- 1Program for the Study and Control of Tropical Diseases—PECET, School of Medicine, University of Antioquia, Medellín, Colombia
- 2Laboratory of Environmental Microbiology, Embrapa Environment, Jaguariúna, Brazil
The huge biological diversity of the Brazilian Cerrado is an important source of economically interesting microbial agents. The phylum Actinobacteria plays an important role in nutrient cycling, potentially improving their availability to plants. In this study, we isolated an actinobacteria (strain 3AS4) from wheat rhizospheres of crops cultivated in the Cerrado biome. Strain 3AS4 was identified as belonging to the genus Streptomyces and had phosphorus mobilization ability, mineralizing approximately 410 μg ml–1 from phytate, 300 μg ml–1 from calcium phosphate, and 200 μg ml–1 from rock phosphate. The analysis of the actinobacteria crude extract by spectrometric techniques revealed the presence of gluconic and 2-ketogluconic acid, and a greenhouse experiment was carried out to evaluate its plant growth promotion activity in soybean. Soil in its natural condition (with no phosphorus addition), 40 kg ha–1 rock phosphate from Bayovar (RP) added to soil, and triple super phosphate (SPT) added to soil were used. Significant differences in plant height were observed at 6 weeks when the plants were inoculated with the 3AS4 strain. The growth of inoculated plants in natural condition was promoted in 17% compared with the RP and SPT non-inoculated conditions, suggesting that inoculation can enable plants to grow with lower chemical P fertilizers. In the plants that were inoculated with the 3AS4 strain in the RP condition, the plant height increased by approximately 80% and the shoot:root ratio was approximately 30% higher compared to control conditions (non-inoculated plants in natural conditions). 3AS4 has P-solubilizing potential and can be exploited as an inoculant for soybean cultivation. These results suggest that this actinobacterium is a valuable resource for sustainable agriculture and will allow the reduction of phosphate fertilization in the future.
Introduction
The Cerrado (Brazilian Savanna) biome is the second largest Brazilian biome and occupies an area of approximately 2 million km2, which is equivalent to up to 20% of the national territory (Bonanomi et al., 2019). Although it is a hostile environment, this biome has a great wealth of species, genetic resources, and ecosystems (Hidasi-Neto et al., 2019). It is a dry and hot environment, but represents a great source of biodiversity; conversely, it has little global recognition compared with the Amazon basin (Marris, 2005; Fonseca and Venticinque, 2018). Several studies have already investigated this biome as a source of raw materials for drug discovery; potential antifungal, antitumor, and antibiotic agents have been identified from plant and microorganismal origin (Quirino et al., 2009; Calixto Júnior et al., 2016; Souza et al., 2016; de Araujo et al., 2018; Genuário et al., 2019). Owing to the favorable resources of the Cerrado biome, such as the flat topography and appropriate soil depth, amount of rainfall, and light intensity (de Pontes et al., 2017) and despite the low pH, low phosphorus (P) content, and high P fixation capacity (Hinsinger, 2001), the Midwest region of Brazil widely cultivates soybean (Glycine max L. Merrill). Moreover since 1970, soybean cultivation has been increasing in the Cerrado, requiring more chemical fertilizer (Roy et al., 2016).
Soybean is one of the most important crops in Brazil (Figueiredo, 2016; Zortea et al., 2018); in the 2018/2019 period, the overall production was 120.3 million tons/year, with a crop yield of 3.833 kg/h (Conab Companhia Nacional de Abastecimento, 2019). It is important owing to its high content (approximately 40%) of vegetable protein (Xing et al., 2018), which constitutes one of the main raw materials for the food industry. A recent study by Da Costa Leite et al. (2018) established that the maximum P efficiency in a soybean production area in the Cerrado, in the state of Bahia, under the no-tillage system (with subsoiling) is 172 kg ha–1. Despite this, in Brazil, the input of phosphate fertilizers is high. Withers et al. (2018) showed that P is applied at 25 kg ha–1 in the state of Paraná, 35 kg ha–1 in the state of Goias, and 50 kg ha–1 in the Matopiba Region; 4.6 tg of P will be applied in Brazil by 2050. The frequent input of phosphate into soils contributes to a build-up of legacy P, which will be of great importance during a P crisis (Kochian, 2012; Withers et al., 2018). The mobilization of soil phosphate by microorganisms has been explored as an alternative to increased phosphate fertilizer application (Khan et al., 2017; Sathya et al., 2017) and is an important tool for exploring legacy P in soils. Microorganisms that belong to the phylum Actinobacteria produce biologically active compounds via complex biosynthetic mechanisms, which enable them to adapt to different ecosystems (Singh et al., 2018), and may act as plant growth promotion molecules (El-Tarabily et al., 2008; Saif et al., 2014). The role of Actinobacteria in promoting plant growth owing to the solubilization of phosphates in the soil has been explored (Palaniyandi et al., 2013; Vurukonda et al., 2018). Microorganisms in this phylum are involved in several biological processes, producing a wide range of bioactive compounds, such as chitinases (Lacombe-Harvey et al., 2018) and cellulase (Bettache et al., 2018). Thus, the aim of the present study was to evaluate the P mobilization potential of Actinobacteria from the Cerrado biome, investigate the possible mechanisms of action, and verify the potential of Actinobacteria to promote the growth of soybean plants.
Results
Isolation and Purification of the Isolates
Sixteen strains belonging to the phylum of actinobacteria were isolated from the rhizospheres of wheat. Screening to verify P mobilization ability showed that three isolates were able to mineralize phytate (3AS4, 2BS5, and 3BS2) (Supplementary Table 1); isolate 3AS4 also had the ability to solubilize calcium and rock phosphate (Supplementary Figures 1A,B) and was selected for phenotypic description, molecular identification, and testing under greenhouse conditions.
Genotypic and Phenotypic Characterization of Strain 3AS4
The phylogenetic tree assembled from the 16S rRNA sequence (1.493 nt) indicated that the isolate belongs to the genus Streptomyces and has a 99% of similarity with Streptomyces rishiriensis NBRC 13407T (Figure 1). With this strain, a defined phylogenetic branch was formed (Figure 1). The accession number for the 3AS4 isolate was MG797670. Isolate 3AS4 had similar morphology and physiology (Supplementary Figures 2, 3) to S. rishiriensis, which produces diffusible pigments in the medium, grows slowly in solid media, and does not produce abundant aerial mycelia or spores (Charousová et al., 2015).
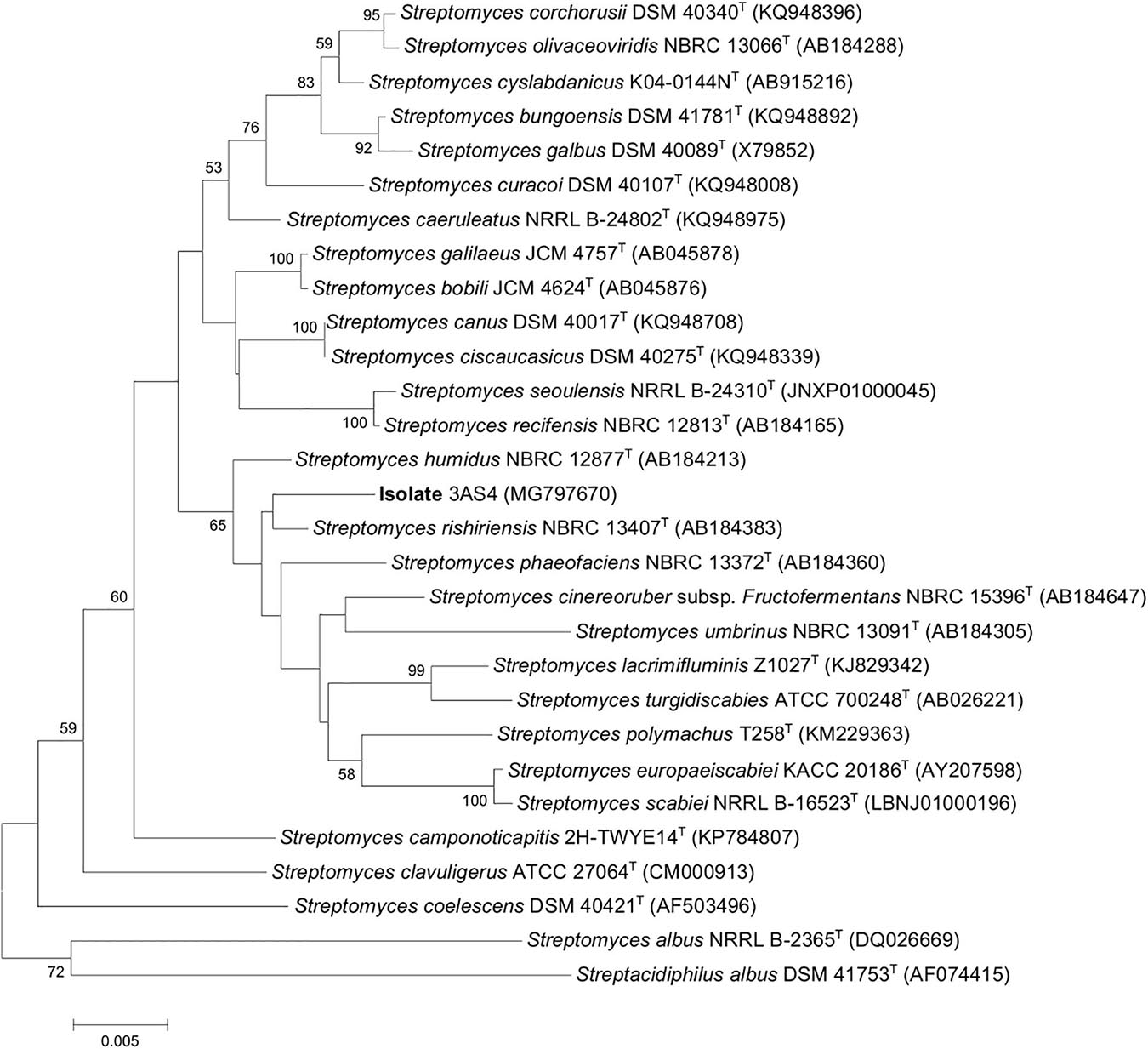
Figure 1. Neighbor-joining phylogenetic tree based on nearly complete 16S rRNA gene sequences showing relationships between 3AS4 strain and closely related type strains of Streptomyces species.
