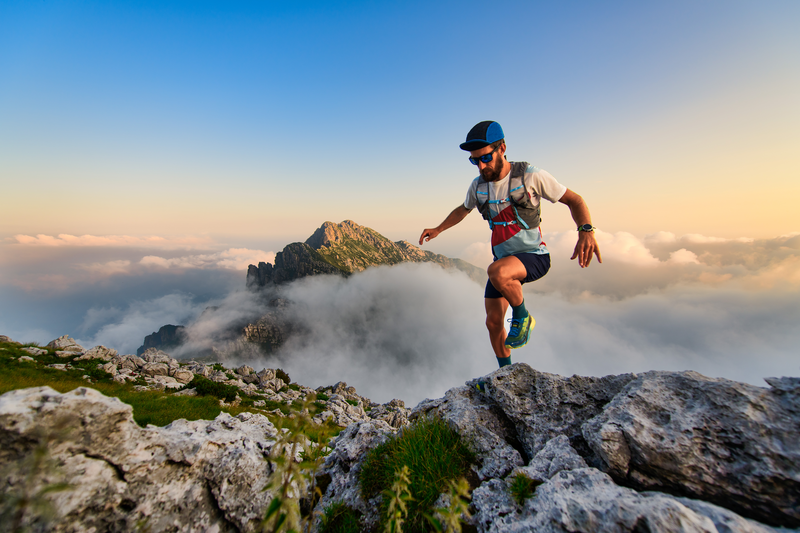
94% of researchers rate our articles as excellent or good
Learn more about the work of our research integrity team to safeguard the quality of each article we publish.
Find out more
ORIGINAL RESEARCH article
Front. Bioeng. Biotechnol. , 18 October 2021
Sec. Bioprocess Engineering
Volume 9 - 2021 | https://doi.org/10.3389/fbioe.2021.761510
This article is part of the Research Topic Biodegradation of Plastics View all 10 articles
Millions of tonnes of tyre waste are discarded annually and are considered one of the most difficult solid wastes to recycle. A sustainable alternative for the treatment of vulcanised rubber is the use of microorganisms that can biotransform polymers and aromatic compounds and then assimilate and mineralise some of the degradation products. However, vulcanised rubber materials present great resistance to biodegradation due to the presence of highly hydrophobic cross-linked structures that are provided by the additives they contain and the vulcanisation process itself. In this work, the biodegradation capabilities of 10 fungal strains cultivated in PDA and EM solid medium were studied over a period of 4 weeks. The growth of the strains, the mass loss of the vulcanised rubber particles and the surface structure were analysed after the incubation period. With the white rot fungi Trametes versicolor and Pleurotus ostreatus, biodegradation percentages of 7.5 and 6.1%, respectively, were achieved. The FTIR and SEM-EDS analyses confirmed a modification of the abundance of functional groups and elements arranged on the rubber surface, such as C, O, S, Si, and Zn, due to the biological treatment employed. The availability of genomic sequences of P. ostreatus and T. versicolor in public repositories allowed the analysis of the genetic content, genomic characteristics and specific components of both fungal species, determining some similarities between both species and their relationship with rubber biodegradation. Both fungi presented a higher number of sequences for laccases and manganese peroxidases, two extracellular enzymes responsible for many of the oxidative reactions reported in the literature. This was confirmed by measuring the laccase and peroxidase activity in cultures of T. versicolor and P. ostreatus with rubber particles, reaching between 2.8 and 3.3-times higher enzyme activity than in the absence of rubber. The integrative analysis of the results, supported by genetic and bioinformatics tools, allowed a deeper analysis of the biodegradation processes of vulcanised rubber. It is expected that this type of analysis can be used to find more efficient biotechnological solutions in the future.
The automotive industry has generated an enormous amount of tyre waste, where landfill is not a viable or sustainable alternative (Bowles et al., 2020). In nature, we can find biological degradation mechanisms carried out by microorganisms with the capacity to use a wide range of compounds such as natural polymers. However, tyre waste has a highly complex structure, preventing effective natural biodegradation processes. Since only 20–25% w/w of a tyre corresponds to natural rubber and the remaining percentage to a mixture of synthetic rubber, carbon black, antioxidants, accelerators, retardants, elemental sulphur, among other compounds (Stevenson et al., 2008), cell colonisation and, consequently, biodegradation is inhibited. In addition to the highly resistant chemicals as part of the tyre, the high degree of crosslinking and the low or no reactivity of the functional groups in the tyre structure make the mixing of this residue with other materials a major challenge (Andler, 2020; Simon-Stőger and Varga, 2021).
Several tyre-recycling processes have been described, and most of them are focused on the modification of the chemical structure and, thus, achieving devulcanisation. These include chemical processes using different peroxides (Rooj et al., 2011; Sabzekar et al., 2015; Hejna et al., 2019), physical processes such as grinding, ultrasound, microwaves or thermo-mechanical processes (Feng and Isayev, 2006; Wang et al., 2013; Formela and Cysewska, 2014; Garcia et al., 2015; Asaro et al., 2018; Formela et al., 2019; Li et al., 2020) and biological processes using microorganisms, mainly bacteria (Tatangelo et al., 2016; Ghavipanjeh et al., 2018; Aboelkheir et al., 2019; Kaewpetch et al., 2019).
The biodegradation of unvulcanised rubber, such as natural rubber, latex or poly (cis-1, 4-isoprene), has previously been studied in detail. Those studies pointed out that the main microorganisms responsible for performing polymer cleavage into oligomers are actinomycetes belonging to the genera Gordonia, Streptomyces, Nocardia, Actinoplanes, among others (Jendrossek et al., 1997). The metabolic pathways involved during the oxidation of oligo (cis-1,4-isoprene) molecules by β-oxidation (Hiessl et al., 2012) and cell cultures, using the polymer as sole carbon source, have also been described (Andler et al., 2018b). Furthermore, the enzymes responsible for the first oxidative attack, the so-called “rubber oxygenases,” have been studied biochemically (Jendrossek and Birke, 2019) and have been used for in vitro applications (Andler et al., 2018a, 2020). However, biodegradation studies using vulcanised rubber are scarce, and much remains to be understood.
