- 1SMART (Skeleton Movement Analysis and Advanced Rehabilitation Technologies) LAB, Bioengineering and Biomedicine Company Srl, Chieti (CH), Italy
- 2Department of Neuroscience, Imaging and Clinical Sciences University G. D’Annunzio, Chieti, Italy
- 3Chair of Rehabilitation and Physiotherapy, Department of Rehabilitation, University of Medical Sciences, Poznań, Poland
Background: The literature reports evidence of leg length discrepancy (LLD) associated with musculoskeletal disorders, alterations in spinopelvic alignment, and body posture, leading to low back pain and lumbar scoliosis. The most common conservative treatment for LLD is the use of internal or external shoe lifts although no treatment guidelines have been established.
Aim: The study aimed to contribute to low back pain–LLD relationship comprehension, highlighting the benefits of LLD correction in the nonspecific low back pain (NSLBP) population.
Methods: A cross-sectional observational study recruited a cohort of 80 NSLBP patients (48 females, 32 males) with LLD, age (μ = 35 ± 17.2). Entire body posture, including 3-D spine shape reconstruction, was measured using a nonionizing 3-D optoelectronic stereophotogrammetric approach. After the first 3-D posture evaluation, patients were provided with customized orthotics, including 100% LLD heel lift correction. No other therapeutic interventions were considered. Pain level was assessed using the numerical pain rating scale (NPRS). The gender, age-related, and time-dependent effects of LLD equalization treatment in NSLBP patients was investigated during 2 years of follow-up. The statistical analysis was performed at the global level using multivariate methods by Hotelling T2 tests and intrasubject-level using t-test.
Results and Discussion: An initial average NPRS = 7.8 was determined. In the medium-term follow-up group (4 months), the NPRS dramatically decreased (NPRS = 1.1). The pain disappeared in the long-term (2 years) follow-up group (NPRS = 0). The study results highlight that LLD equalization treatment led to clear statistically significant improvements in all the postural parameters of the frontal plane, including the underfoot load asymmetry. No worsening has been detected. An adaptation period long enough is needed to obtain progressive pain relief improvements and structural posture changes. Younger NSLBP patients showed slightly better improvements than older ones. Minimal differences between healthy young adults’ and NSLBP patients’ postures were found either in natural erect standing posture or when LLD equalization is applied.
Conclusion: Heel-lift customized orthotics with 100% LLD correction are an effective short- and long-term treatment in patients with nonspecific LBP, inducing pain symptom recession and stimulating the improvement of postural parameters without contraindications.
1 Introduction
Anisomelia, i.e., limb length discrepancy, is defined as a condition in which paired limbs are noticeably unequal. When the discrepancy is in the lower extremities, it is known as leg length discrepancy (LLD). Recent literature reports increasing evidence of the association of LLD with a variety of musculoskeletal disorders, such as hip or knee osteoarthritis and chronic low back pain (LBP) that impose a high personal and social burden (Defrin et al., 2005; Tallroth et al., 2005; Harvey et al., 2010; Kendall et al., 2014; Murray and Azari, 2015; Tallroth et al., 2017; Gordon and Davis, 2019). Perhaps the most controversial musculoskeletal disorder associated with LLD is LBP. Some authors find a definite association between LLD and LBP (Friberg, 1983; Defrin et al., 2005; Golightly et al., 2007; Kendall et al., 2014; Cambron et al., 2017; Rannisto et al., 2019; Menez et al., 2021; Menez et al., 2020), whereas others find none (Campbell et al., 2018). LLD can be either anatomical, i.e., caused by deformities originating from actual differences in the bony structures of the lower limb, or functional, i.e., possibly derived by mechanical changes affecting the lower limbs (Blake and Ferguson, 1992; Brady et al., 2003; Knutson, 2005a; Knutson, 2005b). However, there is still a lack of agreement on diagnosis (Brady et al., 2003), classification (Gurney, 2002), and treatment (Campbell et al., 2018) among both researchers and clinicians. The existence of limb length inequality is not in doubt. However, its prevalence in the population constitutes an open debate because there is no agreement on what constitutes a clinically significant LLD (Brady et al., 2003). In most cases, the coexistence of anatomical and functional contributions to LLD adds difficulties to the LLD evaluation. An extensive review by Sabharwal and Kumar (2008) shows no agreement on how the LLD has to be measured with either clinical or instrumental methods. Clinical methods, such as the use of a tape measure and standing blocks, (being the latter to be preferred in that they were found to be more reliable and complete giving the possibility to consider also the LLD functional component) are noted as useful screening tools, but not as accurate as imaging modalities. However, even on imaging tools to be used, there is a debate, and they present pros and cons, especially thinking about the risk connected to the use of X-rays for some of them.
Since the early ’80s, the literature describes it as a relatively common phenomenon found in as many as 40% (Subotnick, 1981) to 70% (Woerman and Binder-Macleod, 1984) up to 90% of the adult population (Blake and Ferguson, 1992), whereas the incidence of LLD greater than 20 mm was found to affect at least one in every 1000 people (Guichet et al., 1991). Later on, LLD has been recognized to affect up to 90% of the population with an average value of 5.2 ± 4.1 mm as measured by highly precise radiographic millimetric methods (Knutson, 2005a). More recently, D’Amico et al. (2017b) reported the normative 3-D posture and spine shape data in a healthy young adult population. Using an advanced optoelectronic stereophotogrammetric approach to measure the entire 3-D skeleton posture, including 3-D spine morphology during natural, unconstrained standing erect posture, they found, in a cohort of 124 asymptomatic volunteers, that each subject presented some degree of LLD. Despite male and female groups presenting statistically different heights, the amount of LLD was gender-independent with a mean value µ = 9.37 ± 3.31 mm and in a range of 6–23 mm. LLD was associated with the following factors: unbalanced underfoot loads and/or posture, some amount of spinal deformity and pelvis obliquity in the frontal plane, and pelvis torsion. Some authors classify discrepancies ≤2.0 cm as mild (Ruzbarsky et al., 2017), whereas others consider discrepancies of up to 3.0 cm as mild (Gurney, 2002; Brady et al., 2003; Campbell et al., 2018). A subdivision into mild (up to 30 mm), moderate (30–60 mm), or severe (>60 mm) is also reported (Reid and Smith, 1984; Brady et al., 2003; Knutson, 2005a). These classifications are intended to guide practitioners in treating LLD (Vogt et al., 2020), but there is much disagreement in the literature about the magnitude from which LLD requires treatment. Some authors may consider that LLDs less than 2 cm are usually well-tolerated and often go unnoticed (Ruzbarsky et al., 2017). Conversely, it is suggested that orthotic insoles, shoe lifts, or other clinical interventions to equalize leg length should be considered for LLD ≥1.0 cm (White et al., 2004) or even between 0.5 and 1.0 cm (Friberg, 1982; Friberg, 1983; Khamis and Carmeli, 2018; Gordon and Davis, 2019; Menez et al., 2021; Menez et al., 2020).
LLD is associated with alterations in spinopelvic alignment (Knutson, 2005a; Cooperstein, 2010), body posture (Raczkowski et al., 2010; Murray and Azari, 2015; D’Amico et al., 2017b), and balance (D’Amico et al., 2017b; Eliks et al., 2017), thus determining an asymmetrical distribution and magnitude of mechanical stresses and strains within the body (McCaw and Bates, 1991). Particular attention is devoted to LLD effects on pelvic posture and motion. Several authors notice pelvic tilt in the frontal plane due to uneven leveling of lower limbs associated with a forward innominate bone rotation on the short limb and backward rotation on the long limb (McCaw and Bates, 1991; Cooperstein, 2010). Such a complex destabilizing in the pelvis (i.e., pelvic obliquity associated with pelvis torsion) is found to relate to the lower limb loading pattern for healthy, young, asymptomatic individuals. Indeed, D’Amico et al. (2017b) shows a higher probability that longer-leg loaders demonstrate more posterior rotation on the ipsilateral side than shorter-leg loaders who would more likely show anterior rotation on this side. Numerous observational studies reveal correlations between LLD, asymmetrical distribution, and magnitude of mechanical stresses and strains in spine joints, degenerative changes in the lumbar spine, alterations in spinal biomechanics, and LBP. However, they fail to show causation, resulting in limited evidence to guide treatment (Sheha et al., 2018). A certain magnitude of LLD likely plays a role in LBP although it is unclear at this time what degree of LLD is required to cause symptoms. Indeed, given changes in multiple parameters that tend to occur with LLD (e.g., sacral or pelvic tilt and lumbar scoliosis, compensations in the lower limbs), it is likely that confounders are at play. Therefore, the exact relationship linking the LLD, the lumbar spine, and the true drivers of LBP in these patients has yet to be fully elucidated (Sheha et al., 2018).
Treatment of LLD is an open debate in the literature. It spans from no intervention to conservative approaches to various surgical techniques (Gurney, 2002; Brady et al., 2003). Conclusions in a recent review (Vogt et al., 2020) recommend the following: “It must be discussed with each patient individually whether the treatment should be conservative or surgical. The extent of the discrepancy is not the sole determining factor for the mode of treatment. The decision to treat is always elective.”
The most common conservative treatment for mild LLD is the use of internal or external shoe lifts (Brady et al., 2003). Even on such a simple intervention, there are a plethora of opinions regarding shoe lift efficacy, the best thickness to be used, and even the strategies for their application (Brady et al., 2003; Golightly et al., 2007; D’Amico et al., 2012; Chuter et al., 2014). Many are the studies reporting benefits of shoe insert use inducing symmetrical limb loading, pain, and functional disability reduction and lumbar scoliotic curve reduction (Friberg, 1983; Defrin et al., 2005; Golightly et al., 2007; D’Amico et al., 2012; Chuter et al., 2014). Conversely, others find limited data to support their use (Brady et al., 2003; Chuter et al., 2014).
In this context of uncertainty, the present research aims to study the LBP–LLD relationship. Our primary hypothesis is that the uncertainty debated in the literature is mainly due to the lack of detailed, rigorous, biomechanical-functional information on 3-D natural erect standing posture characteristics and how the presence of an LLD influences them.
To tackle the several confounders at play, we use the nonionizing optoelectronic stereophotogrammetric approach associated with baropodometry presented in D’Amico et al. (2017a, 2017b). Such a measurement technique shows several advantages, allowing, in a very short time, the evaluation of the 3-D entire skeleton posture, including the 3-D spine shape, analyzed with many clinically useful 3-D and 2-D anatomical, biomechanical, and clinical parameters. In this way, it is easy and fast to quantify the eventual presence of an LLD and the whole skeleton functional postural adjustments when a heel-lift LLD correction is applied. At the same time, the optimal LLD correction can be immediately identified by evaluating the effects of different thickness heel lifts on the patient’s posture by statistically comparing the LLD equalized erect posture with the actual neutral unconstrained upright standing. We collected data on a cohort of nonspecific low back pain (NSLBP) patients presenting an LLD to investigate the immediate, medium, and long-term answers of LLD equalization considering the effects on different age groups. Finally, to identify whether there are specific 3-D postural characteristics for the NSLBP population and specific behavior of such population in response to LLD equalization, we compared the results obtained in the present study with those obtained for a population of healthy young adults determined in the study of D’Amico et al. (2017b).
2 Materials and Methods
2.1 Study Design
The present study is a prospective, cross-sectional observational research (according to the STROBE guidelines (von Elm et al., 2007) and the Helsinki Declaration), evaluating the LLD equalization effect through custom-made foot orthotics in nonspecific chronic and subacute LBP patients using 3-D stereophotogrammetric quantitative posture analysis. The ethics committee of the University of Medical Sciences in Poznan, Poland, approved this study (resolution number: 376/17). Written informed consent was obtained from the individuals for the publication of any potentially identifiable images or data included in this article. Data collection took place between May 2017 and December 2019.
2.2 Participants
Before the measurement session, participants were given a thorough clinical postural examination by an experienced physiotherapist, during which pain intensity was rated via the numerical pain rating scale (NPRS) (Jensen and Karoly, 2001).
The inclusion/exclusion criteria were as follows: diagnosis of NSLBP both subacute (≥6 weeks) and chronic (≥12 weeks) nonspecific lumbar pain history, males and females older than 18 years (Caucasian), no neurologic problems, no history of musculoskeletal system injury or surgery, LLD presence measurable through the used stereophotogrammetric approach.
A cohort of 80 NSLBP patients (48 females, 32 males), 18–72 years (μ = 35 ± 17.2) were recruited in a cross-sectional observational study at the Clinic of Rehabilitation, University of Medical Sciences, Poznan-Poland. Table 1 summarizes the characteristics of the NSLBP patients compared by gender to healthy young adults.
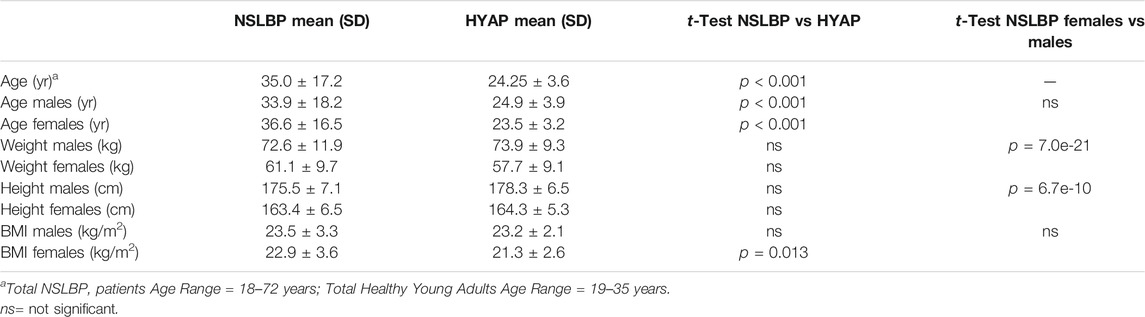
TABLE 1. NSLBP Patients Characteristics Compared to Healthy Young Adults Characteristics. The last column reports the comparison between males and female characteristics in the NSLBP patients’ cohort (total = 80 NSLBP patients).