Strain 3AS4 grew well on ISP1, 3, and 4 media and moderately on ISP2, 5, 6, and 7 media. In all ISP media, the reverse of the colony was light brown, except for ISP2 (yellowish/white). The substrate mycelium was whitish to light brown, and the aerial mycelium was present in ISP1, 3, and 4 media, but diffusible pigments were observed only in ISP1 and 3 media (Supplementary Figure 3). A branched, whitish substrate mycelium and pale grayish aerial hypha, which divided into straight to flexuous (rectiflexibilis) chains with smooth surfaces and rod-shaped spores, formed on oatmeal agar (ISP3 medium; Supplementary Figure 4). The strain 3AS4 grew at 16–37°C (optimal, 28°C) and pH 5–10 (optimal, 7.0) and was tolerant of 15% NaCl. Additionally, 3AS4 grew with hydrolyzed starch, casein, cellobiose, D-arabinose, D-galactose, D-maltose, glucose, guanine, hypoxanthine, raffinose, rhamnose, mannitol, myo-inositol, sucrose, and xylitol but not adenine, D-mannose, and D-ribose. Moreover, it grew in degraded tyrosine and Tween 20 and 80 but not xanthine. The 3AS4 strain showed sensitivity (μg ml–1) to rifampicin (10), streptomycin (16), and ampicillin (10) but not erythromycin (15). The strain possessed enzymatic activity against acid phosphatase, alkaline phosphatase, α-glucosidase, β-galactosidase, β-glucosidase, esterase (C4), esterase lipase (C8), leucine arylamidase, valine arylamidase, N-acetyl-β-glucosaminidase, and naphthol-phosphohydrolase.
Potential for P Mobilization
The liquid culture in both calcium and rock phosphate media showed a decrease in pH with time (Figure 2A). This indicates that solubilization occurred owing to the liberation of organic acids by microorganisms during siderophore excretion. The major liberation of phosphate occurred after 12 days in both cases; moreover, the largest amount of phosphate was liberated from phytate (Figure 2B). Phosphate release by the 3AS4 isolate (on days 2–12) ranged from 150 to 410 μg ml–1 in the phytate medium, 190–300 μg ml–1 in the calcium phosphate medium, and 170–200 μg ml–1 in the rock phosphate medium.
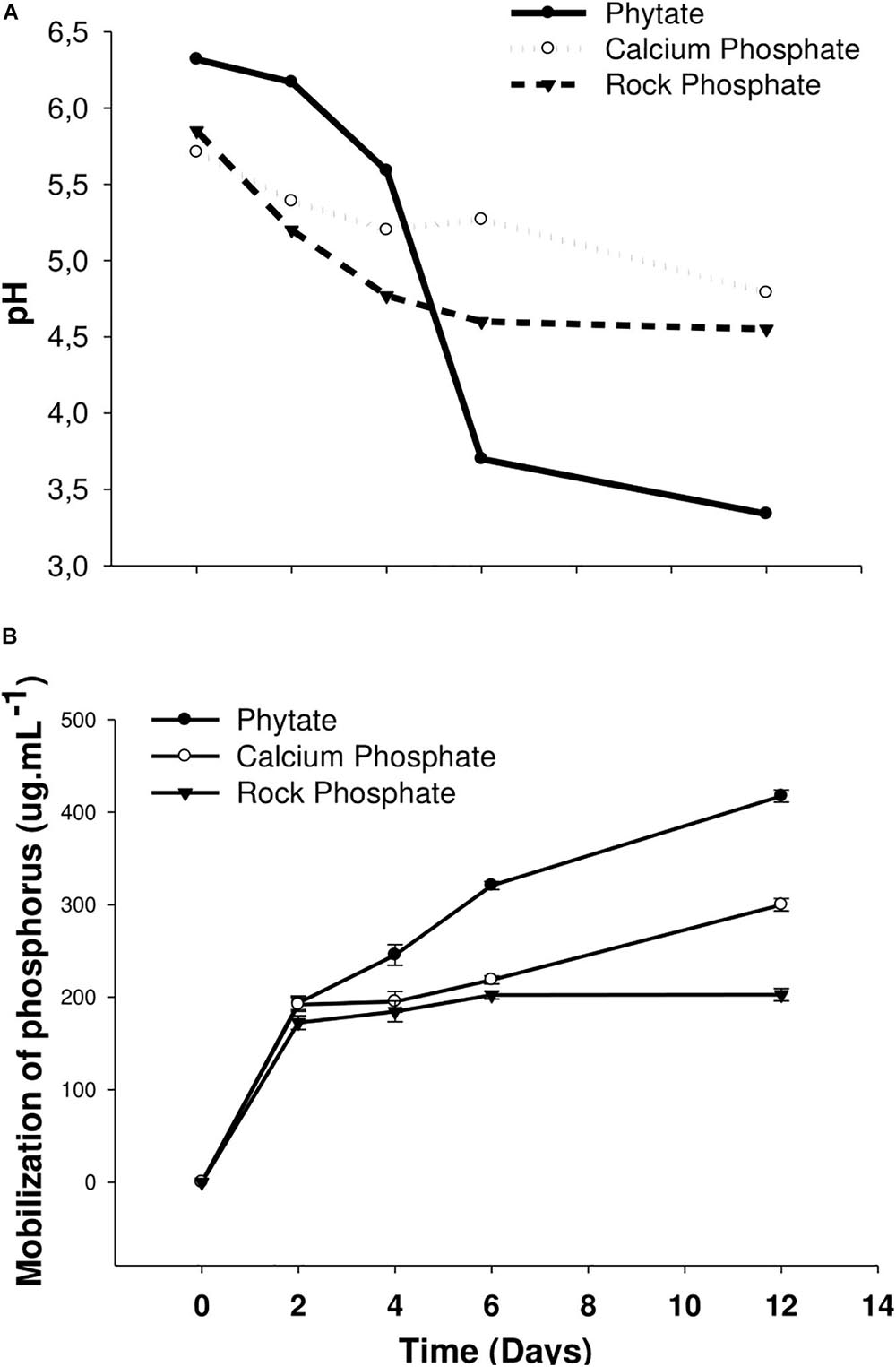
Figure 2. Analysis of the behavior of pH (A) and mobilization of phosphorus (B) in three different media {Phytate, Calcium Phosphate [Ca3(PO4)2], and Rock Phosphate} inoculated with the 3AS4 strain, grown for 12 days at 28°C under stirring.
Identification of Organic Acids
As shown by high-performance liquid chromatography (HPLC), the gluconic acid standard and the aqueous extract of 3AS4 had similar chromatographic profiles after 12 days of incubation (Figure 3).
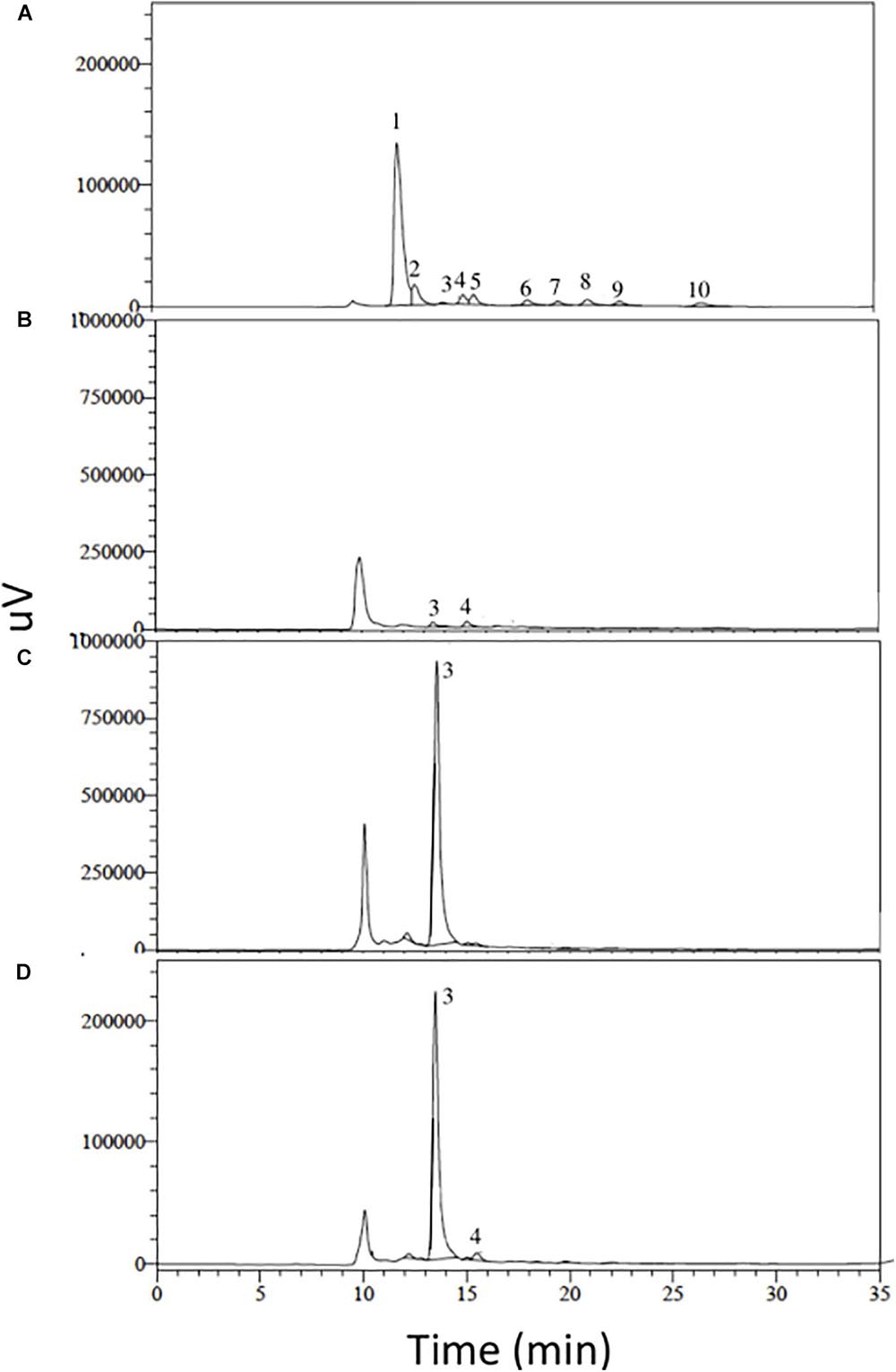
Figure 3. HPLC analysis of organic acids involved in phosphorus solubilization. (A) Chromatographic profile of organic acid standards. (B) Extract of the 3AS4 strain obtained in phytate medium. (C) Extract of the 3AS4 strain obtained in calcium phosphate medium [Ca3(PO4)2]. (D) Extract of the 3AS4 strain obtained in Rock Phosphate medium. All extracts were obtained after 12 days of growth in liquid medium under agitation (140 rpm) at 28°C. Numbers represent the different organic acids used, 1: oxalic acid, 2: citric acid, 3: gluconic acid, 4: 2-ketogluconic acid, 5: malonic acid, 6: succinic acid, 7: lactic acid, 8: formic acid, 9: malic acid, 10: propionic acid.