Fungi are characterised by their biochemical and ecological capacity to degrade contaminants of different nature and varied chemical structures (Harms et al., 2011). They present a series of unique characteristics for the biodegradation of pollutants or toxic substances, including their ability to extend through substrates because of their filamentous structure, powerful enzyme production system and the capacity to produce natural surfactants that favour the degradative process (Sánchez, 2020). The biodegradation of synthetic polymers using fungi has been extensively studied using polyethylene terephthalate (PET), polyethylene (PE), polypropylene (PP), polyvinyl chloride (PVC) and polystyrene (PS) plastics. While commercial plastics also contain additives that impart the physicochemical properties of interest to the final product and prolong the life of plastic products (Hahladakis et al., 2018), the amount of these additives relative to the polymer is considerably lower when compared to the amount of additives present in tyres (Altenhoff et al., 2019).
Given the similarity between lignocellulosic biomass and the additives present in tyres, with many of them being aromatic compounds, white-rot fungi are excellent candidates for detoxifying tyre waste. In a previous study, the degradative capacity of 15 fungi was analysed using the additive Poly R-478 as a model aromatic compound. The authors found that the fungi Pleurotus sajor-caju, Trametes versicolor and Resinicium bicolor had a higher incidence of decolorising the additive and maintained their extracellular enzymatic activity after cultivation (Bredberg et al., 2002).
Despite the important degradative capacity of fungi, given their high metabolic rate and tremendous capacity for the degradation and mineralisation of various environmental pollutants, there is little information regarding their potential use as catalysts for rubber wastes. In this study, we analysed the effects generated on the surface of vulcanised rubber particles after incubation with different fungal species and their linkage with the possible enzymes responsible for these modifications, using molecular and bioinformatics tools.
Rubber particles (RP) were purchased from Trelleborg AB, Germany. For all experiments performed, a particle size between 1 and 2 mm was used. To avoid contamination, rubber particles were washed with 70% ethanol, dried at 80°C for 24 h and sterilised at 121°C for 20 min in separate aluminium envelopes.
Ten different fungal species, namely Coriolus multicolor, Gonnoderma aplanatum, Lentinula edodes, Lenzites trabea, Lenzites betulinus, Pleurotus ostreatus, Pleurotus eryngii, Postia placenta, Stereum hirsutum and Trametes versicolor, were studied. The strains were incubated at 28°C using plates with potato dextrose agar (PDA) and malt extract agar (MEA). After obtaining a fully grown plate, 0.5 g of rubber particles was added to each plate under sterile conditions and incubated for 4 weeks. Each experiment was performed in triplicate.
After the incubation period, the rubber particles were carefully collected from the agar plate under sterile conditions and washed with 70% ethanol and 10% sodium hypochlorite until total removal of the fungi. Subsequently, the solvent was removed, and the rubber particles were dried at 50°C for 72 h. Control samples were treated under the same conditions to prevent the potential oxidative effect of sodium hypochlorite from affecting subsequent analyses.
The mass of the rubber particles was analysed before and after the incubation period with the fungi. The loss of mass was calculated as follows:
where mi is the initial mass of the rubber particles and mf is the final mass of the rubber particles.
10 mg of dried rubber particles were analysed by attenuated total reflectance (ATR-FTIR). The infrared spectra were obtained using an FTIR spectrometer (Agilent, Cary 630) with the ATR technique. Absorbance was measured in the wavelength range of 400–40,00 cm−1, with a resolution of 4 cm−1. For each sample, 64 scans were performed, and the background was subtracted using the Agilent MicroLab PC software.
An electron microscope TESCAN VEGA 3 with probe EDS BRUKER QUANTAX was used. The method was based on the ASTM E1508 standard “Standard Guide for Quantitative Analysis by Energy-Dispersive Spectroscopy”. Samples were covered by a cathodic spray system with Palladium Gold in the Hummer 6.2 equipment.
Liquid cultures of T. versicolor and P. ostreatus were performed by cutting three 1 × 1 cm2 plugs from fully grown PDA plates and poured into 250-ml shake flasks with 50 ml of potato dextrose broth (PDB). Sterile rubber particles were incorporated to the flasks while the corresponding controls did not contain rubber particles. Cultivations were performed at 30 °C, 100 rpm for 5 days. Supernatants were obtained by centrifugation at 10,000 × g for 20 min at 4°C and used for enzyme activity measurements.
Laccase and peroxidase activities were quantified spectrophotometrically (Agilent, Cary 100 Bio) at 415 nm by detecting the oxidation of 2,20-azino-bis(3-ethylbenzothiazoline-6-sulfonate) (ABTS). For laccase activity, 100 μL of sample were mixed with a solution containing 900 μL of 10 mM ABTS and 0.2 M sodium acetate pH 5.0. For peroxidase activity, 100 μL of sample were mixed with a solution containing 800 μL of 10 mM ABTS and 100 M sodium acetate pH 5.0 and 100 μL of 20 mM H2O2.
Two Basidiomycetes species were selected in this study as sample sets of the biodegradation of vulcanised rubber. Publicly available genomic assemblies were downloaded from the Joint Genome Institute’s fungal genome portal MycoCosm (Grigoriev et al., 2014), JGI Genome Portal - unified access to all JGI genomic datasets (Mukherjee et al., 2021) and NCBI Genome Assembly (Schoch et al., 2020).
Two specific enzymes (laccase EC 1.10.3.2 and manganese peroxidase EC 1.11.1.13) were searched in sequences deposited in a public database for the 10 fungal species analysed in this study. The database used consisted of identical protein groups of the National Center for Biotechnology Information (www.ncbi.nlm.nih.gov).
For analysis of the similarity at the amino acid level among the sequences of laccases and manganese peroxidase, we used the MEGA-X (Kumar et al., 2018) roand T-COFFEE (https://coffee.crg.cat/apps/tcoffee/) (Notredame et al., 2000) software packages with the default parameters. To generate the protein alignment figures, the ESPript 3.0 software (https://espript.ibcp.fr/) (Robert and Gouet, 2014) was used, highlighting the regions with higher similarity among the sequences: black represents 100% similarity, grey represents 90 to 80% and white similarity below 70%. Each analysis used sequences of laccases and manganese peroxidase separately from Pleurotus ostreatus and Trametes versicolor.
To evaluate the presence of a conserved domain among the sequences of laccases and manganese peroxidase from P. ostreatus and T. versicolor, we used the Conserved Domain Database (CDD) from the NCBI (https://www.ncbi.nlm.nih.gov/Structure/cdd/cdd.shtml) and PFAM (http://pfam.xfam.org/) programmes (Lu et al., 2020).