2.3 Data Measurement
Patients’ entire body posture, including 3-D spine shape reconstruction, has been measured using a nonionizing 3-D optoelectronic stereophotogrammetric approach. Eleven quantitative biomechanical parameters describing the nature of body posture have been computed. Our experimental recordings were based on a six-TV-camera Global Opto-electronic Approach for Locomotion and Spine1 (GOALS) stereophotogrammetric optoelectronic system derived from Optitrack System2 [resolution 1.3 Mpix, 120 fps, error range 0.3 mm, calibrated volume 3 × 3 × 2 meters (D’Amico et al., 2017b; D’Amico et al., 2018a; Kinel et al., 2018)]. One synchronous baropodometric platform (Zebris FDM-SX)3 (active area dimensions: 400 mm × 330 mm, total 1920 square capacitive sensors; 1.4 sensors/cm2) was used to measure bilateral foot pressure maps and underfoot vertical forces exerted on each foot in the standing position. The platform is rectangular, and the sensors are arranged in rows and columns parallel to the shorter and longer edge, respectively. The manufacturer grants the calibrated pressure measuring range (1–120 N/cm2) with ±5% of the maximum range accuracy. An essential step of the calibration procedure is to establish the relative position of the baropodometric platform within the calibrated volume. This position is essential for all the following calculations related to underfoot pressure maps and associated vertical forces. Further details about the calibration procedure can be found in D’Amico et al. (2021a).
The 27 body landmarks protocol, labeled by passive retroreflective markers (D’Amico et al., 2018b), was used to measure the subjects’ 3-D whole skeleton posture (Figure 1). The model’s accuracy and precision are founded on in-house original signal processing and optimization procedures (D’Amico et al., 1995a; D’Amico et al., 2007; D’Amico et al., 2021a) and anatomical studies listed in the literature (cadaver dissections, in vivo X-ray, and parametric regression equations from gamma-ray measurements) (Liu and Wickstrom, 1973; Zatsiorsky et al., 1990; Seidel et al., 1995; de Leva, 1996). The model was formulated in a parametric form to scale any subject’s characteristics by fitting each given skeletal segment to the 3-D measured positions of its corresponding body landmarks (D’Amico et al., 1995a). The model was tested extensively in the clinical environment to analyze human posture (D’Amico et al., 2017a; D’Amico et al., 2017b; D’Amico et al., 2018a; Kinel et al., 2018; D’Amico et al., 2021a; Kinel et al., 2021).
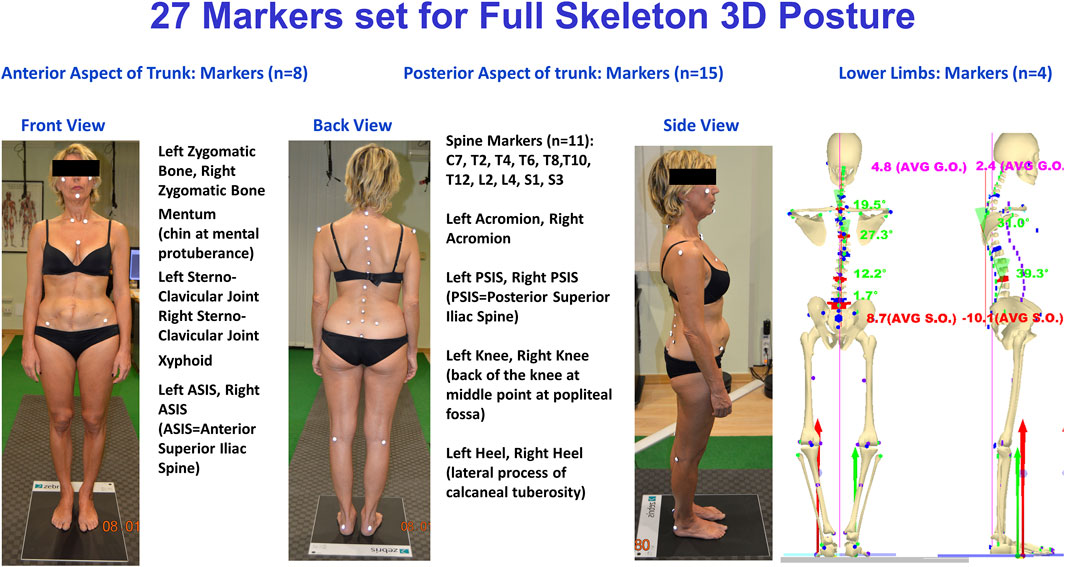
FIGURE 1. The experimental setup used for 3-D posture analysis: GOALS system and baropodometric platform configuration.
The software package called ASAP 3D Skeleton Model,1 implementing a full 3-D parametric biomechanical human skeleton model (3-D spine included), was used to process data.
2.4 Acquisition Protocol
The standard trial session aimed to define the participant’s erect posture. In order to reduce potential postural effects resulting from circadian rhythms, all measurements were taken between 12 noon and 7 pm. The subjects were asked to avoid training/therapeutic exercise and/or demanding physical activity before the postural assessment. The assessment/measurement session aimed to fully capture and record the subject’s neutral standing posture with the upper arms relaxed along the side of the body and eyes looking directly ahead in the horizontal plane. Such posture has been defined as indifferent orthostasis (IO). For all subjects, marker positioning was performed by a single operator with more than 20 years’ experience. Each marker-positioning session was of approximately 10 min duration, after which the subject was asked to sit for a few minutes. Afterward, the subject was asked to keep an IO standing with both feet on the baropodometric platform (D’Amico et al., 2017b; Kinel et al., 2018).
Different positions of the feet can influence standing postures. Thus, the subject was asked to align heels on a line parallel to the frontal plane (i.e., on a line parallel to the X-axis of the laboratory reference system) and keep feet apart at about pelvis width (i.e., with feet under the hip joints’ projection) without restricting feet direction to avoid feet position influence. Real-time baropodometric measurement availability allows controlling feet alignment straightforwardly by checking that the heels’ most prominent tip lay on the same row in the foot pressure maps. The subject was positioned in front of a blank wall to avoid visual feedback or reference during measurements.
Given the inclusion criteria, every subject presented with some degree of LLD. In other words, a difference in right vs. left hip center heights as assessed by anterior and posterior iliac spine (ASIS and PSIS) landmark positions (D’Amico, 2002; D’Amico et al., 2012; D’Amico et al., 2017b). Therefore, an additional measurement was then conducted following the same protocol as for IO but by placing a suitable wedge under the subject’s shorter leg as a corrective to equalize any found LLD. Such a measurement was called wedge-corrected orthostasis (WCO). In order to take into account both “anatomical” and “functional” LLD (Knutson, 2005a), it was decided that the optimal size for the corrective wedge was the degree of thickness that provided the best outcome for the individual subject concerning frontal-plane postural parameters (see below). If an overall improvement of the frontal-plane postural parameters was not obtained, the optimal corrective-wedge thickness was chosen as the one providing the best equalization regarding the PSIS landmarks (D’Amico et al., 2017b). In general, no more than three subsequent WCO measurements were necessary to establish the wedge optimal thickness. Such optimal thickness is considered the LLD value in the subsequent statistical computations.
Between each series of measurements (IO and the subsequent WCO trials), the patient was free to sit and relax for a few minutes. The entire acquisition session for each subject was generally completed within 30 min from the time of the subject’s arrival at the posture analysis laboratory, through the complete assessment process, to the final biomechanical report containing a complete set of IO plus WCO measures.
At least five subsequent 2-s lasting acquisitions at a sampling rate of 120 Hz were recorded for each IO and WCO attitude. A total of 1200 3-D measurements for each static posture was averaged. Before averaging, an amount of preprocessing is needed on the acquired 3-D raw data to define the subject’s local coordinate system and its orientation relative to the global coordinate system (D’Amico et al., 2017a; D’Amico et al., 2017b; D’Amico et al., 2018a; Kinel et al., 2018). We used the general definitions provided by the Scoliosis Research Society (Stokes, 1994). However, in distinction to such recommendations, PSIS rather than ASIS landmarks are considered in defining the subject’s local coordinate system to reduce propagation errors and/or other interference deriving from pelvis torsion in the subsequent calculation of spinal parameters. Once having determined this individual system, a rotation is performed within each frame to align the subject’s coordinates with the global reference coordinates. When the alignment is complete, it is possible to average all acquired frames properly. The complete analytical and mathematical descriptions of the entire procedure to reconstruct the whole 3-D skeleton are beyond this paper’s aim, wherein only the main features of 3-D spine reconstruction are described. Full details can be found in D’Amico et al. (1995a,2017a, 2017b, 2018a, 2021a) and Kinel et al. (2018, 2021).
Based on the 11 3-D spinous process measurements, data are interpolated using cubic splines (de Boor, 2001) to assess the position of each unlabeled spinous process and intervertebral disc. After interpolation, the space-curve modeling of the spine is analytically represented using three parametric functions x(t), y(t), z(t) (the parameter being t > 0). A smoothing and differentiation procedure specially developed for interpolated data with cubic splines is applied to these functions (D’Amico et al., 1995a; D’Amico et al., 2017b; D’Amico et al., 2021a). Once the three parametric functions x(t), y(t), z(t) and their derivatives are assessed, the 3-D position of each vertebra from C7 down to S3 is derived. The maxima and minima of the assessed first derivative allow selecting, under analytical constraint, all the inflection points defining the limit vertebrae. After determining the limit vertebrae, the Cobb and kypho-lordotic angle computation is straightforward, computing the angle between the tangents in such points (D’Amico et al., 1995a; D’Amico et al., 2017a; D’Amico et al., 2017b; Kinel et al., 2018). Worth noting, as it happens for the curve identified in the frontal plane, also the kyphosis and lordosis in the sagittal plane are appropriately identified according to the actual spine curvature spatial changes at the limit-vertebrae; i.e., they are no longer restricted to specific thoracic or lumbar anatomical regions (D’Amico et al., 2017b). The accuracy and precision of such signal processing procedures are demonstrated through numerical simulation (D’Amico et al., 2021a), showing excellent performance. In particular, using 3-D analytical helixes, it is demonstrated that, when white noise stereophotogrammetric error (σ = 0.3 mm) was superimposed, the mean angle error computation was only a fraction of degree (0.65°) on a deformity angle up to 66°. Interestingly, the algorithm showed a substantial insensibility to the marker misplacement error (added to the noisy helix σ = 5 mm) along the longitudinal direction. In this case, the resulting mean estimation error presented the same magnitude (0.68°) as the previous test. Finally, when the added misplacement error was considered along random 3-D positions, the computed mean error resulted in 2.52° (D’Amico et al., 2021a).
Figures 2, 3 Example of data elaboration outcome and the related graphical report of the IO1 vs. WCO2 measurement comparison in the frontal (Figure 2) and sagittal (Figure 3) planes.
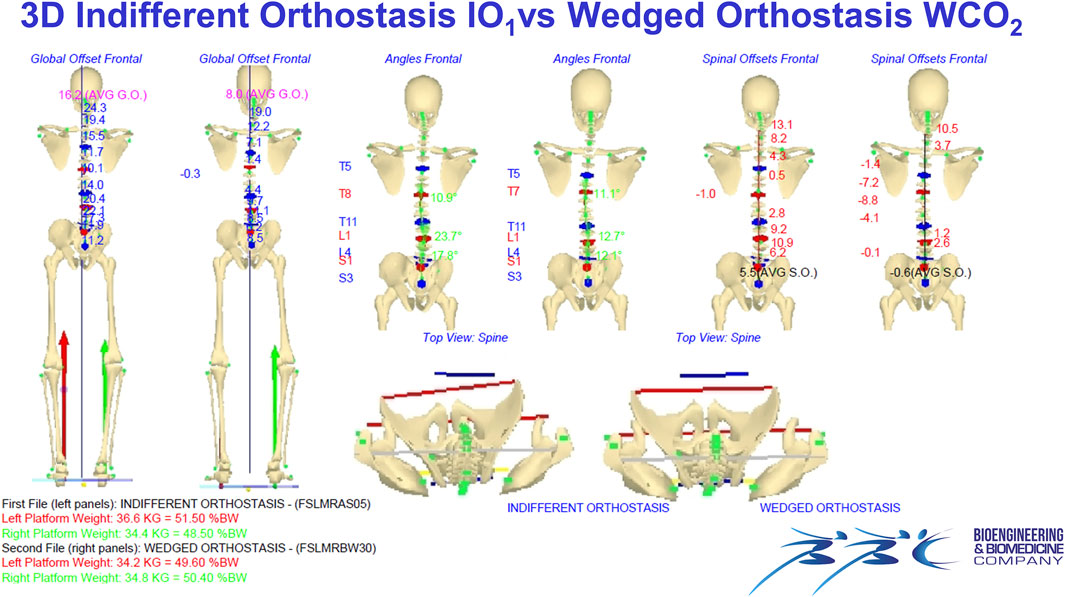
FIGURE 2. Example of data elaboration outcome and the related graphical report of the IO1 vs. WCO2 measurement comparison in the frontal (Panel 2) and sagittal (Figure 3) planes.
