- 1Department of Microbiology and Immunology, School of Medicine & Holistic Integrative Medicine, Nanjing University of Chinese Medicine, Nanjing, China
- 2State Key Laboratory of Natural Medicines, China Pharmaceutical University, Nanjing, China
- 3Jiangsu Collaborative Innovation Center of Chinese Medicinal Resources Industrialization, Nanjing, China
- 4Key Laboratory of Advanced Drug Preparation Technologies, Ministry of Education and School of Pharmaceutical Sciences, Zhengzhou University, Zhengzhou, China
- 5The Affiliated Hospital of Nanjing University of Chinese Medicine, Jiangsu Province Hospital of Chinese Medicine, Nanjing, China
Terpenoids are a large diverse group of natural products which play important roles in plant metabolic activities. Monoterpenoids are the main components of plant essential oils and the active components of some traditional Chinese medicinal herbs. Some monoterpenoids are widely used in medicine, cosmetics and other industries, and they are mainly obtained by plant biomass extraction methods. These plant extraction methods have some problems, such as low efficiency, unstable quality, and high cost. Moreover, the monoterpenoid production from plant cannot satisfy the growing monoterpenoids demand. The development of metabolic engineering, protein engineering and synthetic biology provides an opportunity to produce large amounts of monoterpenoids eco-friendly using microbial cell factories. This mini-review covers current monoterpenoids production using Saccharomyces cerevisiae. The monoterpenoids biosynthetic pathways, engineering of key monoterpenoids biosynthetic enzymes, and current monoterpenoids production using S. cerevisiae were summarized. In the future, metabolically engineered S. cerevisiae may provide one possible green and sustainable strategy for monoterpenoids supply.
Introduction
Terpenoids are the largest and most structurally diverse class of natural products widely distributed in plants, microorganisms, and insects. More than 55,000 terpenoids have been identified so far (Christianson, 2008). Terpenoids have diverse biological activities, and they can function as chemical defense agents against predation and anti-pathogenic agents (Hijaz et al., 2016; Mahizan et al., 2019). Many terpenoids play critical roles in the interactions of plant-plant and plant-environment (Abbas et al., 2017). Among them, monoterpenoids are a kind of terpenoids composed with two isoprene units, which are widely distributed in plant and used in both pharmacy and medicine (Wojtunik-Kulesza et al., 2019). Recently, sabinene, one kind of monoterpene, has the great potential to be used as advanced biofuel (Zhang et al., 2014). Nowadays, monoterpenoids are mainly extracted from plants using chemical methods. The monoterpenoid contents in natural or engineered plants are low, and the extraction processes are high-cost. Moreover, many medicinal plants grow slowly, and the planting area is limited due to the climate and other environmental conditions (Wang J. et al., 2015). The structure of most monoterpenoids is complex, thus, chemical synthesis is difficult and high-cost (Fu et al., 2018). Therefore, other sustainable supply of monoterpenoids is of great interest.
The development of synthetic biology and metabolic engineering provides opportunities for the microbial biosynthesis of monoterpenoids (Chandran et al., 2011). Industrial-scale production of some terpenoids using engineered microorganisms have been realized, such as artemisinin acid and ginsenosides (Paddon et al., 2013; Yan et al., 2014; Wang P. et al., 2015; Wei et al., 2015; Yang et al., 2020). Recovery of the monoterpenoid biosynthetic pathways and introduction of essential key enzymes into proper microbial host can lead to the production of targeted monoterpenoids (Zebec et al., 2016; Ignea et al., 2019). These provide an alternative way to sustainably supply of monoterpenoids and other Chinese herb-derived natural product (Guan et al., 2020; Wang et al., 2020).
The Function of Plant-Derived Terpenoids
Plant-derived terpenoids (also known as isoprenoids) are secondary metabolites, and they are widely used in the cosmetic, pharmaceutical, fragrance, and flavor industries (Goto et al., 2010; Caputi and Aprea, 2011). The basic skeleton of plant-derived terpenoids are the five-carbon unit of isoprene. According to the number of isoprene unit, terpenoids are classified into monoterpenoids, sesquiterpenoids, diterpenoids, triterpenoids, etc. (Ashour et al., 2010). Plant-derived terpenoids have diverse biological properties, for example, artemisinin (sesquiterpenoid) has anti-malarial effects (Talman et al., 2019); Taxol (diterpenoid) is used to treat ovarian cancer and breast cancer (Weaver, 2014); Glycyrrhetinic acid (triterpenoid) has antiviral and antimicrobial function (Kowalska and Kalinowska-Lis, 2019); Lycopene (tetraterpenoid) has antioxidant effects (Costa-Rodrigues et al., 2018) and helps reduce the risk of osteoporosis (Oliveira et al., 2019).
