- 1Department of Biomedical Engineering, The Chinese University of Hong Kong, Shatin, Hong Kong
- 2Institute for Tissue Engineering & Regenerative Medicine, The Chinese University of Hong Kong, Shatin, Hong Kong
- 3School of Biomedical Sciences, Faculty of Medicine, The Chinese University of Hong Kong, Shatin, Hong Kong
- 4Department of Orthopaedics & Traumatology, Faculty of Medicine, The Chinese University of Hong Kong, Shatin, Hong Kong
The synthesis of Metal-organic Frameworks (MOFs) and their evaluation for various applications is one of the largest research areas within materials sciences and chemistry. Here, the use of MOFs in biomaterials and implants is summarized as narrative review addressing primarely the Tissue Engineering and Regenerative Medicine (TERM) community. Focus is given on MOFs as bioactive component to aid tissue engineering and to augment clinically established or future therapies in regenerative medicine. A summary of synthesis methods suitable for TERM laboratories and key properties of MOFs relevant to biomaterials is provided. The use of MOFs is categorized according to their targeted organ (bone, cardio-vascular, skin and nervous tissue) and whether the MOFs are used as intrinsically bioactive material or as drug delivery vehicle. Further distinction between in vitro and in vivo studies provides a clear assessment of literature on the current progress of MOF based biomaterials. Although the present review is narrative in nature, systematic literature analysis has been performed, allowing a concise overview of this emerging research direction till the point of writing. While a number of excellent studies have been published, future studies will need to clearly highlight the safety and added value of MOFs compared to established materials for clinical TERM applications. The scope of the present review is clearly delimited from the general ‘biomedical application’ of MOFs that focuses mainly on drug delivery or diagnostic applications not involving aspects of tissue healing or better implant integration.
Introduction
MOFs are new synthetic materials that emerged over the past three decades (Yaghi and Li, 1995; Yaghi et al., 1995). MOFs comprise of organic ligands that bridge metal ions. This leads to highly ordered, porous and 3-dimensional crystalline structures. The pore size and aperture is significantly smaller compared to polymeric structures (Pan et al., 2013) and can be accurately controlled (Eddaoudi et al., 2002; Banerjee et al., 2009). This accurate control makes MOFs predestined for drug loading and sustained or triggered release from nano-particulate drug formulations or coatings (Wang et al., 2020). This has led to the classical understanding of “biomedical MOF applications” that primarily focused on diagnostic applications (Chapartegui-Arias et al., 2019) or drug delivery (Keskin and Kizilel, 2011; Banerjee et al., 2020). The present review is clearly delimited from these established fields of investigation by focusing on MOFs for TERM applications. In particular, the present review addresses MOFs that modulate the foreign body response to implants, MOFs that influence the inflammatory wound environment, or MOFs that improve the host tissue-implant interface and aid healing.
A systematic literature analysis was performed using Clarivate Analytics Web of Science database in combination with a structured key word search. In brief, a comprehensive list of works on MOFs was generated and those interfacing with biomaterials, tissue engineering, regenerative medicine or MOFs interfacing with neuronal repair were identified. Works solely focusing on drug-delivery or cancer treatment were excluded if not reporting aspect of tissue healing, integration or improvement of the foreign body response.
In addition to the drug loading capacity, the present review aims to highlight that MOFs may also have intrinsic bioactivity through delivering specific metal ions (Su et al., 2019), ligands (Rojas et al., 2017) or through catalytically generating messenger molecules such as nitric oxide in copper-based MOFs (Harding and Reynolds, 2012). Beyond individual components, structural aspects of MOFs are essential since they determine release kinetics as well as the accessibility of the metal centers providing catalytic effects. Most studies investigating MOFs for TERM application focused on orthopedic applications, followed by cardiovascular treatment, cutaneous wound healing, and applications in nervous tissue (Figure 1). Beyond these targeted organs, a specialized MOF was proposed for treatment of periodontitis (Li et al., 2019), while materials for other organs seem not yet to have been evaluated. In summary, the use of MOFs for TERM applications is an emerging field of research with only very few works published before the year 2018.
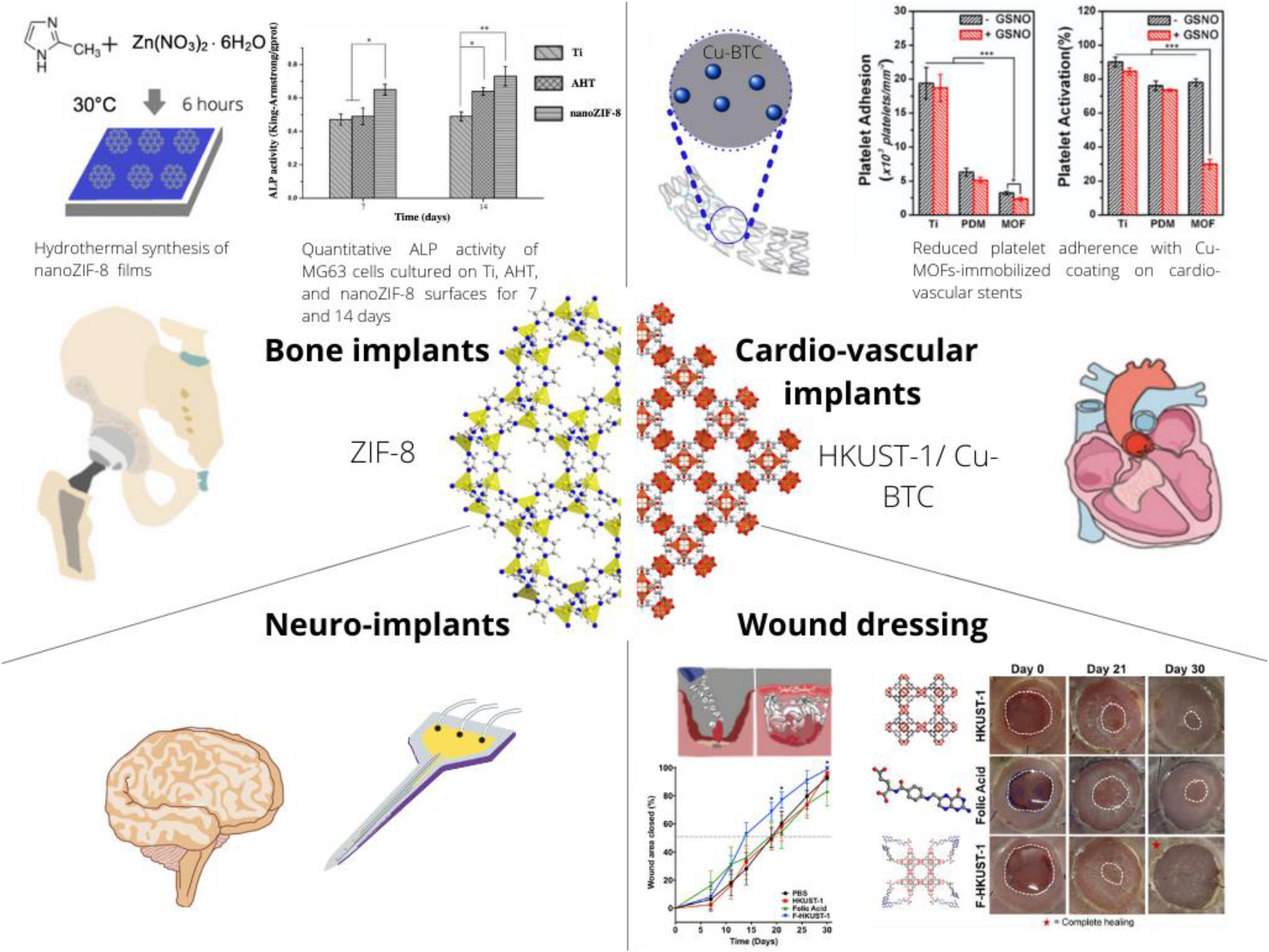
Figure 1. Schematic on the present and envisioned use of MOFs as a component of implants or biomaterial compositions with applications in Tissue Engineering and Regenerative Medicine (TERM). The crystal structure of the prototypical MOF Zeolitic Imidazolate Framework 8 (ZIF-8) is depicted along the [111] crystallographic direction (left side) and HKUST-1 also known as Cu-BTC is depicted along the [001] crystallographic direction. These two different structures have been most frequently applied to TERM research and represent the diverse class of MOFs. In particular, simple exposure of osteoblast like cells to ZIF-8 increases ALP activity (Chen et al., 2017a), Cu-BTC can decrease adherence and activation of platelets (Fan et al., 2019) and also accelerates skin wound closure (Xiao et al., 2018).
