- School of Pharmaceutical Sciences (Shenzhen), Sun Yat-sen University, Shenzhen, China
Transplanting stem cells with the abilities of self-renewal and differentiation is one of the most effective ways to treat many diseases. In order to optimize the therapeutic effect of stem cell transplantation, it is necessary to intervene in stem cell differentiation. Inorganic nanomaterials (NMs), due to their unique physical and chemical properties, can affect the adhesion, migration, proliferation and differentiation of stem cells. In addition, inorganic NMs have huge specific surface area and modifiability that can be used as vectors to transport plasmids, proteins or small molecules to further interfere with the fate of stem cells. In this mini review, we summarized the recent advances of common inorganic NMs in regulating stem cells differentiation, and the effects of the stiffness, size and shape of inorganic NMs on stem cell behavior were discussed. In addition, we further analyzed the existing obstacles and corresponding perspectives of the application of inorganic NMs in the field of stem cells.
Introduction
Stem cells refer to cells with self-renewal and differentiation capacity, which can be roughly divided into embryonic stem cells and somatic stem cells. Embryonic stem cells are derived from blastocysts (Reubinoff et al., 2000). Typical somatic stem cells include mesenchymal stem cells (MSCs), neural stem cells (NSCs), hematopoietic stem cells (HSCs) and so on. Terminally differentiated somatic cells can be reprogrammed into induced pluripotent stem cells (iPSC) with embryonic stem cells (ESCs)-like properties by transfection of defined factors (Takahashi and Yamanaka, 2006), and further differentiate into different cell types. With the increasing of research on stem cells in recent years, more and more evidence is emerging that stem cell transplantation is one of the most effective methods to treat neurological diseases, bone injury and other diseases (Yang et al., 2018; Krukiewicz et al., 2020). The growth and differentiation of stem cells are easily affected by their surrounding matrix. Changing the size, hydrophilicity, roughness, and arrangement of the cell attachment surface can directly affect cell behavior (Zanden et al., 2014). To be able to fully realize the therapeutic potential of stem cells in the field of regenerative medicine, precise control of the fate of stem cells is one of the first issues to be addressed (Solanki et al., 2013b).
Inorganic NMs have been widely used in bioimaging, drug delivery, biosensing, photothermal therapy, and 3D printing due to their own excellent properties (Wu et al., 2018; Mashayekhi et al., 2020; Tavares et al., 2021). In recent years, inorganic NMs have been widely used to manipulate the fate of stem cells. Inorganic NMs exert their influence on stem cell behavior as unique biomolecules, besides that, as modifiable non-viral transfection vectors, inorganic NMs can carry various bioactive molecules that regulate stem cell behavior, including RNA, plasmids, proteins, or polypeptides, etc., thereby further stimulating the proliferation, migration, differentiation and paracrine behavior of stem cells. Various inorganic NMs, including graphene (Rostami et al., 2020), carbon dots (Shao et al., 2017), gold nanoparticles (AuNPs) (Heo et al., 2014), silver nanoparticles (AgNPs) (Wan et al., 2020), nano titanium-based alloys (Jalali et al., 2020), strontium nanoparticles (Hu et al., 2017), iron oxides nanoparticles (Zhang et al., 2020), manganese dioxide (MnO2) nanoparticles (Wang et al., 2017), silicon dioxide (SiO2) nanoparticles (Gandhimathi et al., 2019), and black phosphorus (BP) nanosheets (Xu et al., 2020), have been extensively explored in stem cells regeneration medicine. The stiffness, size and shape of inorganic NMs can directly affect the bioactivity of materials and, in turn, affect the differentiation of stem cells (Huang et al., 2020). In this paper, we summarized the progress of various inorganic NMs on the regulation of stem cells differentiation, and the physical properties of inorganic NMs in regulating stem cell differentiation were discussed. In addition, the obstacles to the application of inorganic NMs and the corresponding solutions were further analyzed.
Carbon-Based Nanomaterials
Carbon-based materials such as carbon nanotubes (CNTs) and graphene have good physical properties, stability and biocompatibility, which can maintain the adhesion and proliferation of stem cells and can influence the differentiation fate of stem cells (Lee et al., 2011; Shao et al., 2018; Gupta et al., 2019). But different types of carbon-based materials show different effects on stem cells. Compared with graphene, iPSCs are more likely to grow on the surface of graphene oxide (GO) because the oxygen-containing functional groups of GO greatly improve the surface hydrophilicity, which is conducive to cell adhesion, growth and differentiation (Liu et al., 2011; Feng et al., 2018; Yang et al., 2018) compared the effects of CNTs, GO, and graphene on dopamine neural differentiation of mESCs. Only GO was found to significantly enhance the differentiation of ESCs into dopaminergic neurons (Yang et al., 2014a). GO can improve biological activity during biomineralization and promote osteoblast adhesion (Krukiewicz et al., 2020). Go can also promote the attachment and proliferation of MSCs, which promotes spontaneous and stimulated osteogenic differentiation (Rostami et al., 2020). In addition, carbonaceous nanomaterials called fullerenes are a potential material for inducing osteogenic differentiation of MSCs (Yang et al., 2014b). Gadofullerene nanoparticles effectively reduce reactive oxygen species (ROS) levels in blood and promote erythrocyte maturation (Jia et al., 2020).
Being able to simultaneously monitor and direct stem cell differentiation is important for the application of stem cells. Kim et al. (2013a) reported a non-invasive and rapid electrochemical method to detect the differentiated state of NSCs using 3D GO encapsulated AuNPs based on the feature that there are many C=C unsaturated groups on the surface of NSCs, while the unsaturated groups significantly decrease after differentiation. Shao et al. (2017) used citric acid-based carbon dots (CDs) to label rat bone marrow mesenchymal stem cells (BMSCs) without affecting cell viability to provide real-time monitoring of cell activities. Meanwhile, the presence of CDs could enhance the osteogenic differentiation efficiency of rBMSCs by promoting matrix mineralization and up-regulating the expression of osteoblast gene markers alkaline phosphate (ALP), runt related transcription factor 2, osteocalcin and bone sialoprotein. Similarly, (Meng et al., 2019; Yang et al., 2019), synthesized Mg2+-doped CDs and Zn2+-doped CDs, which were internalized by cells as a biomarker and simultaneously promoted the osteogenic differentiation of mouse embryo osteoblast precursor cells (MC3T3-E1) by increasing their ALP activity.
Metal-Based Nanomaterials
Gold Nanoparticles
AuNPs possess good biocompatibility and can serve as an ideal alternative material to promote bone tissue regeneration. AuNPs significantly promote osteogenic differentiation and mineral deposition in MSCs (Suarasan et al., 2015; Mahmoud et al., 2020). In vivo experiments have shown that AuNPs can promote bone regeneration at bone defect sites and play a positive role in bone healing (Heo et al., 2014).
AuNPs as vehicles also play an important role in the applications of stem cells. AuNPs deliver basic fibroblast growth factor and bone morphogenetic protein-2 (BMP2) to promote osteogenic differentiation of MSCs (Qi et al., 2017). Patel et al. (2014) constructed a mimic transcription factor NanoScript based on AuNPs, which could achieve nuclear localization and initiate the transcriptional activity of both reporter plasmids and endogenous genes, and successfully initiate selective differentiation of adipose derived mesenchymal stem cells (ADMSCs) into myoblasts (Patel et al., 2015c). Next, they designed NanoScript to depress the expression of SOX9 gene in NSCs and promote the formation of functional neurons (Patel et al., 2015a). After that, they modified the NanoScript which specifically enhanced SOX9 gene expression with a small molecule to enhance the chondrogenic differentiation of ADMSCs by increasing the activity of histone acetyltransferases (Patel et al., 2015b). Wu et al. (2020) developed multifunctional AuNPs to control and detect osteogenic differentiation of hMSCs in real time, giving AuNPs multiple applications in stem cell regenerative medicine.