Many medicinal plants contain volatile monoterpenoids (Zhu et al., 2020). Among them, menthone and pulegone have anti-inflammatory and antiviral pharmacological effects (Božović and Ragno, 2017); (+)-Menthol and other monoterpenoids have antibacterial effects on Staphylococcus aureus and Escherichia coli (Trombetta et al., 2005). Monoterpenoids have been used as ingredients of soap, perfume and food, and some monocyclic monoterpenes can be used as insecticides (Rajput et al., 2018).
Terpenoid Production Strategies
Currently, three possible terpenoid production strategies have been applied, including plant extraction, chemical synthesis, and microbial biosynthesis (Li et al., 2020). Extraction from biomass of herb plants is the traditional terpenoid production method. The monoterpenoids of menthone and pulegone are the effective medicinal ingredients of Nepeta cataria (Wang et al., 2017). The volatile components of N. cataria are mainly extracted by the physical methods of distillation and extraction, including reflux method, and temperature-programmed microwave extraction method (Li et al., 2012). However, the volatile oil contents in Nepeta are low, and extraction of monoterpenoids from Nepeta biomass is difficult (Wen et al., 2019). The chemical structures of terpenoids are relatively complex. Chemical synthesis of terpenoids needs many steps, and the purity and yield of the products are not high enough, making chemical synthesis of terpenoids is difficult and high-cost. With the development of synthetic biology and metabolic engineering, many terpenoids have been successfully synthesized by engineered microorganisms (Sun et al., 2017; Guan et al., 2020). The most successful example is the synthesis of artemisinic acid using metabolically engineered yeasts (Paddon et al., 2013; Kai et al., 2018). In China, the most successful large-scale production of terpenoids is the synthesis of rare ginsenosides with engineered S. cerevisiae (Yan et al., 2014; Wang P. et al., 2015; Wei et al., 2015). Due to the complex structures of monoterpenoids, microbial production of monoterpenoids is of great interest.
Recovery of Monoterpenoid Biosynthetic Pathway
The recovery of monoterpenoid biosynthetic pathway is essential for microbial monoterpenoid biosynthesis. The carbon skeleton of monoterpenoid is formed by the condensation of isopentenyl pyrophosphate (IPP) and dimethylallyl pyrophosphate (DMAPP) (Wang, 2013). There are two distinct biochemical pathways for the synthesis of IPP and DMAPP, the 2C-methyl-D-Erythritol-4-phosphate (MEP) pathway and the mevalonic acid (MVA) pathway (Figure 1 and Supplementary Figure 1). In the plastids of plant cells, protists and most microorganisms, MEP pathway is used to synthesize IPP and DMAPP; While in the cytoplasm of higher eukaryotes, the MVA pathway is used to synthesize IPP and DMAPP (Laule et al., 2003; Vranová et al., 2013).
