- 1National Engineering Laboratory for Cereal Fermentation Technology, Jiangnan University, Wuxi, China
- 2Science Center for Future Foods, Jiangnan University, Wuxi, China
- 3Key Laboratory of Industrial Biotechnology, Ministry of Education, School of Biotechnology, Jiangnan University, Wuxi, China
- 4Jiangsu Provisional Research Center for Bioactive Product Processing Technology, Jiangnan University, Wuxi, China
Scleroglucan is a non-ionic water-soluble polysaccharide, and has been widely used in the petroleum, food, medicine and cosmetics industries. Currently, scleroglucan is mainly produced by Sclerotium rolfsii. A higher level of scleroglucan (42.0 g/L) was previously obtained with S. rolfsii WSH-G01. However, the production of scleroglucan was reduced despite a higher glucose concentration remaining. Additionally, the molecular weight of scleroglucan was large, thus restricted its application. In this study, by adjusting the state of seeds inoculated, the degradation issue of scleroglucan during the fermentation process was solved. By comparing different fed-batch strategies, 66.6 g/L of scleroglucan was harvested by a two-dose fed-batch mode, with 53.3% glucose conversion ratio. To modify the molecular weight of scleroglucan, a combination method with HCl and high-pressure homogenization treatment was established. Finally, scleroglucan with molecular weight of 4.61 × 105 Da was obtained. The developed approaches provide references for the biosynthesis and molecular weight modification of polysaccharides.
Introduction
Scleroglucan is a microbial exopolysaccharide, and is a typical β-glucan (Bai et al., 2021; Valdez et al., 2021). It consists of backbone β-1,3-linked-glucopyranosyl residues and branches of β-1,6-linked-glucopyranosyl residues. Due to its special structure of every three sugar residues of the main chain bearing a single β-1,6-linked-glucopyranosyl residue, the branching degree of scleroglucan is up to 0.33 (Castillo et al., 2015). This characteristic of high branching frequency endows scleroglucan with high water solubility different to other types of β-glucans. In addition, scleroglucan is reported to have a higher molecular weight (Tan et al., 2019; Elsehemy et al., 2020). Due to these outstanding properties of a unique chemical structure and higher molecular weight, scleroglucan has significant advantages in terms of water solubility, biocompatibility, pseudoplasticity, resistance to hydrolysis, salt tolerance, moisture retention and viscosity stability (Barcelos et al., 2020; Song et al., 2020). It has been applied in the petroleum, food, medicine and cosmetics industries (Giavasis, 2014; Li et al., 2020c).
Scleroglucan is mainly produced by the genus Sclerotium sp., including S. rolfsii, S. glucanicum, and S. delphinii (Schmid et al., 2011; Gao et al., 2021). S. rolfsii is the dominant producer, which can accumulate high concentrations of scleroglucan with diverse substrates, such as glucose, sucrose, xylose and molasses (Survase et al., 2007b; Taskin et al., 2010; Leonor Valdez et al., 2019). Strategies have been introduced to enhance its production, most of which were focused on the selection of medium components and cost-effective optimization of controlling fermentation conditions (Survase et al., 2007a; Zhang et al., 2017; Valdez et al., 2021). In our previous research, 42.0 g/L of scleroglucan was obtained with the screened strain S. rolfsii WSH-G01 (Tan et al., 2019). The molecular weight of the obtained scleroglucan reached 108 Da and even 109 Da, while application of the polysaccharide was greater when its molecular weight was below 106. The function of scleroglucan was reported to be closely related to its molecular weight. Water solubility is poor when the molecular weight is too large, while the physiological function is lost when the molecular weight is too low (Kulicke et al., 1997; Farina et al., 2001; Castillo et al., 2015). Therefore, the modification of scleroglucan to obtain an ideal molecular weight is significant for improving its water solubility while maintaining its original function.
Currently, the commonly used methods for polysaccharide hydrolysis include physical hydrolysis, acid hydrolysis and enzymatic hydrolysis (He et al., 2018; Hu et al., 2021). Physical hydrolysis is a relatively fast and clean method, and includes microwave, irradiation and ultrasonication; however, the application of these methods is limited due to low yield and high cost (Liu et al., 2021a). Recently, high-pressure homogenization (HPH) as an emerging technology was used for polysaccharide hydrolysis (Belmiro et al., 2018; Xie et al., 2021). Acid hydrolysis is usually used for the degradation of polysaccharides by controlling the pH value. However, this method has some drawbacks, such as a wide distribution of product molecular weight and poor homogeneity formation (Lin et al., 2017). Enzymatic hydrolysis is considered the optimal choice for the high regional-selectivity and stereoselectivity of enzymes, but it also requires special conditions for storage, reaction and removal from the polysaccharide system (Cristina Vallejo-Garcia et al., 2019; Zheng et al., 2020). For modification of the molecular weight of scleroglucan, a suitable hydrolase with efficient capacity for scleroglucan degradation was not found after the expression and identification of β-glucanases from different microorganisms (Zeng et al., 2021). Co-culture with Pichia pastoris GS115 expressed an endo-β-1,3-glucanase (glycoside hydrolase family 55) from Trichoderma harzianum with S. rolfsii WSH-G01, and the final polymerization degree was only 2–17 (Gao et al., 2021), which did not fulfill the common requirement that the molecular weight be between 105 and 106 Da.
In S. rolfsii, it was reported that β-glucanases and β-1,3-glucanases were expressed to degrade scleroglucan in the late fermentation stage (Farina et al., 2009; Tan et al., 2019). Based on the whole genome sequencing, some β-glucanases and β-1,3-glucanases were discovered and then overexpressed in Pichia pastoris in our previous study. However, the results showed that the hydrolysis effects of these identified β-glucanases on scleroglucan degradation were extremely weak (Zeng et al., 2021). In addition, the utilization of the acid hydrolysis method alone to obtain the appropriate molecular weight of modified scleroglucan was also ineffective. In the present study, the fermentation process was optimized to further enhance scleroglucan production. Furthermore, the scleroglucan degradation method based on HPH treatment was investigated to obtain the appropriate molecular weight of scleroglucan. Finally, 66.6 g/L of scleroglucan was produced by a two-stage fed-batch fermentation strategy. In addition, an HCl-HPH combination method was also established for scleroglucan degradation, which resulted in the molecular weight of scleroglucan being degraded to 4.61 × 105 Da. The methods developed in this study could provide a reference for the efficient fermentation, production and degradation of scleroglucan and other polysaccharides.
Materials and Methods
Strains
The wild-type strain S. rolfsii WSH-G01 (CCTCC M2017646), which is a scleroglucan overproducer screened in our previous work, was used in this study (Gao et al., 2018).