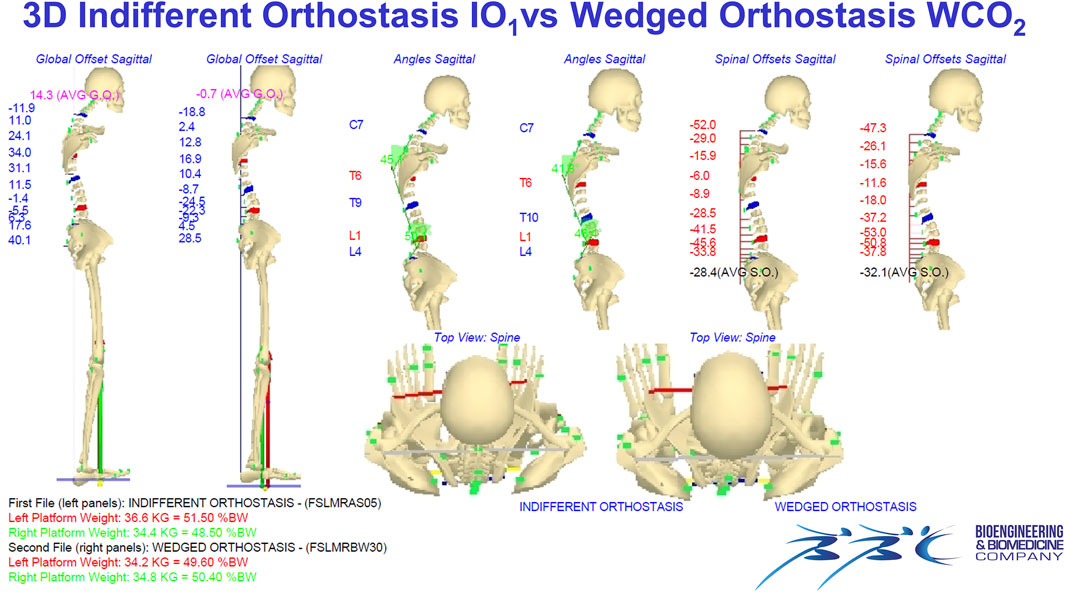
FIGURE 3. Example of data elaboration outcome and the related graphical report of the IO1 vs. WCO2 measurement comparison in the frontal (Figure 2) and sagittal (Panel 3) planes. IO1 (IO at first evaluation); WCO2 (WCO at control session).
A video showing the acquisition/elaboration processes can be found in the supplementary material (Supplementary Material Video S1).
A set of 11 significant parameters detailing the 3-D body posture structure is computed from the 3-D biomechanical human skeleton model reconstruction (D’Amico et al., 2017b; D’Amico et al., 2018a). Such variables were subdivided into three groups as reported in Table 2.
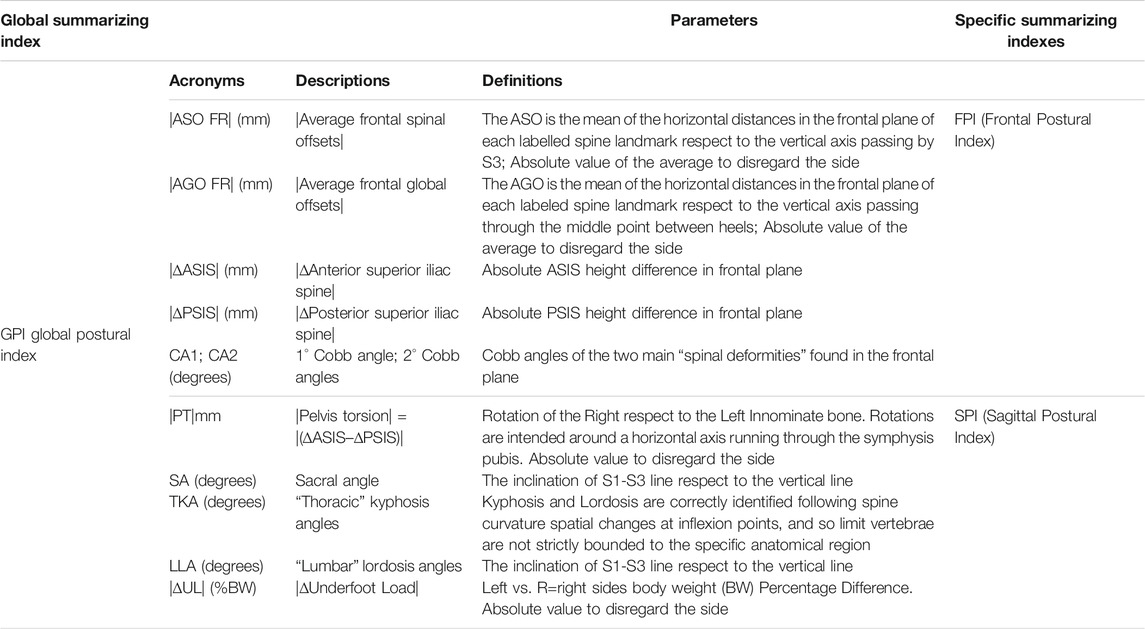
TABLE 2. List of considered parameters (definitions and corresponding acronyms) for IO vs. WCO comparison and Summarizing Indexes.
We decided to consider the Cobb angle value of the two major curves (CA1, CA2, Table 2) for statistical analysis regarding the spinal deformities in the frontal plane.
2.5 Baropodometric Measurements and LLD Equalization by Customized Foot Orthotics
After the first evaluation session, each patient was provided with custom-made foot orthotics to be donned in their usual shoes. As described, during the stereophotogrammetric measurement of static erect postures, simultaneous baropodometric measurements were collected. After the wedge optimal thickness was determined, further baropodometric dynamic measurements were collected during gait trials to complete the information about dynamic foot-floor interaction for each patient’s foot. Indeed, in addition to LLD, each subject presented an individual foot-floor interaction given his/her foot shape and functional behavior (flat foot, arched foot, etc.). Worth underlining, individual behavior was analyzed per foot given the loading asymmetry characteristics linked to LLD (Menez et al., 2021; Menez et al., 2020). A 3-D foot scan complemented such information to proceed to manufacturing customized foot orthotics using a CAD-CAM milling technique as described in detail in D’Amico et al. (2021b). The materials used in the customized orthotics were ethinyl vinyl acetate hand-finished by applying a 1.5 mm PPT top cover. Thus, the customized foot orthotics were made according to the therapeutic needs of the subjects and incorporated a heel lift on the short leg side. The heel lifts were corrective of the 100% LLD (D’Amico et al., 2012) and were shaped from the calcaneus to the Lisfranc joint. No other therapeutic interventions were considered.
2.6 Group Statistical Analysis
The group statistical analysis was performed using a multivariate method provided the checked correlation (through the computation of correlation matrices) among the considered 11 quantitative postural parameters.
According to the specific test, all the following comparisons have been assessed using either the independent samples or the paired samples versions of Hotelling’s T2 test. In the results section, the kind of test used is reported in the table caption.
The simultaneous 95% confidence intervals (derived from Hotelling’s T2 tests) were undertaken to determine the statistical significance of the difference of means for each of the 11 quantitative parameters (Rencher, 2003). Such a method is preferable compared with setting a battery of separate t-tests for each variable with Bonferroni correction on the type I error (α’ = α/k) because the latter approach does not take into account the correlation between the variables, and therefore, it results in an overcorrection of the significance value α (Rencher, 2003).
2.6.1 NSLBP Patients’ Postural Characteristics and Reactions to LLD Equalization, Evaluated by Gender
The following group comparisons have been performed: 1) two female vs. male comparisons, one in IO1 at first evaluation and the other in WCO2 at control sessions; 2) the IO1 vs WCO1 at first evaluation and IO2 vs. WCO2 at control sessions evaluated by gender; such comparisons provide information about the immediate-term postural reaction to LLD equalization and how the adaptation period influences such immediate-term answer; 3) the IO1 at first evaluation vs. IO2 at the control sessions evaluated by gender; 4) the IO1 at first evaluation vs. WCO2 at the control sessions evaluated by gender. The last two comparisons allow assessing structural postural changes induced by custom foot orthotics.
Moreover, to study time-dependent (medium and long-term) effects of LLD equalization induced by customized foot orthotics, the sample has been subdivided into two groups of equal size (24 females and 16 males). The IO2 and WCO2 were evaluated after 4 months in the medium-term group. The follow-up lasted up to 2 years for the long-term group when the final IO2 and WCO2 evaluation was performed. The NPRS (Jensen and Karoly, 2001) was readministered at the medium and long-term evaluations.
Finally, to highlight the age-dependent effects of LLD equalization induced by customized foot orthotics, the sample was subdivided into two groups: younger adults 18–40 years (51 total, 23 males 28 females) and older adults >40 years (29 total, 9 males 20 females).
Only the IO1 vs. WCO2 comparison has been considered for the time and age-dependent effects. The males and females have been pooled together to increase the test power and effect size.
2.6.2 Differences Between NSLBP Patients and Healthy Young Adults in Postural Characteristics and Reactions to LLD Equalization, Evaluated by Gender
To study any differences between NSLBP patients compared with healthy young adults (measured in a previous study (D’Amico et al., 2017b)) in both basic postural characteristics and reactions when an LLD equalization is applied, the following series of comparisons by gender were performed: IO1H vs. WCO1H, IO1NSLBP vs. IO1H, WCO1NSLBP vs. WCO1H, and finally WCO2NSLBP vs. WCO1H. The subscript NSLBP and H indicate NSLBP and healthy young adults groups, whereas the subscripts 1 and 2 indicate, as above, the first or the control session.
Worth noting, control session was not considered for the healthy subjects in the study of (D’Amico et al., 2017b), in which only the immediate-term of LLD equalization by the application of a simple underfoot wedge of optimal thickness (see above) has been scrutinized. For consistency with the statistical approach followed in this study, the immediate-term answer of LLD equalization of the healthy young adults, i.e., the IO1H vs. WCO1H comparison, is performed applying the multivariate approach (T2 Hotelling test for paired samples version) on the data published in (D’Amico et al., 2017b). Because the sacral angle (SA) parameter was not considered in the study of healthy young adults, all the comparisons involving the healthy subject group are performed considering the remaining 10 parameters.
2.6.3 Correlation Between Heel Lift Thickness and Changes in the Main Cobb Angle (CA1)
The correlation between the changes of the main CA1 passing from IO1 to WCO2 and the heel lift correction determined at first evaluation has been assessed. As the data was not normally distributed, the Kendall’s Tau correlation was used.
2.7 Intrasubject Statistical Analysis
At the intrasubject level, we investigated how LLD equalization changed the subject’s posture as assessed in two different temporal circumstances: 1) immediate effect, i.e., when the measurement is made by comparing the within-session IO vs. WCO both at the first evaluation and at the control (i.e., IO1 vs. WCO1 and IO2 vs. WCO2); 2) medium and long-term effect, i.e., when the measurement is made by comparing the IO1 of the first evaluation with the IO2 and the WCO2 in the control session (i.e., IO1 vs. IO2 and IO1 vs. WCO2).
The comparisons were performed through t-tests between the mean values of the 11 considered quantitative parameters obtained per participant in the postural conditions listed above (IO1, IO2, WCO1, WCO2). Such a series of comparisons has been analyzed in terms of “improvement,” “worsening,” or “unchanged” concerning the original IO1 attitude measured at first evaluation.
Thus, each of the 11 postural parameters was classified as “unchanged” if no statistically significant difference between the two currently compared postural attitudes is found.
Conversely, we defined the following as “improvement”:
• Frontal plane parameters: When passing from the first to the second postural attitude (e.g., IO1 vs. WCO1, IO2 vs. WCO2, etc.), the parameter values approached the optimal theoretical zero value (D’Amico et al., 2018a).
• Sagittal plane parameters: In this case (except for pelvis torsion (|PT|) that should be zero), there are no theoretical optimal reference values, so we decided to consider the normative data determined in previous studies in healthy young adults as reference values to be approached (D’Amico et al., 2017b; D’Amico et al., 2018a; Kinel et al., 2018).
• |∆UL| (i.e., the difference of underfoot load between the feet): The optimal theoretical condition is achieved when there is a perfect balance of underfoot load distribution between the left and right sides; therefore, there was “improvement” when changes approached this condition passing from the first to the second postural attitude.
“Worsening”: Each time a statistically significant change differed from the definitions of “improvement,” it was concluded that a “worsening” had occurred.
A summarizing index was defined for each patient, assigning a +1, −1, or 0 scores when improvement, worsening, or unchanged was, respectively, determined (D’Amico et al., 2017b; D’Amico et al., 2018a; Kinel et al., 2021). Henceforth, a “global postural index” (GPIi) given by the sum of scores obtained for all the variables for the ith participant was defined. The frontal plane index (FPIi) and the sagittal plane index (SPIi) were defined by the sum of scores for the variables of the related group (Table 2).
Each of the summarizing indexes was regarded as “improvement” if the summed parameters got a positive score ≥50% of the maximum obtainable positive score; conversely, “worsening” if such sum got a negative score ≥50% of the maximum obtainable negative score; and “unchanged” in the other cases (D’Amico et al., 2018a; Kinel et al., 2021).
By counting the number of “improvement,” “worsening,” and “unchanged” obtained for each participant in each parameter, it is possible to determine the percentages of “improvement,” “worsening,” and “unchanged” achieved in each of the above-listed comparisons.
Finally, as in the group analysis, differences between NSLBP patients and healthy young adults [raw data from (D'Amico et al., 2017b)] were also investigated at the intrasubject level. Because there was no control session in the healthy young adults’ study, for such a group, only the IO1H vs. WCO1H could be included in the intrasubject analysis.
2.8 Power Analysis and Sample Size
The most critical condition among the various multivariate comparison tests is when NSLBP males vs. NSLBP females are compared in IO and WCO. Using GPower software (Faul et al., 2007), it can be established that being that the NSLBP patients’ sample composed of 32 males and 48 females, fixing the required power = 80%, α = 5%, and k = 11 (number of variates), the effect size is d = 1.00 (Mahalanobis distance).
Conversely, for Hotelling’s T2 paired version, the IO1 vs. WCO2 test performed on 29 older NSLBP patients is the most critical condition. For such a case, d = 0.96 is the effect size.
3 Results
First of all, it is essential to underline the time-dependent action of LLD equalization on pain symptoms. Indeed, the NPRS average score was relatively high (NPRS = 7.8) at the first evaluation. In the medium-term follow-up group (4 months), the NPRS score dramatically decreased (NPRS = 1.1). The pain completely disappeared in the long-term (2 years) follow-up group (NPRS = 0).
The mean LLD optimal thickness found in the cohort of NSLBP patients slightly varied between the first evaluation and at control after the adaptation period. An LLD = 10.4 ± 6.2 mm for males and females LLD = 10.7 ± 7.2 mm was determined at the initial assessment. Conversely, at control, the LLD increased to 11.8 ± 6.6mm and 11.1 ± 8.4 mm for males and females, respectively. No statistical difference resulted between males and females.
