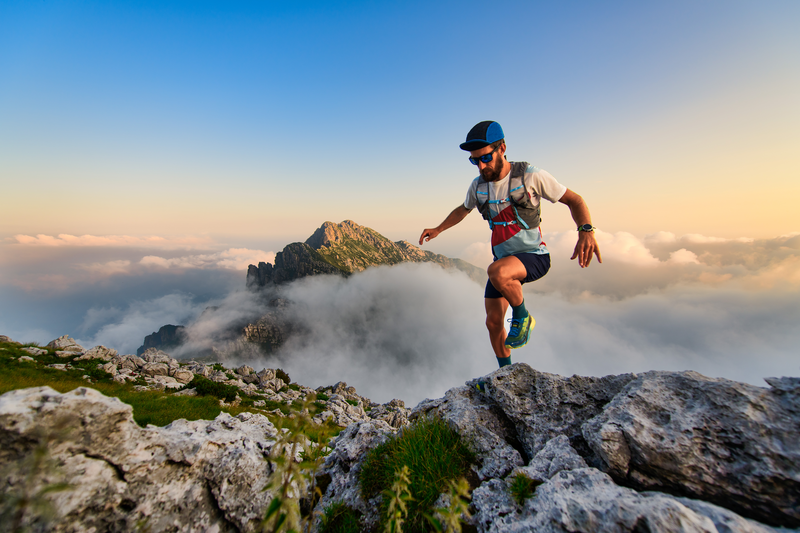
94% of researchers rate our articles as excellent or good
Learn more about the work of our research integrity team to safeguard the quality of each article we publish.
Find out more
ORIGINAL RESEARCH article
Front. Bioeng. Biotechnol. , 18 February 2021
Sec. Bioprocess Engineering
Volume 9 - 2021 | https://doi.org/10.3389/fbioe.2021.628719
This article is part of the Research Topic Advances and Trends in Polyhydroxyalkanoate (PHA) Biopolymer Production View all 11 articles
Polyhydroxyalkanoates (PHAs) production at pilot scale has been recently investigated and carried out exploiting different process configurations and organic wastes. More in detail, three pilot platforms, in Treviso (North-East of Italy), Carbonera (North-East of Italy) and Lisbon, produced PHAs by open mixed microbial cultures (MMCs) and different organic waste streams: organic fraction of municipal solid waste and sewage sludge (OFMSW-WAS), cellulosic primary sludge (CPS), and fruit waste (FW), respectively. In this context, two stabilization methods have been applied, and compared, for preserving the amount of PHA inside the cells: thermal drying and wet acidification of the biomass at the end of PHA accumulation process. Afterward, polymer has been extracted following an optimized method based on aqueous-phase inorganic reagents. Several PHA samples were then characterized to determine PHA purity, chemical composition, molecular weight, and thermal properties. The polymer contained two types of monomers, namely 3-hydroxybutyrate (3HB) and 3-hydroxyvalerate (3HV) at a relative percentage of 92.6–79.8 and 7.4–20.2 w/w, respectively, for Treviso and Lisbon plants. On the other hand, an opposite range was found for 3HB and 3HV monomers of PHA from Carbonera, which is 44.0–13.0 and 56.0–87.0 w/w, respectively. PHA extracted from wet-acidified biomass had generally higher viscosity average molecular weights (Mv) (on average 424.8 ± 20.6 and 224.9 ± 21.9 KDa, respectively, for Treviso and Lisbon) while PHA recovered from thermally stabilized dried biomass had a three-fold lower Mv.
Among different kinds of biopolymers, polyhydroxyalkanoates (PHAs) are one of the most interesting and promising thanks to their mechanical properties and biodegradability. As an alternative to industrial scale production based on pure culture (Gholami et al., 2016), the application of low-cost substrates based on organic wastes and by-products is of an extreme interest, specially coupled with the use of mixed microbial cultures (MMCs) that do not require sterile conditions or excessive operation control (Serafim et al., 2008a; Nikodinovic-Runic et al., 2013; Echegoyen et al., 2017). In fact, it is possible to combine the use of both MMCs (Beccari et al., 1998; Dionisi et al., 2004) and economic feedstocks (Dionisi et al., 2005; Bengtsson et al., 2008; Albuquerque et al., 2010; Bengtsson et al., 2010b; Campanari et al., 2014). Recently, the conversion of both wastewaters and volatile fatty acids (VFAs) mixtures obtained from the fermentation of different organic feedstocks, by MMC, have been investigated for the implementation of such a three-step process at pilot scale, by the integration of MMC-PHA production to wastewater treatment (Valentino et al., 2019; Conca et al., 2020; Moretto et al., 2020). However, downstream processing, including PHA-rich biomass stabilization and polymer extraction, are among the most important factors affecting the overall PHA production cost and need to be optimized for pilot scale. The most studied methods for recovering PHAs have been summarized in recent reviews (Kosseva and Rusbandi, 2018; Pérez-Rivero et al., 2019) and can be grouped into two main categories: solvent extraction and digestion of the NPCM (non-PHA cellular material). Up to now, regarding the MMC PHA-rich biomass, the use of several non-chlorinated solvents (Werker et al., 2014; Samorì et al., 2015) and, more recently, the disruption of NPCM through chemical agents (Burniol-Figols et al., 2020) and surfactants (Colombo et al., 2020) have been investigated. In the view of MMC-PHA production at industrial scale and a subsequent market scenario, specific chemo-mechanical and thermal properties of the polymer need to be controlled. In a recent study, two types of stabilization method have been applied at pilot scale on PHA obtained from organic wastes, in order to preserve the amount of PHA produced after the accumulation step and its properties (Lorini et al., 2020). The recovered polymer was extensively characterized and resulted in good thermal and chemical properties, while it showed variable molecular weight (between 100 and 450 kDa) dependent on the applied stabilization method. Indeed, molecular weight should be higher than 200 kDa and it is influenced by the PHA production process and downstream, including the extraction step (Laycock et al., 2014b). The availability in literature regarding the chemo-mechanical and thermal characterization of MMC-PHAs is very limited, however, the published studies highlighted an increase in melting temperature (Tm) for MMC-PHA respect to pure culture derived PHA (Bengtsson et al., 2010b) and a similar decomposition temperatures (270°C) to the commercial poly-3-hydroxybutyrate-co-3-hydroxyvalerate [P(3HB-co-3HV)] (Carrasco et al., 2006). Furthermore, monomeric composition, i.e., 3HV content, showed to have effects on different polymer properties (Arcos-Hernández et al., 2013; Lorini et al., 2020), such as crystallinity.