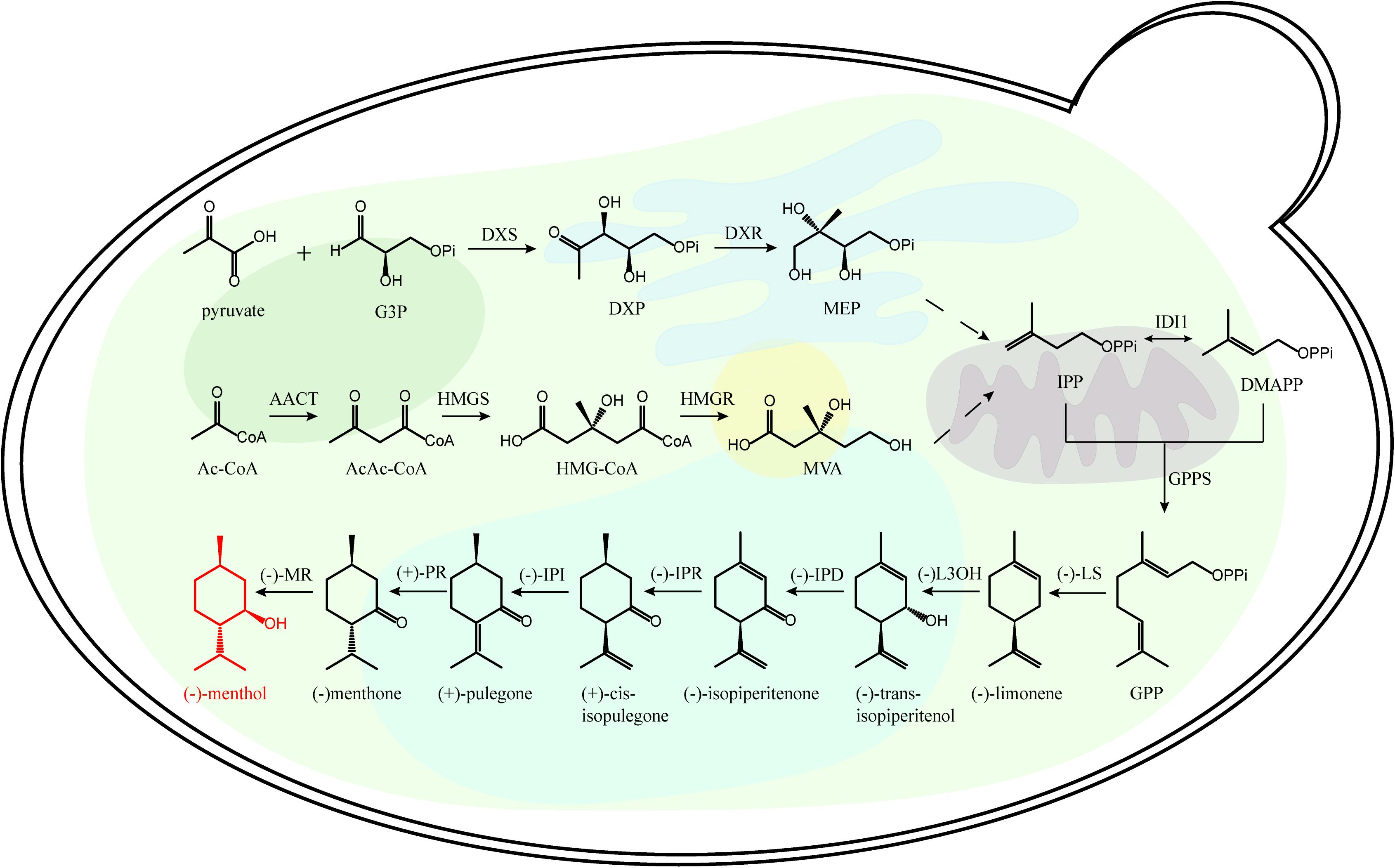
Figure 1. Representative monoterpenoid (menthone) biosynthetic pathway. Ac-CoA, acetyl-CoA; DMAPP dimethylallyl diphosphate; DXP, 1-deoxy-D-xyulose-5-phosphate; DXR, 1-deoxy-D-xylulose 5-phosphate reductoisomerase; DXS, 1-deoxy-D-xylulose 5-phosphate synthase; G3P, glyceraldehyde 3-phosphate; GPP, geranyl diphosphate; GPPS, GPP synthase; HMG-CoA, 3-hydroxy-3-methylglutaryl-CoA; HMGR, 3-hydroxy-3-methylglutaryl-CoA reductase; IDI1, isopentenyl diphosphate isomerase; IPD, trans-isopiperitenol dehydrogenase; IPI, cis-isopulegone isomerase; IPR, isopiperitenone reductase; L3OH, limonene-3-hydroxylase; LS, limonene synthase; MEP, 2C-methyl-D-erythritol 4-phosphate; MR, menthone reductase; MVA, mevalonate; PR, pulegone reductase.
There are several metabolic rate-limiting enzymes in MVA and MEP pathways. In MVA pathway, 3-hydroxy-3-methyl glutaryl coenzyme A reductase (HMGR) is a rate-limiting enzyme, which catalyzes HMG-CoA to form MVA (Asadollahi et al., 2010); In MEP pathway, 1-deoxy-D-xyulose-5-phosphate synthase (DXS) is the rate-limiting enzyme, which catalyzes the formation of branched MEP using 1-deoxy-D-xyulose-5-phosphate (DXP) (Bergman et al., 2019). IPP and DMAPP are generated by the MEP pathway or MVA pathway, then they are catalyzed by GPPS (geranyl diphosphate synthase) to form geranyl diphosphate (GPP) with C10 backbone. Diverse terpene synthases (TPSs) catalyze the production of different monoterpenoids using the acyclic monoterpene precursor of GPP (Liao et al., 2016).
Some monoterpenoids exhibit significant toxicity to bacteria by interfering with bacterial cell membranes. Besides, the accumulation of terpenoids precursors (such as IPP) has a negative impact on bacterial growth (Paramasivan and Muttur, 2017). Production of monoterpenoids often needs to express cytochrome P450 genes, which is not easy to achieve in bacteria. In view of these, S. cerevisiae is becoming an attractive host for monoterpenoid bio-production, due to its robustness, well-studied genetic background, applicability to industrial bioprocesses, and the possibility for terpene scaffold decoration by the functional expression of cytochrome P450 enzymes (Zhang et al., 2008). In particular, S. cerevisiae is classified as generally regarded as safe (GRAS) by the U.S. Food and Drug Administration (FDA) (Nevoigt, 2008). However, compared with plant cells, S. cerevisiae lacks monoterpene synthetase, so it has no ability to synthesize monoterpenoids directly.
