEGF165 biomarker.
Materials and Methods
Materials and Reagents
The anti-VEGF165 sequence (aptamer: 5′-CCGTCTTCCAGACAAGAGTGCAGGG -3′) and the assembled sequence (G4-Apt: 5′-AGGGACGGGACCGTCTTCCAGACAAGAGTGCAGGGAGGGACGGGA-3′) in this study were synthesized by Sangon Biotech Co., Ltd. (Shanghai, China). Recombinant human VEGF165 protein and VEGF165 ELISA kits were purchased from Sino Biological Inc. (Beijing, China). Human serum albumin (HSA), streptavidin (SA), bovine serum albumin (BSA), adenosine triphosphate (ATP), and thioflavin T (ThT) were obtained from Sigma-Aldrich Co. LLC (United States). Gliotoxin (GTX) was purchased from Puhuashi Technology Development Co., Ltd. (Beijing, China). SA biosensors were obtained from ForteBio (Menlo Park, CA). All solutions were prepared using Milli-Q ultrapure water (Millipore, Billerica, MA).
Binding Affinity Determination by Biolayer Interferometry
In this study, the binding affinity of the aptamer sequence was measured using biolayer interferometry in an Octet RED96 system (ForteBio, Shanghai). The principle and analysis procedures were detailed in our previously reported protocol (Gao et al., 2019b; Gao et al., 2019c). All steps were performed at 25°C with shaking at 1,000 rpm in a 96-well plate containing 200 μL of binding buffer in each well. The response data obtained from the reaction surface were normalized by subtracting the signal simultaneously acquired from the reference surface to eliminate nonspecific binding and a buffer-induced interferometry spectrum shift using Octet Data Analysis Software CFR Part 11 Version 10.0.0.5. Finally, the affinity parameter, KD, was obtained.
Conformation Analysis by Circular Dichroism
The conformations of the aptamer and G4-Apt were analyzed using a Chirascan™ V100 Circular Dichroism (CD) Spectrometer. Before the assay, the chamber was deoxygenated with dry purified nitrogen (99.99%) and kept in the nitrogen atmosphere during experiments. Each CD spectrum was collected from 320 to 220 nm, representing the accumulation of two scans at 50 nm/min, with a 1-nm bandwidth and a time-per-point of 0.5 s.
VEGF165 Detection by the Fluorescent Aptasensor
This study was approved by the Ethics Committee of the Eye and ENT Hospital of Fudan University, and consent was obtained from all participants. Aptasensor preparation and VEGF165 detection were conducted according to the following procedure. G4-Apt was diluted to a concentration of 2.5 μM and then heated to 95 °C for 10 min, cooled in an ice bath for 5 min, and kept at room temperature for 10 min. The 25 μL of each aliquot G4-Apt was added to 50 μL of ThT (5 μM), and the mixture was incubated for 30 min. Subsequently, 25 μL of VEGF165 at different concentrations was added, and the mixture was incubated at room temperature for another 40 min. Finally, the fluorescence spectra were recorded immediately by a Spark® Multimode Microplate Reader (Tecan Trading AG) at 25°C. The fluorescence emission spectra of the developed detection system were monitored from 450 to 600 nm, with an excitation wavelength of 390 nm. Fluorescence intensity at 490 nm was used for quantitative analysis.
Results and DISCUSSION
Construction and Characterization of G-Quadruplex Structure
As shown in Figure 1A, G-rich DNA fragments (AGGGACGGGA) were assembled at both ends of the anti-VEGF165 aptamer to construct a G-quadruplex structure aptamer (G4-Apt) (Potty et al., 2009; Gao H. et al., 2019; Gao et al., 2019c). The binding ability of the aptamer and assembled G4-Apt was first evaluated by biolayer interferometry (BLI). As shown in Figures 1B,C, various concentrations of VEGF165 (100, 50, 25, 12.5, and 6.25 nM) were analyzed for both an association time and a dissociation time of over 5 min, along with a blank sample containing only BSA for reference. The BLI results suggest that the aptamer and G4-Apt can strongly bind to VEGF165, with binding affinities of 0.287 and 0.538 nM, respectively. Although the aptamer was modified using G-rich DNA fragments, the G4-Apt maintained a consistent strong affinity and specificity for VEGF165. To further analyze the conformational changes, the tertiary structures of the aptamer and G4-Apt were determined by circular dichroism (CD). In Figure 1D, the CD spectrum shows that the aptamer folded into a B-DNA structure according to a positive peak at 276 nm and a negative peak at 240 nm. Compared with the CD spectrum of the aptamer, the G4-Apt largely possessed a parallel G-quadruplex structure, with a characteristic positive peak at 262 nm and a negative peak at 240 nm (Figure 1E). Therefore, an anti-VEGF165 aptamer with a B-DNA form was successfully assembled into a G-quadruplex structure that maintained binding ability at the sub-nanomolar level.