Medium and Culture Conditions
The medium for slant, seed cultures contained the following: 30.0 g/L glucose, 1.0 g/L yeast extract, 3.0 g/L NaNO3, 1.0 g/L KH2PO4, 0.5 g/L KCl, and 0.5 g/L MgSO4·7H2O, at pH 4.0. In the slant medium, 20 g/L agar was added. The fermentation medium contained the following: 95.0 g/L initial glucose, 1.0 g/L yeast extract, 3.0 g/L NaNO3, 0.5 g/L KCl, 0.5 g/L MgSO4·7H2O, 1.0 g/L KH2PO4, 1.5 g/L citric acid, at an initial pH of 4.0. All the components were autoclaved for 20 min at 115°C (Tan et al., 2019). In addition, different concentrations of glucose sterilized before addition to the medium were added based on the different fermentation controlling strategies.
S. rolfsii was activated on PDA (potato dextrose agar) plates at 30°C for 96 h and then inoculated into 500 ml shaking flasks containing 50 ml culture medium for 60 h at 200 r/min and 30°C on a reciprocal shaker (Zhichu, Shanghai, China). The fed-batch fermentation was performed in a 5 L fermenter (T&J Bioengineering, Shanghai, China) with a 3.5 L working volume at 400 r/min and 1.0 vvm (volume air per volume). The pH was controlled automatically by adding 4.0 mol/L NaOH or 4.0 mol/L HCl according to the different strategies. The size of the inoculation was 5% (v/v) and all cultivations were carried out at 30°C. Different fed-batch strategies are investigated. The initial glucose content was 95 g/L and the total concentration of glucose was 125 g/L. For the two-dose fed-batch mode, glucose was intermittently fed twice with 15 g/L each time at 36 and 60 h, respectively. For the three-dose fed-batch mode, glucose was intermittently fed three times with 10 g/L each time at 36, 48, and 60 h, respectively. For the constant rate feeding fed-batch mode, glucose was constantly fed at a rate of 1.25 g/(L·h) during 36–60 h. All fermentations were performed in triplicate and the results presented as mean values.
Combined HCl-HPH Treatment of Scleroglucan
The pure sample of scleroglucan extracted from fermentation broth (the extraction method of scleroglucan was showed in Analytical Method) was re-dissolved in distilled water. Then, 0.20 mol/L of HCl was added and the solution was hydrolyzed in a water bath at 90°C for different times (2, 4, 6, 8, and 10 h). After cooling to room temperature, the pH of the hydrolysis system was adjusted to 7.0 with 2.0 mol/L NaOH. The HPH treatment was then conducted at 80 MPa for 25 s and the operation was repeated nine cycles.
Analytical Method
Determination of dry cell weight (DCW): The samples of fermentation broth were diluted five times with distilled water and the pH was adjusted to 7.0 with 2.0 mol/L NaOH or 2.0 mol/L HCl. The diluent solutions were then centrifuged at 10,000 × g for 30 min after being heated at 80°C for 30 min in a water bath. The cells were washed three times with ultrapure water and then dried at 105°C to a constant weight.
Extraction of scleroglucan and determination of its production: The fermentation broths obtained at different time points were diluted 3-fold by distilled water and mixed well. The supernatant was then obtained by centrifuging at 10,000 × g after being heated at 80 C in a water bath for 30 min. The pH of the supernatant was adjusted to 7.0 with 2.0 mol/L NaOH or 2.0 mol/L HCl. After adding an equal volume of anhydrous ethanol, scleroglucan was harvested by the alcohol deposition method at 4 C for 16 h. The production of scleroglucan was determined after freeze-drying to constant weight.
Determination of the concentrations of glucose and organic acids: Glucose and oxalic acid were determined by high performance liquid chromatography (HPLC, Agilent 1260, CA, United States) with an Aminex HPX-87H column (Bio-Rad, CA, United States). The elution conditions were as follows: 5 mmol/L H2SO4 of mobile phase, 0.4 ml/min flow rate, 40°C column temperature and 10 µl injection volume. Glucose was detected by applying the differential refraction index detector, and oxalic acid was detected by an UV detector at 210 nm (Gao et al., 2018; Tan et al., 2019).
Determination of the molecular weight of the polysaccharide: The molecular weight of scleroglucan treated with HPH was determined by high performance gel penetration chromatography (HPGPC, Waters, MA, United States) with a Shodex OHpak SB-806M HQ (Shodex, Tokyo, Japan). The detection conditions were as follows: parallax detector, 0.1 mol/L NaNO3 of mobile phase, 1.0 ml/min flow rate, 40°C column temperature and 50 µl injection volume. The differential refraction index detector was used.
Results and Discussion
Solution of the Scleroglucan Degradation During the Fermentation Process
In a previous study, 42.0 g/L of scleroglucan was produced by S. rolfsii WSH-G01, and further enhancement of scleroglucan production was difficult. The production of scleroglucan was gradually decreased and the content of substrate glucose was increased in the late stage of the fermentation process (Tan et al., 2019). The expression of β-glucanases and β-1,3-glucanases was initially considered to be the main reason for this phenomenon, as studies have reported that S. rolfsii can express some glucanohydrolases that degrade scleroglucan into glucose, providing energy to maintain basic cell growth and metabolism (Bateman, 1972; Martin et al., 2007). However, the effect of β-glucanases on scleroglucan degradation was determined to be weak through mining the autologous β-glucanase genes in S. rolfsii (Zeng et al., 2021). In addition, it was reported that other factors could also affect accumulation of the target product during the fermentation process, such as the seed culture and fermentation conditions (Sun et al., 2020b; Tian et al., 2020).
To solve the issue of scleroglucan degradation, adjustments to the fermentation process were introduced. The seeds inoculated were in the mid-log phase, and the seed culture time was adjusted from 72 to 60 h on the reciprocal shaker. In addition, to better suit the changed state of the seeds inoculated, three different time points for pH adjustment (36, 45, and 54 h) were compared based on a previously established pH-shift strategy and one-dose fed-batch strategy. The results are shown in Figure 1. The titer of scleroglucan was 27.7 g/L, 32.2 g/L and 32.2 g/L according to the three time points of pH adjustment at 36, 45, and 54 h, respectively. Compared with the previous results (Tan et al., 2019), the titer of scleroglucan was reduced, but the decrease in scleroglucan production did not appear in the late stage of the fermentation process. In addition, accumulation of the by-product oxalic acid was below 5 g/L. Considering the increased rate of scleroglucan in the late stage of the fermentation process, the time point for pH adjustment at 54 h was selected for the subsequent optimization process.
