- 1Co-Innovation Center for Efficient Processing and Utilization of Forest Products, College of Chemical Engineering, Nanjing Forestry University, Nanjing, China
- 2Nexus of Rare Neurodegenerative Diseases, School of Life Sciences, Faculty of Science, The Chinese University of Hong Kong, Hong Kong, SAR China
Biomass polyphenols are bio-active macromolecules with distinct chemical structures in a variety of biomass. In recent years, the study of biomass polyphenols and their application in food and medicine fields has become a research hotspot, which predominantly focuses on the preparation, purification, structural identifications, and measurements of biological activities. Many studies describe methodologies for extraction and application of polyphenols, but comprehensive work to review its physiological activities like drugs and health products are lacking. This paper comprehensively unlocks the bioactivities of antioxidant, antibacterial, antitumor, anticancer, neuroprotection, control of blood sugar, regulation of blood fat, and promotion of gastrointestinal health functions of polyphenols from different biomass sources. This review will serve as an illuminating resource for the global scientific community, especially for those who are actively working to promote the advances of the polyphenols research field.
Introduction
The growth and development of biomass are sustained by the regulation of metabolism and resistance against different biological stresses. The endogenous metabolites of biomass are composed of primary and secondary metabolites. In the different biomass, the major constituents are cellulose, hemicellulose, lignin, which can be applied to prepare various value-added products and bio-materials (Sun et al., 2019; Zhou et al., 2019; Chen et al., 2020; Geng et al., 2020; Chen et al., 2021; Liu et al., 2021; Luo et al., 2021). Apart from these constituents, there is existed various minor constituents, such as flavonoids and polyphenols (Si et al., 2009; Gironi and Piemonte, 2011; Quideau et al., 2011; Goodman, 2020). Biomass polyphenols are one type of secondary metabolites synthesized primarily through the shikimic acid and phenylpropane pathways (Hidalgo-Liberona et al., 2020; Wang et al., 2020). Biomass polyphenols are widely found in plant skins, roots, leaves, and fruits, with an abundance of as much as 20% by weight (Quideau et al., 2011).
There has been a long history of utilization of biomass polyphenols, which have been used in tanning and as medicines starting from ancient times (Quideau et al., 2011). The natural feelings and intrinsic properties of biomass polyphenols make them remarkable amongst plant-derived products. In recent years, biomass polyphenols have attracted much more attention in green/sustainable scientific fields due to their broad distribution, natural abundance, diverse chemical structures, and biological functions. A range of studies has demonstrated that biomass polyphenols comprise multiple phenolic hydroxyl groups, which have been reported to elicit prominent physiological functions such as free radical scavenging and radical sequestration activities (Gironi and Piemonte, 2011; Dong et al., 2020; Pei et al., 2020). These functionalities thus highlight biomass polyphenols as effective antioxidants. In addition to executing antioxidant activities, biomass polyphenols dramatically inhibit the growth of different strains of bacteria, fungi, and viruses while not affecting the growth and development of beneficial microorganisms under weak acidic and neutral environments. This indicates the potential applications of biomass polyphenols as bacteriostatic and anti-tumor agents. Moreover, biomass polyphenols also effectively protect against cardiovascular diseases via lowering the levels of several key pathogenic factors in the blood, including blood lipid, oxidation of low-density lipoprotein, and blood pressure (Gironi and Piemonte, 2011; Quideau et al., 2011; Camargo et al., 2019; Delgado et al., 2019; Michaličková et al., 2019).
Biomass Polyphenols
Biomass polyphenols are a class of natural compounds widely distributed in biomass with an abundance second to lignin, cellulose, and hemicellulose. Polyphenols are predominantly accumulated in the leaves, vascular tissues, bark, immature fruits, seed coat, and disinfected tissues of biomass. China is rich in biomass polyphenol resources varieties (Figure 1), including Larch (100–150 mg/g, Yashunsky et al., 2014), Black wax (20–50 mg/g, Xu et al., 2016), Waxberry (20–50 mg/g, Chen et al., 2002), Yu Gan (200–300 mg/g, Yang and Liu, 2019), Houpixia (400–1,500 mg/g, Dai et al., 2006), Mangrove (500–600 mg/g, Dahibhate et al., 2020), Gallnut (300–500 mg/g, Ge et al., 2015).
Structure of Polyphenols
Polyphenols share common structural features, their basic framework includes the polyhydroxy substitution of a benzene ring, as well as the absence of any nitrogen functional groups. Biomass polyphenols can be divided into the classes of 1) hydrolyzed tannins (gallate polyphenols) and 2) condensed tannins (polyflavanol polyphenols or proanthocyanidins) (Gironi and Piemonte, 2011). Hydrolyzed tannins are products of tannin hydrolysis, revolving around cleavage ester linkages. Condensed tannins are mainly composed of polyflavanol polyphenols or proanthocyanidins, which contain hydroxyl flavanol monomers connected by C-C bonds (Porter, 1992). Since hydrolyzed tannins and condensed tannins are completely distinct in the aspect of the unit skeleton, there are significant differences in their functional properties and applications (Figure 2). For example, hydrolyzed tannins are unstable and prone to be hydrolyzed under various conditions (acid, alkali, and under the presence of certain enzymes). Condensed tannins are not readily hydrolyzed, but can be further condensed into insoluble upon contact with a strong acid (Gessner and Steiner, 2005). When polyphenols interact with proteins, alkaloids, or polysaccharides, the polyphenol molecules initially approach the surface of protein molecules through hydrophobic bonds. The entry of polyphenols to the hydrophobic bag enables the following formation of multi-point hydrogen bonds. Due to a large number of coordination groups, most metal ions also tend to form precipitates if allowed to complex with polyphenols. Under alkaline conditions, polyphenols and metal ions readily form polycomplexes. In addition, the phenolic hydroxyl in the phenolic structure of biomass polyphenols (catechol or catechol) is easily oxidized to the quinone structure via consuming oxygen in the environment (Zhang et al., 2005).
Classification of Polyphenols
More than 8,000 different kinds of polyphenols and their derivatives have been identified in the biomass kingdom (Boadas-Vaello et al., 2017). The name of polyphenols is assigned due to the presence of multiple phenolic groups in their chemical structures. In terms of structural differences, polyphenols can be further divided into four categories: phenolic acids, astragalus, lignans, and flavonoids (Table 1). A couple of studies have demonstrated the dominant biomass polyphenols that are found in common foods, including gallic catechins in green tea, resveratrol in grapes, capsaicin in chilis and peppers, curcumin in turmeric, genistein in soybean, and gingerol in ginger (Bhuyan, 2018). Investigation of the biological outcomes of these particular edible goods allows for a better understanding of the structural details related to the functionalities of polyphenols.
Bioactivity of Polyphenols
Polyphenols are usually ingested as mixtures of different compounds that are immersed in a complex food substrate. The material then undergoes digestion, which exerts changes in structure and activity, before the mixture eventually reaches and acts upon target organs. After ingestion, absorption from the digestive tract usually requires intestinal enzymes, such as lactase rhizopericoside hydrolase and cytosolute β-glucosidase, to hydrolyze glycoside binders and produce the corresponding aglycones (Day et al., 2000; Gee et al., 2000). These aglycones can be further metabolized by second-stage enzymes to produce methylated, sulfurated, and gluconaldized compounds (Manach et al., 2004). Meanwhile, polyphenols that are not absorbed in the small intestine reach the colon, where they are converted into simpler metabolites by colonic microbiota and consequently being absorbed and get involved in further metabolic reactions (Liu et al., 2018).
Due to the diversity of biomass polyphenols, a variety of biological activities has been reported, including antioxidant (Hu et al., 2020; Ji et al., 2020), anti-inflammatory (Myint et al., 2021), bacteriostatic (Martin and Bolling, 2015; Gullon et al., 2016; Liu et al., 2019), anti-tumor (Sharma et al., 2017; Sajadimajd et al., 2020), regulation of intestinal flora (Cardona et al., 2013; Suzuki, 2013) and prevention of cardiovascular diseases (Kang, 2013; Tangney and Rasmussen, 2013; Kitai and Tang, 2017; Orr et al., 2020). Biomass polyphenols have also been widely used in the fields of the development of drugs and health products.
Antioxidant Activity
Redox is an essential class of metabolic reaction that occurs in living organisms. However, when the electron flow becomes decoupled, the generation of harmful free radicals results in detrimental outcomes (Fiedor and Burda, 2014; Zhao et al., 2021). Free radicals are atoms, molecules, or ions with unpaired electrons. They are highly unstable, will rapidly attack molecules in adjacent cells, and are prone to chemically react with other molecules (Yu et al., 2020). These reactions in turn contribute to various forms of impairments to cells. Most of the impairments can be repaired, but the entire reaction can be avoided if the free radical interacts with an antioxidant in cells. Antioxidants play a vital role in inhibiting molecular oxidation reactions to reduce the harmful accumulation of reactive oxygen species (Fiedor and Burda, 2014). Antioxidants also protect human;somatic cells from the deteriorating effects of free radicals and reactive oxygen species (ROS) by altering the expression of sensor proteins that are involved in oxidative stress (Figure 3) (Fiocchetti et al., 2019). The different kinds of chronic diseases and the process of lipid peroxidation are thus delayed. In recent years, there has been a great interest in unveiling natural plant-derived novel and safe dietary antioxidants.