Silver Nanoparticles
AgNPs have promising anti-inflammatory and antimicrobial activities (Hebeish et al., 2014; Xia et al., 2020). Topical coating facilitates the healing of wounds (Tian et al., 2007). Implanting stem cells into dressings containing AgNPs also promotes cell growth and wound healing (Gao et al., 2020). Silver nanomultilayers decorated on the surface of titanium alloy implants enhance osteogenic differentiation of rBMSCs (Wan et al., 2020). Therefore, AgNPs which possess both antibacterial and osteogenic differentiation abilities are potential biomaterials for treating infectious bone defects (Li et al., 2020a). AgNPs induce osteogenic differentiation independent of Ag+ (Qin et al., 2014), but associated with the increase of intracellular ROS (Chowdhury et al., 2018; Dayem et al., 2018).
Nano Titanium Alloys
Titanium (Ti)-based alloys are a common type of bone implants. Inert Ti alloy implants can quickly form TiO2 film on the surface in vivo, and the film can be recovered in a short time after disruption. Therefore, Ti alloy implants have good biocompatibility (Zhang and Chen, 2019).TiO2 films enhance the osseointegrative properties of the orthopedic implant material (Blendinger et al., 2021). Ti-based materials such as Ti-modified TiO2 nanotubes can promote the adhesion, proliferation and osteogenic differentiation of MSCs, and in vivo transplantation can improve osteoporosis (Yu et al., 2017). At the same time, they promote the adhesion of macrophages and the transformation of M1-to-M2, which induces a favorable immune microenvironment for bone fusion (Yang et al., 2020). TiO2 nanotubes can promote F-actin polymerization and osteogenic differentiation in BMSCs (Liu et al., 2021). Apart from that, TiO2 nanotubes can target drugs to bone and enhance osteoblast differentiation (Hashemi et al., 2020; Tong et al., 2020).
Strontium Nanoparticles
Strontium (Sr) has biological effects to promote osteogenesis, and moderate supplementation of Sr enhances calcium absorption (Nielsen, 2004). Zhang et al. (2013) loaded Sr on TiO2 nanotubes to obtain Crystalline SrTiO3, which can realize the slow release of Sr. It further enhances the ALP activity and matrix mineralization ability of MSCs. SrTiO3 nanotube arrays have good biocompatibility and are ideal implants for osteoporotic bone (Xin et al., 2009). Meanwhile Sr can depress the activity of osteoclasts, which greatly improves the osteogenesis (Hu et al., 2017). In addition, Sr also promotes M2 type polarization of macrophages and reduces proinflammatory factor to create a favorable environment for bone healing (Li et al., 2018).
Iron and its Oxides-Based Nanoparticles
Fe3O4 nanoparticles (Fe3O4 NPs) are typical magnetic materials for promoting bone tissue regeneration (Zhang et al., 2020). Fe3O4 NPs composite scaffolds could enhance adhesion, proliferation and osteoconduction of hMSCs (Bock et al., 2010; Kim et al., 2020b). Magnetite-modified scaffolds facilitate the adhesion and proliferation of cells, which in turn promotes osteogenic differentiation of MSCs and osteogenesis in vivo (Lee et al., 2019b; Pistone et al., 2019; Xia et al., 2019). (Li et al., 2020c) treated hMSCs with Fe3O4 NPs and obtained exosomes labeled with Fe3O4 NPs, which significantly promoted proliferation, migration, and angiogenesis of human umbilical vein endothelial cells (hUVECs) in the skin injury model.
Manganese Dioxide-Based Nanoparticles
One of the most important applications of MnO2 in stem cell regenerative medicine is bio-imaging, in which MRI is used to track the distribution of stem cells in vivo (Yang et al., 2018). MnO2 nanotubes are reduced to Mn2+ in acidic solution or by intracellular glutathione and further activate magnetic resonance imaging (MRI) (Lu et al., 2017; Wu et al., 2018). Mn2+ can promote neural differentiation and neurite growth of rat pheochromocytoma cells (PC12). Moreover, the ππ stacking interaction affects the charge-carrier density between catecholamines and MnO2 nanoellipsoids through which the catecholamines secreted by PC12 cells can be monitored in real time (Kim et al., 2013a).
MnO2 as an antioxidant can alleviate the oxidative environment of injured tissues. Modification of MnO2 on the surface of MSCs can improve cell survival in oxidative disease tissue while secreting more proangiogenic factors (Teo et al., 2019). MnO2-modified hydrogel significantly reduces the ROS at the site of spinal cord transection injury in rats and promotes the neural differentiation of the implanted MSCs (Li et al., 2019). Based on the large specific surface area and molecular characteristics, (Yang et al., 2018), used MnO2 3D nanoscaffolds to deliver hNSCs and small molecules to the spinal cord injury site in mice, improving cell survival and facilitating repair. BMP2 loaded MnO2 nanoparticles can enhance the recruitment of skeletal stem cells to promote bone repair (Li et al., 2020b).
Non-Metallic Nanomaterials
Silicon Dioxide Nanoparticles
Silicon is one of the essential mineral elements in human body, which plays an important role in the formation and maintenance of human bones. Soluble silicon dioxide plays a dual role in bone metabolism. On the one hand, it can promote osteoblasts. On the other hand, it can inhibit the formation of osteoclasts and bone resorption (Mladenovic et al., 2014). Therefore silicon holds great promise for osteoporosis therapy (Price et al., 2013). SiO2 can enhance the hydrophilicity of nanofibers and favor the adhesion and growth of MSCs, which in turn promote osteogenic differentiation (Gandhimathi et al., 2019).
Mesoporous silica nanoparticles (MSNs), with excellent adsorption properties, stability and biocompatibility, are often used as vehicles for various active molecules to direct the fate of stem cells (Mashayekhi et al., 2020). BMP2 delivery by MSNs can promote the differentiation of MSCs towards osteoblasts (Zhou et al., 2015). Solanki et al. (2013a) developed a SiO2 nanoparticle-mediated reverse uptake platform that delivered siRNA to depress SOX9 expression and enable NSCs to differentiate into neurons. Tavares et al. (2021) used functionalized MSNs as inorganic bone building blocks of multi-bioactive nanocomposite bio-ink, and used 3D bio-printing technology to generate biomaterials containing MSCs, which opened up great potential for bone tissue engineering to fabricate living 3D structures.
Black Phosphorus Nanomaterials
Black phosphorus, as a new type of semiconductor material, possesses good optical and electrical properties. In addition, BPNMs have many advantages such as large specific surface area, high photothermal conversion efficiency, good biocompatibility and biodegradability, which are widely used in biological fields (Yin et al., 2017). BPNMs are not only widely used in photothermal therapy of tumors (Luo et al., 2020), (Chen et al., 2018) also reported neuroprotective effects of BPNMs. BP hydrogel scaffolds combined with electrical stimulation can significantly promote the transformation of BMSCs into neuro-like cells (Xu et al., 2020). In addition, BPNMs can promote the proliferation, migration and osteogenic differentiation of stem cells and is widely used in bone repair (Lee et al., 2019a; Raucci et al., 2019). BP degradation produces phosphate ions. Hydrogels encapsulated with BPNMs can capture free calcium ions in vivo to form calcium salts, thus accelerating the biomineralization of bone defects and enhancing bone regeneration (Huang et al., 2019). BP hydrogel promotes osteogenic differentiation of hMSCs in vitro and shows the fastest rate of bone formation when transplanted into a rat model of skull defects (Miao et al., 2019). Pan et al. (2020) synthesized a chitosan thermosensitive hydrogel containing BPNMs for rheumatoid arthritis treatment. BP can eliminate the hyperplastic synovial tissue under the irradiation of near-infrared light, so as to relieve inflammation. Meanwhile, BPNMs continue to degrade in situ and release phosphate ions to realize the mineralization of calcium for bone regeneration.
Physical Properties of Inorganic Nanomaterials Modulate Stem Cell Differentiation
Stiffness
The stiffness and elasticity of the extracellular matrix (ECM) determine the differentiation fate of stem cells (Trappmann et al., 2012). Modulating the stiffness of nanomaterials can direct the fate of stem cells. Neural crest stem cells differentiate into smooth muscle cells around stiff substrates and into glial cells in softer matrix (Zhu et al., 2019). Similarly, MSCs mainly differentiate toward neurons in the soft matrix similar to nerve tissue, toward myocytes in the medium hardness matrix, and toward osteoblasts in the high hardness matrix (Engler et al., 2006). Wang et al. (2020) enhanced the efficiency of osteogenic differentiation by improving the hardness of gradient nanostructured Ti materials.
