- 1Vaccinology & Immunotherapeutic Program, School of Public Health, University of Saskatchewan, Saskatoon, SK, Canada
- 2Vaccine & Infectious Disease Organization-International Vaccine Centre (VIDO-InterVac), University of Saskatchewan, Saskatoon, SK, Canada
- 3College of Agriculture, Fisheries and Forestry, Fiji National University, Nausori, Fiji
Polyphosphazenes are a class of experimental adjuvants that have shown great versatility as vaccine adjuvants in many animal species ranging from laboratory rodents to large animal species. Their adjuvant activity has shown promising results with numerous viral and bacterial antigens, as well as with crude and purified antigens. Vaccines adjuvanted with polyphosphazenes can be delivered via systemic and mucosal administration including respiratory, oral, rectal, and intravaginal routes. Polyphosphazenes can be used in combination with other adjuvants, further enhancing immune responses to antigens. The mechanisms of action of polyphosphazenes have not fully been defined, but several systematic studies have suggested that they act primarily by activating innate immunity. In the present review, we will highlight progress in the development of polyphosphazenes as adjuvants in animals and their other medical applications.
Introduction
Polyphosphazenes are high molecular weight, water-soluble, synthetic polymers that have been investigated for various biomedical applications including tissue regeneration and scaffolding, drug delivery, and stent and denture coatings. More recently, they have been extensively investigated as adjuvants to improve immune responses to vaccines. Vaccination continues to be a very important public health tool in the control of infectious diseases worldwide (Greenwood, 2014). Adjuvants are critical components of vaccines because they enhance antigen-specific immune responses that contribute to protection against disease. Subunit vaccines contain antigen components with varying degrees of purity. Highly purified antigens are poor immunogens and require the addition of adjuvants to generate protective immune responses. Polyphosphazenes are a relatively new class of adjuvants that enhance the magnitude, quality and duration of immune responses when co-administered with bacterial and viral antigens in several animal species including mice, pigs, sheep and cattle (McNeal et al., 1999; Andrianov et al., 2006, 2009, 2011; Mutwiri et al., 2007, 2008; Eng et al., 2010; Garlapati et al., 2011; Dar et al., 2012; Magiri et al., 2018). The two most investigated polyphosphazenes are poly [di(carboxylatophenoxy)phosphazene] (PCPP) and poly[di(sodiumcarboxylatoethylphenoxy)phosphazene] (PCEP) (Mutwiri and Babiuk, 2009). Changes in the synthesis and formulation as a soluble adjuvant or microparticle impacts how they influence immune responses (Andrianov et al., 2004). PCEP has been shown to significantly increase IgG1 and IgG2a antibody production compared to PCPP (Mutwiri et al., 2008) and also to induce 1,000-fold higher antibody titers compared to alum when co-administered subcutaneously with an influenza antigen in mice (Mutwiri et al., 2007). Relative to PCPP, PCEP also promotes a stronger mixed Th1/Th2-type T cell response leading to broad spectrum immunity (Mutwiri et al., 2007).
Polyphosphazenes
Chemical Composition and Structure
Polyphosphazenes are synthetic polymers that consist of a backbone with alternating phosphorus and nitrogen atoms and organic side groups attached to the phosphorus (Payne et al., 1998). The structures of the polyphosphazene molecules has been altered to incorporate ionic moieties resulting in water soluble salts. This, along with the potential hydrolytic degradability of their main chain make them attractive candidates for vaccine adjuvants and delivery systems. In this regard, two of the most investigated polyphosphazene adjuvants are PCEP and PCPP shown in Figure 1.
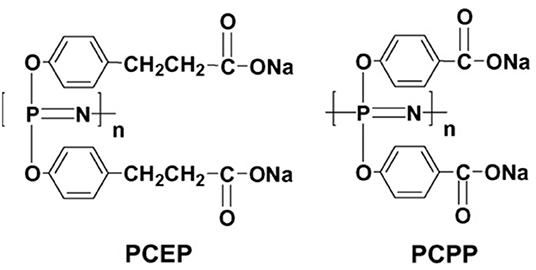
Figure 1. Chemical structures of the polyphosphazene polymers PCEP and PCPP (reprinted from Mutwiri et al., 2007, copyright 2007, with permission from Elsevier).
Synthesis Methods
The synthesis of PCEP was described in a previous publication (Mutwiri et al., 2007) as follows: PCEP was prepared by reacting a solution of sodium salt of methyl 3-(4-oxyphenyl) propionate with polydichlorophosphazene in diglyme at 120°C for 10 h. The resulting polymer, containing ester groups in side chains was hydrolyzed using aqueous potassium hydroxide at 85°C. Polymer was recovered by precipitating in ethanol and additionally purified by precipitating in sodium chloride solution and then in ethanol in its salt form. The high molecular weight nature of the polymer was confirmed by gel permeation chromatography, which was configured as follows: Waters 600 HPLC pump, Waters 717 plus Autosampler, an Ultra- hydrogel Linear column, a multi-angle laser light scattering (MALLS) detector (DAWNDSP-F, Wyatt Technology, Santa Barbara, CA), a Waters 996 Photo Diode Array detector, and a Waters 410 refractive index detector (Waters, Milford, MA). Phosphate buffered saline (PBS, pH 7.4) containing 5% acetonitrile was used as a mobile phase. Polyphosphazene adjuvants PCPP and PCEP were designed and synthesized by Parallel Solutions Inc. (Cambridge, MA). Aqueous solutions of both polymers were stored at room temperature in the dark and were found to retain activity for over several months under these storage conditions. Batches of polyphosphazenes were tested and found to have endotoxin levels that were below 0.034 ng/ml as assessed by Limulus Amebocyte Lysate assay (Biowhittaker, Walkersville, MD, USA). The synthesis of PCPP was described previously [13] and will not be repeated here.
Preparation of Antigen-Polyphosphazene Formulations
One of the most attractive features of polyphosphazene adjuvant is the simplicity with which an antigen-adjuvant formulation can be prepared. These polyphosphazenes are water soluble and can be simply mixed with antigen solutions at room temperature. Antigens spontaneously self-assemble with the polyphosphazenes into complexes predominantly formed through electrostatic interactions between negatively charged polyphosphazene and positively charged antigens, and stabilization of complexes is achieved through formation of hydrogen bonds and hydrophobic interactions (Andrianov et al., 2016). Details of interactions between polyphosphazenes and various antigens and the sizes of the resulting antigen-polyphosphazene complexes (generally 70–150 nm in diameter) have been reported in a recent review by Andrianov and colleagues (Andrianov and Langer, 2021).
Mechanisms of Action of Polyphosphazene Adjuvants
The MOA of PCEP or PCPP has not been fully elucidated. Early studies suggested that PCPP did not act by depot effect, since excision of injection sites in mice had no effect on the ensuing immune responses (Payne et al., 1998). While there are still gaps in our understanding of its MOA, we have gained significant knowledge on how PCEP interacts with the immune system to enhance antigen-specific immune responses. Most of the information on the MOA of polyphosphazene adjuvants has been from studies in which animals were injected with antigen alone, in the absence of antigen (Supplementary Table 2). Based on evidence from several MOA studies in mice, we have proposed a model to explain the MOA of PCEP, which is summarized in a diagram (Figure 2). Evidence in support of this model is provided below under five (5) subheadings.