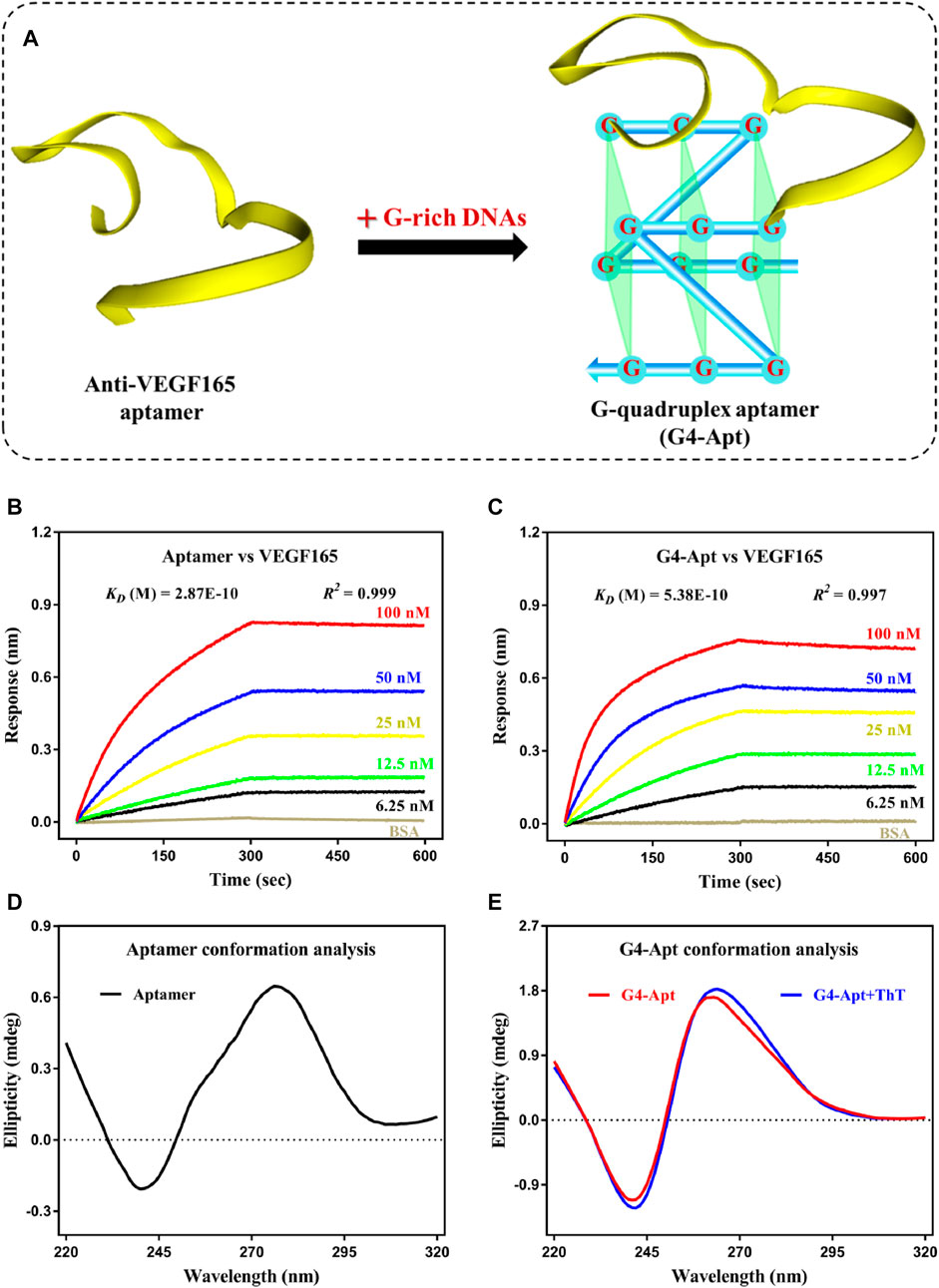
FIGURE 1. (A) Schematic diagram of the construction of the G-quadruplex aptamer. (B,C) Affinity and specificity analysis of the anti-VEGF165 aptamer and the assembled G4-Apt by biolayer interferometry. (D,E) Conformation analysis of the anti-VEGF165 aptamer and the assembled G4-Apt by circular dichroism.
Detection Mechanism of the Fluorescent Aptasensor
ThT is a commercially available benzothiazole cationic dye with good chemical stability, ideal water solubility, excellent discrimination, and a low-fluorescence background in the free state. It exhibits highly specific interactions with G-quadruplexes through intercalation, groove-binding, and end stacking, and further leads to a significant fluorescence enhancement effect (Mohanty et al., 2013; de la Faverie et al., 2014). On this basis, ThT was introduced in this study as a signal indicator for construction of the aptasensor. The BLI interaction results show that ThT did not bind to the aptamer (Figure 2A) and had a moderately applicable binding force to G4-Apt (KD = 52.9 μM). Obviously, this is significantly lower than the binding affinity of G4-Apt to VEGF165 (KD = 0.538 nM). The CD spectrum suggests that the intensity and width of the characteristic absorption peak of G4-Apt increased in the presence of ThT (Figure 1E). These results indicate that ThT can not only specifically bind to G4-Apt but may also induce the formation of more G-quadruplex structures. Therefore, ThT was used as an ideal fluorescent signal indicator to construct the following detection system.