Parallels of MOFs to Natural Minerals in TERM Applications
The concept of MOFs originates from natural minerals, which have a substantial track record in TERM applications. Calcium phosphate has intrinsic osteogenic effect (Albee, 1920) and its admixtures to biodegradable polymers led to clinical implants (de Groot, 1993). A calcium and strontium based MOF with bisphosphonate linker (Matlinska et al., 2019) appears to be the evolution of minerals with engineered bioactivity.
Another naturally occurring mineral is zinc oxide (ZnO), a non-porous solid devoid of any drug loading capacity, that has long been used to treat cutaneous wounds and pathological skin conditions (Lansdown et al., 2007). In contrast to ZnO, versatile drug loading, release and disintegration rate of porous Zeolitic Imidazolate Frameworks (ZIFs) can be designed leading to various envisioned biomedical applications (Maleki et al., 2020). The most prominent member of the ZIF family is ZIF-8, which is a polymorph of zinc(II)2-methylimidazolate and has a ligand-metal-ligand bonding angle similar to that of O-Si-O. In addition, ZIF-8 shares the crystal topology (SOD) with Sodalite, a naturally occurring zeolite of the sodium aluminum silicate framework family.
Preparation Methods of MOFs Suitable for Term Laboratories and Their Key Properties
Solution Precipitation Synthesis of ZIF-8 and Loading of Bioactive Agents
ZIF-8 is easily synthesized through simple precipitation reactions facilitated by mixing the ligand (2-methylimidazole) and zinc salt solution at room temperature (Park et al., 2006; Beyer et al., 2016, 2018b; Kulow et al., 2019). ZIF-8 can be engineered to acquire bioactivity beyond that of zinc ions through encapsulation of drugs. Admixtures of Dexamethasone (Sarkar et al., 2019) or non-steroidal anti-inflammatory drugs (Ho et al., 2020) to these precursor mixtures during precipitation reactions led to their firm entrapment within ZIF-8. ZIF-8 shows a sustained release behavior for small molecules up to several weeks (Liédana et al., 2012). An interesting aspect of ZIF-8 is the observation that it crystallizes around biomacromolecules in aqueous solution by a process termed biomimetic mineralization (Liang et al., 2015a,b; Gao et al., 2019). This approach has been used for delivery of insulin (Duan et al., 2018) and may provide opportunities for e.g., growth factor delivery.
Mechanochemical Synthesis of MOFs and Potential Use as Filler in Polymer Composites
MOFs may also be synthesized mechanochemically (Stolar and Užareviæ, 2020) by mixing organic linkers and metal precursors in a small planetary ball-mill (Akhmetova et al., 2019), immediately yielding MOF powder in large quantities and short time. So far, the mechanochemical synthesis of calcium and strontium MOFs has been reported (Al-Terkawi et al., 2017), which is an excellent route to produce MOFs as bioactive fillers for e.g., polymeric biomaterials. However, mechanochemical syntheses routes for earth alkaline metal MOFs specifically for TERM applications have yet to be developed.
Solvothermal Synthesis of CU-BTC
During solvo-thermal synthesis, two precursor solutions of organic ligands and metal ions are mixed and heated in a micro-autoclave beyond the boiling point of the solvent (Ni and Masel, 2006). Copper(II)1,3,5-benzenetricarboxylate (Cu-BTC), also known as HKUST-1 (Chui, 1999) is typically synthesized under solvo-thermal conditions. The porous structure of Cu-BTC makes the copper metal center accessible to solutes. This renders Cu-BTC an interesting catalyst for the conversion of blood borne nitrosothiols to continuously release nitric oxide (NO) (Harding and Reynolds, 2012). Nitric oxide is a key messenger molecule during inflammation (Sharma et al., 2007), platelet adhesion (Radomski et al., 1987), ECM deposition (Myers and Tanner, 1998), angiogenesis and other aspects of tissue healing and foreign body response (Schäffer et al., 1996). Beyond the intrinsic catalytic activity, constant release of copper ions is crucial for the biological activity of Cu-BTC. Copper ions are known to stimulate angiogenesis (Sen et al., 2002) and to increase extracellular matrix (ECM) deposition (Gérard et al., 2010). These properties together hold potential to benefit TERM applications of copper-based MOFs when correctly dosed for the intended application.
Electrochemical MOF Coating With Potential Use in Sensors or Electrodes
Electro-deposition of MOFs (Campagnol et al., 2016) may be useful for coating of conductive, implantable electrodes and sensors especially with future applications in neuro-interface engineering.
Applications of Mofs or Mof Based Biomaterials in Term
MOFs for Orthopedic Implants
Most reports on use of MOFs in TERM applications focus on the improvement of structural bone implants. Indeed, a small but significant fraction of clinical implants fail due to an unfavorable, non-healing wound environment that may be aggravated by bacterial infection (Inacio et al., 2013).
Intrinsic Bioactivity of MOFs and Influence on Bone Tissue Biology
In vitrostudies showed significantly improved corrosion resistance of magnesium alloys upon coating with bio-MOF-1, comprising of zinc ions and adenine as well as interconnections of 4,4-biphenyldicarboxylate linkers (Liu et al., 2019). Besides engineering corrosion resistance for better structural integrity, the ability to prevent bacterial infection is crucial for successful integration of bone implants. Sr-HA (Sr-substituted hydroxyapatite) with MOF74 has demonstrated excellent abilities to inhibit S. Aureus and E. Coli bacteria and simultaneously induced apoptosis in Saos-2 cancer cells while promoting proliferation and maturation of primary osteoblasts (Zhang et al., 2019).
The effect of different metal ions on bone mineralization and osteogenesis is long known and mechanistic insights have been obtained e.g., for zinc (Kwun et al., 2010) or magnesium (Zhang et al., 2016). Consequently, MOFs were shown to promote osteogenic differentiation in vitro, an indicator for a better osteointegration of bone implants. ZIF-8 coatings on titanium implants enhanced ALP activity, better extracellular matrix mineralization and upregulation of osteogenic gene expression in MG63 cells, while suppressing Streptococcus Mutans proliferation (Chen et al., 2017a). Beyond zinc and magnesium, Ca-Sr MOFs could upregulate the expression of ALP, whereas simultaneous release of Ca and Sr provided osteo-inductive signals (Joseph et al., 2019). Further, simultaneous delivery of calcium and strontium ions as well as bisphosphonate linkers was noted to allow better bone mineralization (Matlinska et al., 2019). In the context of regenerative medicine, Mg/HCOOH-MOF was investigated for the treatment of osteoarthritis. This MOF was shown to up-regulate the expression of OCN, Axin2 and down-regulate iNOS and IL-1β, indicating a potentially favorable effect to ease osteoarthritis (Li et al., 2020).