Thus, the evaluation of the quality of waste-derived PHA and the comparison with the properties of traditional plastics are necessary for commercial purposes and they must meet the standards required for various applications. Furthermore, for the scaling up of MMC-PHA production it is important to optimize an extractive protocol in order to minimize the overall production costs. On the other hand, the concept of novel biorefineries for urban waste valorization allows to integrate MMC–PHA production with wastewater treatment and other biowaste collection, reducing the production costs and the environmental impact. In this regard, the present study shows the results of a wide characterization, including thermal and chemical properties, of several samples of three grades of PHAs, produced by three pilot plants for PHA production from biowaste and recovered with the same extractive method (based on the use of aqueous-phase inorganic reagents). Specifically, the three pilot units produced PHA from different organic feedstocks: the organic fraction of municipal solid waste (OFMSW) and waste activated sludge (WAS) in Treviso plant; fruit waste in Lisbon; cellulosic primary sludge (CPS) in Carbonera.
The effects of an extraction method based on aqueous-phase inorganic reagents were evaluated on PHAs recovered from different biomasses, coming from three pilot plants using three types of feedstock, named TV-samples (from Treviso, OFMSW-WAS), L-samples (from Lisbon, FW), and C-samples (from Carbonera, CPS).
Within the pilot platform of Treviso (northeast Italy), the PHA was produced from a feedstock composed by a mixture of the liquid slurry coming from squeezed OFMSW and WAS from the treatment of municipal wastewater. For the sake of simplicity, this feedstock mixture is indicated as OFMSW-WAS. The latter was characterized by a volatile/total solids ratio of 80 ± 1% (VS/TS); a soluble chemical oxygen demand (CODSOL) of 22 ± 1 (gCOD L–1); total Kjeldahl nitrogen (TKN) equal to 29 ± 3 (g N kg–1 TS) and total phosphorus (P) equal to 2.3 ± 0.1 (g P kg–1 TS) (Moretto et al., 2020). The main process setup and operative conditions are extensively described in Valentino et al. (2019) and Moretto et al. (2020). The pilot plant consisted in a first anaerobic fermentation reactor (380 L) for PHA-precursors production (VFA), a second aerobic reactor (sequencing batch reactor, SBR; 100 L) for biomass cultivation, and a third fed-batch aerobic reactor (70–90 L) for PHA accumulation inside the cells (40–50% wt). This PHA-rich biomass (raw biomass) was collected following two different protocols of biomass stabilization, both addressed to the long-term PHA conservation inside the cells before the extraction/purification steps.
Following the first protocol, at the end of each accumulation, the PHA-rich biomass was left to settle under gravity and then the thickened slurry was centrifuged in a Heraeus Megafuge 40 with a Swinging Bucket Rotor (maximum radius: 195 mm; minimum radius: 83 mm) from Thermo Fisher Scientific (Waltham, MA, United States) for 15 min at 4,500 rpm. The wet pellet was thermally pre-treated at 145°C for 15 min, and then dried overnight in the same oven at 60°C (sample TV20).
In the second protocol, at the end of each accumulation, the PHA-rich biomass was acidified with H2SO4 down to pH 2.0, and left to settle under gravity overnight. The thickened slurry was then centrifuged in a Heraeus Megafuge 40 with a Swinging Bucket Rotor (maximum radius: 195 mm; minimum radius: 83 mm) from Thermo Fisher Scientific (Waltham, MA, United States) for 15 min at 4,500 rpm. Finally, the wet pellet was stored in the fridge (4°C) (samples TV1-12).
The concept of PHA production process is similar to that previously described. The main difference is the kind of the feedstock, which in this case was organic waste coming from fruit processing. The FW was characterized by a high VS/TS ratio (98.0 ± 2.27%); a CODSOL equal to 3.76 gCOD/L; very low ammonia (N-NH4+) and phosphate (P-PO43–) concentration, 1.26 ± 0.07 mg N/L and 2.39 ± 0.67 mg P/L, respectively. The pilot units in Lisbon’s facility consists in three reactors: an upflow anaerobic sludge bioreactor (UASB) with a volume of 60 L, a sequential batch reactor (SBR) with a volume of 100 L and a stirring tank reactor operating in fed-batch mode with 60 L of volume. Fermented FW was the feedstock for both biomass cultivation and PHA accumulation steps. The selected biomass was able to accumulate the polymer up to 70% of its cell dry weight. At the end of accumulation operation, the second stabilization protocol (described in section “PHA From Treviso Pilot Plant”) was applied: the reactor slurry was settled to remove as much water as possible and the biomass was acidified down to pH of 2.0–2.5, in order to prevent polymer degradation. The PHA-rich biomass was then stored in a fridge (4°C) before extraction.
The pilot plant located in Carbonera wastewater treatment plant (WWTP) (Treviso, Italy) integrated the via-nitrite nitrogen removal with PHAs production from the sidestream of Carbonera WWTP (Treviso, Italy). A novel substrate derived from fermentation of CPS was used as carbon source to evaluate the impacts on PHAs production and it was characterized by a VS/TS ratio equal to 92%; a relatively high content in terms of COD (1.5 g O2/g TS) and a low content of TKN (16/22 mg N/g TS) and P (1.7/4.7 mg P/g TS) (Da Ros et al., 2020). Process setup and operative conditions are extensively described in Conca et al. (2020). The pilot plant comprised the following units: i) rotating belt dynamic filter (RBDF) for the recovery of CPS; ii) fermentation unit for the production of VFAs; iii) ultrafiltration unit (UF) for solid/liquid separation of the fermented sludge; iv) nitritation sequencing batch reactor (N-SBR) for the oxidation of ammonia to nitrite; v) selection SBR (S-SBR) where aerobic-feast and anoxic-famine conditions were established to select PHA-accumulating biomass; and vi) an accumulation SBR (A-SBR) were intracellular PHA content was maximized through the feed-on-demand strategy. The S-SBR consisted in a stainless-steel SBR with a total volume of 2.8 m3 and operated under (aerobic)-feast and (anoxic)-famine conditions. The accumulation reactor (A-SBR) consisted in a stainless-steel tank with a volume of 1 m3 which operated as a fed-batch reactor. At the end of accumulation operation, the second stabilization protocol (described in section “PHA From Treviso Pilot Plant”) was applied: the reactor slurry was settled to remove as much water as possible and the biomass was acidified down to pH of 2.0–2.5, in order to prevent polymer degradation. The PHA-rich biomass was then stored in a fridge (4°C) before extraction.