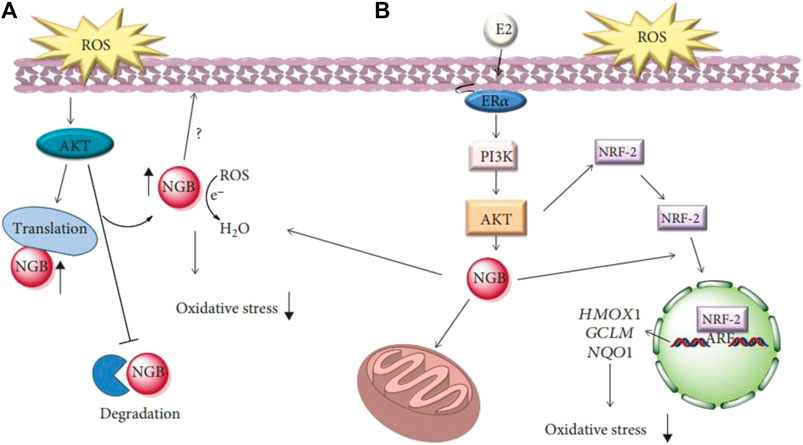
FIGURE 3. (A) Schematic model of ROS-activated signaling involved in the rapid modulation of neuroglobin (NGB) levels, its localization, and function on the redox balance outside mitochondria. (B) Schematic model of the impact of E2 intracellular-activated pathway on NGB expression, localization. The NRF-2 pathway describes how NGB affects the E2-dependent activation of the antioxidant NRF-2 system. E2: 17β-estradiol; ERα: estrogen receptor α; PI3K: phosphatidylinositol three kinase (Fiocchetti et al., 2019).
Biomass polyphenols have strong activity due to their ability to delocalize uncoupled electrons, which can scavenge free radicals, chelate metal ions and inhibiting oxidase activity, and protect endogenous antioxidant enzymes in the body (Kim et al., 2014; Croft, 2016). Most natural antioxidants are phenolic compounds. The most important natural antioxidants are tocopherols, flavonoids, and phenolic acids. Among the phenolic hydroxyl groups, the phenolic hydroxyl group is the most easily oxidized, exhibiting the capacity to capture free radicals such as ROS and active nitrogen species (Geng et al., 2016; Zheng et al., 2021). This functionality enables polyphenols to scavenge free radicals and quench ROS, thus providing strong antioxidant capacity (Fraga, 2007; Dugasani et al., 2010; Losada and Dí az, 2017). These antioxidants, which are commonly used as food supplements, prevent the free radical chain reaction of oxidation and inhibit the initiation and propagation steps. All of these lead to the termination of the reaction and delay of the oxidation process. Antioxidants have the unique property of extending the shelf life of foods without any adverse effect on their sensory or nutritional qualities. Antioxidants used as food additives are non-toxic and effective at low concentrations. Other outstanding properties include high stability, robustness to the various stages of food processing, possess no smell, taste, or color, are easy to be mixed into foodstuffs, and have sufficient solubility.
Biomass polyphenols have been widely used in various fields due to their strong antioxidant activities. Hu et al. (2020) impregnated tea polyphenols (Gallic acid) into tea seed oil with ethanol and removed the ethanol by vacuum distillation to produce tea polyphenol colloids. It was found that no chemical changes occurred after the addition of tea polyphenols to tea seed oil. The antioxidant stability of colloidal tea polyphenols in tea seed oil was superior to that of synthetic antioxidants and tea polyphenol palmitate, and the optimal addition of tea polyphenols to tea seed oil ranged from 0.1–0.2 g/kg. Ji et al. (2020) found that the major phenolics in sea buckthorn were flavonoids, phenolic acids, and tannins, which showed antioxidant functions via regulating the activities of cellular enzymes. Myint et al. (2021) found that stevia leaves were demonstrated to possess the highest antioxidant capacity among plant foods due to the abundance of polyphenols (PPS). The stevia leaves PPS showed antioxidant activity similar to epigallocatechin gallate (EGCG), and their antioxidant activity, hydrophilic activity, and stability are stronger than ascorbic acid (VC), vitamin E, and chlorogenic acid. The antioxidant activity of stevia leaves PPS is stable under various physical conditions, except for in the presence of potassium sorbate or sucrose. In addition, the combination of PPS and VC improves their antioxidant stabilities. Taken together, PPS has the potential to be a natural, inexpensive, and abundant antioxidant for use in pharmaceuticals and cosmetics. In animal studies, Gerasopoulos et al. (2015) have included polyphenols extracted from olive oil processing wastewater to feed 20-day-old piglets for 30 days. The authors found that the polyphenol-rich diet significantly increased levels of total antioxidant capacity, catalase activity, and glutathione in the pig’s blood, as well as reducing oxidative stress. Liu et al. (2018) and Cimmino et al. (2018) showed that biomass polyphenols reduced the content of malondialdehyde in mutton, the fat oxidation was inhibited followed by the improvement of meat quality. More importantly, the biomass flavonoid polyphenol fisetin has been shown to relieve allodynia in a reserpine-induced rat model with fibromyalgia, hyperalgesia, and depression. Through evaluating multiple parameters, the researchers suggest that fisetin lowered biogenic amine (5-hydroxytryptamine, noradrenaline, and dopamine) levels, inhibited the oxidation of nitroso stress to downregulate ROS level, to exert its resistance to hurt feelings and antidepressant potential.
In conclusion, biomass polyphenols show prominent antioxidant performance and free radical scavenging capabilities, which is of great significance to broadening their fields of research and various applications.
Antibacterial Activity
In recent years, consumers are increasingly intended to use natural extracts and other substances as potential antibiotics to inhibit the growth of pathogenic bacteria due to the concerns on the destruction of nutrition by sterilization technology and the abuse of synthetic antibiotics, as shown in Table 2 (Xu et al., 2019; Liu W. et al., 2020). Polyphenols are considered to be one of the intriguing natural extracts to hinder the growth and proliferation of bacteria via multiple modes of action, which include alteration of the bacterial membrane permeabilization, inhibition of the bacterial DNA gyrase, interference with the energy metabolism, and perturbation of the functions of bacterial porins (An et al., 2004; Gradisar et al., 2007; Wang et al., 2020; Yun et al., 2021). In addition, the presence of phenolic hydroxyl groups potentiates the antibacterial activities of polyphenols on damaging the structural integrity and functionality of bacterial membranes (Sousa et al., 2015).
Emerging evidence has demonstrated the beneficial effect of biomass polyphenols against bacteria. Pani et al. (2014) studied the toxicities of 29 polyphenols at different concentrations of the monophototoxin produced from Fusarium oxysporum in wheat. Most of the polyphenols exhibited an inhibitory rate of 70% against deoxynivalenol ranging from 1 to 1.5 mm. A serial of biomass polyphenols shows prominent inhibition on distinct strains of bacteria, fungi, and yeasts. Tea polyphenols are a kind of antimicrobial agent with a broad inhibitory spectrum on multiple pathogenic bacteria, such as Proteus common, Staphylococcus epidermidis, and Staphylococcus aureus. In addition, apple polyphenol extract elicits a suppression effect on the growth of Bacillus aerobics, Escherichia coli, Pseudomonas, and Bacillus subtilis. Pomegranate pulp is rich in eight different kinds of polyphenol compounds, all of which exert strong bacteriostatic abilities against Salmonella and Escherichia coli (Gullon et al., 2016). Flavonoids alone or in combination with known therapeutic agents effectively control S. aureus infection (Elmasri et al., 2015). More interestingly, Williams et al. (2017) found that oligoproanthocyanidins in grape dregs modulate intestinal microflora and alleviates intestinal Ascaris suum infection when grape dregs were added to the pig’s diet. When 10–40 g/kg grape seed powder was added to the chicken diet, it was found that the total number of Streptococcus, Escherichia coli, and microbial colonies in the chicken intestines decreased while the number of beneficial lactic acid bacteria increased in a dose-dependent manner (Hafsa and Ibrahim, 2017). Bearing this antibacterial activity in mind, researchers have endeavored to further investigate the mechanisms behind polyphenols’ bacteriostatic functionality.
Yang and Zhang (2019) delineated the bacteriostatic mechanism of tea polyphenols as shown in Figure 4. Their results showed that the electrolyte leakage rate of bacteria was significantly enhanced after treatment with different concentrations of tea polyphenols, indicating the impairment of bacterial membrane permeability in the presence of polyphenols. The bacterial membrane is mainly composed of lipid bilayers containing hydrophilic and hydrophobic ends. The binding between phenolic hydroxyl groups and hydrophilic ends triggers agglomeration of membrane lipids, thus destroying the bacterial membrane. Intriguingly, when inoculated in plants and fruits, polyphenolic compounds induce the activities of a couple of antibacterial enzymes, including phenylalanine aminase, catalase, peroxidase, polyphenol oxidase, chitinase and β-1, 3-glucanase, thereby improving the antibacterial abilities of plants and fruits (Ramadas et al., 2020).
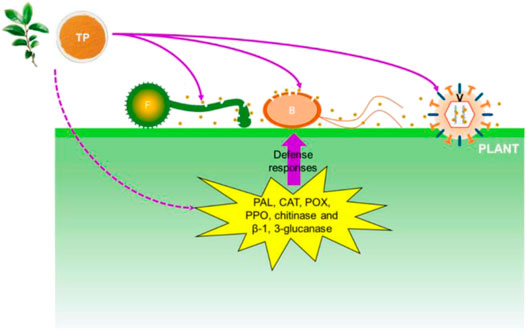
FIGURE 4. Antibacterial mechanism of polyphenols. Brown dots represent tea polyphenol. TP: tea polyphenol; F: fungi; B: bacteria; V: virus; PAL: phenylalanine ammonia-lyase; CAT: catalase; POX: peroxidase; PPO: polyphenoloxidase (Yang and Liu, 2019).
Currently, the studies on the antibacterial properties of biomass polyphenols continue to be carried out in breadth and depth. However, the structure-function relationship of polyphenols and the combinational applications of polyphenols with more prominent antibacterial effects require further investigation.
Antitumor and Anticancer Activity
Reactive oxygen free radicals are the metabolites of the Redox reaction in biological organisms. Under normal physiological conditions, the generation and scavenging of free radicals are finely balanced in a dynamic equilibrium. However, when the imbalance occurs, excessive free radicals will deteriorate the organisms, leading to aging and the increased incidence of a range of disorders (Zhang et al., 2020; Wang et al., 2021). The accumulation of free radicals generates direct damage on the genetic materials and other biological macromolecules, including the aberrant gene transcriptional activation, changes in the structural and functional identities of proteins, breakage, and polymerization of peptide bonds, and lipid peroxidation, leading to the occurrence of tumors and cancers (Figure 5) (Ríos-Arrabal et al., 2013). Polyphenols have attracted broad attention in cancer therapeutics due to their chemopreventive roles as both blocking and suppressing agents (Sharma et al., 2017; Sajadimajd et al., 2020). In terms of their blocking functions, polyphenols can avoid the activation of carcinogens, prevent the reactive carcinogens from interacting with critical DNA sites, and facilitate the metabolic clearance of carcinogens. Moreover, polyphenols are capable of suppressing oncogenesis and cancer progression, to elicit their chemopreventive functions on multiple stages of carcinogenesis (Zhou et al., 2016).