Cellulolytic Enzymatic Complex Activity
The 3AS4 strain showed cellulase activity, 2BS5 showed chitinase activity, and 3BS2 showed cellulase, glucanase, and xylanase activities (Supplementary Table 1). All three isolates could inhibit the mycelial growth of Sclerotinia sclerotiorum in vitro by 48, 35, and 59%, respectively. Of the three pre-selected isolates, only the 3AS4 strain produced indole acetic acid (Supplementary Table 1), which is associated with the promotion of plant growth (Fialho de Oliveira et al., 2010). Actinobacteria isolates showed a positive association with some other strains of microorganisms (data not shown) isolated from the soybean culture, such as Bradyrhizobium sp., Rhizobium sp., and Azospirillum sp.
Greenhouse Experiment
The 3SA4 strain was efficient at P solubilization and mineralization in vitro. To verify the performance in colonizing the rhizosphere and promoting plant growth, a greenhouse experiment was carried out. 3AS4 inoculation into soil without the addition of P promoted a 17% increase in the height of plants compared with control plants grown under the same conditions (Figures 4A, 5).
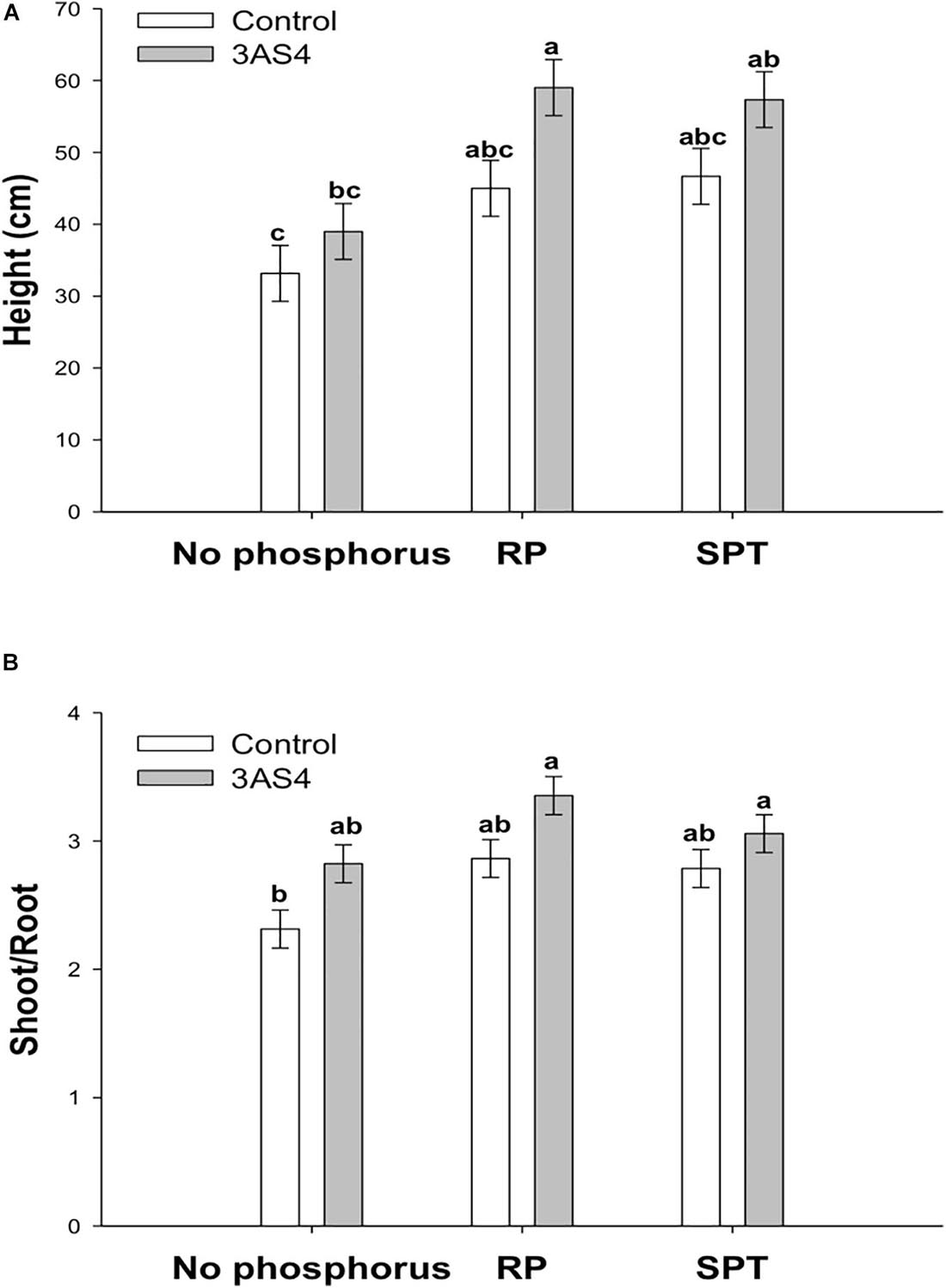
Figure 4. Comparison of the height (A) and shoot:root ratio (B) of the soybean plants inoculated with the 3AS4 strain with two different sources of phosphorus. Rock phosphate (RP) and Single super phosphate (SPT) at 40 kg ha–1. The histograms with the same letter at each case are not significantly different at P < 0.05 (Tukey’s test).
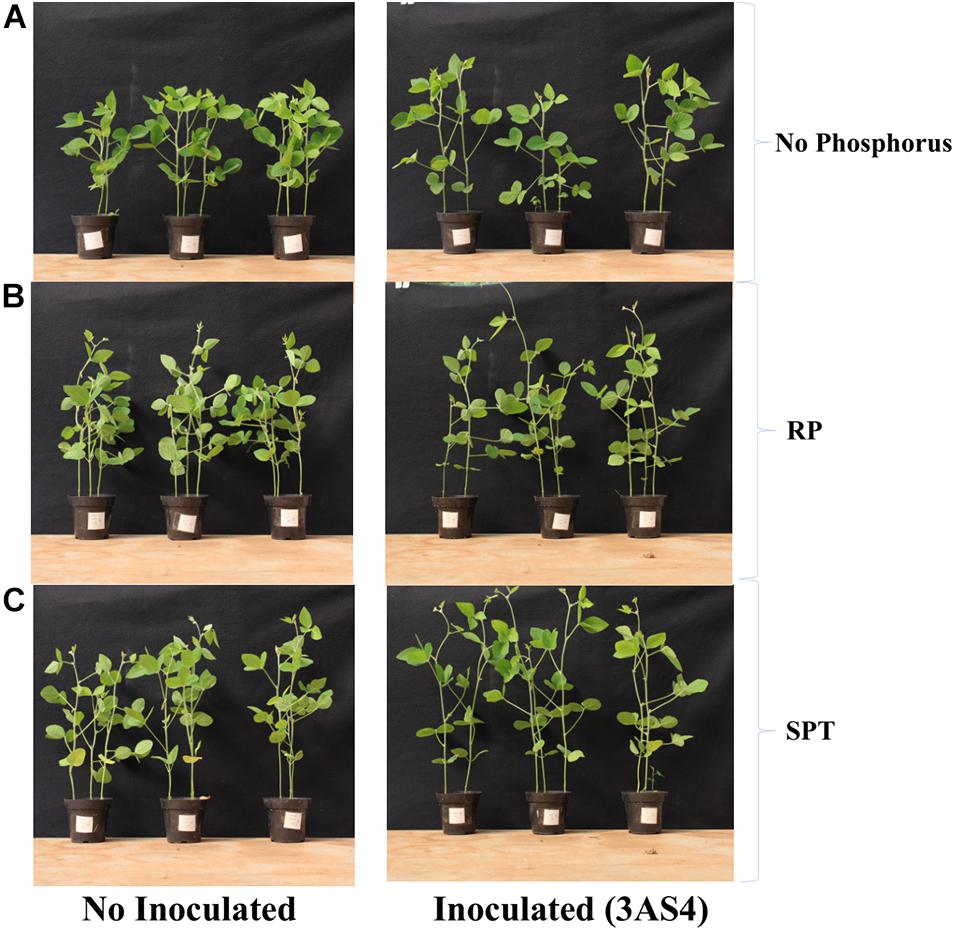
Figure 5. Evaluation of the height of the soybean plants 6 weeks after inoculated with the 3AS4 strain with two different sources of phosphorus. (A) Soybean plants without inoculation of 3AS4 (left), inoculated with 3AS4 isolate and no additional phosphorus. (B) Soybean plants without inoculation of 3AS4 (left), inoculated with 3AS4 isolate and Rock phosphate (RP) at 40 kg ha–1. (C) Soybean plants without inoculation of 3AS4 (left), inoculated with 3AS4 isolate and Single super phosphate (SPT) at 40 kg ha–1.
When the 3AS4 strain was inoculated with an insoluble P source (RP), shoot growth was almost 80% greater than that in plants grown under P-limiting conditions (Figure 4A). The shoot:root ratio was approximately 30% higher when rock phosphate was added with the isolate than when only phosphate was used (Figure 4B).
Discussion
Genotypic Characterization
The morphological and genetic characteristics of the 3AS4 strain indicate that it is part of the Streptomyces genus. The 16S rRNA sequence data supported the assignment of the isolate 3AS4 to S. rishiriensis (NBRC 13407T) with 99.03% similarity. S. rishiriensis produces antibiotics (Matsumoto et al., 1999; Wang et al., 2000; Charousová et al., 2015); however, to the best of our knowledge, this is the first report of this species as a growth stimulant in soybean plants.