In order to realize the heterologous expression of target monoterpenoids in S. cerevisiae, exogenous plant monoterpene synthetases need to be introduced (Figure 1). Dudareva et al. (1996) identified one plant monoterpene synthetase gene responsible for the synthesis of terpene alcohol synthase. Chen et al. (2010) isolated and identified two monoterpene synthetases of AaLS1p and ApLS1p, from Actinidia argute and Actinidia polygama, respectively, which can synthesize (S)-linalool. Oswald et al. (2007) co-expressed linalool synthase from Clarkia breweri and geraniol synthetase from Ocimum basilicum in S. cerevisiae, and these enzymes improved the biosynthetic ability of monoterpene linalool and geraniol in S. cerevisiae. Herrero et al. (2008) successfully introduced linalool biosynthetic pathway to S. cerevisiae, and linalool was effectively produced in S. cerevisiae. These studies showed that S. cerevisiae has great potential to act as a cell factory for monoterpenoids synthesis.
Recently, transcriptomic analyses of the mint adenoids successfully identified the key enzymes of menthol biosynthetic pathway, further cloning and expression verified the biological functions of these key enzymes in the GPP to (−)-menthol pathway (Figure 1 and Supplementary Figure 1; Vining et al., 2017). Different chiral terpenoids often have different biological activities (Hajagos-Tóth et al., 2015), and the key enzymes involved in different terpenoid biosynthetic pathway need to be identified. Therefore, global omics analyses of different monoterpenoid producing plants will give insights into key enzymes for diverse monoterpenoid biosynthesis using engineered microbial cell factories (Wei et al., 2019).
Applications of Protein Engineering, Metabolic Engineering and Structure Biology for Monoterpenoid Production in S. cerevisiae
Although S. cerevisiae has been proven to be able to synthesize monoterpenoids efficiently, the metabolic flux of MVA pathway in S. cerevisiae is still low (Ignea et al., 2019). The metabolic regulation mechanism of the MVA pathway is complex, which greatly affects the final production of monoterpenoids. In order to increase the metabolic flux of the MVA pathway, several important metabolic rate-limiting/key enzymes have been identified and optimized.
3-hydroxy-3-methyl glutaryl coenzyme A reductase is a rate-limiting enzyme in monoterpenoid synthesis, which catalyzes HMG-CoA to generate MVA irreversibly (Figure 1). To relieve the intermediate product inhibition and accumulate of end products, the N-terminal membrane of HMGR was anchored and the C-terminal of HMGR separately (tHMGR) was expressed in S. cerevisiae, which increased the final production of target monoterpenoid (Rico et al., 2010). Overexpression of tHMGR and other genes in S. cerevisiae lead to 22.49 mg/L limonene (Wu et al., 2018). HMGR2 is the predominant isoenzyme that catalyzes HMG-CoA reduction under hypoxia condition (Mantzouridou and Tsimidou, 2010), an engineered stabilized mutant HMGR2 (K6R) successfully improved the production of terpenes (Supplementary Figure 2; Ignea et al., 2012, 2014). These protein engineering studies suggested that HMGR had a significant effect on target monoterpenoids production.
Isoprene pyrophosphate isomerase (IDI1) controls the first step of the monoterpenoid biosynthesis in the conversion process from IPP to DMAPP, which can adjust the proportion of IPP/DMAPP (Figure 1; Cao et al., 2016). Regulating the expression of IDI1 successfully increased the yield of monoterpenoid. Further overexpression of IDI1 gene in engineered S. cerevisiae increased sabinene production by 300% (Ignea et al., 2014). These examples indicate that overexpression of IDI1 in S. cerevisiae could increase the production of desirable monoterpenoid.
The committed step in monoterpenoid biosynthesis involves the conversion of the acyclic isoprenoid diphosphate precursor to cyclic hydrocarbon product catalyzed by limonene synthase (LS) (Figure 1 and Supplementary Figure 1). Limonene is well known for its olfactory characteristics and antibacterial activity, which is often added to soaps or detergents (Jongedijk et al., 2016). Limonene has an unsaturated ring structure, which can be oxidized to menthol, perillyl alcohol and other natural products (Alonso-Gutierrez et al., 2013). Expression of a (−)- limonene synthase from Perilla frutescens and a (+)-limonene synthase from Citrus sinensis resulted in the production of 0.028 mg/l (+)-limonene and 0.060 mg/l (−)-limonene in the engineered yeast cell factory (Jongedijk et al., 2014). The crystal structure of (−)-limonene synthase [(−)-LS] from Mentha spicata was determined at 2.7 Å resolution. The structural and biochemical results show that (−)-LS shares many hallmark features and catalytic characteristic with plant monoterpene synthases, including an all-α-helical domain secondary structure, a two-domain architecture with a catalytic C-terminal domain, an N-terminal domain of unknown function, and conserved divalent metal ion binding residues in the active site (Figure 2A; Hyatt et al., 2007).
