- 1Department of Laboratory Medicine, West China Hospital, Sichuan University, Chengdu, China
- 2The First People’s Hospital of Shuangliu District, Chengdu/West China (Airport)Hospital Sichuan University, Chengdu, China
Infectious diseases are considered as a pressing challenge to global public health. Accurate and rapid diagnostics tools for early recognition of the pathogen, as well as individualized precision therapy are essential for controlling the spread of infectious diseases. Aptamers, which were screened by systematic evolution of ligands by exponential enrichment (SELEX), can bind to targets with high affinity and specificity so that have exciting potential in both diagnosis and treatment of infectious diseases. In this review, we provide a comprehensive overview of the latest development of SELEX technology and focus on the applications of aptamer-based technologies in infectious diseases, such as targeted drug-delivery, treatments and biosensors for diagnosing. The challenges and the future development in this field of clinical application will also be discussed.
Introduction
Infectious diseases which result from pathogenic microorganisms become one of the most important illnesses in the world (Mosing et al., 2005; Giri et al., 2019). Because of the characteristics of contagion and epidemic, infectious diseases not only endanger public health but also pose serious threats and huge losses to social stability and economic development. Over the past decades, the sudden public health crises including Ebola hemorrhagic fevers, avian influenza, severe acute respiratory syndrome (SARS) or Middle East respiratory syndrome (MERS), as well as COVID-19, have swept out the world and caused a significant impact on society inevitably (Del Rio et al., 2014; Liu et al., 2017; Lycett et al., 2020; Wiersinga et al., 2020; Perra, 2021). Existing pathogen detection methods are difficult to achieve a balance between timeliness, accuracy and cost to meet the requirements of large-scale population screening. In addition, the existence of antibiotic-resistant microbes such as multidrug-resistant tuberculosis (MDR-TB), extensively drug-resistant tuberculosis (XDR-TB) and methicillin-and aminoglycoside-resistant Staphylococcus aureus (MARSA) brings greater challenges to the prevention and treatment of infectious diseases (Fauci et al., 2005; Tan Z. M. et al., 2020; Nang et al., 2021). Therefore, there is an urgent demand to develop rapid, economic, and sensitive early diagnostic assays for pathogens, and also adequate therapeutics of precision medicine for infectious diseases.
Aptamers, also known as “chemical antibodies”, are a class of single-stranded DNAs or RNAs which can target various ligands through non-covalent bonds. Those aptamers are synthetic screened in vitro by a selection procedure, commonly known as Systematic Evolution of Ligands by Exponential Enrichment (SELEX). DNA aptamers are more stable and widely used, while RNA aptamers are more likely to form complex structures such as stem, loop, hairpin, G-quadruplex and so on (Breaker, 1997; Lin and Patel, 1997; Wu et al., 2017). These folding 3D structures can increase sequence space coverage and improve space representation, which are beneficial for aptamer-target recognition, thus improving the specificity and affinity of the screened aptamers (Kinghorn et al., 2017). Aptamers have attracted considerable attention because of their exceptional merits such as low synthesis cost, easy chemical modification, high chemical stability and binding affinity, low immunogenicity, good repeatability and reusability (Hong and Sooter, 2015; Yu et al., 2021). To date, thousands of aptamers have been identified, which can be used to identify different targets with high affinity and specificity, such as small metal ions, organic molecules, amino acids, proteins, bacteria, viruses, whole cells and even animals (Cowperthwaite and Ellington, 2008; Zhou and Rossi, 2017). Based on the above advantages, nucleic acid aptamers have been explored as the most promising molecular recognition probes to widely applied in the field of the identification of infectious agents and the therapeutic of infectious diseases.
In this review, the recent advances of SELEX technologies for aptamer selecting of infectious pathogens will be overviewed. Then we will focus on a variety of aptamer-based biosensors for infection detecting and the state-of-the-art aptamer therapeutics and drug delivery systems in the precision treatment of infectious diseases. The current challenges and future prospects of aptamers will also be discussed to provide a direction for the research and development of aptamers.
Discovery of Specific Aptamers by Systematic Evolution of Ligands by Exponential Enrichment
SELEX was originally developed by Gold and Szostak in 1990 (Ellington and Szostak, 1990; Tuerk and Gold, 1990). Before selecting, an oligonucleotide library consisting of two constant regions at 5′ and 3′ ends and a random region in the middle should be synthesized. The primary library usually contains up to 1012–1015 different nucleic acid molecules, of which the random region is about 20–40 bp, and the constant regions that flanked are about 20 bp, including restriction endonuclease sites, primer binding sites and RNA promoter binding sites (Zimbres et al., 2013; Hong and Sooter, 2015). Currently, both DNA and RNA libraries are widely used for SELEX due to their distinct advantages.
General Systematic Evolution of Ligands by Exponential Enrichment Scheme
In brief, a typical SELEX comprises three critical stages: 1) incubate the target molecule with the combinatorial library of nucleic acids in vitro to form an aptamer-target complex; 2) partition the complex from the unbound nucleotides and separate the oligonucleotide chain that binds to the target molecule; 3) obtain a sub-library by employing PCR (DNA SELEX) or reverse transcription PCR (RNA SELEX) to amplifying the target-bound sequence (Figure 1). It is worth mentioning that the negative target is usually introduced to improve target specificity by recovering and amplifying the unbound oligonucleotide chain. In this way, the oligonucleotide chain obtained by iterative circles of selecting and PCR amplification is the nucleic acid aptamer of the target. After cloning and sequencing, the identification, binding ability and the secondary/tertiary structure of aptamer can be analyzed subsequently.
Novel Approaches for Aptamer Selection
Despite the conventional SELEX technology is well-established, the process is tedious and time-consuming, which typically takes up to 20 rounds. At present, various novel SELEX technologies have been developed to improve the shortcomings of the conventional one, further accelerate the speed and efficiency of high-affinity aptamer screening and shorten the selection period. In this respect, the rapid development of SELEX technology provides substantial potential for rapid response to public health emergencies.
The separation of aptamer-bound sequences from the unbound nucleotides is one of the most critical steps in the process of SELEX. Thus, the screening process can be accelerated by optimizing the binding and separation of the target molecule with libraries. Usually, immobilization is involved in binding the targets to a carrier and then incubated with the nucleic acid library (Van Dyke et al., 2016). Common carriers such as magnetic beads, affinity chromatography columns or microfluidic chips. Among them, considerable attention has been given to magnetic beads, as chemical modification of magnetic beads is easy and the magnetic separation method is convenient, fast and effective (McKeague et al., 2010; Hunniger et al., 2014; Xi et al., 2015; Duan et al., 2016; Ma et al., 2018). To date, a variety of aptamers have been successfully selected by magnetic SELEX (Oh et al., 2009; Lai et al., 2014; Wu J.-H. et al., 2018). For example, Hong et al. first proposed a magnetism-controlled chip for Ebola virus aptamers selection by integrating the magnetic bead-based SELEX (Mag-SELEX) with a microfluidic system and by this method they got an aptamer with low dissociation constants and reducing the selecting round to three (Hong et al., 2019). Another novel approach called Capillary Electrophoresis SELEX (CE-SELEX) is based on the difference of electrophoretic mobility between target bounded sequence with the unbound one (Mendonsa and Bowser, 2004a; Mendonsa and Bowser, 2004b). Mosing Renee K et al. successfully obtained aptamers candidate with high affinity to human immunodeficiency virus (HIV) reverse transcriptase by CE-SELEX only after four cycles (Mosing et al., 2005). Although in vitro SELEX technology for a single target has been quite mature, especially for proteins, the clinical applications of these aptamers are still limited. The reason is that the properties of a recombinant protein are not the same as those of natural proteins, including conformation, advanced structure and biological activity. Besides, aptamers screened for artificial recombinant proteins may be much less sensitive in identifying natural targets. To overcome this limitation, cell-SELEX, which employs the whole cell as a target, was first proposed in 2003 (Daniels et al., 2003). Recently, a series of aptamers against various pathogens were generated by cell-SELEX, such as Salmonella typhimurium, Escherichia coli, Vibrio parahaemolyticus, Trypanosoma cruzi, and so on (Duan et al., 2012; Nagarkatti et al., 2012; Dwivedi et al., 2013; Kim et al., 2013; Bitaraf et al., 2016; Saad et al., 2020). In addition to the methods mentioned above, many other innovative SELEX strategies have also been developed in the past decades, such as capture-SELEX, in vivo SELEX, high-throughput sequencing SELEX and so on. The principles and characteristics of those techniques are summarized in Table 1.
SELEX technology determines that the aptamer target molecules have a very wide range which covers a huge range from metal ions, compounds, peptides, nucleic acids, proteins to cells, and even includes some complex targets, such as viruses, bacteria and other pathogens. Based on SELEX technology, aptamers obtained from specific substances of the pathogens such as surface proteins or key enzymes in physiological processes, are expected to be used for pathogen diagnosis or infection treatment.
Application of Aptamers Biosensor in Infectious Diseases Diagnosis
Most infectious diseases progress rapidly, and a clear diagnosis is key for effective treatment. Also, efficient pathogen detection methods help control the spread of infectious diseases. Therefore, early, accurate and rapid pathogen diagnosis is of great significance. Currently, the routine diagnostics for infectious pathogen are bacterial culture, polymerase chain reaction (PCR) and immunological detection (Lampel et al., 2000; Byrne et al., 2009; Arvanitis et al., 2014; Rohr et al., 2016; Balmaseda et al., 2017). These approaches are relatively mature, but they inevitably have limitations such as time-consuming, high cost, and tedious operation. Alternatively, although antibody is indispensable in most routine tests, it inevitably meets many limitations, such as laborious and expensive production and identification, batch-to-batch variation, and its biological activity is susceptible to the environment such as pH and temperature (Jayasena, 1999; Nimjee et al., 2005; Rosenbaum et al., 2012; Yu et al., 2021). Aptamers have a very wide range of target molecules, which can be designed for early disease markers, creating conditions for the early detection of pathogens. At the same time, aptamers can be rapidly chemically synthesized in batches with a long shelf-life for storage at room temperature. The comparison of main characteristics between antibodies and aptamers is shown in Table 2.
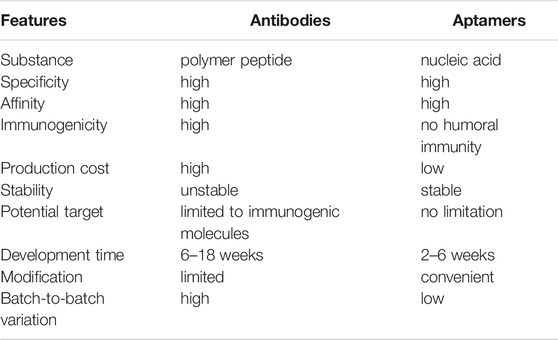
TABLE 2. The characteristics comparison of nucleic acid aptamers and antibodies (Jayasena, 1999; Zhou and Rossi, 2017; Zhuo et al., 2017; Yu et al., 2021).
Due to those advantages, abundant research has integrated aptamers into biosensors as molecular recognition elements over the past decades. The biological signals received by aptamers can be transformed into the optical signal or electrical signal by the signal converter, then the output signal will be amplified by the electronic system and further be used for the detection of pathogenic microorganisms qualitatively or quantitatively. According to the pathogenic targets, we category these aptamer-based biosensors into three classes: bacteria, viruses and others.