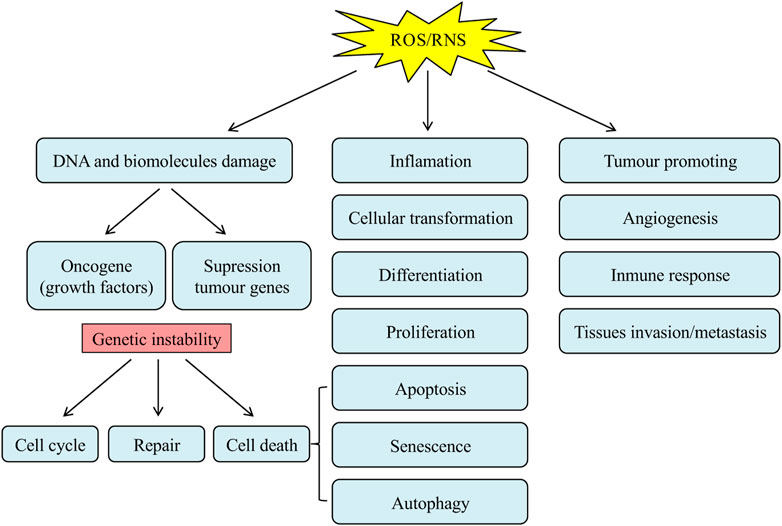
FIGURE 5. The role of ROS/RNS in carcinogenesis (Ríos-Arrabal et al., 2013).
Vegetables and fruits contain a wide variety of polyphenols, and studies have reported that regular consumption of fruits, vegetables, and nuts reduces the risk of various types of cancer, especially with a significant impact on gastric, esophageal, lung, oral, pharyngeal, pancreatic and colon cancers (Yi et al., 2019). Epidemiological and experimental studies have shown that consumption of food and beverages rich in polyphenols (such as catechins, flavonoids, and anthocyanins) is closely associated with a lower incidence of cancer (Naasani et al., 2003). Animal experiments have also demonstrated that food polyphenols effectively suppress chemical-induced tumors and inhibit tumor developments at multiple stages (Sharma et al., 2017; Sajadimajd et al., 2020). An increasing number of studies highlight the role of biomass polyphenols as potential anticancer cell mutagens. Han et al. (2019) showed that cranberry-extracted polyphenols are bioactive anticancer components, and they have dramatic capacities towards inhibiting the viability and colony formation of human colon cancer cells HCT116. Mechanistically, treatment of polyphenols caused the cell cycle arrest at G0/G1 phase and subsequently led to the induction of cell apoptosis. There is ample evidence showing that polyphenols target a variety of molecules that are involved in multiple cellular signaling pathways. Emerging evidence has shown that non-coding RNAs function as oncogenes or tumor suppressors in the regulation of tumorigenesis and tumor progression (Yi et al., 2019). The antitumor mechanisms of polyphenols are multi-targeted and include the activation of different pathways to induce apoptosis in cancer cells. Moreover, three predominant epigenetic changes (alterations in chromatin structure, DNA methylation, and regulation by microRNAs) are also involved in tumor cells treated with biomass polyphenols. As shown in Figure 6 (Yi et al., 2019), EGCG, curcumin, and resveratrol regulate multiple classes of miRNAs to elicit their antitumor potentials.
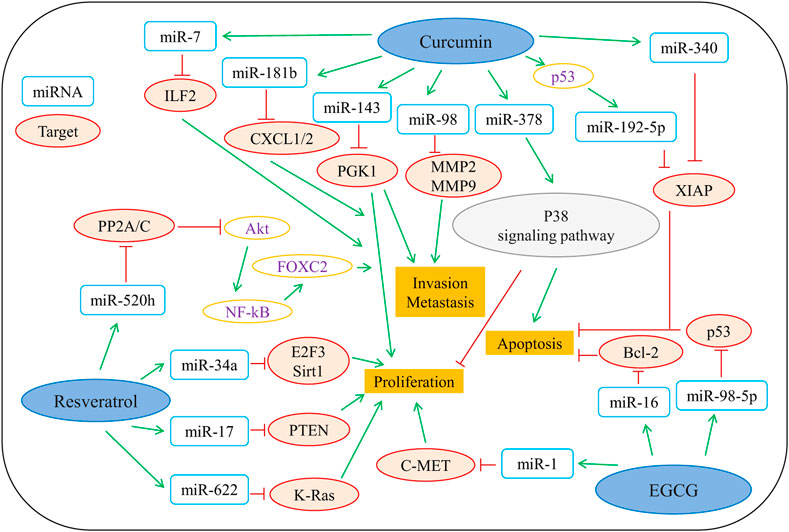
FIGURE 6. Representative polyphenols that are involved in regulating the antitumor mechanisms of microRNAs. MiR, microRNA; ILF2, Interleukin enhancer binding factor 2; CXCL1/2, chemokine (C-X-C motif) ligands 1/2; PGK1, phosphoglycerate kinase 1; MMP2/9, matrix metalloproteinase 2/9; XIAP, X-linked inhibitor of apoptosis; PP2A/C, protein phosphatase 2A/C; E2F3, E2F transcription factor 3; Sirt1, Sirtuin type 1; PTEN, phosphatase and tensin homolog; K-Ras, kirsten rat sarcoma; C-MET, cellular-mesenchymal epithelial transition factor; Bcl-2, B-cell lymphoma-2; p53, protein 53; P38 signaling pathway, protein 38 signaling pathway. reproduced with copyright permission from Elsevier (Yi et al., 2019).
Although multiple targets have been identified in terms of the antitumor/anticancer activities of the biomass polyphenols, the detailed mechanisms of how polyphenols are capable of controlling the expression of these genes/miRNAs remain elusive. It would therefore be interesting to select single or high purity polyphenols from natural product resources with strong antitumor/anticancer activities to further investigate their relationships with antitumor factors.
Neuroprotective Activity
The incidence of neurodegenerative disorders, such as Alzheimer’s disease and Parkinson’s disease, gradually increases with age (Remington et al., 2010; Figueira et al., 2017; Li, 2018). These types of disorders share common pathological hallmarks, including oxidative stress, neuroinflammation, protein aggregation, and mitochondrial dysfunction (Gu et al., 2021). Given their roles in mediating essential biological processes, including signal transduction, cell proliferation and apoptosis, and cell differentiation, polyphenols have been long taken as potential neuroprotective agents. More importantly, the neuroprotective function of polyphenols has been suggested to be associated with their antioxidant activities, especially towards scavenging ROS and nitric oxide (Zhen and Liu, 2018).
A growing number of studies have provided experimental evidence that the consumption of polyphenol-rich berry fruits is beneficial to the nervous system and shows the potential to mitigate age-dependent neurodegeneration via alleviating cognitive and motor dysfunctions (Figueira et al., 2017; Tavares et al., 2013). Moreover, the neuroprotective function has also been demonstrated on Gardenia jasminoides extract (GJE). The medium dose of GJE treatment showed the most effective inhibition of neuronal necrosis in different brain regions of the rat model of chronic cerebral ischemia (Figure 7) (Zhang et al., 2016). Green tea polyphenols have been demonstrated to play a neuroprotective role due to their antioxidant and anti-inflammatory properties (Sutherland et al., 2006; Song et al., 2019). Zhang et al. (2010) showed that a 30-days treatment with green tea polyphenols (200 mg/kg, twice a day) prominently restored blood-brain barrier permeability, rescued cerebral infarction and improved neurological functions in rats underwent cerebral ischemia. Moreover, the induction of caveolin-1 mRNA and hyperphosphorylation of extracellular signal-regulated kinase 1/2, markers of cerebral ischemia, were also found ameliorated in cerebral ischemic tissue. Liu et al. (2019) isolated four catechins, including two new catechin derivatives, from Anhua dark tea. The study showed that the compounds exhibited optimal neuroprotective effects by inhibiting N-methyl-p-aspartate (NMDA) receptors. It protected SH-SY5Y cells from NMDA-induced injury and apoptosis by regulating NR2B expression and activating PI3K/Akt signaling pathway. These compounds are expected to be effective therapeutic agents for the prevention of excitatory brain injury. Taken together, these findings emphasize that the antioxidant, anti-apoptotic, and reduction of brain edema activities of tea polyphenols are prerequisites for their neuroprotective functions.
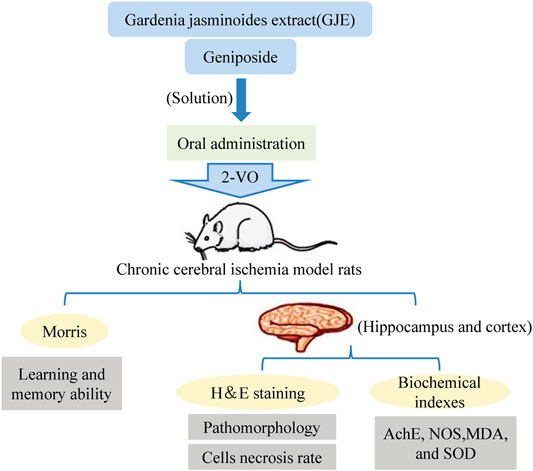
FIGURE 7. The neuroprotective roles of Gardenia jasminoides extract (GJE) and Geniposide on a rat model with chronic cerebral ischemia (Zhang et al., 2016).