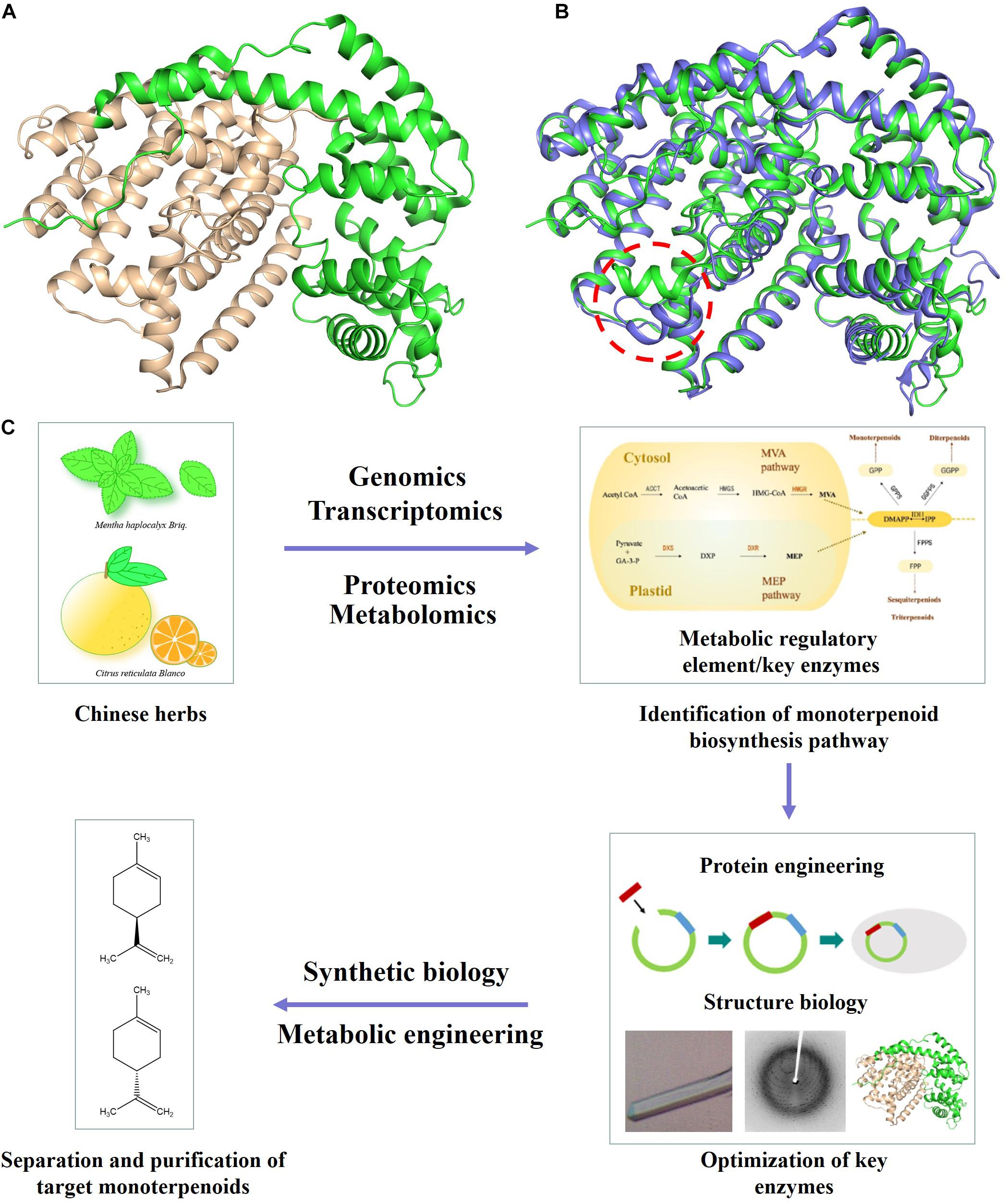
Figure 2. Application of protein engineering and structural biology based approaches in yeast monoterpenoid production. (A) Structure of (–)-LS (PDB ID 2ONG). N-terminal and C-terminal domain are colored in green and wheat, respectively. (B) Comparison of the structure of (–)-LS (colored in green) and (+)-LS (colored in blue). (C) Increasing the production of target monoterpenoid through optimizing important metabolic nodes/key enzymes by enzyme engineering or structure biology.