The fungal strains were evaluated in terms of radial growth when incubated in PDA and EM media in Petri dishes, and no significant differences were found between the culture media used (data not shown). Figure 1A shows some of the fungi incubated with the vulcanised rubber particles already incorporated. There was a different fungus-rubber interaction depending on the fungal strain used. Three different behaviours stand out, namely total coating, partial coating and zero coating. Of the 10 strains analysed, only P. ostreatus and T. versicolor achieved a total coating of the vulcanised rubber particles, whereas the strains C. multicolor, L. edodes, L. betulinus and P. eryngii showed partial coating. The time required to reach a fully-grown plate was different for each fungus, which was qualitatively analysed by the relative growth rate (Figure 1B). Based on this, P. ostreatus obtained complete growth in the shortest time (between 24 and 30 h), followed by P. eryngii and T. versicolor (from 36 to 42 h). In general, the observations of the level of coating were related to the percentage mass loss of the vulcanised rubber particles at the end of the incubation periods, while the relative growth rate was not always a precise indicator. The highest mass loss was 7.5 ± 0.3%, obtained after cultivation with T. versicolor, followed by 6.1 ± 0.4% obtained with P. ostreatus. Based on these results, vulcanised rubber particles treated with T. versicolor and P. ostreatus strains were selected for surface analysis.
FIGURE 1. (A) Growth of fungal species in the presence of vulcanised rubber particles. (B) Relative growth rate and rubber mass reduction of the 10 fungal species after incubation.
Bredberg et al. (2002) studied the detoxification of vulcanised rubber by different wood-rotting fungi, using the aromatic polymeric dye polyvinylamine sulfonate anthrapyridone (Poly-R478) as a model compound. The authors showed that only three strains were able to biodegrade Poly-R478, namely Pleurotus sajor-caju, T. versicolor and Recinicium bicolor. Subsequently, they used the treated rubber in cultures with Acidithiobacillus ferrooxidans and reached higher growth rates compared to rubber without fungal treatment. It was assumed that the extracellular enzymes from the selected fungi degraded aromatic structures and that the rubber obtained had a reduced toxicity. As in our study, only the cultures with white rot fungi obtained positive results.
Changes in the rubber surface were analysed by FTIR (Figure 2). The functional groups observed may indicate the presence of compounds such as black carbon, aromatic oils, antioxidants, accelerators, among others (Altenhoff et al., 2019). Doublet was observed between 2,926 and 2,853 cm−1 (stretching of CH2), which was drastically reduced after the treatment, with the greatest reduction in T. versicolor with EM medium and P. ostreatus with EM medium (Gorassini et al., 2016). A loss of a signal was also observed at approximately 1,715 cm−1 in the treatment with T. versicolor on EM and PDA media and for P. ostreatus on EM and PDA media, corresponding to carbonyl groups (C=O), which are typical of the rubber chain (Gorassini et al., 2016). A band at approximately 1,550 cm−1, associated with carboxylate or conjugated ketone, was totally lost after cultivation of both fungal species (Stelescu et al., 2017). After incubation with T. versicolor, a small band close to 1,455 cm−1, assigned to C-H bending of CH2, was maintained in PDA, but it decreased using EM. However, for P. ostreatus, the band decreased for the assay with PDA and increased significantly in the treatment with EM. Most likely, the sporulation of the fungus was influenced when PDA medium was used, and therefore, the enzymatic metabolism involved in the decrease of the band was influenced by the sporulation process stimulated by PDA (Su et al., 2012). We detected a variation in the polymer structure after treatments corresponding to alkene groups (–C=CH) at a wavelength of 874 cm−1. This band was not present in the control, but it appeared after incubation with T. versicolor and P. ostreatus on both media (Colom et al., 2016). One reason for this may be the exposure of groups related to isoprene and butadiene present in rubber, which, before treatment, were hidden in more interior areas of the material.
FIGURE 2. FTIR spectrum of vulcanised rubber particles after incubation with Trametes versicolor and Pleurotus ostreatus using PDA and EM medium.
These results show that the treatment using T. versicolor with EM allowed a greater transformation of the surface of the rubber material, suggesting that the EM medium favours the degradation in a better way than PDA for T. versicolor. For P. ostreatus, the PDA medium allowed, in general, a greater decrease in bands versus the control. The EM medium could stimulate the generation of secondary metabolites, allowing a more efficient degradation of rubber compared to the type of metabolites stimulated by the PDA medium.
We used SEM analysis to demonstrate the changes in the rubber surface against mechanical, chemical or biological treatments (Li et al., 2012; Aboelkheir et al., 2019). In this study, SEM analysis revealed morphological changes of the analysed rubber surface after biological treatments (P. ostreatus and T. versicolor using PDA medium) in comparison with the control at 500x (Figure 3). In both treatments, particle size was approximately 500 to 1,200 µm and was therefore not influenced by the treatments, in contrast to a previous study (Li et al., 2012). The size reported in this work is compatible with treatments with lower-performance grinding. When comparing the control versus the biological treatments, an increase in the roughness of the material was observed, which was similar for T. versicolor and P. ostreatus. After the treatment, the surface showed cracks, facilitating the breaking of the rubber particles by subsequent treatments, such as mechanical ones, since they can spread rapidly, generating fractures (Kroon, 2011). This result is also related to the elemental analysis of C, O, S, Si, and Zn on the rubber surface (Table 2), where the carbon content decreased in the rubber particles subjected to the treatments. The opposite was observed for O and S; it should be noted that the PDA medium lacks sulphates, and therefore, the increase in S and O is not a result of the culture medium. We therefore recommend that the rubber particles were washed with ethanol and a solution of chlorine before superficial analysis (see Methodology), and therefore, the increases in Si and Zn after the biological treatment are not due to contamination, as both elements are commonly present in tyres (Adathodi et al., 2018; Altenhoff et al., 2019). Zinc is used in the non-accelerated vulcanisation of rubber, whereas silicium is used as a filler (Rattanasom et al., 2007). With P. ostreatus, a higher percentage of Si was detected compared to T. versicolor, whereas for Zn, the percentage was higher with the use of T. versicolor. The changes at the surface can be attributed to oxidation-reduction reactions by the enzymes secreted by the microorganisms, exposing a different proportion of the elements initially arranged on the surface. It is suggested that the observed changes were due to enzymatic processes related to the assimilation of microorganisms towards the components present on the surface of the treated material, which is corroborated by the loss of rubber mass after treatment.