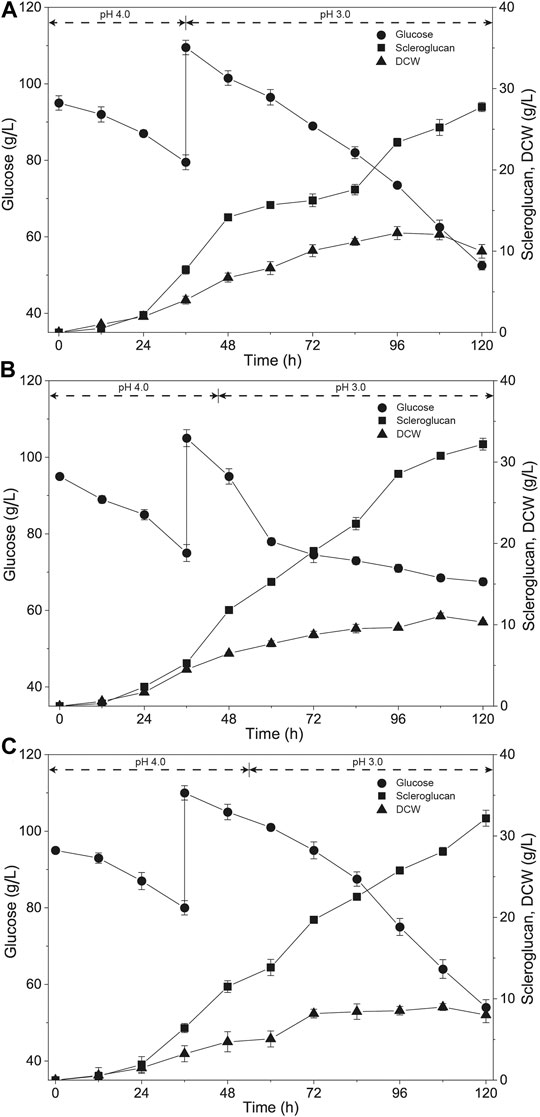
FIGURE 1. Time course of scleroglucan production using different pH-shift controlling fermentation strategies. The pH was controlled at 4.0 in the early fermentation process, and then adjusted to 3.0 at different times. (A) The pH was controlled at 4.0 before 36 h, and maintained at 3.0 after 36 h. (B) The pH was controlled at 4.0 before 45 h, and maintained at 3.0 after 45 h. (C) The pH was controlled at 4.0 before 54 h, and maintained at 3.0 after 54 h.
Comparison of Different Fed-Batch Strategies on Scleroglucan Production
To further enhance the production of scleroglucan, various feeding strategies were tested with the exception for the previously applied single-dose fed-batch mode. These strategies included the two-dose fed-batch mode, three-dose fed-batch mode and constant rate feeding fed-batch mode. The results are shown in Figure 2. The two-dose fed-batch mode yielded the best results, with the highest scleroglucan production being 66.6 g/L, a glucose conversion ratio of 53.3%, and productivity of 0.4 g/(L·h). Compared with the fed-batch process, the titer of scleroglucan was enhanced by 106.8%, and compared with the previous result (Tan et al., 2019), the titer of scleroglucan was enhanced by 58.7%.
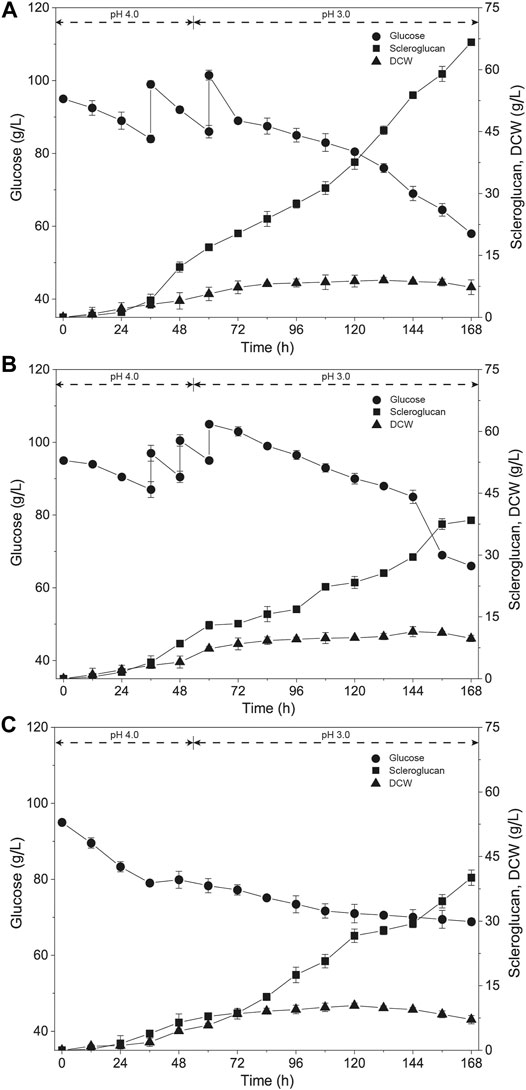
FIGURE 2. Time course of scleroglucan production using different feeding methods. The initial glucose concentration was 95.0 g/L and 30.0 g/L of glucose was added with different feeding approaches. (A) Two-dose fed-batch strategy (15.0 g/L of glucose was added at 36 and 60 h, respectively). (B) Three-dose fed-batch strategy, (10.0 g/L of glucose was added at 36, 48, and 60 h, respectively). (C) Constant speed feeding strategy (1.25 g/(L·h) of glucose was continuously added from 36 to 60 h).
The fed-batch mode is a usual strategy for improving the output of some target products, including the feeding of substrate or other ingredients. Different feeding modes also have diverse influences, such as the intermittent fed-batch mode and constant rate feeding fed-batch mode (Sun et al., 2020a; Liu et al., 2021b). In our previous research, diverse fed-batch strategies were established for specific products with different microorganisms, such as the one-dose fed-batch mode for enhancement of 2-phenylethanol (Tian et al., 2020), a multi-intermittent fed-batch mode for 2-keto-D-gluconic acid production (Zeng et al., 2019), and a constant rate feeding fed-batch mode for the simultaneous biosynthesis of α-ketoglutarate and pyruvate (Zeng et al., 2017). In this study, the production and glucose conversion efficiency were significantly enhanced with the intermittent fed-batch mode, while the productivity showed a slight decline. In the future, optimization could be conducted to further enhance the productivity and scleroglucan production, such as adding nitrogen sources, and improving the performance of strain and fermentation parameters.
Effects of HPH Treatment on Scleroglucan Molecular Weight
Homogenization technology is the most widely used method for emulsifying and refining. The high-pressure homogenization (HPH) procedure possesses distinguishing advantages in high shear stress, high-frequency oscillation, and cavitation (Xie et al., 2018; Li et al., 2020b). It is often employed in food processing to reduce the particle size of solid substances and improve the quality of products, such as yogurt, fruit and vegetable juice (Wellala et al., 2020; Levy et al., 2021). HPH technology has been increasingly used in polysaccharide processing, and is mainly used to reduce the molecular weight of polysaccharides. For example, the structural characteristics of tamarind seed polysaccharides were obviously changed after HPH treatment, and therefore, affected the physicochemical properties of corn starch (Xie et al., 2021). In addition, it was reported that the viscosity of polysaccharides could also be reduced by HPH treatment (Villay et al., 2012).