In vivo investigations were less frequently reported, indicating that the field of MOFs for orthopedic applications is just at the beginning of being explored. Mg/Zn-MOF74, was demonstrated to have low toxicity, intrinsic anti-inflammatory and antibacterial features, while improving early osteogenic promotion and angiogenesis in vivo (Zhu et al., 2020).
Acquired Bioactivity of MOFs Through Drug Loading for Enhanced Bone Regeneration
Acquired bioactivity refers to any additional biological effect that does not originate from the MOF itself, which can be acquired through encapsulation of drugs, ions or other bioactive agents. MOF-based drug carriers are interesting for orthopedic implant coatings due to their high drug encapsulation capacity with a negligible premature release (Tan et al., 2016).
In vitro studies showed that Poly-l-lactic acid (PLLA) scaffolds coated with copper loaded ZIF-8 promoted osteogenic differentiation as compared to pure PLLA scaffolds while preventing bacterial infection in the presence of Zn2+ and Cu2+ ions (Telgerd et al., 2019).
Beyond the release of ions, MOFs have unique sustained release abilities for small molecular weight drugs. A sustained release of dexamethasone for 4 weeks was achieved through integrating dexamethasone loaded ZIF-8 into cellulose-hydroxyapatite nanocomposites envisioned for load bearing orthopedic applications (Sarkar et al., 2019). A similar approach has led to silk fibroin-dexamethasone@zeolitic imidazolate framework-8-titanium (SF-DEX@ZIF-8-Ti) composite materials. MC3T3-E1 osteoblast precursor cells cultured on the SF-DEX@ZIF-8-Ti showed enhanced osteo-differentiation (Ran et al., 2018). Hence, current research indicates a good potential of MOFs to facilitate osteointegration of bone implants, thereby improving their clinical outcome. Examples for treating bone diseases include the release of naringin, which improves osseointegration and prevents bacterial infection (Yu et al., 2017), delivery of antitubercular drug for treatment of osteoarticular tuberculosis (Pei et al., 2018) and release of vancomycin for treatment of osteomyelitis (Karakeçili et al., 2019). The versatility of MOF-based drug carriers principally allow the design of elaborate drug release mechanisms tailored to specific triggers. However, only one study describes pH and Ca2+ ion dependent release of drugs into the bone environment using a Zr-MOFs capped with CP5-based pseudorotaxanes (Tan et al., 2016).
In vivostudies are less frequently reported. One study investigated implant materials modified with levofloxacin loaded ZIF-8 particles, that resulted in better attachment, proliferation, and differentiation of osteoblasts in vitro. Special focus was given on the pH-triggered release in slightly acidic environments resulting from inflammation and bacterial infection. The effectiveness of this implant material was confirmed in terms of osseointegration and antibacterial properties in a rat infected femur model (Tao et al., 2020).
In conclusion, MOFs have been shown to be promising to enhance bone healing through intrinsic and/or acquired properties (Figure 2). While the here presented initial studies provide excellent perspectives for the use of MOFs for bone healing, more studies are needed toward their clinical applicability.
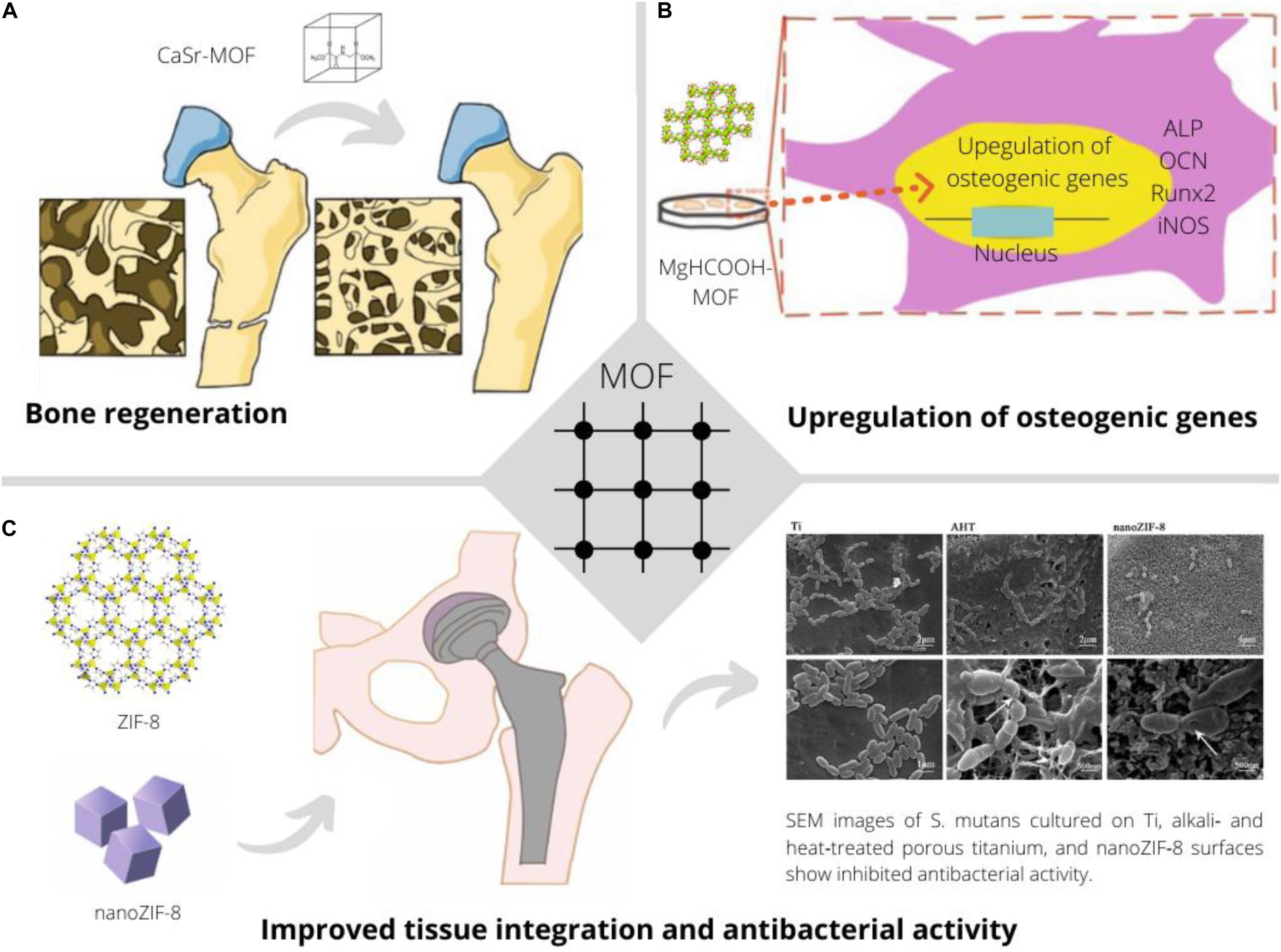
Figure 2. Application of MOFs to improve bone implants through continuous release of earth alkaline ions promoting bone regeneration and biomineralization (Joseph et al., 2019) (A), facilitating osteo-induction and -integration through favorable upregulation of relevant genes in presence of specific MOFs (Li et al., 2020) (B) and providing improved tissue integration and an anti-bacterial effect (Chen et al., 2017a) (C).