Hypoglycemic and Lipid-Lowering Activity
High blood lipid content is one of the essential risk factors of fatty liver, cerebral infarction, coronary heart disease, and the formation of vascular sclerosis. Excessive accumulation of blood glucose in diabetic patients easily leads to acute severe metabolic disorders, for example, life-threatening hyperosmolar hyperglycemia syndrome. In the meantime, diabetic patients also suffer from infectious diseases, which lead to chronic complications including microangiopathy, diabetic nephropathy, and diabetic retinopathy. The nervous system complications may also be accompanied, such as peripheral neuropathy, autonomic neuropathy, and diabetic feet. All of these symptoms and complications severely ruin the quality of life of diabetic patients (Monika L. et al., 2019). Diabetic patients with combined neuropathy also develop pancreatic sclerosis and atrophy (He et al., 2020). Medicinal biomass has been applied to control diabetes and hyperlipidemia in different countries (Sugiyama et al., 2007; Yang et al., 2010; Saeed et al., 2012), and has become the major source of safe and effective hypoglycemic and hyperlipidemic drugs. Importantly, the hypoglycemic activity has been assigned to biomass polyphenols due to their capabilities in exerting antioxidant functions, promoting the synthesis and secretion of insulin, perturbing the activities of intestinal digestive enzymes, and inhibiting the glucose transport (Mahmood et al., 2013).
Chakraborty et al. (2012) emphasized that the role of 6-gingerol in controlling insulin responsiveness via regulating insulin secretion of mouse pancreas is essential for protecting the hyperglycemia and oxidative stress caused by arsenic. When the mice were fed with 6-gingerol for 12 days, Singh et al. (2009) reported a significant reduction of fasting blood glucose, accompanied by increased glucose tolerance and downregulation of plasma triglyceride (TG), total cholesterol (TC), insulin, low-density lipoprotein cholesterol (LDL-C) and free fatty acid (FFA) levels. These findings support the anti-hyperglycemia and cholesterol-lowering activities of 6-gingerol. The other kinds of biomass polyphenols function to increase insulin sensitivity and improve insulin resistance. Manzano et al. (Manzano et al., 2016) showed that apple polyphenols (APE, mainly quercetin and rutin) have therapeutic potential in the rat model of insulin resistance. Nutritional intervention with APE resulted in increased insulin sensitivity and a 45% increase in glucose infusion rate (GIR). Furthermore, in vitro results showed a synergistic effect between APE and insulin to increase glucose uptake through GLUT4 translocation in muscle cells. This translocation is mediated by the phosphatidylinositol 3-kinase (PI3K) and peroxisome proliferator-activated receptor-γ (PPARγ) signaling pathways. Xiong et al. (2020) also demonstrated that catechins, procyanidin Al and procyanidin A2 extracted from lychee seed LSF could activate the insulin signaling pathway and inhibit GSK-3β activity via the IRS-1/PI3K/Akt pathway, which in turn inhibited Tau hyperphosphorylation and ultimately improved cognitive function in AD rats. Kim et al. (2016) provided a summary of potential mechanisms by which dietary polyphenol metabolites improve glucose homeostasis (Figure 8).
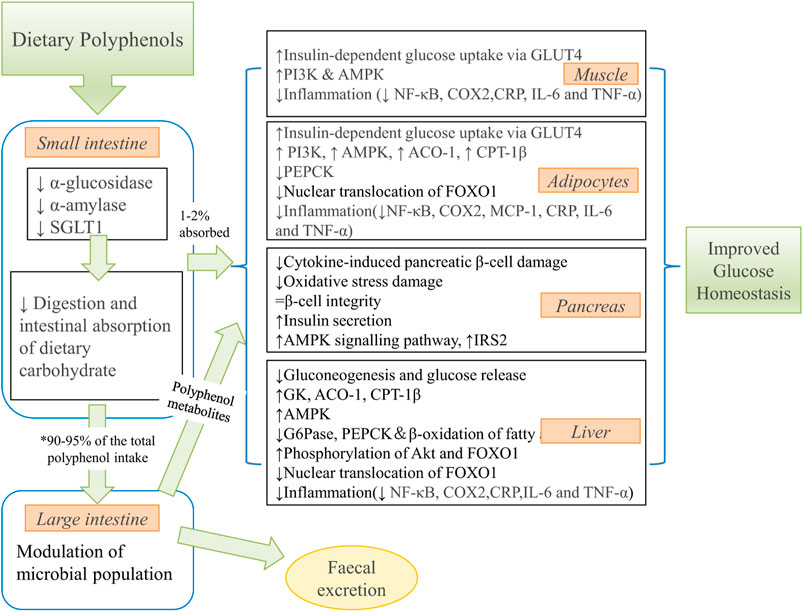
FIGURE 8. The summary of potential mechanisms linking dietary polyphenol metabolites to improved glucose homeostasis. ↑, increase; ↓, decrease. * 90–95% of the ingested polyphenols reach the colon. SGLT1, sodium-dependent glucose transporter; GLUT4, glucose transporter four; PI3K, phosphoinositide 3-kinase; AMPK, 5′ adenosine monophosphate-activated protein kinase; NF-κB, nuclear factor kappaB; COX2, cyclooxygenase-2 protein; CRP, C-reactive protein; IL-6, interleukin 6; TNFα, tumor necrosis factor α; ACO-1, acyl CoA oxidase-1; CPT-1β, carnitine palmitoyl transferase-1β; PEPCK, phosphoenolpyruvate carboxykinase; FOXO1, forkhead box protein O1; MCP-1, monocyte chemoattractant protein-1; IRS2, insulin receptor substrate two; GK, glucokinase; G6Pase, glucose-6-phosphatase. (reproduced with copyright permission from MPDI (Kim et al., 2016)).
Vroegrijk et al. (2011) found that male C57BL/J6 rats fed with a high-fat diet containing 1% pomegranate seed oil for 12 weeks showed reduced fat content and body weight compared with those fed with a full-fat diet. Additionally, Xu et al. (2009) studied the effect of pomegranate flower extract on hepatic fat accumulation in Zucker diabetic obese rats with severe fatty liver disease and highlighted the hypolipidemic effect of the isolated polyphenols. Yu et al. (2014) found that pomegranate leaves (PGL) have a similar modulating effect on lipid metabolism. Pomegranate leaves and their major active components (ellagic acid, gallic acid, pyrogallic gallic acid, and tannic acid) showed the effect of inhibiting pancreatic lipase activity in vitro. High doses of PGL inhibited intestinal lipase activity while promoting the expression of tight junction proteins, thereby inhibiting lipid absorption and reducing blood serum total cholesterol (TC) and triglyceride (TG) levels to prevent intestinal mucosal damage due to lipid overload.
Green tea polyphenols, grape polyphenols, citrus juice polyphenols, and sand buckthorn leaf polyphenols play similar roles in lowering blood sugar via multiple modes of action. In addition, several common fruit and vegetable polyphenols, such as pomegranate polyphenols, tea polyphenols, hawthorn polyphenols, and apple polyphenols, exert similar effects on downregulating TG, TC, and LDL-C levels while upregulating the level of high-density lipoprotein cholesterol. Currently, the study on the mechanisms of glucose- and lipid-lowering capabilities of biomass polyphenols has attracted much more attention but still requires further investigations.
Promotion of Gastrointestinal Health
Intestinal barriers refer to the intact structure and function of the intestine to prevent harmful substances such as bacteria and toxins from passing through the intestinal mucosa and entering other tissues, organs, and blood circulation in the human body. The normal intestinal mucosal barriers are composed of a mechanical barrier, a chemical barrier, an immune barrier, and a biological barrier, and the integrity of each intestinal barrier is indispensable to human health. The intestinal barriers maintain the normal intestinal permeability and regulate the transportation and absorption of nutrients (such as sugar, vitamins, amino acids, fatty acids, and other lipids) and other food-related compounds (such as polyphenols). In addition, intestinal barriers regulate the composition of bacteria from the lumen to the blood flow of transfer (Tangney and Rasmussen, 2013; Hidalgo-Liberona et al., 2020). The intestinal permeability is under control of a complex system of junctions known as tight junctions (TJ), gap junctions, and adhesion junctions. The system has consisted of numerous TJ proteins and junction adhesion molecules that control the flow among adjacent intestinal cells. It has been reported previously that polyphenols can mitigate leaky bowel disease by directly adjusting TJ function, enhancing the synthesis and redistribution of TJ proteins (such as occludin, claudins, and occludula), and suppressing the activities of different kinases involved in controlling TJ expression (Hidalgo-Liberona et al., 2020).
Gastrointestinal dysfunction is one of the major factors that contribute to type II diabetes, cardiovascular disease, insomnia, obesity, and other disorders (Kang, 2013; Kitai and Tang, 2017; Orr et al., 2020). Therefore, improvement of gastrointestinal function requires much more investigation. Previous studies (Pandey and Rizvi, 2009) have reported that polyphenols show antioxidant, anti-inflammatory, anti-fat, anti-diabetes, cardioprotective, neuroprotective, and anticarcinogenic effects via collaborating with the intestinal microbiota. Biomass polyphenols influence the activities of intestinal microflora, repair gastrointestinal mucosal damage, optimize the intestinal structure, and interact with other macromolecules to affect gastrointestinal function. Chen et al. (2018) showed that the addition of chlorogenic acid to weaning piglets led to an increase of immune globulin level, the expression of antiapoptotic protein B-cell lymphoma-2 was simultaneously upregulated in the duodenum and jejunum. This indicates that the intestinal beneficial effect of chlorogenic acid depends on the enhancement of immune function and suppression of excessive intestinal epithelial cell apoptosis. Liao et al. (2016) showed that upon treatment of tea polyphenols, the reduction of atherosclerosis plaque in mice negatively correlated with the increased number of bifidobacteria in their intestine, suggesting that tea polyphenols promote the proliferation of bifidobacteria and prevent lipid metabolism, thereby suppressing atherosclerosis. Biomass polyphenols were found to accelerate beneficial bacteria proliferation to improve the function of the intestines and stomach and repair the damaged intestinal cells. Zhao et al. (2018) showed that when treated with bitter butyl tea polyphenols, the reduction of gastric acid secretion and increase of gastric juice pH was detected in mice with gastric mucosa damage, indicating that bitter butyl tea polyphenols supplement was an effective approach to combat against gastric mucosa damage. With the increasing number of studies on the relationship between biomass polyphenols and gastrointestinal function, the development and utilization of biomass polyphenols as functional factors for the improvement of gastrointestinal function is expected to be broadened. Liu et al. (2020a) depicted the metabolic mechanisms of dietary polyphenols in the intestine (Figure 9). In the body, a small percentage of dietary polyphenols is first absorbed in the small intestine. They are then deconjugated, circulated, and distributed among organs or excreted in the urine. The remaining unabsorbed polyphenols reach the colon where they are catabolized by bacteria to produce metabolites either absorbed or excreted in feces. After intestinal and hepatic Phase I and II metabolism, the microbial-derived polyphenolic metabolites enter the systemic circulation. The metabolites in the liver could be excreted via the biliary duct and re-absorbed throughout the enterohepatic recirculation. In animal studies, the addition of polyphenols to diets reduced high-fat diet-induced obesity and modulated the gut microbiota by increasing the growth of short-chain fatty acid-producing bacteria and decreasing the growth of lipopolysaccharide-producing bacteria. More clinical trials are required to investigate the application of dietary polyphenols as nutritional or functional foods in the prevention and treatment of obesity in humans, and studies that aim at elucidating the mode of action of specific bacteria strains in mediating dietary polyphenols would be necessary.