3.1 Group Statistical Analysis
3.1.1 NSLBP Patients’ Postural Characteristics and Reactions to LLD Equalization, Evaluated by Gender
In group statistical analysis, we investigated gender differences in the IO1 representing the initial indifferent orthostasis and the WCO2 representing the erect corrected standing posture outcome after the adaptation period to the LLD equalization provided by the customized foot orthotics (Table 3). The T2 Hotelling test shows that there are differences between males and females in IO1, in particular the CA2 (second main CA), the SA (sacral angle), and the lumbar lordosis angle (LLA) present greater values in the females. At the control WCO2, only the LLA resulted greater in the females. Such a postural characteristic must be considered physiological because it is typically observed in the healthy young population in IO and WCO (D’Amico et al., 2017b).
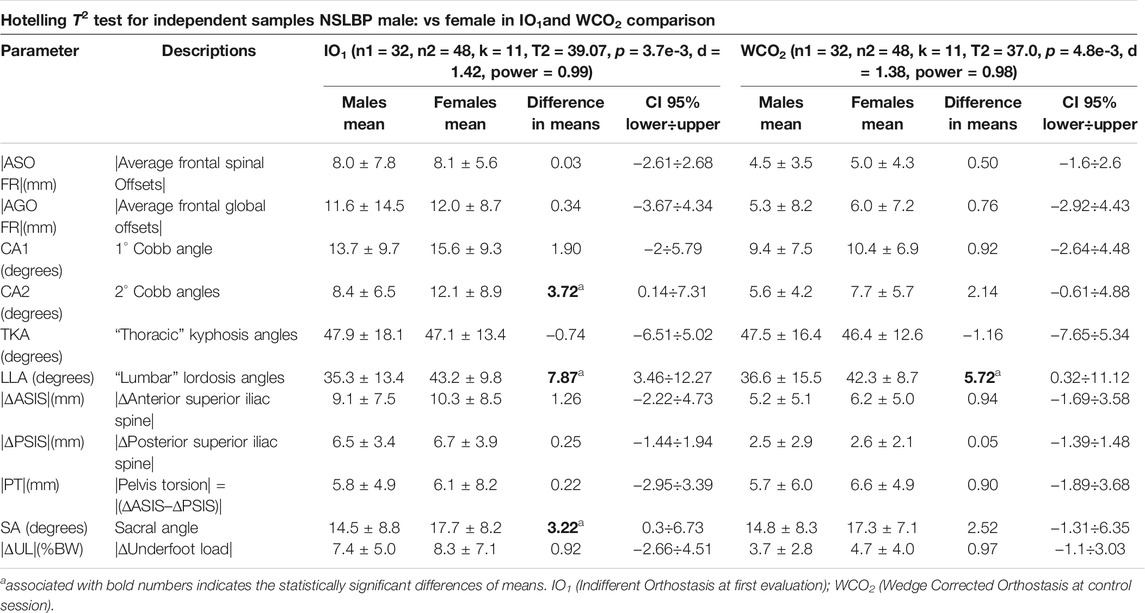
TABLE 3. NSLBP Males vs. Females comparisons in IO1 and WCO2: Hotelling T2 tests results, 95% confidence intervals and difference of means.
The IO1 vs. WCO1 at first evaluation and IO2 vs. WCO2 at control sessions, after the adaptation period, evaluated by gender, provide information about the immediate-term postural reaction to LLD equalization (Table 4).
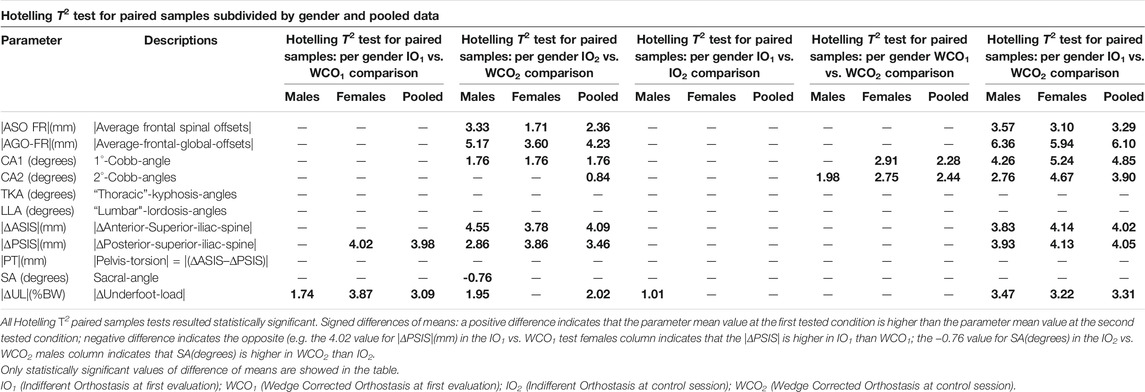
TABLE 4. Hotelling T2 test for paired samples subdivided by gender and pooled data: IO1 vs. WCO1, IO2 vs. WCO2, IO1 vs. IO2, WCO1 vs. WCO2 and IO1 vs. WCO2.
The IO1 vs. WCO1 (immediate-term answer) shows that, at the beginning, at the group level, the statistically significant changes are few. Specifically, in the IO1 vs. WCO1, males present differences (improvements) only in |ΔUL| (underfoot load asymmetry). Conversely, in the females, the statistically significant changes (improvements) are in the |ΔUL| (underfoot load asymmetry) and |ΔPSIS| (pelvis obliquity as measured at the PSIS). When males and females are pooled together, the improvements are the same as in the females’ group.
More differences are evident in the immediate-term answer after the adaptation period. In the IO2 vs. WCO2 comparison, males show significant improvements in CA1, both the spinal and global frontal offsets (|ASO FR| and |AGO FR|), the pelvic obliquity (|ΔASIS| and |ΔPSIS|), the underfoot load asymmetry (|ΔUL|) and the SA. The same differences, except for SA, are found in females. When pooled together, in addition to the above parameters (excluding SA), also CA2 shows statically significant improvements.
The IO1 vs. WCO2 and the IO1 vs. IO2 and WCO1 vs. WCO2 (evaluated by gender) provide information about the structural postural changes induced by custom foot orthotics after the adaptation period. In the IO1 vs. WCO2 comparison, the results highlight clear statistically significant improvements in all the postural parameters of the frontal plane, including the underfoot load asymmetry (|ΔUL|), and it holds for all such parameters for males, females, and in the pooled females–males group as well (Table 4).
Conversely, the IO1 vs. IO2 comparison shows statistically significant improvement only in the underfoot load asymmetry (|ΔUL|) and only in men. In the WCO1 vs. WCO2 comparison, the improvements appear statistically significant in spine deformity angles (CA1 and CA2) for both genders.
The time-dependent (medium and long-term) and the age-dependent (older vs. young adults) effects of LLD equalization induced by customized foot orthotics are summarized in Table 5.
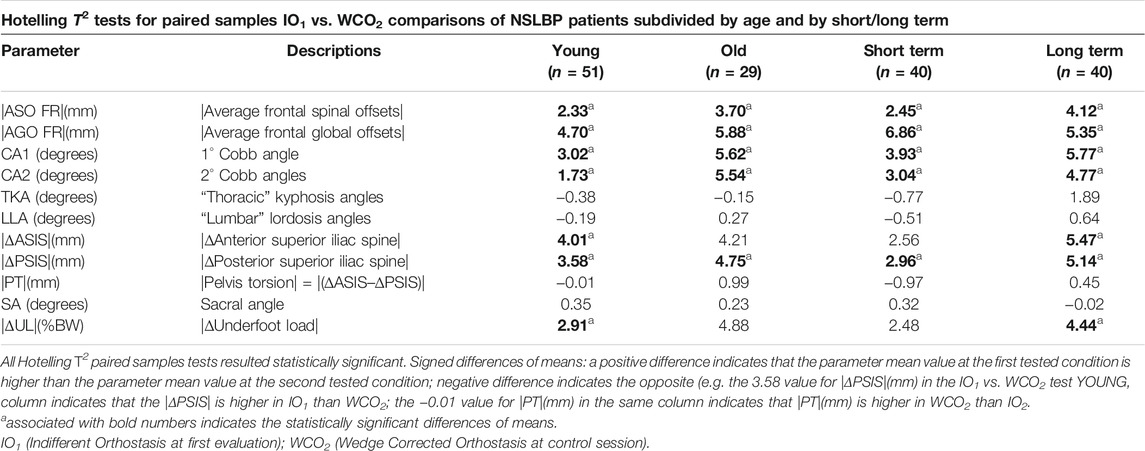
TABLE 5. Hotelling T2 tests for paired samples for the IO1 vs. WCO2 comparisons of NSLBP patients subdivided by age and by short/long term.
The medium-term results highlight clear statistically significant improvements in all the postural parameters of the frontal plane except for the underfoot load asymmetry |ΔUL| and the |ΔASIS| that require a more extended adaptation period as demonstrated by the long-term group in which also these two latter parameters become significantly improved.
The age-dependent (older vs. younger adults) comparison shows similar results to the time-dependent comparison. Indeed, the older adults group present statistically significant improvements in all the postural parameters of the frontal plane except for the underfoot load asymmetry |ΔUL| and the |ΔASIS|. Conversely, in the younger adults, all the postural parameters of the frontal plane present statistically significant improvements.
3.1.2 Differences Between NSLBP Patients and Healthy Young Adults in Postural Characteristics and Reactions to LLD Equalization, Evaluated by Gender
The IO1H vs. WCO1H (immediate-term answer) shows that the female healthy young adults present significant improvements in both the frontal offsets |ASO FR| and |AGO FR|, in the pelvic obliquity by both the |ΔASIS| and |ΔPSIS|, and in the reduction of underfoot load asymmetry |ΔUL| (Table 6). The same improvements are also present for the healthy young males, but in this case, the |ΔASIS| and |ΔUL| do not reach statistical significance.
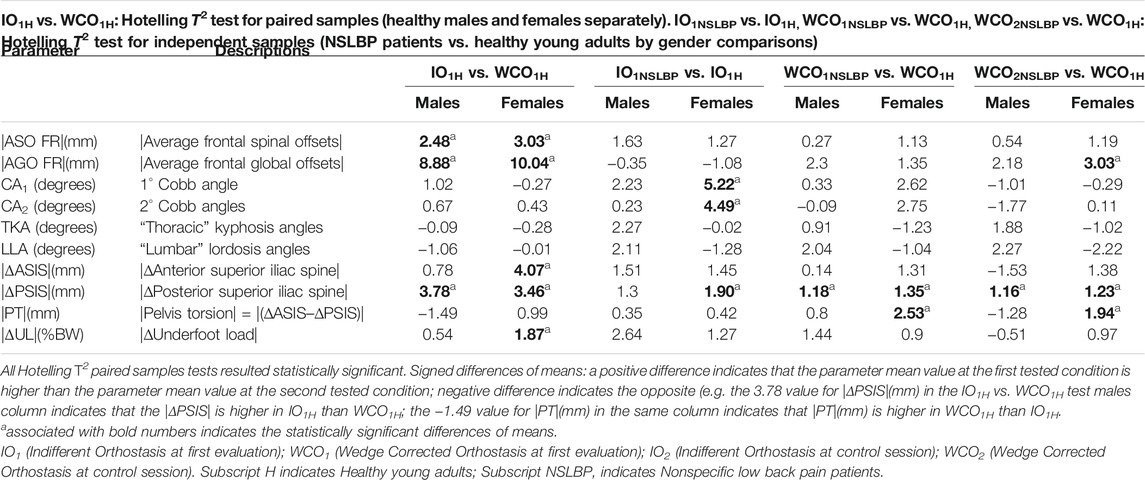
TABLE 6. NSLBP patients vs. healthy young adults subdivided by gender for the following comparisons: IO1H vs. WCO1H, IO1NSLBP vs. IO1H, WCO1NSBLP vs. WCO1H, and WCO2NSBLP vs. WCO1H.
The IO1NSLBP vs. IO1H (evaluated by gender) provides information about the two groups’ structural postural characteristics. NSLBP females show significantly greater values in CA1, CA2, and |ΔPSIS| respect to healthy young women. Conversely, no differences are present for the males.
Comparing the immediate and medium-long term answers to LLD equalization, the NSLBP patients show residual difference only in |ΔPSIS| and |PT| for females and |ΔPSIS| for males. This happens in both WCO1NSBLP vs. WCO1H and WCO2NSBLP vs. WCO1H comparisons. Furthermore, it is possible to evaluate that the adaptation over time to LLD equalization reduces the differences between NSLBP patients and healthy subjects (Table 6).
3.1.3 Correlation Between Heel Lift Thickness and Changes in CA1
At the first evaluation, the value of optimal thickness for LLD correction in NSLBP patients resulted in 10.4 ± 6.2 mm for males, 10.7 ± 7.2 mm for females, and 10.6 ± 4.8 mm pooled. No LLD statistical difference between genders has been found. At the control session, after the adaptation period, the LLD values presented a slight increase for males 11.8 ± 6.6 mm and females 11.1 ± 8.4 mm (still without statistical difference between genders). The mean main CA1 variation passing from IO1 to WCO2 is 4.8° ± 6.1°.
The correlation between the changes of the main CA1 passing from IO1 to WCO2 and the heel lift correction determined at first evaluation is low Tau = 0.17 but statistically significant (p = 0.032).
3.2 Intrasubject Statistical Analysis
Figures 4, 5 report the results of the intrasubject analysis.