Shape
The microstructure of the cellular matrix greatly influences the growth and differentiation of cells. Solanki et al. (2010) found that the arrangement of the ECM affects the differentiation of NSCs, which are more likely to differentiate into neurons on grid-shaped ECM than strip or square. Later, they proved that the nanotopographic cues of the carbon nanotube network could cooperatively induce the selective growth of hNSCs (Park et al., 2011). Nanostructure modification on the surface of titanium grafts can promote osseointegration (Souza et al., 2019). Zhao et al. (2010) found that titanium microstructure surface can promote osteogenesis-related gene expression in osteoblasts, but has a down-regulation trend in cell proliferation, total protein formation, ALP activity and cell matrix mineralization. However, the addition of nanostructures on the surface of titanium microstructures significantly promotes osteogenic differentiation. At the same time, there is no significant difference between the total protein content and ALP activity on the nanostructured surface and the smooth surface, but the micro/nano surface features significantly increase the expression of both. In vivo transplantation can also promote new bone formation and osseointegration at the femoral defect (Zhang et al., 2013; Li et al., 2016a; Yuan et al., 2018). These results indicate that the micro/nano structure has a synergistic effect on promoting bone regeneration.
Size
There have been many studies reporting the effect of the size of nanomaterials on stem cell behavior. Stem cells exhibit different adhesion, proliferation, migration and environmental stress responses on different sizes of nanostructured substrates (Kim et al., 2020a). The osteoinduction activity of AuNPs at 20 nm is higher than that at 40 nm (Li et al., 2016b). However, (Li et al., 2017), reported that AuNPs below 10 nm significantly decreased osteogenesis-related gene expression in BMSCs, but increased the expression of genes related to adipogenesis and the formation of oil droplets. Zhang et al. (2015) demonstrated that AgNPs with an average diameter of 10 ± 5 nm could promote the proliferation and osteogenic differentiation of mMSCs in vitro. However, AgNPs with a mean diameter of 43 ± 11 nm could promote adipogenic differentiation of hMSCs and inhibit osteogenic differentiation in the early stage of differentiation (He et al., 2016). So, the size of AgNPs has a significant effect on the differentiation of MSCs. Shen et al. (2015) demonstrated that Ti nanoparticles with large particles (80 nm) greatly promoted the proliferation and differentiation of MSCs compared with other small particles (20 and 40 nm). The ability of TiO2 to promote osteogenic differentiation of MSCs is enhanced with the increase of nanotube diameter in a certain range (74–148 nm) (Tong et al., 2020). In contrast, when compared with 100 nm, TiO2 nanotubes with a size of 15 nm were reported by (Park et al., 2009) to be the best for both mMSCs and HSCs to promote adhesion, proliferation and differentiation. These conflicting conclusions led us to be cautious when looking at the size issue, and further determine the optimal size in combination with the preparation process of nanomaterials and cell types. In addition, the size of nanomaterials is often closely related to biological toxicity, which should not be ignored.
Taken together, a variety of inorganic NMs are capable of intervening in the differentiation of stem cells and are used for the treatment of specific diseases. Meanwhile, the physicochemical characteristics including stiffness, shape and size of inorganic NMs greatly influence their differentiation guidance (see Figure 1).
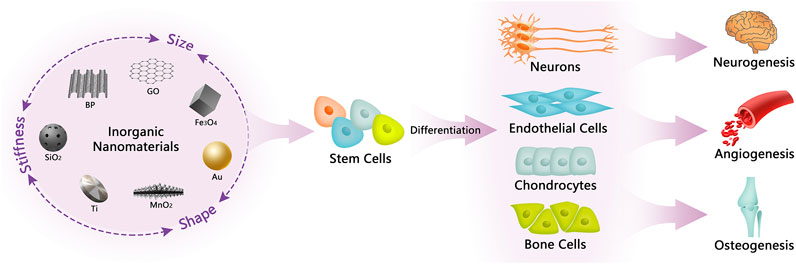
FIGURE 1. Illustration of inorganic NMs in regulating stem cell differentiation and their biological applications. The physical properties of inorganic NMs, including stiffness, shape, and size, can influence the guidance of inorganic NMs to the fate of stem cells, which in turn mediate the differentiation of stem cells to produce different kinds of functional cells that are beneficial for neurogenesis, angiogenesis, and osteogenesis.
Obstacles and Solutions for the Application of Inorganic Nanomaterials
We searched the literatures through the PubMed website using “stem cells and nanomaterials” as keywords in the past 5 years. A total of 3,526 results were displayed, and only two remained after adjusting article type to clinical trial, both about nanocurcumin. In addition, our keywords search for “nanomaterials and nanoparticles” in the ClinicalTrials.gov database yielded only four results and none of the clinical studies were related to stem cells, so we can see the extremely low clinical translation of nanomaterials. Combined with the analysis of the current published papers on the effects of inorganic NMs on stem cell differentiation (see Table 1), it is not difficult to find that the research methods and contents are monotonous and repetitive. Most of the papers just simply characterize some differentiation markers in vitro and in vivo. Articles usually emphasize that inorganic NMs possess good biocompatibility. However, these experiments only carry out short-term tests in vivo and in vitro, and even do not detect the distribution of nanoparticles in various organs in the body, which is far from the actual clinical application. In addition, due to the profound differences in anatomy, physiology and genetics between humans and animals (Su et al., 2018), successful animal experiments will not necessarily be replicated in humans. The safety problem is one of the important reasons for the failure of clinical translation (Arrowsmith, 2011). In the absence of complete safety data, clinical failure of nanomaterials is inevitable. As for the research strategies of nanomedicine, (Su et al., 2018), put forward critical and balanced suggestions, including multi-field cooperation, encouraging research to focus more on the biosafety of nanoparticles rather than the efficacy, and establishing standardized evaluation methods.
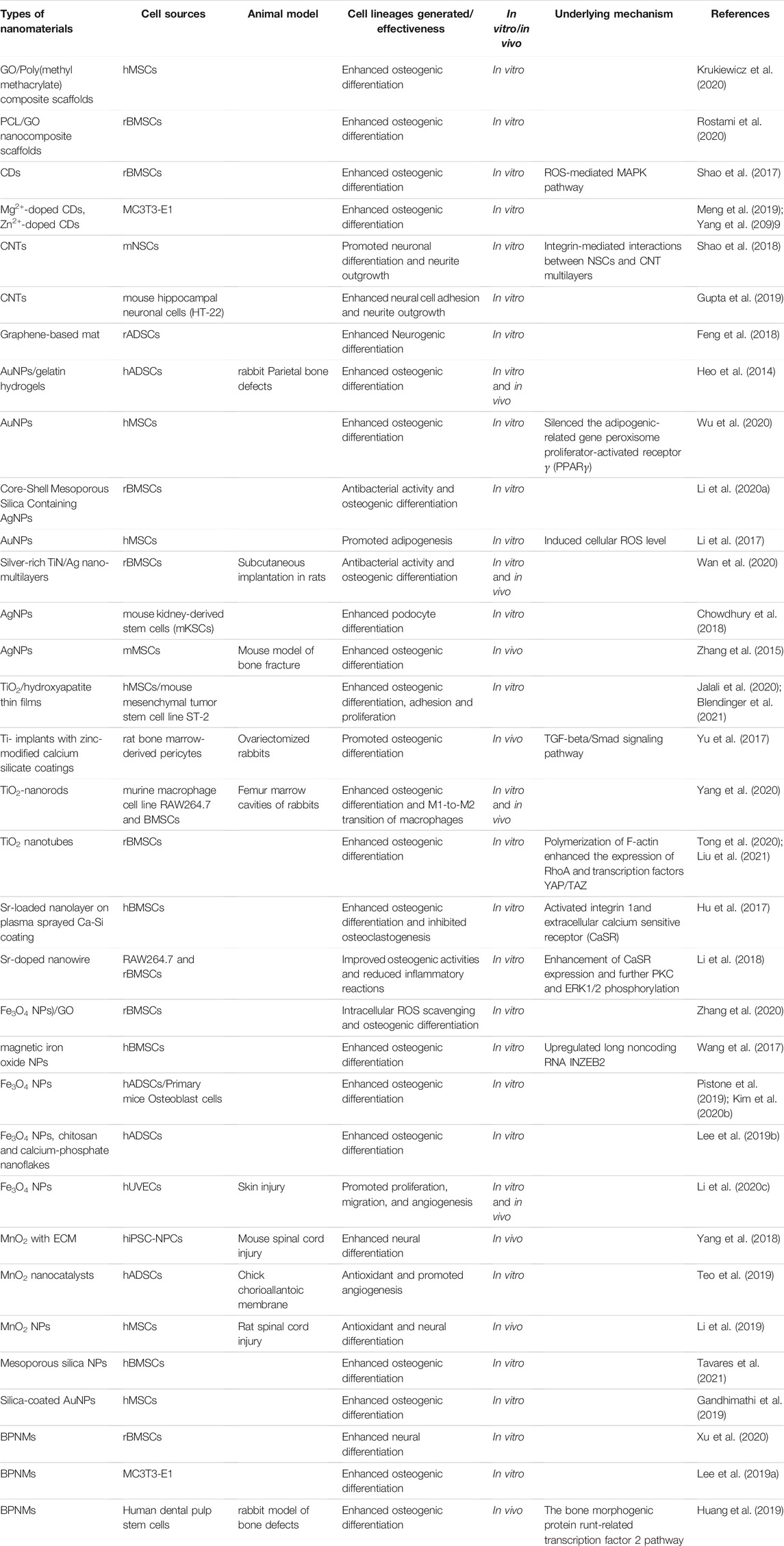
TABLE 1. A summary of inorganic nanomaterials guiding stem cell differentiation and its application in regenerative medicine.