Detection of Bacteria
The current diagnostic gold standard of bacterial identification is still bacterial culture, subsequent biochemical identification and serological typing (Rauch and Nauen, 2003; Andini et al., 2018; Takeuchi et al., 2018). However, they are time-consuming, usually several days, and have some limitations in the identification of some certain species. In addition, it is less convenient for point-of-care detection. To meet this need, aptamer-based biosensors were widely developed. As an example, an electrochemical sensor for the detection of Mycobacterium tuberculosis reference strain H37Rv ATCC 27294 using aptamer technology was introduced by Zhang X. et al. (2019). In this method, H37Rv aptamers layer modified on the bare Au interdigital electrode are recognition probe and oligonucleotides modified with gold nanoparticles (AuNPs-DNA) are signal probe. When H37Rv bacteria was present, it competitively bounds to aptamers, and the displacement of AuNPs-DNA dramatically changing the conductivity. The detection limit of this method is 100 CFU/ml, and it can be used for rapid detection of H37Rv only in 2 h. In one of recent studies, electrochemical aptasensor was devloped for the detection of Escherichia coli O157:H7 (E. coli) (Qaanei et al., 2021). In this system, the aptamer is employed to improve the selectivity while a reduced graphene oxide–poly (vinyl alcohol) and gold nanoparticles nanocomposite (AuNPs/rGO–PVA/GCE) is used to raise the sensor sensitivity. Consequently, this aptasensor is able to detect E. coli as low as 9.34 CFU/ml, with an excellent specificity.
Detection of Viruses
Many infectious diseases are caused by viral infection, such as acquired immunodeficient syndrome (AIDS), influenza and COVID-19, which has dealt a heavy blow to the world in 2020. These pathogens widely distribute in open systems and are endanger human health and the public environment. For example, the current COVID-19 pandemic in more than 100 countries around the world has infected an untold number of people and caused large numbers of deaths. Thus, it is urgently desirable for cost-effective, rapid and reliable diagnostic methods. Woo et al. designed an aptamer-based fluorescent sensor to detect SARS-CoV-2 RNA in human nasopharyngeal samples (Woo et al., 2020). They intelligently designed a one-pot, ligation-dependent isothermal reaction cascade that consists of a ligation reaction by SplintR ligase and subsequent transcription by T7 RNA polymerase. When target RNA existing, the RNA aptamers of the isothermal reaction products bind to fluorescent dyes and produce a significant fluorescence signal. The detection limit is 0.1 aM. Interestingly, only by redesigning the hybridization regions of the probes, a series of viruses including influenza viruses, MERS and SARS can be detected by this method. Another recent approach was proposed by Liu et al. (2020), which designed a qPCR amplification reaction triggered by two aptamers probes for ultrasensitive detection of serum COVID-19-associated antigens. This method exhibits excellent detection performance and can be conducted within 2 h. In another study by Babamiri et al. (2018), aptamer against HIV-1 was used for the development of an electrochemiluminescence (ECL) sensor. This aptamer-based biosensor showed excellent sensitivity and specificity, with a detection limit as low as 0.3 fM, and can be successfully applied to clinical serum samples analysis.
More importantly, some viruses have multiple subtypes and mutant quickly, which are highly infectious and transmissible pathogens (Shim, 2011; Li et al., 2019; Ma and Ma, 2020). Therefore, the detection of mutation is becoming a top priority in the field of methodological research. At present, molecular methods such as PCR and DNA sequencing are powerful tools to obtain information on mutant status, but these methods could not satisfy the expectation for extensive disease screening because of the need for special equipment and expensive consumables (Escadafal et al., 2014; Ma et al., 2015). Recently, Wang et al. established a highly sensitive platform for detecting SARS-CoV-2 and its mutated variants based on a CRISPR-Cas13 transcription amplification principle (Wang et al., 2021). They employed light-up RNA aptamers as the sensitive output of amplification signals, achieving sensing of as low as 82 copies of SARS-CoV-2. Moreover, this platform was applied to strictly identify the key mutation of the SARS-CoV-2 variant, D614G, which increases viral stability and flexibility and further enhances replication and transmission.
Detection of Other Pathogens
Aptamer-based biosensors are used to detect several other pathogens alternatively. Protozoan parasite infection remains one of the major public health problems in some underdeveloped and developing countries with poor sanitation and economic backwardness. Thus, the application of detections that require expensive equipment or complex laboratory sites is significantly limited in these areas. In this case, a deal of aptamer-based biosensors has been developed for the identification of parasites due to their low cost, simplicity, portability (Lee et al., 2012; Singh et al., 2019a; Singh et al., 2019b; Frezza et al., 2020; Minopoli et al., 2020). Take the diagnosis of malaria as an example, Singh et al. established instrument-based and instrument-free approaches for pan malaria and P. falciparum species based on aptamers specific to Plasmodium lactate dehydrogenase (PLDH) and Plasmodium falciparum glutamate dehydrogenase (PfGDH) respectively (Singh et al., 2019a). They successfully overcame the false-negative limitation of traditional microscopic examination of Giemsa-stained thick blood films and achieved an ultrasensitive detection with a low cost (∼0.10 $ per test) (Mikhail et al., 2011; Thongdee et al., 2014; Bin Dajem, 2015; Sumari et al., 2016; Habyarimana and Ramroop, 2020). In addition, Fu developed an indirect blocking enzyme linked aptamer assay (ib-ELAA) for the detection of Mycoplasma gallisepticum (M. gallisepticum), which was the major pathogen of chronic respiratory disease (Fu et al., 2021b; Fu et al., 2021a). In this method, they initially screened out the aptamer Apt-236 which can bind to PvpA protein of M. gallisepticum with high affinity, and further integrated Apt-236 into ib-ELAA and successfully applied in the detection of clinical chicken sera sample. Similarly, a great many of aptamer-based methods for the detection of pathogenic parasites (Homann et al., 2006; Bruno et al., 2014; Ospina-Villa et al., 2018; Ospina, 2020), mycoplasma (Fu et al., 2014; Liu Y. et al., 2019; Wan et al., 2020), several fungal species (McKeague et al., 2010; Barthelmebs et al., 2011; Ma et al., 2014; Wu S. et al., 2018; Liu M. et al., 2019; Han et al., 2021) have also been developed.
The comparison of representative aptasensor performance in the detection of the various pathogen is summarized in Table 3.
Application of Aptamers in Infectious Diseases Treatment
At present, the therapeutic of infectious diseases is mainly based on the principle of symptomatic treatment or specific anti-pathogen treatment. However, antimicrobial resistance, high viral genomes mutation variability and escaping the host immune response make most medications and vaccines inefficient (Finlay and McFadden, 2006; Labella and Merel, 2013; Dunning et al., 2014a; Fall-Malick et al., 2014; Ferir et al., 2014; Lazarevic, 2014; Marascio et al., 2014; Sahu, 2015; Wandtke et al., 2015). It is worth mentioning that the effect of antiviral therapy is not ideal for all patients and side effects caused by many existing antiviral drugs may lead to other diseases than primary affection. For instance, the most effective therapy for patients with hepatitis C (interferon alfa-2b plus ribavirin) benefits only about 50% of cases (Manns et al., 2001; Sarhan et al., 2020), whereas such therapeutic regimen usually be associated with numerous adverse effects (Fried, 2002; Nishimura et al., 2002; Fisher et al., 2004; Negro, 2010; Pazienza, 2011; Gull et al., 2019). Many studies confirmed that aptamer, as a promising candidate, can target the key molecules in bacterial physiological processes or viral surface proteins, and treat the infection effectively by inhibiting viruses penetrating the cells, disrupting the activity of enzymes related to viral replication or regulating immune response (Bellecave et al., 2008; Gopinath et al., 2012; Hwang et al., 2012; Torabi et al., 2020).
Aptamer-Based Therapeutics
Precision medicine holds great promise to harness the benefits of aptamers that can bound to targets with high specificity and affinity for targeted treatment of a variety of diseases. Such therapeutic aptamers function mainly in the following two ways: 1) aptamers function as antagonists to disrupt the function of a pathologic target protein and block the interaction of disease-associated targets by specifically binding to target; 2) aptamers function as agonists to increase the ability of the target receptors. For instance, Lee et al. reported an RNA aptamer against the Hepatitis C virus (HCV) nonstructural protein 5B can effectively inhibit HCV replication and suppressed HCV infectious virus particle formation (Lee et al., 2015). HIV integrase is considered necessary for retroviral replication, which is a primary target for the therapy of AIDS (Shoji et al., 2002). Thus, aptamers as potential anti-HIV integrase inhibitors have drawn much attention from researchers. Pang and his colleague designed an anti-HIV lentivirus vector consist of shRNA, ribozyme and RNA decoy (Pang et al., 2018). By screening aptamers against integrase and incorporating these aptamers in shRNA, they successfully observed interference and inhibition to transcription of HIV in cell cultures. Also, many other aptamers against Tat protein, gp120, reverse transcriptase, nucleocapsid protein were developed for further exploit research of antivirus therapy (Mufhandu et al., 2012; Aeksiri et al., 2014; Nguyen et al., 2020; Zhang et al., 2020). COVID-19 has wreaked havoc all over the world, but no specific treatment has been developed yet. Liu and his colleague developed an aptamer that specifically targets the spike protein of the coronavirus SARS-CoV-2, which is the critical role of viral infection (Liu et al., 2021). When the receptor-binding domain (RBD) of the spike protein of the coronavirus SARS-CoV-2 binds to the human angiotensin-converting enzyme 2 (ACE2), an infection cascade is triggered (Schmitz et al., 2021). They proved that this aptamer effectively protects host cells from infection by blocking the interaction between spike protein and ACE2 receptor. This exciting report is fueling hope in the field of COVID-19’s therapy, it also brings up new opportunities for aptamer-based treatment.
Despite aptamer-based therapy shows huge potential, their inherent physicochemical characteristics affect pharmacokinetic properties in some way, which may limit their widespread clinical application. The most critical problems are nuclease degradation and rapid renal filtration. Unmodified nucleic acid aptamers have an average half-life of fewer than 10 min for the susceptibility to nucleases which abundantly exist in biological fluids (Lakhin et al., 2013). To increase its biostability and prolong the in vivo half-life, chemical modifications are typically introduced such as replacing 2′-OH with fluoro (F), amino (NH2), or O-methyl (OCH3) groups at the 2′ position (Morita et al., 2018). Since the average diameter of 5–30 kDa aptamers is less than 5nm, which is smaller than the glomerular filtration threshold (i.e., 30–50 kDa), aptamers are inevitably rapidly excreted through renal filtration (Guo, 2010; Morita et al., 2018). To overcome this disadvantage, many macromolecular substances such as proteins, cholesterol, liposomes, high molecular mass PEG or nanomaterials are involved to modify aptamers, and there is indeed a significant improvement in some reports (Fisher et al., 1976; Drolet et al., 2000; Rusconi et al., 2004; Burmeister et al., 2005; Chen et al., 2015; Heo et al., 2016). However, chemical modification is a double-edged sword. Serious allergic responses caused by biomaterial, non-specific immune activation, tissue toxicity caused by drug metabolism and other undesirable side effects have been reported (Geary et al., 2003; Waring, 2010; Wong and Goldberg, 2014; Lincoff et al., 2016; Morita et al., 2016). Thus, it is necessary to cautiously improve and optimize the formulations or administration routines of aptamer therapy.