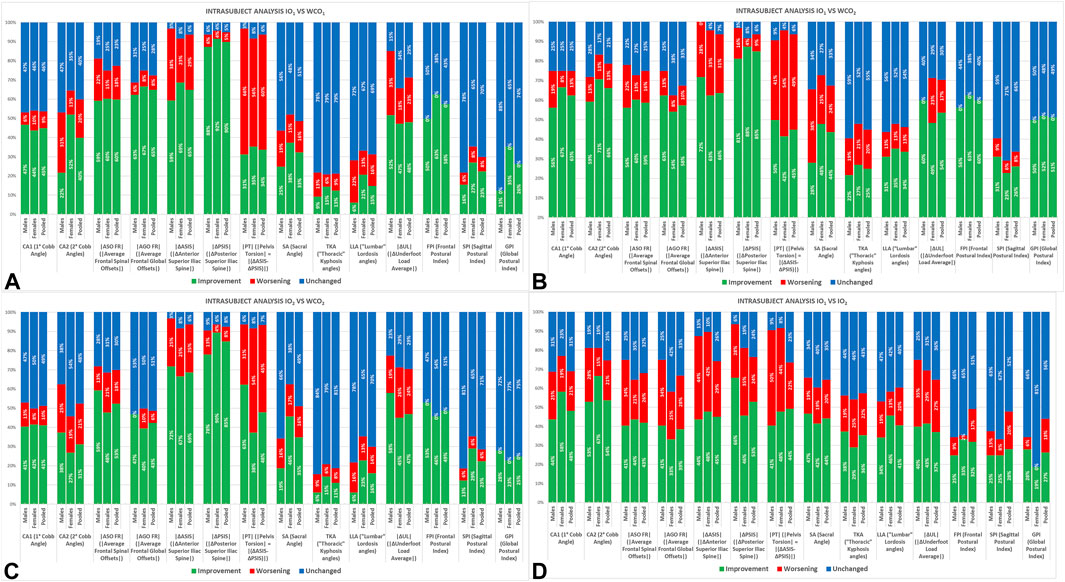
FIGURE 4. Intrasubject analysis subdivided by gender and pooled in NSLBP patient for the following comparisons: IO1 vs.WCO1 (A), IO1 vs. WCO2 (B), IO2 vs.WCO2 (C), and IO1 vs. IO2 (D). IO1 (IO at first evaluation); WCO1 (WCO at first evaluation); IO2 (IO at control session); WCO2 (WCO at control session).
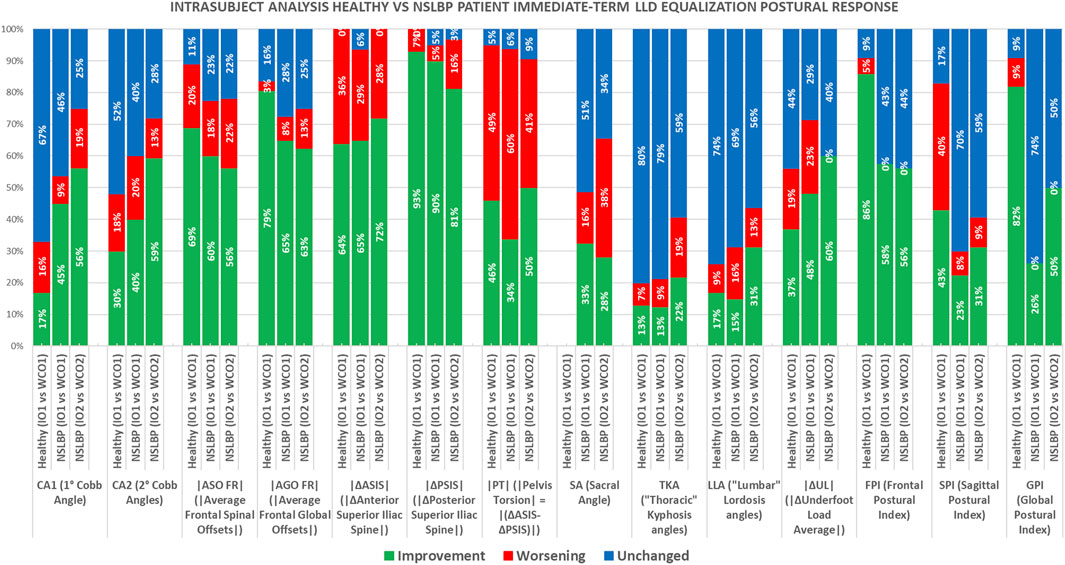
FIGURE 5. Intrasubject analysis healthy young adults’ vs. NSLBP patients’ pooled data immediate-term LLD equalization postural response comparisons: IO1H vs. WCO1H, IO1NSLBP vs. WCO1 NSLBP, IO2 NSLBP vs. WCO2 NSLBP. Healthy young adults’ pooled data for the IO1 vs. WCO1 (data from (D’Amico et al., 2017b)). IO1 (IO at first evaluation); WCO1 (WCO at first evaluation); IO2 (IO at control session); WCO2 (WCO at control session). Subscript H indicates healthy young adults; subscript NSLBP indicates nonspecific low back pain patients.
The IO1 vs. WCO1 intrasubject analysis (Figure 4A) shows the immediate effect of the LLD equalization. Almost all frontal plane parameters and, additionally, |ΔUL| improve in both sexes (FPI shows “improvements” in 50% of males and 63% of females). Females show more “improvements” than males displaying higher percentages and favorable changes in a higher number of parameters.
Such results are consolidated at control after the adaptation period (IO1 vs. WCO2), in which males tend to reach the same effects as females (Figure 4B).
The IO2 vs. WCO2 intrasubject analysis (Figure 4C) is analogous to the IO1 vs. WCO1 but shows further “improvements” in pelvis torsion (|PT|).
In the IO1 vs. IO2 (Figure 4D), almost all parameters display higher percentages of “improvements” than “worsening” as witnessed by the summarizing indexes: FPI “improvements” (33% for females and 25% for males), SPI “improvements” (25% both for females and males), GPI “improvements” (19% for females and 28% for males).
Worth underlining with the LLD equalization, the “worsening” percentage for FPI and GPI equals zero in all the conditions IO1 vs. WCO1, IO2 vs. WCO2, IO1 vs. WCO2.
By analyzing the comparison with the healthy young adults (Figure 5), it results that, at immediate term, NSLBP patients improve more than the healthy subjects in those parameters in which they present the higher structural deviations from “healthy status,” such as CA1, CA2, and |ΔUL|, whereas the healthy individuals improve more on |ASO FR| and |AGO FR|.
4 Discussion
The present study aimed to define if LLD equalization treatment is effective in NSLBP patients to remove pain symptoms and structural-biomechanical asymmetries.
Eleven quantitative biomechanical parameters describing the nature of 3-D entire body posture were considered to study the gender, age-related, and time-dependent effects of LLD equalization treatment in NSLBP patients during 2 years of follow-up.
Our basic hypothesis was that the uncertainty about LLD measurement and treatment in LBP debated in the literature is mainly due to the lack of detailed, rigorous biomechanical-functional information on 3-D natural standing posture and gait characteristics and how the presence of an LLD influences them.
Indeed, given changes in multiple parameters that tend to occur with LLD (e.g., sacral or pelvic tilt and lumbar scoliosis, compensations in the lower limbs), it is likely that confounders are at play. Therefore, it is difficult to identify the true drivers of LBP in the LLD–LBP relationship (Sheha et al., 2018).
To tackle the several confounders at play, we used the nonionizing optoelectronic stereophotogrammetric approach associated with baropodometry presented in D’Amico et al. (2017a, 2017b). Such a method provides the evaluation of the 3-D entire skeleton posture, including the 3-D spine shape. In this way, it is easy and fast to quantify the eventual presence of an LLD and the whole skeleton functional postural adjustments when a heel-lift LLD correction is applied. As explained in the “Materials and Methods” section, to take into account both “anatomical” and “functional” LLD (Knutson, 2005a; Knutson, 2005b), the corrective wedge optimal size was determined as the degree of thickness that provided the best postural outcome for the individual in the frontal-plane parameters. Conversely, when it was impossible to achieve an overall improvement of the frontal-plane postural parameters, the optimal corrective-wedge thickness was chosen as the one providing the best equalization regarding the PSIS landmarks (D’Amico et al., 2017b).
In the present paper, the investigation has been limited to studying a 3-D entire skeleton standing posture in various conditions. No gait analysis has been performed.
At the first evaluation, the value of optimal thickness for LLD correction in NSLBP patients resulted in 10.4 ± 6.2 mm for males and 10.7 ± 7.2 mm for females. Such values are only about 1 mm higher than those found for healthy young adults (9.7 mm) in D’Amico et al. (2017b), and as in this previous study, no LLD statistical difference between genders has been found. Worth noting, at the control session, after the adaptation period, we found a slight increase of the LLD values resulting for males 11.8 ± 6.6 mm and females 11.1 ± 8.4 mm (still without statistical difference between genders). Such an outcome is likely to be connected to the pelvis torsion reduction after the LLD equalization adaptation period.
The patients were provided with customized foot orthotics incorporating a heel lift on the short leg side. The foot orthotics customization was supplied according to patients’ therapeutic needs, inducing LLD equalization and better biomechanical foot function (D’Amico et al., 2021b) (by considering the foot deformities and foot-floor interaction using baropodometry and 3-D foot scans). The heel lifts were corrective of the 100% LLD (D’Amico et al., 2012; D’Amico et al., 2017b) and were shaped from the calcaneus to the Lisfranc joint.
This study’s set of results leads to defining a coherent framework of postural responses to LLD equalization. A straightforward answer is that the heel-lift custom-made orthotics to equalize LLD is effective in NSLBP to remove pain symptoms and structural-biomechanical asymmetries.
The data confirm that the use of orthotics with 100% LLD equalization has no contraindications. In addition to the progressive total disappearance of painful symptoms, LLD equalization produces progressive improvements in postural parameters in the immediate, medium, and long terms. Specifically, the frontal plane parameters are the most strongly influenced by the orthotics corrections showing improvements for the majority of the subjects. Moreover, a not negligible percentage of NSLBP patients (31.3% of males and 22.9% of females) presented improvements in the sagittal plane after the medium-long term adaptation.
No patient presented any worsening in the frontal plane as witnessed by the FPI = 0% for “worsening” in each intrasubject comparison involving LLD equalization, i.e., IO1 vs. WCO1, IO2 vs. WCO2, IO1 vs. WCO2.
It is evident that there are immediate-term responses (IO1 vs. WCO1) to LLD equalization stimulus reducing unbalancing and asymmetries; medium-term (IO1 vs. WCO2 4 months follow up group), and long-term (IO1 vs. WCO2 2 years follow up group) responses that lead to structural changes.
From the group statistical analysis (confirmed at intrasubject level), on the gender, age-related, time-dependent effects of LLD equalization treatment, it can be noted that the adaptation times are individual, being connected to the relative joint mobility of the pelvis (in particular the sacroiliac joint) and spine. For such reasons, there could be some light differences between genders.
For example, the differences between genders in NSLBP patients are evaluated in two occurrences (Table 3): i.e., through the comparison of the indifferent orthostasis at the first evaluation (IO1) and through the comparison of the wedge-corrected orthostasis at the follow up (WCO2). In both assessments, the females tended to have more significant asymmetries and spinal deformities than males even if such difference does not reach the statistical significance except for CA2 in IO1 (Table 3).
The value of LLA is significantly higher in women than in men as it happens for healthy young adults (D’Amico et al., 2017b) in both IO1 and WCO2.
In any case, at follow-up (WCO2), both the genders converge toward a similar final improved and more balanced standing erect posture. Starting from a worse postural condition, the women show a more marked improvement, particularly spinal deformities reduction.
The intrasubject statistical analysis (Figure 4) confirmed the difference between the sexes in IO1 vs. WCO1 and IO1 vs. WCO2. Indeed, the women present a higher percentage of “improvements” in almost all frontal plane values (or positive changes in a higher number of parameters) as witnessed by the summarizing indexes starting from the immediate effect (FPI shows “improvements” in 50% of males and 63% of females in IO1 vs. WCO1). Even in the sagittal plane, females perform better than males. Such results are consolidated at control after the adaptation period when the IO1 vs. WCO2 shows “improvements” in most patients, and males tend to reach the same results as females. Some parameters show a faster answer to the LLD equalization as it happens to |ΔPSIS| (WCO1), and some others need a more extended adaptation period leading to a higher percentage of “improvements” at control (WCO2) as it happens for |ΔASIS| and so |PT|, CA1, CA2. The pelvis obliquity at posterior-superior iliac spine (|ΔPSIS|) level disappeared for more than 85% of subjects with the women improving in a more significant percentage than men (women 88%, men 81% of improvements). Conversely, only about 66% of the patients achieved the anterior-superior iliac spine (|ΔASIS|) leveling. In this case, men present a more significant percentage of improvements than women (women 63%, men 72%). Women demonstrated a better reduction of the spinal deformities (67% vs. 56% for primary CA1 and 71% vs. 59% for secondary CA2). For the underfoot load asymmetry (|ΔUL|), an overall improvement is obtained for 54% of subjects (women 49%, men 60%). This condition is also confirmed by the global body side-leaning (|AGO FR|), which globally improved in 58% of the sample (women 54%, men 63%). Regarding the trunk side-leaning (|ASO FR|), the results showed improvements in 59% of subjects (women 60%, men 56%).
Regarding the time-dependent LLD equalization effects, it is possible to see that the immediate response (IO1 vs. WCO1) induces high percentages of improvements in the intrasubject analysis (Figure 4) in almost all the frontal plane parameters (especially in women). However, such high percentages are not enough to obtain a general statistical significance in the group analysis. Indeed, only |∆UL| for both genders and |∆PSIS| for females are significantly different (Table 4), thus indicating the considerable variability of individual responses.
Conversely, in the medium–long term LLD equalization (i.e., IO1 vs. WCO2), the effects after the adaptation period show the convergence to an overall statistically significant improvement for all the frontal parameters at group level in males and females. The most relevant improvements as highlighted by Hotelling’s T2 (Table 4) are the reductions of spinal deformities (CA1: 5.24° females 4.26° males, 4.85° pooled, and CA2: 4.67° females, 2.76° males, 3.9° pooled), underfoot load asymmetry (|∆UL|: -body weight percentage - 3.22% females, 3.47% males, 3.31% pooled) and pelvis horizontality realignment (|∆PSIS|: 4.13 mm females, 3.93 mm males, 4.05 mm pooled, and |∆ASIS|: 4.14 mm females, 3.83 mm males, 4.02 mm pooled).
Such behavior is likely related to structural changes in the pelvis, spine, proprioception, and motor control that induce better postural balance and more symmetrical distribution of underfoot loads.