Previous studies have suggested that the efficacy of stem cell transplantation depends on the differentiation into specific cell types, but there is growing evidence that the efficacy depends on paracrine behavior, which produces neuroprotective, angiogenesis, and immunomodulatory effects through the secretion of a large number of cytokines and proteins. MnO2 nanoparticles increase the secretion level of pro-angiogenic factors in MSCs (Teo et al., 2019). Li et al. (2020c) reported that exosomes produced by Fe3O4 NPs-treated hMSCs significantly promoted the angiogenesis of hUVECs. In addition, cell-derived nanoparticles have emerged as a promising alternative to synthetic nanocarriers for safer clinical outcomes (Chakravarti et al., 2020). Exosom-based therapies can effectively circumvent the toxicity of nanomaterials and the immune rejection problems associated with cell transplantation, with a broader application prospect in the field of regenerative medicine.
Conclusion and Perspectives
Stem cell transplantation has enabled the cure of many diseases. Based on previous studies, we know that the unique physicochemical characteristics of inorganic NMs greatly influence stem cell fate (Shao et al., 2018; Hashemi et al., 2020), and the combination of inorganic NMs and stem cells provides new insights into the treatment of several diseases, such as bone injury and neurological disorders (Gandhimathi et al., 2019; Zhang et al., 2019). Inorganic NMs, as vehicles, can effectively deliver soluble factors such as growth factors and cytokines to induce stem cell differentiation, and can also interfere stem cell survival, homing and paracrine behaviors by forming specific patterns with fibrous/hydrogel scaffolds (Qi et al., 2017; Zhang et al., 2019).
Biosafety issues are one of the main reasons for the low clinical translation efficiency of inorganic NMs in the field of regenerative medicine, so we should also perform more comprehensive and systematic studies on the biosafety of inorganic NMs, which are not limited to superficial cytotoxicity tests, but should pay more attention to the in vivo distribution, visceral toxicity, as well as metabolic pathways of the NMs. Next, HSCs are known to play an important role in the field of regenerative medicine. But there are few publications related to inorganic NMs’ role in HSCs (England et al., 2013; Bari et al., 2015). Based on the advantages of inorganic NMs, it is significant to explore the effect of inorganic NMs on the fate of HSCs and the derived therapeutic effect. Furthermore, most of the researches on inorganic NMs focus on the differentiation of stem cells into terminal functional cells, such as bone cells or neurons (Shao et al., 2018; Tong et al., 2020). The limited efficacy is accompanied by safety problems. Therefore, it is more promising to turn the research hotspot to the exosomes secreted by stem cells stimulated by inorganic NMs. Exosomes are rich in active molecules while having lower toxicity and can be used for the treatment of more diseases.
In conclusion, inorganic NMs enrich the applications of stem cells, and there are still many problems to be solved, but nanomaterials combined with stem cell therapy is promising and will lead to major breakthroughs in the near future.
Author Contributions
FH, GL, and WD were responsible for the conception and design of the review and revised the article. FH drafted the article. JC drew the diagram. JQ and ZL revised the article. All authors contributed to the article and approved the submitted version.
Funding
This work was supported by the grants from Shenzhen Science and Technology Program (Grant No. KQTD20190929173853397), Science, Technology and Innovation Commission of Shenzhen Municipality (Grant No. JCYJ20180307154606793), National Natural Science Foundation of China (Grant Nos. 81772449 and 81971081).
Conflict of Interest
The authors declare that the research was conducted in the absence of any commercial or financial relationships that could be construed as a potential conflict of interest.
Publisher’s Note
All claims expressed in this article are solely those of the authors and do not necessarily represent those of their affiliated organizations, or those of the publisher, the editors and the reviewers. Any product that may be evaluated in this article, or claim that may be made by its manufacturer, is not guaranteed or endorsed by the publisher.
References
Abdal Dayem, A., Lee, S., Choi, H., and Cho, S.-G. (2018). Silver Nanoparticles: Two-Faced Neuronal Differentiation-Inducing Material in Neuroblastoma (SH-Sy5y) Cells. Int. J. Mol. Sci. 19, 1470. doi:10.3390/ijms19051470
Arrowsmith, J. (2011). Phase III and Submission Failures: 2007-2010. Nat. Rev. Drug Discov. 10, 87. doi:10.1038/nrd3375
Bari, S., Chu, P. P. Y., Lim, A., Fan, X., Bunte, R. M., Li, S., et al. (2015). Mitochondrial Superoxide Reduction and Cytokine Secretion Skewing by Carbon Nanotube Scaffolds Enhance Ex Vivo Expansion of Human Cord Blood Hematopoietic Progenitors. Nanomedicine: Nanotechnology, Biol. Med. 11, 1643–1656. doi:10.1016/j.nano.2015.06.005
Blendinger, F., Seitz, D., Ottenschläger, A., Fleischer, M., and Bucher, V. (2021). Atomic Layer Deposition of Bioactive TiO2 Thin Films on Polyetheretherketone for Orthopedic Implants. ACS Appl. Mater. Inter. 13, 3536–3546. doi:10.1021/acsami.0c17990
Bock, N., Riminucci, A., Dionigi, C., Russo, A., Tampieri, A., Landi, E., et al. (2010). A Novel Route in Bone Tissue Engineering: Magnetic Biomimetic Scaffolds. Acta Biomater. 6, 786–796. doi:10.1016/j.actbio.2009.09.017
Chakravarti, A. R., Pacelli, S., and Paul, A. (2020). Investigation of Human Adipose Stem Cell-Derived Nanoparticles as a Biomimetic Carrier for Intracellular Drug Delivery. Nanoscale 12, 24273–24284. doi:10.1039/d0nr06571d
Chen, W., Ouyang, J., Yi, X., Xu, Y., Niu, C., Zhang, W., et al. (2018). Black Phosphorus Nanosheets as a Neuroprotective Nanomedicine for Neurodegenerative Disorder Therapy. Adv. Mater. 30, 1703458. doi:10.1002/adma.201703458