To modify the molecular weight of scleroglucan for wider application value, the effects of HPH treatment on scleroglucan molecular weight was investigated. The content of scleroglucan in the sample was 1.0 g/L. The effects of different pressures (20, 40, 60, and 80 MPa) and different cycles (1, 3, 5, 7, and 9) were compared (Figures 3A–E). The result showed that HPH treatment has an effect on reducing the molecular weight of scleroglucan. The molecular weight of scleroglucan in the range of 106–108 was determined after HPH treatment, and the range of molecular weight was gradually degraded with increasing treatment pressure and cycle. A type of scleroglucan with a molecular weight of 5.6 × 106 Da was obtained under the treatment conditions of 80 MPa and nine cycles. However, scleroglucan with the highest molecular weight was not degraded. Degradation was not obviously affected by increasing the number of cycles below 80 MPa (Figure 3F). In addition, it was not feasible to obtain the desired scleroglucan with lower molecular weight by further increasing the processing pressure, which was limited by the experimental equipment.
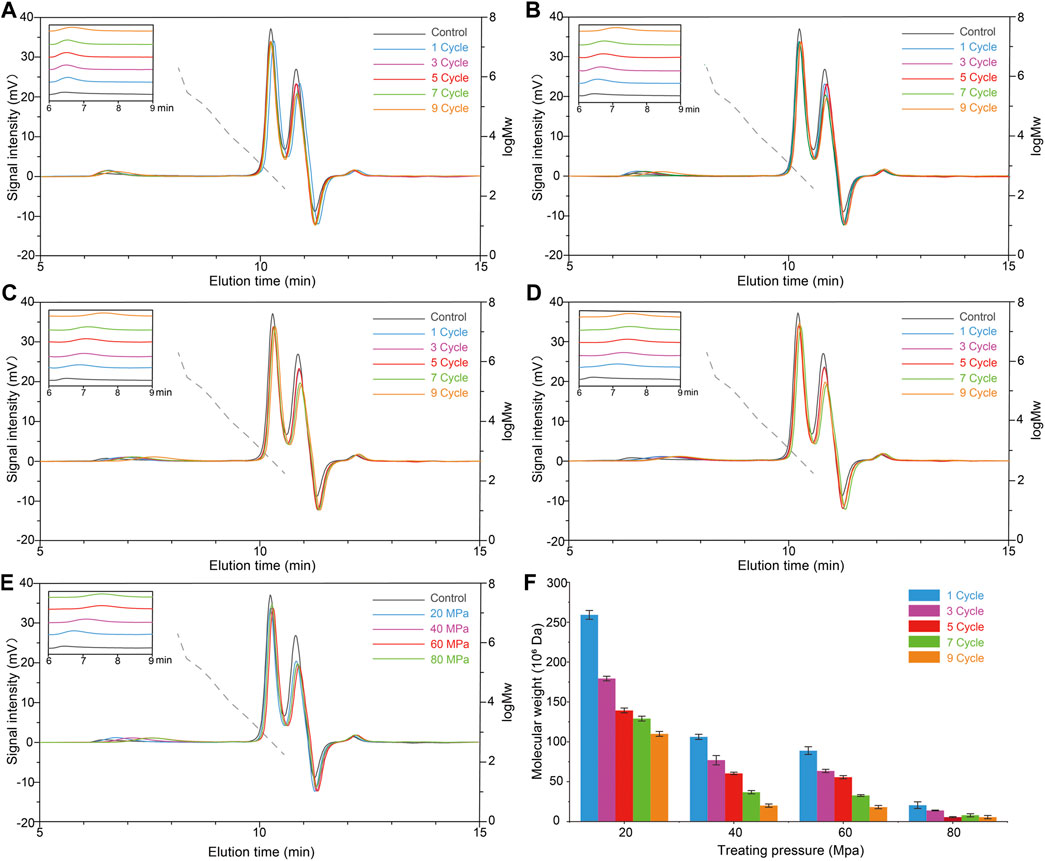
FIGURE 3. Effects of different pressures and cycles with HPH treatment on the molecular weight of scleroglucan. (A) Molecular weight (MW) chromatogram of scleroglucan treated with 20 MPa. (B) Molecular weight chromatogram of scleroglucan treated with 40 MPa. (C) Molecular weight chromatogram of scleroglucan treated with 60 MPa. (D) Molecular weight chromatogram of scleroglucan treated with 80 MPa. (E) Molecular weight chromatogram of scleroglucan treated with different pressures for nine cycles. (F) Distribution of scleroglucan in the molecular weight range of 106–108. The control was the sample not treated with HPH. The dotted lines represent the standard curve of scleroglucan molecular weight (1.80 × 102–2.00 × 106 Da). MW, Molecular weight.
Establishment of the HCl-HPH Combination Method for Scleroglucan Degradation
The molecular weight of the obtained scleroglucan was still too large, which was limited by utilization of the HPH treatment alone. Other strategies should be included to further modify scleroglucan. Recently, several combination methods with different single processes were established for polysaccharide modification, such as enzymatic hydrolysis combined with hydrothermal pretreatment for pectin (Wang et al., 2021), acid hydrolysis combined with an induced electric field for guar gum, chitosan and pectin (Li et al., 2020a). Acid hydrolysis with HCl, H2SO4 or organic acids is an effective method for polysaccharide degradation (Nguyen et al., 2020; Shi et al., 2020; Liu et al., 2021a). In this study, an HCl-HPH combination method was attempted to establish scleroglucan degradation. Based on the obtained conditions of HPH treatment, the parameters of HCl treatment were further optimized.
Firstly, the effects of different HCl concentrations (0.05, 0.1, 0.20, 0.30, and 0.50 mol/L) on the molecular weight of scleroglucan were investigated. Treatment with HCL only at 60°C for 2 h was not obtained the desired molecular weight of scleroglucan (Supplementary Table S1). Combining with HPH treatment, the molecular weight could be modified, and reduced to 1.62 × 106 Da with 0.2 mol/L HCl pretreatment, but it still did not reach the desired range. It was found that the molecular weight decreased with increased HCl concentration at 0.05–0.20 mol/L, while it increased with continuous enhanced HCl concentration (Figure 4A, Supplementary Table S2). It was speculated that the higher molecular weight of scleroglucan was decomposed by high HCl concentrations, which usually has better capacity in polysaccharide degradation (Nguyen et al., 2020). Additionally, the degradation effects were further tested with different HCl concentrations (0.1, 0.2, and 0.3 mol/L) under higher treatment temperatures (70, 80, and 90°C) (Figures 4B–D). The results showed that the effect of pretreatment with 0.2 mol/L HCl at 90°C was best, producing scleroglucan of 1.32 × 106 Da (Supplementary Table S3).