MOFs for Cardio-Vascular Implants
Approximately 4% of the population in industrialized societies suffer from coronary artery disease (CAD) requiring intervention (Sanchis-Gomar et al., 2016). Traditionally, anti-thrombotic medication and small molecular weight nitric oxide donors acting as vasodilators are administrated to prevent artery occlusion. In more severe cases, drug-eluting stents are implanted into the artery to prevent occlusion permanently. Copper-based MOFs recently emerged as component in anti-thrombotic coatings for cardio-vascular implants.
In vitro: Cu-BTC was established to be an efficient catalyst for the conversion of blood borne s-nitroso-cysteine to nitric oxide and cysteine (Harding and Reynolds, 2012). More complex Cu-MOFs have subsequently been investigated for catalytic conversion of s-ntirosoglutathione (Neufeld et al., 2017b). This has led to works on MOF/polymer composite materials for nitric oxide release as suitable leads for novel implant materials (Harding and Reynolds, 2014; Neufeld et al., 2015, 2016, 2017a; Zang et al., 2020). In addition to these initial studies, Cu-BTC was directly grown on stent surfaces as SURMOF and investigated in vitro showing favorable hemocompatibility (Zhao et al., 2019).
Evaluating non-copper based MOFs for cardiovascular implants, a MIL-101 (Fe) poly(ε−caprolactone) composite was investigated as material for metal free stents, using the MOF as mechanical re-enforcement, sustained drug release vehicle and Magnetic Resonance Imaging (MRI) contrast agent (Hamideh et al., 2020). Further, few studies exist that address the interaction of MOFs with cardiomyocytes (Al-Ansari et al., 2020).
In vivo, Cu-BTC was shown effective as cardiovascular stent surface modification through coating together with polydopamine (Fan et al., 2019). This significantly reduced protein absorption, thrombus formation, platelet adhesion and an increased vasodilating effect, especially upon systemic injection of nitric oxide donors requiring catalytic degradation. Using a 5-(1H-tetrazol-5-yl)isophthalic acid based MOF with Ni2+ and K+ ions in another study prevented arrhythmia through reducing the sympathetic excitability in a rat model (Ding et al., 2020). Future studies will need to compare the effectiveness and safety of MOFs with that of clinically used materials.
MOF-Based Biomaterials for Cutaneous Wound Care
The clinical healing outcome of chronic wounds arising from pressure ulcers or injuries of diabetic patients is often unsatisfactory, which motivates research for better cutaneous wound care material. In addition, achieving fast hemostasis and antibacterial properties is important for emergency care equipment and the potential role of MOFs for these two applications is elucidated in the following.
In vitro studies investigated polymer meshes with enclosed Cu-BTC (Zhang et al., 2020) and cellulose based biomaterials with the ability to release nitric oxide (Darder et al., 2020) as wound dressing. To ascribe increased mitochondrial respiration solely to nitric oxide, a titanium-based MOF devoid of intrinsic bioactivity and with controlled release of pre-loaded nitric oxide was proposed to be a copper free alternative (Pinto et al., 2020).
In vivostudies have shown the positive effect of Cu-BTC on skin healing using full-thickness splinted skin wounds in rodents. Dosage forms included folate stabilized Cu-BTC crystal suspensions (Xiao et al., 2018) and hydrogel compositions (Xiao et al., 2017).
Supplementation of zinc ions from ZIF-8 has shown to be similarly effective in a ZIF-8 hydrogel composite membrane (Yao et al., 2020) for wound healing. Combination of zinc and copper ions in MOFs that further encapsulated bioactive molecules promised to be most effective. Along this line of thought, the encapsulation of niacin loaded copper-/zinc MOFs within alginate shells has shown antibacterial, antioxidant and angiogenic properties, which resulted in significant improvement of wound closure in an infected full-thickness skin defect model (Chen et al., 2019).
Future studies are necessary to ascribe these effects to the synergistic potential of MOFs and not to the presence of its individual components upon dissolution.
MOFs for Neuronal Tissue Engineering and Implants
Traumatic injuries to the nervous system result in dysfunctional regeneration and glial scaring in an inflammatory process leading to loss of nervous tissue. The consequences include physical paralysis of muscles, neurosensory or mental impairment. This has motivated the development of biomaterials for nervous tissue regeneration (Liu and Hsu, 2020), and MOFs may be suitable to further augment positive materials characteristic toward this goal.
In vitro studies focused on assessing the response of sensory neurons to nano-particulate MOFs by employing a neurite outgrowth assay, indicating the potential of a material to promote axonal outgrowth, thus nervous regeneration (Wuttke et al., 2017). The MOFs investigated included iron and chromium salts of trimesic acid (MIL-100) and Zirconium fumarate MOFs. These MOFs did not show reduction in neurite outgrowth at a concentration of 100 μg/ml, indicating their suitability for drug delivery applications. In line with the thought that MOFs are compatible with neuronal biology, treatment of neuronal stem cells with a cobalt MOF having 3,3’,5,5’ -azobenzenetetracarboxylic acid as linker was envisioned to benefit therapies for spinal cord injury. This was due to molecular docking studies revealing potential binding of the MOF with the trkA receptor, which led to activation of PI3K/Akt signaling and enhanced nerve growth factor (NGF) production in vitro. NGF is responsible for neuron growth and survival (Cao et al., 2020).
MOF based drug delivery vehicles include cationic MOF-74-Fe(III) with low cytotoxicity on PC12 neuron cells and high drug loading due to electrostatic accumulation (Hu et al., 2014) of ibuprofen, which is known to reduce neuroinflammation (Wixey et al., 2019) and is passively released from the MOF. An example for actively triggered dopamine release by magnetophoretic means was demonstrated using MIL-88A (iron(III) fumarate) encapsulated iron oxide particles (Pinna et al., 2018). A drug delivery mechanism that is triggered upon synaptic transmission can be achieved using adenosine triphosphate (ATP) responsive release of drugs. Here, a MOF based on Zr4+ ions and amino-triphenyldicarboxylic acid was capped with aptamers specific for ATP, leading to an un-locking effect upon binding and drug release (Chen et al., 2017b). These three examples of passive-, triggered- and targeted drug release show the versatility of MOF based drug delivery for neurobiological applications. Systemic injection requires MOF particles to cross the blood-brain barrier. This ability was assessed for e.g., ZIF-8 particles through crossing an hCMEC/D3 brain endothelial cell membrane (Chiacchia et al., 2015). Future studies in this direction would benefit from microfluidic vascular transport models for nanoparticles (Ho et al., 2017) involving pericytes to better emulate the blood-brain barrier and to study the biological response of further cell types in inflammation and healing (Beyer et al., 2018a; Blocki et al., 2018).
In vivostudies reported the use of a Cu(II) MOF in a mixed linker approach with 5-methylisophthalic acid and 1,3-bis(5,6-dimethylbenzimidazol-1-yl)propane (Tian and Tian, 2020). This MOF inhibited dopaminergic neurons, decreased expression of dopamine reporters, decreased dopamine release, reduced apoptosis and increased Wnt1-Nkx2.2 activation. The MOF was presumably injected on a systemic level into LgDel ± mice and biological effects were observed in a dose dependent manner up to 5 mg/kg body weight. No comparison of the biological effect upon injection of the MOF or a solution containing only the ligand was made.