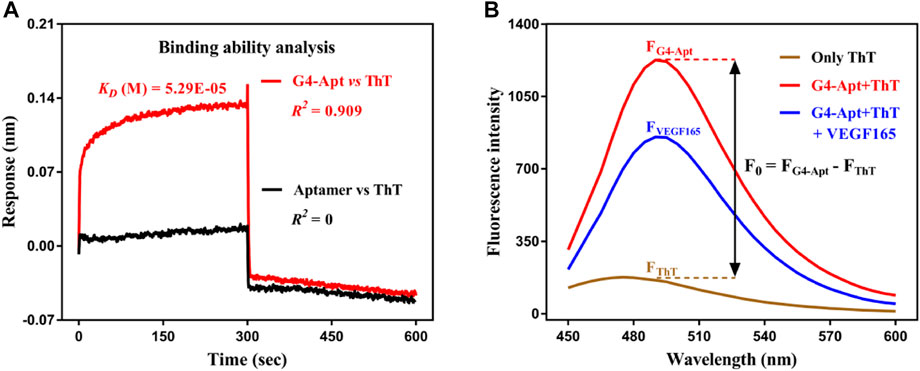
FIGURE 2. (A) Binding affinity analysis of the assembled G4-Apt and the aptamer to thioflavin T (ThT) by biolayer interferometry. (B) Fluorescence spectra of ThT in the free state (brown curve), ThT with G4-Apt (red curve), and ThT with G4-Apt and VEGF165 (blue curve).
To achieve rapid, sensitive, and effective detection of the VEGF165 biomarker, a fluorescent aptasensor based on the assembled G-quadruplex and ThT was developed. As shown in Figure 3, the G-rich DNA fragments assembled at both ends of the anti-VEGF165 aptamer formed the parallel G-quadruplex aptamer G4-Apt. When ThT was present, its fluorescence intensity was substantially enhanced through specific intercalation into the G-quadruplex (Figure 2B), while ThT emitted only weak fluorescence in the free state. When VEGF165 was added, because of the higher affinity of the aptamer to the target protein, it promoted the structural transformation of G4-Apt and resulted in loss of the G-quadruplex structure. The G4-Apt/VEGF165 binding complex was further formed, and ThT was released (Figure 3), which led to a significant decrease in the fluorescence intensity of the detection system (Figure 2B). Therefore, the efficiency of the change in the fluorescence intensity during this process could be used for detecting VEGF165.
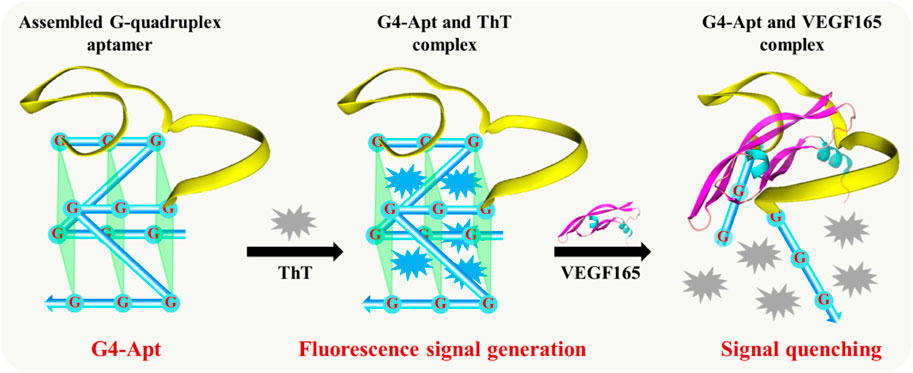
FIGURE 3. Illustration of the developed fluorescent aptasensor based on the assembled G-quadruplex aptamer and thioflavin T (ThT).
Parameter Optimization of the Fluorescent Aptasensor
To achieve the best detection efficiency of the fluorescent aptasensor, the concentration ratio of the G4-Apt to ThT was first studied in the detection system. Theoretically, a higher concentration of G4-Apt (high ratio) will result in a decrease in detection sensitivity, while a lower G4-Apt concentration will not have enough detection windows. To balance this effect, the optimal G4-Apt to ThT ratio was analyzed by titrating the G4-Apt concentration (ranging from 39.06 to 1,250 nM) with a fixed concentration of ThT. As shown in Figure 4A, as the G4-Apt concentration continued to increase, the available detection fluorescence also increased significantly. A turning point occurred when the G4-Apt concentration was 625 nM. Although a higher concentration of G4-Apt could produce a wider detection window, it might also greatly reduce the detection sensitivity. Therefore, a concentration of 625 nM G4-Apt was selected to balance the detection window and the sensitivity of the fluorescent aptasensor. In addition, it is important that the detection time allows VEGF165 to induce the structural conversion of the G-quadruplex and specifically bind to the aptamer to form the stable detection system. Generally, a longer detection time is conducive to the formation of a highly stable complex for a greater fluorescence change effect. However, a longer detection time will also reduce the application prospects of the aptasensor. To balance this influence, a binding time ranging from 5 to 60 min was evaluated. As shown in Figure 4B, the intensity of fluorescence change increased with higher VEGF165 incubation times, reaching equilibrium at 40 min. Therefore, 40 min was selected as the optimal detection time for VEGF165 in the following experiments.