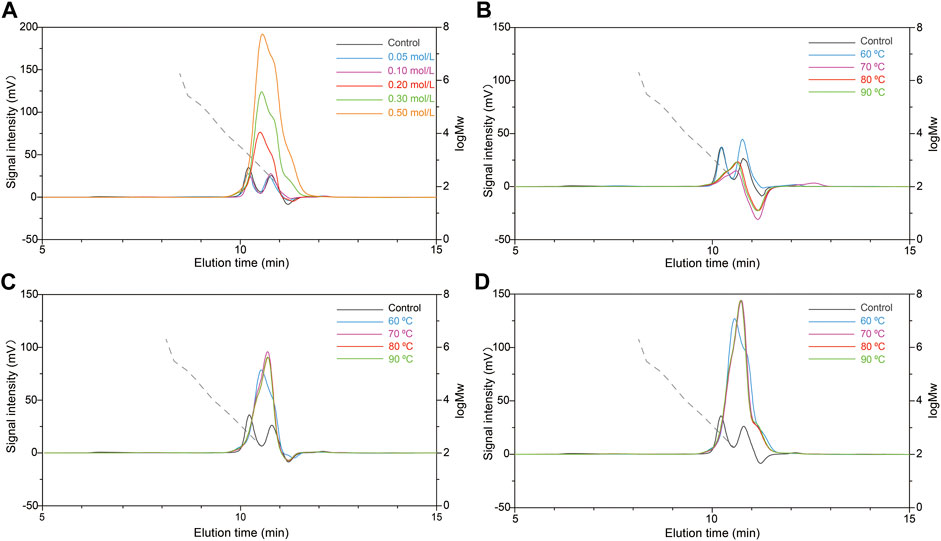
FIGURE 4. Effects of different HCl pretreatment conditions with the combination HCl-HPH method on molecular weight of scleroglucan. (A) Molecular weight chromatogram of scleroglucan pretreated with different concentrations of HCl (0.05, 0.10, 0.2, 0.30, and 0.50 mol/L) using the HCl-HPH method. (B) Molecular weight chromatogram of scleroglucan pretreated with 0.10 mol/L HCl using the HCl-HPH method at different temperatures. (C) Molecular weight chromatogram of scleroglucan pretreated with 0.20 mol/L HCl using the HCl-HPH method at different temperatures. (D) Molecular weight chromatogram of scleroglucan pretreated with 0.30 mol/L HCl using the HCl-HPH method at different temperatures. The control was the sample not pretreated with HCl. The dotted lines represent the curve of scleroglucan molecular weight (1.80 × 102–2.00 × 106 Da). MW, Molecular weight.
The influence of prolonged HCl pretreatment was further investigated. Simultaneously, different initial concentrations of scleroglucan (1.0, 2.5, 5.0, 7.5, and 10.0 g/L) with higher molecular weight were selected, which were extracted from samples in the middle of the scleroglucan fermentation process. Figure 5 shows the molecular weight changes in scleroglucan at different times with HCl pretreatment. The specific molecular weight of scleroglucan ranged from 105 to 106 Da and its peak proportion in the samples are shown in Table 1. These results showed that the established HCl-HPH combination method could be applied to modify scleroglucan. The desired molecular weight of modified scleroglucan was 4.61 × 105 Da and 8.88 × 105 Da by treating an initial 2.5 g/L of scleroglucan and 5.0 g/L of scleroglucan for 10 h, respectively, and the peak proportions were 4.80 and 2.55%, respectively. In the future, several optimizations should be introduced to enhance the peak proportion of the target modified scleroglucan. For example, because of limiting by the experimental equipment, the effect of higher processing pressure on the scleroglucan modification was not implemented in this study. The results showed that the degradation effect was obvious with the increased pressure, which could be considered for further dealing with the large amounts and high production. In addition, the enzymatic hydrolysis could also be combined with the established HCl-HPH method, though there are still no reports about enzymes that can efficiently degrade scleroglucan.
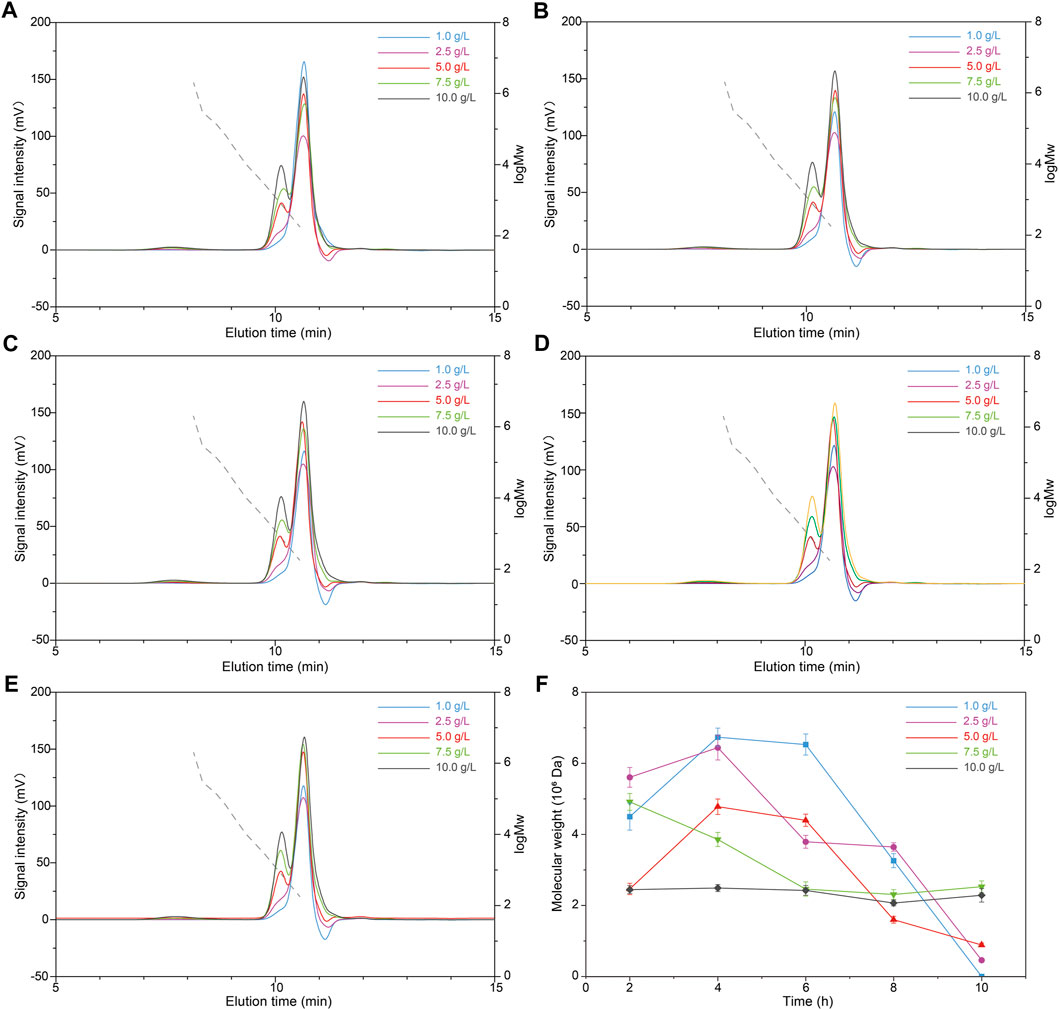
FIGURE 5. Effects of different scleroglucan concentrations with the combination HCl-HPH method on the molecular weight of scleroglucan. (A) Molecular weight chromatogram of different scleroglucan concentrations treated using the HCl-HPH method for 2 h. (B) Molecular weight chromatogram of different scleroglucan concentrations treated using the HCl-HPH method for 4 h. (C) Molecular weight chromatogram of different scleroglucan concentrations treated using the HCl-HPH method for 6 h. (D) Molecular weight chromatogram of different scleroglucan concentrations treated using the HCl-HPH method for 8 h. (E) Molecular weight chromatogram of different scleroglucan concentrations treated using the HCl-HPH method for 10 h. (F) Molecular weight change of scleroglucan (with high or medium molecular weight) treated using the HCl-HPH method for the samples with different scleroglucan concentrations. The dotted lines represent the curve of scleroglucan molecular weight (1.80 × 102–2.00 × 106 Da). MW, Molecular weight.