A very inspiring study used MIL-100 (Fe) as a drug carrier for siSOX9 and retinoic acid in combination with co-encapsulated ceria based nanozymes to reduce oxidative stress (Yu et al., 2020). This complex drug formulation was incubated with neuronal stem cells (NSCs) isolated from newborn mice prior to NSC transplantation at sites of lesions. The co-release of soSOX9 and retinoic acid promoted neuro-differentiation and reduced oxidative stress prevented newborn neurons from apoptosis. The studies were carried out with 11-month old APPswe/PS1M146V/TauP301L triple transgenic mice, a model for Alzheimer’s disease. The positive effect of the MOF based drug combination was shown through cognitive improvements in a Morris Water Maze test and histopathological improvements.
These inspiring in vitro and in vivo works have highlighted a clear potential of MOFs to treat neurological disorders. Very few macroscopic biomaterials have been developed along this thought and future studies should include evaluating the opportunity to improve implant-nervous tissue interfaces with these materials.
Conclusion and Outlook
The presented in vitro and in vivo data are impressive in terms of the strong biological response that MOFs elicit toward a positive healing outcome in various tissues and applications. However, more efforts are required to understand the basic dose dependent response of individual cell types and tissues to different MOFs. In addition, concerns about the safety of some organic linkers of MOFs need to be eliminated or alternative linkers considered save for clinical applications must be shown effective in yielding MOFs with similar properties. This is essential before MOF based biomaterials for TERM applications will commence improving healing outcomes in the treatment of human patients.
Author Contributions
MS reviewed literature related to orthopedic implants and cutaneous wound care and designed the figures. JR reviewed literature related to neuronal TERM applications. XL reviewed literature related to nitric oxide producing biomaterials. JM reviewed literature related to MOF & polymer composite materials. AB and SB wrote the manuscript. SB guided the review process. All authors contributed to the article and approved the submitted version.
Funding
This work was supported by grant 4055120 to SB through Faculty of Engineering of The Chinese University of Hong Kong (CUHK). SB appreciates the research budget provided by the Department of Biomedical Engineering of CUHK.
Conflict of Interest
The authors declare that the research was conducted in the absence of any commercial or financial relationships that could be construed as a potential conflict of interest.
References
Akhmetova, I., Beyer, S., Schutjajew, K., Tichter, T., Wilke, M., Prinz, C., et al. (2019). Cadmium benzylphosphonates – the close relationship between structure and properties. Cryst. Eng. Comm. 21, 5958–5964. doi: 10.1039/C9CE00776H
Al-Ansari, D. E., Mohamed, N. A., Marei, I., Zekri, A., Kameno, Y., Davies, R. P., et al. (2020). Internalization of metal–organic framework nanoparticles in human vascular cells: implications for cardiovascular disease therapy. Nanomaterials 10:1028. doi: 10.3390/nano10061028
Albee, F. H. (1920). Studies in bone growth triple calcium phosphate as a stimulus to osteogenesis. Ann. Surg. 71, 32–39.
Al-Terkawi, A.-A., Scholz, G., Buzanich, A. G., Reinsch, S., Emmerling, F., and Kemnitz, E. (2017). Ca- and Sr-tetrafluoroisophthalates: mechanochemical synthesis, characterization, and ab initio structure determination. Dalt. Trans. 46, 6003–6012. doi: 10.1039/C7DT00734E
Banerjee, R., Furukawa, H., Britt, D., Knobler, C., O’Keeffe, M., and Yaghi, O. M. (2009). Control of pore size and functionality in isoreticular zeolitic imidazolate frameworks and their carbon dioxide selective capture properties. J. Am. Chem. Soc. 131, 3875–3877. doi: 10.1021/ja809459e
Banerjee, S., Lollar, C. T., Xiao, Z., Fang, Y., and Zhou, H. C. (2020). Biomedical integration of metal–organic frameworks. Trends Chem. 2, 467–479. doi: 10.1016/j.trechm.2020.01.007
Campagnol, N., Van Assche, T. R. C., Li, M., Stappers, L., Dinca, M., Denayer, J. F. M., et al. (2016). On the electrochemical deposition of metal-organic frameworks. J. Mater. Chem. A 4, 3914–3925. doi: 10.1039/c5ta10782b
Beyer, S., Koch, M., Lee, Y., Jung, F., and Blocki, A. (2018a). An in vitro model of angiogenesis during wound healing provides insights into the complex role of cells and factors in the inflammatory and proliferation phase. Int. J. Mol. Sci. 19:2913. doi: 10.3390/ijms19102913
Beyer, S., Schürmann, R., Feldmann, I., Blocki, A., Bald, I., Schneider, R. J., et al. (2018b). Maintaining stable Zeolitic Imidazolate Framework (ZIF) templates during polyelectrolyte multilayer coating. Colloids Interface Sci. Commun. 22, 14–17. doi: 10.1016/j.colcom.2017.11.004
Beyer, S., Prinz, C., Schürmann, R., Feldmann, I., Zimathies, A., Blocki, A. M., et al. (2016). Ultra-Sonication of ZIF-67 crystals results in ZIF-67 nano-flakes. ChemistrySelect 1, 5905–5908. doi: 10.1002/slct.201601513
Blocki, A., Beyer, S., Jung, F., and Raghunath, M. (2018). The controversial origin of pericytes during angiogenesis - implications for cell-based therapeutic angiogenesis and cell-based therapies. Clin. Hemorheol. Microcirc. 69, 215–232. doi: 10.3233/CH-189132
Cao, C., Li, Z. Z., Zhang, Z. Q., and Chen, X. H. (2020). A new porous cationic metal–organic framework: selective sorption of organic dyes and treatment on neural stem cells during spinal cord injury treatment. J. Iran. Chem. Soc. 17, 2933–2942. doi: 10.1007/s13738-020-01969-1960
Chapartegui-Arias, A., Villajos, J. A., Myxa, A., Beyer, S., Falkenhagen, J., Schneider, R. J., et al. (2019). Covalently fluorophore-functionalized ZIF-8 colloidal particles as a sensing platform for endocrine-disrupting chemicals such as phthalates plasticizers. ACS Omega 4, 17090–17097. doi: 10.1021/acsomega.9b01051
Chen, G., Yu, Y., Wu, X., Wang, G., Gu, G., Wang, F., et al. (2019). Microfluidic electrospray niacin metal-organic frameworks encapsulated microcapsules for wound healing. Research 2019:6175398. doi: 10.34133/2019/6175398