Aptamers as Intelligent Chemical Drug-Delivery Systems
The other ingenious anti-infective therapeutic strategy is to employ aptamers as intelligent messengers of therapeutic agents, such as small interfering RNA molecules and ribozymes (Dey et al., 2005; Romero-López et al., 2012; Zhu et al., 2012; Wandtke et al., 2015). In the broad area of drug delivery system, aptamers have also been extensively sought after due to the inherited merits: relatively small physical size, versatile structure, quick chemical production, flexible chemical modification, high stability, and lack of immunogenicity. Through chemical modification and bioconjugation, a variety of therapeutic agents increase their stability and bioactivity without change the primary characteristics (Fattal et al., 2018). The targeting of aptamer increases the local drug concentration, thus improving the therapeutic efficacy whilst reducing the systemic toxic and side effects of the drug. Therefore, many cell-specific aptamers are explored to conjugate with chemical entities including chemotherapeutic agents, siRNA, nanoparticles for targeted delivery of drugs.
For the development of aptamers as drug-delivery systems, one example is anti-gp120 aptamer for the treatment of AIDS (Zhou J. et al., 2013). A viral surface protein, gp120, is closely related to viral infection. HIV-1 infects target cells through binding gp120 to cellular receptor CD4 and chemokine receptors such as CCR5 or CXCR4. In this research, Zhou and his colleague employed an anti-gp120 aptamers as siRNA delivery vehicles, effective delivery viral inhibiting siRNA in vivo and potent inhibition of HIV-1 replication. Similarly, Pan et al. designed an ingenious system which employed bispecific circular aptamers (bc-apts) to specifically tether protein cargoes and cellular membrane proteins (Pan et al., 2020). This strategy achieved the specific delivery of functional therapeutic proteins, and the deactivation of functional proteins was also avoided. Furthermore, the bioactivity of the drug in the lesion was specifically increased. Yan et al. reviewed the design and application of aptamers as drug-delivery systems in the photodynamic platform of targeted therapy (Yan et al., 2021). In this review, they focused on the application of aptamers-targeted photodynamic therapies which achieve controlled and accurate delivery of therapeutic drugs to the lesion sites and obtained excellent photodynamic therapy efficiency. Another advantage of an aptamer-based targeted delivery system is that it can delay the evolution of resistance and improve the efficiency of the antimicrobials on already resistant pathogens. Ucak et al. used Staphylococcus aureus-specific aptamer to functionalize methicillin, which is an antibiotic for serious infectious caused by Gram-positive bacteria (Ucak et al., 2020). By limiting the amount and dosage during therapy, they proved that the novel delivery system was significantly effective in reducing minimum inhibitory concentration (MIC) values. Therefore, the aptamer-based targeted delivery system is a promising method for the treatment of infections caused by antibiotic-resistant bacteria. In addition to the examples above, multiple types of research have shown the extraordinary ability of aptamer in drug target-delivering (Nimjee et al., 2005; Shiang et al., 2013; Hahn, 2017; Chonco et al., 2018; Fattal et al., 2018; Tan X. et al., 2020).
Conclusion and Future Perspectives
Taking advantage of low cost, easy chemical modification, high specificity and binding affinity, low immunogenicity, aptamers have been used as an alternative to antibodies in the development of aptamer-based technologies in the past decades. The development of biomedical technology has enabled a comprehensive exploration of the screening technologies and practical applications of aptamers. In this review, we comprehensively discussed the recent progress in the development of SELEX technology and aptamer-based applied research in various types of infectious diseases.
Up to now, aptamers were intensively integrated into the biosensor strategies as molecular recognition elements. Compared with conventional diagnosis methods, aptamers-based biosensors strategies had obvious advancement in sensitivity and reliability which could improve diagnostic performance, thus lead to intervention at an earlier stage and avoid the spread of infectious diseases. Furthermore, as a class of single-stranded nucleic acid, aptamers showed outstanding advantages on cost and manufacturability, so that the development of aptamer-based biosensors could be conducive promote the popularization and improvement of infectious diseases diagnosis techniques in community hospitals. Last but not the least, the portability makes biosensors an alternative to traditional methods in point-of-care diagnostics and even more diverse medical settings such as epidemic areas. Aptamers could be also applied in biotherapy and drug-delivery systems. Due to low immunogenicity and high targeting ability, aptamer-based therapy could increase the drug concentration in local lesions, thus improve the therapeutic effect and reduce the toxic and side effects of drugs. Aptamers were also intelligent in solving problems of antimicrobial resistance and viral genomes mutation variability. Therefore, aptamers are expected to be promising in the therapeutic of infectious diseases.
However, there remain several challenges limiting the clinical application of aptamers. Firstly, aptamers for some complex pathogens are still limited because of the limitations of the current SELEX technology. Nevertheless, those problems can be ameliorated by optimizing the critical factors in the SELEX process in near future, such as the concentration of target molecules, the separation method of aptamers, PCR reaction conditions, the number of screening rounds and so on. Besides, the biostability and toxicity of aptamers, as well as the degradation of unmodified aptamers by nuclease in serum and rapid removal by renal filtration remain to be explored. The side effects caused by the metabolism of aptamer are also a problem to be reckoned with. Therefore, significant refinements of biochemical modification and rigorous administration routines of aptamer-based therapy are still needed in future research. Fortunately, in spite of all the challenges mentions above, the transition from aptamer-based basic research to clinical application is taking place, although slowly.
Overall, we foresee a promising prospect for aptamer-based technologies in precision medicine of infectious diseases. Shortly, with many research and development activities going on in this field, we envision that practical and commercial biosensors and novel drugs for clinical diagnosis and precise therapy are very close to realization, and consequently, that will significantly reduce the human diseases and economic burdens.
Author Contributions
YX and XJ wrote the manuscript. YZ and MM contributed to the literature research. MW and BY revised and approved the manuscript. All authors have read and agreed to the published version of the manuscript.
Funding
This work was supported by the National Natural Science Foundation of China (Grant numbers 81672095); National Science and Technology Major Project of the Ministry of Science and Technology of China (Grant numbers 2018ZX10715,003-001).
Conflict of Interest
The authors declare that the research was conducted in the absence of any commercial or financial relationships that could be construed as a potential conflict of interest.
Publisher’s Note
All claims expressed in this article are solely those of the authors and do not necessarily represent those of their affiliated organizations, or those of the publisher, the editors and the reviewers. Any product that may be evaluated in this article, or claim that may be made by its manufacturer, is not guaranteed or endorsed by the publisher.
References
Aeksiri, N., Songtawee, N., Gleeson, M. P., Hannongbua, S., and Choowongkomon, K. (2014). Insight into HIV-1 Reverse Transcriptase-Aptamer Interaction from Molecular Dynamics Simulations. J. Mol. Model. 20 (8), 2380. doi:10.1007/s00894-014-2380-8
Andini, N., Hu, A., Zhou, L., Cogill, S., Wang, T.-H., Wittwer, C. T., et al. (2018). A "Culture" Shift: Broad Bacterial Detection, Identification, and Antimicrobial Susceptibility Testing Directly from Whole Blood. Clin. Chem. 64 (10), 1453–1462. doi:10.1373/clinchem.2018.290189
Ansari, N., Ghazvini, K., Ramezani, M., Shahdordizadeh, M., Yazdian-Robati, R., Abnous, K., et al. (2017). Selection of DNA Aptamers against Mycobacterium tuberculosis Ag85A, and its Application in a Graphene Oxide-Based Fluorometric Assay. Microchim Acta 185 (1), 21. doi:10.1007/s00604-017-2550-3
Arvanitis, M., Anagnostou, T., Fuchs, B. B., Caliendo, A. M., and Mylonakis, E. (2014). Molecular and Nonmolecular Diagnostic Methods for Invasive Fungal Infections. Clin. Microbiol. Rev. 27 (3), 490–526. doi:10.1128/CMR.00091-13