England, T. J., Bath, P. M. W., Abaei, M., Auer, D., and Jones, D. R. E. (2013). Hematopoietic Stem Cell (CD34+) Uptake of Superparamagnetic Iron Oxide Is Enhanced by but Not Dependent on a Transfection Agent. Cytotherapy 15, 384–390. doi:10.1016/j.jcyt.2012.10.016
Engler, A. J., Sen, S., Sweeney, H. L., and Discher, D. E. (2006). Matrix Elasticity Directs Stem Cell Lineage Specification. Cell 126, 677–689. doi:10.1016/j.cell.2006.06.044
Feng, Z.-Q., Yan, K., Shi, C., Xu, X., Wang, T., Li, R., et al. (2018). Neurogenic Differentiation of Adipose Derived Stem Cells on Graphene-Based Mat. Mater. Sci. Eng. C 90, 685–692. doi:10.1016/j.msec.2018.05.019
Gandhimathi, C., Quek, Y. J., Ezhilarasu, H., Ramakrishna, S., Bay, B. H., and Srinivasan, D. K. (2019). Osteogenic Differentiation of Mesenchymal Stem Cells with Silica-Coated Gold Nanoparticles for Bone Tissue Engineering. Int. J. Mol. Sci. 20, 5135. doi:10.3390/ijms20205135
Gao, T., Tian, C., Ma, Z., Chu, Z., Wang, Z., and Zhang, P. (2020). Stem Cell Seeded and Silver Nanoparticles Loaded Bilayer PLGA/PVA Dressings for Wound Healing. Macromol. Biosci. 20, 2000141. doi:10.1002/mabi.202000141
Gupta, P., Agrawal, A., Murali, K., Varshney, R., Beniwal, S., Manhas, S., et al. (2019). Differential Neural Cell Adhesion and Neurite Outgrowth on Carbon Nanotube and Graphene Reinforced Polymeric Scaffolds. Mater. Sci. Eng. C 97, 539–551. doi:10.1016/j.msec.2018.12.065
Hashemi, A., Ezati, M., Mohammadnejad, J., Houshmand, B., and Faghihi, S. (2020). Chitosan Coating of TiO2 Nanotube Arrays for Improved Metformin Release and Osteoblast Differentiation. I. J. Nanomedicine. 15, 4471–4481. doi:10.2147/IJN.S248927
He, W., Elkhooly, T. A., Liu, X., Cavallaro, A., Taheri, S., Vasilev, K., et al. (2016). Silver Nanoparticle Based Coatings Enhance Adipogenesis Compared to Osteogenesis in Human Mesenchymal Stem Cells through Oxidative Stress. J. Mater. Chem. B 4, 1466–1479. doi:10.1039/c5tb02482j
Hebeish, A., El-Rafie, M. H., El-Sheikh, M. A., Seleem, A. A., and El-Naggar, M. E. (2014). Antimicrobial Wound Dressing and Anti-inflammatory Efficacy of Silver Nanoparticles. Int. J. Biol. Macromolecules 65, 509–515. doi:10.1016/j.ijbiomac.2014.01.071
Heo, D. N., Ko, W.-K., Bae, M. S., Lee, J. B., Lee, D.-W., Byun, W., et al. (2014). Enhanced Bone Regeneration with a Gold Nanoparticle-Hydrogel Complex. J. Mater. Chem. B 2, 1584–1593. doi:10.1039/c3tb21246g
Hu, D., Li, K., Xie, Y., Pan, H., Zhao, J., Huang, L., et al. (2017). The Combined Effects of Nanotopography and Sr Ion for Enhanced Osteogenic Activity of Bone Marrow Mesenchymal Stem Cells (BMSCs). J. Biomater. Appl. 31, 1135–1147. doi:10.1177/0885328217692140
Huang, K., Wu, J., and Gu, Z. (2019). Black Phosphorus Hydrogel Scaffolds Enhance Bone Regeneration via a Sustained Supply of Calcium-free Phosphorus. ACS Appl. Mater. Inter. 11, 2908–2916. doi:10.1021/acsami.8b21179
Huang, Y.-R., Wu, I.-T., Chen, C.-C., and Ding, S.-J. (2020). In Vitro comparisons of Microscale and Nanoscale Calcium Silicate Particles. J. Mater. Chem. B 8, 6034–6047. doi:10.1039/d0tb01202e
Jalali, F., Oveisi, H., and Meshkini, A. (2020). Enhanced Osteogenesis Properties of Titanium Implant Materials by Highly Uniform Mesoporous Thin Films of Hydroxyapatite and Titania Intermediate Layer. J. Mater. Sci. Mater. Med. 31. doi:10.1007/s10856-020-06450-1
Jia, W., Zhen, M., Li, L., Zhou, C., Sun, Z., Liu, S., et al. (2020). Gadofullerene Nanoparticles for Robust Treatment of Aplastic Anemia Induced by Chemotherapy Drugs. Theranostics 10, 6886–6897. doi:10.7150/thno.46794
Kim, H.-S., Lee, B. N., Choi, S., Kim, M. S., and Kim, J.-H. (2020a). Behavior of Muscle-Derived Stem Cells on Silica Nanostructured Substrates. Nanomaterials 10, 1651. doi:10.3390/nano10091651
Kim, M., Jee, S.-C., Sung, J.-S., and Kadam, A. A. (2020b). Supermagnetic Sugarcane Bagasse Hydrochar for Enhanced Osteoconduction in Human Adipose Tissue-Derived Mesenchymal Stem Cells. Nanomaterials 10, 1793. doi:10.3390/nano10091793
Kim, S., Oh, W.-K., Jeong, Y. S., and Jang, J. (2013a). Dual-Functional Poly(3,4-ethylenedioxythiophene)/MnO2Nanoellipsoids for Enhancement of Neurite Outgrowth and Exocytosed Biomolecule Sensing in PC12 Cells. Adv. Funct. Mater. 23, 1947–1956. doi:10.1002/adfm.201202198
Kim, T.-H., Lee, K.-B., and Choi, J.-W. (2013b). 3D Graphene Oxide-Encapsulated Gold Nanoparticles to Detect Neural Stem Cell Differentiation. Biomaterials 34, 8660–8670. doi:10.1016/j.biomaterials.2013.07.101
Krukiewicz, K., Putzer, D., Stuendl, N., Lohberger, B., and Awaja, F. (2020). Enhanced Osteogenic Differentiation of Human Primary Mesenchymal Stem and Progenitor Cultures on Graphene Oxide/Poly(methyl Methacrylate) Composite Scaffolds. Materials 13, 2991. doi:10.3390/ma13132991
Lee, W. C., Lim, C. H. Y. X., Shi, H., Tang, L. A. L., Wang, Y., Lim, C. T., et al. (2011). Origin of Enhanced Stem Cell Growth and Differentiation on Graphene and Graphene Oxide. ACS Nano 5, 7334–7341. doi:10.1021/nn202190c
Lee, Y.-J., Lee, S.-C., Jee, S. C., Sung, J.-S., and Kadam, A. A. (2019b). Surface Functionalization of Halloysite Nanotubes with Supermagnetic Iron Oxide, Chitosan and 2-D Calcium-Phosphate Nanoflakes for Synergistic Osteoconduction Enhancement of Human Adipose Tissue-Derived Mesenchymal Stem Cells. Colloids Surf. B: Biointerfaces 173, 18–26. doi:10.1016/j.colsurfb.2018.09.045
Lee, Y. B., Song, S.-J., Shin, Y. C., Jung, Y. J., Kim, B., Kang, M. S., et al. (2019a). Ternary Nanofiber Matrices Composed of PCL/black Phosphorus/collagen to Enhance Osteodifferentiation. J. Ind. Eng. Chem. 80, 802–810. doi:10.1016/j.jiec.2019.06.055
Li, D., Qiu, Y., Zhang, S., Zhang, M., Chen, Z., and Chen, J. (2020a). A Multifunctional Antibacterial and Osteogenic Nanomedicine: QAS-Modified Core-Shell Mesoporous Silica Containing Ag Nanoparticles. Biomed. Res. Int. 2020, 1–15. doi:10.1155/2020/4567049
Li, G., Cao, H., Zhang, W., Ding, X., Yang, G., Qiao, Y., et al. (2016a). Enhanced Osseointegration of Hierarchical Micro/Nanotopographic Titanium Fabricated by Microarc Oxidation and Electrochemical Treatment. ACS Appl. Mater. Inter. 8, 3840–3852. doi:10.1021/acsami.5b10633