Chen, J., Zhang, X., Huang, C., Cai, H., Hu, S., Wan, Q., et al. (2017a). Osteogenic activity and antibacterial effect of porous titanium modified with metal-organic framework films. J. Biomed. Mater. Res. - Part A. 105, 834–846. doi: 10.1002/jbm.a.35960
Chen, W.-H., Yu, X., Liao, W.-C., Sohn, Y. S., Cecconello, A., Kozell, A., et al. (2017b). ATP-Responsive aptamer-based metal-organic framework nanoparticles (NMOFs) for the controlled release of loads and drugs. Adv. Funct. Mater. 27:1702102. doi: 10.1002/adfm.201702102
Chiacchia, M., Cerutti, C., Gromnicova, R., Rietdorf, K., Romero, I. A., and Bradshaw, D. (2015). Zinc-imidazolate polymers (ZIPs) as a potential carrier to brain capillary endothelial cells. J. Mater. Chem. B 3, 9053–9059. doi: 10.1039/C5TB01814E
Chui, S. S. Y. (1999). A chemically functionalizable nanoporous material [Cu3(TMA)2(H2O)3]n. Science 283, 1148–1150. doi: 10.1126/science.283.5405.1148
Darder, M., Karan, A., Real, G., del, and DeCoster, M. A. (2020). Cellulose-based biomaterials integrated with copper-cystine hybrid structures as catalysts for nitric oxide generation. Mater. Sci. Eng. C 108:110369. doi: 10.1016/j.msec.2019.110369
de Groot, K. (1993). Clinical applications of calcium phosphate biomaterials: a review. Ceram. Int. 19, 363–366. doi: 10.1016/0272-8842(93)90050-90052
Ding, C., Zhang, W., Xu, Z., Zhang, H., and Zheng, J. (2020). A new heterometallic MOF with 1D nano-sized channels for cyanosilylation reaction and prevention effect against arrhythmia by reducing the sympathetic excitability. J. Exp. Nanosci. 15, 87–96. doi: 10.1080/17458080.2020.1729356
Duan, Y., Ye, F., Huang, Y., Qin, Y., He, C., and Zhao, S. (2018). One-pot synthesis of a metal–organic framework-based drug carrier for intelligent glucose-responsive insulin delivery. Chem. Commun. 54, 5377–5380. doi: 10.1039/C8CC02708K
Eddaoudi, M., Kim, J., Rosi, N., Vodak, D., Wachter, J., O’Keeffe, M., et al. (2002). Systematic design of pore size and functionality in isoreticular MOFs and their application in methane storage. Science 295, 469–472. doi: 10.1126/science.1067208
Fan, Y., Zhang, Y., Zhao, Q., Xie, Y., Luo, R., Yang, P., et al. (2019). Immobilization of nano Cu-MOFs with polydopamine coating for adaptable gasotransmitter generation and copper ion delivery on cardiovascular stents. Biomaterials 204, 36–45. doi: 10.1016/j.biomaterials.2019.03.007
Gao, S., Hou, J., Deng, Z., Wang, T., Beyer, S., Buzanich, A. G., et al. (2019). Improving the acidic stability of zeolitic imidazolate frameworks by biofunctional molecules. Chemistry 5, 1597–1608. doi: 10.1016/j.chempr.2019.03.025
Gérard, C., Bordeleau, L. J., Barralet, J., and Doillon, C. J. (2010). The stimulation of angiogenesis and collagen deposition by copper. Biomaterials 31, 824–831. doi: 10.1016/j.biomaterials.2009.10.009
Hamideh, R. A., Akbari, B., Fathi, P., Misra, S. K., Sutrisno, A., Lam, F., et al. (2020). Biodegradable MRI visible drug eluting stent reinforced by metal organic frameworks. Adv. Healthc. Mater. 9:2000136. doi: 10.1002/adhm.202000136
Harding, J. L., and Reynolds, M. M. (2012). Metal organic frameworks as nitric oxide catalysts. J. Am. Chem. Soc. 134, 3330–3333. doi: 10.1021/ja210771m
Harding, J. L., and Reynolds, M. M. (2014). Composite materials with embedded metal organic framework catalysts for nitric oxide release from bioavailable S-nitrosothiols. J. Mater. Chem. B 2, 2530–2536. doi: 10.1039/C3TB21458C
Ho, Y. T., Adriani, G., Beyer, S., Nhan, P. T., Kamm, R. D., and Kah, J. C. Y. (2017). A facile method to probe the vascular permeability of nanoparticles in nanomedicine applications. Sci. Rep. 7:707. doi: 10.1038/s41598-017-00750-753
Ho, P. H., Salles, F., Di Renzo, F., and Trens, P. (2020). One-pot synthesis of 5-FU@ZIF-8 and ibuprofen@ZIF-8 nanoparticles. Inorganica Chim. Acta 500:119229. doi: 10.1016/j.ica.2019.119229
Hu, Q., Yu, J., Liu, M., Liu, A., Dou, Z., and Yang, Y. (2014). A low cytotoxic cationic metal–organic framework carrier for controllable drug release. J. Med. Chem. 57, 5679–5685. doi: 10.1021/jm5004107
Inacio, M. C. S., Ake, C. F., Paxton, E. W., Khatod, M., Wang, C., Gross, T. P., et al. (2013). Sex and risk of hip implant failure. JAMA Intern. Med. 173:435. doi: 10.1001/jamainternmed.2013.3271
Joseph, N., Lawson, H. D., Overholt, K. J., Damodaran, K., Gottardi, R., Acharya, A. P., et al. (2019). Synthesis and characterization of CaSr-Metal organic frameworks for biodegradable orthopedic applications. Sci. Rep. 9:13024. doi: 10.1038/s41598-019-49536-49539
Karakeçili, A., Topuz, B., Korpayev, S., and Erdek, M. (2019). Metal-organic frameworks for on-demand pH controlled delivery of vancomycin from chitosan scaffolds. Mater. Sci. Eng. C 105:110098. doi: 10.1016/j.msec.2019.110098
Keskin, S., and Kizilel, S. (2011). Biomedical applications of metal organic frameworks. Ind. Eng. Chem. Res. 50, 1799–1812. doi: 10.1021/ie101312k
Kulow, A., Witte, S., Beyer, S., Guilherme Buzanich, A., Radtke, M., Reinholz, U., et al. (2019). A new experimental setup for time- and laterally-resolved X-ray absorption fine structure spectroscopy in a ‘single shot.’. J. Anal. At. Spectrom. 34, 239–246. doi: 10.1039/C8JA00313K
Kwun, I.-S., Cho, Y.-E., Lomeda, R.-A. R., Shin, H.-I., Choi, J.-Y., Kang, Y.-H., et al. (2010). Zinc deficiency suppresses matrix mineralization and retards osteogenesis transiently with catch-up possibly through Runx 2 modulation. Bone 46, 732–741. doi: 10.1016/j.bone.2009.11.003
Lansdown, A. B. G., Mirastschijski, U., Stubbs, N., Scanlon, E., and Ågren, M. S. (2007). Zinc in wound healing: theoretical, experimental, and clinical aspects. Wound Repair Regen. 15, 2–16. doi: 10.1111/j.1524-475X.2006.00179.x
Li, X., Qi, M., Li, C., Dong, B., Wang, J., Weir, M. D., et al. (2019). Novel nanoparticles of cerium-doped zeolitic imidazolate frameworks with dual benefits of antibacterial and anti-inflammatory functions against periodontitis. J. Mater. Chem. B 7, 6955–6971. doi: 10.1039/C9TB01743G
Li, Z., Peng, Y., Pang, X., and Tang, B. (2020). Potential therapeutic effects of Mg/HCOOH metal organic framework on relieving osteoarthritis. Chem. Med. Chem. 15, 13–16. doi: 10.1002/cmdc.201900546