Asif, M., and Orenstein, Y. (2020). DeepSELEX: Inferring DNA-Binding Preferences from HT-SELEX Data Using Multi-Class CNNs. Bioinformatics 36 (Suppl. l_2), i634–i642. doi:10.1093/bioinformatics/btaa789
Babamiri, B., Salimi, A., and Hallaj, R. (2018). A Molecularly Imprinted Electrochemiluminescence Sensor for Ultrasensitive HIV-1 Gene Detection Using EuS Nanocrystals as Luminophore. Biosens. Bioelectron. 117, 332–339. doi:10.1016/j.bios.2018.06.003
Balmaseda, A., Stettler, K., Medialdea-Carrera, R., Collado, D., Jin, X., Zambrana, J. V., et al. (2017). Antibody-based Assay Discriminates Zika Virus Infection from Other Flaviviruses. Proc. Natl. Acad. Sci. USA 114 (31), 8384–8389. doi:10.1073/pnas.1704984114
Barthelmebs, L., Jonca, J., Hayat, A., Prieto-Simon, B., and Marty, J.-L. (2011). Enzyme-Linked Aptamer Assays (ELAAs), Based on a Competition Format for a Rapid and Sensitive Detection of Ochratoxin A in Wine. Food Control 22 (5), 737–743. doi:10.1016/j.foodcont.2010.11.005
Bellecave, P., Cazenave, C., Rumi, J., Staedel, C., Cosnefroy, O., Andreola, M.-L., et al. (2008). Inhibition of Hepatitis C Virus (HCV) RNA Polymerase by DNA Aptamers: Mechanism of Inhibition of In Vitro RNA Synthesis and Effect on HCV-Infected Cells. Antimicrob. Agents Chemother. 52 (6), 2097–2110. doi:10.1128/AAC.01227-07
Bin Dajem, S. M. (2015). Molecular Investigation of Mixed Malaria Infections in Southwest Saudi Arabia. Saudi Med. J. 36 (2), 248–251. doi:10.15537/smj.2015.2.10874
Bitaraf, F. S., Rasooli, I., and Mousavi Gargari, S. L. (2016). DNA Aptamers for the Detection of Haemophilus Influenzae Type B by Cell SELEX. Eur. J. Clin. Microbiol. Infect. Dis. 35 (3), 503–510. doi:10.1007/s10096-015-2567-7
Boussebayle, A., Groher, F., and Suess, B. (2019a). RNA-based Capture-SELEX for the Selection of Small Molecule-Binding Aptamers. Methods 161, 10–15. doi:10.1016/j.ymeth.2019.04.004
Boussebayle, A., Torka, D., Ollivaud, S., Braun, J., Bofill-Bosch, C., Dombrowski, M., et al. (2019b). Next-level Riboswitch Development-Implementation of Capture-SELEX Facilitates Identification of a New Synthetic Riboswitch. Nucleic Acids Res. 47 (9), 4883–4895. doi:10.1093/nar/gkz216
Breaker, R. (1997). DNA Aptamers and DNA Enzymes. Curr. Opin. Chem. Biol. 1 (1), 26–31. doi:10.1016/s1367-5931(97)80105-6
Bruno, J. G., Richarte, A. M., Phillips, T., Savage, A. A., Sivils, J. C., Greis, A., et al. (2014). Development of a Fluorescent Enzyme-Linked DNA Aptamer-Magnetic Bead sandwich Assay and Portable Fluorometer for Sensitive and Rapid Leishmania Detection in Sandflies. J. Fluoresc 24 (1), 267–277. doi:10.1007/s10895-013-1315-6
Burmeister, P. E., Lewis, S. D., Silva, R. F., Preiss, J. R., Horwitz, L. R., Pendergrast, P. S., et al. (2005). Direct In Vitro Selection of a 2′-O-Methyl Aptamer to VEGF. Chem. Biol. 12 (1), 25–33. doi:10.1016/j.chembiol.2004.10.017
Byrne, B., Stack, E., Gilmartin, N., and O’Kennedy, R. (2009). Antibody-based Sensors: Principles, Problems and Potential for Detection of Pathogens and Associated Toxins. Sensors 9 (6), 4407–4445. doi:10.3390/s90604407
Cai, R., Yin, F., Zhang, Z., Tian, Y., and Zhou, N. (2019). Functional Chimera Aptamer and Molecular beacon Based Fluorescent Detection of Staphylococcus aureus with Strand Displacement-Target Recycling Amplification. Analytica Chim. Acta 1075, 128–136. doi:10.1016/j.aca.2019.05.014
Cella, L. N., Sanchez, P., Zhong, W., Myung, N. V., Chen, W., and Mulchandani, A. (2010). Nano Aptasensor for Protective Antigen Toxin of Anthrax. Anal. Chem. 82 (5), 2042–2047. doi:10.1021/ac902791q
Chand, R., and Neethirajan, S. (2017). Microfluidic Platform Integrated with Graphene-Gold Nano-Composite Aptasensor for One-step Detection of Norovirus. Biosens. Bioelectron. 98, 47–53. doi:10.1016/j.bios.2017.06.026
Chen, L., He, W., Jiang, H., Wu, L., Xiong, W., Li, B., et al. (2019). In Vivo SELEX of Bone Targeting Aptamer in Prostate Cancer Bone Metastasis Model. Int. J. Nanomedicine 14, 149–159. doi:10.2147/IJN.S188003
Chen, L., Rashid, F., Shah, A., Awan, H. M., Wu, M., Liu, A., et al. (2015). The Isolation of an RNA Aptamer Targeting to P53 Protein with Single Amino Acid Mutation. Proc. Natl. Acad. Sci. USA 112 (32), 10002–10007. doi:10.1073/pnas.1502159112
Chonco, L., Fernández, G., Kalhapure, R., Hernáiz, M. J., García-Oliva, C., Gonzalez, V. M., et al. (2018). Novel DNA Aptamers against CCL21 Protein: Characterization and Biomedical Applications for Targeted Drug Delivery to T Cell-Rich Zones. Nucleic Acid Ther. 28 (4), 242–251. doi:10.1089/nat.2017.0689
Cowperthwaite, M. C., and Ellington, A. D. (2008). Bioinformatic Analysis of the Contribution of Primer Sequences to Aptamer Structures. J. Mol. Evol. 67 (1), 95–102. doi:10.1007/s00239-008-9130-4
Daniels, D. A., Chen, H., Hicke, B. J., Swiderek, K. M., and Gold, L. (2003). A Tenascin-C Aptamer Identified by Tumor Cell SELEX: Systematic Evolution of Ligands by Exponential Enrichment. Proc. Natl. Acad. Sci. 100 (26), 15416–15421. doi:10.1073/pnas.2136683100
Dao, P., Hoinka, J., Takahashi, M., Zhou, J., Ho, M., Wang, Y., et al. (2016). AptaTRACE Elucidates RNA Sequence-Structure Motifs from Selection Trends in HT-SELEX Experiments. Cel Syst. 3 (1), 62–70. doi:10.1016/j.cels.2016.07.003
Del Rio, C., Mehta, A. K., Lyon, G. M., and Guarner, J. (2014). Ebola Hemorrhagic Fever in 2014: the Tale of an Evolving Epidemic. Ann. Intern. Med. 161 (10), 746–748. doi:10.7326/M14-1880
Dey, A. K., Griffiths, C., Lea, S. M., and James, W. (2005). Structural Characterization of an Anti-gp120 RNA Aptamer that Neutralizes R5 Strains of HIV-1. RNA 11 (6), 873–884. doi:10.1261/rna.7205405
Dittmar, K. A., Jiang, P., Park, J. W., Amirikian, K., Wan, J., Shen, S., et al. (2012). Genome-wide Determination of a Broad ESRP-Regulated Posttranscriptional Network by High-Throughput Sequencing. Mol. Cel Biol 32 (8), 1468–1482. doi:10.1128/MCB.06536-11
Ditzler, M. A., Lange, M. J., Bose, D., Bottoms, C. A., Virkler, K. F., Sawyer, A. W., et al. (2013). High-throughput Sequence Analysis Reveals Structural Diversity and Improved Potency Among RNA Inhibitors of HIV Reverse Transcriptase. Nucleic Acids Res. 41 (3), 1873–1884. doi:10.1093/nar/gks1190
Dong, L., Tan, Q., Ye, W., Liu, D., Chen, H., Hu, H., et al. (2015). Screening and Identifying a Novel ssDNA Aptamer against Alpha-Fetoprotein Using CE-SELEX. Sci. Rep. 5, 15552. doi:10.1038/srep15552
Drolet, D. W., Nelson, J., Tucker, C. E., Zack, P. M., Nixon, K., Bolin, R., et al. (2000). Pharmacokinetics and Safety of an Anti-vascular Endothelial Growth Factor Aptamer (NX1838) Following Injection into the Vitreous Humor of Rhesus Monkeys. Pharm. Res. 17 (12), 1503–1510. doi:10.1023/a:1007657109012
Duan, N., Gong, W., Wu, S., and Wang, Z. (2017). An ssDNA Library Immobilized SELEX Technique for Selection of an Aptamer against Ractopamine. Analytica Chim. Acta 961, 100–105. doi:10.1016/j.aca.2017.01.008
Duan, N., Wu, S., Chen, X., Huang, Y., and Wang, Z. (2012). Selection and Identification of a DNA Aptamer Targeted to Vibrio Parahemolyticus. J. Agric. Food Chem. 60 (16), 4034–4038. doi:10.1021/jf300395z
Duan, N., Wu, S., Chen, X., Huang, Y., Xia, Y., Ma, X., et al. (2013). Selection and Characterization of Aptamers against Salmonella typhimurium Using Whole-Bacterium Systemic Evolution of Ligands by Exponential Enrichment (SELEX). J. Agric. Food Chem. 61 (13), 3229–3234. doi:10.1021/jf400767d
Duan, Y., Gao, Z., Wang, L., Wang, H., Zhang, H., and Li, H. (2016). Selection and Identification of Chloramphenicol-specific DNA Aptamers by Mag-SELEX. Appl. Biochem. Biotechnol. 180 (8), 1644–1656. doi:10.1007/s12010-016-2193-6
Dunning, J., Baillie, J. K., Cao, B., Hayden, F. G., and I, I. S. A. R. E. (2014a). Antiviral Combinations for Severe Influenza. Lancet Infect. Dis. 14 (12), 1259–1270. doi:10.1016/S1473-3099(14)70821-7
Dunning, J., Baillie, J. K., Cao, B., Hayden, F. G., International Severe Acute, R., and Emerging Infection, C. (2014b). Antiviral Combinations for Severe Influenza. Lancet Infect. Dis. 14 (12), 1259–1270. doi:10.1016/S1473-3099(14)70821-7
Dwivedi, H. P., Smiley, R. D., and Jaykus, L.-A. (2013). Selection of DNA Aptamers for Capture and Detection of Salmonella Typhimurium Using a Whole-Cell SELEX Approach in Conjunction with Cell Sorting. Appl. Microbiol. Biotechnol. 97 (8), 3677–3686. doi:10.1007/s00253-013-4766-4
Ellington, A. D., and Szostak, J. W. (1990). In Vitro selection of RNA Molecules that Bind Specific Ligands. Nature 346 (6287), 818–822. doi:10.1038/346818a0
Escadafal, C., Faye, O., Sall, A. A., Faye, O., Weidmann, M., Strohmeier, O., et al. (2014). Rapid Molecular Assays for the Detection of Yellow Fever Virus in Low-Resource Settings. Plos Negl. Trop. Dis. 8 (3), e2730. doi:10.1371/journal.pntd.0002730