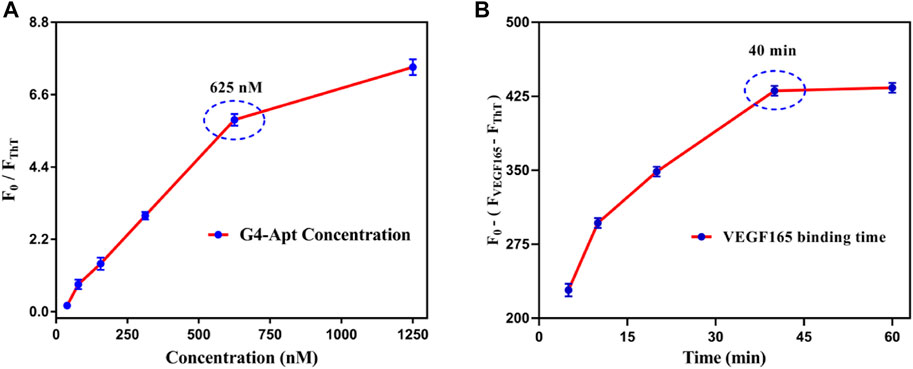
FIGURE 4. (A) Optimization of the concentration for the assembled G4-Apt. (B) Optimization of binding time for VEGF165 in the detection system.
Detection Range, Sensitivity, and Specificity of the Fluorescent Aptasensor
To further evaluate the detection of VEGF165 using the fluorescent aptasensor, dose-dependent changes in the signal range of 1.56–25 nM were investigated under optimal experimental conditions. As shown in Figure 5A, the intensity of the fluorescence spectrum gradually decreased in the presence of VEGF165. The aptasensor signal was quantified as
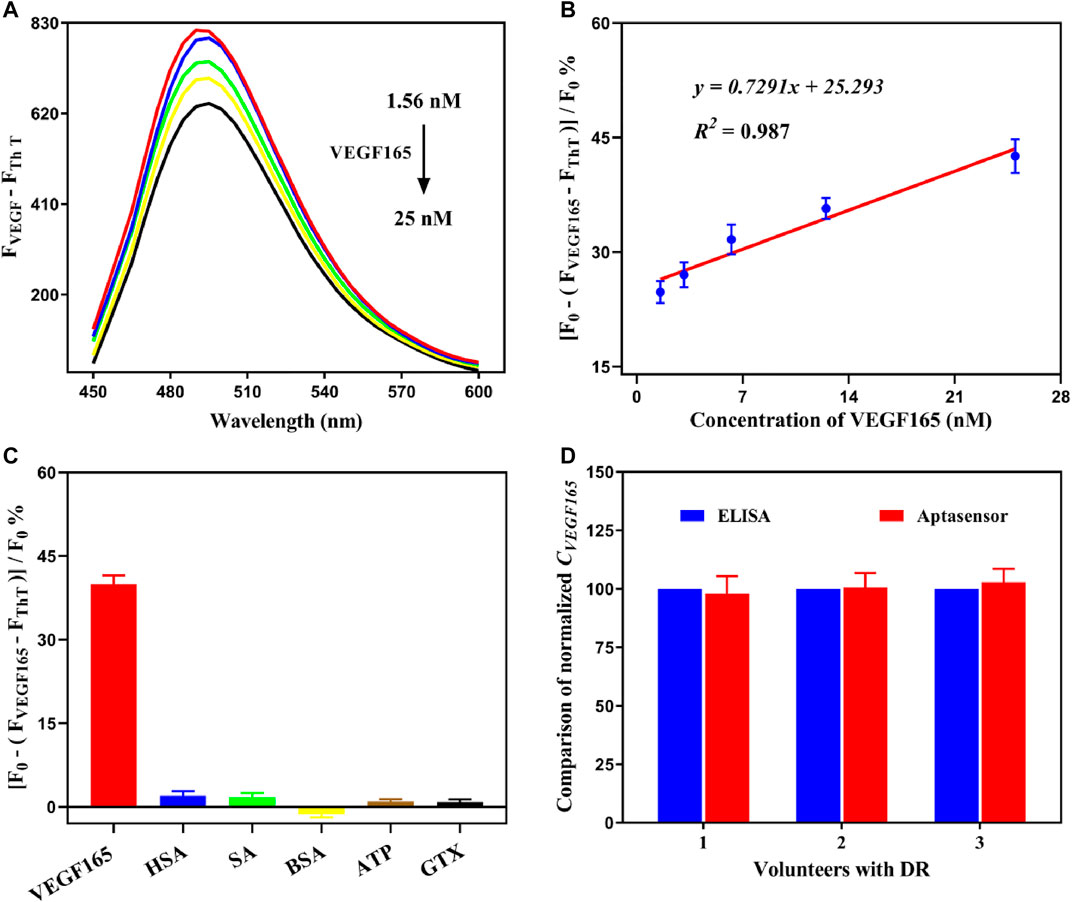
FIGURE 5. (A) Fluorescence spectra of the detection system at various concentrations of VEGF165. (B) Linear relationship between the aptasensor signal and the concentration of VEGF165 in the range of 1.56–25 nM. (C) Cross-reactivity experiments of the fluorescent aptasensor with HAS, SA, BSA, ATP, and GTX. The error bars represent the standard deviation of three independent measurements. (D) Comparison of the normalized concentration of VEGF165.