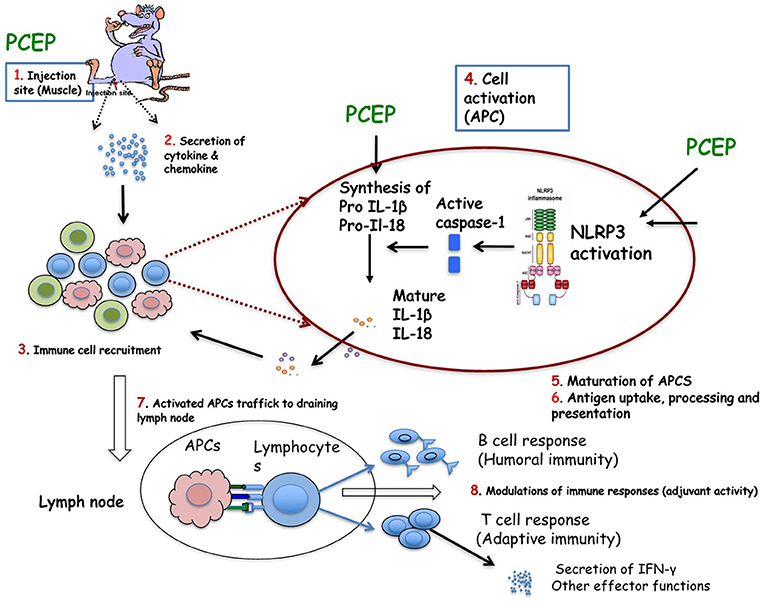
Figure 2. Proposed mechanism of action of PCEP: (1) PCEP injected into muscle is transiently retained locally, induces genes involved in innate immunity. (2) PCEP induces local cells to secret cytokines and chemokines. (3) The secreted cytokines and chemokines initiate recruitment of various immune cells, including APCs (such as DCs) to the injection site. The recruited cells are activated and secrete more cytokines and chemokines, which in turn chemoattracts other immune cells. All these events lead to formation of local immunocompetent environment at the injection site. (4) Recruited DCs (which express various PRRs both on the surface (TLRs, CLRs) and intracellularly (NLRs and RLRs) are recognized and/or activated by PCEP resulting in activation of inflammasome. It is not yet known whether PCEP modulates (5) Antigen uptake, process and presentation, or (6) maturation of APCs. (7) PCEP increases the trafficking of APCs to the draining lymph nodes to interact with antigen-specific B or T cell to (8) Resulting in potent antibody secreting B cells and/or effector CD8+ T cell responses. These events constitute adjuvant activity.
Upregulation of Cytokines and Chemokines
IM injection of mice with PCEP induced gene expression of many genes associated several adjuvants including chemokine genes CCL-2, CCL-4, CCL-5, CCL-12 and CXCL-10 in mice (Awate et al., 2012). Additionally, a major transcription factor NF-kB gene and the inflammatory cytokine TNF-α gene were also up-regulated in response to PCEP in mice (Awate et al., 2012). At the protein level, PCEP promoted significant local production of Th1-type proinflammatory cytokines (IL-1β, Il-6, IL-18 IFN-γ and TNF-α) and Th2-type cytokines (IL-4 and monocyte chemo-attractants CCL-2 and CXCL-10) at the site of injection in mice but not systemically (Awate et al., 2012).
In pigs, PCEP induced significant production of interleukin IL-1β and IL-13 at the site of injection and IL-1β and IL-6 at the draining lymph nodes. Emulsigen (a commercially available oil-in-water emulsion adjuvant for animal vaccines) promoted the production of IL-1β, IL-6, and IL-12 at the site of injection but not in the draining lymph nodes (Magiri et al., 2019).
Cell Recruitment to the Site of Injection
PCEP have been shown to induce recruitment of immune cells to the site of injection in mice. This local cell recruitment was presumably mediated by the several chemokines released as noted in Upregulation of Cytokines and Chemokines above. The recruited cells peaked within a week and were predominantly neutrophils, but also macrophages, CD4+ T cells, CD8+ T cells and CD19+ B cells, monocytes and DCs to the injection site (Awate et al., 2014b). The numbers of recruited cells were significantly higher in mice immunized with PCEP adjuvanted vaccines than in the alum-adjuvanted vaccines. Confocal analysis revealed that many recruited myeloid cells (but only a few lymphocytes) showed evidence of intracytoplasmic lysosomal localization of PCEP (Awate et al., 2014b) which PCEP potentiates immune responses.
In pigs, PCEP induced recruitment of macrophages, T and B cells, leucocytes and necrotic debris at the site of injection (Magiri et al., 2019).
Activation and Maturation of Dendritic Cells
In in vitro studies, PCEP activated the NLRP3 inflammasome in a Caspase 1-depedent manner which led to the processing of interleukin IL-1β, IL-18 and IL-33 stimulated splenic DCs in mice (Awate et al., 2014a). However, while inflammasome activation may contribute to adjuvant activity by promoting a pro-inflammatory environment at the site of injection, it is yet to be confirmed whether inflammasome activation is required for the adjuvant activity of PCEP. Interestingly, PCEP injection in mice increased the expression of TLR4 and TLR9 at the site of injection (Awate et al., 2012). The biologic significance of this observation is unclear, given that PCEP is not of microbial origin. PCEP has strong avidity for TLR7, TLR8, and TLR9 (Sasai and Yamamoto, 2013; Andrianov et al., 2016). Furthermore, direct activation of immune cells by PCPP and PCEP through TLR signaling pathway, both on the external cell surface (TLR4) and endosome (TLR3 and TLR9) receptors has been reported (Reed et al., 2013; Sasai and Yamamoto, 2013). It was reported that polyphosphazene adjuvants also induced the maturation of DCs in vitro (Andrianov et al., 2006, 2016).
Antigen Uptake, Processing and Presentation
In vitro studies suggested that PCPP promoted the uptake of Gag antigen of HIV, increased expression of co-stimulatory molecules in DCs and enhanced Gag antigen presentation to CD4+ T cells (Palmer et al., 2014). In a separate study, it was reported that PCEP, but not PCPP, disrupted early endosomes, suggesting that PCEP may promote cross-presentation of antigen (Andrianov et al., 2016). If this is the case, then PCEP promotes the ability of DCs to take up, process, and present extracellular antigens with MHC class I molecules to CD8 T cells, a pathway critical for the development of Th1 responses. This response may explain, at least in part, why PCEP induces a mixed Th1/Th2 while PCPP induces predominantly Th2 and no Th1 type immune responses.
Trafficking of Activated Cells to the Draining Lymph Nodes
IM injection of mice with PCEP resulted in increased numbers of myeloid cells and lymphocytes in draining lymph nodes (Awate et al., 2014b). Neutrophils and DCs were detected in the lymph nodes as early as 3 h after injection, while macrophages followed in the next 24 h. Interestingly, lymphocytes (mainly CD4+ and CD8+ T cells) were also increased in the lymph nodes after injection of PCEP (Awate et al., 2014b). All the cells types were significantly higher in mice injected with PCEP compared to those injected with alum. In pigs, PCEP induced infiltration of leucocytes, resulting in a local inflammatory response in the draining lymph nodes (Magiri et al., 2019).
Therefore, the MOA of PCEP involves early activation innate immune responses that involves cell recruitment to site of injection cell activation promoting a strong immunostimulatory environment at the site of injection. and then trafficking of activated cells to the draining lymph nodes.
Application of Polyphosphazenes as Vaccine Adjuvants in Various Animal Species
Viral Antigens
Polyphosphazenes have been used as experimental adjuvants in a variety of viral antigens. Immunization of mice with influenza virus X:31 antigen co-formulated in PCPP increased IgG titers upon subcutaneous (SC) immunization in mice (Andrianov et al., 2004; Mutwiri et al., 2007). When compared to the conventional adjuvant alum, PCPP and PCEP both induced antibody titers that were 100-1,000-fold higher than alum (Figure 3). The differences between the three adjuvants were more dramatic when IgG2a titers were examined. PCEP induced IgG2a titers that were 100-fold higher than PCPP and several orders of magnitude (more than 10,000-fold) higher than alum (Figure 1). Furthermore, PCEP promoted both IgG1 and IgG2a titers (indicative of a mixed Th1/Th2 type immune response), while both PCPP and alum promoted a Th2 type but no Th1, as indicated by poor IgG2a antibody response (Mutwiri et al., 2007). In addition, PCEP required only 1/5 of the dose of X:31 antigen to induce antibody titers similar to alum (Figure 3). Thus, PCEP has the potential to reduce the cost of vaccination by reducing the number of vaccinations and since only minimal doses of antigen are required to induce significant immunity, the cost of vaccination could be dramatically reduced. In a separate study influenza, PR8 antigen of influenza Virus H1N1 adjuvanted with PCPP increased mucosal IgA, enhanced IgG1 and IgG2a antibody responses, induced a mixed Th1/Th2 cytokine responses which contributed to long term B cell memory response following both systemic and mucosal immunization in mice (Shim et al., 2010). Furthermore, the immune responses induced were protective against lethal H1N1 challenge (Shim et al., 2010). In ferrets, PCPP adjuvanted influenza vaccine HA H5N1 demonstrated dose sparring, increased vaccine stability, and an increased survival rate in lethally challenged animals (Andrianov et al., 2011).