Fall-Malick, F.-Z., Tchiakpé, E., Ould Soufiane, S. A., Diop-Ndiaye, H., Mouhamedoune Baye, A., Ould Horma Babana, A., et al. (2014). Drug Resistance Mutations and Genetic Diversity in Adults Treated for HIV Type 1 Infection in Mauritania. J. Med. Virol. 86 (3), 404–410. doi:10.1002/jmv.23860
Fan, L., Wang, T., Hua, C., Sun, W., Li, X., Grunwald, L., et al. (2020). A Compendium of DNA-Binding Specificities of Transcription Factors in Pseudomonas syringae. Nat. Commun. 11 (1), 4947. doi:10.1038/s41467-020-18744-7
Fattal, E., Hillaireau, H., and Ismail, S. I. (2018). Aptamers in Therapeutics and Drug Delivery. Adv. Drug Deliv. Rev. 134, 1–2. doi:10.1016/j.addr.2018.11.001
Fauci, A. S., Touchette, N. A., and Folkers, G. K. (2005). Emerging Infectious Diseases: a 10-year Perspective from the National Institute of Allergy and Infectious Diseases. Emerg. Infect. Dis. 11 (4), 519–525. doi:10.3201/eid1104.041167
Férir, G., Gordts, S., and Schols, D. (2014). HIV-1 and its Resistance to Peptidic Carbohydrate-Binding Agents (CBAs): an Overview. Molecules 19 (12), 21085–21112. doi:10.3390/molecules191221085
Finlay, B. B., and McFadden, G. (2006). Anti-immunology: Evasion of the Host Immune System by Bacterial and Viral Pathogens. Cell 124 (4), 767–782. doi:10.1016/j.cell.2006.01.034
Fisher, M. E., Rossini, M., Simmons, E., Harris, R. C., Moeckel, G., and Zent, R. (2004). A Woman with Chronic Hepatitis C Infection and Nephrotic Syndrome Who Developed Multiple Renal Lesions after Interferon Alfa Therapy. Am. J. Kidney Dis. 44 (3), 567–573. doi:10.1016/s0272-6386(04)00828-5
Fisher, R. I., Jaffe, E. S., Braylan, R. C., Andersen, J. C., and Tan, H. K. (1976). Immunoblastic Lymphadenopathy. Am. J. Med. 61 (4), 553–559. doi:10.1016/0002-9343(76)90337-5
Frezza, V., Pinto-Díez, C., Fernández, G., Soto, M., Martín, M. E., García-Sacristán, A., et al. (2020). DNA Aptamers Targeting Leishmania Infantum H3 Protein as Potential Diagnostic Tools. Analytica Chim. Acta 1107, 155–163. doi:10.1016/j.aca.2020.02.012
Fried, M. W. (2002). Side Effects of Therapy of Hepatitis C and Their Management. Hepatology 36 (5 Suppl. 1), S237–s244. doi:10.1053/jhep.2002.3681010.1002/hep.1840360730
Fu, P., Sun, Z., Yu, Z., Zhang, Y., Shen, J., Zhang, H., et al. (2014). Enzyme Linked Aptamer Assay: Based on a Competition Format for Sensitive Detection of Antibodies to Mycoplasma Bovis in Serum. Anal. Chem. 86 (3), 1701–1709. doi:10.1021/ac4042203
Fu, P., Wang, F., Zhang, Y., Qiao, X., Zhang, Y., Zhou, W., et al. (2021a). The Application of Aptamer Apt-236 Targeting PvpA Protein in the Detection of Antibodies against Mycoplasma Gallisepticum. Anal. Methods 13 (27), 3068–3076. doi:10.1039/d1ay00515d
Fu, P., Wang, F., Zhang, Y., Qiao, X., Zhang, Y., Zhou, W., et al. (2021b). The Application of Aptamer Apt-236 Targeting PvpA Protein in the Detection of Antibodies against Mycoplasma Gallisepticum. Anal. Methods 13, 3068–3076. doi:10.1039/d1ay00515d
Gao, T., Ding, P., Li, W., Wang, Z., Lin, Q., and Pei, R. (2020). Isolation of DNA Aptamers Targeting N-Cadherin and High-Efficiency Capture of Circulating Tumor Cells by Using Dual Aptamers. Nanoscale 12 (44), 22574–22585. doi:10.1039/d0nr06180h
Geary, R. S., Yu, R. Z., Watanabe, T., Henry, S. P., Hardee, G. E., Chappell, A., et al. (2003). Pharmacokinetics of a Tumor Necrosis Factor-Alpha Phosphorothioate 2’-O-(2-Methoxyethyl) Modified Antisense Oligonucleotide: Comparison across Species. Drug Metab. Dispos 31 (11), 1419–1428. doi:10.1124/dmd.31.11.1419
Giri, B. R., Mahato, R. I., and Cheng, G. (2019). Roles of microRNAs in T Cell Immunity: Implications for Strategy Development against Infectious Diseases. Med. Res. Rev. 39 (2), 706–732. doi:10.1002/med.21539
Gopinath, S. C. B., Hayashi, K., and Kumar, P. K. R. (2012). Aptamer that Binds to the gD Protein of Herpes Simplex Virus 1 and Efficiently Inhibits Viral Entry. J. Virol. 86 (12), 6732–6744. doi:10.1128/JVI.00377-12
Gull, I., Aslam, M. S., Tipu, I., Mushtaq, R., Ali, T. Z., and Athar, M. A. (2019). Development of Latent Interferon Alpha 2b as a Safe Therapeutic for Treatment of Hepatitis C Virus Infection. Sci. Rep. 9 (1), 10867. doi:10.1038/s41598-019-47074-y
Guo, P. (2010). The Emerging Field of RNA Nanotechnology. Nat. Nanotech 5 (12), 833–842. doi:10.1038/nnano.2010.231
Guo, Y., Zhao, C., Liu, Y., Nie, H., Guo, X., Song, X., et al. (2020). A Novel Fluorescence Method for the Rapid and Effective Detection of Listeria Monocytogenes Using Aptamer-Conjugated Magnetic Nanoparticles and Aggregation-Induced Emission Dots. Analyst 145 (11), 3857–3863. doi:10.1039/d0an00397b
Habyarimana, F., and Ramroop, S. (2020). Prevalence and Risk Factors Associated with Malaria Among Children Aged Six Months to 14 Years Old in Rwanda: Evidence from 2017 Rwanda Malaria Indicator Survey. Int. J. Environ. Res. Public Health 17 (21), 7975. doi:10.3390/ijerph17217975
Hahn, U. (2017). Charomers-Interleukin-6 Receptor Specific Aptamers for Cellular Internalization and Targeted Drug Delivery. Int. J. Mol. Sci. 18 (12), 2641. doi:10.3390/ijms18122641
Han, B., Fang, C., Sha, L., Jalalah, M., Al-Assiri, M. S., Harraz, F. A., et al. (2021). Cascade Strand Displacement Reaction-Assisted Aptamer-Based Highly Sensitive Detection of Ochratoxin A. Food Chem. 338, 127827. doi:10.1016/j.foodchem.2020.127827
Han, X., Zhang, Y., Nie, J., Zhao, S., Tian, Y., and Zhou, N. (2017). Gold Nanoparticle Based Photometric Determination of Tobramycin by Using New Specific DNA Aptamers. Microchim Acta 185 (1), 4. doi:10.1007/s00604-017-2568-6
He, J., Wang, J., Zhang, N., Shen, L., Wang, L., Xiao, X., et al. (2019). In Vitro selection of DNA Aptamers Recognizing Drug-Resistant Ovarian Cancer by Cell-SELEX. Talanta 194, 437–445. doi:10.1016/j.talanta.2018.10.028
Heo, K., Min, S.-W., Sung, H. J., Kim, H. G., Kim, H. J., Kim, Y. H., et al. (2016). An Aptamer-Antibody Complex (Oligobody) as a Novel Delivery Platform for Targeted Cancer Therapies. J. Controlled Release 229, 1–9. doi:10.1016/j.jconrel.2016.03.006
Homann, M., Lorger, M., Engstler, M., Zacharias, M., and Göringer, H. U. (2006). Serum-stable RNA Aptamers to an Invariant Surface Domain of Live African Trypanosomes. Comb. Chem. High Throughput Screen. 9 (7), 491–499. doi:10.2174/138620706777935324
Hong, K. L., and Sooter, L. J. (2015). Single-Stranded DNA Aptamers against Pathogens and Toxins: Identification and Biosensing Applications. Biomed. Res. Int. 2015, 1–31. doi:10.1155/2015/419318
Hong, S.-L., Xiang, M.-Q., Tang, M., Pang, D.-W., and Zhang, Z.-L. (2019). Ebola Virus Aptamers: From Highly Efficient Selection to Application on Magnetism-Controlled Chips. Anal. Chem. 91 (5), 3367–3373. doi:10.1021/acs.analchem.8b04623
Hünniger, T., Wessels, H., Fischer, C., Paschke-Kratzin, A., and Fischer, M. (2014). Just in Time-Selection: A Rapid Semiautomated SELEX of DNA Aptamers Using Magnetic Separation and BEAMing. Anal. Chem. 86 (21), 10940–10947. doi:10.1021/ac503261b
Hwang, S.-Y., Sun, H.-Y., Lee, K.-H., Oh, B.-H., Cha, Y. J., Kim, B. H., et al. (2012). 5′-Triphosphate-RNA-independent Activation of RIG-I via RNA Aptamer with Enhanced Antiviral Activity. Nucleic Acids Res. 40 (6), 2724–2733. doi:10.1093/nar/gkr1098
Ishida, R., Adachi, T., Yokota, A., Yoshihara, H., Aoki, K., Nakamura, Y., et al. (2020). RaptRanker: In Silico RNA Aptamer Selection from HT-SELEX experiment Based on Local Sequence and Structure Information. Nucleic Acids Res. 48 (14), e82. doi:10.1093/nar/gkaa484
Jayasena, S. D. (1999). Aptamers: an Emerging Class of Molecules that Rival Antibodies in Diagnostics. Clin. Chem. 45 (9), 1628–1650. doi:10.1093/clinchem/45.9.1628
Jing, M., and Bowser, M. T. (2013). Tracking the Emergence of High Affinity Aptamers for rhVEGF165 during Capillary Electrophoresis-Systematic Evolution of Ligands by Exponential Enrichment Using High Throughput Sequencing. Anal. Chem. 85 (22), 10761–10770. doi:10.1021/ac401875h
Kang, J., Yeom, G., Jang, H., Oh, J., Park, C.-J., and Kim, M.-G. (2019). Development of Replication Protein A-Conjugated Gold Nanoparticles for Highly Sensitive Detection of Disease Biomarkers. Anal. Chem. 91 (15), 10001–10007. doi:10.1021/acs.analchem.9b01827
Kim, Y. S., Song, M. Y., Jurng, J., and Kim, B. C. (2013). Isolation and Characterization of DNA Aptamers against Escherichia coli Using a Bacterial Cell-Systematic Evolution of Ligands by Exponential Enrichment Approach. Anal. Biochem. 436 (1), 22–28. doi:10.1016/j.ab.2013.01.014
Kinghorn, A., Fraser, L., Liang, S., Shiu, S., and Tanner, J. (2017). Aptamer Bioinformatics. Int. J. Mol. Sci. 18 (12), 2516. doi:10.3390/ijms18122516
Labella, A. M., and Merel, S. E. (2013). Influenza. Med. Clin. North America 97 (4), 621–645, x. doi:10.1016/j.mcna.2013.03.001
Lai, H.-C., Wang, C.-H., Liou, T.-M., and Lee, G.-B. (2014). Influenza A Virus-specific Aptamers Screened by Using an Integrated Microfluidic System. Lab. Chip 14 (12), 2002–2013. doi:10.1039/c4lc00187g
Lai, J.-C., and Hong, C.-Y. (2014). A Novel Protocol for Generating High-Affinity ssDNA Aptamers by Using Alternating Magnetic fields. J. Mater. Chem. B 2 (26), 4114–4121. doi:10.1039/c3tb21729a