An extraction method involving a mixture of aqueous phase inorganic reagents has been optimized by Biotrend S. A. for PHA recovery from the cellular material of enriched biomass. The optimized method was carried out on PHA-rich biomasses coming from the three previously described pilot plants, stabilized at the end of the accumulation step following the thermal (sample TV20) and the acidification protocol (samples TV 1-12; L-samples; C-samples). At the end of the extraction, the polymer was dried obtaining a white powder. The optimized extraction method is reserved by Biotrend S. A. and under patent application procedure.
Approximately 3.5 mg of dried biomass or 5.0 mL of re-suspended samples (wet and dried thermally treated biomasses) were suspended in 2 mL of acidified methanol solution (at 3% v/v H2SO4) containing benzoic acid (at 0.005% w/v) as internal standard and 1 mL of chloroform in a screw-capped test tube. Then, an acid-catalyzed methanolysis of the PHA occurred and the released methyl esters were quantified by gas-chromatography (GC-FID Perkin Elmer 8410) according to the method described in Braunegg et al. (1978). The relative abundance of 3HB and 3HV monomers was determined using a commercial P(3HB-co-3HV) copolymer with a 3HV content of 5 wt. % (Sigma–Aldrich, Milan, Italy) as reference standard. The 3HV content in PHA was calculated as the ratio of 3HV and (3HB + 3HV) monomers (as wt. %).
A volume of 25 mL of PHA solution at the concentration of 0.5% w/v in chloroform were prepared for all the samples of extracted polymer. The equipment for the measure of the gravimetric flow time of each solution was composed by a SCHOTT AVS 350 viscosimeter with AVS/SHT sensor, a LAUDA CD15 thermostatic bath (working at 30°C) and a SCHOTT GERÄTE Ubbelohde capillary viscosimeter (ID = 0.46 mm). For each sample, 15 mL of solution 0.5% w/v were transferred in the Ubbelohde capillary viscosimeter and the flow time was measured through the optical sensor. Then, at least four dilutions were made directly in the viscosimeter by adding predetermined aliquots of solvent. From the flow time of each solution the intrinsic viscosity [η] of polymer samples was determined. The viscosity average molecular weight (Mv) was calculated according to the Mark–Houwink equation:
with K = 7.7 × 10–5 e α = 0.82 (Marchessault et al., 1970)
The thermal stability of the samples was evaluated by TGA using a Mettler TG50 thermobalance. Approximately 5 mg of dried samples were weighted on the balance. The analysis was conducted in nitrogen flow (20 mL min–1) by heating the samples from 30 to 500°C at 10°C min–1.
The thermal properties of the PHA samples were characterized by a differential scanning calorimeter Mettler Toledo DSC 822e. All the experiments were carried out under N2 flux (30 mL min–1) on about 3–6 mg of polymer, weighted in aluminum pans. TV-samples were analyzed applying a single heating scan at 10°C min–1 from room temperature to 190°C, in order to evaluate the real thermal behavior of the final product.
The sample crystallinity (Xc) was evaluated from the equation:
where ΔHm is the melting enthalpy obtained from the single DSC heating scan and ΔHm0 = 146 J g–1 is the enthalpy of fusion of 100% crystalline PHB sample (Barham et al., 1984).
On the contrary, in order to evaluate the intrinsic thermal properties, the following temperature program was selected for the analysis of C-samples:
1. a heating scan at 10°C min–1 from RT to 190°C;
2. a rapid cooling at 30°C min–1 from 190 to −70°C;
3. a second heating up to 190°C at 10°C min–1.
The first heating is necessary to erase all the previous sample history.
Principal component analysis (PCA) was conducted using the software The Unscrambler ver. 10.5 (CAMO Software AS, Oslo, Norway).
Three grades PHA produced from three different organic wastes have been extracted and characterized. For the sake of a deeper discussion, the characteristics of fermented streams and the results in terms of overall PHA production yield of the three pilot plants are here reported. Within the pilot plant located in Treviso, the fermented mixture of OFMSW – WAS showed a predominance of acetic acid (24–34%) and propionic acid (10–20%), along with butyric acid (30–40%) and caproic acid (25–35%) fed to both selection and accumulation steps for PHA production. The overall process yield was calculated and resulted of 110 g PHA/kg VS (Moretto et al., 2020). On the other hand, pilot plant in Lisbon produced a fermented stream, starting from FW, characterized by a predominance of butyric and acetic acid, along with propionic acid, valeric acid, lactic acid and ethanol, with a resulting fermentative products concentration of 21.2 ± 1.6 gCOD L–1. As for Treviso process, the fermented stream fed both the selection and accumulation steps. It was estimated that 37.4 L of FW are necessary to produce 1.0 kg of PHA and then the resulted global yield of conversion was 26% on COD basis (data under submission). Finally, the fermentation of CPS in Carbonera pilot plant led to a fermented stream characterized by the presence of acetic, propionic, butyric and valeric acid and a quite high molar ratio between even and odd VFAs [0.49–0.52% mol/mol C3/(C2 + C3)]. The fermented stream CPS fed both the selection and the accumulation steps. As a result, the PHA production represented up to 17.5% of the COD conversion and a potential PHA production of 1.2 kg PHA/PE year was calculated (Conca et al., 2020).
Results obtained by GC-FID analysis conducted on extracted samples (Table 1) showed that the optimized extraction method allowed to reach very high purity, with an average value of 103.4 ± 2.1% w/w. The high PHA content in the recovered material was confirmed through TGA analysis. In fact the PHA content (% w/w), that indicates the purity of the sample, showed an average value of 98.9 ± 0.7% w/w. Sample TV20, extracted from a thermal stabilized biomass, had the lowest purity (85.5 ± 3.6% w/w). In Table 1, the 3HV content quantified from GC-FID analysis is also reported. It is possible to notice that samples from OFMSW-WAS and from FW are characterized by a 3HV content ranging between 16.3 and 19.1% w/w, with some exceptions. More in detail, samples TV20, L1, L2, and L3 (having 3HV content between 7.4 and 7.7% w/w) have been produced during the first operative year, while the remaining samples have been produced during the following operative years. In both cases, the two pilot platforms in Treviso and in Lisbon were operated in parallel, in order to obtain polymers with the same monomeric composition. Indeed, VFAs composition of the fermented mixtures, characterized by the predominance of even VFAs, led to a high 3HB percentage. On the other hand, a higher variability and an opposite trend were found for C-samples, in fact 3HV content is higher than 3HB, varying from 56 to 87% w/w, as a direct consequence of the high odd VFAs content in the feedstock (Albuquerque et al., 2011).