Laccase and peroxidase activities were detected in all the conducted experiments (Figure 4). In cultures of T. versicolor, the presence of rubber particles revealed a 2.8-fold increase of laccase activity and 3.3-fold increase of peroxidase activity compared to the cultures without rubber particles. Likewise in cultures of P. ostreatus, laccase and peroxidase activities increased by double when rubber particles were in the cultivation medium. A maximum laccase activity of 0.128 U ml−1 was calculated for T. versicolor cultures, a 18% more than for P. ostreatus cultures. An enhanced laccase and peroxidase activity can be achieved by the addition of different mediators into the cultivation medium such as xylidine, ferulic acid, veratrylalcohol, pyrogallol and copper (Vranska et el. 2016), enzymes like feruloyl esterase, aryl-alcohol oxidase, quinone reductases, lipases, catechol 2, 3-dioxygenase (Kumar and Chandra 2020) or by culture media optimization (Wang et al., 2014; Hahn Schneider 2018). The increase of the laccase and peroxidase activity in the cultures of T. versicolor and P. ostreatus predicts the affinity of these ligninolytic enzymes for aromatic substrates present in the rubber composition.
FIGURE 4. Laccase and peroxidase activity in cultures of Trametes versicolor and Pleurotus ostreatus in the presence and the absence of vulcanized rubber particles. L, laccasse, P, peroxidase. Measurements were carried out in triplicates.
The presence of laccase and peroxidase enzymes has been described during the degradation process of natural rubber by the bacteria Bacillus subtilis (Nayanashree and Thippeswamy, 2015). Nevertheless, the enzymatic mechanism is still unknown and rubber oxygenases (Lcp, RoxA, RoxB) have been well characterized for the cleavage of natural rubber (Andler et al., 2018a; Jendrossek and Birke, 2020). The complexity of working with multi-components substrates as rubber is to reveal which of those parts are directly related to the activity of the extracellular enzymes. The current work has shown that the presence of rubber act as an inducer of laccase and peroxidase activities, and provides a more concrete result in the role of these groups of enzymes for vulcanized rubber biodegradation. However, it would be necessary to study the effect of laccases and peroxidases with each of the different components of vulcanized rubber to gain a deeper understanding of the reaction mechanisms.
Obtaining genome data is the initial step in understanding the biology of P. ostreatus and T. versicolor. Fungi present a wide variety of functional proteins that play different roles in the acquisition of nutrients and protection against nearby organisms or unfavourable environmental conditions (Kim et al., 2016).
The genomes of P. ostreatus and T. versicolor have been sequenced, and an analysis of the published fungal genomes, sourced from the public database (Grigoriev et al., 2014; Schoch et al., 2020; Mukherjee et al., 2021), revealed that the P. ostreatus haploid genome contains 11 chromosomes, with a length of 34.3 Mbp and a G + C content of ∼51%. For T. versicolor, there is no information in the public database about the number of chromosomes; however, the total genomic size is 44.8 Mb, with a G + C content of ∼58% (Table 1). Although there is a large variation in the genome size in fungi, the average genome size of fungal species taken during this study was 40.0 Mb. Fungal species harbour small genomes with highly specific genic regions and reduced non-coding regions (Galagan et al., 2003; Martinez et al., 2004). Fungi belonging to the phylum Basidiomycota have an average genome size of 46 Mb (Mohanta and Bae, 2015). In line with this, the sequenced genomes of Pleurotus taxa were assembled to less than 50 M bp but exhibited large numbers of annotated protein-coding genes and few constituent TEs (Table 1). However, as mentioned before, there were genome size variations among the two species, which could have arisen from unequal TE variations. The number of transposable elements (TEs), including retrotransposons and DNA transposons, accounted for approximately 253 of the P. ostreatus genome and 233 of the T. versicolor genome. For P. ostreatus, it was possible to determine notable genomic differences in the regions corresponding to TEs, where 80 identified TE families represented 2.5–6.2% of the genome sizes (Téllez-Téllez and Díaz-Godínez, 2019). Transposable elements are undoubtedly an important source of genetic variation in fungi, as previously found for other fungal species (Labbé et al., 2012).
Considering the coding gene sequences in fungi, on average, the Basidiomycota group encodes for 15,431.51 genes in their genomes. The average numbers of annotated genes are 12,330 for P. ostreatus per genome and 14,572 for the T. versicolor genome (Table 1). For the noncoding gene, we found scaffolds of the genome assembly, with 315 and 234 genes, respectively. There were three and two pseudogenes predicted, corresponding to 0.24% of the genome assembly of P. ostreatus and 0.014% of the genome assembly of T. versicolor.
Comparative analysis of fungal genomes showed that fungi are highly divergent. The P. ostreatus genome sequence assembly was distributed across of 12 and 572 scaffolds. Of these, 56 were smaller than 1 kb (Alfaro et al., 2016; Castanera et al., 2016). The genome of T. versicolor comprised only 283 scaffolds. The average protein coding genes in P. ostreatus were 12,330, with 14,302 for T. versicolor. These coding genes have homologues with known proteins deposited in the NCBI nr, Pfam, SwissProt and TrEMBL databases.
Both fungal species can partially degrade vulcanised rubber, and genome data revealed that both of fungi encode for a large set of enzymes involved in the degradation of rubber materials. The protein coding genes with enzymes for P. ostreatus included 1,677 genes (Table 1). For T. versicolor, such data were not available in the public repositories. However, based on a previous study, T. versicolor has an expansion of the AA2 gene family (26 genes), a feature that is also found in the central polyporoid clade (Miyauchi et al., 2018). The putative peroxidase genes (PoPOD) were obtained from the Joint Genome Institute (JGI) (Mukherjee et al., 2021). The gene density and the mean size of the protein-coding genes in both species of fungi used in this study secrete different enzymes, among which are laccases, manganese peroxidases, versatile peroxidases, glycosylhydrolases, peptidases and fungal esterases/lipases (Ruiz-Dueñas et al., 2011). These enzymes can degrade complex compounds such as lignin as well as certain industrial pollutants contaminating vulcanised rubber. Despite the advances in comparative genomics, the genera Pleurotus and Trametes are under-exploited, and a variety of potential biotechnological applications in different industries can be elucidated based on their genomes.