Liang, K., Coghlan, C. J., Bell, S. G., Doonan, C., and Falcaro, P. (2015a). Enzyme encapsulation in zeolitic imidazolate frameworks: a comparison between controlled co-precipitation and biomimetic mineralisation. Chem. Commun. 52, 473–476. doi: 10.1039/c5cc07577g
Liang, K., Ricco, R., Doherty, C. M., Styles, M. J., Bell, S., Kirby, N., et al. (2015b). Biomimetic mineralization of metal-organic frameworks as protective coatings for biomacromolecules. Nat. Commun. 6:7240. doi: 10.1038/ncomms8240
Liédana, N., Galve, A., Rubio, C., Téllez, C., and Coronas, J. (2012). CAF@ZIF-8: one-step encapsulation of caffeine in MOF. ACS Appl. Mater. Interfaces 4, 5016–5021. doi: 10.1021/am301365h
Liu, W., Yan, Z., Zhang, Z., Zhang, Y., Cai, G., and Li, Z. (2019). Bioactive and anti-corrosive bio-MOF-1 coating on magnesium alloy for bone repair application. J. Alloys Compd. 788, 705–711. doi: 10.1016/j.jallcom.2019.02.281
Liu, Y., and Hsu, S. (2020). Biomaterials and neural regeneration. Neural Regen. Res. 15:1243. doi: 10.4103/1673-5374.272573
Maleki, A., Shahbazi, M., Alinezhad, V., and Santos, H. A. (2020). The progress and prospect of zeolitic imidazolate frameworks in cancer therapy, antibacterial activity, and biomineralization. Adv. Healthc. Mater. 9:2000248. doi: 10.1002/adhm.202000248
Matlinska, M. A., Ha, M., Hughton, B., Oliynyk, A. O., Iyer, A. K., Bernard, G. M., et al. (2019). Alkaline earth metal–organic frameworks with tailorable ion release: a path for supporting biomineralization. ACS Appl. Mater. Interfaces 11, 32739–32745. doi: 10.1021/acsami.9b11004
Myers, P. R., and Tanner, M. A. (1998). Vascular endothelial cell regulation of extracellular matrix collagen: role of nitric oxide. Arterioscler. Thromb. Vasc. Biol. 18, 717–722. doi: 10.1161/01.ATV.18.5.717
Neufeld, M. J., Harding, J. L., and Reynolds, M. M. (2015). Immobilization of metal-organic framework copper(II) benzene-1,3,5-tricarboxylate (CuBTC) onto cotton fabric as a nitric oxide release catalyst. ACS Appl. Mater. Interfaces 7, 26742–26750. doi: 10.1021/acsami.5b08773
Neufeld, M. J., Lutzke, A., Jones, W. M., and Reynolds, M. M. (2017a). Nitric oxide generation from endogenous substrates using metal-organic frameworks: inclusion within poly(vinyl alcohol) membranes to investigate reactivity and therapeutic potential. ACS Appl. Mater. Interfaces. 9, 35628–35641. doi: 10.1021/acsami.7b11846
Neufeld, M. J., Lutzke, A., Tapia, J. B., and Reynolds, M. M. (2017b). Metal-Organic framework/chitosan hybrid materials promote nitric oxide release from S-nitrosoglutathione in aqueous solution. ACS Appl. Mater. Interfaces. 9, 5139–5148. doi: 10.1021/acsami.6b14937
Neufeld, M. J., Ware, B. R., Lutzke, A., Khetani, S. R., and Reynolds, M. M. (2016). Water-Stable metal-organic framework/polymer composites compatible with human hepatocytes. ACS Appl. Mater. Interfaces. 8, 19343–19352. doi: 10.1021/acsami.6b05948
Ni, Z., and Masel, R. I. (2006). Rapid production of metal-organic frameworks via microwave-assisted solvothermal synthesis. J. Am. Chem. Soc. 128, 12394–12395. doi: 10.1021/ja0635231
Pan, H. M., Beyer, S., Zhu, Q., and Trau, D. (2013). Inwards interweaving of polymeric layers within hydrogels: assembly of spherical multi-shells with discrete porosity differences. Adv. Funct. Mater. 23, 5108–5115. doi: 10.1002/adfm.201300733
Park, K. S., Ni, Z., Côté, A. P., Choi, J. Y., Huang, R., Uribe-Romo, F. J., et al. (2006). Exceptional chemical and thermal stability of zeolitic imidazolate frameworks. Proc. Natl. Acad. Sci. U S A. 103, 10186–10191. doi: 10.1073/pnas.0602439103
Pei, P., Tian, Z., and Zhu, Y. (2018). 3D printed mesoporous bioactive glass/metal-organic framework scaffolds with antitubercular drug delivery. Microporous Mesoporous Mater. 272, 24–30. doi: 10.1016/j.micromeso.2018.06.012
Pinna, A., Ricco’, R., Migheli, R., Rocchitta, G., Serra, P. A., Falcaro, P., et al. (2018). A MOF-based carrier for in situ dopamine delivery. RSC Adv. 8, 25664–25672. doi: 10.1039/C8RA04969F
Pinto, R. V., Wang, S., Tavares, S. R., Pires, J., Antunes, F., Vimont, A., et al. (2020). Tuning cellular biological functions through the controlled release of NO from a porous Ti-MOF. Angew. Chemie Int. Ed. 59, 5135–5143. doi: 10.1002/anie.201913135
Radomski, M. W., Palmer, R. M. J., and Moncada, S. (1987). Endogenous nitric oxide inhibits human platelet adhesion to vascular endothelium. Lancet 2, 1057–1058. doi: 10.1016/S0140-6736(87)91481-91484
Ran, J., Zeng, H., Cai, J., Jiang, P., Yan, P., Zheng, L., et al. (2018). Rational design of a stable, effective, and sustained dexamethasone delivery platform on a titanium implant: an innovative application of metal organic frameworks in bone implants. Chem. Eng. J. 333, 20–33. doi: 10.1016/j.cej.2017.09.145
Rojas, S., Devic, T., and Horcajada, P. (2017). Metal organic frameworks based on bioactive components. J. Mater. Chem. B 5, 2560–2573. doi: 10.1039/C6TB03217F
Sanchis-Gomar, F., Perez-Quilis, C., Leischik, R., and Lucia, A. (2016). Epidemiology of coronary heart disease and acute coronary syndrome. Ann. Transl. Med. 4:256. doi: 10.21037/atm.2016.06.33
Sarkar, C., Chowdhuri, A. R., Garai, S., Chakraborty, J., and Sahu, S. K. (2019). Three-dimensional cellulose-hydroxyapatite nanocomposite enriched with dexamethasone loaded metal–organic framework: a local drug delivery system for bone tissue engineering. Cellulose 26, 7253–7269. doi: 10.1007/s10570-019-02618-2613
Schäffer, M. R., Tantry, U., Gross, S. S., Wasserkrug, H. L., and Barbul, A. (1996). Nitric oxide regulates wound healing. J. Surg. Res. 63, 237–240. doi: 10.1006/jsre.1996.0254
Sen, C. K., Khanna, S., Venojarvi, M., Trikha, P., Christopher Ellison, E., Hunt, T. K., et al. (2002). Copper-induced vascular endothelial growth factor expression and wound healing. Am. J. Physiol. - Hear. Circ. Physiol. 282, H1821–H1827. doi: 10.1152/ajpheart.01015.2001
Sharma, J. N., Al-Omran, A., and Parvathy, S. S. (2007). Role of nitric oxide in inflammatory diseases. Inflammopharmacology 15, 252–259. doi: 10.1007/s10787-007-0013-x
Stolar, T., and Užareviæ, K. (2020). Mechanochemistry: an efficient and versatile toolbox for synthesis, transformation, and functionalization of porous metal-organic frameworks. CrystEngComm 22, 4511–4525. doi: 10.1039/d0ce00091d