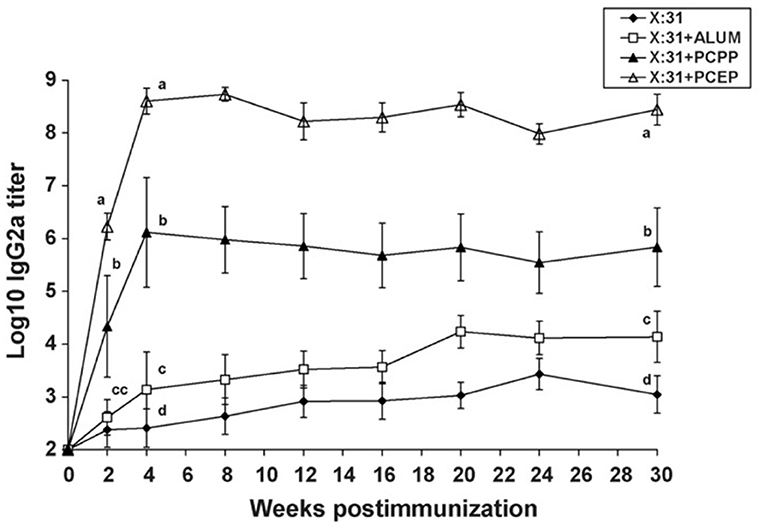
Figure 3. Immune responses to low dose of influenza virus X:31 antigen: IgG2a antibody responses were assayed in serum of BALB/c mice (n = 5 per group) given a single s.c. immunization with a low dose (0.2_g) of X:31 antigen alone, X:31 + alum, X:31 + PCPP or X:31 + PCEP. Each data point represents mean ± S.E.M. for titers as determined by ELISA. Groups with different letters are significantly different from each other (p < 0.05). Data are representative of two independent experiments (reprinted from Mutwiri et al., 2007, Copyright 2007, with permission from Elsevier).
Coformulation of the viral antigens such as HBsAg, PR8, or influenza X:31 with PCEP resulted in significant increases in both IgG1 and IgG2a antibody responses in mice (Mutwiri et al., 2007, 2008; Shim et al., 2010). Recombinant MV-H (Measles Virus recombinant “H”) protein of measles virus adjuvanted with PCEP induced significant IgG1 and IgG2a antibody responses and neutralization titers in vivo (Lobanova et al., 2012). In vitro, increased MV-H-specific IFN-γ and IL-5 was observed in the splenocytes indicating strong cell mediated immunity (Lobanova et al., 2012). Further, the recombinant protein's immunogenicity was retained 2 weeks after storage, suggesting the vaccine formulation was stable (Andrianov et al., 2011; Lobanova et al., 2012). PCEP efficiently promoted a balanced Th1/Th2 type response, following both mucosal and systemic immunization, indicating that PCEP is versatile as an adjuvant (Mutwiri et al., 2007; Eng et al., 2010).
In recent studies in pigs, PCEP was shown to be an effective adjuvant with an experimental swine influenza vaccine. PCEP promoted strong immune responses and protection against homologous swine influenza H1N1 virus but failed to cross-protect against the heterologous H3N2 virus (Magiri et al., 2018, 2020). A vaccine with PCEP administered intradermally was superior to that injected intramuscularly (IM), suggesting the importance of route of administration in inducing protective immunity (Magiri et al., 2020). Respiratory syncytial virus (RSV-F), HN of parainfluenza virus 3 and inclusion body hepatitis virus (IBHV) antigens have also been used with polyphosphazene adjuvants, but these are discussed under combination adjuvants further below in this review.
Bacterial and Other Types of Antigens
Polyphosphazene adjuvants have also been used with bacterial antigens. Pertussis toxoid (PTd) antigen elicits a Th-1 biased response when adjuvanted with PCEP (Gracia et al., 2011), or even multiple adjuvants such as a triple adjuvant formula like PCEP+CpG+IDR when given by IN administration in mice (Garlapati et al., 2011). Subcutaneous injection of pigs with an Actinobacillus pleuorpnemoniae (APP) outer membrane antigen (OmlA) adjuvanted with PCEP showed significantly increased IgG1 and IgG2a antibody responses and also increased IFN-γ when compared to pigs vaccinated with OmlA adjuvanted with the commercial adjuvant, Emulsigen (Dar et al., 2012).
A large proportion of studies performed using polyphosphazenes as adjuvants have been centered around academic antigens such as ovalbumin (OVA). Because these antigens alone do not induce strong immune responses, they are often used alongside adjuvant formulations such as the triple adjuvant formulation (Garlapati et al., 2011) to better relay the abilities of the adjuvant in relation to an antigen. Studies involving academic antigens Bovine Serum Albumin (BSA) with PCPP in mice promoted increased serum IgG, however such titers were dependent on the structure of the PCPP backbone (Andrianov et al., 2005). PCPP administered with OVA promoted increased IgG1 and IgG2a antibody response and ultimately a predominantly Th1 response (Garlapati et al., 2010), findings which have been further corroborated by more recent studies involving PCEP as a combination adjuvant. Oral administration of PCEP with OVA induced significant anti-OVA specific IgM and IgG, alongside enhanced IgG1 and IgG2a serum antibodies in pigs (Pasternak et al., 2014).
Comparison of Routes of Administration
Polyphosphazene-adjuvanted vaccines have been tested in a variety of routes including IN, SC, intrauterine (IU), Oral, and intrarectal (IR) with various antigens and in different animal models and in humans (Supplementary Table 1). However, parenteral immunization has been the predominant method of vaccination for animals (Zhang et al., 2015). Historically, subcutaneous injection has been the most commonly used method as it represents the route of application of many animal vaccines to date (Cook, 2008).
In a study evaluating various routes of administration of influenza virus X:31 antigen, IN and SC had superior adjuvant activity compared to the Oral and IR routes; significantly higher IgG1 titers and increased IgG and IgA in nasal secretions yielded results conferring systemic and humoral response not seen with IR or Oral vaccination (Eng et al., 2010). Intranasal (IN) immunization with PCEP+X:31 induced significantly higher IgA titers in all mucosal secretions (nasal, lung, vaginal) compared to the other routes, particularly the IR route which showed negligible immunogenicity (Eng et al., 2010).
While IN and SC have proven to have more optimal response than Oral or IR, the distinction between the two is also significant. In a study where IN immunization was compared to SC, there were distinct differences in antibody response, neutralizing titers, and viral replication and protection against challenge. A two-dose study comparing IN/IN, IN/SC, SC/IN, and SC/SC, revealed that two IN immunizations (IN/IN) were the most effective in inducing increased IgG1 and IgG2a, as well as reducing viral replication in the lungs and conferring protection in experimentally infected mice (Mapletoft et al., 2010).
Intradermal (ID) immunization outperforming IM injection in pigs is a theme documented by a 2018 study comparing ID and IM with swine influenza virus (SIV) antigen. PCEP adjuvanted pigs injected ID displayed greater induction of humoral immunity than IM injected pigs (Magiri et al., 2018). Furthermore, the ID vaccinated group also developed greater neutralizing titers compared to IM (Magiri et al., 2018) hence ID was proven to be the superior route in pigs. The superior performance of the ID route is presumably due to the presence of professional antigen presenting cells (APCs) such as dendritic cells (DC) are more frequent in the skin relative to muscle tissue.
In another study in pigs, it was noted that when Hepatitis B Surface Antigen (HBsAg) was injected alongside PCPP, ID vaccination yielded 10-times higher IgG titers; and also induced significantly higher HBsAg-specific titers than IM injection (Andrianov et al., 2009). ID injection of HBsAg adjuvanted with PCPP displayed a significant dose sparring effect, where a 10 μg ID dose induced 10-fold higher antibody titer than 20 μg of the antigen alone (Andrianov et al., 2009). The significance of PCPP as a part of vaccine formulation was also confirmed by 100-fold increase in antibody titers for the polyphosphazene adjuvanted microneedle system over the ID injection of non-adjuvanted antigen (Andrianov et al., 2009).
Intrauterine vaccination has produced mixed results between pigs and rabbit species as it pertains to the use of Tri-Adj formulations containing PCEP. In pigs, inactivated PPV and PEDV+FliC antigens formulated with Tri-Adj failed to elicit a humoral immune response, despite the adjuvant's ability to directly stimulate chemokines CCL2, IFN-β, and CCL4 in uterine epithelial cells (UECs) (Hamonic et al., 2020). In rabbits, when Tri-Adj formulation PCEP+poly:I:C+HDP was administered with three different antigens, OVA, tGD, and vP2-TrX, IM and IU routes both demonstrated increased IgG and IgA serum titers for OVA and tGD antigens (Pasternak et al., 2017). However, in this same experiment it became apparent that the IU route was not as effective in stimulating systemic immunity, as indicated by increased IgG only via the IM route for the rVP2-TrX antigen (Pasternak et al., 2017).
Combination of Polyphosphazenes With Other Adjuvants
Why Use Combinations of Adjuvants?