The genomic data of several species evaluated in this work revealed information concerning the presence of laccases and manganese peroxidase enzymes. For the species L. edodes, L. betulinus, P. eryngii, P. ostreatus, P. placenta, S. hirsutum and T. versicolor, database searches revealed the presence of several sequences of laccase already sequenced; the species L. edodes, P. ostreatus, T. versicolor and P. eryngii showed the highest number of laccase sequences (Table 2). In addition, the species G. applanatum, L. edodes, P. eryngii, P. ostreatus, S. hirsutum and T. versicolor also presented several sequences of manganese peroxidase already characterised. Species such as L. edodes, P. ostreatus and T. versicolor presented the highest number of characterised enzymes of manganese peroxidase in the evaluated database (Table 2). In our experiments, these species showed a high ability to degrade rubber. The high number of deposited sequences of laccase and manganese peroxidase for these species could reinforce their role as microorganisms that can degrade rubber and other polymers naturally, using laccase and manganese peroxidase enzymes (Nayanashree and Thippeswamy, 2015). However, the species C. multicolor and L. trabea presented no sequences of both mentioned enzymes. Also, G. applanatum had no deposited sequence of laccase; L. betulinus and P. placenta had no sequences of manganese peroxidase in the database used in this study.
Laccase presented three multicopper oxidase-conserved domains in its structure, namely Cu-oxidase, Cu-oxidase 2 and Cu-oxidase 3. For all sequences, these domains were located in the same region. For Cu-oxidase 3 domain, its location in the sequences started around the 31 amino acid position; it had a size of around 119 amino acids. The Cu-oxidase domain started from the 160 to 169 amino acid position and presented about 159 amino acids. The Cu-oxidase 2 domain started in the 366 to 388 amino acid position and generally had a size of 137 amino acids. These domains can use copper ions as cofactors to oxidise a broad range of substrates (Nayanashree and Thippeswamy, 2015; Arregui et al., 2019).
Manganese peroxidase has two conserved domains: a peroxidase domain with 229 amino acids and another domain, the so-called “peroxidase extension region,” with a size of 79 amino acids. The peroxidase domain in the sequences starts from 49 to 53 amino acid position and the peroxidase extension region from 279 to 286 amino acid position. These enzymatic domains are involved in oxidative reactions that use hydrogen peroxide as the electron acceptor (Nayanashree and Thippeswamy, 2015; Wang et al., 2016; Arregui et al., 2019).
For alignment at the amino acid level of laccase sequences from P. ostreatus, 18 sequences were used, and from T. versicolor, 23 sequences were used. All partial sequences were removed from the alignment analysis. Laccases from P. ostreatus showed a size of around 530 amino acids and a different pattern of the amino acid content; they were divided into four group of laccases. For T. versicolor, the laccases showed an average size of 520 amino acids and showed the same behaviour as those from P. ostreatus regarding the amino acid content; they were divided into three groups. In the evaluated database, the information about the specific name of each laccase in both species was inconsistent, making it difficult to predict the correct name per group. However, based on the amino acid alignment and the similarity, the laccase groups were determined.
In P. ostreatus, the groups of alignment represented for A and B presented high similarity among their amino acid contents of the studied sequences; however, for the groups C and D, the sequences were almost identical (see Supplementary Figure S1 online). In T. versicolor, group A also presented a high similarity among the amino acid contents of the sequences, and groups B and C were almost identical (see Supplementary Figure S2 online).
Regarding manganese peroxidase, we also evaluated its amino acid conservation among all sequences deposited in the NCBI database. Both species presented manganese peroxidase with a size of around 360 amino acids. For P. ostreatus, we evaluated 11 sequences and for T. versicolor 10 sequences. Similar to the laccase analysis, all partial sequences were removed from the alignment analysis. The species P. ostreatus presented four groups based on the amino acid contents of the sequences (see Supplementary Figure S3 online). The groups A, B and C were almost identical, and group D of manganese peroxidase presented high similarity regarding the amino acid content. The species T. versicolor had two groups of manganese peroxidases enzymes (see Supplementary Figure S4 online), presenting high similarity among the sequences for the group representing A, while for the group B, the sequences are almost identical. When generating an alignment using all sequences together, one analysis for laccase and another analysis for manganese peroxidase, the level of amino acid similarity decreased (data not shown). Only separated regions of conserved domains were maintained. These results indicate the presence of different isoforms of laccases and manganese peroxidase in the genomes of these two studied fungi or the lack of genomic information in the accessed database (Yuan et al., 2016).
Sustainable solutions for the management of toxic solid wastes, such as vulcanised rubber, are of great importance, and the use of microorganisms has great potential. However, the hydrophobic nature and the large amounts of additives present in the material prevent high biodegradation rates. In this study, we analysed the degradation potential of different fungal strains, finding two white rot fungi that stood out, namely T. versicolor and P. ostreatus. The effects of biological treatments in terms of surface modifications and the presence of laccase and peroxidase activity were linked to genomic and bioinformatic analyses. We found a strong relationship between the percentage of biodegradation of rubber particles and the availability of laccase and manganese peroxidase enzymes in these species, two of the main enzymes used in the bioremediation of xenobiotics. The results obtained are promising, highlighting white rot fungi as potent biodegrading agents. Among the most interesting considerations is the non-specificity of the enzymatic attack provided by these fungi, which is necessary in highly recalcitrant materials such as tyre waste. Given the complexity of the biodegradation of vulcanised rubber, integrated and comprehensive studies are required to achieve a deeper understanding and to postulate new biotechnological processes for more effective biodegradation or biotransformation.
The original contributions presented in the study are included in the article/Supplementary Material, further inquiries can be directed to the corresponding author.
RA conceptualised, analyzed the data and edited the manuscript, VD conducted the experiments, analyzed the data and drafted the manuscript, JP conducted the experiments, helped in providing information and preparation of tables and figures, CV analyzed the data and drafted the manuscript, MS analyzed the data and drafted the manuscript. All authors read and approved the final manuscript.
This research was financially supported by FONDECYT Grant 11190220 from ANID (Chile).
The authors declare that the research was conducted in the absence of any commercial or financial relationships that could be construed as a potential conflict of interest.
All claims expressed in this article are solely those of the authors and do not necessarily represent those of their affiliated organizations, or those of the publisher, the editors and the reviewers. Any product that may be evaluated in this article, or claim that may be made by its manufacturer, is not guaranteed or endorsed by the publisher.
The authors acknowledge the receipt of the Fondecyt Grant 11190220 from ANID (Chile).