Potential of Phosphorus Mobilization
Previous studies have reported high numbers of Actinobacteria in Cerrado soils (Nascimento et al., 2005; Suela Silva et al., 2013; de Sales et al., 2017; Vieira et al., 2018; Vargas Hoyos et al., 2019). These soils are acidic and have a low availability of macronutrients (nitrogen, P, potassium, calcium, magnesium, and sulfur) and micronutrients (boron, copper, molybdenum, and zinc) (Cruz Ruggiero et al., 2002; Oliveira and Marquis, 2002; Genuário et al., 2019). Moreover, these soils usually show high aluminum saturation and P fixation potential (Lopes, 1996; Junio de Jesús Lacerda et al., 2015; Mariussi et al., 2019). The presence of P-mobilizing bacteria in the rhizosphere might allow a higher availability of P to plants in a beneficial association, where bacteria are provided with nutrients and niche to thrive in the rhizosphere (Oliveira et al., 2009; Estrada-Bonilla et al., 2017; Zheng et al., 2019).
Therefore, this microhabitat should be explored for potential inoculants. Previous studies have reported the liberation of phosphate by Actinobacteria, especially by Streptomyces species. Hamdali et al. (2008) reported the capacity of several strains isolated from three Moroccan phosphate mining centers to solubilize phosphate in a synthetic minimum medium supplemented with rock phosphate; two had values of 21.43 and 29.67 μg L–1 after a 5 day incubation at 28°C. Oliveira et al. (2009) described six strains that belong to the Streptomyces genus isolated from Cerrado rhizosphere maize plants; S. platensis, S. tumescens, S. chartreusis, S. griseochromogenes, S. collinus, and S. avermitillis are capable of solubilizing 68, 43.1, 23.5, 4.1, 1.8, and 3.7 mg phosphate L–1 tricalcium phosphate, respectively. In the same study, two Arthrobacter strains solubilized 28 and 30.5 mg phosphate L–1 sodium phytate after a 10 day incubation at 27°C. Wang et al. (2018) describes a strain of S. griseoplanus isolated from the maize rhizosphere that solubilized 284.80 μg phosphate ml–1 tricalcium phosphate after a 20 day incubation at 28°C. Similarly, Solans et al. (2019) evaluated five actinobacterial strains, two of which, belonging to the genus Streptomyces, were the most efficient solubilizers of Ca3PO4 of 2.27 and 2.149 mg L–1 (PO4–2) g–1, respectively, after 14 days at 28°C.
The results obtained in this study showed that, after 3AS4 inoculation, 410 μg ml–1 phosphate was released from phytate, 300 μg ml–1 was released from calcium phosphate, and 200 μg. ml–1 was released from rock phosphate in vitro. It is important to highlight that the time required for P mobilization was relatively short (12 days) and the temperature required was moderate (28°C).
Soils vary greatly in pH and other characteristics; therefore, there is no phosphate compound most suitable to screen the most efficient mobilizing microorganisms. Therefore, more than one source of phosphate should be used to screen organic compounds and rock phosphate (Bashan et al., 2013). The most frequently evaluated source of P is (tri-)calcium phosphate. The results of this study showed the ability of S. rishiriensis to solubilize both organic and inorganic P, demonstrating the great potential of the 3AS4 strain for P solubilization. Furthermore, positive results for the acid and alkaline phosphatase enzymes suggest that more than one mobilization process could be carried out by this isolate.
Identification of Organic Acids
Microorganisms that mobilize P at low pH levels, often owing to the production of organic acids, have been widely documented. Different genera of actinobacteria, such as Micromonospora and Streptomyces, have been reported to solubilize phosphate through siderophore production (Hamdali et al., 2008, 2010; Gopalakrishnan et al., 2014). Moreover, genera such as Arthrobacter and Actinomadura are able to produce organic acids, such as lactic and citric acids (Sharma et al., 2013). However, gluconic acid is the most commonly reported and most efficient solubilizing agent (Alori et al., 2017).
Recent studies have demonstrated the presence of organic acids in Streptomyces isolates with the capacity to stimulate growth in plants through the release of free phosphate. Jog et al. (2014) reported two Streptomyces strains capable of producing gluconic and malic acids. Similarly, Farhat et al. (2015) evaluated 128 isolates of actinobacteria and selected two, belonging to the genus Streptomyces, that possessed a remarkable capacity to produce gluconic acid. Prieto-Correal et al. (2015) evaluated 15 isolates of Streptomyces, 14 of which were able to produce organic acids in both tricalcium phosphate and aluminum phosphate media, most frequently citric, tartaric, and gluconic acids. Solans et al. (2019) evaluated five Streptomyces isolates, all of which were able to produce organic acids in both calcium phosphate and aluminum phosphate media, most frequently gluconic, oxalic, citric, and malic acids. In this study, we identified a S. rishiriensis isolate with the ability to solubilize P and identified that both gluconic and 2-ketogluconic acids were involved in the process. In general, the majority of P-solubilizing microorganisms produce gluconic acid but not 2-ketogluconic acid (Kumar et al., 2013). However, there is a common organic acid profile in the isolates of Streptomyces sp., in which gluconic acid is often found, whereas 2-ketogluconic acid is less frequently reported. It would be useful to search for an operon associated with the production of 2-ketogluconic acid, as is the case for gluconate dehydrogenase (gad), to enhance the production of this organic acid, as has been successfully identified for some gram negative bacterial genera (Kumar et al., 2013).
Cellulolytic Enzymatic Complex Activities
Enzymatic activity might be one of the main mechanisms of the control of soil-borne pathogens by actinobacteria (El-Tarabily et al., 2009; Kaur et al., 2013; Swiontek Brzezinska et al., 2013), such as S. sclerotiorum, which is responsible for the loss of 20–30% and, in some cases, at least 70% of soybean crops in Brazil (de Oliveira et al., 2019; Meyer et al., 2019). It is estimated that approximately 28% of the total area of production is infected, compromising almost 10 million ha (Meyer et al., 2019).
In addition to the ability to mobilize phosphate, microorganisms usually show potential to produce indole acetic acid (AIA) and siderophores, which also promote plant growth (Fialho de Oliveira et al., 2010; Shrivastava et al., 2017). The selected isolate in this study also showed the capacity to produce AIA; this result is consistent with those of previous studies that demonstrated the liberation of this hormone by Streptomyces sp. with plant growth-promoting activity (Abd-Alla et al., 2013; Myo et al., 2019; Chukwuneme et al., 2020). The siderophore is also responsible for the mobilization of phosphate by the formation of stable complexes with P from aluminum, iron, and calcium compounds (Hamdali et al., 2008; Soumare et al., 2020).
Greenhouse Experiment
Soybean crops require a lot of nutrients, especially nitrogen and P. Since 1960, when soybeans began to be produced on a commercial scale in Brazil, measures were adopted, emphasizing biological nitrogen fixation (Hungria and Vargas, 2000). Currently, the soybean crop obtains the greatest proportion of nitrogen from biological fixation (Ambrosini et al., 2019). This was achieved by the selection of rhizobia for the formulation of inoculants and selection of plants predisposed to nodulation. The second nutrient limiting soybean production is P. In Brazil, soybean cultivation is responsible for more of the input of phosphate fertilizers to the soil than any other crop (Withers et al., 2018). P is strongly attached to colloids in tropical acidic soils, mainly by iron and aluminum; thus, less than 0.1% of soil P is in its soluble form (Silva et al., 2019). As with nitrogen, the application of inoculants based on microorganisms capable of releasing soil-bound P has great potential to increase the availability of nutrients for agriculture (Kumar et al., 2018; Kalayu, 2019; Sammauria et al., 2020).
One strategy to reduce P fixation in the soil is the use of less soluble P sources. The application of rock phosphates presents several advantages compared with the application of other phosphate fertilizers (Van Straaten, 2006; Hellal et al., 2019). Because they do not undergo the same chemical process as the production of conventional fertilizers, phosphate rocks are usually cheaper and have lower environmental impact processes (Zapata and Roy, 2004; Schröder et al., 2011). Additionally, owing to their variable and complex chemical composition, they are sources of several nutrients in addition to P. The slow release of nutrients from the rock to the soil supplies the nutritional requirements of agricultural crops and reduces the rate of P fixation (Zapata and Roy, 2004; Mishra and Nayak, 2020). However, a major problem with the use of rock phosphates as a source of nutrients is that in the initial years, production tends to be lower than that with soluble phosphates (Hellal et al., 2019).
Sustainable agriculture is being promoted by practices such as soil management, the genetic engineering of plants, and the application of inoculants to increase nutrient intake (Nimnoi et al., 2014; de Sousa and Olivares, 2016). The solubilization, mobilization, assimilation, and use of P have been the focus of several studies in recent years (Tian et al., 2012; Nesme et al., 2014; Kalayu, 2019). The application of inoculants to the soil has been shown to be sustainable and beneficial to the environment owing to the reduction in the need for P fertilizer application (Kaur et al., 2013; Sharma et al., 2013). The response of the plant to inoculated microorganisms is usually related to complex interactions with the soil and rhizospheric communities adapted to the plant (Jones and Oburger, 2011; Ahkami et al., 2017; Vurukonda et al., 2018).
In this study, isolate 3AS4 played a significant role in the promotion of soybean growth; it increased the height of plants by 17% (Figures 4A, 5), which agrees with Henri (2014), who indicated that the addition of microorganisms that solubilize P can increase plant growth by between 10 and 15%.
When the 3AS4 strain was inoculated into a pot with an insoluble phosphorus source (RP), soybean plants presented an almost 80% increase in shoot growth and 30% increase in the shoot:root ratio (Figures 4B, 5). These results are complementary to those already reported by Fatmawati et al. (2019), who described six isolates of actinobacteria that stimulated plant growth in vitro in soybean by exerting significant effects on root elongation, the number of lateral roots, and root dry weight. Mba (1994) described an increase in soybean production under field conditions after the co-inoculation of rock phosphate and two isolates of actinobacteria (by 43% and 17%, respectively). Thus, the synergistic effect of 3AS4 and P can be explained by the release of hormones that promote plant growth (Gopalakrishnan et al., 2014; Htwe et al., 2018). We showed that 3AS4 also releases IAA (Supplementary Table 1), and as previously suggested, Actinobacteria that produce this phytohormone aid plant growth (Sathya et al., 2017). There are several reports of actinobacteria as promoters of plant growth in different species; these emphasize the use of these microorganisms and their metabolic products to increase production and control diseases in grain legumes (Sathya et al., 2017) and existing commercial techniques that are routinely employed in vegetable farming (Chaurasia et al., 2018).