Li, H., Wang, H., Pan, J., Li, J., Zhang, K., Duan, W., et al. (2020b). Nanoscaled Bionic Periosteum Orchestrating the Osteogenic Microenvironment for Sequential Bone Regeneration. ACS Appl. Mater. Inter. 12, 36823–36836. doi:10.1021/acsami.0c06906
Li, J., Chen, Y., Yang, Y., Kawazoe, N., and Chen, G. (2017). Sub-10 Nm Gold Nanoparticles Promote Adipogenesis and Inhibit Osteogenesis of Mesenchymal Stem Cells. J. Mater. Chem. B 5, 1353–1362. doi:10.1039/c6tb03276a
Li, J., Li, J. E. J., Zhang, J., Wang, X., Kawazoe, N., and Chen, G. (2016b). Gold Nanoparticle Size and Shape Influence on Osteogenesis of Mesenchymal Stem Cells. Nanoscale 8, 7992–8007. doi:10.1039/c5nr08808a
Li, K., Hu, D., Xie, Y., Huang, L., and Zheng, X. (2018). Sr-doped Nanowire Modification of Ca-Si-Based Coatings for Improved Osteogenic Activities and Reduced Inflammatory Reactions. Nanotechnology 29, 084001. doi:10.1088/1361-6528/aaa2b4
Li, L., Xiao, B., Mu, J., Zhang, Y., Zhang, C., Cao, H., et al. (2019). A MnO2 Nanoparticle-Dotted Hydrogel Promotes Spinal Cord Repair via Regulating Reactive Oxygen Species Microenvironment and Synergizing with Mesenchymal Stem Cells. Acs Nano 13, 14283–14293. doi:10.1021/acsnano.9b07598
Li, X., Wang, Y., Shi, L., Li, B., Li, J., Wei, Z., et al. (2020c). Magnetic Targeting Enhances the Cutaneous Wound Healing Effects of Human Mesenchymal Stem Cell-Derived Iron Oxide Exosomes. J. Nanobiotechnol 18, 113. doi:10.1186/s12951-020-00670-x
Liu, Q., Shi, J., Sun, J., Wang, T., Zeng, L., and Jiang, G. (2011). Graphene and Graphene Oxide Sheets Supported on Silica as Versatile and High-Performance Adsorbents for Solid-phase Extraction. Angew. Chem. Int. Ed. 50, 5913–5917. doi:10.1002/anie.201007138
Liu, Y., Tong, Z., Wang, C., Xia, R., Li, H., Yu, H., et al. (2021). TiO2 Nanotubes Regulate Histone Acetylation through F-Actin to Induce the Osteogenic Differentiation of BMSCs. Artif. Cell Nanomedicine, Biotechnol. 49, 398–406. doi:10.1080/21691401.2021.1910282
Lu, Q., Ericson, D., Song, Y., Zhu, C., Ye, R., Liu, S., et al. (2017). MnO2 Nanotube-Based NanoSearchlight for Imaging of Multiple MicroRNAs in Live Cells. ACS Appl. Mater. Inter. 9, 23325–23332. doi:10.1021/acsami.6b15387
Luo, M., Zhou, Y., Gao, N., Cheng, W., Wang, X., Cao, J., et al. (2020). Mesenchymal Stem Cells Transporting Black Phosphorus-Based Biocompatible Nanospheres: Active Trojan Horse for Enhanced Photothermal Cancer Therapy. Chem. Eng. J. 385, 123942. doi:10.1016/j.cej.2019.123942
Mahmoud, N. S., Ahmed, H. H., Mohamed, M. R., Amr, K. S., Aglan, H. A., Ali, M. A. M., et al. (2020). Role of Nanoparticles in Osteogenic Differentiation of Bone Marrow Mesenchymal Stem Cells. Cytotechnology 72, 1–22. doi:10.1007/s10616-019-00353-y
Mashayekhi, S., Rasoulpoor, S., Shabani, S., Esmaeilizadeh, N., Serati-Nouri, H., Sheervalilou, R., et al. (2020). Curcumin-loaded Mesoporous Silica Nanoparticles/nanofiber Composites for Supporting Long-Term Proliferation and Stemness Preservation of Adipose-Derived Stem Cells. Int. J. Pharmaceutics 587, 119656. doi:10.1016/j.ijpharm.2020.119656
Meng, Y., Yang, M., Liu, X., Yu, W., and Yang, B. (2019). Zn2+-Doped Carbon Dots, a Good Biocompatibility Nanomaterial Applied for Bio-Imaging and Inducing Osteoblastic Differentiation In Vitro. Nano 14, 1950029. doi:10.1142/S1793292019500292
Miao, Y., Shi, X., Li, Q., Hao, L., Liu, L., Liu, X., et al. (2019). Engineering Natural Matrices with Black Phosphorus Nanosheets to Generate Multi-Functional Therapeutic Nanocomposite Hydrogels. Biomater. Sci. 7, 4046–4059. doi:10.1039/c9bm01072f
Mladenović, Ž., Johansson, A., Willman, B., Shahabi, K., Björn, E., and Ransjö, M. (2014). Soluble Silica Inhibits Osteoclast Formation and Bone Resorption In Vitro. Acta Biomater. 10, 406–418. doi:10.1016/j.actbio.2013.08.039
Pan, W. Z., Dai, C. B., Li, Y., Yin, Y. M., Gong, L., Machuki, J. O., et al. (2020). PRP-chitosan Thermoresponsive Hydrogel Combined with Black Phosphorus Nanosheets as Injectable Biomaterial for Biotherapy and Phototherapy Treatment of Rheumatoid Arthritis. Biomaterials 239, 119851. doi:10.1016/j.biomaterials.2020.119851
Park, J., Bauer, S., Schlegel, K. A., Neukam, F. W., von der Mark, K., and Schmuki, P. (2009). TiO2Nanotube Surfaces: 15 Nm-An Optimal Length Scale of Surface Topography for Cell Adhesion and Differentiation. Small 5, 666–671. doi:10.1002/smll.200801476
Park, S. Y., Choi, D. S., Jin, H. J., Park, J., Byun, K.-E., Lee, K.-B., et al. (2011). Polarization-Controlled Differentiation of Human Neural Stem Cells Using Synergistic Cues from the Patterns of Carbon Nanotube Monolayer Coating. Acs Nano 5, 4704–4711. doi:10.1021/nn2006128
Patel, S., Chueng, S.-T. D., Yin, P. T., Dardir, K., Song, Z., Pasquale, N., et al. (2015a). Induction of Stem-Cell-Derived Functional Neurons by NanoScript-Based Gene Repression. Angew. Chem. Int. Ed. 54, 11983–11988. doi:10.1002/anie.201504902
Patel, S., Jung, D., Yin, P. T., Carlton, P., Yamamoto, M., Bando, T., et al. (2014). NanoScript: A Nanoparticle-Based Artificial Transcription Factor for Effective Gene Regulation. Acs Nano 8, 8959–8967. doi:10.1021/nn501589f
Patel, S., Pongkulapa, T., Yin, P. T., Pandian, G. N., Rathnam, C., Bando, T., et al. (2015b). Integrating Epigenetic Modulators into NanoScript for Enhanced Chondrogenesis of Stem Cells. J. Am. Chem. Soc. 137, 4598–4601. doi:10.1021/ja511298n
Patel, S., Yin, P. T., Sugiyama, H., and Lee, K.-B. (2015c). Inducing Stem Cell Myogenesis Using NanoScript. Acs Nano 9, 6909–6917. doi:10.1021/acsnano.5b00709
Pistone, A., Celesti, D., Piperopoulos, C., Ashok, E., Cembran, D., Tricoli, A., et al. (2019). Engineering of Chitosan-Hydroxyapatite-Magnetite Hierarchical Scaffolds for Guided Bone Growth. Materials 12, 2321. doi:10.3390/ma12142321
Pors Nielsen, S. (2004). The Biological Role of Strontium. Bone 35, 583–588. doi:10.1016/j.bone.2004.04.026
Price, C. T., Koval, K. J., and Langford, J. R. (2013). Silicon: A Review of its Potential Role in the Prevention and Treatment of Postmenopausal Osteoporosis. Int. J. Endocrinol. 2013, 1–6. doi:10.1155/2013/316783
Qi, W., Yan, J., Sun, H., and Wang, H. (2017). Multifunctional Nanocomposite Films for Synergistic Delivery of bFGF and BMP-2. Acs Omega 2, 899–909. doi:10.1021/acsomega.6b00420