The Supplementary Material for this article can be found online at: https://www.frontiersin.org/articles/10.3389/fbioe.2021.761510/full#supplementary-material
Aboelkheir, M. G., Bedor, P. B., Leite, S. G., Pal, K., Toledo Filho, R. D., and Gomes de Souza, F. (2019). Biodegradation of Vulcanized SBR: A Comparison between Bacillus Subtilis, Pseudomonas aeruginosa and Streptomyces Sp. Sci. Rep. 9. doi:10.1038/s41598-019-55530-y
Alfaro, M., Castanera, R., Lavín, J. L., Grigoriev, I. V., Oguiza, J. A., Ramírez, L., et al. (2016). Comparative and Transcriptional Analysis of the Predicted Secretome in the Lignocellulose-Degrading Basidiomycete fungusPleurotus Ostreatus. Environ. Microbiol. 18, 4710–4726. doi:10.1111/1462-2920.13360
Altenhoff, A.-L., de Witt, J., Andler, R., and Steinbüchel, A. (2019). Impact of Additives of Commercial Rubber Compounds on the Microbial and Enzymatic Degradation of Poly(cis-1,4-Isoprene). Biodegradation 30, 13–26. doi:10.1007/s10532-018-9858-5
Andler, R., Altenhoff, A.-L., Mäsing, F., and Steinbüchel, A. (2018a). In Vitro studies on the Degradation of Poly(cis -1,4-isoprene). Biotechnol. Prog. 34, 890–899. doi:10.1002/btpr.2631
Andler, R. (2020). Bacterial and Enzymatic Degradation of Poly(cis-1,4-Isoprene) Rubber: Novel Biotechnological Applications. Biotechnol. Adv. 44. doi:107606 doi:10.1016/j.biotechadv.2020.107606
Andler, R., Hiessl, S., Yücel, O., Tesch, M., and Steinbüchel, A. (2018b). Cleavage of Poly(cis-1,4-Isoprene) Rubber as Solid Substrate by Cultures of Gordonia Polyisoprenivorans. New Biotechnol. 44, 6–12. doi:10.1016/j.nbt.2018.03.002
Andler, R., Valdés, C., Díaz-Barrera, A., and Steinbüchel, A. (2020). Biotransformation of Poly(cis-1,4-Isoprene) in a Multiphase Enzymatic Reactor for Continuous Extraction of Oligo-Isoprenoid Molecules. New Biotechnol. 58, 10–16. doi:10.1016/j.nbt.2020.05.001
Arregui, L., Ayala, M., Gómez-Gil, X., Gutiérrez-Soto, G., Hernández-Luna, C. E., Herrera De Los Santos, M., et al. (2019). Laccases: Structure, Function, and Potential Application in Water Bioremediation. Microb. Cel Fact 18. doi:10.1186/s12934-019-1248-0
Asaro, L., Gratton, M., Seghar, S., and Aït Hocine, N. (2018). Recycling of Rubber Wastes by Devulcanization. Resour. Conservation Recycling 133, 250–262. doi:10.1016/j.resconrec.2018.02.016
Bowles, A. J., Fowler, G. D., O'Sullivan, C., and Parker, K. (2020). Sustainable Rubber Recycling from Waste Tyres by Waterjet: A Novel Mechanistic and Practical Analysis. Sustain. Mater. Tech. 25. doi:e00173 doi:10.1016/j.susmat.2020.e00173
Bredberg, K., Erik Andersson, B., Landfors, E., and Holst, O. (2002). Microbial Detoxification of Waste Rubber Material by wood-rotting Fungi. Bioresour. Technol. 83, 221–224. doi:10.1016/S0960-8524(01)00218-8
Castanera, R., López-Varas, L., Borgognone, A., LaButti, K., Lapidus, A., Schmutz, J., et al. (2016). Transposable Elements versus the Fungal Genome: Impact on Whole-Genome Architecture and Transcriptional Profiles. Plos Genet. 12. doi:e1006108 doi:10.1371/journal.pgen.1006108
Colom, X., Faliq, A., Formela, K., and Cañavate, J. (2016). FTIR Spectroscopic and Thermogravimetric Characterization of Ground Tyre Rubber Devulcanized by Microwave Treatment. Polym. Test. 52, 200–208. doi:10.1016/j.polymertesting.2016.04.020
Feng, W., and Isayev, A. I. (2006). Recycling of Tire-Curing Bladder by Ultrasonic Devulcanization. Polym. Eng. Sci. 46, 8–18. doi:10.1002/pen.20449
Formela, K., and Cysewska, M. (2014). Efficiency of Thermomechanical Reclaiming of Ground Tire Rubber Conducted in Counter-rotating and Co-rotating Twin Screw Extruder. Polimery 59, 231–238. doi:10.14314/polimery.2014.231
Formela, K., Hejna, A., Zedler, L., and Colom, J. (2019). Microwave Treatment in Waste Rubber Recycling - Recent Advances and Limitations. Express Polym. Lett. 13, 565–588. doi:10.3144/expresspolymlett.2019.48
Galagan, J. E., Calvo, S. E., Borkovich, K. A., Selker, E. U., Read, N. O., Jaffe, D., et al. (2003). The Genome Sequence of the Filamentous Fungus Neurospora Crassa. Nature 422. doi:10.1038/nature01554
Garcia, P. S., de Sousa, F. D. B., de Lima, J. A., Cruz, S. A., and Scuracchio, C. H. (2015). Devulcanization of Ground Tire Rubber: Physical and Chemical Changes after Different Microwave Exposure Times. Express Polym. Lett. 9, 1015–1026. doi:10.3144/expresspolymlett.2015.91
Ghavipanjeh, F., Ziaei Rad, Z., and Pazouki, M. (2018). Devulcanization of Ground Tires by Different Strains of Bacteria: Optimization of Culture Condition by Taguchi Method. J. Polym. Environ. 26, 3168–3175. doi:10.1007/s10924-017-1169-0
Gorassini, A., Adami, G., Calvini, P., and Giacomello, A. (2016). ATR-FTIR Characterization of Old Pressure Sensitive Adhesive tapes in Historic Papers. J. Cult. Heritage 21, 775–785. doi:10.1016/j.culher.2016.03.005
Grigoriev, I. V., Nikitin, R., Haridas, S., Kuo, A., Ohm, R., Otillar, R., et al. (2014). MycoCosm portal: Gearing up for 1000 Fungal Genomes. Nucl. Acids Res. 42, D699–D704. doi:10.1093/nar/gkt1183
Hahladakis, J. N., Velis, C. A., Weber, R., Iacovidou, E., and Purnell, P. (2018). An Overview of Chemical Additives Present in Plastics: Migration, Release, Fate and Environmental Impact during Their Use, Disposal and Recycling. J. Hazard. Mater. 344, 179–199. doi:10.1016/j.jhazmat.2017.10.014