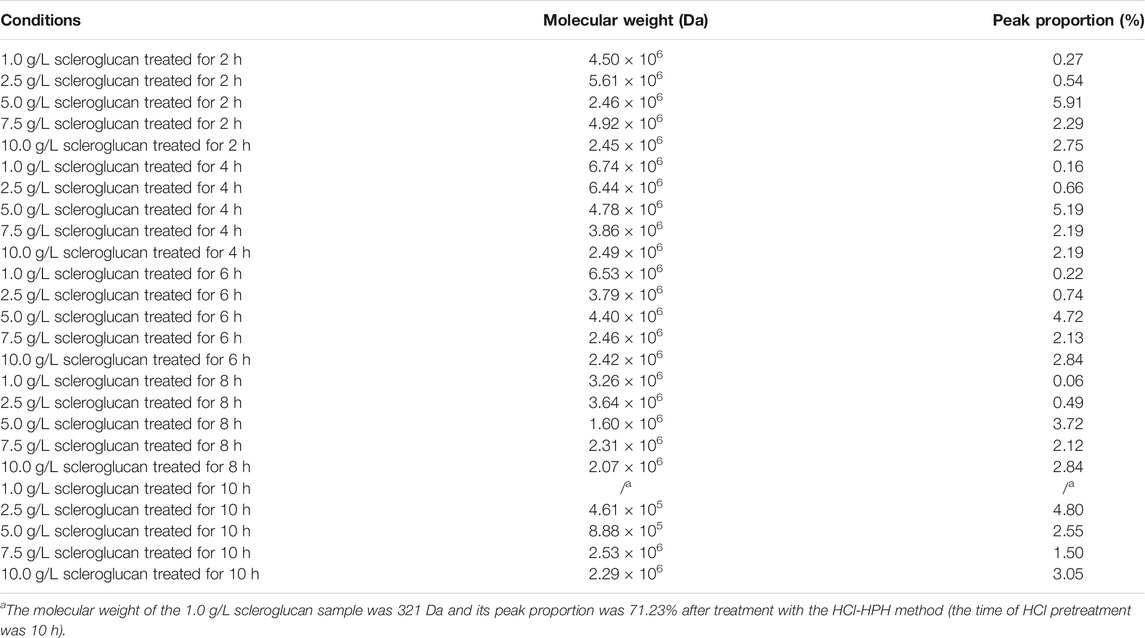
TABLE 1. Molecular weight of scleroglucan (105–106) and its peak proportion in the samples with different scleroglucan concentrations.
Conclusion
In this study, the decrease in scleroglucan production during the fermentation process was resolved by adjusting the state of the seeds inoculated. Additionally, by establishing a two-dose fed-batch mode, the production of scleroglucan was further enhanced, reaching 66.6 g/L, with a 53.3% glucose conversion ratio and productivity of 0.40 g/(L·h). Furthermore, based on optimizing the conditions of HPH treatment and HCl treatment, a combination HCl-HPH method was established for modifying the molecular weight of scleroglucan, which produced scleroglucan of 4.61 × 105 Da. These established methods could provide a reference for the biosynthesis and molecular weight modification of scleroglucan and other polysaccharides.
Data Availability Statement
The original contributions presented in the study are included in the article/Supplementary Material, further inquiries can be directed to the corresponding author.
Author Contributions
WZ, JW, and XS performed the experiments and data analysis. WZ, SY, and JZ wrote the manuscript and conceived the study. WZ and JZ coordinated the project. All authors contributed to the article and approved the submitted version.
Funding
This work was supported by the National Key Research and Development Program of China (2017YFC1600403), the Foundation for Innovative Research Groups of the National Natural Science Foundation of China (32021005), the National Science Fund for Excellent Young Scholars (21822806) and the Fundamental Research Funds for the Central Universities (JUSRP521005).
Conflict of Interest
The authors declare that the research was conducted in the absence of any commercial or financial relationships that could be construed as a potential conflict of interest.
Publisher’s Note
All claims expressed in this article are solely those of the authors and do not necessarily represent those of their affiliated organizations, or those of the publisher, the editors and the reviewers. Any product that may be evaluated in this article, or claim that may be made by its manufacturer, is not guaranteed or endorsed by the publisher.
Supplementary Material
The Supplementary Material for this article can be found online at: https://www.frontiersin.org/articles/10.3389/fbioe.2021.748213/full#supplementary-material
References
Bai, T., Wang, T., Li, Y., Gao, N. L., Zhang, L., Chen, W.-H., et al. (2021). Optimization of Scleroglucan Production by Sclerotium Rolfsii by Lowering pH during Fermentation via Oxalate Metabolic Pathway Manipulation Using CRISPR/Cas9. Fungal Biol. Biotechnol. 8, 1. doi:10.1186/s40694-021-00108-5
Barcelos, M. C. S., Vespermann, K. A. C., Pelissari, F. M., and Molina, G. (2020). Current Status of Biotechnological Production and Applications of Microbial Exopolysaccharides. Crit. Rev. Food Sci. Nutr. 60, 1475–1495. doi:10.1080/10408398.2019.1575791
Bateman, D. F. (1972). The Polygalacturonase Complex Produced by Sclerotium Rolfsii. Physiol. Plant Pathol. 2, 175–184. doi:10.1007/s00253-011-3438-510.1016/0048-4059(72)90025-2
Belmiro, R. H., Tribst, A. A. L., and Cristianini, M. (2018). Application of High-Pressure Homogenization on Gums. J. Sci. Food Agric. 98, 2060–2069. doi:10.1002/jsfa.8695
Castillo, N. A., Valdez, A. L., and Fariña, J. I. (2015). Microbial Production of Scleroglucan and Downstream Processing. Front. Microbiol. 6, 1106. doi:10.3389/fmicb.2015.01106
Elsehemy, I. A., Noor El Deen, A. M., Awad, H. M., Kalaba, M. H., Moghannem, S. A., Tolba, I. H., et al. (2020). Structural, Physical Characteristics and Biological Activities Assessment of Scleroglucan from a Local Strain Athelia Rolfsii TEMG. Int. J. Biol. Macromolecules 163, 1196–1207. doi:10.1016/j.ijbiomac.2020.06.272
Farina, J. I., Sineriz, F., Molina, O. E., and Perotti, N. I. (2001). Isolation and Physicochemical Characterization of Soluble Scleroglucan from Sclerotium Rolfsii. Rheological Properties, Molecular Weight and Conformational Characteristics. Carbohyd Polym. 44, 41–50. doi:10.1016/s0144-8617(00)00189-2
Fariña, J. I., Viñarta, S. C., Cattaneo, M., and Figueroa, L. I. C. (2009). Structural Stability of Sclerotium rolfsii ATCC 201126 β-glucan with Fermentation Time: a Chemical, Infrared Spectroscopic and Enzymatic Approach. J. Appl. Microbiol. 106, 221–232. doi:10.1111/j.1365-2672.2008.03995.x
Gao, M., Xu, Y., Yang, G., Jin, S., Hu, X., Jiang, Y., et al. (2021). One-step Production of Functional Branched Oligoglucosides with Coupled Fermentation of Pichia pastoris GS115 and Sclerotium Rolfsii WSH-G01. Bioresour. Technol. 335, 125286. doi:10.1016/j.biortech.2021.125286
Gao, R., Zhou, J., and Xu, G. (2018). Strategies for Feeding Control in Microbial Production of Scleroglucan by Sclerotium Rolfsii WSH-G01. Food Fermen Ind. 44, 51–56. doi:10.13995/j.cnki.11-1802/ts.017234