A further understanding of how the medium–long term equalization acts structurally in modifying the standing posture can be obtained by associating the results of the following group comparisons (Table 4) IO1 vs. WCO2, IO1 vs. IO2, and WCO1 vs. WCO2.
In the IO1 vs. IO2 comparison, only the underfoot load asymmetry (|ΔUL|) is statistically significant and only for men. Whereas in the WCO1 vs. WCO2 comparison, improvements are statistically significant only in spine deformity angles (both the CA1 and CA2) for both genders.
Such outcomes, considered together with the IO1 vs. WCO2 comparison, demonstrate that the improvements obtained after the adaptation period using the customized foot orthotics determine significant changes in the postural structure of the body and also that these structural changes are maintained only through the continuous use of LLD and foot posture corrections. Otherwise, the patients’ posture tends to return to the initial conditions.
Such structural changes modify the immediate term answer to LLD equalization as it is possible to evaluate by considering the IO1 vs. WCO1 and the IO2 vs. WCO2 comparisons. Indeed, the IO1 vs. WCO1 comparison displays that the immediate response to LLD equalization acts significantly only on the |∆UL| and |∆PSIS| parameters. Conversely, in IO2 vs. WCO2 (which represents the immediate response to LLD equalization at control after the adaptation period) the same parameters, also significant in the IO1 vs. WCO2 comparison, are statistically significant.
This set of outcomes leads us to conclude that the adaptation period induces progressive changes in joint mobility and neuromotor control, particularly at the pelvis and spine level. This is achieved through the various functional activities (walking, standing in various conditions, etc.) performed while wearing the customized corrective insoles, inducing changes in body balance and proprioception, stimulating new motor control strategies. These changes modify the response to the stimulus in those parts of the body that showed structural alterations at the first evaluation (spinal deformities, torsion of the pelvis, etc.). The |∆UL| is the only parameter in which the motor control system shows a memory of the “adaptation-learning” phenomenon even without LLD equalization as highlighted by the IO1 vs. IO2 comparison. When the stimulus is applied again (i.e., in the IO2 vs. WCO2), the response involves the entire structure, inducing significant changes in all the frontal plane postural parameters and partially also in sagittal plane parameters. Therefore, it is evident that equalization acts on the whole-body structure and the neuromotor system. It is also evident that when the stimulus is missing, the posture returns to the initial state of imbalance (IO1 vs. IO2). Thus, LLD equalization needs some adaptation time (the duration of which varies on an individual basis) to be effective on the entire body posture, and it must be maintained over time to prevent the body structure from returning to its original asymmetric unbalanced state. Indeed, if LLD equalization is removed, the process of progressive formation of structural deformities and asymmetries restarts.
Other aspects of the coherent framework of postural responses to LLD equalization derive from the analysis of young vs. older adults’ behavior and the medium- vs. long-term adaptation period.
From Table 5, it is possible to highlight that older adults start from conditions of more significant postural asymmetries and spinal deformities. LLD equalization induces significant improvements in all frontal plane parameters in younger adults, including the underfoot load asymmetry (|∆UL|). Conversely, LLD equalization induces improvements of greater magnitude in older adults than in younger adults though they reach the statistical significance in a reduced number of parameters.
Comparing the medium to the long-term adjustment period shows that the longer, the better as the long-term group reaches the statistical significance in all the frontal plane parameters, including the |∆UL| (Table 5). Thus, confirming the need for an adaptation period long enough to obtain structural posture changes.
Comparing healthy young adults and NSLBP patients adds another piece to the jigsaw puzzle.
Interestingly, the comparison of indifferent orthostases (i.e., the IO1H vs. IO1NSLBP) between healthy young adults and NSLBP patients shows very few differences in the natural upright posture. NSLBP men present no significant difference compared with healthy young adults. In contrast, women display significant differences in spinal deformities and |ΔPSIS| parameters. Minimal differences between healthy young adults’ and NSLBP patients’ postures are also confirmed when LLD equalization is applied. In such a condition, the differences between healthy young subjects and NSLBP patients tend to vanish in almost all the considered parameters except for residual difference only in |ΔPSIS| and |PT| for females and |ΔPSIS| for males (Table 6). Worth noting such convergence is already at the immediate term response, i.e., WCO1H vs. WCO1NSLBP and successively confirmed at medium–long term WCO1H vs. WCO2NSLBP when the adaptation over time to LLD equalization produces the effect of a further reduction of the residual differences between NSLBP patients and healthy subjects.
Comparing the intrasubject performance of healthy young adults and NSLBP patients shows that, in the short term, the latter present higher percentages of “improvements” compared with healthy subjects in those parameters in which the structural deviations from the “healthy status” are the most remarkable (Figure 5), such as CA1, CA2, and |ΔUL|. Healthy young adults show higher rates of “improvements” on |ASO FR| and |AGO FR| and a very high percentage of “unchanged” in the sagittal plane. In such latter plane, patients with NSLBP show better performance with relevant percentages of “improvements.”
LLD is reported as relatively common in the literature, affecting up to 90% of the population with an average value of 5.2 ± 4.1 mm as measured by highly precise radiographic millimetric methods (Knutson, 2005a). The relationship between LLD and LBP is an open debate. LLD is correlated with asymmetrical distribution and magnitude of mechanical stresses and strains in spine joints, degenerative changes in the lumbar spine, alterations in spinal biomechanics, and LBP (Giles, 1981; Friberg, 1982; Friberg, 1983; Gofton, 1985; Helliwell, 1985; Rossvoll et al., 1992; ten Brinke et al., 1999; Defrin et al., 2005; Golightly et al., 2007; Kendall et al., 2014; Murray and Azari, 2015; Cambron et al., 2017; Menez et al., 2021; Menez et al., 2020).
LLD is associated with some degree of pelvic tilt in the coronal plane and very often with pelvis torsion in the sagittal plane, deriving from distinct inter-ASIS and inter-PSIS height differences. Pelvis torsion is defined as an intrasegmental pelvic pattern in which one ilium is more tilted toward the anterior than the other (Beaudoin et al., 1999; Knutson, 2005a; D’Amico et al., 2017b).
Authors Gurney (2002) and Raczkowski et al. (2010) describe that alterations in pelvis symmetry, the tilt on the frontal plane, and the 3-D pelvis torsion induce changes in the sacrum attitude, producing variations in the dynamics of the lumbar vertebrae and possibly developing LBP and lumbar scoliosis with a higher probability to present convexity toward the short limb (Giles and Taylor, 1982; Murray et al., 2017; Sheha et al., 2018; Gordon and Davis, 2019). Even mild extents of LLD can change pelvic posture (Betsch et al., 2012, 2013; Kwon et al., 2015; D’Amico et al., 2017b). This latter can be associated with several postural compensations. These compensations may include foot pronation and/or hip and knee flexion on the longer limb, foot supination and/or hip and knee extension on the shorter limb. These induce muscle imbalance, which may cause several dysfunctions at various levels such as the sacroiliac joint (Perttunen et al., 2004), hip flexor contracture on the long limb, or short limb plantar flexor contracture (Raczkowski et al., 2010).
Such compensations induce biomechanical-functional changes in the foot–ground interaction that must be corrected with customized insoles.
As a consequence, the coexistence, in most cases, of anatomical and functional contributions adds difficulties to the LLD evaluation. Among the clinical methods, the tape method has been criticized. Indeed, potential sources of error of such a technique relate to differences in leg circumference, angular deformities, and difficulty in accurately palpating bony prominences as well as joint contractures (Cleveland et al., 1988; Beattie et al., 1990; Rondon et al., 1992; Terry et al., 2005; Sabharwal and Kumar, 2008). In contrast, the use of standing blocks under the short leg to level the pelvis is more reliable and complete than tape measurement by giving the possibility also to consider the LLD functional component but not as accurate as imaging modalities. However, even on imaging tools to be used, there is a debate, and they present pros and cons, especially thinking about the risk connected to X-ray use (Sabharwal and Kumar, 2008) for some of them.
The magnitude of LLD likely plays a role in LBP although it is unclear what degree of LLD is required to cause symptoms (Friberg, 1982; Friberg, 1983; Defrin et al., 2005; Golightly et al., 2007; Kendall et al., 2014; Murray and Azari, 2015; Cambron et al., 2017; Menez et al., 2021; Menez et al., 2020). Some investigators have tried to quantify a significant LLD, accepting as much as 20 (Gross, 1978) to 30 mm (Reid and Smith, 1984) as the minimal difference to be corrected, whereas others define a significant discrepancy in terms of functional outcomes (Abraham and Dimon, 1992). Various authors find postural unbalancing and disorders associated with LLD lower than 10 mm (Giles and Taylor, 1976; Giles and Taylor, 1982; Friberg, 1983; Defrin et al., 2005; D’Amico et al., 2012).
However, there is still no convergence of opinions leading to the shared definition of treatment guidelines (Sheha et al., 2018). For such a reason, more recently, it has been suggested that “it must be discussed with each patient individually whether the treatment should be conservative or surgical. The extent of the discrepancy is not the sole determining factor for the mode of treatment. The decision to treat is always elective” (Vogt et al., 2020).
Indeed, also body functional activity likely plays a role in the LLD–LBP relationship. Rannisto et al. (2015) established that workers with LLD who have to stand for many hours for their work activity are more likely to have LBP than seated workers. This result looks pretty reasonable, especially from the biomechanical point of view, as the LLD can induce high load asymmetries and, therefore, high asymmetrical stresses that can be distributed at the spine level leading to postural imbalances and spinal deformities as our results describe.
It is worth noting that the same group (Rannisto et al., 2019) conducted a randomized controlled study on those standing work activity workers (meat cutters) presenting LBP associated with LLD. They obtained reduced subjective pain and the probability of taking sick leave days by applying an LLD heel lift of 5 mm or more.
Even the best corrective thickness of shoe lifts is a matter of debate. Indeed, there is no agreement on the LLD measurement method and the rationale to apply for the correction given the difficulty of evaluating its global influence on standing posture from a functional point of view. Studies present the following approaches: lifts equal to the amount of LLD (Raczkowski et al., 2010; D’Amico et al., 2012; D’Amico et al., 2017b), lifts few millimeters less than the amount of LLD (Friberg, 1982), lifts equal to LLD minus 10% (Chuter et al., 2014; Ashour et al., 2019), a lift of 50% of LLD (Menez et al., 2021; Menez et al., 2020), and lifts that caused resolution of LBP symptoms (Golightly et al., 2007). It is not a surprise that the strategies for lift application were variable. Researchers provide lifts to correct LLD completely at once (Gurney, 2002; D’Amico et al., 2012; D’Amico et al., 2017b), whereas others (Defrin et al., 2005; Golightly et al., 2007) provided lifts that were gradually adjusted over time.
The quantitative functional evaluation of posture helps to overcome all the above issues. Indeed, it highlights a strong relationship between LLD and postural unbalancing associated with NSLBP and allowed to find the optimal LLD equalization.
Given the individual biomechanical-functional compensations due to LLD and the individual responses to LLD equalization as also witnessed by the low correlation between heel lift thickness and changes in main CA1, identifying the minimum LLD to treat is an “ill-posed” problem. Indeed, the same LLD can produce different outcomes for subjects with different anthropometric, proprioception, and motor control characteristics. Thus, the treatment must be individualized through appropriate measurement approaches.
The proposed approach helps to overcome also the controversial debate on functional vs. anatomical LLD nature (Knutson, 2002; Knutson, 2005a; Knutson, 2005b). The optimal LLD correction induces overall postural parameter improvement, disregarding either the functional or anatomical origin of LLD.
The suitable heel rise correction was included in the customized orthotics to fix the foot functional behavior using the complementary information derived from baropodometry and 3-D foot scan. Furthermore, the present study demonstrates that the application of the correction equal to 100% of the measured LLD, which was included in the orthotics soon after the first evaluation in a complete way (i.e., without starting with smaller thicknesses, which were progressively increased up to the final desired thickness), led to improvements without any overall worsening or adverse effects. Quick pain relief responses to LLD equalization have been noted in the agreement with many other studies (including also randomized control studies) (Friberg, 1982; Friberg, 1983; ten Brinke et al., 1999; Defrin et al., 2005; Golightly et al., 2007; Kendall et al., 2014; Murray and Azari, 2015; Cambron et al., 2017; Sheha et al., 2018; Rannisto et al., 2019; Menez et al., 2021; Menez et al., 2020) up to the complete pain remission after an adaptation period, the duration of which is strictly individual. Personalized therapy/rehabilitation treatments would surely help to speed up and stabilize the recovery process (Giles and Taylor, 1976). Such treatment should consider the clinical and postural evaluation and focus on increasing joint mobility and muscle strength to reduce spinal deformities and improve proprioception and motor control to equalize the asymmetric muscular efforts associated with LLD.
Studying the posture in healthy young adults, D’Amico et al. (2017b) established that “to be asymptomatic does not mean to have an optimal posture. It seems that asymmetry (associated with LLD unbalanced postural and underfoot loads, spinal curvature in the frontal plane, and pelvis torsion) is standard in both sexes.” The comparison of the NSLBP sample with the healthy young adult population showed that the postural-structural characteristics of the two groups were very similar except for the presence of more pronounced spinal deformities in NSLBP females and generally of a slightly higher LLD (1–2 mm) in NSLBP patients. Depending on individual morphological-physiological characteristics and proprioceptive and motor control traits, the prolonged condition of imbalance due to LLD could lead to several postural-functional compensations, accelerating degenerative processes leading to musculoskeletal injury disorders and spinal deformities accentuation (Friberg, 1983; Defrin et al., 2005; Tallroth et al., 2005; Harvey et al., 2010; Kendall et al., 2014; Murray and Azari, 2015; Tallroth et al., 2017; Sheha et al., 2018; Gordon and Davis, 2019). We also observed that older NSLBP patients presented greater asymmetry values than the younger NSLBP patients. The long-term LLD equalization produced better postural outcomes than the immediate and medium-term. Such results bring us to strengthen the hypothesis, presented many times in literature that there is likely a biomechanical component in the development of NSLBP and other spinal and lower limb musculoskeletal disorders, such as hip or knee osteoarthritis (Chuter et al., 2014; Cambron et al., 2017; Campbell et al., 2018; Sheha et al., 2018; Gordon and Davis, 2019; Rothschild et al., 2020). However, as demonstrated, if the LLD is equalized, the degenerative process can be slowed down or even reversed, at least from a postural-functional point of view.