Table 1. Chemical and thermal properties of PHA samples produced in Treviso, Lisbon, and Carbonera pilot plants.
Thermal characteristics of the extracted polymers, such as decomposition temperatures and related thermal stability, melting temperature and melting enthalpy have been determined through TGA and DSC. The results of the TGA experiments, including the PHA content (discussed in the previous section), the temperature at 10% decomposition (Td10%) and the temperature at maximum decomposition rate (Tdmax), are reported in Table 1, while melting temperature (Tm) and melting enthalpy (ΔHm) from DSC are reported in Table 2. The comparison of the results showed that PHA thermal stability seems not to be affected by the feedstock used for PHA production. In fact, the average Td10% and Tdmax of extracted TV-samples were 265 ± 3°C and 288 ± 3°C while for L-samples the corresponding values were 257 ± 5°C (Td10%) and 276 ± 5°C (Tdmax) respectively. On the contrary, C-samples showed a lower thermal stability, with an average Td10% and Tdmax at 238 ± 4°C and 257 ± 3°C, respectively. The DSC profiles of sample TV1 is reported in Figure 1. The thermograms of the other TV samples did not remarkably change from that of TV1. In all the thermograms the main transition is the melting process occurring in the 160–175°C temperature range, but also a low temperature transition at about 60°C occurred.
Table 2. Thermal properties and crystallinity of PHA samples produced in Treviso and Carbonera pilot plants.
On the other hand, C-samples showed a different thermal behaviour, indeed the melting process occurred in a wider range shifted at lower temperature (80–105°C). Moreover, for C-samples, the glass transition occurred after the cooling step and it ranges between −22 and −12.5°C.
From the integrated melting enthalpy, the sample crystallinity was evaluated by Eq. 2 for TV-samples and then reported in Table 2. On the contrary, while there is adequate agreement between the results obtained from DSC for the P(3HB) homopolymer, in PHBV copolymers, it is quite difficult to measure a true crystallinity, particularly for copolymers with 3HV content higher than 20% w/w (Laycock et al., 2014b). Thus, in order to compare the melting behavior, the melting enthalpies (ΔHm) of TV and C-samples, determined by DSC, are reported as a function of 3HV content in Figure 2. Moreover, for a more complete evaluation of the 3HV content effects, results from a previous work (Lorini et al., 2020) are reported, too. In this way, it was possible to have a wider range of 3HV percentage, specifically between 7.7 and 87% w/w. The results clearly show that ΔHm linearly increased with the 3HV content in the C-samples copolymer (3HV % w/w = 56-87), while it linearly decreased for the whole TV-samples (3HV % w/w = 7.7-20.2).
The determination of viscosity average molecular weight (Mv) of each extracted sample gave different results for the three grades of collected material.
The results, reported in Figure 3, clearly appeared divided in two main groups: TV-samples and L and C-samples, with average values of 430 ± 24 and 208 ± 20 kDa, respectively, and one outlier for each group (102 and 508 kDa, respectively, for TV20 and C2).
Figure 3. Comparison of viscosity average molecular weights (Mv). Starred sample TV20 is thermal stabilized.
Principal component analysis was defined as an effective statistical method used to establish relationships between different observations, such as similarities and inequalities. According to the PCA results, the first principal component (PC1) accounted for 48% of the total variance, 31% for the 2nd component (PC2). Figure 4A shows the score plot graphic and Figure 4B shows the loading plot graphic for PC1 and PC2 in PHA samples. When Figures 4A and B are evaluated together, analysis of the data obtained from the complete characterization of the PHA samples with the PCA allowed grouping the PHA samples into three groups to express and demonstrate their similarity and differences.
Recovery procedures for PHA from MMC, including non-chlorinated solvents extraction and disruption of NPCM through chemical or oxidizing agents, were widely investigated in the last decade as alternatives to traditional chloroform extraction (Werker et al., 2012; Villano et al., 2014; Samorì et al., 2015).
More recently, disruption of NPCM was obtained by using non-ionic surfactants as pretreatment before PHA extraction with DMC (Colombo et al., 2020) and by treating the biomass with ammonia solutions at high temperatures (Burniol-Figols et al., 2020). In a previous study (Lorini et al., 2020), NaClO digestion was investigated for PHA recovery from MMC produced at pilot scale. More in detail, PHA was produced in the pilot plant operating in Treviso, exploiting the same feedstock (OFMSW-WAS) and operative conditions and applying the same biomass stabilization methods described in this work. In this study, the effects of the extraction method optimized by Biotrend S. A. were evaluated on PHAs recovered from different biomasses. The extraction has been conducted on the wet acid stabilized centrifuged biomass for the whole set of PHA sample, except for sample TV20. Indeed, the latter was extracted from a thermal-stabilized dry biomass, produced during the first operative year of the pilot platform together with the samples analyzed elsewhere (Lorini et al., 2020). Results of the latter study are reported in Table 1 for a comparison. As explained in section “Purity and Monomeric Composition of Results,” sample TV20 had a lower purity, comparable with those thermal dried determined in the reference work by Lorini et al. (2020), than the rest of the whole set. Compared to other reference studies, in which NPCM disruption was conducted, the extraction method discussed in this work shows better performances in terms of polymer purity (higher than 92.0% w/w), in fact using NH3 (Burniol-Figols et al., 2020) or a combination of non-ionic surfactants and DMC (as PHA solvent) (Colombo et al., 2020) allowed to reach a PHA purity of 86 and 57 − 92% w/w, respectively. This evidence suggests that the optimized extraction method can be considered effective on all the types of biomass, in fact the same results were obtained independently from the PHA origin, that is kind of feedstock and operative conditions.
The purity obtained by GC-FID was confirmed through TGA analysis, in fact the polymer content in the samples was estimated from the main weight loss occurring between 250 and 320°C, as reported in literature (Hahn and Chang, 1995).
Moreover, in terms of thermal stability, the C-samples, with the highest 3HV content (56-87% w/w), showed the lowest degradation temperatures (on average Td10% and Tdmax at 238 ± 4°C and 257 ± 3°C, respectively). On the other hand, samples TV20 and L1, L2, L3, L4, with the lowest 3HV content (7.6% w/w) gave non-homogeneous results, indeed TV20 exhibited the highest Td10% (289°C) and Tdmax (307°C), while L-samples had lower values. Hence, the variability of the reported available data (Table 1) did not allow to enunciate a statement on the possible effect of monomeric composition on thermal stability.