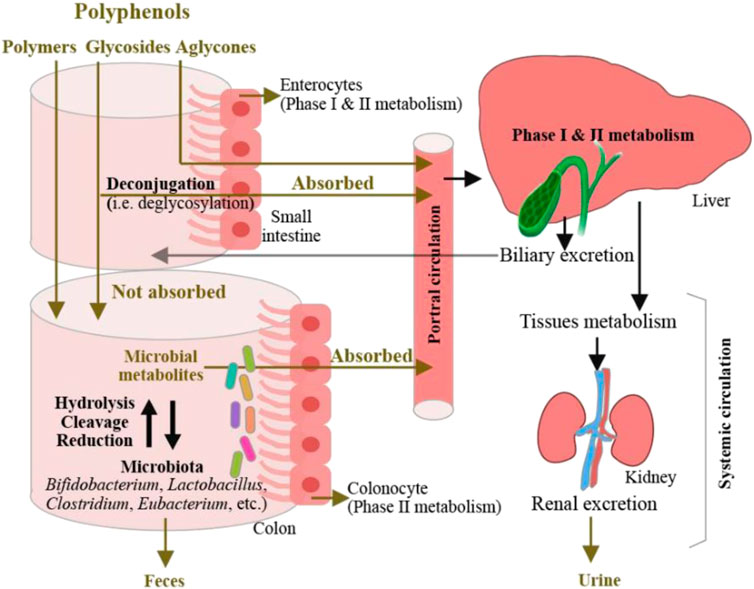
FIGURE 9. Absorption of dietary polyphenols and their microbial transformation in the intestine. [reproduced with copyright permission from ACS Publications (Liu J. et al., 2020)]
Conclusion and Perspectives
Although polyphenols have been considered as chemical impurities, recent studies and findings underlined its biological activities in terms of exerting antioxidant, antibacterial, antitumor, neuroprotection, regulation of blood lipid, and promotion of gastrointestinal health functions. This thus attracts more attention from researchers worldwide to further investigate the pharmacological applications of biomass polyphenols and use them as one of the major components in natural products-derived drugs. More interestingly, given that biomass polyphenols are enriched in daily food, this further highlights the essential contribution of polyphenols to human life and makes biomass polyphenols one of the research hotspots. A range of studies has demonstrated efficient extraction of polyphenols from tea, grape, pomegranate, rapeseed, and other raw materials, which are coincidentally used in medical treatments and as functional food supplements. Many diseases are associated with antioxidants, but given the purity of the extract and the complexity of the structure, polyphenols are currently only used as supplements for the treatment of diseases, and research into their use as medicines for the treatment of diseases still requires innovative extraction techniques and in-depth research into anti-disease mechanisms, to explore their therapeutic potential. Hence, the mechanisms of polyphenols’ pharmacological actions still require further investigation. It is hoped that with the increasing attention from researchers on natural drugs and the progress of scientific technology, more methods of rapid separation and preparation of polyphenols can be developed, and the underlying pharmacological mechanisms of polyphenols will be further elucidated to provide the material basis for further pharmacological examination and clinical investigation.
Author Contributions
ZC proposed the idea. BY and YH wrote the manuscript. ZC and QY wrote and revised the manuscript.
Funding
This work was supported by Natural Science Foundation of Jiangsu Province (BK20180772) and Qinglan project of Jiangsu Province. ZC was supported by a Postdoctoral Fellowship in Clinical Neurosciences program between The Chinese University of Hong Kong and University of Oxford (Nuffield Department of Clinical Neurosciences and Pembroke College).
Conflict of Interest
The authors declare that the research was conducted in the absence of any commercial or financial relationships that could be construed as a potential conflict of interest.
Publisher’s Note
All claims expressed in this article are solely those of the authors and do not necessarily represent those of their affiliated organizations, or those of the publisher, the editors and the reviewers. Any product that may be evaluated in this article, or claim that may be made by its manufacturer, is not guaranteed or endorsed by the publisher.
References
Abu Hafsa, S. H., and Ibrahim, S. A. (2017). Effect of Dietary Polyphenol-Rich Grape Seed on Growth Performance, Antioxidant Capacity and Ileal Microflora in Broiler Chicks. J. Anim. Physiol. Anim. Nutr. 102, 268–275. doi:10.1111/jpn.12688
An, B.-J., Kwak, J.-H., Son, J.-H., Park, J.-M., Lee, J.-Y., Jo, C., et al. (2004). Biological and Anti-microbial Activity of Irradiated green tea Polyphenols. Food Chem. 88, 549–555. doi:10.1016/j.foodchem.2004.01.070
Bhuyan, D. J. (2018). Phytochemicals Derived from Australian Eucalypts as Anticancer Agents for Pancreatic Malignancies (Doctoral Dissertation. Newcastle, England: The University of Newcastle.
Boadas-Vaello, P., Vela, J. M., and Verdú, E. (2017). New Pharmacological Approaches Using Polyphenols on the Physiopathology of Neuropathic Pain. Curr. Drug Targets 18, 160–173. doi:10.2174/1389450117666160527142423
Cardona, F., Andrés-Lacueva, C., Tulipani, S., Tinahones, F. J., and Queipo-Ortuño, M. I. (2013). Benefits of Polyphenols on Gut Microbiota and Implications in Human Health. J. Nutr. Biochem. 24, 1415–1422. doi:10.1016/j.jnutbio.2013.05.001
Chakraborty, D., Mukherjee, A., Sikdar, S., Paul, A., Ghosh, S., and Khuda-Bukhsh, A. R. (2012). [6]-gingerol Isolated from Ginger Attenuates Sodium Arsenite Induced Oxidative Stress and Plays a Corrective Role in Improving Insulin Signaling in Mice. Toxicol. Lett. 210, 34–43. doi:10.1016/j.toxlet.2012.01.002
Chen, J., Xie, H., Chen, D., Yu, B., Mao, X., Zheng, P., et al. (2018). Chlorogenic Acid Improves Intestinal Development via Suppressing Mucosa Inflammation and Cell Apoptosis in Weaned Pigs. ACS Omega 3, 2211–2219. doi:10.1021/acsomega.7b01971
Chen, J., Yu, Y., Zhu, B., Han, J., Liu, C., Liu, C., et al. (2021). Synthesis of Biocompatible and Highly Fluorescent N-Doped Silicon Quantum Dots from Wheat Straw and Ionic Liquids for Heavy Metal Detection and Cell Imaging. Sci. Total Environ. 765, 142754. doi:10.1016/j.scitotenv.2020.142754
Chen, S., Wang, G., Sui, W., Parvez, A. M., Dai, L., and Si, C. (2020). Novel Lignin-Based Phenolic Nanosphere Supported Palladium Nanoparticles with Highly Efficient Catalytic Performance and Good Reusability. Ind. Crops Prod. 145, 112164. doi:10.1016/j.indcrop.2020.112164
Chen, W., Chen, F., and Tian, J. (2002). Study on Heavy Sulfite Modification and Application of Bayberry Tannin Extract. Chem. Ind. For. Prod. 22, 55–58. doi:10.1097/00006982-200202000-00010
Cimmino, R., Barone, C. M. A., Claps, S., Varricchio, E., Rufrano, D., Caroprese, M., et al. (2018). Effects of Dietary Supplementation with Polyphenols on Meat Quality in Saanen Goat Kids. Bmc. Vet. Res. 14, 181. doi:10.1186/s12917-018-1513-1
Croft, K. D. (2016). Dietary Polyphenols: Antioxidants or Not? Arch. Biochem. Biophys. 595, 120–124. doi:10.1016/j.abb.2015.11.014
Dahibhate, N. L., Kumar, D., and Kumar, K. (2020). Determination of Bioactive Polyphenols in Mangrove Species and Their In-Vitro Anti-Candida Activities by Ultra-high-performance Liquid Chromatography - Electrospray Ionization - Tandem Mass Spectrometry (UPLC-ESI-MS/MS). Anal. Lett. 54 (4), 608–624. doi:10.1080/00032719.2020.1774600
Dai, L., Su, X., Lu, Y., Chen, S., and Wu, Z. (2006). Determination of Tannin Content of Valonea Tannin Extracts by Colloidal Titration. Biomass Chem. Eng. 40, 15–17.