Detection of VEGF165 in Serum Samples by the Fluorescent Aptasensor
To further verify the effectiveness of our developed fluorescent aptasensor, free VEGF165 was added to the clinical serum sample and analyzed using the preset calibration curve. A good recovery percentage of 95.33–109.18% was obtained, indicating that the detection accuracy of the aptasensor was not significantly affected by clinical serum samples. The coefficient of variation range of 3.39–7.69% was acceptable (less than 10%), suggesting that the proposed detection system has high reproducibility and stability. In addition, the concentrated serum samples, which were obtained from diabetic retinopathy (DR) patients, were simultaneously analyzed using an ELISA kit and the fluorescent aptasensor. As shown in Figure 5D, the results were highly consistent, which indicates that our developed aptasensor can be reliably applied to actual sample analysis. Therefore, the developed fluorescent aptasensor has significant potential as a reliable, convenient, and cost-effective approach for the sensitive, specific, and rapid detection of the VEGF165 biomarker.
Conclusion
In this study, a reliable, convenient, and cost-effective aptasensor was established for the sensitive, specific, and rapid detection of VEGF165 biomarker using an assembled G-quadruplex and signal molecule ThT. The developed fluorescent aptasensor shows reliable diagnostic performance. Its sub-nanomolar LOD can provide sufficiently high sensitivity levels comparable to ELISA. Although ELISA is the most common method for biomarker detection, it requires at least 3 h to complete VEGF165 analysis, whereas our fluorescent aptasensor can be used to complete this quantification in about 1 h. Further comparisons between this work and previously reported aptasensors for analyzing VEGF165 are shown in Table 1. Compared with fluorescence-based aptasensors (Wang and Si, 2013; Wang et al., 2014), although they have similar detection windows and sensitivity, but the construction scheme of our aptasensor has universal structures that can broadly extend the development and application of biosensors based on the assembled G-quadruplex. Compared with sensors based on colorimetric, electrochemical, and surface plasmon resonance methodologies (Chen et al., 2014; Pan et al., 2017; Dong et al., 2020), the aptasensor is still insufficient in automation, visualization, and sensitivity. However, our developed aptasensor does not require enzyme labeling or rely on expensive equipment and professional operators. Its simple, convenient, and fast detection process makes it particularly suitable for the point-of-care testing needed for disease biomarkers. We believe that this aptasensor design may soon promote its clinical translational research in the future.
Data Availability Statement
The raw data supporting the conclusion of this article will be made available by the authors, without undue reservation.
Author Contributions
XZ, SG, JW, and XH contributed to conception and design of the study. XZ and SG wrote the first draft of the manuscript. All authors contributed to manuscript revision, read, and approved the submitted version.
Funding
This work was supported by the National Natural Science Foundation of China (No. 82071011) and Science and Technology Commission of Shanghai Municipality (Nos 21Y11909900, 19ZR1471900).
Conflict of Interest
The authors declare that the research was conducted in the absence of any commercial or financial relationships that could be construed as a potential conflict of interest.
Publisher’s Note
All claims expressed in this article are solely those of the authors and do not necessarily represent those of their affiliated organizations, or those of the publisher, the editors and the reviewers. Any product that may be evaluated in this article, or claim that may be made by its manufacturer, is not guaranteed or endorsed by the publisher.
References
Abu-Yaghi, N. E., Abu Tarboush, N. M., Abojaradeh, A. M., Al-Akily, A. S., Abdo, E. M., and Emoush, L. O. (2020). Relationship between Serum Vascular Endothelial Growth Factor Levels and Stages of Diabetic Retinopathy and Other Biomarkers. J. Ophthalmol. 2020, 8480193. doi:10.1155/2020/8480193