Historically, vaccines have employed a model of “one adjuvant, one vaccine,” mainly because of regulatory hurdles on safety and/or for purely economic reasons. It is unlikely that a single adjuvant will be able to fulfill all the required immunologic properties in the many different vaccines. For example, alum is known to only stimulate Th2 immune cells leading to increased production of antigen-specific antibodies but is poor or even inhibitory to Th1 or cytotoxic responses. This limitation in single adjuvants can be alleviated by the development of adjuvant combinations such as MF59, AS03, or AS04 that seek to further strengthen antigen-specific humoral (Th2) responses and also broaden responses by increasing Th1 or cytotoxic responses. Evidence has been accumulating over the last two decades that formulation of a vaccine with multiple adjuvants may act synergistically to elicit dramatic increases in mixed Th1 and Th2 types of immune responses (Kindrachuk et al., 2009; Mutwiri et al., 2011; Salvador et al., 2012; Mount et al., 2013; Levast et al., 2014; Ciabattini et al., 2016; Didierlaurent et al., 2017; Madan-Lala et al., 2017). Furthermore, different adjuvants are required to stimulate immune responses required following different vaccination strategies taking into account the pathogen, the type of antigen, the immune status and age of the vaccine and the application route in order to improve vaccine efficacy (Garçon and Di Pasquale, 2017). Most importantly, the stimulation of a cell-mediated Th1 response and cytotoxic T lymphocytes (CTLs) is highly sought since actual vaccines antigen mostly stimulate predominantly humoral (Th2) immune responses. Combination adjuvants are particularly used to enhance and/or direct the immune responses toward a Th1-, Th2-, or Th17-type responses (Kindrachuk et al., 2009; Salvador et al., 2012; Levast et al., 2014). Adjuvants target different pattern-recognition receptors (PRR), both endosomal and intracellular. Their combinations based on these targets can enhance antigen-specific humoral and cellular immune responses (Gutjahr et al., 2016). For instance, Toll-like receptor (TLR) agonist MPL A adsorbed on aluminum salts (AS04TM, GlaxoSmithKline) in a combination results in the stimulation of increased production of antigen-specific antibodies and an enhanced cell-mediated response by causing a local and temporary cytokine response (Reed et al., 2009; Garcon et al., 2011; Del Giudice et al., 2018). Due to the short half-life of most immunostimulatory adjuvants in vivo, combining a delivery vehicle adjuvant with an immunostimulatory adjuvant like polyphosphazene may increase the magnitude and modulate the quality of immune responses (Weiner et al., 1997).
Examples of Combination Adjuvants Containing Polyphosphazenes
As demonstrated with numerous other adjuvants, the adjuvant potential of polyphosphazenes can be greatly enhanced by combining with other adjuvants. Subcutaneous immunization of mice with Hepatitis B surface antigen (HBsAg) plus PCEP or PCPP combined with the TLR agonist CpG ODN resulted in enhanced production of HBsAg-specific antibody responses compared with mice immunized with HBsAg plus any of the three adjuvants when used alone (Mutwiri et al., 2008). When IgG2a antibody titers were compared, CpG ODN and PCEP both induced comparable titers, but the combination of PCEP+CpG ODN produced ~100-fold higher titers than the individual adjuvants (Figure 3). The titers were maintained for almost 6 months (Figure 4). Similarly, immunization of mice with PCPP microparticles encapsulating OVA and CpG ODN generated higher antigen-specific antibody responses compared to antigen alone (Garlapati et al., 2010; Wilson et al., 2010).
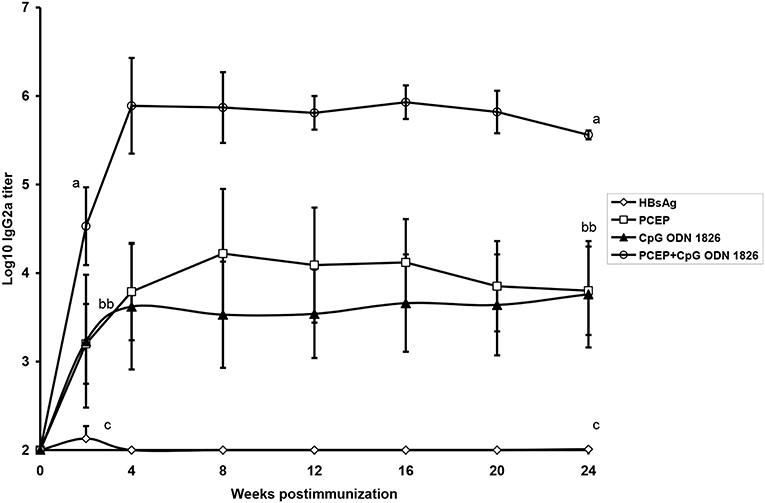
Figure 4. Serum HBsAg-specific IgG2a antibody titers in mice given a single SC immunization with a dose of 0.2 _g of HBsAg alone, HBsAg + PCEP, HBsAg + CpG or HBsAg + PCEP + CpG. Each data point represents mean ± S.E.M. of titers of anti-HBsAg as determined by ELISA. Groups with different letters are significantly different (p < 0.05) (reprinted from Mutwiri et al., 2008, Copyright 2007, with permission from Elsevier).
In several studies it has been demonstrated that the use of polyphosphazenes in triple adjuvant combination (TriAdj) consisting of PCEP or PCPP plus TLR agonist (CpG or poly:I:C) and Host Defense Peptide (HDP) induced robust immune responses with various antigens, in multiple species and in diverse routes of delivery. Similar observations were made in a separate study, mice immunized with OVA plus TriAdj combination significantly enhanced antibody and cell mediated immune responses via both MHC class-I and MHC class-II pathways, promoting a more balanced Th1/Th2 immune response, compared to mice immunized with OVA plus any of the individual adjuvants (Kovacs-Nolan et al., 2009a). Further, S.C. immunization of mice with Bordetella pertussis antigen plus TriAdj reduced bacterial load after challenge and increased antigen-specific IL-17 secreting cells relative to vaccine containing only one or two of the other adjuvants (Garlapati et al., 2011). Furthermore, TriAdj formulation with PTd increased IgG1 responses in adult mice in addition to superior serum IgG2a antibody titers in both adult and neonatal mice compared to mice immunized with each adjuvants alone (Gracia et al., 2011). Additionally, recombinant truncated bovine respiratory syncytial virus (bRSV) fusion protein (Δ-F) plus TriAdj enhanced secretion of antigen-specific serum antibody titres compared with mice immunized with antigen alone (Kovacs-Nolan et al., 2009c).
IN vaccination with a formalin-inactivated bRSV antigen plus TriAdj induced systemic and mucosal immunity in mice (Mapletoft et al., 2010) which then significantly reduced viral replication upon virulent bRSV virus challenge in mice (Mapletoft et al., 2008). OVA antigen has also been formulated with lipidic (L) Tri-Adj, and microparticle formulated (MP) Tri-Adj and given intranasally in mice. These formulations all resulted in balanced Th1/Th2 responses, but with L-Tri-Adj being the most effective and inducing significantly greater levels of IgG1 titers than the other formulations, as well as enhanced IgA response (Wasan et al., 2019). L-Tri-Adj and MP Tri-Adj both also promoted increased IgG2a and IFN- γ from lymphocytes indicating a stronger CMI than that of Tri-Adj alone (Wasan et al., 2019).
Subcutaneous immunization of cattle on days 0 and 90 with hen egg lysozyme (HEL) antigen adjuvanted with TriAdj showed superior antigen-specific humoral responses and cell-mediated immune responses relative to immunization with antigen alone (Kovacs-Nolan et al., 2009b). Purified E2 antigen of BVDV-2 (Bovine viral diarrhea virus) formulated in TriAdj and administered IM significantly increased E2-specific VN antibodies along with IFN-γ, CD4+/CD8+ T cells, indicative of a mixed Th1/Th-2 type immune responses (Snider et al., 2014). The purified E2 antigen also stimulated significant protection against virulent BVDV-2 4 weeks after vaccination; compared to the control PBS-immunized cattle, those immunized with the E2 vaccine had lower body temperatures, significantly less weight loss, and significantly higher WBC counts than the PBS treated cows during days 6–12 of the trial (Snider et al., 2014). Furthermore, increased expression of the CD25+ marker which indicated the presence of CTLs was also detected and with the exception of a single animal, vaccinated calves did not show evidence of viral replication (Snider et al., 2014). A similar robust antibody response was also noted in an ID immunization with the same adjuvant and antigen formulation where APCs and DCs were significantly increased in number upon challenge with no clinical sickness, leukopenia, or viral shedding observed (Sadat et al., 2017).