(+)-Limonene is an abundant monoterpene in the essential oils of most citrus fruit, which is commonly used as an industrial and household solvent. In particular, the citrus smell enables its use in the fragrance and flavoring industries. The (+)-LS from Citrus sinensis was purified, and its crystal structure was further determined. The structural comparison of (+)-LS and (−)-LS showed that the conformation of short α1 helix played critical roles in determining (+)-LS or (−)-LS enantiomer. The structural and catalytic mechanism studies of (+)-LS and (−)-LS provided theoretical basis and new options for designing and achieving (+) or (−)-limonene rationally (Figure 2B; Morehouse et al., 2017; Ren et al., 2020). Based on the LS crystal structures and the proposed catalytic mechanism, a triple mutant (S454G, C457V, M458I) of Mentha spicata (−)-LS was successfully generated, which showed a new catalytic activity of producing more complex bicyclic monoterpenes (Supplementary Figure 2; Leferink et al., 2018).
Farnesyl pyrophosphate synthase (FPPS) condenses one molecule of DMAPP and one molecule of IPP to form GPP, then condenses GPP with one molecule of IPP to form FPP in S. cerevisiae (Figure 1). It is reported that FPPS of S. cerevisiae is encoded by the gene ERG20, which is responsible for the biosynthesis of GPP and FPP. GPP is usually closely bound to the catalytic pocket of FPPS and results in the low content of free GPP (Dai et al., 2013). Therefore, the precursor GPP for monoterpenoid synthesis was insufficient, which greatly restricted the biosynthesis of monoterpenoids. In addition, S. cerevisiae also lacks specific and efficient GPP synthase (Herrero et al., 2008). Sequence alignment of FPPS from different organisms showed that there were two ASP rich sequences in the conserved region of this enzyme. The first ASP rich sequence is DDXXD or DDXXXXD, which could bind DMAPP, GPP, and FPP in catalytic reaction. The second ASP rich sequence is DDXXD, which is mainly responsible for binding IPP. Previous studies showed that site directed mutagenesis in the conservative region of FPPS can cause GPP released from the binding site of FPPS, which increased the intracellular free GPP content (Supplementary Figure 2). By mutating the catalytic residue K197 of yeast farnesyl diphosphate synthase erg20p and expressing heterologous geraniol synthetase gene in engineered S. cerevisiae, the yield of geraniol increased significantly, suggesting that the regulation of ERG20 gene can increase the GPP amount and balance GPP and FPP ratio for geraniol production (Fischer et al., 2011). Sabinene is seen as a potential component for the next generation of aircraft fuels (Rude and Schirmer, 2009). Ignea et al. (2014) identified that erg20p is an rate-limiting enzyme for monoterpene production, and integrating erg20p with a geranyl diphosphate synthase increased sabinene titer (17.5 mg/L). Compared with the canonical GPP-based monoterpenoid biosynthetic pathway, a synthetic orthogonal monoterpenoid pathway using neryl diphosphate as substrate was designed, and further dynamic regulation of some engineered enzymes in the synthetic monoterpenoid improved monoterpenoid production by seven-fold (Ignea et al., 2019).
The protein engineering and structural studies of key enzymes involved in monoterpenoid biosynthetic pathway provided new options for efficient monoterpenoid biosynthesis in S. cerevisiae (Figure 2C and Supplementary Figure 2).
Conclusion and Future Perspective
Plant-derived monoterpenoids are widely used in daily life, and the demand is increasing. Microbial biosynthesis of monoterpenoid provides a new feasible strategy for the monoterpenoid production. S. cerevisiae might be an ideal microbial cell factory for monoterpenoid biosynthesis. With the help of omics technologies, systems biology, structural biology and protein engineering, it will further lead to the identification of highly efficient monoterpenoid biosynthetic enzymes, which can help to enable high yield of monoterpenoid in S. cerevisiae.
Author Contributions
QG, LW, and MZ contributed to figures and manuscript draft preparations. YW and WL wrote and revised the manuscript. WL conceived the project. All authors approved it for publication.
Funding
This work was supported in part by the National Natural Science Foundation of China (Grant Nos. 81903526, 81991523, and 82072240 to WL and NO. 31800079 for YW), Fok Ying Tung Education Foundation, Natural Science Foundation of Jiangsu Province of China (Grant No. BK20190798 to WL), The Open Project of State Key Laboratory of Natural Medicines (No. SKLNMKF202004 to WL), and Jiangsu Specially-Appointed Professor Talent Program to WL.
Conflict of Interest
The authors declare that the research was conducted in the absence of any commercial or financial relationships that could be construed as a potential conflict of interest.