The set of results of the present study provide strong support to the hypothesis that even asymptomatic healthy young people with LLD, asymmetrical underfoot load distributions, and associated mechanical stresses and strains in spine joints, over time, could evolve into alterations in spinal biomechanics, inducing degenerative changes in the lumbar spine and, therefore, develop LBP or other pathologies. Such considerations confirm the hypothesis of D’Amico et al. (2017b) that “as a rule of thumb, it could be argued that to prevent eventual possible long term negative consequences of asymmetry due to musculoskeletal imbalance on joints and spine, clinicians could apply a suitable form of LLD equalization and prescribe regular/periodic performance of focused physical activity, in order to reduce asymmetry in the subject.”
A limitation of this study is that the analysis of the postural response to LLD equalization in the medium and long term was conducted by pooling together people of different ages not separated by gender although we verified the existence of different responses due to both sex and age. Similarly, the analysis of the postural response to LLD equalization in younger and older adults was conducted by grouping together medium and long-term follow-up NSLBP patients not separated by gender. Such a choice was due to the limited size of the sample. Such kind of further detail will be a matter of future study. In the literature, it is suggested that gait and range of movements analysis for patients with LBP provide better functional-clinical observation and treatment (Bratton, 1999). In previous papers, we describe how the proposed methodology, also in association with surface electromyography, can quantitatively detail the spine range of movement and the affected flexion-relaxation phenomenon in the trunk forward bending as well as a thorough evaluation of gait characteristics in LBP patients (D’Amico et al., 1995b; D’Amico et al., 2008; D’Amico et al., 2021a). Such evaluations were not included in the present study. This will be a matter for future research.
5 Conclusion
Heel-lift customized orthotics with 100% LLD correction are an effective short- and long-term treatment in patients with NSLBP, inducing complete recession of pain symptoms and stimulating postural parameter improvement without contraindications. LLD equalization needs some adaptation time, the duration of which varies on an individual basis, to be effective on the entire body posture. Such benefits are maintained if the customized orthotics are donned. The interruption of their use can lead to progressive regression with the return to the starting postural conditions.
The 3-D stereophotogrammetry is demonstrated to be a helpful tool to perform the postural measurement, quantifying LLD magnitude and the LLD effects on whole skeleton posture and spine deformities, correction, and monitoring. Indeed, it highlights a strong relationship between LLD and postural unbalancing associated with NSLBP and allows finding the optimal LLD equalization.
Given the individual biomechanical-functional compensations due to LLD and the individual responses to LLD equalization, identifying the minimum LLD to treat is an ill-posed problem. The same LLD can produce different outcomes for subjects with different anthropometric, proprioception, and motor control characteristics. Thus, the treatment must be individualized through appropriate measurement approaches.
Healthy young adults and NSLBP patients show very few differences in the natural upright posture. Minimal differences between healthy young adults’ and NSLBP patients’ postures are also confirmed when LLD equalization is applied, showing the tendency to vanish in almost all the considered parameters.
Data Availability Statement
The raw data supporting the conclusion of this article will be made available by the authors, without undue reservation.
Ethics Statement
The studies involving human participants were reviewed and approved by The Ethics Committee of the University of Medical Sciences in Poznan, Poland, approved this study. Resolution number: 376/17. The patients/participants provided their written informed consent to participate in this study.
Author Contributions
All authors listed have made a substantial, direct, and intellectual contribution to the work and approved it for publication.
Funding
The authors received funding and support in the form of GOALS System free use from Bioengineering and Biomedicine Company Srl.
Conflict of Interest
MD and PR own shares of the Bioengineering and Biomedicine Company Srl. This does not alter our adherence to Frontiers in Bioengineering and Biotechnology policies on sharing data and materials. The Bioengineering and Biomedicine Company Srl did not play any direct role in the study design, data collection and analysis, decision to publish, or manuscript preparation.
The remaining author declares that the research was conducted in the absence of any commercial or financial relationships that could be construed as a potential conflict of interest.
Publisher’s Note
All claims expressed in this article are solely those of the authors and do not necessarily represent those of their affiliated organizations, or those of the publisher, the editors and the reviewers. Any product that may be evaluated in this article, or claim that may be made by its manufacturer, is not guaranteed or endorsed by the publisher.
Acknowledgments
The authors wish to acknowledge the Bioengineering and Biomedicine Company Srl, Italy, for providing GOALS System use for free, letting the authors freely use it without any limitations to carry out the study measurements the accomplishment of the present study.
Supplementary Material
The Supplementary Material for this article can be found online at: https://www.frontiersin.org/articles/10.3389/fbioe.2021.743132/full#supplementary-material
Abbreviations
IO, indifferent orthostasis; LBP, low back pain; LLD, leg length discrepancy; NSLBP, nonspecific low back pain; WCO = Wedge corrected orthostasis.
Footnotes
1Bioengineering and Biomedicine Company Srl Pescara-Italy.
2NaturalPoint Inc. Corvallis, OR-USA.
3Zebris Gmbh Isny-Germany.
References
Abraham, W. D., and Dimon, J. H. (1992). Leg Length Discrepancy in Total Hip Arthroplasty. Orthop. Clin. North Am. 23, 201–209. doi:10.1016/s0030-5898(20)31731-4
Ashour, R., Abdelraouf, O., Abdallah, A., and Sweif, R. (2019). Effect of Footwear Modification on Postural Symmetry and Body Balance in Leg Length Discrepancy: A Randomized Controlled Study. Int. J. Osteopathic Med. 32, 13–20. doi:10.1016/j.ijosm.2019.02.001
Beattie, P., Isaacson, K., Riddle, D. L., and Rothstein, J. M. (1990). Validity of Derived Measurements of Leg-Length Differences Obtained by Use of a Tape Measure. Phys. Ther. 70, 150–157. doi:10.1093/ptj/70.3.150
Beaudoin, L., Zabjek, K. F., Leroux, M. A., Coillard, C., and Rivard, C. H. (1999). Acute Systematic and Variable Postural Adaptations Induced by an Orthopaedic Shoe Lift in Control Subjects. Eur. Spine J. 8, 40–45. doi:10.1007/s005860050125
Betsch, M., Wild, M., Große, B., Rapp, W., and Horstmann, T. (2012). The Effect of Simulating Leg Length Inequality on Spinal Posture and Pelvic Position: a Dynamic Rasterstereographic Analysis. Eur. Spine J. 21, 691–697. doi:10.1007/s00586-011-1912-5
Betsch, M., Rapp, W., Przibylla, A., Jungbluth, P., Hakimi, M., Schneppendahl, J., et al. (2013). Determination of the Amount of Leg Length Inequality that Alters Spinal Posture in Healthy Subjects Using Rasterstereography. Eur. Spine J. 22, 1354–1361. doi:10.1007/s00586-013-2720-x
Blake, R., and Ferguson, H. (1992). Limb Length Discrepancies. J. Am. Podiatr Med. Assoc. 82, 33–38. doi:10.7547/87507315-82-1-33
Brady, R. J., Dean, J. B., Skinner, T. M., and Gross, M. T. (2003). Limb Length Inequality: Clinical Implications for Assessment and Intervention. J. Orthop. Sports Phys. Ther. 33, 221. doi:10.2519/jospt.2003.33.5.221
Bratton, R. L. (1999). Assessment and Management of Acute Low Back Pain. Am. Fam. Physician 60, 2299–2308.
Cambron, J. A., Dexheimer, J. M., Duarte, M., and Freels, S. (2017). Shoe Orthotics for the Treatment of Chronic Low Back Pain: A Randomized Controlled Trial. Arch. Phys. Med. Rehabil. 98, 1752–1762. doi:10.1016/j.apmr.2017.03.028
Campbell, T. M., Ghaedi, B. B., Tanjong Ghogomu, E., and Welch, V. (2018). Shoe Lifts for Leg Length Discrepancy in Adults with Common Painful Musculoskeletal Conditions: A Systematic Review of the Literature. Arch. Phys. Med. Rehabil. 99, 981–993. doi:10.1016/j.apmr.2017.10.027
Chuter, V., Spink, M., Searle, A., and Ho, A. (2014). The Effectiveness of Shoe Insoles for the Prevention and Treatment of Low Back Pain: a Systematic Review and Meta-Analysis of Randomised Controlled Trials. BMC Musculoskelet. Disord. 15, 140. doi:10.1186/1471-2474-15-140
Cleveland, R. H., Kushner, D. C., Ogden, M. C., Herman, T. E., Kermond, W., and Correia, J. A. (1988). Determination of Leg Length Discrepancy. A Comparison of Weight-Bearing and Supine Imaging. Invest. Radiol. 23, 301. doi:10.1097/00004424-198804000-00010
Cooperstein, R. (2010). The Relationship between Pelvic Torsion and Anatomical Leg Length Inequality: a Review of the Literature. J. Chiropr. Med. 9, 96–97. doi:10.1016/j.jcm.2010.04.002
D’Amico, M., D’Amico, G., and Roncoletta, P. (1995a). Algorithm for Estimation, Classification and Graphical Representation of Clinical Parameters in the Measurement of Scoliosis and Spinal Deformities by Means of Non-ionising Device. Three Dimensional Anal. Spinal Deformities 15, 33–38. doi:10.3233/978-1-60750-859-5-33
D’Amico, M., Grillet, C., Mariotti, S., and Roncoletta, P. (1995b). Functional Evaluation of the Spine through the Analysis of Lateral Bending Test Kinematics by Mean of Non-ionising Technique. Three Dimensional Anal. Spinal Deformities 15, 197–202. doi:10.3233/978-1-60750-859-5-197
D’Amico, M., D’Amico, G., Roncoletta, P., Tomassini, M., Ciarrocca, F., and Vallasciani, M. (2007). A 3-D Biomechanical Skeleton Model and Processing Procedure. Eur. Med. Phys. 43, 1–6.
D’Amico, M., D’Amico, G., Frascarello, M., Paniccia, M., Roncoletta, P., and Vallasciani, M. (2008). A 3-D Skeleton Model & SEMG Approach for Integrated Neck and Low Back Pain Analysis Test Batteries. Stud. Health Technol. Inform. 140, 79–84.
D'Amico, M., Roncoletta, P., Di Felice, F., Porto, D., Bellomo, R., and Saggini, R. (2012). LBP and Lower Limb Discrepancy: 3D Evaluation of Postural Rebalancing via Underfoot Wedge Correction. Stud. Health Technol. Inform. 176, 108–112. doi:10.3233/978-1-61499-067-3-146
D’Amico, M., Kinel, E., D’Amico, G., and Roncoletta, P. (2017a). “A 3D Spine and Full Skeleton Model for Opto-Electronic Stereo- Photogrammetric Multi-Sensor Biomechanical Analysis in Posture and Gait,” in Innovations in Spinal Deformities and Postural Disorders (Rijeka, Croatia: INTECH). doi:10.5772/intechopen.68633
D'Amico, M., Kinel, E., and Roncoletta, P. (2017b). Normative 3D Opto-Electronic Stereo-Photogrammetric Posture and Spine Morphology Data in Young Healthy Adult Population. PLOS ONE 12, e0179619. doi:10.1371/journal.pone.0179619
D'Amico, M., Kinel, E., and Roncoletta, P. (2018a). 3D Quantitative Evaluation of Spine Proprioceptive Perception/motor Control through Instinctive Self-Correction Maneuver in Healthy Young Subjects' Posture: an Observational Study. Eur. J. Phys. Rehabil. Med. 54, 428. doi:10.23736/S1973-9087.17.04738-4
D’Amico, M., Kinel, E., Roncoletta, P., and D’Amico, G. (2018b). ASAP Posture. Available at: https://www.protocols.io/view/asap-posture-q5zdy76 (Accessed June 22, 2018).
D’Amico, M., Kinel, E., D’Amico, G., and Roncoletta, P. (2021a). A Self-Contained 3D Biomechanical Analysis Lab for Complete Automatic Spine and Full Skeleton Assessment of Posture, Gait and Run. Sensors 21, 3930. doi:10.3390/s21113930
D’Amico, M., Kinel, E., Roncoletta, P., Gnaldi, A., Ceppitelli, C., Belli, F., et al. (2021b). Data-driven CAD-CAM vs Traditional Total Contact Custom Insoles: A Novel Quantitative-Statistical Framework for the Evaluation of Insoles Offloading Performance in Diabetic Foot. PLOS ONE 16, e0247915. doi:10.1371/journal.pone.0247915
D’Amico, M. (2002). Scoliosis and Leg Asymmetries: a Reliable Approach to Assess Wedge Solutions Efficacy. Stud. Health Technol. Inform. 88, 285–289.
de Leva, P. (1996). Joint center Longitudinal Positions Computed from a Selected Subset of Chandler's Data. J. Biomech. 29, 1231–1233. doi:10.1016/0021-9290(96)00021-8
Defrin, R., Benyamin, S. B., Aldubi, R. D., and Pick, C. G. (2005). Conservative Correction of Leg-Length Discrepancies of 10mm or Less for the Relief of Chronic Low Back Pain. Arch. Phys. Med. Rehabil. 86, 2075–2080. doi:10.1016/j.apmr.2005.06.012
Eliks, M., Ostiak-Tomaszewska, W., Lisiński, P., and Koczewski, P. (2017). Does Structural Leg-Length Discrepancy Affect Postural Control? Preliminary Study. BMC Musculoskelet. Disord. 18, 346. doi:10.1186/s12891-017-1707-x
Faul, F., Erdfelder, E., Lang, A.-G., and Buchner, A. (2007). G*Power 3: A Flexible Statistical Power Analysis Program for the Social, Behavioral, and Biomedical Sciences. Behav. Res. Methods 39, 175–191. doi:10.3758/BF03193146
Friberg, O. (1982). Leg Length Asymmetry in Stress Fractures. A Clinical and Radiological Study. J. Sports Med. Phys. Fitness 22, 485–488.