Respiratory syncytial virus F (RSV-F) protein formulated with Tri-Adj (CpG ODN+PCEP+HDP) induced a robust immune response in mice immunized via IN route. Mucosal and systemic immunity was effectively induced as indicated by the overall antibody responses including IgA, IgG1, and IgG2a (Garg et al., 2014, 2016, 2017, 2019; Sarkar and Garg, 2016). Significant antigen-specific CD8+T cell production was also observed (Garg et al., 2014, 2017), as well as reduction in viral replication in the lungs (Sarkar and Garg, 2016; Garg et al., 2017). Furthermore, interferon secretion, monocyte and DC trafficking to lymph nodes and recruitment were also observed with this formulation (Garg et al., 2014; Sarkar and Garg, 2016). This experimental vaccine formulation activated prolonged immunity in both arms of adaptive immunity and ultimately provided protection against a virulent RSV challenge (Garg et al., 2017). In a separate study, RSV-F and HN of para-influenza virus 3 (PIV3) antigens administered IM adjuvanted with Tri-Adj resulted in more efficient transfer of maternal antibodies from ewes to newborn lambs (Garg et al., 2019).
In rabbits, IM or IU immunization with a single dose of OVA, truncated glycoprotein D (tGD) from bovine herpesvirus, and a fusion protein of porcine parvovirus protein VP2 and bacterial thioredoxin (rVP2-TrX) formulated with TriAdj induced antigen-specific antibody responses systemically (serum) and within the local (uterus) and distal mucosa (lungs and vagina) (Pasternak et al., 2017). Thus, PZ as part of the TriAdj combination dramatically enhanced the magnitude of immune responses in variety of viral and bacterial antigens resulting in balanced immunity for broader protection.
Chickens have also been the subject of studies in combination adjuvant studies. Eighteen-day-old embryonating eggs were inoculated with PCPP/PCEP+CpG ODN, and 4 days after exposure to the adjuvants or 1 day post hatch, they were inoculated SC with E. coli (Taghavi et al., 2009). No adverse reactions or impacts on hatchability were observed, along with significant decrease in mortality in embryos immunized compared to the control group (Taghavi et al., 2009). Birds that received CpG ODN + PCEP had a significantly higher survival rate compared to the other groups (55%), and relative risk of mortality was significantly reduced in PCPP (0.25) and PCEP (0.33) formulations as well (Taghavi et al., 2009). In a separate study, immunization of chickens with inactivated IBHV vaccine formulated in PCEP with avian beta defensin (ABD) induced significant increases in IgG antibody response, IFN-γ, IL-12 (p40), IL-6, and overall a balanced Th1/Th2 immune response (Dar et al., 2015).
Discussion
In the last few decades, there has been increased interest in research on adjuvants leading to identification of numerous substances with adjuvant potential. Some of these new adjuvants have undergone pre-clinical development, but few ever make it into clinical trials, and even fewer ever become used in commercial vaccines.
There are several challenges to using new adjuvants in commercial vaccines, and these challenges go beyond proof of efficacy. Most, if not all, vaccine companies have their own adjuvants. One of the widely known portfolio of adjuvants is GSK's (Glaxo Smith Kline) adjuvant system (AS), which has relatively new adjuvants AS01 and AS03 incorporated in several new and existing vaccines.
It would take a significant investment to make such a change of adjuvant from existing vaccines. The vaccine company would have to buy rights for using the new adjuvant from the party that owns the IP rights. Also, including a new adjuvant to an existing vaccine will require regulatory approvals as adjuvants are only approved as part of a vaccine, adding another layer of substantial cost. It may also require that changes be made in the manufacturing processes. These enormous costs alone make it extremely challenging for new adjuvants to penetrate the vaccine market.
While we have gained knowledge in how adjuvants work, there are still gaps in our understanding of the MOA of adjuvants. Many factors are bound to affect how adjuvants mediate their immunomodulatory effects. We now know that adjuvant activity can vary remarkably depending on the animal species. For example, CpG ODN used alone is a strong adjuvant in mice and chicken, but is a poor adjuvant in large animals, unless it is used in combination with other adjuvants (reviewed in Mutwiri et al., 2011). The reasons for these remarkable species differences remains unknown.
It should be noted that immunostimulatory adjuvant work by activating innate immune responses which constitute an inflammation response, which if excessive can be deleterious to the host. Thus, too much inflammation will cause pathology and disease, while too little or none may result in lack of adjuvant activity. However, there is no evidence to support this notion. Then the question arises, what is the relationship between degree or nature of the innate immune responses and adjuvant activity? The answer to this question will be valuable in the development of effective and safe adjuvants.
Furthermore, in MOA studies adjuvants are typically used alone with no antigen (Supplementary Table 2), and essentially no studies have explored the possibility that the nature or composition of antigen may influence whether or not a substance has adjuvant activity. The question that we rarely ask is: what is the contribution of the antigen in the overall adjuvant activity of a vaccine? Vaccines typically comprise of antigen + adjuvant, and vaccine antigens can vary in origin and composition. Un-purified microbial antigens may contain components such as CpG DNA or LPS that have known adjuvant activity. In this regard, the resulting antigen-specific immune responses seen in vaccinated animals may be a result of a combination of adjuvant activity from the combination of adjuvant and antigen, such as seen with polyphosphazene plus CpG ODN discussed earlier (Mutwiri et al., 2008). This may explain why a particular adjuvant may promote antigen-specific immune responses in some antigens and not in others. This is a critical piece of information in the rational design of adjuvant+antigen pairing for effective vaccines.
Conclusion
Over the last decade, evidence has accumulated that polyphosphazenes are potent adjuvants for viral and bacterial vaccine candidates in several animal species. Furthermore, they are effective given by the various routes, and in adults and neonatal animals. These experimental adjuvants are relatively safe, although systematic safety studies are needed. Going forward, there is sufficient pre-clinical data with the newer, more potent polyphosphazene PCEP and its combinations to warrant clinical trials in animals.
Author Contributions
DC reviewed literature and Prepared first draft of manuscript. GM primary author with overall oversight of the manuscript, conceived idea, checked accuracy of contributions in the manuscript, and edited manuscript. HW contributed material on medical applications of polyphosphazenes. RM contributed material on combination adjuvants. All authors contributed to the article and approved the submitted version.
Funding
Funding in the authors' laboratories was provided by Natural Sciences and Engineering Research Council (# 402993), Government of Saskatchewan through Agricultural Development Fund (# 20150263) and Government of Alberta through Alberta Livestock and Meat Agency (# 2012F055R).
Conflict of Interest
GM holds a patent in polyphosphazene adjuvants and their combinations.
The remaining authors declare that the research was conducted in the absence of any commercial or financial relationships that could be construed as a potential conflict of interest.
Acknowledgments
We would like to thank Dr. Sunita Awate for her help in preparing Figure 2. This manuscript is published as VIDO-InterVac manuscript series number 919.