Giavasis, I. (2014). Bioactive Fungal Polysaccharides as Potential Functional Ingredients in Food and Nutraceuticals. Curr. Opin. Biotechnol. 26, 162–173. doi:10.1016/j.copbio.2014.01.010
He, Y., Zhang, M., Shan, M., Zeng, P., Li, X., Hao, C., et al. (2018). Optimizing Microwave-Assisted Hydrolysis Conditions for Monosaccharide Composition Analyses of Different Polysaccharides. Int. J. Biol. Macromolecules 118, 327–332. doi:10.1016/j.ijbiomac.2018.06.077
Hu, T.-G., Zou, Y.-X., Li, E.-N., Liao, S.-T., Wu, H., and Wen, P. (2021). Effects of Enzymatic Hydrolysis on the Structural, Rheological, and Functional Properties of Mulberry Leaf Polysaccharide. Food Chem. 355, 129608. doi:10.1016/j.foodchem.2021.129608
Kulicke, W.-M., Lettau, A. I., and Thielking, H. (1997). Correlation between Immunological Activity, Molar Mass, and Molecular Structure of Different (1→3)-β-D-Glucans. Carbohydr. Res. 297, 135–143. doi:10.1016/s0008-6215(96)00273-x
Levy, R., Okun, Z., Davidovich-Pinhas, M., and Shpigelman, A. (2021). Utilization of High-Pressure Homogenization of Potato Protein Isolate for the Production of Dairy-free Yogurt-like Fermented Product. Food Hydrocolloids 113, 106442. doi:10.1016/j.foodhyd.2020.106442
Li, D.-D., Yang, N., Tao, Y., Xu, E.-B., Jin, Z.-Y., Han, Y.-B., et al. (2020a). Induced Electric Field Intensification of Acid Hydrolysis of Polysaccharides: Roles of thermal and Non-thermal Effects. Food Hydrocolloids 101, 105484. doi:10.1016/j.foodhyd.2019.105484
Li, H., Yan, S., Ji, J., Xu, M., Mao, H., Wen, Y., et al. (2020b). Insights into maize Starch Degradation by High Pressure Homogenization Treatment from Molecular Structure Aspect. Int. J. Biol. Macromolecules 161, 72–77. doi:10.1016/j.ijbiomac.2020.06.037
Li, X., Lu, Y., Adams, G. G., Zobel, H., Ballance, S., Wolf, B., et al. (2020c). Characterisation of the Molecular Properties of Scleroglucan as an Alternative Rigid Rod Molecule to Xanthan Gum for Oropharyngeal Dysphagia. Food Hydrocolloids 101, 105446. doi:10.1016/j.foodhyd.2019.105446
Lin, Q., Li, H., Ren, J., Deng, A., Li, W., Liu, C., et al. (2017). Production of Xylooligosaccharides by Microwave-Induced, Organic Acid-Catalyzed Hydrolysis of Different Xylan-type Hemicelluloses: Optimization by Response Surface Methodology. Carbohydr. Polym. 157, 214–225. doi:10.1016/j.carbpol.2016.09.091
Liu, D., Tang, W., Yin, J.-Y., Nie, S.-P., and Xie, M.-Y. (2021a). Monosaccharide Composition Analysis of Polysaccharides from Natural Sources: Hydrolysis Condition and Detection Method Development. Food Hydrocolloids 116, 106641. doi:10.1016/j.foodhyd.2021.106641
Liu, E., Segato, F., and Wilkins, M. R. (2021b). Fed-batch Production of Thermothelomyces Thermophilus Lignin Peroxidase Using a Recombinant Aspergillus nidulans Strain in Stirred-Tank Bioreactor. Bioresour. Technol. 325, 124700. doi:10.1016/j.biortech.2021.124700
Martin, K., Mcdougall, B. M., Mcilroy, S., Jayus, J., and Chen, R. J. (2007). Biochemistry and Molecular Biology of Exocellular Fungal β-(1,3)- and β-(1,6)-glucanases. FEMS Microbiol. Rev. 31, 168–192. doi:10.1111/j.1574-6976.2006.00055.x
Nguyen, H. S. H., Heinonen, J., Laatikainen, M., and Sainio, T. (2020). Evolution of the Molar Mass Distribution of Oat β-glucan during Acid Catalyzed Hydrolysis in Aqueous Solution. Chem. Eng. J. 382, 122863. doi:10.1016/j.cej.2019.122863
Schmid, J., Meyer, V., and Sieber, V. (2011). Scleroglucan: Biosynthesis, Production and Application of a Versatile Hydrocolloid. Appl. Microbiol. Biotechnol. 91, 937–947. doi:10.1007/s00253-011-3438-5
Shi, H., Wan, Y., Li, O., Zhang, X., Xie, M., Nie, S., et al. (2020). Two-step Hydrolysis Method for Monosaccharide Composition Analysis of Natural Polysaccharides Rich in Uronic Acids. Food Hydrocolloids 101, 105524. doi:10.1016/j.foodhyd.2019.105524
Song, J., Jia, Y.-X., Su, Y., Zhang, X.-Y., Tu, L.-N., Nie, Z.-Q., et al. (2020). Initial Analysis on the Characteristics and Synthesis of Exopolysaccharides from Sclerotium Rolfsii with Different Sugars as Carbon Sources. Polymers 12, 348. doi:10.3390/polym12020348
Sun, H., Ren, Y., Lao, Y., Li, X., and Chen, F. (2020a). A Novel Fed-Batch Strategy Enhances Lipid and Astaxanthin Productivity without Compromising Biomass of Chromochloris Zofingiensis. Bioresour. Technol. 308, 123306. doi:10.1016/j.biortech.2020.123306
Sun, T., Wang, R., Sun, D., Li, S., Xu, H., Qiu, Y., et al. (2020b). High-efficiency Production of Tremella Aurantialba Polysaccharide through Basidiospore Fermentation. Bioresour. Technol. 318, 124268. doi:10.1016/j.biortech.2020.124268
Survase, S. A., Saudagar, P. S., and Singhal, R. S. (2007a). Enhanced Production of Scleroglucan by Sclerotium Rolfsii MTCC 2156 by Use of Metabolic Precursors. Bioresour. Technol. 98, 410–415. doi:10.1016/j.biortech.2005.12.013
Survase, S. A., Saudagar, P. S., and Singhal, R. S. (2007b). Use of Complex media for the Production of Scleroglucan by Sclerotium Rolfsii MTCC 2156. Bioresour. Technol. 98, 1509–1512. doi:10.1016/j.biortech.2006.05.022