Day, A. J., Cañada, F. J., Dı́az, J. C., Kroon, P. A., Mclauchlan, R., Faulds, C. B., et al. (2000). Dietary Flavonoid and Isoflavone Glycosides Are Hydrolysed by the Lactase Site of Lactase Phlorizin Hydrolase. Febs. Lett. 468, 166–170. doi:10.1016/s0014-5793(00)01211-4
de Camargo, A. C., Favero, B. T., Morzelle, M. C., Franchin, M., Alvarez-Parrilla, E., de la Rosa, L. A., et al. (2019). Is Chickpea a Potential Substitute for Soybean? Phenolic Bioactives and Potential Health Benefits. Ijms 20 (11), 2644. doi:10.3390/ijms20112644
de Sousa, J. P., de Oliveira, K. Á. R., de Figueiredo, R. C. B. Q., and de Souza, E. L. (2015). Influence of Carvacrol and 1,8-cineole on Cell Viability, Membrane Integrity, and Morphology of Aeromonas Hydrophila Cultivated in a Vegetable-Based Broth. J. Food Prot. 78, 424–429. doi:10.4315/0362-028x.jfp-14-242
Delgado, N. T. B., Rouver, W. N., and Santos, R. L. D. (2019). Protective Effects of Pomegranate in Endothelial Dysfunction. Curr. Pharm. Design. 26 (30), 3684–3699.
Dong, H., Zheng, L., Yu, P., Jiang, Q., Wu, Y., Huang, C., et al. (2020). Characterization and Application of Lignin-Carbohydrate Complexes from Lignocellulosic Materials as Antioxidants for Scavenging In Vitro and In Vivo Reactive Oxygen Species. ACS Sust. Chem. Eng. 8 (1), 256–266. doi:10.1021/acssuschemeng.9b05290
Dugasani, S., Pichika, M. R., Nadarajah, V. D., Balijepalli, M. K., Tandra, S., and Korlakunta, J. N. (2010). Comparative Antioxidant and Anti-inflammatory Effects of [6]-gingerol, [8]-gingerol, [10]-gingerol and [6]-shogaol. J. Ethnopharmacology 127 (2), 515–520. doi:10.1016/j.jep.2009.10.004
Elmasri, W. A., Zhu, R., Peng, W., Al-Hariri, M., Kobeissy, F., Tran, P., et al. (2015). Multitargeted Flavonoid Inhibition of the Pathogenic Bacterium Staphylococcus aureus: a Proteomic Characterization. J. Proteome Res. 16 (7), 2579–2586. doi:10.1021/acs.jproteome.7b00137
Fiedor, J., and Burda, K. (2014). Potential Role of Carotenoids as Antioxidants in Human Health and Disease. Nutrients 6, 466–488. doi:10.3390/nu6020466
Figueira, I., Tavares, L., Jardim, C., Costa, I., Terrasso, A. P., Almeida, A. F., et al. (2017). Blood-brain Barrier Transport and Neuroprotective Potential of Blackberry-Digested Polyphenols: an In Vitro Study. Eur. J. Nutr. 58 (1), 113–130. doi:10.1007/s00394-017-1576-y
Fiocchetti, M., Fernandez, V. S., Montalesi, E., and Marino, M. (2019). Neuroglobin: a Novel Player in the Oxidative Stress Response of Cancer Cells. Oxidative Med. Cell Longevity 2019, 1–9. doi:10.1155/2019/6315034
Fraga, C. G. (2007). Plant Polyphenols: How to Translate Their In Vitro Antioxidant Actions to In Vivo Conditions. Tbmb 59, 308–315. doi:10.1080/15216540701230529
Ge, G., Luo, S., and Ta, N. (2015). Extraction of Total Tannins and Determination of Amino Acids in Chinese Gallnut, Cinnamon and Pomegranate Bark. Inner. Mongolia. Pet. Ind. 6, 11–13.
Gee, J. M., Dupont, M. S., Day, A. J., Plumb, G. W., Williamson, G., and Johnson, I. T. (2000). Intestinal Transport of Quercetin Glycosides in Rats Involves Both Deglycosylation and Interaction with the Hexose Transport Pathway. J. Nutr. 130, 2765–2771. doi:10.1093/jn/130.11.2765
Geng, W., Narron, R., Jiang, X., Pawlak, J. J., Chang, H. M., Park, S., et al. (2016). The Influence of Lignin Content and Structure on Hemicellulose Alkaline Extraction for Non-wood and Hardwood Lignocellulosic Biomass. Cellulose 26, 3219–3230.
Geng, W., Venditti, R. A., Pawlak, J. J., Chang, H.-m., Pal, L., and Ford, E. (2020). Carboxymethylation of Hemicellulose Isolated from poplar (Populus Grandidentata) and its Potential in Water-Soluble Oxygen Barrier Films. Cellulose 27, 3359–3377. doi:10.1007/s10570-020-02993-2
Gerasopoulos, K., Stagos, D., Petrotos, K., Kokkas, S., Kantas, D., Goulas, P., et al. (2015). Feed Supplemented with Polyphenolic Byproduct from Olive Mill Wastewater Processing Improves the Redox Status in Blood and Tissues of Piglets. Food Chem. Toxicol. 86, 319–327. doi:10.1016/j.fct.2015.11.007
Gessner, M. O., and Steiner, D. (2005). Acid Butanol Assay for Proanthocyanidins (Condensed Tannins). Methods to Study Litter Decomposition. Springer Netherlands.
Gironi, F., and Piemonte, V. (2011). Temperature and Solvent Effects on Polyphenol Extraction Process from Chestnut Tree wood. Chem. Eng. Res. Des. 89, 857–862. doi:10.1016/j.cherd.2010.11.003
Goodman, B. A. (2020). Utilization of Waste Straw and Husks from rice Production: A Review. J. Bioresources Bioproducts 5 (3), 143–162. doi:10.1016/j.jobab.2020.07.001
Gradisar, H., Pristovsek, P., Plaper, A., and Jerala, R. (2007). Green tea Catechins Inhibit Bacterial DNA Gyrase by Interaction with its ATP Binding Site. J. Med. Chem. 50, 264–271. doi:10.1021/jm060817o
Gu, J., Guo, M., Huang, C., Wang, X., Zhu, Y., Wang, L., et al. (2021). Titanium Dioxide Nanoparticle Affects Motor Behavior, Neurodevelopment and Axonal Growth in Zebrafish (Danio rerio) Larvae. Sci. Total Environ. 754, 142315. doi:10.1016/j.scitotenv.2020.142315
Gullon, B., Pintado, M. E., Pérez-Álvarez, J. A., and Viuda-Martos, M. (2016). Assessment of Polyphenolic Profile and Antibacterial Activity of Pomegranate Peel (Punica Granatum) Flour Obtained from Co-product of Juice Extraction. Food Control 59, 94–98. doi:10.1016/j.foodcont.2015.05.025
Han, Y., Huang, M., Li, L., Cai, X., Gao, Z., Li, F., et al. (2019). Non-extractable Polyphenols from Cranberries: Potential Anti-inflammation and anti-colon-cancer Agents. Food Funct. 10, 7714–7723. doi:10.1039/c9fo01536a
He, Y., Jin, Y., Li, X., Wu, L., and Jin, C. (2020). Quantification of Pancreatic Elasticity in Type 2 Diabetes: a New Potential Imaging Marker for Evaluation of Microangiopathy. Eur. J. Radiol. 124, 108827. doi:10.1016/j.ejrad.2020.108827
Hidalgo-Liberona, N., González-Domínguez, R., Vegas, E., Riso, P., Del Bo’, C., Bernardi, S., et al. (2020). Increased Intestinal Permeability in Older Subjects Impacts the Beneficial Effects of Dietary Polyphenols by Modulating Their Bioavailability. J. Agric. Food Chem. 68, 12476–12484. doi:10.1021/acs.jafc.0c04976
Hu, L. W., Li, T., Luo, Q., and Zhang, J. S. (2020). Antioxidant Stability of Colloidal tea Polyphenols in tea Seed Oil. J. Food Process. Pres. 45 (2), e15130. doi:10.1111/jfpp.15130
Ji, M., Gong, X., Li, X., Wang, C., and Li, M. (2020). Advanced Research on the Antioxidant Activity and Mechanism of Polyphenols from Hippophae Species-A Review. Molecules 25 (4), 917. doi:10.3390/molecules25040917
Kang, Y. S. (2013). Obesity Associated Hypertension: New Insights into Mechanism. Electrolyte Blood Press. 11, 46–52. doi:10.5049/ebp.2013.11.2.46
Kim, H.-S., Quon, M. J., and Kim, J.-a. (2014). New Insights into the Mechanisms of Polyphenols beyond Antioxidant Properties; Lessons from the green tea Polyphenol, Epigallocatechin 3-gallate: Lessons from the green tea Polyphenol, Epigallocatechin 3-ganate. Redox Biol. 2, 187–195. doi:10.1016/j.redox.2013.12.022
Kim, Y., Keogh, J., and Clifton, P. (2016). Polyphenols and Glycemic Control. Nutrients 8 (1), 17. doi:10.3390/nu8010017
Kitai, T., and Tang, W. H. W. (2017). The Role and Impact of Gut Microbiota in Cardiovascular Disease. Revista Española de Cardiología (English Edition) 70, 799–800. doi:10.1016/j.rec.2017.04.007
Li, J. (2018). Neuroprotective Effect of (-)-Epigallocatechin-3-Gallate on Autoimmune Thyroiditis in a Rat Model by an Anti-inflammation Effect, Anti-apoptosis and Inhibition of TRAIL Signaling Pathway. Exp. Ther. Med. 15, 1087–1092. doi:10.3892/etm.2017.5511