Supplementary Material
The Supplementary Material for this article can be found online at: https://www.frontiersin.org/articles/10.3389/fbioe.2021.625482/full#supplementary-material
References
Andrianov, A. K., DeCollibus, D. P., Gillis, H. A., Henry, H. K., Marin, A., Prausnitz, M. R., et al. (2009). Poly [di (carboxylatophenoxy) phosphazene] is a potent adjuvant for intradermal immunization. Proc. Natl. Acad. Sci. U.S.A. 106, 18936–18941. doi: 10.1073/pnas.0908842106
Andrianov, A. K., Decollibus, D. P., Marin, A., Webb, A., Griffin, Y., Webby, R., et al. (2011). PCPP-formulated H5N1 influenza vaccine displays improved stability and dose-sparing effect in lethal challenge studies. J. Pharm. Sci. 100, 1436–1443. doi: 10.1002/jps.22367
Andrianov, A. K., and Langer, R. (2021). Polyphosphazene immunoadjuvants: historical perspective and recent advances. J. Control. Release 329, 299–315. doi: 10.1016/j.jconrel.2020.12.001
Andrianov, A. K., Marin, A., and Chen, J. (2006). Synthesis, properties, and biological activity of poly [di (sodium carboxylatoethylphenoxy) phosphazene]. Biomacromolecules 7, 394–399. doi: 10.1021/bm050790a
Andrianov, A. K., Marin, A., and Fuerst, T. R. (2016). Molecular-level interactions of polyphosphazene immunoadjuvants and their potential role in antigen presentation and cell stimulation. Biomacromolecules 17, 3732–3742. doi: 10.1021/acs.biomac.6b01251
Andrianov, A. K., Marin, A., and Roberts, B. E. (2005). Polyphosphazene polyelectrolytes: a link between the formation of noncovalent complexes with antigenic proteins and immunostimulating activity. Biomacromolecules 6, 1375–1379. doi: 10.1021/bm049329t
Andrianov, A. K., Svirkin, Y. Y., and LeGolvan, M. P. (2004). Synthesis and biologically relevant properties of polyphosphazene polyacids. Biomacromolecules 5, 1999–2006. doi: 10.1021/bm049745d
Awate, S., Eng, N. F., Gerdts, V., Babiuk, L. A., and Mutwiri, G. (2014a). Caspase-1 dependent IL-1β secretion and antigen-specific T-Cell activation by the novel adjuvant, PCEP. Vaccine 2, 500–514. doi: 10.3390/vaccines2030500
Awate, S., Wilson, H. L., Lai, K., Babiuk, L. A., and Mutwiri, G. (2012). Activation of adjuvant core response genes by the novel adjuvant PCEP. Mol. Immunol. 51, 292–303. doi: 10.1016/j.molimm.2012.03.026
Awate, S., Wilson, H. L., Singh, B., Babiuk, L. A., and Mutwiri, G. (2014b). The adjuvant PCEP induces recruitment of myeloid and lymphoid cells at the injection site and draining lymph node. Vaccine 32, 2420–2427. doi: 10.1016/j.vaccine.2014.03.014
Batista-Duharte, A., Martínez, D. T., and Carlos, I. Z. (2018). Efficacy and safety of immunological adjuvants. Where is the cut-off? Biomed. Pharmacother. 105, 616–624. doi: 10.1016/j.biopha.2018.06.026
Capodanno, D., and Tamburino, C. (2010). Properties and clinical development of a novel coating technology: the poly[bis(trifluoroethoxy)phosphazene]. Recent Patents Drug Delivery Formula 4, 18–22. doi: 10.2174/187221110789957264
Ciabattini, A., Pettini, E., Fiorino, F., Pastore, G., Andersen, P., Pozzi, G., et al. (2016). Modulation of primary immune response by different vaccine adjuvants. Front. Immunol. 7:427. doi: 10.3389/fimmu.2016.00427
Cook, I. F. (2008). Evidence based route of administration of vaccines. Hum. Vaccin. 4, 67–73. doi: 10.4161/hv.4.1.4747
Dar, A., Lai, K., Dent, D., Potter, A., Gerdts, V., Babiuk, L. A., et al. (2012). Administration of poly[di(sodium carboxylatoethylphenoxy)]phosphazene (PCEP) as adjuvant activated mixed Th1/Th2 immune responses in pigs. Vet. Immunol. Immunopathol. 146, 289–295. doi: 10.1016/j.vetimm.2012.01.021
Dar, A., Tipu, M., Townsend, H., Potter, A., Gerdts, V., and Tikoo, S. (2015). Administration of poly [di (sodium carboxylatoethylphenoxy) phosphazene](PCEP) and avian beta defensin as adjuvants in inactivated inclusion body hepatitis virus and its hexon protein-based experimental vaccine formulations in chickens. Avian Dis. 59, 518–524. doi: 10.1637/11202-052815-Reg.1
Del Giudice, G., Rappuoli, R., and Didierlaurent, A. M. (2018). “Correlates of adjuvanticity: a review on adjuvants in licensed vaccines,” in Seminars in Immunology (Siena: Elsevier), 14–21. doi: 10.1016/j.smim.2018.05.001
Di Pasquale, A., Bonanni, P., Garçon, N., Stanberry, L. R., El-Hodhod, M., Da Silva, F., et al. (2016). Vaccine safety evaluation: practical aspects in assessing benefits and risks. Vaccine 34, 6672–6680. doi: 10.1016/j.vaccine.2016.10.039
Didierlaurent, A. M., Laupèze, B., Di Pasquale, A., Hergli, N., Collignon, C., and Garçon, N. (2017). Adjuvant system AS01: helping to overcome the challenges of modern vaccines. Expert Rev. Vaccines 16, 55–63. doi: 10.1080/14760584.2016.1213632
Dubensky, T. W., Kanne, D. B., and Leong, M. L. (2013). Rationale, progress and development of vaccines utilizing STING-activating cyclic dinucleotide adjuvants. Ther. Adv. Vaccines 1, 131–143. doi: 10.1177/2051013613501988
Ebensen, T., Delandre, S., Prochnow, B., Guzmán, C. A., and Schulze, K. (2019). The combination vaccine adjuvant system Alum/c-di-AMP results in quantitative and qualitative enhanced immune responses post immunization. Front. Cell. Infect. Microbiol. 9, 1–9. doi: 10.3389/fcimb.2019.00031
Eng, F. N., Garlapati, S., Lai, K., Gerdts, V., and Mutwiri, G. K. (2009). Polyphosphazenes enhance mucosal and systemic immune responses in mice immunized intranasally with influenza antigens. Open Vaccine J. 2, 1476–1485. doi: 10.2174/1875035400902010134
Eng, N. F., Garlapati, S., Gerdts, V., Babiuk, L. A., and Mutwiri, G. K. (2010). PCEP enhances IgA mucosal immune responses in mice following different immunization routes with influenza virus antigens. J. Immune Based Therapeutic Vaccines 8:4. doi: 10.1186/1476-8518-8-4
Garçon, N., and Di Pasquale, A. (2017). From discovery to licensure, the Adjuvant System story. Hum. Vaccines Immunotherapeutics 13, 19–33. doi: 10.1080/21645515.2016.1225635
Garcon, N., Morel, S., Didierlaurent, A., Descamps, D., Wettendorff, M., and Van Mechelen, M. (2011). Development of an AS04-adjuvanted HPV vaccine with the adjuvant system approach. BioDrugs 25, 217–226. doi: 10.2165/11591760-000000000-00000
Garg, R., Latimer, L., Gerdts, V., Potter, A., and van Drunen Littel-van den Hurk, S. (2017). Intranasal immunization with a single dose of the fusion protein formulated with a combination adjuvant induces long-term protective immunity against respiratory syncytial virus. Hum. Vaccines Immunotherapeutics 13, 2894–2901. doi: 10.1080/21645515.2017.1349584
Garg, R., Latimer, L., Gomis, S., Gerdts, V., and Potter, A. (2019). Maternal vaccination with a novel chimeric glycoprotein formulated with a polymer-based adjuvant provides protection from human parainfluenza virus type 3 in newborn lambs. Antiviral Res. 162, 54–60. doi: 10.1016/j.antiviral.2018.12.010
Garg, R., Latimer, L., Simko, E., Gerdts, V., and Potter, A. (2014). Induction of mucosal immunity and protection by intranasal immunization with a respiratory syncytial virus subunit vaccine formulation. J. General Virol. 95, 301–306. doi: 10.1099/vir.0.058461-0
Garg, R., Theaker, M., and Martinez, E. C. (2016). A single intranasal immunization with a subunit vaccine formulation induces higher mucosal IgA production than live respiratory syncytial virus. Virology 499, 288–297. doi: 10.1016/j.virol.2016.09.023
Garlapati, S., Eng, N. F., Kiros, T. G., Kindrachuk, J., Mutwiri, G. K., Hancock, R. E., et al. (2011). Immunization with PCEP microparticles containing pertussis toxoid, CpG ODN and a synthetic innate defense regulator peptide induces protective immunity against pertussis. Vaccine 29, 6540–6548. doi: 10.1016/j.vaccine.2011.07.009