Harms, H., Schlosser, D., and Wick, L. Y. (2011). Untapped Potential: Exploiting Fungi in Bioremediation of Hazardous Chemicals. Nat. Rev. Microbiol. 9, 177–192. doi:10.1038/nrmicro2519
Hejna, A., Klein, M., Saeb, M. R., and Formela, K. (2019). Towards Understanding the Role of Peroxide Initiators on Compatibilization Efficiency of Thermoplastic Elastomers Highly Filled with Reclaimed GTR. Polym. Test. 73, 143–151. doi:10.1016/j.polymertesting.2018.11.005
Hiessl, S., Schuldes, J., Thürmer, A., Halbsguth, T., Bröker, D., Angelov, A., et al. (2012). Involvement of Two Latex-Clearing Proteins during Rubber Degradation and Insights into the Subsequent Degradation Pathway Revealed by the Genome Sequence of Gordonia Polyisoprenivorans Strain VH2. Appl. Environ. Microbiol. 78, 2874–2887. doi:10.1128/AEM.07969-11
Jendrossek, D., and Birke, J. (2019). Rubber Oxygenases. Appl. Microbiol. Biotechnol. 103, 125–142. doi:10.1007/s00253-018-9453-z
Jendrossek, D., Tomasi, G., and Kroppenstedt, R. (1997). Bacterial Degradation of Natural Rubber: A Privilege of Actinomycetes. FEMS Microbiol. Lett. 150, 179–188. doi:10.1016/S0378-1097(97)00072-4
Kaewpetch, B., Prasongsuk, S., and Poompradub, S. (2019). Devulcanization of Natural Rubber Vulcanizates by Bacillus Cereus TISTR 2651. Express Polym. Lett. 13, 877–888. doi:10.3144/expresspolymlett.2019.76
Kim, K.-T., Jeon, J., Choi, J., Cheong, K., Song, H., Choi, G., et al. (2016). Kingdom-wide Analysis of Fungal Small Secreted Proteins (SSPs) Reveals Their Potential Role in Host Association. Front. Plant Sci. 7. doi:10.3389/fpls.2016.00186
Kroon, M. (2011). Steady-state Crack Growth in Rubber-like Solids. Int. J. Fract. 169, 49–60. doi:10.1007/s10704-010-9583-5
Kumar, A., and Chandra, R. (2020). Ligninolytic Enzymes and its Mechanisms for Degradation of Lignocellulosic Waste in Environment. Heliyon 6. doi:e03170 doi:10.1016/j.heliyon.2020.e03170
Kumar, S., Stecher, G., Li, M., Knyaz, C., and Tamura, K. (2018). MEGA X: Molecular Evolutionary Genetics Analysis across Computing Platforms. Mol. Biol. Evol. 35, 1547–1549. doi:10.1093/molbev/msy096
Labbé, J., Murat, C., Morin, E., Tuskan, G. A., Le Tacon, F., and Martin, F. (2012). Characterization of Transposable Elements in the Ectomycorrhizal Fungus Laccaria Bicolor. PLoS One 7. doi:e40197 doi:10.1371/journal.pone.0040197
Lakshmi Adathodi et al., L., Raja Murugadoss, L. A. e. a., and Gaddam, K. (2018). A Comparative Study on Vehicular Tyre Rubber and Aircraft Tyre Rubber, A Review Based on SEM, EDS and XRD Analysis. Ijmperd 8, 1227–1234. doi:10.24247/ijmperdapr2018141
Li, X., Xu, X., and Liu, Z. (2020). Cryogenic Grinding Performance of Scrap Tire Rubber by Devulcanization Treatment with ScCO2. Powder Tech. 374, 609–617. doi:10.1016/j.powtec.2020.07.026
Li, Y., Zhao, S., and Wang, Y. (2012). Microbial Desulfurization of Ground Tire Rubber by Sphingomonas sp.: A Novel Technology for Crumb Rubber Composites. J. Polym. Environ. 20, 372–380. doi:10.1007/s10924-011-0386-1
Lu, S., Wang, J., Chitsaz, F., Derbyshire, M. K., Geer, R. C., Gonzales, N. R., et al. (2020). CDD/SPARCLE: The Conserved Domain Database in 2020. Nucleic Acids Res. 48, D265–D268. doi:10.1093/nar/gkz991
Martinez, D., Larrondo, L. F., Putnam, N., Gelpke, M. D. S., Huang, K., Chapman, J., et al. (2004). Genome Sequence of the Lignocellulose Degrading Fungus Phanerochaete Chrysosporium Strain RP78. Nat. Biotechnol. 22, 695–700. doi:10.1038/nbt967
Miyauchi, S., Rancon, A., Drula, E., Hage, H., Chaduli, D., Favel, A., et al. (2018). Integrative Visual Omics of the white-rot Fungus Polyporus Brumalis Exposes the Biotechnological Potential of its Oxidative Enzymes for Delignifying Raw Plant Biomass. Biotechnol. Biofuels. 11. doi:10.1186/s13068-018-1198-5
Mohanta, T. K., and Bae, H. (2015). The Diversity of Fungal Genome. Biol. Proced. Online 17. doi:10.1186/s12575-015-0020-z
Mukherjee, S., Stamatis, D., Bertsch, J., Ovchinnikova, G., Sundaramurthi, J. C., Lee, J., et al. (2021). Genomes OnLine Database (GOLD) v.8: Overview and Updates. Nucleic Acids Res. 49, D723–D733. doi:10.1093/nar/gkaa983
Nayanashree, G., and Thippeswamy, B. (2015). Biodegradation of Natural Rubber by Laccase and Manganese Peroxidase Enzyme of Bacillus Subtilis. Environ. Process. 2, 761–772. doi:10.1007/s40710-015-0118-y
Notredame, C., Higgins, D. G., and Heringa, J. (2000). T-coffee: a Novel Method for Fast and Accurate Multiple Sequence Alignment 1 1Edited by J. Thornton. J. Mol. Biol. 302, 205–217. doi:10.1006/jmbi.2000.4042
Rattanasom, N., Saowapark, T., and Deeprasertkul, C. (2007). Reinforcement of Natural Rubber with Silica/carbon Black Hybrid Filler. Polym. Test. 26, 369–377. doi:10.1016/j.polymertesting.2006.12.003
Robert, X., and Gouet, P. (2014). Deciphering Key Features in Protein Structures with the New ENDscript Server. Nucleic Acids Res. 42, W320–W324. doi:10.1093/nar/gku316
Rooj, S., Basak, G. C., Maji, P. K., and Bhowmick, A. K. (2011). New Route for Devulcanization of Natural Rubber and the Properties of Devulcanized Rubber. J. Polym. Environ. 19, 382–390. doi:10.1007/s10924-011-0293-5