Su, Y., Cockerill, I., Wang, Y., Qin, Y.-X., Chang, L., Zheng, Y., et al. (2019). Zinc-Based biomaterials for regeneration and therapy. Trends Biotechnol. 37, 428–441. doi: 10.1016/j.tibtech.2018.10.009
Tan, L.-L., Song, N., Zhang, S. X.-A., Li, H., Wang, B., and Yang, Y.-W. (2016). Ca 2+, pH and thermo triple-responsive mechanized Zr-based MOFs for on-command drug release in bone diseases. J. Mater. Chem. B 4, 135–140. doi: 10.1039/C5TB01789K
Tao, B., Zhao, W., Lin, C., Yuan, Z., He, Y., Lu, L., et al. (2020). Surface modification of titanium implants by ZIF-8@Levo/LBL coating for inhibition of bacterial-associated infection and enhancement of in vivo osseointegration. Chem. Eng. J. 390:124621. doi: 10.1016/j.cej.2020.124621
Telgerd, M. D., Sadeghinia, M., Birhanu, G., Daryasari, M. P., Zandi-Karimi, A., Sadeghinia, A., et al. (2019). Enhanced osteogenic differentiation of mesenchymal stem cells on metal–organic framework based on copper, zinc, and imidazole coated poly- l -lactic acid nanofiber scaffolds. J. Biomed. Mater. Res. Part A 107, 1841–1848. doi: 10.1002/jbm.a.36707
Tian, S., and Tian, D. (2020). A water-stable Cu(II)-based coordination polymer for clinical nursing effect on schizophrenia by inhibiting over-activity of the dopaminergic neurons. Supramol. Chem. 32, 365–372. doi: 10.1080/10610278.2020.1739285
Wang, Y., Yan, J., Wen, N., Xiong, H., Cai, S., He, Q., et al. (2020). Metal-organic frameworks for stimuli-responsive drug delivery. Biomaterials 230:119619. doi: 10.1016/j.biomaterials.2019.119619
Wixey, J. A., Sukumar, K. R., Pretorius, R., Lee, K. M., Colditz, P. B., Bjorkman, S. T., et al. (2019). Ibuprofen treatment reduces the neuroinflammatory response and associated neuronal and white matter impairment in the growth restricted newborn. Front. Physiol. 10:541. doi: 10.3389/fphys.2019.00541
Wuttke, S., Zimpel, A., Bein, T., Braig, S., Stoiber, K., Vollmar, A., et al. (2017). Validating metal-organic framework nanoparticles for their nanosafety in diverse biomedical applications. Adv. Healthc. Mater. 6:1600818. doi: 10.1002/adhm.201600818
Xiao, J., Chen, S., Yi, J., Zhang, H. F., and Ameer, G. A. (2017). A cooperative copper metal–organic framework-hydrogel system improves wound healing in diabetes. Adv. Funct. Mater. 27:1604872. doi: 10.1002/adfm.201604872
Xiao, J., Zhu, Y., Huddleston, S., Li, P., Xiao, B., Farha, O. K., et al. (2018). Copper metal-organic framework nanoparticles stabilized with folic acid improve wound healing in diabetes. ACS Nano 12, 1023–1032. doi: 10.1021/acsnano.7b01850
Yaghi, O. M., Li, G., and Li, H. (1995). Selective binding and removal of guests in a microporous metal–organic framework. Nature 378, 703–706. doi: 10.1038/378703a0
Yaghi, O. M., and Li, H. (1995). Hydrothermal synthesis of a metal-organic framework containing large rectangular channels. J. Am. Chem. Soc. 117, 10401–10402. doi: 10.1021/ja00146a033
Yao, X., Zhu, G., Zhu, P., Ma, J., Chen, W., Liu, Z., et al. (2020). Omniphobic ZIF-8@Hydrogel membrane by microfluidic-emulsion-templating method for wound healing. Adv. Funct. Mater. 30:1909389. doi: 10.1002/adfm.201909389
Yu, D., Ma, M., Liu, Z., Pi, Z., Du, X., Ren, J., et al. (2020). MOF-encapsulated nanozyme enhanced siRNA combo: control neural stem cell differentiation and ameliorate cognitive impairments in Alzheimer’s disease model. Biomaterials 255:120160. doi: 10.1016/j.biomaterials.2020.120160
Yu, M., You, D., Zhuang, J., Lin, S., Dong, L., Weng, S., et al. (2017). Controlled release of naringin in metal-organic framework-loaded mineralized collagen coating to simultaneously enhance osseointegration and antibacterial activity. ACS Appl. Mater. Interfaces 9, 19698–19705. doi: 10.1021/acsami.7b05296
Zang, Y., Roberts, T. R., Batchinsky, A. I., and Reynolds, M. M. (2020). Metal–Organic framework polymer coating inhibits staphylococcus aureus attachment on medical circulation tubing under static and dynamic flow conditions. ACS Appl. Bio Mater. 3, 3535–3543. doi: 10.1021/acsabm.0c00151
Zhang, P., Li, Y., Tang, Y., Shen, H., Li, J., Yi, Z., et al. (2020). Copper-Based metal–organic framework as a controllable nitric oxide-releasing vehicle for enhanced diabetic wound healing. ACS Appl. Mater. Interfaces 12, 18319–18331. doi: 10.1021/acsami.0c01792
Zhang, Y., Shen, X., Ma, P., Peng, Z., and Cai, K. (2019). Composite coatings of Mg-MOF74 and Sr-substituted hydroxyapatite on titanium substrates for local antibacterial, anti-osteosarcoma and pro-osteogenesis applications. Mater. Lett. 241, 18–22. doi: 10.1016/j.matlet.2019.01.033
Zhang, Y., Xu, J., Ruan, Y. C., Yu, M. K., O’Laughlin, M., Wise, H., et al. (2016). Implant-derived magnesium induces local neuronal production of CGRP to improve bone-fracture healing in rats. Nat. Med. 22, 1160–1169. doi: 10.1038/nm.4162
Zhao, Q., Fan, Y., Zhang, Y., Liu, J., Li, W., and Weng, Y. (2019). Copper-Based SURMOFs for nitric oxide generation: hemocompatibility, vascular cell growth, and tissue response. ACS Appl. Mater. Interfaces 11, 7872–7883. doi: 10.1021/acsami.8b22731
Keywords: metal-organic frameworks, biomaterial, tissue engineering, regenerative medicine, bone, cardio-vascular, nervous tissue
Citation: Shyngys M, Ren J, Liang X, Miao J, Blocki A and Beyer S (2021) Metal-Organic Framework (MOF)-Based Biomaterials for Tissue Engineering and Regenerative Medicine. Front. Bioeng. Biotechnol. 9:603608. doi: 10.3389/fbioe.2021.603608
Received: 07 September 2020; Accepted: 27 January 2021;
Published: 11 March 2021.
Edited by:
Xin Zhao, Hong Kong Polytechnic University, Hong KongReviewed by:
Jose Mauro Granjeiro, National Institute of Metrology, Quality and Technology, BrazilJunling Guo, Sichuan University, China
Dariusz Matoga, Jagiellonian University, Poland
Copyright © 2021 Shyngys, Ren, Liang, Miao, Blocki and Beyer. This is an open-access article distributed under the terms of the Creative Commons Attribution License (CC BY). The use, distribution or reproduction in other forums is permitted, provided the original author(s) and the copyright owner(s) are credited and that the original publication in this journal is cited, in accordance with accepted academic practice. No use, distribution or reproduction is permitted which does not comply with these terms.
*Correspondence: Sebastian Beyer, c2ViYXN0aWFuLmJleWVyQGN1aGsuZWR1Lmhr