Overall, such values indicated the high thermal stability of the polymer, comparable to those observed in other studies on MMC-PHA (Table 1) and on commercial P(3HB-co-3HV) (Tdmax = 256–290°C; Morgan-Sagastume et al., 2015). DSC analysis allowed to evaluate the thermal behaviour of the three grades PHA. The melting temperature determined for TV-samples, ranging in 130–175°C, resulted comparable or higher than those reported in literature (Table 2). The low temperature exothermic transition at about 60°C could be considered as a cold crystallization (Figure 1), probably due to a partial crystallization started at low temperature during the extraction process (Wellen et al., 2015). Different thermal properties were found for PHA from Carbonera, in fact C-samples presented a lower Tm than TV and L-samples, and a Tg comparable or even lower than those reported in previous studies (Table 2). In fact, Bengtsson et al. (2010b) reported a Tg of −10.3°C and −14°C for PHA with 53 and 63% mol 3HV, produced from molasses and synthetic VFA solution, respectively. On the other hand, Serafim et al. (2008b) characterized a PHA, produced with a synthetic feedstock and having a 3HV content of 72% mol, which presented a Tm of 99.2°C and a Tg of −29.6°C, more similar to those of C-samples. For TV-samples, the determination of melting enthalpy allowed to calculate the crystallinity and to confirm the semicrystalline behavior of copolymer P(3HB-co-3HV) (Bengtsson et al., 2010a). Indeed, TV-samples are characterized by a χc (%) comparable to that reported in the previous work of Lorini et al. (2020) and in a study from Colombo et al. (2020) (Table 2). As reported in literature, it is quite difficult to determine the crystallinity of PHBV copolymers having 3HV content higher than 20%, since the melting process is affected by the presence of amorphous regions (Laycock et al., 2014b). Thus, Figure 2 shows a typical trend of ΔHm as a function of 3HV content, indeed, as reported in Laycock et al. (2014b), a minimum in the melting enthalpy at 30–55% HV occurs.
Overall, these results highlight that the PHA samples, obtained with different process configuration and different feedstock, did not differ in thermal behaviour from other MMC-PHAs, independently from the kind of substrate exploited.
As mentioned in section “Viscosity Average Molecular Weight” of Results, Figure 3 shows the results of Mv determination. They are divided in two main groups having average values of 430 ± 24 and 208 ± 20 kDa.
To better understand the reason of this marked difference, it is useful to make a comparison between TV-samples of the present work and those of Lorini et al. (2020) (same pilot plant, feedstock, and stabilization methods).
As a main result of the previous work, the average Mv values of thermal dried samples were 127 ± 16 and 132 ± 10 kDa for NaClO- and CHCl3-extracted samples, respectively. Hence, no effects of the extraction step were found. Indeed, chloroform extraction was considered as a benchmark and it was concluded that NPCM disruption had not damaged the polymer. In this sense, we can consider NaClO extraction of the previous work as a benchmark, because it was applied on biomasses obtained in the same operative periods and with the same operative conditions. More in detail, sample TV-20 was thermally stabilized and obtained in parallel with thermal dried samples of the previous work, while samples TV-1-12 were acid stabilized and obtained in parallel with two acid stabilized samples of the previous work. These latter had a significantly higher Mv (370 and 424 kDa) than that obtained from thermal dried samples.
Hence, the Mv determination has allowed to evidence any possible effects of the biomass pre-treatment on the PHA and the effective capacity of acidification to stabilize PHA for downstream processing without affecting its molecular weight was confirmed (Werker et al., 2012). This evidence can be further confirmed by the results obtained in the present study. In fact, the same trend was found for sample TV-20 (Mv = 102 kDa) and TV-1-12 (341-615 kDa). Moreover, a study on PHB produced from crude glycerol and MMC reports significantly different Mw values for PHB after drying at 65°C and after lyophilization, respectively, 84-195 and 200-380 kDa. The authors justified the Mw decrease after drying with the occurrence of a partial hydrolysis due to heating (Hu et al., 2013). Besides, it can be concluded that the extraction method applied by Biotrend S. A. had no negative effects on PHAs chains, since the results of TV-samples are similar those obtained from the benchmark NaClO extraction (Lorini et al., 2020). On the other hand, L and C-samples showed a significantly lower Mv, ranging between 134-354 and 92-203 kDa with C2-sample as an outlier (508 kDa). Taking into account that the stabilization method applied on PHA-rich biomass at the end of the accumulation and the extraction method are the same for all the three grades PHA (TV, L, and C-samples), it is possible to infer that the marked difference in Mv is probably due to the different feedstocks and operative conditions applied in the pilot plants. According to what has been reported in recent studies of MMC-PHA produced from wastes and/or synthetic VFA mixture there is no correlation between the Mw and the 3HV content in the copolymer (Frison et al., 2015; Hilliou et al., 2016). Literature data on commercial polymers from pure culture report that molecular weight (Mw) ranges in 200–660 kDa (Morgan-Sagastume et al., 2015; Colombo et al., 2017). On the contrary, it is often reported that MMC are able to produce PHA with even higher Mw, comparable to the highest Mv determined for TV-samples. Previous studies reported Mw of 340–540 kDa (Villano et al., 2014), 440–630 kDa (Laycock et al., 2014a) for PHA produced from synthetic VFA mixture; 400–600 kDa (Burniol-Figols et al., 2020) from crude glycerol fermentation liquid; 650 kDa (Frison et al., 2015) from WAS; 800 kDa (Colombo et al., 2017) from percolates of the OFMSW; 800 kDa (Colombo et al., 2020) from dairy wastes. The cited works have been conducted at laboratory scale, while a study from full-scale is noteworthy and it reported a PHA from activated sludge with Mw of 400–500 kDa (Arcos-Hernández et al., 2015; Werker et al., 2018).
Considering Figures 4A and B together, analysis of the data obtained from the complete characterization of the PHA samples with the PCA allowed grouping the PHA samples into three groups to express and demonstrate their similarity and differences. According to that discussed in the previous sections, it is possible to conclude that the main differences among the three grades PHA are due to the different feedstocks and pilot plants, with more remarkable evidence in C-samples. Indeed, PC1 presents a direct correlation with 3HV content that is determining for separate C-samples as a single group, since 3HV is even higher than 80% w/w, namely the opposite composition of TV and L-copolymer. Loadings plot (Figure 4B) shows that Mv is also a relevant parameter, in fact TV and L-samples are separated mainly because of the marked difference in Mv values and the two groups are shown in scores plot (Figure 4A).