Supplementary Material
The Supplementary Material for this article can be found online at: https://www.frontiersin.org/articles/10.3389/fbioe.2020.609800/full#supplementary-material
References
Abbas, F., Ke, Y., Yu, R., Yue, Y., Amanullah, S., Jahangir, M. M., et al. (2017). Volatile terpenoids: multiple functions, biosynthesis, modulation and manipulation by genetic engineering. Planta 246, 803–816. doi: 10.1007/s00425-017-2749-x
Alonso-Gutierrez, J., Chan, R., Batth, T. S., Adams, P. D., Keasling, J. D., Petzold, C. J., et al. (2013). Metabolic engineering of Escherichia coli for limonene and perillyl alcohol production. Metab. Eng. 19, 33–41. doi: 10.1016/j.ymben.2013.05.004
Asadollahi, M. A., Maury, J., Schalk, M., Clark, A., and Nielsen, J. (2010). Enhancement of farnesyl diphosphate pool as direct precursor of sesquiterpenes through metabolic engineering of the mevalonate pathway in Saccharomyces cerevisiae. Biotechnol. Bioeng. 106, 86–96. doi: 10.1002/bit.22668
Ashour, M. L., Wink, M., and Gershenzon, J. (2010). Biochemistry of Terpenoids: monoterpenes, Sesquiterpenes and Diterpenes. Annu. Plant Rev. Biochem. Plant Sec. Metab. 40, 259–303.
Bergman, M. E., Davis, B., and Phillips, M. A. (2019). Medically useful plant terpenoids: biosynthesis, occurrence, and mechanism of action. Molecules 24:3961. doi: 10.3390/molecules24213961
Božović, M., and Ragno, R. (2017). Calamintha nepeta (L.) savi and its main essential oil constituent pulegone: biological activities and chemistry. Molecules 22:290. doi: 10.3390/molecules22020290
Cao, X., Lv, Y.-B., Chen, J., Imanaka, T., Wei, L.-J., and Hua, Q. (2016). Metabolic engineering of oleaginous yeast Yarrowia lipolytica for limonene overproduction. Biotechnol. Biofuels 9:214. doi: 10.1186/s13068-016-0626-7
Caputi, L., and Aprea, E. (2011). Use of terpenoids as natural flavouring compounds in food industry. Recent Patents Food Nutr. Agricult. 3, 9–16. doi: 10.2174/2212798411103010009
Chandran, S., Kealey, J., and Reeves, C. (2011). Microbial production of isoprenoids. Process Biochem. 46, 1703–1710. doi: 10.1016/j.procbio.2011.05.012
Chen, X., Yauk, Y.-K., Nieuwenhuizen, N. J., Matich, A. J., Wang, M. Y., Perez, R. L., et al. (2010). Characterisation of an (S)-linalool synthase from kiwifruit (Actinidia arguta) that catalyses the first committed step in the production of floral lilac compounds. Funct. Plant Biol. 37:232. doi: 10.1071/FP09179
Christianson, D. W. (2008). Unearthing the roots of the terpenome. Curr. Opin. Chem. Biol. 12, 141–150. doi: 10.1016/j.cbpa.2007.12.008
Costa-Rodrigues, J., Pinho, O., and Monteiro, P. R. R. (2018). Can lycopene be considered an effective protection against cardiovascular disease? Food Chem. 245, 1148–1153. doi: 10.1016/j.foodchem.2017.11.055
Dai, Z., Liu, Y., Zhang, X., Shi, M., Wang, B., Wang, D., et al. (2013). Metabolic engineering of Saccharomyces cerevisiae for production of ginsenosides. Metab. Eng. 20, 146–156. doi: 10.1016/j.ymben.2013.10.004
Dudareva, N., Cseke, L., Blanc, V. M., and Pichersky, E. (1996). Evolution of floral scent in Clarkia: novel patterns of S-linalool synthase gene expression in the C. breweri flower. Plant Cell 8, 1137–1148. doi: 10.1105/tpc.8.7.1137
Fischer, M. J. C., Meyer, S., Claudel, P., Bergdoll, M., and Karst, F. (2011). Metabolic engineering of monoterpene synthesis in yeast. Biotechnol. Bioeng. 108, 1833–1892. doi: 10.1002/bit.23129
Fu, B., Zhao, J., Li, C., Liu, X., Bao, X., and Hou, J. (2018). Research progresses on monoterpene synthesis in Saccharomyces cerevisiae. Biotechnol. Bull. 34, 60–69.