Liao, Z.-L., Zeng, B.-H., Wang, W., Li, G.-H., Wu, F., Wang, L., et al. (2016). Impact of the Consumption of Tea Polyphenols on Early Atherosclerotic Lesion Formation and Intestinal Bifidobacteria in High-Fat-Fed ApoE−/− Mice. Front. Nutr. 3, 42. doi:10.3389/fnut.2016.00042
Liu, D., Meng, S., Xiang, Z., He, N., and Yang, G. (2019). Antimicrobial Mechanism of Reaction Products of Morus Notabilis (mulberry) Polyphenol Oxidases and Chlorogenic Acid. Phytochemistry 163, 1–10. doi:10.1016/j.phytochem.2019.03.026
Liu, H. W., Zhao, J. S., Li, K., and Deng, W. (2018). Effects of Chlorogenic Acids-Enriched Extract from Eucommia Ulmoides Leaves on Growth Performance, Stress Response, Antioxidant Status and Meat Quality of Lambs Subjected or Not to Transport Stress, Stress Response, Antioxidant Status and Meat Quality of Lambs Subjected or Not to Transport Stress. Anim. Feed Sci. Tech. 238, 47–56. doi:10.1016/j.anifeedsci.2018.02.003
Liu, J., Fan, Y., Kim, D., Zhong, T., Yi, P., Fan, C., et al. (2019). Neuroprotective Effect of Catechins Derivatives Isolated from Anhua Dark tea on NMDA-Induced Excitotoxicity in SH-Sy5y Cells. Fitoterapia 137, 104240. doi:10.1016/j.fitote.2019.104240
Liu, J., He, Z., Ma, N., and Chen, Z.-Y. (2020a). Beneficial Effects of Dietary Polyphenols on High-Fat Diet-Induced Obesity Linking with Modulation of Gut Microbiota. J. Agric. Food Chem. 68 (1), 33–47. doi:10.1021/acs.jafc.9b06817
Liu, K., Du, H., Zheng, T., Liu, H., Zhang, M., Xie, H., et al. (2021). Recent Advances in Cellulose and its Derivatives for Oilfield Applications. Carbohyd. Polym. 117740. doi:10.1016/j.carbpol.2021.117740
Liu, W., Du, H., Zhang, M., Liu, K., Liu, H., Xie, H., et al. (2020b). Bacterial Cellulose-Based Composite Scaffolds for Biomedical Applications: a Review. ACS Sust. Chem. Eng. 8 (20), 7536–7562. doi:10.1021/acssuschemeng.0c00125
Liu, Z., Bruins, M. E., Ni, L., and Vincken, J.-P. (2018). Green and Black tea Phenolics: Bioavailability, Transformation by Colonic Microbiota, and Modulation of Colonic Microbiota. J. Agric. Food Chem. 66, 8469–8477. doi:10.1021/acs.jafc.8b02233
Losada-Barreiro, S., and Bravo-Díaz, C. (2017). Free Radicals and Polyphenols: the Redox Chemistry of Neurodegenerative Diseases. Eur. J. Med. Chem. 133, 379–402. doi:10.1016/j.ejmech.2017.03.061
Luo, Y., Li, Y., Cao, L., Zhu, J., Deng, B., Hou, Y., et al. (2021). High Efficiency and Clean Separation of eucalyptus Components by Glycolic Acid Pretreatment. Bioresour. Tech. 341, 125757. doi:10.1016/j.biortech.2021.125757
Mahmood, D. F. D., Abderrazak, A., El Hadri, K., Simmet, T., and Rouis, M. (2013). The Thioredoxin System as a Therapeutic Target in Human Health and Disease. Antioxid. Redox Signaling 19 (11), 1266–1303. doi:10.1089/ars.2012.4757
Manach, C., Scalbert, A., Morand, C., Rémésy, C., and Jiménez, L. (2004). Polyphenols: Food Sources and Bioavailability. Am. J. Clin. Nutr. 79, 727–747. doi:10.1093/ajcn/79.5.727
Martin, D. A., and Bolling, B. W. (2015). A Review of the Efficacy of Dietary Polyphenols in Experimental Models of Inflammatory Bowel Diseases. Food Funct. 6, 1773–1786. doi:10.1039/c5fo00202h
Michaličková, D., Belović, M., Ilić, N., Kotur-Stevuljević, J., Slanař, O., and Šobajić, S. (2019). Comparison of Polyphenol-Enriched Tomato Juice and Standard Tomato Juice for Cardiovascular Benefits in Subjects with Stage 1 Hypertension: a Randomized Controlled Study. Plant Food Hum. Nutr. 74 (1), 122–127.
Monika, L., Heidemarie, A., Claudia, F., Markus, K., Wolfgang, S., and Gerd, K. (2019). Diabetische neuropathie und diabetischer fuß (update 2019). Wien. Klin. Wochenschr. 131, 141–150.
Myint, K. Z., Zhou, Z. Y., Xia, Y. M., Fang, Y., Wu, M. N., Song, Z., et al. (2021). Stevia Polyphenols: A Stable Antioxidant that Presents a Synergistic Effect with Vitamin C. J. Food Process. Pres. 45 (4), e15317. doi:10.1111/jfpp.15317
Naasani, I., Oh-Hashi, F., Oh-Hara, T., Feng, W. Y., Johnston, J., Chan, K., et al. (2003). Blocking Telomerase by Dietary Polyphenols Is a Major Mechanism for Limiting the Growth of Human Cancer Cells In Vitro and In Vivo. Cancer Res. 63, 824–830.
Orr, W. C., Fass, R., Sundaram, S. S., and Scheimann, A. O. (2020). The Effect of Sleep on Gastrointestinal Functioning in Common Digestive Diseases. Lancet Gastroenterol. Hepatol. 5, 616–624. doi:10.1016/s2468-1253(19)30412-1
Pandey, K. B., and Rizvi, S. I. (2009). Plant Polyphenols as Dietary Antioxidants in Human Health and Disease. Oxidative Med. Cell Longevity 2, 270–278. doi:10.4161/oxim.2.5.9498
Pani, G., Scherm, B., Azara, E., Balmas, V., Jahanshiri, Z., Carta, P., et al. (2014). Natural and Natural-like Phenolic Inhibitors of Type B Trichothecene In Vitro Production by the Wheat (Triticum sp.) Pathogen fusarium Culmorum. J. Agric. Food Chem. 62, 4969–4978. doi:10.1021/jf500647h
Pei, W., Chen, Z. S., Chan, H. Y. E., Zheng, L., Liang, C., and Huang, C. (2020). Isolation and Identification of a Novel Anti-protein Aggregation Activity of Lignin-Carbohydrate Complex from Chionanthus Retusus Leaves. Front. Bioeng. Biotechnol. 8, 573991. doi:10.3389/fbioe.2020.573991
Porter, L. J. (1992). Structure and Chemical Properties of the Condensed Tannins. Plant Polyphenols, 245–258. doi:10.1007/978-1-4615-3476-1_14
Quideau, S., Deffieux, D., Douat-casassus, C., and Pouységu, L. (2011). Plant Polyphenols: Chemical Properties, Biological Activities, and Synthesis, Biological Activities, and Synthesis. Angew. Chem. Int. Ed. 50, 586–621. doi:10.1002/anie.201000044
Ramadas, D., Kowti, R., Ramesh, B., Nune, S. K., Joshi, V., and District, M. (2020). Antibacterial Effect of Polyphenols Enriched Drumstick Plant Leaves (Moringa Oleifera) Extract: A Research Study. Int. J. Innovative Sci. Res. Tech. 5, 1281–1283.
Remington, R., Chan, A., Lepore, A., Kotlya, E., and Shea, T. B. (2010). Apple Juice Improved Behavioral but Not Cognitive Symptoms in Moderate-To-Late Stage Alzheimer's Disease in an Open-Label Pilot Study. Am. J. Alzheimers Dis. Other Demen. 25, 367–371. doi:10.1177/1533317510363470
Ríos-Arrabal, S., Artacho-Cordón, F., León, J., Román-Marinetto, E., del Mar Salinas-Asensio, M., Calvente, I., et al. (2013). Involvement of Free Radicals in Breast Cancer. SpringerPlus 2, 404. doi:10.1186/2193-1801-2-404
Saeed, S., Mosa-Al-Reza, H., Fatemeh, A., and Saeideh, D. (2012). Antihyperglycemic and Antihyperlipidemic Effects of Guar Gum on Streptozotocin-Induced Diabetes in Male Rats. Phcog Mag. 8, 65–72. doi:10.4103/0973-1296.93328
Sajadimajd, S., Bahramsoltani, R., Iranpanah, A., Kumar Patra, J., Das, G., Gouda, S., et al. (2020). Advances on Natural Polyphenols as Anticancer Agents for Skin Cancer. Pharmacol. Res. 151, 104584. doi:10.1016/j.phrs.2019.104584
Salto, R., and Lopez, J. (2016). Apple Polyphenol Extract Improves Insulin Sensitivity In Vitro and In Vivo in Animal Models of Insulin Resistance. Nutr. Metab. 13, 1–10.
Sharma, P., Montes de Oca, M. K., Alkeswani, A. R., McClees, S. F., Das, T., Elmets, C. A., et al. (2017). Tea Polyphenols for the Prevention of UVB-Induced Skin Cancer. Photodermatol. Photoimmunol Photomed. 34 (1), 50–59. doi:10.1111/phpp.12356
Si, C.-L., Kim, J.-K., Bae, Y.-S., and Li, S.-M. (2009). Phenolic Compounds in the Leaves of Populus Ussuriensisand Their Antioxidant Activities. Planta Med. 75, 1165–1167. doi:10.1055/s-0029-1185476
Singh, A., Akanksha Singh, N., Maurya, R., and Srivastava, A. K. (2009). Anti-hyperglycaemic, Lipid Lowering and Anti-oxidant Proper- Ties of (6)-gingerol in Db/db Mice. Int. J. Med. Med. Sci. 1 (12), 536–544.