Polyhydroxyalkanoates produced in Treviso showed Mv in line with values for good mechanical properties, required for thermoplastic applications (Laycock et al., 2014b). Hence the PHA produced in Treviso pilot plant deserves further investigation. Up to now, tests for future applications evaluated the possibility of compounding PHA from OFMSW-WAS and other biodegradable polymers, as PBS (polybutylene succinate) or PBAT (polybutylene adipate terephthalate). Preliminary results showed that it is possible to obtain biodegradable films (for agricultural purposes) with acceptable mechanical and permeability properties through melt compounding and blown extrusion, starting from mixtures at different PHA content. Also packaging applications have been explored by Melendez-Rodriguez et al. (2020) on PHA from Treviso, carrying out a wide characterization on fiber-based continuous films obtained starting from electrospun PHA. The combination of electrospinning and a mild annealing postprocessing below the biopolymer’s Tm was able to yield unique biomaterials with balanced mechanical, thermal and barrier properties (Melendez-Rodriguez et al., 2020). Furthermore, one of the main concerns relating to the use of waste as feedstock is the content of pollutants that can migrate from the waste, through the technology chain, and into the end-products. PHAs from Treviso and Lisbon were analyzed in order to determine the presence of relevant contaminants, such as heavy metals (Astolfi et al., 2020) and polychlorinated biphenyls (PCB) (Riccardi et al., 2020). The total content of the analyzed metals and PCBs for all tested PHA types complied with the current regulations and guidelines. Therefore, the use of such waste as raw material for PHA production process appears to be safe for the environment and human health. As an added value, PHA characterized by low Mv or purity may be exploited as electron donor for biological reductive dechlorination, applied into permeable reactive barriers (PRBs) for groundwater remediation from chlorinated hydrocarbons (Baric et al., 2014).
This study showed the possibility to exploit different organic biowaste as raw material for biodegradable polymers, characterized by thermal and chemical properties comparable to commercial plastics. Indeed, the application of a stabilization method at the end of the accumulation step followed by an efficient extraction allowed to reach high purity of the end-product and to preserve the polymer properties, mostly the molecular weight. This latter was influenced by the type of feedstock, indeed on equal terms of monomeric composition with PHA from FW, a PHA with significantly higher Mv was produced from OFMSW-WAS. Monomeric composition was strongly influenced by the VFAs composition and the operative conditions, since C-samples have an opposite 3HV content (56–87% w/w) than TV and L-samples. Thermal properties are all in the same range for the whole samples set and comparable to those reported in literature. Moreover, melting enthalpy is dependent from 3HV content and the showed trend complies with that reported in literature. As a conclusion, the present study underlines that, from three different processes at pilot scale, different PHAs having adjustable properties can be produced with a long time stability. The real novelty of the work is to show how waste-derived biopolymers can achieve good characteristics and workability, and this information has a pivotal role in future development and optimization of these processes, including the kind of downstream and applications.
The raw data supporting the conclusions of this article will be made available by the authors, without undue reservation.
LL: investigation, data curation, and writing – original draft. AM: supervision and visualization. GC: formal analysis. NF and BSF: resources. MR: resources and funding acquisition. MV: visualization. MM: funding acquisition and project administration. FV: conceptualization, data curation, writing – original draft, and supervision. All authors contributed to the article and approved the submitted version.
BSF was employed by company Biotrend SA.
The remaining authors declare that the research was conducted in the absence of any commercial or financial relationships that could be construed as a potential conflict of interest.
This work was supported by the “REsources from URban BIowaSte” – RES URBIS (GA 7303499) and by the “Scale-up of low-carbon footprint MAterial Recovery Techniques in existing wastewater treatment PLANTs” – SMART-PLANT (GA 690323) projects in the European Horizon 2020 program.
Albuquerque, M. G. E., Martino, V., Pollet, E., Avérous, L., and Reis, M. A. M. (2011). Mixed culture polyhydroxyalkanoate (PHA) production from volatile fatty acid (VFA)-rich streams: effect of substrate composition and feeding regime on PHA productivity, composition and properties. J. Biotechnol. 151, 66–76. doi: 10.1016/j.jbiotec.2010.10.070
Albuquerque, M. G. E., Torres, C. A. V., and Reis, M. A. M. (2010). Polyhydroxyalkanoate (PHA) production by a mixed microbial culture using sugar molasses: effect of the influent substrate concentration on culture selection. Water Res. 44, 3419–3433. doi: 10.1016/j.watres.2010.03.021
Arcos-Hernández, M., Montaño-Herrera, L., Murugan Janarthanan, O., Quadri, L., Anterrieu, S., Hjort, M., et al. (2015). Value-added bioplastics from services of wastewater treatment. Water Pract. Technol. 10, 546–555. doi: 10.2166/wpt.2015.063
Arcos-Hernández, M. V., Laycock, B., Donose, B. C., Pratt, S., Halley, P., Al-Luaibi, S., et al. (2013). Physicochemical and mechanical properties of mixed culture polyhydroxyalkanoate (PHBV). Eur. Poly. J. 49, 904–913. doi: 10.1016/j.eurpolymj.2012.10.025
Astolfi, M. L., Marconi, E., Lorini, L., Valentino, F., Silva, F., Sommer Ferreira, B., et al. (2020). Elemental concentration and migratability in bioplastics derived from organic waste. Chemosphere 259:127472. doi: 10.1016/j.chemosphere.2020.127472
Barham, P. J., Keller, A., Otun, E. L., and Holmes, P. A. (1984). Crystallization and morphology of a bacterial thermoplastic: Poly-3-hydroxybutyrate. J. Mater. Sci. 19, 2781–2794. doi: 10.1007/BF01026954