Garlapati, S., Eng, N. F., Wilson, H. L., Buchanan, R., Mutwiri, G. K., Babiuk, L. A., et al. (2010). PCPP (poly[di(carboxylatophenoxy)-phosphazene]) microparticles co-encapsulating ovalbumin and CpG oligo-deoxynucleotides are potent enhancers of antigen specific Th1 immune responses in mice. Vaccine 28, 8306–8314. doi: 10.1016/j.vaccine.2010.09.080
Gilbert, P., Chiu, Y.-L., Allen, M., Lawrence, D., Chapdu, C., Israel, H., et al. (2003). Long-term safety analysis of preventive HIV-1 vaccines evaluated in AIDS vaccine evaluation group NIAID-sponsored Phase I and II clinical trials. Vaccine 21, 2933–2947. doi: 10.1016/S0264-410X(03)00158-0
Gracia, A., Polewicz, M., Halperin, S. A., Hancock, R. E., Potter, A. A., Babiuk, L. A., et al. (2011). Antibody responses in adult and neonatal BALB/c mice to immunization with novel Bordetella pertussis vaccine formulations. Vaccine 29, 1595–1604. doi: 10.1016/j.vaccine.2010.12.083
Greenwood, B. (2014). The contribution of vaccination to global health: past, present and future. Philos. Trans. Royal Soc. B 369, 20130433. doi: 10.1098/rstb.2013.0433
Gutjahr, A., Tiraby, G., Perouzel, E., Verrier, B., and Paul, S. (2016). Triggering intracellular receptors for vaccine adjuvantation. Trends Immunol. 37, 573–587. doi: 10.1016/j.it.2016.07.001
Hamonic, G., Pasternak, J. A., Ng, S. H., Fourie, K. R., Simko, O. M., Deluco, B., et al. (2020). Assessment of immunological response and impacts on fertility following intrauterine vaccination delivered to swine in an artificial insemination dose. Front. Immunol. 11:1015. doi: 10.3389/fimmu.2020.01015
He, P., Zou, Y., and Hu, Z. (2015). Advances in aluminum hydroxide-based adjuvant research and its mechanism. Hum. Vaccines Immunotherapeutics 11, 477–488. doi: 10.1080/21645515.2014.1004026
Htay, T., and Liu, M. W. (2005). Drug-eluting stent: a review and update. Vasc. Health Risk Manag. 1, 263–276. doi: 10.2147/vhrm.2005.1.4.263
Jin, W., and Dong, C. (2013). IL-17 cytokines in immunity and inflammation. Emerging Microbes Infect. 2, 1–5. doi: 10.1038/emi.2013.58
Jun, Y. J., Kim, J. I., Jun, M. J., and Sohn, Y. S. (2005). Selective tumor targeting by enhanced permeability and retention effect. Synthesis and antitumor activity of polyphosphazene-platinum (II) conjugates. J. Inorganic Biochem. 99, 1593–1601. doi: 10.1016/j.jinorgbio.2005.04.019
Kawano, F., Dootz, E. R., Koran, A. III., and Craig, R. G. (1994). Sorption and solubility of 12 soft denture liners. J. Prosthet. Dent. 72, 393–398. doi: 10.1016/0022-3913(94)90559-2
Kindrachuk, J., Jenssen, H., Elliott, M., Townsend, R., Nijnik, A., Lee, S. F., et al. (2009). A novel vaccine adjuvant comprised of a synthetic innate defence regulator peptide and CpG oligonucleotide links innate and adaptive immunity. Vaccine 27, 4662–4671. doi: 10.1016/j.vaccine.2009.05.094
Kovacs-Nolan, J., Latimer, L., Landi, A., Jenssen, H., Hancock, R., and Babiuk, L. (2009a). The novel adjuvant combination of CpG ODN, indolicidin and polyphosphazene induces potent antibody-and cell-mediated immune responses in mice. Vaccine 27, 2055–2064. doi: 10.1016/j.vaccine.2009.01.118
Kovacs-Nolan, J., Mapletoft, J., Latimer, L., and Babiuk, L. (2009b). CpG oligonucleotide, host defense peptide and polyphosphazene act synergistically, inducing long-lasting, balanced immune responses in cattle. Vaccine 27, 2048–2054. doi: 10.1016/j.vaccine.2009.01.117
Kovacs-Nolan, J., Mapletoft, J., Lawman, Z., and Babiuk, L. (2009c). Formulation of bovine respiratory syncytial virus fusion protein with CpG oligodeoxynucleotide, cationic host defence peptide and polyphosphazene enhances humoral and cellular responses and induces a protective type 1 immune response in mice. J. General Virol. 90, 1892–1905. doi: 10.1099/vir.0.011684-0
Lalor, S. J., and McLoughlin, R. M. (2016). Memory γδ T Cells–Newly Appreciated Protagonists in Infection and Immunity. Trends Immunol. 37, 690–702. doi: 10.1016/j.it.2016.07.006
Lee, S., and Nguyen, M. T. (2015). Recent advances of vaccine adjuvants for infectious diseases. Immune Netw. 15, 51–57. doi: 10.4110/in.2015.15.2.51
Levast, B., Awate, S., Babiuk, L., Mutwiri, G., Gerdts, V., and van Drunen Littel-van den Hurk, S. (2014). Vaccine potentiation by combination adjuvants. Vaccines 2, 297–322. doi: 10.3390/vaccines2020297
Lobanova, L. M., Eng, N. F., Satkunarajah, M., Mutwiri, G. K., Rini, J. M., Zakhartchouk, A., et al. (2012). The recombinant globular head domain of the measles virus hemagglutinin protein as a subunit vaccine against measles. Vaccine 30, 3061–3067. doi: 10.1016/j.vaccine.2012.02.067
Madan-Lala, R., Pradhan, P., and Roy, K. (2017). Combinatorial delivery of dual and triple TLR agonists via polymeric pathogen-like particles synergistically enhances innate and adaptive immune responses. Sci. Rep. 7:2530. doi: 10.1038/s41598-017-02804-y
Magiri, R., Lai, K., Chaffey, A., Zhou, Y., Pyo, H.-M., Gerdts, V., et al. (2018). Intradermal immunization with inactivated swine influenza virus and adjuvant polydi (sodium carboxylatoethylphenoxy) phosphazene (PCEP) induced humoral and cell-mediated immunity and reduced lung viral titres in pigs. Vaccine 36, 1606–1613. doi: 10.1016/j.vaccine.2018.02.026
Magiri, R., Lai, K., Huang, Y., Mutwiri, G., and Wilson, H. L. (2019). Innate immune response profiles in pigs injected with vaccine adjuvants polydi (sodium carboxylatoethylphenoxy) phosphazene (PCEP) and Emulsigen. Vet. Immunol. Immunopathol. 209, 7–16. doi: 10.1016/j.vetimm.2019.02.004
Magiri, R. B., Lai, K. J., Mutwiri, G. K., and Wilson, H. L. (2020). Experimental PCEP-adjuvanted swine influenza H1N1 vaccine induced strong immune responses but did not protect piglets against heterologous H3N2 virus challenge. Vaccines 8:235. doi: 10.3390/vaccines8020235
Mapletoft, J. W., Latimer, L., and Babiuk, L. A. (2010). Intranasal immunization of mice with a bovine respiratory syncytial virus vaccine induces superior immunity and protection compared to those by subcutaneous delivery or combinations of intranasal and subcutaneous prime-boost strategies. Clin. Vaccine Immunol. 17, 23–35. doi: 10.1128/CVI.00250-09
Mapletoft, J. W., Oumouna, M., Kovacs-Nolan, J., Latimer, L., Mutwiri, G., Babiuk, L., et al. (2008). Intranasal immunization of mice with a formalin-inactivated bovine respiratory syncytial virus vaccine co-formulated with CpG oligodeoxynucleotides and polyphosphazenes results in enhanced protection. J. General Virol. 89, 250–260. doi: 10.1099/vir.0.83300-0
McNeal, M. M., Rae, M. N., and Ward, R. L. (1999). Effects of different adjuvants on rotavirus antibody responses and protection in mice following intramuscular immunization with inactivated rotavirus. Vaccine 17, 1573–1580. doi: 10.1016/S0264-410X(98)00359-4
Mosca, F., Tritto, E., Muzzi, A., Monaci, E., Bagnoli, F., Iavarone, C., et al. (2008). Molecular and cellular signatures of human vaccine adjuvants. Proc. Natl. Acad. Sci. U.S.A. 105, 10501–10506. doi: 10.1073/pnas.0804699105
Mount, A., Koernig, S., Silva, A., Drane, D., Maraskovsky, E., Morelli, A., et al. (2013). Combination of adjuvants: the future of vaccine design. Expert Rev. Vaccines 12, 733–746. doi: 10.1586/14760584.2013.811185
Mutwiri, G., and Babiuk, L. A. (2009). 5 potential of polyphosphazenes in modulating vaccine-induced immune responses. Polyphosphazenes Biomed. Applications 77, 65–75. doi: 10.1002/9780470478882.ch5