Ahuja, S., Saxena, S., Akduman, L., Meyer, C. H., Kruzliak, P., and Khanna, V. K. (2019). Serum Vascular Endothelial Growth Factor Is a Biomolecular Biomarker of Severity of Diabetic Retinopathy. Int. J. Retin. Vitr 5, 29. doi:10.1186/s40942-019-0179-6
Anthony, F. W., Evans, P. W., Wheeler, T., and Wood, P. J. (1997). Variation in Detection of VEGF in Maternal Serum by Immunoassay and the Possible Influence of Binding Proteins. Ann. Clin. Biochem. 34 (Pt 3), 276–280. doi:10.1177/000456329703400309
Chen, H., Hou, Y., Qi, F., Zhang, J., Koh, K., Shen, Z., et al. (2014). Detection of Vascular Endothelial Growth Factor Based on Rolling circle Amplification as a Means of Signal Enhancement in Surface Plasmon Resonance. Biosens. Bioelectron. 61, 83–87. doi:10.1016/j.bios.2014.05.005
Chinnappan, R., Zaghloul, N. S., AlZabn, R., Malkawi, A., Abdel Rahman, A., Abu-Salah, K. M., et al. (2021). Aptamer Selection and Aptasensor Construction for Bone Density Biomarkers. Talanta 224, 121818. doi:10.1016/j.talanta.2020.121818
Dehghani, S., Nosrati, R., Yousefi, M., Nezami, A., Soltani, F., Taghdisi, S. M., et al. (2018). Aptamer-based Biosensors and Nanosensors for the Detection of Vascular Endothelial Growth Factor (VEGF): A Review. Biosens. Bioelectron. 110, 23–37. doi:10.1016/j.bios.2018.03.037
Dong, J., He, L., Wang, Y., Yu, F., Yu, S., Liu, L., et al. (2020). A Highly Sensitive Colorimetric Aptasensor for the Detection of the Vascular Endothelial Growth Factor in Human Serum. Spectrochimica Acta A: Mol. Biomol. Spectrosc. 226, 117622. doi:10.1016/j.saa.2019.117622
Ellington, A. D., and Szostak, J. W. (1990). In Vitro Selection of RNA Molecules that Bind Specific Ligands. Nature 346 (6287), 818–822. doi:10.1038/346818a0
Fu, Y., Zou, K., Liu, M., Zhang, X., Du, C., and Chen, J. (2020). Highly Selective and Sensitive Photoelectrochemical Sensing Platform for VEGF165 Assay Based on the Switching of Photocurrent Polarity of CdS QDs by Porous Cu2O-CuO Flower. Anal. Chem. 92 (1), 1189–1196. doi:10.1021/acs.analchem.9b04319
Gao, H., Zhao, J., Huang, Y., Cheng, X., Wang, S., Han, Y., et al. (2019a). Universal Design of Structure-Switching Aptamers with Signal Reporting Functionality. Anal. Chem. 91 (22), 14514–14521. doi:10.1021/acs.analchem.9b03368
Gao, L., Teng, R., Zhang, S., Zhou, Y., Luo, M., Fang, Y., et al. (2020a). Zinc Ion-Stabilized Aptamer-Targeted Black Phosphorus Nanosheets for Enhanced Photothermal/Chemotherapy against Prostate Cancer. Front. Bioeng. Biotechnol. 8, 769. doi:10.3389/fbioe.2020.00769
Gao, S., Hu, W., Zheng, X., Cai, S., and Wu, J. (2019b). Functionalized Aptamer with an Antiparallel G-Quadruplex: Structural Remodeling, Recognition Mechanism, and Diagnostic Applications Targeting CTGF. Biosens. Bioelectron. 142, 111475. doi:10.1016/j.bios.2019.111475
Gao, S., Zhang, S., Sun, X., Zheng, X., and Wu, J. (2020b). Fluorescent Aptasensor Based on G-Quadruplex-Assisted Structural Transformation for the Detection of Biomarker Lipocalin 1. Biosens. Bioelectron. 169, 112607. doi:10.1016/j.bios.2020.112607
Gao, S., Zheng, X., Tang, Y., Cheng, Y., Hu, X., and Wu, J. (2019c). Development of a Fluorescently Labeled Aptamer Structure-Switching Assay for Sensitive and Rapid Detection of Gliotoxin. Anal. Chem. 91 (2), 1610–1618. doi:10.1021/acs.analchem.8b05094
Gao, S., Zheng, X., and Wu, J. (2021). A Graphene Oxide-Based Aptasensor for ANGPTL4 Biomarker Detection. Sensors Actuators B: Chem. 345, 130389. doi:10.1016/j.snb.2021.130389
Goel, H. L., and Mercurio, A. M. (2013). VEGF Targets the Tumour Cell. Nat. Rev. Cancer 13 (12), 871–882. doi:10.1038/nrc3627
Goncalves, V., Gautier, B., Garbay, C., Vidal, M., and Inguimbert, N. (2007). Development of a Chemiluminescent Screening Assay for Detection of Vascular Endothelial Growth Factor Receptor 1 Ligands. Anal. Biochem. 366 (1), 108–110. doi:10.1016/j.ab.2007.03.027
Greenberg, D. A., and Jin, K. (2004). VEGF and ALS: The Luckiest Growth Factor? Trends Mol. Med. 10 (1), 1–3. doi:10.1016/j.molmed.2003.11.006
Hassani, S., Rezaei Akmal, M., Salek Maghsoudi, A., Rahmani, S., Vakhshiteh, F., Norouzi, P., et al. (2020). High-Performance Voltammetric Aptasensing Platform for Ultrasensitive Detection of Bisphenol A as an Environmental Pollutant. Front. Bioeng. Biotechnol. 8, 574846. doi:10.3389/fbioe.2020.574846