Baric, M., Pierro, L., Pietrangeli, B., and Petrangeli Papini, M. (2014). Polyhydroxyalkanoate (PHB) as a slow-release electron donor for advanced in situ bioremediation of chlorinated solvent-contaminated aquifers. New Biotechnol. 31, 377–382. doi: 10.1016/j.nbt.2013.10.008
Beccari, M., Majone, M., Massanisso, P., and Ramadori, R. (1998). A bulking Sludge with high storage response selected under intermittent feeding. Water Res. 32, 3403–3413. doi: 10.1016/S0043-1354(98)00100-6
Bengtsson, S., Pisco, A. R., Johansson, P., Lemos, P. C., and Reis, M. A. M. (2010a). Molecular weight and thermal properties of polyhydroxyalkanoates produced from fermented sugar molasses by open mixed cultures. J. Biotechnol. 147, 172–179. doi: 10.1016/j.jbiotec.2010.03.022
Bengtsson, S., Pisco, A. R., Reis, M. A. M., and Lemos, P. C. (2010b). Production of polyhydroxyalkanoates from fermented sugar cane molasses by a mixed culture enriched in glycogen accumulating organisms. J. Biotechnol. 145, 253–263. doi: 10.1016/j.jbiotec.2009.11.016
Bengtsson, S., Werker, A., Christensson, M., and Welander, T. (2008). Production of polyhydroxyalkanoates by activated sludge treating a paper mill wastewater. Bioresour. Technol. 99, 509–516. doi: 10.1016/j.biortech.2007.01.020
Braunegg, G., Sonnleitner, B., and Lafferty, R. (1978). Rapid gas-chromatographic method for determination of poly-beta-hydroxybutyric acid in microbial biomass. Appl. Microbiol. Biotechnol. 6, 29–37. doi: 10.1007/BF00500854
Burniol-Figols, A. I, Skiadas, V., Daugaard, A. E., and Gavala, H. N. (2020). Polyhydroxyalkanoate (PHA) purification through dilute aqueous ammonia digestion at elevated temperatures. J. Chem. Technol. Biotechnol. 95, 1519–1532. doi: 10.1002/jctb.6345
Campanari, S., Silva, F., Bertin, L., Villano, M., and Majone, M. (2014). Effect of the organic loading rate on the production of polyhydroxyalkanoates in a multi-stage process aimed at the valorization of olive oil mill wastewater. Int. J. Biol. Macromol. 71, 34–41. doi: 10.1016/j.ijbiomac.2014.06.006
Carrasco, F., Dionisi, D., Martinelli, A., and Majone, M. (2006). Thermal stability of polyhydroxyalkanoates. J. Appl. Polym. Sci. 100, 2111–2121. doi: 10.1002/app.23586
Colombo, B., Favini, F., Scaglia, B., Pepè Sciarria, T., D’Imporzano, G., Pognani, M., et al. (2017). Enhanced polyhydroxyalkanoate (PHA) production from the organic fraction of municipal solid waste by using mixed microbial culture. Biotechnol. Biofuels 10:201. doi: 10.1186/s13068-017-0888-8
Colombo, B., Pereira, J., Martins, M., Torres-Acosta, M. A., Dias, A. C. R. V., Lemos, P. C., et al. (2020). Recovering PHA from mixed microbial biomass: using non-ionic surfactants as a pretreatment step. Separat. Purificat. Technol. 253:117521. doi: 10.1016/j.seppur.2020.117521
Conca, V., Da Ros, C., Valentino, F., Eusebi, A. L., Frison, N., and Fatone, F. (2020). Long-term validation of polyhydroxyalkanoates production potential from the sidestream of municipal wastewater treatment plant at pilot scale. Chem. Eng. J. 390:124627. doi: 10.1016/j.cej.2020.124627
Da Ros, C. V., Conca, A., Eusebi, L., Frison, N., and Fatone, F. (2020). Sieving of municipal wastewater and recovery of bio-based volatile fatty acids at pilot scale. Water Res. 174:115633. doi: 10.1016/j.watres.2020.115633
Dionisi, D., Carucci, G., Petrangeli Papini, M., Riccardi, C., Majone, M., and Carrasco, F. (2005). Olive oil mill effluents as a feedstock for production of biodegradable polymers. Water Res. 39, 2076–2084. doi: 10.1016/j.watres.2005.03.011
Dionisi, D., Majone, M., Papa, V., and Beccari, M. (2004). Biodegradable polymers from organic acids by using activated sludge enriched by aerobic periodic feeding. Biotechnol. Bioeng. 85, 569–579. doi: 10.1002/bit.10910
Echegoyen, Y., Fabra, M. J., Castro-Mayorga, J. L., Cherpinski, A., and Lagaron, J. M. (2017). High throughput electro-hydrodynamic processing in food encapsulation and food packaging applications: viewpoint. Trends Food Sci. Technol. 60, 71–79. doi: 10.1016/j.tifs.2016.10.019
Frison, N., Katsou, E., Malamis, S., Oehmen, A., and Fatone, F. (2015). Development of a Novel process integrating the treatment of sludge reject water and the production of polyhydroxyalkanoates (PHAs). Environ. Sci. Technol. 49, 10877–10885. doi: 10.1021/acs.est.5b01776
Gholami, A., Mohkam, M., Rasoul-Amini, S., and Ghasemi, Y. (2016). Industrial production of polyhydroxyalkanoates by bacteria: opportunities and challenges. Miner. Biotecnol. 28, 59–74.
Hahn, S. K., and Chang, Y. K. (1995). A themogravimetric analysis for Poly(3-Hydroxybutyrate) quantification. Biotechnol. Techn. 9, 873–878. doi: 10.1007/BF00158539
Hilliou, L., Machado, D., Oliveira, C. S. S., Gouveia, A. R., Reis, M. A. M., Campanari, S., et al. (2016). Impact of fermentation residues on the thermal, structural, and rheological properties of polyhydroxy(Butyrate-Co-Valerate) produced from cheese whey and olive oil mill wastewater. J. Appl. Polym. Sci. 132:42818. doi: 10.1002/app.42818
Hu, S., McDonald, A. G., and Coats, E. R. (2013). Characterization of polyhydroxybutyrate biosynthesized from crude glycerol waste using mixed microbial consortia. J. Appl. Polym. Sci. 129, 1314–1321. doi: 10.1002/app.38820
Kosseva, M. R., and Rusbandi, E. (2018). Trends in the biomanufacture of polyhydroxyalkanoates with focus on downstream processing. Int. J. Biol. Macromol. 107:762. doi: 10.1016/j.ijbiomac.2017.09.054
Laycock, B., Arcos-Hernandez, M. V., Langford, A., Pratt, S., Werker, A., Halley, P. J., et al. (2014a). Crystallisation and fractionation of selected polyhydroxyalkanoates produced from mixed cultures. New Biotechnol. 31, 345–356. doi: 10.1016/j.nbt.2013.05.005
Laycock, B., Halley, P., Pratt, S., Werker, A., and Lant, P. (2014b). The chemomechanical properties of microbial polyhydroxyalkanoates. Progr. Polym. Sci. 39, 397–442. doi: 10.1016/j.progpolymsci.2013.06.008