Conclusion
Brazil has biomes with distinct environmental characteristics, which can serve as a source of microorganisms with significant biotechnological potential. The 3AS4 isolate showed important P-solubilizing potential and can be exploited as an inoculant for soybean cultivation. Moreover, inoculation of this microorganism with rock phosphate may be a promising strategy to promote crop growth, minimizing losses related to fertilization with soluble P sources and reducing P fertilizer use in soils. There is interest to discover new microorganisms that can facilitate productive processes in agriculture. In this sense, the phylum Actinobacteria requires additional focus, as the species in this phylum are versatile and have the capacity to survive in different environments. To effectively utilize the process of plant nutrient acquisition, efficient mechanisms in the cycling of nutrients from the soil must be identified; then, biofertilizers obtained from soils can be developed.
In conclusion, the 3AS4 strain was isolated, characterized, and shown to be a strain of S. rishiriensis with outstanding biological capabilities, among them, the solubilization of organic and inorganic P. Thus, this strain is an appropriate candidate as an alternative tool for crop fertilization, to be combined with chemical fertilizers, which will result in less consumption of chemical fertilizers and greater availability of phosphorus for soybean plants.
Materials and Methods
Isolation and Purification
Two sampling sites were performed to obtain the isolates. The first one occurred in Palmital (S 22∘ 47′ 30″; W 50∘ 12′ 18″) São Paulo State, and Planaltina (S 15∘ 36′; W 47∘ 42′) Brasília, DF. The sites had historic of rotation management between soybean crop and wheat crop. The collected material consisted in rhizosphere of the wheat crop. The soil strongly adhered to the wheat roots were collected in plastic bags. The samples were diluted in sterile saline solution (NaCl 0.85%), submitted to agitation for 10 min and sonicated during 20 s, to release the attached microorganisms from soil particles (Shirling and Gottlieb, 1966). Serial dilutions were performed and a subsample of 0.1 ml at 10–4 was cultured in Glucose Yeast Agar (GYA) (Gordon and Mihm, 1962). After the isolation, the Actinobacteria were cultivated in international Streptomyces project Media 2, ISP2, to confirm the purity and stored at 4°C until downstream analysis.
Genotypic and Phenotypic Characterization of Strain 3AS4
DNA extraction was made using Ultra Clean® Microbial DNA Isolation, MO Bio (Calif, United States), according to the manufacturer’s instructions. 16S rRNA gene was amplified with primers: 27F (5′-AGA GTT TGA TCM TGG CTC AG-3′) and 1492R (5′-TAC GGY TAC CTT GTT ACG ACT T-3′). Amplification products were purified using Promega Wizard SV gel and PCR clean-up system. The sequencing reaction was performed with primers 27F, 704F (5′-AGA TTT TCC GAC GGC AGG TT-3′), 1114R (5′-GGG TTG CGC TCG TTG C-3′), and 1492R, using BIGDYE protocol (Applied biosystems, Foster City, Calif.) in 3500 Genomic Analyzer Sequencer (Applied Biosystems). The sequences were assembled using the CLC Software1 and compared to strains with the Ez-Taxón e-server (Kim et al., 2012). The alignment of sequences was performed with Clustal W (Thompson et al., 1994) and phylogenetic trees were inferred by neighbor-joining method (Saitou and Nei, 1987) in MEGA 7 (Kumar et al., 2016).
The growth cultural characteristics were assessed using standard ISP media, after inoculation at 28∘C for 3 weeks. Characterization of the temperature growth, pH, NaCl tolerance, and antibiotic sensitivity was conducted in Glucose Yeast Extract Agar (GYEA) (Shirling and Gottlieb, 1966). The assimilation of carbon sources and degradation of compounds were checked using the basal culture medium (Sigma) and GYEA, respectively (Gordon and Mihm, 1962). Morphological observations of spore arrangement and ornamentation were conducted by scanning electron microscopy of oatmeal agar (ISP-3) cultures incubated at 28∘C for 3 weeks, using the gold-coated dehydrated samples that were made as described by O’Donnell et al. (1993). Enzymatic activities were determined using API®ZYM strips (BioMérieux), according to the manufacturer’s instructions. Dual culture assay was performed against S. sclerotiorum as described by Cheng et al. (2014).
Phosphorus Mobilization Activity
The actinobacteria isolates were submitted to assays for assessing the potential for mobilization and mineralization of different sources of phosphates such as phytate and rock phosphates using the reference medium NBRIP (National Botanical Research Institute’s Phosphate) (Nautiyal, 1999) and modified NBRIP (Gadagi and Sa, 2002; Singh et al., 2013).
To quantify the amount of phosphate liberated by actinobacteria from the media, 100 μl of 1 × 108 cells of the strain 3AS4 (OD560 = 0.5; Marra et al., 2011) were inoculated in liquid Calcium Phosphate, Phytate, and Rock Phosphate media and incubated at 28oC at 140 rpm; pH measurements and subsamples were taken after 0, 2, 4, 6, and 12 days. The samples were centrifuged at 15,000g for 5 min, and the amount of phosphorus in the supernatant was quantified by the phosphomolybdenum method (Murphy and Riley, 1962). The aqueous phase was filtered on a Millipore® 0.02 μm membrane and applied in high-performance liquid chromatography (HPLC) to verify and identify the production of organic acids. For the separation, we used an Aminex HPX 87H-300 × 7.8 mm column (125-0140 Bio Rad), mobile phase Water:Sulfuric acid 0.005 M, with a flow rate of 0.4 ml min–1, an oven temperature of 50°C, and 20 μl injection volume. Samples were analyzed at the 210 nm length. Analytical curves were performed with the organic acid standards: Oxalic, Citric, Gluconic, 2-ketogluconic, Malonic, Succinic, Lactic, Formic, Malic, and Propionic (Sigma-Aldrich).
Evaluation of Cellulolytic Enzymatic Complex Activity
The isolates were evaluated for cellulase, chitinase, glucanase, and xylanase activity in SMM (Supplemented Minimal Media) with the addition of 1% of specific substrate: CMC (Sigma C5013), Chitin (Sigma C7170), Laminarin (Sigma L9634), and Xilan (Sigma X4252), respectively. The presence of hydrolysis halo around the isolate confirmed the enzymatic activity, which was classified as follows: 1–10 mm as low (+), 11–20 mm as medium (++), and >21 mm as high (+++) enzymatic activity. Qualitative screening of IAA in vitro production was determined by the previous methodology of Kavamura et al. (2013). Compatibility assays were performed between Actinobacteria and beneficial soybean microorganisms, namely, Bradyrhizobium sp., Rhizobium sp., and Azospirillum sp. (Kavamura et al., 2013).
Greenhouse Experiment
Soil was obtained at Embrapa Environment experimental field, Jaguariúna SP; this soil does not have a history of previous fertilization practices and is considered phosphorus (P)-poor soil. Eighteen pots, each containing 400 g of soil, were cultivated with three soybean seed var. Potencia 99 (Embrapa-Soja) under glasshouse conditions (i.e., ±35°C, natural luminosity ±11 h light, 70% field capacity). The potential effect of the actinobacteria was evaluated comparing the inoculated and non-inoculated pots in the absence of phosphorus addition and with the addition of two different sources of phosphorus in a total dose of 40 kg ha–1 P2O5, a total of six treatments each with three replicates. The phosphate sources used in this experiment was Triple Superphosphate (TSP) containing 46% of P2O5 and rock phosphate (RP) containing 31% P2O5, 4.42% SiO2, 0.96% Al2O3, 0.87% Fe2O3, 46% CaO, 0.53% MgO, 1.98% Na2O, 0.3% K2O, 0.01% MnO, and 10.57% LOI. The inoculum was obtained from the filtered biomass of the actinobacteria culture growing in Potato Dextrose (PD; Becton Dickinson) under constant agitation of 135 rpm at 28°C for 7 days. Three milliliters of spore suspension (108 cells) was applied to the pots near each plant. Evaluations occurred after 6 weeks, at the beginning of the flowering stage (R1), considering plant height; after that, shoots and roots were oven dried at 70°C for 72 h to record their dry biomass. Percentage of effect caused by each treatment was calculated according to Henri (2014), considering the negative control and the treatment with no phosphorus addition and no inoculation.
Statistical Analysis
Data obtained were analyzed by Sigma Plot (Version 10; Systat Software). A one-way ANOVA was used to determine the effects of the treatments, which was assumed to be different when the contrast showed a significance level of P ≤ 0.05 using the Tukey test.
Data Availability Statement
The datasets presented in this study can be found in online repositories. The GenBank accession number for the 16S rRNA sequence of Streptomyces sp. 3AS4 is MG797670.
Author Contributions
HV, JC, SNo, and IS designed the experiments. HV, AB-C, and JF performed antagonistic and enzymatic evaluations. HV and JC performed phosphorus mobilization and greenhouse experiments. HV, JP-J, and SNo performed genetic characterization. HV, JC, SNo, and SNa performed chemical work and data analysis. HV, JC, JP-J, and IS wrote the manuscript with contributions from all authors.
Funding
This work was supported by the FAPESP—The São Paulo Research Foundation (Grant Nos. 2013/25076-0 and 2013/16037-0).
Conflict of Interest
The authors declare that the research was conducted in the absence of any commercial or financial relationships that could be construed as a potential conflict of interest.
Supplementary Material
The Supplementary Material for this article can be found online at: https://www.frontiersin.org/articles/10.3389/fbioe.2021.579906/full#supplementary-material
Footnotes
References
Abd-Alla, M. H., Elsayed, E. A., and Rasmey, A. M. (2013). Indole-3-acetic acid (IAA) production by Streptomyces atrovirens isolated from rhizospheric soil in Egypt. J. Biol. Earth Sci. 3, B182–B193.