Lee, J., Tatsumi, A., Tsukakoshi, K., Wilson, E. D., Abe, K., Sode, K., et al. (2020). Application of a Glucose Dehydrogenase-Fused with Zinc Finger Protein to Label DNA Aptamers for the Electrochemical Detection of VEGF. Sensors 20 (14), 3878. doi:10.3390/s20143878
Li, L., Xu, S., Yan, H., Li, X., Yazd, H. S., Li, X., et al. (2021). Nucleic Acid Aptamers for Molecular Diagnostics and Therapeutics: Advances and Perspectives. Angew. Chem. Int. Ed. 60 (5), 2221–2231. doi:10.1002/anie.202003563
Liu, Y., Xu, Q., Zhang, Y., Ren, B., Huang, L., Cai, H., et al. (2021). An Electrochemical Aptasensor Based on AuPt alloy Nanoparticles for Ultrasensitive Detection of Amyloid-β Oligomers. Talanta 231, 122360. doi:10.1016/j.talanta.2021.122360
Manaviat, M. R., Rashidi, M., Afkhami-Ardekani, M., Mohiti-Ardekani, J., and Bandala-Sanchez, M. (2011). Effect of Pan Retinal Photocoagulation on the Serum Levels of Vascular Endothelial Growth Factor in Diabetic Patients. Int. Ophthalmol. 31 (4), 271–275. doi:10.1007/s10792-011-9448-6
Manibalan, S., Shobana, A., Kiruthika, M., Achary, A., Swathi, M., Venkatalakshmi, R., et al. (2020). Protein Network Studies on PCOS Biomarkers with S100A8, Druggability Assessment, and RNA Aptamer Designing to Control its Cyst Migration Effect. Front. Bioeng. Biotechnol. 8, 328. doi:10.3389/fbioe.2020.00328
Mohanty, J., Barooah, N., Dhamodharan, V., Harikrishna, S., Pradeepkumar, P. I., and Bhasikuttan, A. C. (2013). Thioflavin T as an Efficient Inducer and Selective Fluorescent Sensor for the Human Telomeric G-Quadruplex DNA. J. Am. Chem. Soc. 135 (1), 367–376. doi:10.1021/ja309588h
Nakahara, H., Song, J., Sugimoto, M., Hagihara, K., Kishimoto, T., Yoshizaki, K., et al. (2003). Anti-Interleukin-6 Receptor Antibody Therapy Reduces Vascular Endothelial Growth Factor Production in Rheumatoid Arthritis. Arthritis Rheum. 48 (6), 1521–1529. doi:10.1002/art.11143
Niu, G., and Chen, X. (2010). Vascular Endothelial Growth Factor as an Anti-Angiogenic Target for Cancer Therapy. Cdt 11 (8), 1000–1017. doi:10.2174/138945010791591395
Olsson, A.-K., Dimberg, A., Kreuger, J., and Claesson-Welsh, L. (2006). VEGF Receptor Signalling ? in Control of Vascular Function. Nat. Rev. Mol. Cel Biol 7 (5), 359–371. doi:10.1038/nrm1911
Pan, L.-H., Kuo, S.-H., Lin, T.-Y., Lin, C.-W., Fang, P.-Y., and Yang, H.-W. (2017). An Electrochemical Biosensor to Simultaneously Detect VEGF and PSA for Early Prostate Cancer Diagnosis Based on Graphene oxide/ssDNA/PLLA Nanoparticles. Biosens. Bioelectron. 89 (Pt 1), 598–605. doi:10.1016/j.bios.2016.01.077
Paradowska-Gorycka, A., Pawlik, A., Malinowski, D., Romanowska-Prochnicka, K., Haladyj, E., Manczak, M., et al. (2015). AB0003 Relationship between Vegfa Gene Polymorphisms and Serum Vegf Protein Levels in Patients with Rheumatoid Arthritis. Ann. Rheum. Dis. 74, 1–892. doi:10.1136/annrheumdis-2015-eular.3274
Potty, A. S. R., Kourentzi, K., Fang, H., Jackson, G. W., Zhang, X., Legge, G. B., et al. (2009). Biophysical Characterization of DNA Aptamer Interactions with Vascular Endothelial Growth Factor. Biopolymers 91 (2), 145–156. doi:10.1002/bip.21097
Radom, F., Jurek, P. M., Mazurek, M. P., Otlewski, J., and Jeleń, F. (2013). Aptamers: Molecules of Great Potential. Biotechnol. Adv. 31 (8), 1260–1274. doi:10.1016/j.biotechadv.2013.04.007
Renaud de la Faverie, A., Guédin, A., Bedrat, A., Yatsunyk, L. A., and Mergny, J. L. (2014). Thioflavin T as a Fluorescence Light-Up Probe for G4 Formation. Nucleic Acids Res. 42 (8), e65. doi:10.1093/nar/gku111
Rud’ko, A. S., Efendieva, M. K., Budzinskaya, M. V., and Karpilova, M. A. (2017). Influence of Vascular Endothelial Growth Factor on Angiogenesis and Neurogenesis. Vestn. Oftal'mol. 133 (3), 75–81. doi:10.17116/oftalma2017133375-80
Simons, M., Gordon, E., and Claesson-Welsh, L. (2016). Mechanisms and Regulation of Endothelial VEGF Receptor Signalling. Nat. Rev. Mol. Cel Biol 17 (10), 611–625. doi:10.1038/nrm.2016.87
Takahashi, H., Nomura, Y., Nishida, J., Fujino, Y., Yanagi, Y., and Kawashima, H. (2016). Vascular Endothelial Growth Factor (VEGF) Concentration Is Underestimated by Enzyme-Linked Immunosorbent Assay in the Presence of Anti-VEGF Drugs. Invest. Ophthalmol. Vis. Sci. 57 (2), 462–466. doi:10.1167/iovs.15-18245