Lorini, L., Martinelli, A., Pavan, P., Majone, M., and Valentino, F. (2020). Downstream processing and characterization of polyhydroxyalkanoates (PHAs) produced by mixed microbial culture (MMC) and organic urban waste as substrate. Biom. Conver. Bioref. (in press). doi: 10.1007/s13399-020-00788-w
Marchessault, R. H., Okamura, K., and Su, C. J. (1970). Physical properties of poly(β-Hydroxy Butyrate). II. conformational aspects in solution. Macromolecules 3, 735–740. doi: 10.1021/ma60018a005
Melendez-Rodriguez, B., Torres-Giner, S., Lorini, L., Valentino, F., Sammon, C., Cabedo, L., et al. (2020). Valorization of municipal biowaste into electrospun poly(3-Hydroxybutyrate- Co -3-Hydroxyvalerate) Biopapers for food packaging applications. ACS Appl. Bio Mater. 3, 6110–6123. doi: 10.1021/acsabm.0c00698
Moretto, G., Lorini, L., Pavan, P., Crognale, S., Tonanzi, B., Rossetti, S., et al. (2020). Biopolymers from urban organic waste: influence of the solid retention time to cycle length ratio in the enrichment of a mixed microbial culture (MMC). ACS Sustain. Chem. Eng. 8:0c04980. doi: 10.1021/acssuschemeng.0c04980
Moretto, G., Russo, I., Bolzonella, D., Pavan, P., Majone, M., and Valentino, F. (2020). An Urban biorefinery for food waste and biological sludge conversion into polyhydroxyalkanoates and biogas. Water Res. 170:115371. doi: 10.1016/j.watres.2019.115371
Morgan-Sagastume, F., Hjort, M., Cirne, D., Gérardin, F., Lacroix, S., Gaval, G., et al. (2015). Integrated production of polyhydroxyalkanoates (PHAs) with municipal wastewater and sludge treatment at pilot scale. Bioresour. Technol. 181, 78–89. doi: 10.1016/j.biortech.2015.01.046
Nikodinovic-Runic, J., Guzik, M., Kenny, S. T., Babu, R., Werker, A., and Connor, K. E. O. (2013). Carbon-rich wastes as feedstocks for biodegradable polymer (Polyhydroxyalkanoate) production using bacteria. Adv. Appl. Microbiol. 84, 139–200. doi: 10.1016/B978-0-12-407673-0.00004-7
Pérez-Rivero, C., López-Gómez, J. P., and Roy, I. (2019). A Sustainable approach for the downstream processing of bacterial polyhydroxyalkanoates: state-of-the-art and latest developments. Biochem. Eng. J. 150:107283. doi: 10.1016/j.bej.2019.107283
Riccardi, C., Buiarelli, F., Castellani, F., Di Filippo, P., Lorini, L., Majone, M., et al. (2020). Polychlorinated biphenyl profile in polyhydroxy-alkanoates synthetized from urban organicwastes. Polymers 2:659. doi: 10.3390/polym12030659
Samorì, C., Abbondanzi, F., Galletti, P., Giorgini, L., Mazzocchetti, L., Torri, C., et al. (2015). Extraction of polyhydroxyalkanoates from mixed microbial cultures: impact on polymer quality and recovery. Bioresour. Technol. 189, 195–202. doi: 10.1016/j.biortech.2015.03.062
Serafim, L. S., Lemos, P. C., Albuquerque, M. G. E., and Reis, M. A. M. (2008a). Strategies for PHA production by mixed cultures and renewable waste materials. Appl. Microbiol. Biotechnol. 81, 615–628. doi: 10.1007/s00253-008-1757-y
Serafim, L. S., Lemos, P. C., Torres, C., Reis, M. A. M., and Ramos, A. M. (2008b). The influence of process parameters on the characteristics of polyhydroxyalkanoates produced by mixed cultures. Macromol. Biosci. 8, 355–366. doi: 10.1002/mabi.200700200
Valentino, F., Moretto, G., Lorini, L., Bolzonella, D., Pavan, P., and Majone, M. (2019). Pilot-scale polyhydroxyalkanoate production from combined treatment of organic fraction of municipal solid waste and sewage sludge. Industr. Eng. Chem. Res. 58, 12149–12158. doi: 10.1021/acs.iecr.9b01831
Villano, M., Valentino, F., Barbetta, A., Martino, L., Scandola, M., and Majone, M. (2014). Polyhydroxyalkanoates production with mixed microbial cultures: from culture selection to polymer recovery in a high-rate continuous process. New Biotechnol. 31, 289–296. doi: 10.1016/j.nbt.2013.08.001
Wellen, R. M. R., Rabello, M. S. I, Araujo Júnior, C., Fechine, G. J. M., and Canedo, E. L. (2015). Melting and crystallization of poly(3-Hydroxybutyrate): effect of heating/cooling rates on phase transformation. Polímeros 25, 296–304. doi: 10.1590/0104-1428.1961
Werker, A., Bengtsson, S., Korving, L., Hjort, M., Anterrieu, S., Alexandersson, T., et al. (2018). Consistent production of high quality PHA using activated sludge harvested from full scale municipal wastewater treatment - PHARIO. Water Sci. Technol. 78, 2256–2269. doi: 10.2166/wst.2018.502
Werker, A., Johansson, P. S. T., and Magnusson, P. O. G. (2014). Process for the Extraction of Polyhydroxyalkanoates from Biomass. Patent No: US2015/0368393A1.
Keywords: polyhydroxyalakanoates, mixed microbial culture (MMC), thermal properties, molecular weight, urban waste
Citation: Lorini L, Martinelli A, Capuani G, Frison N, Reis M, Sommer Ferreira B, Villano M, Majone M and Valentino F (2021) Characterization of Polyhydroxyalkanoates Produced at Pilot Scale From Different Organic Wastes. Front. Bioeng. Biotechnol. 9:628719. doi: 10.3389/fbioe.2021.628719
Received: 12 November 2020; Accepted: 18 January 2021;
Published: 18 February 2021.
Edited by:
Prasun Kumar, Chungbuk National University, South KoreaReviewed by:
Rashmi Chandra, University of Waterloo, CanadaCopyright © 2021 Lorini, Martinelli, Capuani, Frison, Reis, Sommer Ferreira, Villano, Majone and Valentino. This is an open-access article distributed under the terms of the Creative Commons Attribution License (CC BY). The use, distribution or reproduction in other forums is permitted, provided the original author(s) and the copyright owner(s) are credited and that the original publication in this journal is cited, in accordance with accepted academic practice. No use, distribution or reproduction is permitted which does not comply with these terms.
*Correspondence: Laura Lorini, bGF1cmEubG9yaW5pQHVuaXJvbWExLml0
Disclaimer: All claims expressed in this article are solely those of the authors and do not necessarily represent those of their affiliated organizations, or those of the publisher, the editors and the reviewers. Any product that may be evaluated in this article or claim that may be made by its manufacturer is not guaranteed or endorsed by the publisher.
Research integrity at Frontiers
Learn more about the work of our research integrity team to safeguard the quality of each article we publish.