Ahkami, A. H., Allen White, R., Handakumbura, P. P., and Jansson, C. (2017). Rhizosphere engineering: enhancing sustainable plant ecosystem productivity. Rhizosphere 3, 233–243. doi: 10.1016/j.rhisph.2017.04.012
Alori, E. T., Glick, B. R., and Babalola, O. O. (2017). Microbial phosphorus solubilization and its potential for use in sustainable agriculture. Front. Microbiol. 8:971. doi: 10.3389/fmicb.2017.00971
Ambrosini, V. G., Fontoura, S. M. V., de Moraes, R. P., Tamagno, S., Ciampitti, I. A., and Bayer, C. (2019). Soybean yield response to Bradyrhizobium strains in fields with inoculation history in Southern Brazil. J. Plant Nutr. 42, 1941–1951. doi: 10.1080/01904167.2019.1648680
Bashan, Y., Kamnev, A. A., and de-Bashan, L. E. (2013). Tricalcium phosphate is inappropriate as a universal selection factor for isolating and testing phosphate-solubilizing bacteria that enhance plant growth: a proposal for an alternative procedure. Biol. Fertil. Soils 49, 465–479. doi: 10.1007/s00374-012-0737-7
Bettache, A., Azzouz, Z., Boucherba, N., Bouiche, C., and Hamma, S. (2018). Lignocellulosic biomass and cellulolytic enzymes of actinobacteria. SAJ Biotechnol. 5:203.
Bonanomi, J., Tortato, F. R., Gomes, R., de, S. R., Penha, J. M., Bueno, A. S., et al. (2019). Protecting forests at the expense of native grasslands: land-use policy encourages open-habitat loss in the Brazilian cerrado biome. Perspect. Ecol. Conserv. 17, 26–31. doi: 10.1016/J.PECON.2018.12.002
Calixto Júnior, J. T., de Morais, S. M., Gomez, C. V., Molas, C. C., Rolon, M., Boligon, A. A., et al. (2016). Phenolic composition and antiparasitic activity of plants from the Brazilian Northeast “Cerrado.”. Saudi J. Biol. Sci. 23, 434–440. doi: 10.1016/J.SJBS.2015.10.009
Charousová, I., Javoreková, S., and Wink, J. (2015). Isolation and characterization of Streptomyces rishiriensis (vy31) with antibiotic activity against various pathogenic microorganisms. J. Microbiol. Biotechnol. Food Sci. 04, 23–27. doi: 10.15414/jmbfs.2015.4.special1.23-27
Chaurasia, A., Meena, B. R., Tripathi, A. N., Pandey, K. K., Rai, A. B., and Singh, B. (2018). Actinomycetes: an unexplored microorganisms for plant growth promotion and biocontrol in vegetable crops. World J. Microbiol. Biotechnol. 34, 1–16. doi: 10.1007/s11274-018-2517-5
Cheng, G., Huang, Y., Yang, H., and Liu, F. (2014). Streptomyces felleus YJ1: potential biocontrol agents against the sclerotinia stem rot (Sclerotinia sclerotiorum) of Oilseed Rape. J. Agric. Sci. 6:91. doi: 10.5539/jas.v6n4p91
Chukwuneme, C. F., Babalola, O. O., Kutu, F. R., and Ojuederie, O. B. (2020). Characterization of actinomycetes isolates for plant growth promoting traits and their effects on drought tolerance in maize. J. Plant Interact. 15, 93–105. doi: 10.1080/17429145.2020.1752833
Conab Companhia Nacional de Abastecimento (2019). Boletim da Safra de Grãos. Available online at: https://www.conab.gov.br/info-agro/safras/graos/boletim-da-safra-de-graos (accessed June 4, 2020)
Cruz Ruggiero, P. G., Batalha, M. A., Pivello, V. R., and Meirelles, S. T. (2002). Soil-vegetation relationships in cerrado (Brazilian savanna) and semideciduous forest, Southeastern Brazil. Plant Ecol. 160, 1–16. doi: 10.1023/A:1015819219386
Da Costa Leite, R., Da Costa Leite, R., da Silva Carneiro, J. S., de Freitas, G. A., dos Santos, A. C. M., da Silva, R. R., et al. (2018). No-tillage and phosphate fertilization management on soybean culture in Brazilian Cerrado. J. Agric. Sci. 10:125. doi: 10.5539/jas.v10n5p125
de Araujo, A. S. F., Mendes, L. W., Bezerra, W. M., Nunes, L. A. P. L., de Lyra, M. C. C. P., Figueiredo, M., et al. (2018). Archaea diversity in vegetation gradients from the Brazilian Cerrado. Braz. J. Microbiol. 49, 522–528. doi: 10.1016/J.BJM.2017.08.010
de Oliveira, C. S., Lião, L. M., and Alcantara, G. B. (2019). Metabolic response of soybean plants to Sclerotinia sclerotiorum infection. Phytochemistry 167:112099. doi: 10.1016/j.phytochem.2019.112099
de Pontes, J. S., Oehl, F., Pereira, C. D., de Toledo Machado, C. T., Coyne, D., da Silva, D. K. A., et al. (2017). Diversity of arbuscular mycorrhizal fungi in the Brazilian’s Cerrado and in soybean under conservation and conventional tillage. Appl. Soil Ecol. 117–118, 178–189. doi: 10.1016/J.APSOIL.2017.04.023
de Sales, A. N., de Souza, A. C., Moutta, R., de, O., Ferreira-Leitão, V. S., Schwan, R. F., et al. (2017). Use of lignocellulose biomass for endoxylanase production by Streptomyces termitum. Prep. Biochem. Biotechnol. 47, 505–512. doi: 10.1080/10826068.2016.1275015
de Sousa, J. A., and Olivares, F. L. (2016). Plant growth promotion by Streptomycetes: ecophysiology, mechanisms and applications. Chem. Biol. Technol. Agric. 3, 1–12. doi: 10.1186/s40538-016-0073-5
El-Tarabily, K. A., Nassar, A. H., Hardy, G. E. S. J., and Sivasithamparam, K. (2009). Plant growth promotion and biological control of Pythium aphanidermatum, a pathogen of cucumber, by endophytic actinomycetes. J. Appl. Microbiol. 106, 13–26. doi: 10.1111/j.1365-2672.2008.03926.x
El-Tarabily, K. A., Nassar, A. H., and Sivasithamparam, K. (2008). Promotion of growth of bean (Phaseolus vulgaris L.) in a calcareous soil by a phosphate-solubilizing, rhizosphere-competent isolate of Micromonospora endolithica. Appl. Soil Ecol. 39, 161–171. doi: 10.1016/J.APSOIL.2007.12.005
Estrada-Bonilla, G. A., Lopes, C. M., Durrer, A., Alves, P. R. L., Passaglia, N., and Cardoso, E. J. B. N. (2017). Effect of phosphate-solubilizing bacteria on phosphorus dynamics and the bacterial community during composting of sugarcane industry waste. Syst. Appl. Microbiol. 40, 308–313. doi: 10.1016/j.syapm.2017.05.003
Farhat, M. B., Boukhris, I., and Chouayekh, H. (2015). Mineral phosphate solubilization by Streptomyces sp. CTM396 involves the excretion of gluconic acid and is stimulated by humic acids. FEMS Microbiol. Lett. 362, fnv008. doi: 10.1093/femsle/fnv008
Fatmawati, U., Meryandini, A., Nawangsih, A. A., and Wahyudi, A. T. (2019). Screening and characterization of actinomycetes isolated from soybean rhizosphere for promoting plant growth. Biodivers. J. Biol. Divers. 20, 2970–2977.
Fialho de Oliveira, M., Germano da Silva, M., and Van Der Sand, S. T. (2010). Anti-phytopathogen potential of endophytic actinobacteria isolated from tomato plants (Lycopersicon esculentum) in southern Brazil, and characterization of Streptomyces sp. R18(6), a potential biocontrol agent. Res. Microbiol. 161, 565–572. doi: 10.1016/j.resmic.2010.05.008
Figueiredo, P. N. (2016). New challenges for public research organisations in agricultural innovation in developing economies: evidence from Embrapa in Brazil’s soybean industry. Q. Rev. Econ. Financ. 62, 21–32. doi: 10.1016/J.QREF.2016.07.011
Fonseca, C. R., and Venticinque, E. M. (2018). Biodiversity conservation gaps in Brazil: a role for systematic conservation planning. Perspect. Ecol. Conserv. 16, 61–67. doi: 10.1016/J.PECON.2018.03.001
Gadagi, R. S., and Sa, T. (2002). New isolation method for microorganisms solulbilizing iron and aluminum phosphates using dyes. Soil Sci. Plant Nutr. 48, 615–618. doi: 10.1080/00380768.2002.10409246
Genuário, D. B., Vaz, M. G. M. V., Santos, S. N., Kavamura, V. N., and Melo, I. S. (2019). “Cyanobacteria From Brazilian extreme environments: toward functional exploitation,” in Microbial Diversity in the Genomic Era, (Amsterdam: Elsevier), 265–284. doi: 10.1016/B978-0-12-814849-5.00016-2
Gopalakrishnan, S., Vadlamudi, S., Bandikinda, P., Sathya, A., Vijayabharathi, R., Rupela, O., et al. (2014). Evaluation of Streptomyces strains isolated from herbal vermicompost for their plant growth-promotion traits in rice. Microbiol. Res. 169, 40–48. doi: 10.1016/j.micres.2013.09.008
Gordon, R. E., and Mihm, J. M. (1962). The type species of the genus Nocardia. J. Gen. Microbiol. 27, 1–10. doi: 10.1099/00221287-27-1-1
Hamdali, H., Bouizgarne, B., Hafidi, M., Lebrihi, A., Virolle, M. J., and Ouhdouch, Y. (2008). Screening for rock phosphate solubilizing Actinomycetes from Moroccan phosphate mines. Appl. Soil Ecol. 38, 12–19. doi: 10.1016/j.apsoil.2007.08.007
Hamdali, H., Smirnov, A., Esnault, C., Ouhdouch, Y., and Virolle, M. J. (2010). Physiological studies and comparative analysis of rock phosphate solubilization abilities of Actinomycetales originating from Moroccan phosphate mines and of Streptomyces lividans. Appl. Soil Ecol. 44, 24–31. doi: 10.1016/J.APSOIL.2009.09.001