Tsai, M.-J., Wang, T.-N., Lin, Y.-S., Kuo, P.-L., Hsu, Y.-L., and Huang, M.-S. (2015). Aryl Hydrocarbon Receptor Agonists Upregulate VEGF Secretion from Bronchial Epithelial Cells. J. Mol. Med. 93 (11), 1257–1269. doi:10.1007/s00109-015-1304-0
Tuerk, C., and Gold, L. (1990). Systematic Evolution of Ligands by Exponential Enrichment: RNA Ligands to Bacteriophage T4 DNA Polymerase. Science 249 (4968), 505–510. doi:10.1126/science.2200121
Vigneshvar, S., Sudhakumari, C. C., Senthilkumaran, B., and Prakash, H. (2016). Recent Advances in Biosensor Technology for Potential Applications - an Overview. Front. Bioeng. Biotechnol. 4, 11. doi:10.3389/fbioe.2016.00011
Wang, J.-Y., Kwon, J.-S., Hsu, S.-M., and Chuang, H.-S. (2020). Sensitive Tear Screening of Diabetic Retinopathy with Dual Biomarkers Enabled Using a Rapid Electrokinetic Patterning Platform. Lab. Chip 20 (2), 356–362. doi:10.1039/c9lc00975b
Wang, S.-E., Huang, Y., Hu, K., Tian, J., and Zhao, S. (2014). A Highly Sensitive and Selective Aptasensor Based on Fluorescence Polarization for the Rapid Determination of Oncoprotein Vascular Endothelial Growth Factor (VEGF). Anal. Methods 6 (1), 62–66. doi:10.1039/c3ay41697f
Wang, S.-E., and Si, S. (2013). A Fluorescent Nanoprobe Based on Graphene Oxide Fluorescence Resonance Energy Transfer for the Rapid Determination of Oncoprotein Vascular Endothelial Growth Factor (VEGF). Appl. Spectrosc. 67 (11), 1270–1274. doi:10.1366/13-07071
Wong, T. Y., Cheung, C. M. G., Larsen, M., Sharma, S., and Simó, R. (2016). Diabetic Retinopathy. Nat. Rev. Dis. Primers 2, 16012. doi:10.1038/nrdp.2016.12
Xu, H., Kou, F., Ye, H., Wang, Z., Huang, S., Liu, X., et al. (2017). Highly Sensitive Antibody-Aptamer Sensor for Vascular Endothelial Growth Factor Based on Hybridization Chain Reaction and pH Meter/indicator. Talanta 175, 177–182. doi:10.1016/j.talanta.2017.04.073
Xu, Y., Jiang, X., Zhou, Y., Ma, M., Wang, M., and Ying, B. (2021). Systematic Evolution of Ligands by Exponential Enrichment Technologies and Aptamer-Based Applications: Recent Progress and Challenges in Precision Medicine of Infectious Diseases. Front. Bioeng. Biotechnol. 9, 704077. doi:10.3389/fbioe.2021.704077
Xu, Z., Zhang, L.-W., Long, L.-L., Zhu, S.-H., Chen, M.-L., Ding, L., et al. (2020). Metal Organic Frame-Upconverting Nanoparticle Assemblies for the FRET Based Sensor Detection of Bisphenol A in High-Salt Foods. Front. Bioeng. Biotechnol. 8, 626269. doi:10.3389/fbioe.2020.626269
Yuan, Q., Islam, N., and Wu, J. (2017). AC Electrokinetics Based Capture of Yeast Cells from Ultra‐Fast Through‐Flow for Sensitive Detection. Micro Nano Lett. 12 (11), 901–906. doi:10.1049/mnl.2017.0424
Zhang, J., Oueslati, R., Cheng, C., Zhao, L., Chen, J., Almeida, R., et al. (2018). Rapid, Highly Sensitive Detection of Gram-Negative Bacteria with Lipopolysaccharide Based Disposable Aptasensor. Biosens. Bioelectron. 112, 48–53. doi:10.1016/j.bios.2018.04.034
Zhao, S., Ma, W., Xu, L., Wu, X., Kuang, H., Wang, L., et al. (2015). Ultrasensitive SERS Detection of VEGF Based on a Self-Assembled Ag Ornamented-AU Pyramid Superstructure. Biosens. Bioelectron. 68, 593–597. doi:10.1016/j.bios.2015.01.056
Keywords: aptamer, biomarker, biosensor, assembled G-quadruplex, thioflavin T
Citation: Zheng X, Gao S, Wu J and Hu X (2021) A Fluorescent Aptasensor Based on Assembled G-Quadruplex and Thioflavin T for the Detection of Biomarker VEGF165. Front. Bioeng. Biotechnol. 9:764123. doi: 10.3389/fbioe.2021.764123
Received: 25 August 2021; Accepted: 29 October 2021;
Published: 16 November 2021.
Edited by:
Han-Sheng Chuang, National Cheng Kung University, TaiwanReviewed by:
Bicheng Zhu, The University of Auckland, New ZealandXiaolin Huang, Nanchang University, China
Copyright © 2021 Zheng, Gao, Wu and Hu. This is an open-access article distributed under the terms of the Creative Commons Attribution License (CC BY). The use, distribution or reproduction in other forums is permitted, provided the original author(s) and the copyright owner(s) are credited and that the original publication in this journal is cited, in accordance with accepted academic practice. No use, distribution or reproduction is permitted which does not comply with these terms.
*Correspondence: Jihong Wu, jihongwu@fudan.edu.cn; Xiaobo Hu, huxiaobo@vip.sina.com
†These authors have contributed equally to this work