Lakhin, A. V., Tarantul, V. Z., and Gening, L. V. (2013). Aptamers: Problems, Solutions and Prospects. Acta Naturae 5 (4), 34–43. doi:10.32607/20758251-2013-5-4-34-43
Lampel, K. A., Orlandi, P. A., and Kornegay, L. (2000). Improved Template Preparation for PCR-Based Assays for Detection of Food-Borne Bacterial Pathogens. Appl. Environ. Microbiol. 66 (10), 4539–4542. doi:10.1128/AEM.66.10.4539-4542.2000
Lauridsen, L. H., Doessing, H. B., Long, K. S., and Nielsen, A. T. (2018). A Capture-SELEX Strategy for Multiplexed Selection of RNA Aptamers against Small Molecules. Methods Mol. Biol. 1671, 291–306. doi:10.1007/978-1-4939-7295-1_18
Lazarevic, I. (2014). Clinical Implications of Hepatitis B Virus Mutations: Recent Advances. World J. Gastroenterol. 20 (24), 7653–7664. doi:10.3748/wjg.v20.i24.7653
Leblebici, P., Leirs, K., Spasic, D., and Lammertyn, J. (2019). Encoded Particle Microfluidic Platform for Rapid Multiplexed Screening and Characterization of Aptamers against Influenza A Nucleoprotein. Analytica Chim. Acta 1053, 70–80. doi:10.1016/j.aca.2018.11.055
Lee, C. H., Lee, S. H., Kim, J. H., Noh, Y. H., Noh, G. J., and Lee, S. W. (2015). Pharmacokinetics of a Cholesterol-Conjugated Aptamer against the Hepatitis C Virus (HCV) NS5B Protein. Mol. Therapy-Nucleic Acids 4, e254. doi:10.1038/mtna.2015.30
Lee, S., Song, K.-M., Jeon, W., Jo, H., Shim, Y.-B., and Ban, C. (2012). A Highly Sensitive Aptasensor towards Plasmodium Lactate Dehydrogenase for the Diagnosis of Malaria. Biosens. Bioelectron. 35 (1), 291–296. doi:10.1016/j.bios.2012.03.003
Li, G., Zhou, L., Zhang, C., Shi, Y., Dong, D., Bai, M., et al. (2019). Insulin-Like Growth Factor 1 Regulates Acute Inflammatory Lung Injury Mediated by Influenza Virus Infection. Front. Microbiol. 10, 2541. doi:10.3389/fmicb.2019.02541
Lin, C. H., and Patei, D. J. (1997). Structural Basis of DNA Folding and Recognition in an AMP-DNA Aptamer Complex: Distinct Architectures but Common Recognition Motifs for DNA and RNA Aptamers Complexed to AMP. Chem. Biol. 4 (11), 817–832. doi:10.1016/s1074-5521(97)90115-0
Lin, H.-I., Wu, C.-C., Yang, C.-H., Chang, K.-W., Lee, G.-B., and Shiesh, S.-C. (2015). Selection of Aptamers Specific for Glycated Hemoglobin and Total Hemoglobin Using On-Chip SELEX. Lab. Chip 15 (2), 486–494. doi:10.1039/c4lc01124d
Lin, N., Wu, L., Xu, X., Wu, Q., Wang, Y., Shen, H., et al. (2021). Aptamer Generated by Cell-SELEX for Specific Targeting of Human Glioma Cells. ACS Appl. Mater. Inter. 13 (8), 9306–9315. doi:10.1021/acsami.0c11878
Lincoff, A. M., Mehran, R., Povsic, T. J., Zelenkofske, S. L., Huang, Z., Armstrong, P. W., et al. (2016). Effect of the REG1 Anticoagulation System versus Bivalirudin on Outcomes after Percutaneous Coronary Intervention (REGULATE-PCI): a Randomised Clinical Trial. The Lancet 387 (10016), 349–356. doi:10.1016/S0140-6736(15)00515-2
Liu, M., Li, X., Li, B., Du, J., and Yang, Z. (2019a). A Fluorometric Aptamer-Based Assay for Ochratoxin A by Using Exonuclease III-Assisted Recycling Amplification. Microchim Acta 187 (1), 46. doi:10.1007/s00604-019-3992-6
Liu, R., He, L., Hu, Y., Luo, Z., and Zhang, J. (2020). A Serological Aptamer-Assisted Proximity Ligation Assay for COVID-19 Diagnosis and Seeking Neutralizing Aptamers. Chem. Sci. 11 (44), 12157–12164. doi:10.1039/d0sc03920a
Liu, W. J., Zhao, M., Liu, K., Xu, K., Wong, G., Tan, W., et al. (2017). T-cell Immunity of SARS-CoV: Implications for Vaccine Development against MERS-CoV. Antiviral Res. 137, 82–92. doi:10.1016/j.antiviral.2016.11.006
Liu, X., Wang, Y. l., Wu, J., Qi, J., Zeng, Z., Wan, Q., et al. (2021). Neutralizing Aptamers Block S/RBD‐ACE2 Interactions and Prevent Host Cell Infection. Angew. Chem. 133 (18), 10361–10366. doi:10.1002/ange.202100345
Liu, Y., Jiang, W., Yang, S., Hu, J., Lu, H., Han, W., et al. (2019b). Rapid Detection of Mycoplasma-Infected Cells by an ssDNA Aptamer Probe. ACS Sens. 4 (8), 2028–2038. doi:10.1021/acssensors.9b00582
Lou, X., Qian, J., Xiao, Y., Viel, L., Gerdon, A. E., Lagally, E. T., et al. (2009). Micromagnetic Selection of Aptamers in Microfluidic Channels. Proc. Natl. Acad. Sci. 106 (9), 2989–2994. doi:10.1073/pnas.0813135106
Lycett, S. J., Pohlmann, A., Staubach, C., Caliendo, V., Woolhouse, M., Beer, M., et al. (2020). Genesis and Spread of Multiple Reassortants during the 2016/2017 H5 Avian Influenza Epidemic in Eurasia. Proc. Natl. Acad. Sci. USA 117 (34), 20814–20825. doi:10.1073/pnas.2001813117
Ma, X., Wang, W., Chen, X., Xia, Y., Wu, S., Duan, N., et al. (2014). Selection, Identification, and Application of Aflatoxin B1 Aptamer. Eur. Food Res. Technol. 238 (6), 919–925. doi:10.1007/s00217-014-2176-1
Ma, X., Xu, H., Shi, L., Yang, P., Zhang, L., Sun, X., et al. (2015). A Multiplex PCR Assay for the Detection of Five Influenza Viruses Using a Dual Priming Oligonucleotide System. BMC Infect. Dis. 15, 93. doi:10.1186/s12879-015-0818-y
Ma, Y., Li, X., Li, W., and Liu, Z. (2018). Glycan-Imprinted Magnetic Nanoparticle-Based SELEX for Efficient Screening of Glycoprotein-Binding Aptamers. ACS Appl. Mater. Inter. 10 (47), 40918–40926. doi:10.1021/acsami.8b14441
Ma, Y., and Ma, J. (2020). Immunotherapy against Prion Disease. Pathogens 9 (3), 216. doi:10.3390/pathogens9030216
Manns, M. P., McHutchison, J. G., Gordon, S. C., Rustgi, V. K., Shiffman, M., Reindollar, R., et al. (2001). Peginterferon Alfa-2b Plus Ribavirin Compared with Interferon Alfa-2b Plus Ribavirin for Initial Treatment of Chronic Hepatitis C: a Randomised Trial. The Lancet 358 (9286), 958–965. doi:10.1016/s0140-6736(01)06102-5
Marascio, N., Torti, C., Liberto, M., and Focà, A. (2014). Update on Different Aspects of HCV Variability: Focus on NS5B Polymerase. BMC Infect. Dis. 14 (Suppl. 5), S1. doi:10.1186/1471-2334-14-S5-S1
McKeague, M., Bradley, C. R., Girolamo, A. D., Visconti, A., Miller, J. D., and Derosa, M. C. (2010). Screening and Initial Binding Assessment of Fumonisin B1 Aptamers. Int. J. Mol. Sci. 11 (12), 4864–4881. doi:10.3390/ijms11124864
Mendonsa, S. D., and Bowser, M. T. (2004a). In Vitro evolution of Functional DNA Using Capillary Electrophoresis. J. Am. Chem. Soc. 126 (1), 20–21. doi:10.1021/ja037832s
Mendonsa, S. D., and Bowser, M. T. (2004b). In Vitro selection of High-Affinity DNA Ligands for Human IgE Using Capillary Electrophoresis. Anal. Chem. 76 (18), 5387–5392. doi:10.1021/ac049857v
Mikhail, A. F., Leslie, T. J., Mayan, M. I., Zekria, R., Mohammad, N., Hasanzai, M. A., et al. (2011). Field Trial of Three Different Plasmodium Vivax- Detecting Rapid Diagnostic Tests with and without Evaporative Cool Box Storage in Afghanistan. Malar. J. 10, 169. doi:10.1186/1475-2875-10-169
Minopoli, A., Della Ventura, B., Lenyk, B., Gentile, F., Tanner, J. A., Offenhäusser, A., et al. (2020). Ultrasensitive Antibody-Aptamer Plasmonic Biosensor for Malaria Biomarker Detection in Whole Blood. Nat. Commun. 11 (1), 6134. doi:10.1038/s41467-020-19755-0
Morita, Y., Kamal, M., Kang, S.-A., Zhang, R., Lokesh, G. L., Thiviyanathan, V., et al. (2016). E-selectin Targeting PEGylated-Thioaptamer Prevents Breast Cancer Metastases. Mol. Ther. - Nucleic Acids 5 (12), e399. doi:10.1038/mtna.2016.103
Morita, Y., Leslie, M., Kameyama, H., Volk, D., and Tanaka, T. (2018). Aptamer Therapeutics in Cancer: Current and Future. Cancers 10 (3), 80. doi:10.3390/cancers10030080
Mosing, R. K., and Bowser, M. T. (2009). Isolating Aptamers Using Capillary Electrophoresis-SELEX (CE-SELEX). Methods Mol. Biol. 535, 33–43. doi:10.1007/978-1-59745-557-2_3
Mosing, R. K., Mendonsa, S. D., and Bowser, M. T. (2005). Capillary Electrophoresis-SELEX Selection of Aptamers with Affinity for HIV-1 Reverse Transcriptase. Anal. Chem. 77 (19), 6107–6112. doi:10.1021/ac050836q
Mufhandu, H. T., Gray, E. S., Madiga, M. C., Tumba, N., Alexandre, K. B., Khoza, T., et al. (2012). UCLA1, a Synthetic Derivative of a Gp120 RNA Aptamer, Inhibits Entry of Human Immunodeficiency Virus Type 1 Subtype C. J. Virol. 86 (9), 4989–4999. doi:10.1128/Jvi.06893-11
Nagarkatti, R., Bist, V., Sun, S., Fortes de Araujo, F., Nakhasi, H. L., and Debrabant, A. (2012). Development of an Aptamer-Based Concentration Method for the Detection of Trypanosoma Cruzi in Blood. PLoS One 7 (8), e43533. doi:10.1371/journal.pone.0043533
Nang, S. C., Azad, M. A. K., Velkov, T., Zhou, Q., and Li, J. (2021). Rescuing the Last-Line Polymyxins: Achievements and Challenges. Pharmacol. Rev. 73 (2), 679–728. doi:10.1124/pharmrev.120.000020
Negro, F. (2010). Adverse Effects of Drugs in the Treatment of Viral Hepatitis. Best Pract. Res. Clin. Gastroenterol. 24 (2), 183–192. doi:10.1016/j.bpg.2009.10.012
Nguyen, P. D. M., Zheng, J., Gremminger, T. J., Qiu, L., Zhang, D., Tuske, S., et al. (2020). Binding Interface and Impact on Protease Cleavage for an RNA Aptamer to HIV-1 Reverse Transcriptase. Nucleic Acids Res. 48 (5), 2709–2722. doi:10.1093/nar/gkz1224