Hellal, F., El-Sayed, S., Zewainy, R., and Amer, A. (2019). Importance of phosphate pock application for sustaining agricultural production in Egypt. Bull. Natl. Res. Cent. 43, 1–11. doi: 10.1186/s42269-019-0050-9
Henri, F. (2014). Rock-phosphate solubilising bacteria and their effect on soybean (Glycine max) growth under pot grown conditions. Am. J. Agric. For. 2:100. doi: 10.11648/j.ajaf.20140204.11
Hidasi-Neto, J., Joner, D. C., Resende, F., Monteiro, L., de, M., Faleiro, F. V., et al. (2019). Climate change will drive mammal species loss and biotic homogenization in the Cerrado Biodiversity Hotspot. Perspect. Ecol. Conserv. 17, 57–63. doi: 10.1016/J.PECON.2019.02.001
Hinsinger, P. (2001). Bioavailability of soil inorganic P in the rhizosphere as affected by root-induced chemical changes: a review. Plant Soil 237, 173–195. doi: 10.1023/A:1013351617532
Htwe, A. Z., Moh, S. M., Moe, K., and Yamakawa, T. (2018). Effects of co-inoculation of Bradyrhizobium japonicum SAY3-7 and Streptomyces griseoflavus P4 on plant growth, nodulation, nitrogen fixation, nutrient uptake, and yield of soybean in a field condition. Soil Sci. Plant Nutr. 64, 222–229. doi: 10.1080/00380768.2017.1421436
Hungria, M., and Vargas, M. A. T. (2000). Environmental factors affecting N2 fixation in grain legumes in the tropics, with an emphasis on Brazil. Field Crop Res. 65, 151–164. doi: 10.1016/S0378-4290(99)00084-2
Jog, R., Pandya, M., Nareshkumar, G., and Rajkumar, S. (2014). Mechanism of phosphate solubilization and antifungal activity of Streptomyces spp. isolated from wheat roots and rhizosphere and their application in improving plant growth. Microbiology 160, 778–788. doi: 10.1099/mic.0.074146-0
Jones, D. L., and Oburger, E. (2011). “Solubilization of phosphorus by soil microorganisms,” in Phosphorus in Action. Soil Biology, Vol. 26, eds E. Bünemann, A. Oberson, and E. Frossard (Berlin: Springer), 169–198. doi: 10.1007/978-3-642-15271-9_7
Junio de Jesús Lacerda, J., Vilela de Resende, Á, Eduardo Furtini Neto, A., Hickmann, C., and Prates da Conceição, O. (2015). Adubação, produtividade e rentabilidade da rotação entre soja e milho em solo com fertilidade construída. Pesq. Agropec. Bras. 50, 769–778. doi: 10.1590/S0100-204X2015000900005
Kalayu, G. (2019). Phosphate solubilizing microorganisms: promising approach as biofertilizers. Int. J. Agron. 2019, 4917256. doi: 10.1155/2019/4917256
Kaur, T., Sharma, D., Kaur, A., and Manhas, R. K. (2013). Antagonistic and plant growth promoting activities of endophytic and soil actinomycetes. Arch. Phytopathol. Plant Prot. 46, 1756–1768. doi: 10.1080/03235408.2013.777169
Kavamura, V. N., Santos, S. N., da Silva, J. L., Parma, M. M., Ávila, L. A., Visconti, A., et al. (2013). Screening of Brazilian cacti rhizobacteria for plant growth promotion under drought. Microbiol. Res. 168, 183–191. doi: 10.1016/j.micres.2012.12.002
Khan, M. S., Rizvi, A., Saif, S., and Zaidi, A. (2017). “Phosphate-solubilizing microorganisms in sustainable production of wheat: current perspective,” in Probiotics in Agroecosystem, eds V. Kumar, M. Kumar, S. Sharma, and R. Prasad (Singapore: Springer Singapore), 51–81. doi: 10.1007/978-981-10-4059-7_3
Kim, O. S., Cho, Y. J., Lee, K., Yoon, S. H., Kim, M., Na, H., et al. (2012). Introducing EzTaxon-e: a prokaryotic 16s rRNA gene sequence database with phylotypes that represent uncultured species. Int. J. Syst. Evol. Microbiol. 62, 716–721. doi: 10.1099/ijs.0.038075-0
Kochian, L. V. (2012). Plant nutrition: rooting for more phosphorus. Nature 488, 466–467. doi: 10.1038/488466a
Kumar, A., Kumar, A., and Patel, H. (2018). Role of microbes in phosphorus availability and acquisition by plants. Int. J. Curr. Microbiol. Appl. Sci. 7, 1344–1347. doi: 10.20546/ijcmas.2018.705.161
Kumar, C., Yadav, K., Archana, G., and Naresh Kumar, G. (2013). 2-Ketogluconic acid secretion by incorporation of Pseudomonas putida KT 2440 gluconate dehydrogenase (gad) Operon in Enterobacter asburiae PSI3 improves mineral phosphate solubilization. Curr. Microbiol. 67, 388–394. doi: 10.1007/s00284-013-0372-z
Kumar, S., Stecher, G., and Tamura, K. (2016). MEGA7: molecular evolutionary genetics analysis version 7.0 for bigger datasets. Mol. Biol. Evol. 33, 1870–1874. doi: 10.1093/molbev/msw054
Lacombe-Harvey, M.-È, Brzezinski, R., and Beaulieu, C. (2018). Chitinolytic functions in actinobacteria: ecology, enzymes, and evolution. Appl. Microbiol. Biotechnol. 102, 7219–7230. doi: 10.1007/s00253-018-9149-4
Lopes, A. S. (1996). Soils under cerrado: a success story in soil management. Better Crops Int. 10, 1–7.
Mariussi, L. M., da Costa Leite, R., Araújo de Freitas, G., da Costa Leite, R., Martins Dos Santos, A. C., da Silva Carneiro, J. S., et al. (2019). Phosphate fertilization on soils with improved fertility in the brazilian cerrado. Agron. Colomb. 37, 85–92. doi: 10.15446/agron.colomb.v37n1.72123
Marra, L. M., de Oliveira, S. M., Soares, C. R. F. S., and de Souza Moreira, F. M. (2011). Solubilisation of inorganic phosphates by inoculant strains from tropical legumes. Sci. Agric. 68, 603–609. doi: 10.1590/S0103-90162011000500015
Matsumoto, N., Tsuchida, T., Maruyama, M., Kinoshita, N., Homma, Y., Iinuma, H., et al. (1999). Lactonamycin, a new antimicrobial antibiotic produced by Streptomyces rishiriensis MJ773-88K4. I. Taxonomy, fermentation, isolation, physico-chemical properties and biological activities. J. Antibiot. (Tokyo). 52, 269–275. doi: 10.7164/antibiotics.52.269
Mba, C. C. (1994). Field studies on two rock phosphate solubilizing actinomycete isolates as biofertilizer sources. Environ. Manag. 18, 263–269. doi: 10.1007/BF02393767
Meyer, M. C., Campos, H. D., Godoy, C. V., Utiamada, C. M., Dias, A. R., De Souza, D., et al. (2019). Eficiência de Fungicidas Para controle de Mofo-Branco (Sclerotinia sclerotiorum) em Soja, Na Safra 2018/19: Resultados Sumarizados dos Experimentos Cooperativos. Brasilia: Brazilian Agricultural Research Corporation.
Mishra, B. B., and Nayak, S. K. (2020). Frontiers in Soil and Environmental Microbiology. Boca Raton, FL: CRC Press.
Murphy, J., and Riley, J. P. (1962). A modified single solution method for the determination of phosphate in natural waters. Anal. Chim. Acta 27, 31–36. doi: 10.1016/S0003-2670(00)88444-5
Myo, E. M., Ge, B., Ma, J., Cui, H., Liu, B., Shi, L., et al. (2019). Indole-3-acetic acid production by Streptomyces fradiae NKZ-259 and its formulation to enhance plant growth. BMC Microbiol. 19:155. doi: 10.1186/s12866-019-1528-1
Nascimento, R. P., D’Avila-Levy, C. M., Souza, R. F., Branquinha, M. H., Bon, E. P. S., Pereira, N., et al. (2005). Production and partial characterization of extracellular proteinases from Streptomyces malaysiensis, isolated from a Brazilian cerrado soil. Arch. Microbiol. 184, 194–198. doi: 10.1007/s00203-005-0030-3
Nautiyal, C. S. (1999). An efficient microbiological growth medium for screening phosphate solubilizing microorganisms. FEMS Microbiol. Lett. 170, 265–270. doi: 10.1111/j.1574-6968.1999.tb13383.x
Nesme, T., Colomb, B., Hinsinger, P., and Watson, C. A. (2014). “Soil phosphorus management in organic cropping systems: from current practices to avenues for a more efficient use of p resources,” in Organic Farming, Prototype for Sustainable Agricultures: Prototype for Sustainable Agricultures, eds S. Bellon and S. Penvern (Dordrecht: Springer Netherlands), 23–45. doi: 10.1007/978-94-007-7927-3_2
Nimnoi, P., Pongsilp, N., and Lumyong, S. (2014). Co-inoculation of Soybean (Glycine Maxx) with Actinomycetes and Bradyrhizobium japonicum Enhances Plant Growth, Nitrogenase Activity and Plant Nutrition. Milton Park: Taylor & Francis Group.
O’Donnell, A. G., Falconer, C., Goodfellow, M., Ward, A. C., and Williams, E. (1993). Biosystematics and diversity amongst novel carboxydotrophic actinomycetes. Antonie Van Leeuwenhoek 64, 325–340. doi: 10.1007/BF00873091
Oliveira, C. A., Alves, V. M. C., Marriel, I. E., Gomes, E. A., Scotti, M. R., Carneiro, N. P., et al. (2009). Phosphate solubilizing microorganisms isolated from rhizosphere of maize cultivated in an oxisol of the Brazilian Cerrado Biome. Soil Biol. Biochem. 41, 1782–1787. doi: 10.1016/j.soilbio.2008.01.012
Oliveira, P. S., and Marquis, R. J. (2002). The cerrados of Brazil: Ecology and Natural History of a Neotropical Savanna. New York, NY: Columbia University Press.