Tan, R., Lyu, Y., Zeng, W., and Zhou, J. (2019). Enhancing Scleroglucan Production by Sclerotium Rolfsii WSH-G01 through a pH-Shift Strategy Based on Kinetic Analysis. Bioresour. Technol. 293, 122098. doi:10.1016/j.biortech.2019.122098
Taskin, M., Erdal, S., and Canli, O. (2010). Utilization of Waste Loquat (Eriobotrya Japonica Lindley) Kernels as Substrate for Scleroglucan Production by Locally Isolated Sclerotium Rolfsii. Food Sci. Biotechnol. 19, 1069–1075. doi:10.1007/s10068-010-0150-7
Tian, S., Liang, X., Chen, J., Zeng, W., Zhou, J., and Du, G. (2020). Enhancement of 2-phenylethanol Production by a Wild-type Wickerhamomyces Anomalus Strain Isolated from rice Wine. Bioresour. Technol. 318, 124257. doi:10.1016/j.biortech.2020.124257
Valdez, A. L., Babot, J. D., Schmid, J., Delgado, O. D., and Fariña, J. I. (2019). Scleroglucan Production by Sclerotium Rolfsii ATCC 201126 from Amylaceous and Sugarcane Molasses-Based media: Promising Insights for Sustainable and Ecofriendly Scaling-Up. J. Polym. Environ. 27, 2804–2818. doi:10.1007/s10924-019-01546-4
Valdez, A. L., Delgado, O. D., and Fariña, J. I. (2021). Cost-effective Optimized Scleroglucan Production by Sclerotium Rolfsii ATCC 201126 at Bioreactor Scale. A Quantity-Quality Assessment. Carbohydr. Polym. 260, 117505. doi:10.1016/j.carbpol.2020.117505
Vallejo-García, L. C., Rodríguez-Alegría, M. E., and López Munguía, A. (2019). Enzymatic Process Yielding a Diversity of Inulin-type Microbial Fructooligosaccharides. J. Agric. Food Chem. 67, 10392–10400. doi:10.1021/acs.jafc.9b03782
Villay, A., Lakkis de Filippis, F., Picton, L., Le Cerf, D., Vial, C., and Michaud, P. (2012). Comparison of Polysaccharide Degradations by Dynamic High-Pressure Homogenization. Food Hydrocolloids 27, 278–286. doi:10.1016/j.foodhyd.2011.10.003
Wang, T., Jiang, M., Tang, S., Lai, C., Huang, C., Fan, Y., et al. (2021). Preparation of Di- and Tri- Galacturonic Acid by Coupling Hydrothermal Pretreatment and Enzymatic Hydrolysis. Process Biochem. 102, 180–185. doi:10.1016/j.procbio.2021.01.002
Wellala, C. K. D., Bi, J., Liu, X., Liu, J., Lyu, J., Zhou, M., et al. (2020). Effect of High Pressure Homogenization Combined with Juice Ratio on Water-Soluble Pectin Characteristics, Functional Properties and Bioactive Compounds in Mixed Juices. Innovative Food Sci. Emerging Tech. 60, 102279. doi:10.1016/j.ifset.2019.102279
Xie, F., Zhang, H., Nie, C., Zhao, T., Xia, Y., and Ai, L. (2021). Structural Characteristics of Tamarind Seed Polysaccharides Treated by High-Pressure Homogenization and Their Effects on Physicochemical Properties of Corn Starch. Carbohydr. Polym. 262, 117661. doi:10.1016/j.carbpol.2021.117661
Xie, F., Zhang, W., Lan, X., Gong, S., Wu, J., and Wang, Z. (2018). Effects of High Hydrostatic Pressure and High Pressure Homogenization Processing on Characteristics of Potato Peel Waste Pectin. Carbohydr. Polym. 196, 474–482. doi:10.1016/j.carbpol.2018.05.061
Zeng, W., Cai, W., Liu, L., Du, G., Chen, J., and Zhou, J. (2019). Efficient Biosynthesis of 2-Keto-D-Gluconic Acid by Fed-Batch Culture of Metabolically Engineered Gluconobacter Japonicus. Synth. Syst. Biotechnol. 4, 134–141. doi:10.1016/j.synbio.2019.07.001
Zeng, W., Tan, R., and Zhou, J. (2021). Discovery and Functional Verification of Endogenous Glucanases for Scleroglucan Hydrolysis in Sclerotium Rolfsii. Chin. J. Biotechnol. 37, 207–217. doi:10.13345/j.cjb.200236
Zeng, W., Zhang, H., Xu, S., Fang, F., and Zhou, J. (2017). Biosynthesis of Keto Acids by Fed-Batch Culture of Yarrowia Lipolytica WSH-Z06. Bioresour. Technol. 243, 1037–1043. doi:10.1016/j.biortech.2017.07.063
Zhang, Y., Wang, W., Zhang, Y., and Dong, X. (2017). Medium Optimization of Scleroglucan Production by Sclerotium Rolfsii SCL2010. China Brewing 36, 49–53. doi:10.11882/j.issn.0254-5071.2017.11.011
Keywords: Sclerotium rolfsii, scleroglucan, fed-batch, molecular weight, high-pressure homogenization
Citation: Zeng W, Wang J, Shan X, Yu S and Zhou J (2021) Efficient Production of Scleroglucan by Sclerotium rolfsii and Insights Into Molecular Weight Modification by High-Pressure Homogenization. Front. Bioeng. Biotechnol. 9:748213. doi: 10.3389/fbioe.2021.748213
Received: 27 July 2021; Accepted: 23 August 2021;
Published: 03 September 2021.
Edited by:
Chao Gao, Shandong University, ChinaReviewed by:
Fei Tao, Shanghai Jiao Tong University, ChinaHui Wu, East China University of Science and Technology, China
Copyright © 2021 Zeng, Wang, Shan, Yu and Zhou. This is an open-access article distributed under the terms of the Creative Commons Attribution License (CC BY). The use, distribution or reproduction in other forums is permitted, provided the original author(s) and the copyright owner(s) are credited and that the original publication in this journal is cited, in accordance with accepted academic practice. No use, distribution or reproduction is permitted which does not comply with these terms.
*Correspondence: Jingwen Zhou, zhoujw1982@jiangnan.edu.cn
†These authors have contributed equally to this work