Nimjee, S. M., Rusconi, C. P., and Sullenger, B. A. (2005). Aptamers: an Emerging Class of Therapeutics. Annu. Rev. Med. 56, 555–583. doi:10.1146/annurev.med.56.062904.144915
Nishimura, S., Miura, H., Yamada, H., Shinoda, T., Kitamura, S., and Miura, Y. (2002). Acute Onset of Nephrotic Syndrome during Interferon-α Retreatment for Chronic Active Hepatitis C. J. Gastroenterol. 37 (10), 854–858. doi:10.1007/s005350200141
Nitta, K. R., Vincentelli, R., Jacox, E., Cimino, A., Ohtsuka, Y., Sobral, D., et al. (2019). High-Throughput Protein Production Combined with High- Throughput SELEX Identifies an Extensive Atlas of Ciona Robusta Transcription Factor DNA-Binding Specificities. Methods Mol. Biol. 2025, 487–517. doi:10.1007/978-1-4939-9624-7_23
Oh, S. S., Qian, J., Lou, X., Zhang, Y., Xiao, Y., and Soh, H. T. (2009). Generation of Highly Specific Aptamers via Micromagnetic Selection. Anal. Chem. 81 (13), 5490–5495. doi:10.1021/ac900759k
Oh, S. S., Ahmad, K. M., Cho, M., Kim, S., Xiao, Y., and Soh, H. T. (2011). Improving Aptamer Selection Efficiency through Volume Dilution, Magnetic Concentration, and Continuous Washing in Microfluidic Channels. Anal. Chem. 83 (17), 6883–6889. doi:10.1021/ac201269f
Ospina, J. D. (2020). Los aptámeros como novedosa herramienta diagnóstica y terapéutica y su potencial uso en parasitología. Biomedica 40 (Suppl. 1), 148–165. doi:10.7705/biomedica.4765
Ospina-Villa, J., López-Camarillo, C., Castañón-Sánchez, C., Soto-Sánchez, J., Ramírez-Moreno, E., and Marchat, L. (2018). Advances on Aptamers against Protozoan Parasites. Genes 9 (12), 584. doi:10.3390/genes9120584
Pan, X., Yang, Y., Li, L., Li, X., Li, Q., Cui, C., et al. (2020). A Bispecific Circular Aptamer Tethering a Built-In Universal Molecular Tag for Functional Protein Delivery. Chem. Sci. 11 (35), 9648–9654. doi:10.1039/d0sc02279a
Pang, K. M., Castanotto, D., Li, H., Scherer, L., and Rossi, J. J. (2018). Incorporation of Aptamers in the Terminal Loop of shRNAs Yields an Effective and Novel Combinatorial Targeting Strategy. Nucleic Acids Res. 46 (1), e6. doi:10.1093/nar/gkx980
Paniel, N., Istamboulié, G., Triki, A., Lozano, C., Barthelmebs, L., and Noguer, T. (2017). Selection of DNA Aptamers against Penicillin G Using Capture-SELEX for the Development of an Impedimetric Sensor. Talanta 162, 232–240. doi:10.1016/j.talanta.2016.09.058
Pazienza, V. (2011). Ophthalmological Complications in Hepatitis C Virus Infection: Side Effect of Interferon Therapy or a Direct Role of HCV?. Biomed. Pharmacother. 65 (4), 317–318. doi:10.1016/j.biopha.2011.04.004
Perra, N. (2021). Non-pharmaceutical Interventions during the COVID-19 Pandemic: A Review. Phys. Rep. 913, 1–52. doi:10.1016/j.physrep.2021.02.001
Qaanei, M., Taheri, R. A., and Eskandari, K. (2021). Electrochemical Aptasensor for Escherichia coli O157:H7 Bacteria Detection Using a Nanocomposite of Reduced Graphene Oxide, Gold Nanoparticles and Polyvinyl Alcohol. Anal. Methods 13 (27), 3101–3109. doi:10.1039/d1ay00563d
Rauch, N., and Nauen, R. (2003). Identification of Biochemical Markers Linked to Neonicotinoid Cross Resistance inBemisia Tabaci (Hemiptera: Aleyrodidae). Arch. Insect Biochem. Physiol. 54 (4), 165–176. doi:10.1002/arch.10114
Reiss, D. J., Howard, F. M., and Mobley, H. L. T. (2012). A Novel Approach for Transcription Factor Analysis Using SELEX with High-Throughput Sequencing (TFAST). PLoS One 7 (8), e42761. doi:10.1371/journal.pone.0042761
Rohr, U.-P., Binder, C., Dieterle, T., Giusti, F., Messina, C. G. M., Toerien, E., et al. (2016). The Value of In Vitro Diagnostic Testing in Medical Practice: A Status Report. PLoS One 11 (3), e0149856. doi:10.1371/journal.pone.0149856
Romero-López, C., Berzal-Herranz, B., Gómez, J., and Berzal-Herranz, A. (2012). An Engineered Inhibitor RNA that Efficiently Interferes with Hepatitis C Virus Translation and Replication. Antiviral Res. 94 (2), 131–138. doi:10.1016/j.antiviral.2012.02.015
Rosenbaum, C. D., Carreiro, S. P., and Babu, K. M. (2012). Here Today, Gone Tomorrow…and Back Again? A Review of Herbal Marijuana Alternatives (K2, Spice), Synthetic Cathinones (Bath Salts), Kratom, Salvia Divinorum, Methoxetamine, and Piperazines. J. Med. Toxicol. 8 (1), 15–32. doi:10.1007/s13181-011-0202-2
Roulet, E., Busso, S., Camargo, A. A., Simpson, A. J. G., Mermod, N., and Bucher, P. (2002). High-throughput SELEX-SAGE Method for Quantitative Modeling of Transcription-Factor Binding Sites. Nat. Biotechnol. 20 (8), 831–835. doi:10.1038/nbt718
Ruan, S., Swamidass, S. J., and Stormo, G. D. (2017). BEESEM: Estimation of Binding Energy Models Using HT-SELEX Data. Bioinformatics 33 (15), 2288–2295. doi:10.1093/bioinformatics/btx191
Ruff, P., Pai, R. B., and Storici, F. (2012). Real-Time PCR-Coupled CE-SELEX for DNA Aptamer Selection. ISRN Mol. Biol. 2012, 1–9. doi:10.5402/2012/939083
Rusconi, C. P., Roberts, J. D., Pitoc, G. A., Nimjee, S. M., White, R. R., Quick, G., et al. (2004). Antidote-mediated Control of an Anticoagulant Aptamer In Vivo. Nat. Biotechnol. 22 (11), 1423–1428. doi:10.1038/nbt1023
Saad, M., Chinerman, D., Tabrizian, M., and Faucher, S. P. (2020). Identification of Two Aptamers Binding to Legionella pneumophila with High Affinity and Specificity. Sci. Rep. 10 (1), 9145. doi:10.1038/s41598-020-65973-3
Sahu, G. K. (2015). Potential Implication of Residual Viremia in Patients on Effective Antiretroviral Therapy. AIDS Res. Hum. Retroviruses 31 (1), 25–35. doi:10.1089/AID.2014.0194
Sarhan, M., El-Bitar, A. M. H., and Hotta, H. (2020). Potent Virucidal Activity of Honeybee "Apis mellifera" Venom against Hepatitis C Virus. Toxicon 188, 55–64. doi:10.1016/j.toxicon.2020.10.014
Schmitz, A., Weber, A., Bayin, M., Breuers, S., Fieberg, V., Famulok, M., et al. (2021). A SARS‐CoV‐2 Spike Binding DNA Aptamer that Inhibits Pseudovirus Infection by an RBD‐Independent Mechanism**. Angew. Chem. Int. Ed. 60 (18), 10279–10285. doi:10.1002/anie.202100316
Shiang, Y.-C., Ou, C.-M., Chen, S.-J., Ou, T.-Y., Lin, H.-J., Huang, C.-C., et al. (2013). Highly Efficient Inhibition of Human Immunodeficiency Virus Type 1 Reverse Transcriptase by Aptamers Functionalized Gold Nanoparticles. Nanoscale 5 (7), 2756–2764. doi:10.1039/c3nr33403a
Shim, B. S. (2011). Current Concepts in Bacterial Sexually Transmitted Diseases. Korean J. Urol. 52 (9), 589–597. doi:10.4111/kju.2011.52.9.589
Shoji, Y., Shimada, J., and Mizushima, Y. (2002). Drug Delivery System to Control Infectious Diseases. Curr. Pharm. Des. 8 (6), 455–465. doi:10.2174/1381612023395934
Singh, N. K., Jain, P., Das, S., and Goswami, P. (2019a). Dye Coupled Aptamer-Captured Enzyme Catalyzed Reaction for Detection of Pan Malaria andP. falciparumSpecies in Laboratory Settings and Instrument-free Paper-Based Platform. Anal. Chem. 91 (6), 4213–4221. doi:10.1021/acs.analchem.9b00670
Singh, N. K., Thungon, P. D., Estrela, P., and Goswami, P. (2019b). Development of an Aptamer-Based Field Effect Transistor Biosensor for Quantitative Detection of Plasmodium Falciparum Glutamate Dehydrogenase in Serum Samples. Biosens. Bioelectron. 123, 30–35. doi:10.1016/j.bios.2018.09.085
Sola, M., Menon, A. P., Moreno, B., Meraviglia-Crivelli, D., Soldevilla, M. M., Cartón-García, F., et al. (2020). Aptamers against Live Targets: Is In Vivo SELEX Finally Coming to the Edge?. Mol. Ther. - Nucleic Acids 21, 192–204. doi:10.1016/j.omtn.2020.05.025
Song, S., Wang, X., Xu, K., Li, Q., Ning, L., and Yang, X. (2019). Selection of Highly Specific Aptamers to Vibrio Parahaemolyticus Using Cell-SELEX Powered by Functionalized Graphene Oxide and Rolling circle Amplification. Analytica Chim. Acta 1052, 153–162. doi:10.1016/j.aca.2018.11.047
Spiga, F. M., Maietta, P., and Guiducci, C. (2015). More DNA-Aptamers for Small Drugs: A Capture-SELEX Coupled with Surface Plasmon Resonance and High-Throughput Sequencing. ACS Comb. Sci. 17 (5), 326–333. doi:10.1021/acscombsci.5b00023
Stoltenburg, R., Nikolaus, N., and Strehlitz, B. (2012). Capture-SELEX: Selection of DNA Aptamers for Aminoglycoside Antibiotics. J. Anal. Methods Chem. 2012, 1–14. doi:10.1155/2012/415697
Sumari, D., Grimberg, B. T., Blankenship, D. A., Mugasa, J., Mugittu, K., Moore, L., et al. (2016). Application of Magnetic Cytosmear for the Estimation of Plasmodium Falciparum Gametocyte Density and Detection of Asexual Stages in Asymptomatic Children. Malar. J. 15, 113. doi:10.1186/s12936-016-1170-4
Takeuchi, N., Segawa, S., Ishiwada, N., Ohkusu, M., Tsuchida, S., Satoh, M., et al. (2018). Capsular Serotyping of Haemophilus Influenzae by Using Matrix-Associated Laser Desorption Ionization-Time of Flight Mass Spectrometry. J. Infect. Chemother. 24 (7), 510–514. doi:10.1016/j.jiac.2018.02.007
Tan, X., Jia, F., Wang, P., and Zhang, K. (2020a). Nucleic Acid-Based Drug Delivery Strategies. J. Controlled Release 323, 240–252. doi:10.1016/j.jconrel.2020.03.040
Tan, Z. M., Lai, G. P., Pandey, M., Srichana, T., Pichika, M. R., Gorain, B., et al. (2020b). Novel Approaches for the Treatment of Pulmonary Tuberculosis. Pharmaceutics 12 (12), 1196. doi:10.3390/pharmaceutics12121196