Friberg, O. (1983). Clinical Symptoms and Biomechanics of Lumbar Spine and Hip Joint in Leg Length Inequality. Spine 8, 643–651. doi:10.1097/00007632-198309000-00010
Giles, L. G., and Taylor, J. (1976). Low-back Pain Associated with Leg Length Inequality. Spine (Phila Pa 1976) 6, 510. doi:10.1097/00007632-198109000-00014
Giles, L. G. F., and Taylor, J. R. (1982). Lumbar Spine Structural Changes Associated with Leg Length Inequality. Spine 7, 159–162. doi:10.1097/00007632-198203000-00011
Giles, L. G. (1981). Lumbosacral Facetal “Joint Angles” Associated with Leg Length Inequality. Rheumatol. Rehabil. 20, 233. doi:10.1093/rheumatology/20.4.233
Golightly, Y. M., Tate, J. J., Burns, C. B., and Gross, M. T. (2007). Changes in Pain and Disability Secondary to Shoe Lift Intervention in Subjects with Limb Length Inequality and Chronic Low Back Pain: a Preliminary Report. J. Orthop. Sports Phys. Ther. 37, 380–388. doi:10.2519/jospt.2007.2429
Gordon, J. E., and Davis, L. E. (2019). Leg Length Discrepancy: The Natural History (And what Do We Really Know). J. Pediatr. Orthop. 39, S10–S13. doi:10.1097/BPO.0000000000001396
Gross, R. H. (1978). Leg Length Discrepancy: How Much Is Too Much? Orthopedics 1, 307–310. doi:10.3928/0147-7447-19780701-08
Guichet, J. M., Spivak, J. M., Trouilloud, P., and Grammont, P. M. (1991). Lower Limb-Length Discrepancy. An Epidemiologic Study. Clin. Orthop. Relat. Res. 272, 235–241.
Gurney, B. (2002). Leg Length Discrepancy. Gait Posture 15, 195–206. doi:10.1016/s0966-6362(01)00148-5
Harvey, W. F., Yang, M., Cooke, T. D. V., Segal, N. A., Lane, N., Lewis, C. E., et al. (2010). Association of Leg-Length Inequality with Knee Osteoarthritis. Ann. Intern. Med. 152, 287–295. doi:10.7326/0003-4819-152-5-201003020-00006
Jensen, M. P., and Karoly, P. (2001). “Self-report Scales and Procedures for Assessing Pain in Adults,” in Handbook of Pain Assessment. 2nd ed (New York, NY, US: The Guilford Press), 15–34.
Kendall, J. C., Bird, A. R., and Azari, M. F. (2014). Foot Posture, Leg Length Discrepancy and Low Back Pain - Their Relationship and Clinical Management Using Foot Orthoses - an Overview. Foot 24, 75–80. doi:10.1016/j.foot.2014.03.004
Khamis, S., and Carmeli, E. (2018). The Effect of Simulated Leg Length Discrepancy on Lower Limb Biomechanics during Gait. Gait Posture 61, 73–80. doi:10.1016/j.gaitpost.2017.12.024
Kinel, E., D’Amico, M., and Roncoletta, P. (2018). Normative 3D Opto-Electronic Stereo-Photogrammetric Sagittal Alignment Parameters in a Young Healthy Adult Population. PLOS ONE 13, e0203679. doi:10.1371/journal.pone.0203679
Kinel, E., D’Amico, M., and Roncoletta, P. (2021). 3D Quantitative Evaluation of Posture and Spine Proprioceptive Perception through Instinctive Self-Correction Maneuver in Adolescent Idiopathic Scoliosis. Front. Bioeng. Biotechnol. 9, 663394. doi:10.3389/fbioe.2021.663394
Knutson, G. A. (2002). Incidence of Foot Rotation, Pelvic Crest Unleveling, and Supine Leg Length Alignment Asymmetry, and Their Relationship to Self-Reported Back. J. Manipulative Physiol. Ther. 25, 110E. doi:10.1067/mmt.2002.121414
Knutson, G. A. (2005a). Anatomic and Functional Leg-Length Inequality: A Review and Recommendation for Clinical Decision-Making. Part I, Anatomic Leg-Length Inequality: Prevalence, Magnitude, Effects and Clinical Significance. Chiropr. Man. Therap. 13, 11. doi:10.1186/1746-1340-13-11
Knutson, G. A. (2005b). Anatomic and Functional Leg-Length Inequality: A Review and Recommendation for Clinical Decision-Making. Part II, the Functional or Unloaded Leg-Length Asymmetry. Chiropr. Man. Therap. 13, 12. doi:10.1186/1746-1340-13-12
Kwon, Y.-J., Song, M., Baek, I.-H., and Lee, T. (2015). The Effect of Simulating a Leg-Length Discrepancy on Pelvic Position and Spinal Posture. J. Phys. Ther. Sci. 27, 689–691. doi:10.1589/jpts.27.689
Liu, Y. K., and Wickstrom, J. K. (1973). “Estimation of the Inertial Property Distribution of the Human Torso from Segmented Cadaveric Data,” in Perspectives in Biomedical Engineering. Editor R. M. Kenedi (London: Palgrave Macmillan UK), 203–213. doi:10.1007/978-1-349-01604-4_33
McCaw, S. T., and Bates, B. T. (1991). Biomechanical Implications of Mild Leg Length Inequality. Br. J. Sports Med. 25, 10–13. doi:10.1136/bjsm.25.1.10
Menez, C., Coquart, J., Dodelin, D., Tourny, C., and L'Hermette, M. (2021). Effects of Orthotic Insoles on Gait Kinematics and Low-Back Pain in Patients with Mild Leg Length Discrepancy. J. Am. Podiatr. Med. Assoc. 111, 1–10. doi:10.7547/18-093
Menez, C., L'Hermette, M., and Coquart, J. (2020). Orthotic Insoles Improve Gait Symmetry and Reduce Immediate Pain in Subjects with Mild Leg Length Discrepancy. Front. Sports Act. Living 2, 1–11. doi:10.3389/fspor.2020.579152
Murray, K. J., and Azari, M. F. (2015). Leg Length Discrepancy and Osteoarthritis in the Knee, Hip and Lumbar Spine. J. Can. Chiropr. Assoc. 59, 226–237. Available at: https://www.ncbi.nlm.nih.gov/pmc/articles/PMC4593034/(Accessed March 2, 2021).
Murray, K. J., Molyneux, T., Le Grande, M. R., Castro Mendez, A., Fuss, F. K., and Azari, M. F. (2017). Association of Mild Leg Length Discrepancy and Degenerative Changes in the Hip Joint and Lumbar Spine. J. Manipulative Physiol. Ther. 40, 320–329. doi:10.1016/j.jmpt.2017.03.001
Perttunene, J. R., Anttila, E., Södergård, J., Merikanto, J., and Komi, P. V. (2004). Gait Asymmetry in Patients with Limb Length Discrepancy. Scand. J. Med. Sci. Sports 14, 49. doi:10.1111/j.1600-0838.2003.00307.x
Raczkowski, J. W., Daniszewska, B., and Zolynski, K. (2010). Clinical Research Functional Scoliosis Caused by Leg Length Discrepancy. AOMS 3, 393–398. doi:10.5114/aoms.2010.14262
Rannisto, S., Okuloff, A., Uitti, J., Paananen, M., Rannisto, P.-H., Malmivaara, A., et al. (2015). Leg-length Discrepancy Is Associated with Low Back Pain Among Those Who Must Stand while Working. BMC Musculoskelet. Disord. 16, 110. doi:10.1186/s12891-015-0571-9
Rannisto, S., Okuloff, A., Uitti, J., Paananen, M., Rannisto, P.-H., Malmivaara, A., et al. (2019). Correction of Leg-Length Discrepancy Among Meat Cutters with Low Back Pain: a Randomized Controlled Trial. BMC Musculoskelet. Disord. 20, 105. doi:10.1186/s12891-019-2478-3
Reid, D. C., and Smith, B. (1984). Leg Length Inequality: a Review of Etiology and Management. Physio Can. 36, 177–182.
Rondon, C. A., González, N., Agreda, L., and Millán, A. (1992). Observer Agreement in the Measurement of Leg Length. Rev. Invest. Clin. 44, 85–89.
Rossvoll, I., Junk, S., and Terjesen, T. (1992). The Effect on Low Back Pain of Shortening Osteotomy for Leg Length Inequality. Int. Orthop. 16, 388–391. doi:10.1007/BF00189625
Rothschild, D., Ng, S. Y., and Ng, Y. L. E. (2020). Indications of Sole Lift and Foot Orthoses in the Management of Mild Idiopathic Scoliosis-A Review. J. Phys. Ther. Sci. 32, 251–256. doi:10.1589/jpts.32.251
Ruzbarsky, J. J., Goodbody, C., and Dodwell, E. (2017). Closing the Growth Plate: a Review of Indications and Surgical Options. Curr. Opin. Pediatr. 29, 80–86. doi:10.1097/MOP.0000000000000438
Sabharwal, S., and Kumar, A. (2008). Methods for Assessing Leg Length Discrepancy. Clin. Orthop. Relat. Res. 466, 2910–2922. doi:10.1007/s11999-008-0524-9
Seidel, G. K., Marchinda, D. M., Dijkers, M., and Soutas-Little, R. W. (1995). Hip Joint center Location from Palpable Bony Landmarks-A Cadaver Study. J. Biomech. 28, 995–998. doi:10.1016/0021-9290(94)00149-X
Sheha, E. D., Steinhaus, M. E., Kim, H. J., Cunningham, M. E., Fragomen, A. T., and Rozbruch, S. R. (2018). Leg-Length Discrepancy, Functional Scoliosis, and Low Back Pain. JBJS Rev. 6, e6. doi:10.2106/JBJS.RVW.17.00148
Stokes, I. A. F. (1994). Three-Dimensional Terminology of Spinal Deformity. Spine 19, 236–248. doi:10.1097/00007632-199401001-00020
Subotnick, S. I. (1981). Limb Length Discrepancies of the Lower Extremity (The Short Leg Syndrome). J. Orthop. Sports Phys. Ther. 3, 11–16. doi:10.2519/jospt.1981.3.1.11
Tallroth, K., Ylikoski, M., Lamminen, H., and Ruohonen, K. (2005). Preoperative Leg-Length Inequality and Hip Osteoarthrosis: a Radiographic Study of 100 Consecutive Arthroplasty Patients. Skeletal Radiol. 34, 136–139. doi:10.1007/s00256-004-0831-5
Tallroth, K., Ristolainen, L., and Manninen, M. (2017). Is a Long Leg a Risk for Hip or Knee Osteoarthritis? Acta Orthop. 88, 512–515. doi:10.1080/17453674.2017.1348066
ten Brinke, A., van der Aa, H. E., van der Palen, J., and Oosterveld, F. (1999). Is Leg Length Discrepancy Associated with the Side of Radiating Pain in Patients with a Lumbar Herniated Disc? Spine 24, 684–686. doi:10.1097/00007632-199904010-00013
Terry, M. A., Winell, J. J., Green, D. W., Schneider, R., Peterson, M., Marx, R. G., et al. (2005). Measurement Variance in Limb Length Discrepancy. J. Pediatr. Orthop. 25, 197–201. doi:10.1097/01.bpo.0000148496.97556.9f
Vogt, B., Gosheger, G., Wirth, T., Horn, J., and Rödl, R. (2020). Leg Length Discrepancy- Treatment Indications and Strategies. Dtsch Arztebl Int. 117, 405–411. doi:10.3238/arztebl.2020.0405
von Elm, E., Altman, D. G., Egger, M., Pocock, S. J., Gøtzsche, P. C., Vandenbroucke, J. P., et al. (2007). The Strengthening the Reporting of Observational Studies in Epidemiology (STROBE) Statement: Guidelines for Reporting Observational Studies. Plos Med. 4, e296. doi:10.1371/journal.pmed.0040296
White, S. C., Gilchrist, L. A., and Wilk, B. E. (2004). Asymmetric Limb Loading with True or Simulated Leg-Length Differences. Clin. Orthop. Relat. Res. 421, 287–292. doi:10.1097/01.blo.0000119460.33630.6d
Woerman, A. L., and Binder-Macleod, S. A. (1984). Leg Length Discrepancy Assessment: Accuracy and Precision in Five Clinical Methods of Evaluation. J. Orthop. Sports Phys. Ther. 5, 230–239. doi:10.2519/jospt.1984.5.5.230
Zatsiorsky, V., Seluyanov, V., and Chugunova, L. (1990). “In Vivo body Segment Inertial Parameters Determination Using a Gamma-Scanner Method,” in Biomechanics of Human Movement : Applications in Rehabilitation, Sports and Ergonomics. Editors N. Berme,, and A. Capozzo (Worthington, Ohio-USA: Bertec Corporation), 186–202.
Keywords: leg length discrepancy, nonspecific low back pain, stereophotogrammetry, posture, spine, customized orthotics
Citation: D’Amico M, Kinel E and Roncoletta P (2022) Leg Length Discrepancy and Nonspecific Low Back Pain: 3-D Stereophotogrammetric Quantitative Posture Evaluation Confirms Positive Effects of Customized Heel-Lift Orthotics. Front. Bioeng. Biotechnol. 9:743132. doi: 10.3389/fbioe.2021.743132
Received: 17 July 2021; Accepted: 23 December 2021;
Published: 10 February 2022.
Edited by:
Bernardo Innocenti, Université libre de Bruxelles, BelgiumReviewed by:
Chi-Wen Lung, Asia University, TaiwanSai Wei Yang, National YangMing ChiaoTung University, Taiwan
Copyright © 2022 D’Amico, Kinel and Roncoletta. This is an open-access article distributed under the terms of the Creative Commons Attribution License (CC BY). The use, distribution or reproduction in other forums is permitted, provided the original author(s) and the copyright owner(s) are credited and that the original publication in this journal is cited, in accordance with accepted academic practice. No use, distribution or reproduction is permitted which does not comply with these terms.
*Correspondence: Moreno D’Amico, damicomoreno@gmail.com
†These authors have contributed equally to this work and share first authorship