Song, Y., Li, X., Gong, X., Zhao, X., Ma, Z., Xia, T., et al. (2019). Green tea Polyphenols Improve Isoflurane-Induced Cognitive Impairment via Modulating Oxidative Stress. J. Nutr. Biochem. 73, 108213. doi:10.1016/j.jnutbio.2019.07.004
Sugiyama, H., Akazome, Y., Shoji, T., Yamaguchi, A., Yasue, M., Kanda, T., et al. (2007). Oligomeric Procyanidins in Apple Polyphenol Are Main Active Components for Inhibition of Pancreatic Lipase and Triglyceride Absorption. J. Agric. Food Chem. 55, 4604–4609. doi:10.1021/jf070569k
Sun, S., Zhang, M., Umemura, K., and Zhao, Z. (2019). Investigation and Characterization of Synthesis Conditions on Sucrose-Ammonium Dihydrogen Phosphate (SADP) Adhesive: Bond Performance and Chemical Transformation. Materials 12, 4078. doi:10.3390/ma12244078
Sutherland, B. A., Rahman, R. M. A., and Appleton, I. (2006). Mechanisms of Action of green tea Catechins, with a Focus on Ischemia-Induced Neurodegeneration. J. Nutr. Biochem. 17 (5), 291–306. doi:10.1016/j.jnutbio.2005.10.005
Suzuki, T. (2013). Regulation of Intestinal Epithelial Permeability by Tight Junctions. Cell. Mol. Life Sci. 70, 631–659. doi:10.1007/s00018-012-1070-x
Tangney, C. C., and Rasmussen, H. E. (2013). Polyphenols, Inflammation, and Cardiovascular Disease. Curr. Atheroscler. Rep. 15, 324. doi:10.1007/s11883-013-0324-x
Tavares, L., Figueira, I., Mcdougall, G. J., Vieira, H. L. A., Stewart, D., Alves, P. M., et al. (2013). Neuroprotective Effects of Digested Polyphenols from Wild Blackberry Species. Eur. J. Nutr. 52, 225–236. doi:10.1007/s00394-012-0307-7
Vroegrijk, I. O. C. M., van Diepen, J. A., van den Berg, S., Westbroek, I., Keizer, H., Gambelli, L., et al. (2011). Pomegranate Seed Oil, a Rich Source of Punicic Acid, Prevents Diet-Induced Obesity and Insulin Resistance in Mice. Food Chem. Toxicol. 49, 1426–1430. doi:10.1016/j.fct.2011.03.037
Wang, P., Yin, B., Dong, H., Zhang, Y., Zhang, Y., Chen, R., et al. (2020). Coupling biocompatible au nanoclusters and cellulose nanofibrils to prepare the antibacterial nanocomposite films. Front. Bioeng. Biotechnol. 8, 986. doi:10.3389/fbioe.2020.00986
Wang, X., Tang, S., Chai, S., Wang, P., Qin, J., Pei, W., et al. (2021). Preparing Printable Bacterial Cellulose Based Gelatin Gel to Promote In Vivo Bone Regeneration. Carbohydr. Polym. 270 (15), 118342. doi:10.1016/j.carbpol.2021.118342
Wang, Z., Li, S., Ge, S., and Lin, S. (2020). Review of Distribution, Extraction Methods, and Health Benefits of Bound Phenolics in Food Plants. J. Agric. Food Chem. 68, 3330–3343. doi:10.1021/acs.jafc.9b06574
Williams, A. R., Krych, L., Fauzan Ahmad, H., Nejsum, P., Skovgaard, K., Nielsen, D. S., et al. (2017). A Polyphenol-Enriched Diet and ascaris Suum Infection Modulate Mucosal Immune Responses and Gut Microbiota Composition in Pigs. Plos One 12, e0186546. doi:10.1371/journal.pone.0186546
Xiong, R., Wang, X.-L., Wu, J.-M., Tang, Y., Qiu, W.-Q., Shen, X., et al. (2020). Polyphenols Isolated from Lychee Seed Inhibit Alzheimer's Disease-Associated Tau through Improving Insulin Resistance via the IRS-1/PI3K/Akt/GSK-3β Pathway. J. Ethnopharmacology 251, 112548. doi:10.1016/j.jep.2020.112548
Xu, H., Zhang, D., and Li, J. (2019). Antibacterial Nanoparticles with Universal Adhesion Function Based on Dopamine and Eugenol. J. Bioresour. Bioprod. 4 (3), 177–182.
Xu, K. Z.-Y., Zhu, C., Kim, M. S., Yamahara, J., and Li, Y. (2009). Pomegranate Flower Ameliorates Fatty Liver in an Animal Model of Type 2 Diabetes and Obesity. J. Ethnopharmacology 123, 280–287. doi:10.1016/j.jep.2009.03.035
Xu, L., Yang, Y., and Wang, F. (2016). Callus culture of acacia mearnsii de wilde and analysis on proanthocyanidin. Chem. Ind. For. Prod. 36, 53–59.
Yang, B., and Liu, X. (2019). Extraction and Antioxidant Activity of Polyphenols from phyllanthus Emblica. Food Ind. Sci. Technol. 40, 151–155.
Yang, D.-J., Chang, Y.-Y., Hsu, C.-L., Liu, C.-W., Lin, Y.-L., Lin, Y.-H., et al. (2010). Antiobesity and Hypolipidemic Effects of Polyphenol-Rich Longan (Dimocarpus Longans lour.) Flower Water Extract in Hypercaloric-Dietary Rats. J. Agric. Food Chem. 58, 2020–2027. doi:10.1021/jf903355q
Yang, Y., and Zhang, T. (2019). Antimicrobial Activities of tea Polyphenol on Phytopathogens: A Review. Molecules 24, 816. doi:10.3390/molecules24040816
Yashunsky, D. V., Men´shov, V. M., Tsvetkov, D. E., Tsvetkov, Y. E., Bel´ko, A. A., Vasiyarov, G. G., et al. (2014). Analysis of Content of (-)-secoisolariciresinol and Related Polyphenols in Different Morphological Parts and Anatomical Structures of Larch wood from Siberia. Russ. Chem. Bull. 63 (11), 2571–2576. doi:10.1007/s11172-014-0780-7
Yi, J., Li, S., Wang, C., Cao, N., Qu, H., Cheng, C., et al. (2019). Potential Applications of Polyphenols on Main ncRNAs Regulations as Novel Therapeutic Strategy for Cancer. Biomed. Pharmacother. 113, 108703. doi:10.1016/j.biopha.2019.108703
Yu, P., Zheng, L., Wang, P., Chai, S., Zhang, Y., Shi, T., et al. (2020). Development of a Novel Polysaccharide-Based Iron Oxide Nanoparticle to Prevent Iron Accumulation-Related Osteoporosis by Scavenging Reactive Oxygen Species. Int. J. Biol. Macromolecules 165, 1634–1645. doi:10.1016/j.ijbiomac.2020.10.016
Yu, Z., Zhang, Z., Wang, Z., Wang, G., Wang, G., and Liu, Z. (2014). The Extraction Technology and Antioxidant Activity of Polyphenol from Larch. Food Sci. Tech. 39, 207–212. doi:10.1021/acsomega.9b02071
Yun, J., Wei, L., Li, W., Gong, D., Qin, H., Feng, X., et al. (2021). Isolating High Antimicrobial Ability Lignin from Bamboo Kraft Lignin by Organosolv Fractionation. Front. Bioeng. Biotech. 9, 683796. doi:10.3389/fbioe.2021.683796
Zhang, H., Lai, Q., Li, Y., Liu, Y., and Yang, M. (2016). Learning and Memory Improvement and Neuroprotection of Gardenia Jasminoides (Fructus Gardenia) Extract on Ischemic Brain Injury Rats. J. Ethnopharmacol. 196, 225–235. doi:10.1016/j.jep.2016.11.042
Zhang, L. L., Zhang, L. F., and Xu, J. G. (2020). Chemical Composition, Antibacterial Activity and Action Mechanism of Different Extracts from Hawthorn (Crataegus Pinnatifida Bge.). Sci. Rep. 10 (1), 8876–8913. doi:10.1038/s41598-020-65802-7
Zhang, L., Sun, C., Li, J., and Liu, Y. (2005). Research Status and Development prospect of Plant Polyphenols. For. Sci. 41, 157–162.
Zhang, S., Liu, Y., Zhao, Z., and Xue, Y. (2010). Effects of green tea Polyphenols on Caveolin-1 of Microvessel Fragments in Rats with Cerebral Ischemia. Neurol. Res. 32, 963–970. doi:10.1179/016164110x12700393823570
Zhao, X., Huang, C., Xiao, D., Wang, P., Luo, X., Liu, W., et al. (2021). Melanin-inspired Design: Preparing Sustainable Photothermal Materials from Lignin for Energy Generation. ACS Appl. Mater. Inter. 13 (6), 7600–7607. doi:10.1021/acsami.0c21256
Zhao, X., Sun, P., Li, G., Yi, R., Qian, Y., and Park, K.-Y. (2018). Polyphenols in Kuding tea Help Prevent Hcl/ethanol-Induced Gastric Injury in Mice. Food Funct. 9, 1713–1725. doi:10.1039/c7fo01754e
Zhen, N., and Liu, M. (2018). Research Progress on Neuroprotective Effects of Polyphenols. South Forum 49, 30–31.
Zheng, L., Yu, P., Zhang, Y., Wang, P., Yan, W., Guo, B., et al. (2021). Evaluating the Bio-Application of Biomacromolecule of Lignin-Carbohydrate Complexes (LCC) from Wheat Straw in Bone Metabolism via ROS Scavenging. Int. J. Biol. Macromolecules 176, 13–25. doi:10.1016/j.ijbiomac.2021.01.103
Zhou, Q., Bennett, L. L., and Zhou, S. (2016). Multifaceted Ability of Naturally Occurring Polyphenols against Metastatic Cancer. Clin. Exp. Pharmacol. Physiol. 43 (4), 394–409. doi:10.1111/1440-1681.12546
Keywords: biomass, polyphenols, biological application, bioactivities, health functions
Citation: Yan B, Chen ZS, Hu Y and Yong Q (2021) Insight in the Recent Application of Polyphenols From Biomass. Front. Bioeng. Biotechnol. 9:753898. doi: 10.3389/fbioe.2021.753898
Received: 05 August 2021; Accepted: 31 August 2021;
Published: 13 September 2021.
Edited by:
Jia-Long Wen, Beijing Forestry University, ChinaReviewed by:
Caili Fu, National University of Singapore Suzhou Research Institute, ChinaChuan-Ling Si, Tianjin University of Science and Technology, China
Wenhui Geng, North Carolina State University, United States
Copyright © 2021 Yan, Chen, Hu and Yong. This is an open-access article distributed under the terms of the Creative Commons Attribution License (CC BY). The use, distribution or reproduction in other forums is permitted, provided the original author(s) and the copyright owner(s) are credited and that the original publication in this journal is cited, in accordance with accepted academic practice. No use, distribution or reproduction is permitted which does not comply with these terms.
*Correspondence: Qiang Yong, swhx@njfu.com.cn
†These authors have contributed equally to this work and share first authorship