Thongdee, P., Chaijaroenkul, W., Kuesap, J., and Na-Bangchang, K. (2014). Nested-PCR and a New ELISA-Based NovaLisa Test Kit for Malaria Diagnosis in an Endemic Area of Thailand. Korean J. Parasitol. 52 (4), 377–381. doi:10.3347/kjp.2014.52.4.377
Torabi, R., Ranjbar, R., Halaji, M., and Heiat, M. (2020). Aptamers, the Bivalent Agents as Probes and Therapies for Coronavirus Infections: A Systematic Review. Mol. Cell Probes 53, 101636. doi:10.1016/j.mcp.2020.101636
Tuerk, C., and Gold, L. (1990). Systematic Evolution of Ligands by Exponential Enrichment: RNA Ligands to Bacteriophage T4 DNA Polymerase. Science 249 (4968), 505–510. doi:10.1126/science.2200121
Ucak, S., Sudagidan, M., Borsa, B. A., Mansuroglu, B., and Ozalp, V. C. (2020). Inhibitory Effects of Aptamer Targeted Teicoplanin Encapsulated PLGA Nanoparticles for Staphylococcus aureus Strains. World J. Microbiol. Biotechnol. 36 (5), 69. doi:10.1007/s11274-020-02845-y
Urak, K. T., Shore, S., Rockey, W. M., Chen, S.-J., McCaffrey, A. P., and Giangrande, P. H. (2016). In Vitro RNA SELEX for the Generation of Chemically-Optimized Therapeutic RNA Drugs. Methods 103, 167–174. doi:10.1016/j.ymeth.2016.03.003
van Bel, N., Das, A. T., and Berkhout, B. (2014). In Vivo SELEX of Single-Stranded Domains in the HIV-1 Leader RNA. J. Virol. 88 (4), 1870–1880. doi:10.1128/JVI.02942-13
Van Dyke, M. W., Beyer, M. D., Clay, E., Hiam, K. J., McMurry, J. L., and Xie, Y. (2016). Identification of Preferred DNA-Binding Sites for the Thermus Thermophilus Transcriptional Regulator SbtR by the Combinatorial Approach REPSA. PLoS One 11 (7), e0159408. doi:10.1371/journal.pone.0159408
Wan, Q., Liu, X., Zeng, Z., Chen, Z., Liu, Y., and Zu, Y. (2020). Aptamer Cocktail to Detect Multiple Species of Mycoplasma in Cell Culture. Int. J. Mol. Sci. 21 (11), 3784. doi:10.3390/ijms21113784
Wandtke, T., Woźniak, J., and Kopiński, P. (2015). Aptamers in Diagnostics and Treatment of Viral Infections. Viruses 7 (2), 751–780. doi:10.3390/v7020751
Wang, H., Zhang, Y., Yang, H., Qin, M., Ding, X., Liu, R., et al. (2018). In Vivo SELEX of an Inhibitory NSCLC-specific RNA Aptamer from PEGylated RNA Library. Mol. Ther. - Nucleic Acids 10, 187–198. doi:10.1016/j.omtn.2017.12.003
Wang, Y., Zhang, Y., Chen, J., Wang, M., Zhang, T., Luo, W., et al. (2021). Detection of SARS-CoV-2 and its Mutated Variants via CRISPR-Cas13-Based Transcription Amplification. Anal. Chem. 93 (7), 3393–3402. doi:10.1021/acs.analchem.0c04303
Waring, M. J. (2010). Lipophilicity in Drug Discovery. Expert Opin. Drug Discov. 5 (3), 235–248. doi:10.1517/17460441003605098
Wiersinga, W. J., Rhodes, A., Cheng, A. C., Peacock, S. J., and Prescott, H. C. (2020). Pathophysiology, Transmission, Diagnosis, and Treatment of Coronavirus Disease 2019 (COVID-19). JAMA 324 (8), 782–793. doi:10.1001/jama.2020.12839
Wong, E., and Goldberg, T. (2014). Mipomersen (Kynamro): a Novel Antisense Oligonucleotide Inhibitor for the Management of Homozygous Familial Hypercholesterolemia. P T 39 (2), 119–122.
Woo, C. H., Jang, S., Shin, G., Jung, G. Y., and Lee, J. W. (2020). Sensitive Fluorescence Detection of SARS-CoV-2 RNA in Clinical Samples via One-Pot Isothermal Ligation and Transcription. Nat. Biomed. Eng. 4 (12), 1168–1179. doi:10.1038/s41551-020-00617-5
Wu, J.-H., Wang, C.-H., Ma, Y.-D., and Lee, G.-B. (2018a). A Nitrocellulose Membrane-Based Integrated Microfluidic System for Bacterial Detection Utilizing Magnetic-Composite Membrane Microdevices and Bacteria-specific Aptamers. Lab. Chip 18 (11), 1633–1640. doi:10.1039/c8lc00251g
Wu, S., Liu, L., Duan, N., Wang, W., Yu, Q., and Wang, Z. (2018b). A Test Strip for Ochratoxin A Based on the Use of Aptamer-Modified Fluorescence Upconversion Nanoparticles. Microchim Acta 185 (11), 497. doi:10.1007/s00604-018-3022-0
Wu, X., Shaikh, A., Yu, Y., Li, Y., Ni, S., Lu, A., et al. (2017). Potential Diagnostic and Therapeutic Applications of Oligonucleotide Aptamers in Breast Cancer. Int. J. Mol. Sci. 18 (9), 1851. doi:10.3390/ijms18091851
Xi, Z., Gong, Q., Wang, C., and Zheng, B. (2018). Highly Sensitive Chemiluminescent Aptasensor for Detecting HBV Infection Based on Rapid Magnetic Separation and Double-Functionalized Gold Nanoparticles. Sci. Rep. 8 (1), 9444. doi:10.1038/s41598-018-27792-5
Xi, Z., Huang, R., Li, Z., He, N., Wang, T., Su, E., et al. (2015). Selection of HBsAg-specific DNA Aptamers Based on Carboxylated Magnetic Nanoparticles and Their Application in the Rapid and Simple Detection of Hepatitis B Virus Infection. ACS Appl. Mater. Inter. 7 (21), 11215–11223. doi:10.1021/acsami.5b01180
Yan, J., Gao, T., Lu, Z., Yin, J., Zhang, Y., and Pei, R. (2021). Aptamer-Targeted Photodynamic Platforms for Tumor Therapy. ACS Appl. Mater. Inter. 13 (24), 27749–27773. doi:10.1021/acsami.1c06818
Yang, J., and Bowser, M. T. (2013). Capillary Electrophoresis-SELEX Selection of Catalytic DNA Aptamers for a Small-Molecule Porphyrin Target. Anal. Chem. 85 (3), 1525–1530. doi:10.1021/ac302721j
Yu, H., Alkhamis, O., Canoura, J., Liu, Y., and Xiao, Y. (2021). Advances and Challenges in Small‐Molecule DNA Aptamer Isolation, Characterization, and Sensor Development. Angew. Chem. Int. Ed. 60 (31), 16800–16823. doi:10.1002/anie.202008663
Zhang, L., Fang, X., Liu, X., Ou, H., Zhang, H., Wang, J., et al. (2020). Discovery of sandwich Type COVID-19 Nucleocapsid Protein DNA Aptamers. Chem. Commun. 56 (70), 10235–10238. doi:10.1039/d0cc03993d
Zhang, X., Feng, Y., Duan, S., Su, L., Zhang, J., and He, F. (2019a). Mycobacterium Tuberculosisstrain H37Rv Electrochemical Sensor Mediated by Aptamer and AuNPs-DNA. ACS Sens. 4 (4), 849–855. doi:10.1021/acssensors.8b01230
Zhang, Y., Zhu, L., He, P., Zi, F., Hu, X., and Wang, Q. (2019b). Sensitive Assay of Escherichia coli in Food Samples by Microchip Capillary Electrophoresis Based on Specific Aptamer Binding Strategy. Talanta 197, 284–290. doi:10.1016/j.talanta.2019.01.040
Zhou, J., Neff, C. P., Swiderski, P., Li, H., Smith, D. D., Aboellail, T., et al. (2013a). Functional In Vivo Delivery of Multiplexed Anti-HIV-1 siRNAs via a Chemically Synthesized Aptamer with a Sticky Bridge. Mol. Ther. 21 (1), 192–200. doi:10.1038/mt.2012.226
Zhou, J., and Rossi, J. (2017). Aptamers as Targeted Therapeutics: Current Potential and Challenges. Nat. Rev. Drug Discov. 16 (3), 181–202. doi:10.1038/nrd.2016.199
Zhou, N., Wang, J., Zhang, J., Li, C., Tian, Y., and Wang, J. (2013b). Selection and Identification of Streptomycin-specific Single-Stranded DNA Aptamers and the Application in the Detection of Streptomycin in Honey. Talanta 108, 109–116. doi:10.1016/j.talanta.2013.01.064
Zhu, C., Li, L., Yang, G., Fang, S., Liu, M., Ghulam, M., et al. (2019a). Online Reaction Based Single-step Capillary Electrophoresis-Systematic Evolution of Ligands by Exponential Enrichment for ssDNA Aptamers Selection. Analytica Chim. Acta 1070, 112–122. doi:10.1016/j.aca.2019.04.034
Zhu, C., Yang, G., Ghulam, M., Li, L., and Qu, F. (2019b). Evolution of Multi-Functional Capillary Electrophoresis for High-Efficiency Selection of Aptamers. Biotechnol. Adv. 37 (8), 107432. doi:10.1016/j.biotechadv.2019.107432
Zhu, Q., Shibata, T., Kabashima, T., and Kai, M. (2012). Inhibition of HIV-1 Protease Expression in T Cells Owing to DNA Aptamer-Mediated Specific Delivery of siRNA. Eur. J. Med. Chem. 56, 396–399. doi:10.1016/j.ejmech.2012.07.045
Zhuo, Z., Yu, Y., Wang, M., Li, J., Zhang, Z., Liu, J., et al. (2017). Recent Advances in SELEX Technology and Aptamer Applications in Biomedicine. Int. J. Mol. Sci. 18 (10), 2142. doi:10.3390/ijms18102142
Keywords: aptamer, SELEX, infectious disease, precision medicine, biosensor
Citation: Xu Y, Jiang X, Zhou Y, Ma M, Wang M and Ying B (2021) Systematic Evolution of Ligands by Exponential Enrichment Technologies and Aptamer-Based Applications: Recent Progress and Challenges in Precision Medicine of Infectious Diseases. Front. Bioeng. Biotechnol. 9:704077. doi: 10.3389/fbioe.2021.704077
Received: 01 May 2021; Accepted: 26 July 2021;
Published: 10 August 2021.
Edited by:
Xinyu Liu, University of Toronto, CanadaReviewed by:
Giuseppe Legname, International School for Advanced Studies (SISSA), ItalyXuemei Ge, Nanjing Forestry University, China
Copyright © 2021 Xu, Jiang, Zhou, Ma, Wang and Ying. This is an open-access article distributed under the terms of the Creative Commons Attribution License (CC BY). The use, distribution or reproduction in other forums is permitted, provided the original author(s) and the copyright owner(s) are credited and that the original publication in this journal is cited, in accordance with accepted academic practice. No use, distribution or reproduction is permitted which does not comply with these terms.
*Correspondence: Minjin Wang, d2FuZy5taW5qaW5Ab3V0bG9vay5jb20=; Binwu Ying, eWluZ2Jpbnd1QHNjdS5lZHUuY24=
†These authors have contributed equally to this work and share first authorship