Mutwiri, G., Benjamin, P., Soita, H., and Babiuk, L. A. (2008). Co-administration of polyphosphazenes with CpG oligodeoxynucleotides strongly enhances immune responses in mice immunized with Hepatitis B virus surface antigen. Vaccine 26, 2680–2688. doi: 10.1016/j.vaccine.2008.03.031
Mutwiri, G., Benjamin, P., Soita, H., Townsend, H., Yost, R., Roberts, B., et al. (2007). Poly[di(sodium carboxylatoethylphenoxy)phosphazene] (PCEP) is a potent enhancer of mixed Th1/Th2 immune responses in mice immunized with influenza virus antigens. Vaccine 25, 1204–1213. doi: 10.1016/j.vaccine.2006.10.011
Mutwiri, G., Gerdts, V., van Drunen Littel-van den Hurk, S., Auray, G., Eng, N., Garlapati, S., et al. (2011). Combination adjuvants: the next generation of adjuvants? Expert Rev. Vaccines 10, 95–107. doi: 10.1586/erv.10.154
Palmer, C. D., Ninković, J., Prokopowicz, Z. M., Mancuso, C. J., Marin, A., Andrianov, A. K., et al. (2014). The effect of stable macromolecular complexes of ionic polyphosphazene on HIV Gag antigen and on activation of human dendritic cells and presentation to T-cells. Biomaterials 35, 8876–8886. doi: 10.1016/j.biomaterials.2014.06.043
Park, M. R., Chun, C., Ahn, S. W., Ki, M. H., Cho, C. S., Song, S., et al. (2010). Sustained delivery of human growth hormone using a polyelectrolyte complex-loaded thermosensitive polyphosphazene hydrogel. J. Control. Release 147, 359–367. doi: 10.1016/j.jconrel.2010.07.126
Pasternak, J. A., Hamonic, G., Forsberg, N. M., Wheler, C. L., Dyck, M. K., Wilson, H., et al. (2017). Intrauterine delivery of subunit vaccines induces a systemic and mucosal immune response in rabbits. Am. J. Reproduc. Immunol. 78:e12732. doi: 10.1111/aji.12732
Pasternak, J. A., Ng, S. H., and Wilson, H. L. (2014). A single, low dose oral antigen exposure in newborn piglets primes mucosal immunity if administered with CpG oligodeoxynucleotides and polyphosphazene adjuvants. Vet. Immunol. Immunopathol. 161, 211–221. doi: 10.1016/j.vetimm.2014.08.006
Payne, L. G., Jenkins, S. A., Woods, A. L., Grund, E. M., Geribo, W. E., Loebelenz, J. R., et al. (1998). Poly [di (carboxylatophenoxy) phosphazene](PCPP) is a potent immunoadjuvant for an influenza vaccine. Vaccine 16, 92–98. doi: 10.1016/S0264-410X(97)00149-7
Radford, D. R., Sweet, S. P., Challacombe, S. J., and Walter, J. D. (1998). Adherence of Candida albicans to denture-base materials with different surface finishes. J. Dent. Res. 26, 577–583. doi: 10.1016/S0300-5712(97)00034-1
Reed, S. G., Bertholet, S., Coler, R. N., and Friede, M. (2009). New horizons in adjuvants for vaccine development. Trends Immunol. 30, 23–32. doi: 10.1016/j.it.2008.09.006
Reed, S. G., Orr, M. T., and Fox, C. B. (2013). Key roles of adjuvants in modern vaccines. Nat. Med. 19:1597. doi: 10.1038/nm.3409
Riese, P., Schulze, K., Ebensen, T., Prochnow, B., and Guzmán, C. A. (2013). Vaccine adjuvants: key tools for innovative vaccine design. Curr. Top. Med. Chem. 13, 2562–2580. doi: 10.2174/15680266113136660183
Sadat, S. M. A., Snider, M., Garg, R., Brownlie, R., and van Drunen Littel-van den Hurk, S. (2017). Local innate responses and protective immunity after intradermal immunization with bovine viral diarrhea virus E2 protein formulated with a combination adjuvant in cattle. Vaccine 35, 3466–3473. doi: 10.1016/j.vaccine.2017.05.029
Salvador, A., Igartua, M., Hernández, R. M., and Pedraz, J. L. (2012). Combination of immune stimulating adjuvants with poly (lactide-co-glycolide) microspheres enhances the immune response of vaccines. Vaccine 30, 589–596. doi: 10.1016/j.vaccine.2011.11.057
Sarkar, I., and Garg, R. (2016). Formulation of the respiratory syncytial virus fusion protein with a polymer-based combination adjuvant promotes transient and local innate immune responses and leads to improved adaptive immunity. Vaccine 34, 5114–5124. doi: 10.1016/j.vaccine.2016.08.053
Sasai, M., and Yamamoto, M. (2013). Pathogen recognition receptors: ligands and signaling pathways by Toll-like receptors. Int. Rev. Immunol. 32, 116–133. doi: 10.3109/08830185.2013.774391
Shim, D.-H., Ko, H.-J., Volker, G., Potter, A. A., Mutwiri, G., Babiuk, L. A., et al. (2010). Efficacy of poly [di (sodium carboxylatophenoxy) phosphazene](PCPP) as mucosal adjuvant to induce protective immunity against respiratory pathogens. Vaccine 28, 2311–2317. doi: 10.1016/j.vaccine.2009.12.069
Snider, M., Garg, R., Brownlie, R., and van den Hurk, V. N. (2014). The bovine viral diarrhea virus E2 protein formulated with a novel adjuvant induces strong, balanced immune responses and provides protection from viral challenge in cattle. Vaccine 32, 6758–6764. doi: 10.1016/j.vaccine.2014.10.010
Sobhani, A., Rafienia, M., Ahmadian, M., and Naimi-Jamal, M. R. (2017). Fabrication and characterization of polyphosphazene/calcium phosphate scaffolds containing chitosan microspheres for sustained release of bone morphogenetic protein 2 in bone tissue engineering. J. Tissue Eng. Regen. Med. 14, 525–538. doi: 10.1007/s13770-017-0056-z
Taghavi, A., Allan, B., Mutwiri, G., Foldvari, M., Kessel, A. V., Willson, P., et al. (2009). Enhancement of immunoprotective effect of CpG-ODN by formulation with polyphosphazenes against E. coli septicemia in neonatal chickens. Current Drug Delivery 6, 76–82. doi: 10.2174/156720109787048221
Tandrup Schmidt, S., Foged, C., Smith Korsholm, K., Rades, T., and Christensen, D. (2016). Liposome-based adjuvants for subunit vaccines: formulation strategies for subunit antigens and immunostimulators. Pharmaceutics 8:7. doi: 10.3390/pharmaceutics8010007
Teasdale, I., and Brüggemann, O. (2013). Polyphosphazenes: multifunctional, biodegradable vehicles for drug and gene delivery. Polymers 5, 161–187. doi: 10.3390/polym5010161
Wasan, E. K., Syeda, J., Strom, S., Cawthray, J., Hancock, R. E., Wasan, K. M., et al. (2019). A lipidic delivery system of a triple vaccine adjuvant enhances mucosal immunity following nasal administration in mice. Vaccine 37. doi: 10.1016/j.vaccine.2019.01.058
Weiner, G. J., Liu, H.-M., Wooldridge, J. E., Dahle, C. E., and Krieg, A. M. (1997). Immunostimulatory oligodeoxynucleotides containing the CpG motif are effective as immune adjuvants in tumor antigen immunization. Proc. Natl. Acad. Sci. U.S.A. 94, 10833–10837. doi: 10.1073/pnas.94.20.10833
Wilson, H. L., Kovacs-Nolan, J., Latimer, L., Buchanan, R., Gomis, S., and Babiuk, L. (2010). A novel triple adjuvant formulation promotes strong, Th1-biased immune responses and significant antigen retention at the site of injection. Vaccine 28, 8288–8299. doi: 10.1016/j.vaccine.2010.10.006
Keywords: adjuvants, polyphosphazenes, vaccines, immunity, animals, viruses, bacteria
Citation: Chand DJ, Magiri RB, Wilson HL and Mutwiri GK (2021) Polyphosphazenes as Adjuvants for Animal Vaccines and Other Medical Applications. Front. Bioeng. Biotechnol. 9:625482. doi: 10.3389/fbioe.2021.625482
Received: 03 November 2020; Accepted: 22 January 2021;
Published: 04 March 2021.
Edited by:
Changyou Gao, Zhejiang University, ChinaReviewed by:
Lulu Han, Dalian University of Technology, ChinaOlga Kammona, Centre for Research and Technology Hellas (CERTH), Greece
Copyright © 2021 Chand, Magiri, Wilson and Mutwiri. This is an open-access article distributed under the terms of the Creative Commons Attribution License (CC BY). The use, distribution or reproduction in other forums is permitted, provided the original author(s) and the copyright owner(s) are credited and that the original publication in this journal is cited, in accordance with accepted academic practice. No use, distribution or reproduction is permitted which does not comply with these terms.
*Correspondence: George K. Mutwiri, Z2VvcmdlLm11dHdpcmlAdXNhc2suY2E=