Ruiz-Dueñas, F. J., Fernández, E., Martínez, M. J., and Martínez, A. T. (2011). Pleurotus Ostreatus Heme Peroxidases: An In Silico Analysis from the Genome Sequence to the Enzyme Molecular Structure. Comptes Rendus Biologies 334, 795–805. doi:10.1016/j.crvi.2011.06.004
Sabzekar, M., Chenar, M. P., Mortazavi, S. M., Kariminejad, M., Asadi, S., and Zohuri, G. (2015). Influence of Process Variables on Chemical Devulcanization of Sulfur-Cured Natural Rubber. Polym. Degrad. Stab. 118, 88–95. doi:10.1016/j.polymdegradstab.2015.04.013
Sánchez, C. (2020). Fungal Potential for the Degradation of Petroleum-Based Polymers: An Overview of Macro- and Microplastics Biodegradation. Biotechnol. Adv. 40. doi:107501 doi:10.1016/j.biotechadv.2019.107501
Schneider, W. D. H., Fontana, R. C., Mendonça, S., de Siqueira, F. G., Dillon, A. J. P., and Camassola, M. (2018). High Level Production of Laccases and Peroxidases from the Newly Isolated white-rot Basidiomycete Marasmiellus Palmivorus VE111 in a Stirred-Tank Bioreactor in Response to Different Carbon and Nitrogen Sources. Process Biochem. 69, 1–11. doi:10.1016/j.procbio.2018.03.005
Schoch, C. L., Ciufo, S., Domrachev, M., Hotton, C. L., Kannan, S., Khovanskaya, R., et al. (2020). NCBI Taxonomy: A Comprehensive Update on Curation, Resources and Tools. Database 2020. doi:10.1093/database/baaa062
Simon-Stőger, L., and Varga, C. (2021). PE-contaminated Industrial Waste Ground Tire Rubber: How to Transform a Handicapped Resource to a Valuable One. Waste Manag. 119, 111–121. doi:10.1016/j.wasman.2020.09.037
Stelescu, M.-D., Manaila, E., Craciun, G., and Chirila, C. (2017, Development and Characterization of Polymer Eco-Composites Based on Natural Rubber Reinforced with Natural Fibers). Development and characterization of polymer eco-composites based on natural rubber reinforced with natural fibers. Materials, 10, 787. doi:10.3390/ma10070787
Stevenson, K., Stallwood, B., and Hart, A. G. (2008). Tire Rubber Recycling and Bioremediation: A Review. Bioremediation J. 12, 1–11. doi:10.1080/10889860701866263
Su, Y. Y., Qi, Y. L., and Cai, L. (2012). Induction of Sporulation in Plant Pathogenic Fungi. Mycology 3. doi:10.1080/21501203.2012.719042
Tatangelo, V., Mangili, I., Caracino, P., Anzano, M., Najmi, Z., Bestetti, G., et al. (2016). Biological Devulcanization of Ground Natural Rubber by Gordonia Desulfuricans DSM 44462T Strain. Appl. Microbiol. Biotechnol. 100, 8931–8942. doi:10.1007/s00253-016-7691-5
Téllez-Téllez, M., and Díaz-Godínez, G. (2019). Omic Tools to Study Enzyme Production from Fungi in the Pleurotus Genus. BioRes 14, 2420–2457. doi:10.15376/biores.14.1.2420-2457
Vrsanska, M., Voberkova, S., Langer, V., Palovcikova, D., Moulick, A., Adam, V., et al. (2016). Induction of Laccase, Lignin Peroxidase and Manganese Peroxidase Activities in White-Rot Fungi Using Copper Complexes. Molecules 21, 1553. doi:10.3390/molecules21111553
Wang, F., Hu, J.-H., Guo, C., and Liu, C.-Z. (2014). Enhanced Laccase Production by Trametes versicolor Using Corn Steep Liquor as Both Nitrogen Source and Inducer. Bioresour. Tech. 166, 602–605. doi:10.1016.j.biortech.2014.05.06810.1016/j.biortech.2014.05.068
Wang, N., Ren, K., Jia, R., Chen, W., and Sun, R. (2016). Expression of a Fungal Manganese Peroxidase in Escherichia coli: A Comparison between the Soluble and Refolded Enzymes. BMC Biotechnol. 16. doi:10.1186/s12896-016-0317-2
Wang, X., Shi, C., Zhang, L., and Zhang, Y. (2013). Effects of Shear Stress and Subcritical Water on Devulcanization of Styrene-Butadiene Rubber Based Ground Tire Rubber in a Twin-Screw Extruder. J. Appl. Polym. Sci. 130, 1845–1854. doi:10.1002/app.39253
Keywords: laccase, peroxidase, Pleurotus ostreatus, rubber biodegradation, rubber recycling, Trametes versicolor, vulcanized rubber
Citation: Andler R, D’Afonseca V, Pino J, Valdés C and Salazar-Viedma M (2021) Assessing the Biodegradation of Vulcanised Rubber Particles by Fungi Using Genetic, Molecular and Surface Analysis. Front. Bioeng. Biotechnol. 9:761510. doi: 10.3389/fbioe.2021.761510
Received: 19 August 2021; Accepted: 06 October 2021;
Published: 18 October 2021.
Edited by:
Aamer Ali Shah, Quaid-i-Azam University, PakistanReviewed by:
Ziaullah Shah, CECOS University, PakistanCopyright © 2021 Andler, D’Afonseca, Pino, Valdés and Salazar-Viedma. This is an open-access article distributed under the terms of the Creative Commons Attribution License (CC BY). The use, distribution or reproduction in other forums is permitted, provided the original author(s) and the copyright owner(s) are credited and that the original publication in this journal is cited, in accordance with accepted academic practice. No use, distribution or reproduction is permitted which does not comply with these terms.
*Correspondence: R. Andler, cmFuZGxlckB1Y20uY2w=
Disclaimer: All claims expressed in this article are solely those of the authors and do not necessarily represent those of their affiliated organizations, or those of the publisher, the editors and the reviewers. Any product that may be evaluated in this article or claim that may be made by its manufacturer is not guaranteed or endorsed by the publisher.
Research integrity at Frontiers
Learn more about the work of our research integrity team to safeguard the quality of each article we publish.