Qin, H., Zhu, C., An, Z., Jiang, Y., Zhao, Y., Wang, J., et al. (2014). Silver Nanoparticles Promote Osteogenic Differentiation of Human Urine-Derived Stem Cells at Noncytotoxic Concentrations. I. J. Nanomedicine. 9, 2469–2478. doi:10.2147/IJN.S59753
Raucci, M. G., Fasolino, I., Caporali, M., Serrano-Ruiz, M., Soriente, A., Peruzzini, M., et al. (2019). Exfoliated Black Phosphorus Promotes In Vitro Bone Regeneration and Suppresses Osteosarcoma Progression through Cancer-Related Inflammation Inhibition. ACS Appl. Mater. Inter. 11, 9333–9342. doi:10.1021/acsami.8b21592
Reubinoff, B. E., Pera, M. F., Fong, C.-Y., Trounson, A., and Bongso, A. (2000). Embryonic Stem Cell Lines from Human Blastocysts: Somatic Differentiation In Vitro. Nat. Biotechnol. 18, 399–404. doi:10.1038/74447
Rostami, F., Tamjid, E., and Behmanesh, M. (2020). Drug-eluting PCL/graphene Oxide Nanocomposite Scaffolds for Enhanced Osteogenic Differentiation of Mesenchymal Stem Cells. Mater. Sci. Eng. C 115, 111102. doi:10.1016/j.msec.2020.111102
Roy Chowdhury, N., Hopp, I., Zilm, P., Murray, P., and Vasilev, K. (2018). Silver Nanoparticle Modified Surfaces Induce Differentiation of Mouse Kidney-Derived Stem Cells. RSC Adv. 8, 20334–20340. doi:10.1039/c8ra02145g
Shao, D., Lu, M., Xu, D., Zheng, X., Pan, Y., Song, Y., et al. (2017). Carbon Dots for Tracking and Promoting the Osteogenic Differentiation of Mesenchymal Stem Cells. Biomater. Sci. 5, 1820–1827. doi:10.1039/c7bm00358g
Shao, H., Li, T., Zhu, R., Xu, X., Yu, J., Chen, S., et al. (2018). Carbon Nanotube Multilayered Nanocomposites as Multifunctional Substrates for Actuating Neuronal Differentiation and Functions of Neural Stem Cells. Biomaterials 175, 93–109. doi:10.1016/j.biomaterials.2018.05.028
Shen, X., Ma, P., Hu, Y., Xu, G., Zhou, J., and Cai, K. (2015). Mesenchymal Stem Cell Growth Behavior on Micro/nano Hierarchical Surfaces of Titanium Substrates. Colloids Surf. B: Biointerfaces 127, 221–232. doi:10.1016/j.colsurfb.2015.01.048
Solanki, A., Shah, S., Yin, P. T., and Lee, K. B. (2013b). Nanotopography-mediated Reverse Uptake for siRNA Delivery into Neural Stem Cells to Enhance Neuronal Differentiation. Sci. Rep. 3, 1553. doi:10.1038/srep01553
Solanki, A., Shah, S., Memoli, K. A., Park, S. Y., Hong, S., and Lee, K.-B. (2010). Controlling Differentiation of Neural Stem Cells Using Extracellular Matrix Protein Patterns. Small 6, 2509–2513. doi:10.1002/smll.201001341
Solanki, A., Shah, S., Yin, P. T., and Lee, K.-B. (2013a). Nanotopography-mediated Reverse Uptake for siRNA Delivery into Neural Stem Cells to Enhance Neuronal Differentiation. Sci. Rep. 3, 1553. doi:10.1038/srep01553
Souza, J. C. M., Sordi, M. B., Kanazawa, M., Ravindran, S., Henriques, B., Silva, F. S., et al. (2019). Nano-scale Modification of Titanium Implant Surfaces to Enhance Osseointegration. Acta Biomater. 94, 112–131. doi:10.1016/j.actbio.2019.05.045
Su, H., Wang, Y., Gu, Y., Bowman, L., Zhao, J., and Ding, M. (2018). Potential Applications and Human Biosafety of Nanomaterials Used in Nanomedicine. J. Appl. Toxicol. 38, 3–24. doi:10.1002/jat.3476
Suarasan, S., Focsan, M., Soritau, O., Maniu, D., and Astilean, S. (2015). One-pot, green Synthesis of Gold Nanoparticles by Gelatin and Investigation of Their Biological Effects on Osteoblast Cells. Colloids Surf. B: Biointerfaces 132, 122–131. doi:10.1016/j.colsurfb.2015.05.009
Takahashi, K., and Yamanaka, S. (2006). Induction of Pluripotent Stem Cells from Mouse Embryonic and Adult Fibroblast Cultures by Defined Factors. Cell 126, 663–676. doi:10.1016/j.cell.2006.07.024
Tavares, M. T., Gaspar, V. M., Monteiro, M. V., S Farinha, J. P., Baleizão, C., and Mano, J. F. (2021). GelMA/bioactive Silica Nanocomposite Bioinks for Stem Cell Osteogenic Differentiation. Biofabrication 13, 035012. doi:10.1088/1758-5090/abdc86
Teo, J. Y., Seo, Y., Ko, E., Leong, J., Hong, Y.-T., Yang, Y. Y., et al. (2019). Surface Tethering of Stem Cells with H2O2-Responsive Anti-oxidizing Colloidal Particles for protection against Oxidation-Induced Death. Biomaterials 201, 1–15. doi:10.1016/j.biomaterials.2019.01.039
Tian, J., Wong, K. K. Y., Ho, C.-M., Lok, C.-N., Yu, W.-Y., Che, C.-M., et al. (2007). Topical Delivery of Silver Nanoparticles Promotes Wound Healing. ChemMedChem 2, 129–136. doi:10.1002/cmdc.200600171
Tong, Z., Liu, Y., Xia, R., Chang, Y., Hu, Y., Liu, P., et al. (2020). F-actin Regulates Osteoblastic Differentiation of Mesenchymal Stem Cells on TiO2 Nanotubes through MKL1 and YAP/TAZ. Nanoscale Res. Lett. 15, 183. doi:10.1186/s11671-020-03415-9
Trappmann, B., Gautrot, J. E., Connelly, J. T., Strange, D. G. T., Li, Y., Oyen, M. L., et al. (2012). Erratum: Extracellular-Matrix Tethering Regulates Stem-Cell Fate. Nat. Mater 11, 742. doi:10.1038/Nmat3387
Wan, R., Chu, S., Wang, X., Lei, L., Tang, H., Hu, G., et al. (2020). Study on the Osteogenesis of Rat Mesenchymal Stem Cells and the Long‐term Antibacterial Activity of Staphylococcus Epidermidis on the Surface of Silver‐rich TiN/Ag Modified Titanium alloy. J. Biomed. Mater. Res. 108, 3008–3021. doi:10.1002/jbm.b.34630
Wang, Q., Chen, B., Ma, F., Lin, S., Cao, M., Li, Y., et al. (2017). Magnetic Iron Oxide Nanoparticles Accelerate Osteogenic Differentiation of Mesenchymal Stem Cells via Modulation of Long Noncoding RNA INZEB2. Nano Res. 10, 626–642. doi:10.1007/s12274-016-1322-4
Wang, W., Wang, Z., Fu, Y., Dunne, N., Liang, C., Luo, X., et al. (2020). Improved Osteogenic Differentiation of Human Amniotic Mesenchymal Stem Cells on Gradient Nanostructured Ti Surface. J. Biomed. Mater. Res. 108, 1824–1833. doi:10.1002/jbm.a.36948
Wu, Q., Wang, K., Wang, X., Liang, G., and Li, J. (2020). Delivering siRNA to Control Osteogenic Differentiation and Real-Time Detection of Cell Differentiation in Human Mesenchymal Stem Cells Using Multifunctional Gold Nanoparticles. J. Mater. Chem. B 8, 3016–3027. doi:10.1039/c9tb02899d
Wu, Y., Li, D., Zhou, F., Liang, H., Liu, Y., Hou, W., et al. (2018). Versatilein Situsynthesis of MnO2nanolayers on Upconversion Nanoparticles and Their Application in Activatable Fluorescence and MRI Imaging. Chem. Sci. 9, 5427–5434. doi:10.1039/c8sc00490k