Palaniyandi, S. A., Yang, S. H., Zhang, L., and Suh, J.-W. (2013). Effects of actinobacteria on plant disease suppression and growth promotion. Appl. Microbiol. Biotechnol. 97, 9621–9636. doi: 10.1007/s00253-013-5206-1
Prieto-Correal, G. C., Prada Salcedo, L. D., Cuervo Patiño, C. L., and Franco Correa, M. (2015). Evaluation of organic acid production by Streptomyces spp. and solubilization of three phosphorus sources by strain T3A. Rev. Colomb. Biotecnol. 17, 111–121. doi: 10.15446/rev.colomb.biote.v17n1.41667
Quirino, B. F., Pappas, G. J., Tagliaferro, A. C., Collevatti, R. G., Neto, E. L., da Silva, M. R. S. S., et al. (2009). Molecular phylogenetic diversity of bacteria associated with soil of the savanna-like Cerrado vegetation. Microbiol. Res. 164, 59–70. doi: 10.1016/j.micres.2006.12.001
Roy, E. D., Richards, P. D., Martinelli, L. A., Coletta, L. D., Lins, S. R. M., Vazquez, F. F., et al. (2016). The phosphorus cost of agricultural intensification in the tropics. Nat. Plants 2:16043. doi: 10.1038/nplants.2016.43
Saif, S., Khan, M. S., Zaidi, A., and Ahmad, E. (2014). “Role of phosphate-solubilizing actinomycetes in plant growth promotion: current perspective,” in Phosphate Solubilizing Microorganisms, eds M. Khan, A. Zaidi, and J. Musarrat (Cham: Springer International Publishing), 137–156. doi: 10.1007/978-3-319-08216-5_6
Saitou, N., and Nei, M. (1987). The neighbor-joining method: a new method for reconstructing phylogenetic trees. Mol. Biol. Evol. 4, 406–425. doi: 10.1093/oxfordjournals.molbev.a040454
Sammauria, R., Kumawat, S., Kumawat, P., Singh, J., and Jatwa, T. K. (2020). Microbial inoculants: potential tool for sustainability of agricultural production systems. Arch. Microbiol. 202, 677–693. doi: 10.1007/s00203-019-01795-w
Sathya, A., Vijayabharathi, R., and Gopalakrishnan, S. (2017). Plant growth-promoting actinobacteria: a new strategy for enhancing sustainable production and protection of grain legumes. 3 Biotech 7:102. doi: 10.1007/s13205-017-0736-3
Schröder, J. J., Cordell, D., Smit, A. L., and Rosemarin, A. (2011). Sustainable Use of Phosphorus. Stockholm: Stockholm Enivronment Institute.
Sharma, S. B., Sayyed, R. Z., Trivedi, M. H., and Gobi, T. A. (2013). Phosphate solubilizing microbes: sustainable approach for managing phosphorus deficiency in agricultural soils. Springerplus 2:587. doi: 10.1186/2193-1801-2-587
Shirling, E. B., and Gottlieb, D. (1966). Methods for characterization of Streptomyces species. Int. J. Syst. Bacteriol. 16, 313–340. doi: 10.1099/00207713-16-3-313
Shrivastava, P., Kumar, R., and Yandigeri, M. S. (2017). In vitro biocontrol activity of halotolerant Streptomyces aureofaciens K20: a potent antagonist against Macrophomina phaseolina (Tassi) Goid. Saudi J. Biol. Sci. 24, 192–199. doi: 10.1016/j.sjbs.2015.12.004
Silva, R. R., Faria, A. J. G., Neto, A. A., Pereira, T. A., Dias, J. D. S., Santos, A. C. M., et al. (2019). High P availability in Brazilian cerrado soils reduces nodulation and grain yield of soybean. Commun. Soil Sci. Plant Anal. 50, 1267–1277. doi: 10.1080/00103624.2019.1614604
Singh, D. P., Patil, H. J., Prabha, R., Yandigeri, M. S., and Prasad, S. R. (2018). “Actinomycetes as potential plant growth-promoting microbial communities,” in Crop Improvement Through Microbial Biotechnology, eds R. Prasad, S. S. Gill, and N. Tuteja (Amsterdam: Elsevier), 27–38. doi: 10.1016/B978-0-444-63987-5.00002-5
Singh, N. K., Joshi, D. K., and Gupta, R. K. (2013). Isolation of phytase producing bacteria and optimization of phytase production parameters. Jundishapur J. Microbiol. 6:6419. doi: 10.5812/jjm.6419
Solans, M., Messuti, M. I., Reiner, G., Boenel, M., Vobis, G., Wall, L. G., et al. (2019). Exploring the response of Actinobacteria to the presence of phosphorus salts sources: Metabolic and co-metabolic processes. J. Basic Microbiol. 59, 487–495. doi: 10.1002/jobm.201800508
Soumare, A., Boubekri, K., Lyamlouli, K., Hafidi, M., Ouhdouch, Y., and Kouisni, L. (2020). From isolation of phosphate solubilizing microbes to their formulation and use as biofertilizers: status and needs. Front. Bioeng. Biotechnol. 7:425. doi: 10.3389/fbioe.2019.00425
Souza, R. C., Mendes, I. C., Reis-Junior, F. B., Carvalho, F. M., Nogueira, M. A., Vasconcelos, A. T. R., et al. (2016). Shifts in taxonomic and functional microbial diversity with agriculture: how fragile is the Brazilian Cerrado? BMC Microbiol. 16:42. doi: 10.1186/s12866-016-0657-z
Suela Silva, M., Naves Sales, A., Teixeira Magalhães-Guedes, K., Ribeiro Dias, D., and Freitas Schwan, R. (2013). Brazilian cerrado soil actinobacteria ecology. Biomed Res. Int. 2013:503805. doi: 10.1155/2013/503805
Swiontek Brzezinska, M., Jankiewicz, U., and Burkowska, A. (2013). Purification and characterization of Streptomyces albidoflavus antifungal components. Appl. Biochem. Microbiol. 49, 451–457. doi: 10.1134/S0003683813050025
Thompson, J. D., Higgins, D. G., and Gibson, T. J. (1994). CLUSTAL W: improving the sensitivity of progressive multiple sequence alignment through sequence weighting, position-specific gap penalties and weight matrix choice. Nucleic Acids Res. 22, 4673–4680.
Tian, J., Wang, X., Tong, Y., Chen, X., and Liao, H. (2012). Bioengineering and management for efficient phosphorus utilization in crops and pastures. Curr. Opin. Biotechnol. 23, 866–871. doi: 10.1016/j.copbio.2012.03.002
Van Straaten, P. (2006). Farming with rocks and minerals: challenges and opportunities. An. Acad. Bras. Cienc. 78, 731–747. doi: 10.1590/S0001-37652006000400009
Vargas Hoyos, H. A., Santos, S. N., Padilla, G., and Melo, I. S. (2019). Genome Sequence of Streptomyces cavourensis 1AS2a, a Rhizobacterium isolated from the Brazilian Cerrado Biome. Microbiol. Resour. Announc. 8:e00065-19. doi: 10.1128/mra.00065-19
Vieira, C. K., Borges, L. G., dos, A., Marconatto, L., Giongo, A., and Stürmer, S. L. (2018). Microbiome of a revegetated iron-mining site and pristine ecosystems from the Brazilian Cerrado. Appl. Soil Ecol. 131, 55–65. doi: 10.1016/j.apsoil.2018.07.011
Vurukonda, S. S. K. P., Giovanardi, D., and Stefani, E. (2018). Plant growth promoting and biocontrol activity of streptomyces spp. As endophytes. Int. J. Mol. Sci. 19:952. doi: 10.3390/ijms19040952
Wang, C., Cui, J., Yang, L., Zhao, C., Wang, T., Yan, L., et al. (2018). Phosphorus-release dynamics by phosphate solubilizing actinomycetes and its enhancement of growth and yields in maize. Int. J. Agric. Biol. 20, 437–444. doi: 10.17957/IJAB/15.0554
Wang, Z. X., Li, S. M., and Heide, L. (2000). Identification of the coumermycin A(1) biosynthetic gene cluster of Streptomyces rishiriensis DSM 40489. Antimicrob. Agents Chemother. 44, 3040–3048.
Withers, P. J. A., Rodrigues, M., Soltangheisi, A., de Carvalho, T. S., Guilherme, L. R. G., Benites, V., et al. (2018). Transitions to sustainable management of phosphorus in Brazilian agriculture. Sci. Rep. 8:2537. doi: 10.1038/s41598-018-20887-z
Xing, Q., de Wit, M., Kyriakopoulou, K., Boom, R. M., and Schutyser, M. A. I. (2018). Protein enrichment of defatted soybean flour by fine milling and electrostatic separation. Innov. Food Sci. Emerg. Technol. 50, 42–49. doi: 10.1016/J.IFSET.2018.08.014
Zheng, B. X., Zhang, D. P., Wang, Y., Hao, X. L., Wadaan, M. A. M., Hozzein, W. N., et al. (2019). Responses to soil pH gradients of inorganic phosphate solubilizing bacteria community. Sci. Rep. 9:25. doi: 10.1038/s41598-018-37003-w
Keywords: Brazilian biodiversity, wheat rhizosphere, Streptomyces rishiriensis, gluconic acid, P-solubilizing potential, plant growth promotion
Citation: Vargas Hoyos HA, Chiaramonte JB, Barbosa-Casteliani AG, Fernandez Morais J, Perez-Jaramillo JE, Nobre Santos S, Nascimento Queiroz SC and Soares Melo I (2021) An Actinobacterium Strain From Soil of Cerrado Promotes Phosphorus Solubilization and Plant Growth in Soybean Plants. Front. Bioeng. Biotechnol. 9:579906. doi: 10.3389/fbioe.2021.579906
Received: 03 July 2020; Accepted: 03 March 2021;
Published: 22 April 2021.
Edited by:
Francisco Dini-Andreote, Pennsylvania State University (PSU), United StatesReviewed by:
Ashok K. Dubey, Netaji Subhas University of Technology, IndiaKit Wayne Chew, Xiamen University Malaysia, Malaysia
Copyright © 2021 Vargas Hoyos, Chiaramonte, Barbosa-Casteliani, Fernandez Morais, Perez-Jaramillo, Nobre Santos, Nascimento Queiroz and Soares Melo. This is an open-access article distributed under the terms of the Creative Commons Attribution License (CC BY). The use, distribution or reproduction in other forums is permitted, provided the original author(s) and the copyright owner(s) are credited and that the original publication in this journal is cited, in accordance with accepted academic practice. No use, distribution or reproduction is permitted which does not comply with these terms.
*Correspondence: Harold Alexander Vargas Hoyos, aGFyb2xkLnZhcmdhc0B1ZGVhLmVkdS5jbw==; Itamar Soares Melo, aXRhbWFyLm1lbG9AZW1icmFwYS5icg==