Xia, D., Wang, X., Wang, Y., Wang, Y., Meng, H., Li, L., et al. (2020). Silver-decorated Mesostructured Cellular Silica Foams as Excellent Antibacterial Hemostatic Agents for Rapid and Effective Treatment of Hemorrhage. Mater. Sci. Eng. C 115, 111105. doi:10.1016/j.msec.2020.111105
Xia, Y., Chen, H., Zhao, Y., Zhang, F., Li, X., Wang, L., et al. (2019). Novel Magnetic Calcium Phosphate-Stem Cell Construct with Magnetic Field Enhances Osteogenic Differentiation and Bone Tissue Engineering. Mater. Sci. Eng. C 98, 30–41. doi:10.1016/j.msec.2018.12.120
Xin, Y., Jiang, J., Huo, K., Hu, T., and Chu, P. K. (2009). Bioactive SrTiO3 Nanotube Arrays: Strontium Delivery Platform on Ti-Based Osteoporotic Bone Implants. Acs Nano 3, 3228–3234. doi:10.1021/nn9007675
Xu, C., Xu, Y., Yang, M., Chang, Y. K., Nie, A. M., Liu, Z. Y., et al. (2020). Black-Phosphorus-Incorporated Hydrogel as a Conductive and Biodegradable Platform for Enhancement of the Neural Differentiation of Mesenchymal Stem Cells. Adv. Funct. Mater. 30. doi:10.1002/adfm.202000177
Yang, D., Li, T., Xu, M., Gao, F., Yang, J., Yang, Z., et al. (2014a). Graphene Oxide Promotes the Differentiation of Mouse Embryonic Stem Cells to Dopamine Neurons. Nanomedicine 9, 2445–2455. doi:10.2217/Nnm.13.197
Yang, H., Yu, M., Wang, R., Li, B., Zhao, X., Hao, Y., et al. (2020). Hydrothermally Grown TiO2-Nanorods on Surface Mechanical Attrition Treated Ti: Improved Corrosion Fatigue and Osteogenesis. Acta Biomater. 116, 400–414. doi:10.1016/j.actbio.2020.09.005
Yang, L., Chueng, S.-T. D., Li, Y., Patel, M., Rathnam, C., Dey, G., et al. (2018). A Biodegradable Hybrid Inorganic Nanoscaffold for Advanced Stem Cell Therapy. Nat. Commun. 9. doi:10.1038/s41467-018-05599-2
Yang, M., Meng, Y., Liu, J., Yu, W., and Yang, B. (2019). Facile Synthesis of Mg2+ -Doped Carbon Dots as Novel Biomaterial Inducing Cell Osteoblastic Differentiation. Part. Part. Syst. Charact. 36, 1800315. doi:10.1002/ppsc.201800315
Yang, X., Li, E., Wan, Y., Smith, P., Shang, G., and Cui, Q. (2014b). Antioxidative Fullerol Promotes Osteogenesis of Human Adipose-Derived Stem Cells. I. J. Nanomedicine. 9, 4023–4031. doi:10.2147/IJN.S66785
Yin, F., Hu, K., Chen, S., Wang, D., Zhang, J., Xie, M., et al. (2017). Black Phosphorus Quantum Dot Based Novel siRNA Delivery Systems in Human Pluripotent Teratoma PA-1 Cells. J. Mater. Chem. B 5, 5433–5440. doi:10.1039/c7tb01068k
Yu, J., Xu, L., Li, K., Xie, N., Xi, Y., Wang, Y., et al. (2017). Zinc-modified Calcium Silicate Coatings Promote Osteogenic Differentiation through TGF-β/Smad Pathway and Osseointegration in Osteopenic Rabbits. Sci. Rep. 7, 3440. doi:10.1038/s41598-017-03661-5
Yuan, Z., Liu, P., Liang, Y., Tao, B., He, Y., Hao, Y., et al. (2018). Investigation of Osteogenic Responses of Fe-Incorporated Micro/nano-Hierarchical Structures on Titanium Surfaces. J. Mater. Chem. B 6, 1359–1372. doi:10.1039/c7tb03071a
Zandén, C., Hellström Erkenstam, N., Padel, T., Wittgenstein, J., Liu, J., and Kuhn, H. G. (2014). Stem Cell Responses to Plasma Surface Modified Electrospun Polyurethane Scaffolds. Nanomedicine: Nanotechnology, Biol. Med. 10, e949–e958. doi:10.1016/j.nano.2014.01.010
Zhang, H., Li, S., Liu, Y., Yu, Y., Lin, S., Wang, Q., et al. (2020). Fe3O4@GO Magnetic Nanocomposites Protect Mesenchymal Stem Cells and Promote Osteogenic Differentiation of Rat Bone Marrow Mesenchymal Stem Cells. Biomater. Sci. 8, 5984–5993. doi:10.1039/d0bm00906g
Zhang, L. C., and Chen, L. Y. (2019). A Review on Biomedical Titanium Alloys: Recent Progress and Prospect. Adv. Eng. Mater. 21, 1801215. doi:10.1002/adem.201801215
Zhang, R., Lee, P., Lui, V. C. H., Chen, Y., Liu, X., Lok, C. N., et al. (2015). Silver Nanoparticles Promote Osteogenesis of Mesenchymal Stem Cells and Improve Bone Fracture Healing in Osteogenesis Mechanism Mouse Model. Nanomedicine: Nanotechnology, Biol. Med. 11, 1949–1959. doi:10.1016/j.nano.2015.07.016
Zhang, T., Li, F., Xu, Q., Wang, Q., Jiang, X., Liang, Z., et al. (2019). Ferrimagnetic Nanochains‐Based Mesenchymal Stem Cell Engineering for Highly Efficient Post‐Stroke Recovery. Adv. Funct. Mater. 29, 1900603. doi:10.1002/adfm.201900603
Zhang, W., Wang, G., Liu, Y., Zhao, X., Zou, D., Zhu, C., et al. (2013). The Synergistic Effect of Hierarchical Micro/nano-Topography and Bioactive Ions for Enhanced Osseointegration. Biomaterials 34, 3184–3195. doi:10.1016/j.biomaterials.2013.01.008
Zhao, L., Mei, S., Chu, P. K., Zhang, Y., and Wu, Z. (2010). The Influence of Hierarchical Hybrid Micro/nano-Textured Titanium Surface with Titania Nanotubes on Osteoblast Functions. Biomaterials 31, 5072–5082. doi:10.1016/j.biomaterials.2010.03.014
Zhou, X., Feng, W., Qiu, K., Chen, L., Wang, W., Nie, W., et al. (2015). BMP-2 Derived Peptide and Dexamethasone Incorporated Mesoporous Silica Nanoparticles for Enhanced Osteogenic Differentiation of Bone Mesenchymal Stem Cells. ACS Appl. Mater. Inter. 7, 15777–15789. doi:10.1021/acsami.5b02636
Keywords: Inorganic nanomaterials, stem cells, differentiation, cell therapy, regenerative medicine
Citation: He F, Cao J, Qi J, Liu Z, Liu G and Deng W (2021) Regulation of Stem Cell Differentiation by Inorganic Nanomaterials: Recent Advances in Regenerative Medicine. Front. Bioeng. Biotechnol. 9:721581. doi: 10.3389/fbioe.2021.721581
Received: 07 June 2021; Accepted: 15 September 2021;
Published: 30 September 2021.
Edited by:
J Mary Murphy, National University of Ireland Galway, IrelandCopyright © 2021 He, Cao, Qi, Liu, Liu and Deng. This is an open-access article distributed under the terms of the Creative Commons Attribution License (CC BY). The use, distribution or reproduction in other forums is permitted, provided the original author(s) and the copyright owner(s) are credited and that the original publication in this journal is cited, in accordance with accepted academic practice. No use, distribution or reproduction is permitted which does not comply with these terms.
*Correspondence: Gan Liu, bGl1Z2FuNUBtYWlsLnN5c3UuZWR1LmNu; Wenbin Deng, ZGVuZ3diNUBtYWlsLnN5c3UuZWR1LmNu