Goto, T., Takahashi, N., Hirai, S., and Kawada, T. (2010). Various terpenoids derived from herbal and dietary plants function as PPAR modulators and regulate carbohydrate and lipid metabolism. PPAR Res. 2010, 1–9. doi: 10.1155/2010/483958
Guan, R., Wang, M., Guan, Z., Jin, C.-Y., Lin, W., Ji, X.-J., et al. (2020). Metabolic engineering for glycyrrhetinic acid production in Saccharomyces cerevisiae. Front. Bioeng. Biotechnol. 8:588255. doi: 10.3389/fbioe.2020.588255
Hajagos-Tóth, J., Hódi, Á, Seres, A. B., and Gáspár, R. (2015). Effects of d- and l-limonene on the pregnant rat myometrium in vitro. Croat Med. J. 56, 431–438. doi: 10.3325/cmj.2015.56.431
Herrero, Ó, Ramón, D., and Orejas, M. (2008). Engineering the Saccharomyces cerevisiae isoprenoid pathway for de novo production of aromatic monoterpenes in wine. Metab. Eng. 10, 78–86. doi: 10.1016/j.ymben.2007.11.001
Hijaz, F., Nehela, Y., and Killiny, N. (2016). Possible role of plant volatiles in tolerance against huanglongbing in citrus. Plant Signal. Behav. 11:e1138193. doi: 10.1080/15592324.2016.1138193
Hyatt, D. C., Youn, B., Zhao, Y., Santhamma, B., Coates, R. M., Croteau, R. B., et al. (2007). Structure of limonene synthase, a simple model for terpenoid cyclase catalysis. Proc. Natl. Acad. Sci. U.S.A. 104, 5360–5365. doi: 10.1073/pnas.0700915104
Ignea, C., Pontini, M., Maffei, M. E., Makris, A. M., and Kampranis, S. C. (2014). Engineering monoterpene production in yeast using a synthetic dominant negative geranyl diphosphate synthase. ACS Synth. Biol. 3, 298–306. doi: 10.1021/sb400115e
Ignea, C., Raadam, M. H., Motawia, M. S., Makris, A. M., Vickers, C. E., and Kampranis, S. C. (2019). Orthogonal monoterpenoid biosynthesis in yeast constructed on an isomeric substrate. Nat. Commun. 10:3799. doi: 10.1038/s41467-019-11290-x
Ignea, C., Trikka, F. A., Kourtzelis, I., Argiriou, A., Kanellis, A. K., Kampranis, S. C., et al. (2012). Positive genetic interactors of HMG2 identify a new set of genetic perturbations for improving sesquiterpene production in Saccharomyces cerevisiae. Microb. Cell Fact. 11:162. doi: 10.1186/1475-2859-11-2
Jongedijk, E., Cankar, K., Buchhaupt, M., Schrader, J., Bouwmeester, H., and Beekwilder, J. (2016). Biotechnological production of limonene in microorganisms. Appl. Microbiol. Biotechnol. 100, 2927–2938. doi: 10.1007/s00253-016-7337-7
Jongedijk, E., Cankar, K., Ranzijn, J., van der Krol, S., Bouwmeester, H., and Beekwilder, J. (2014). Capturing of the monoterpene olefin limonene produced in Saccharomyces cerevisiae: limonene from yeast headspace. Yeast 32, 159–171. doi: 10.1002/yea.3038
Kai, G., Zhou, W., Wang, Y., Hua, Q., and Shi, M. (2018). Research progress in terms of the biosynthesis and regulation of terpenoids from medicinal plants. Sci. Sin. Vitae 48, 352–364. doi: 10.1360/N052017-00194
Kowalska, A., and Kalinowska-Lis, U. (2019). 18 β -Glycyrrhetinic acid: its core biological properties and dermatological applications. Int. J. Cosmet. Sci. 41, 325–331. doi: 10.1111/ics.12548
Laule, O., Furholz, A., Chang, H.-S., Zhu, T., Wang, X., Heifetz, P. B., et al. (2003). Crosstalk between cytosolic and plastidial pathways of isoprenoid biosynthesis in Arabidopsis thaliana. Proc. Natl. Acad. Sci. U.S.A. 100, 6866–6871. doi: 10.1073/pnas.1031755100
Leferink, N. G. H., Ranaghan, K. E., Karuppiah, V., Currin, A., van der Kamp, M. W., Mulholland, A. J., et al. (2018). Experiment and simulation reveal how mutations in functional plasticity regions guide plant monoterpene synthase product outcome. ACS Catal. 8, 3780–3791. doi: 10.1021/acscatal.8b00692
Li, J., Cai, Q., and Wu, J. (2020). Research progresses on the synthetic biology of terpenes in Saccharomyces cerevisiae. Biotechnol. Bull. 36, 1–9.
Li, X., Huang, J., Shi, H., Xu, S., and Li, Z. (2012). Comparison Study on Extraction Methods of Volatile Components from Schizonepeta tenuifolia Briq. J. Anhui Agri. 40, 792–794. doi: 10.13989/j.cnki.0517-6611.2012.02.086
Liao, P., Hemmerlin, A., Bach, T. J., and Chye, M.-L. (2016). The potential of the mevalonate pathway for enhanced isoprenoid production. Biotechnol. Adv. 34, 697–713. doi: 10.1016/j.biotechadv.2016.03.005
Mahizan, N. A., Yang, S.-K., Moo, C.-L., Song, A. A.-L., Chong, C.-M., Chong, C.-W., et al. (2019). Terpene derivatives as a potential agent against antimicrobial resistance (AMR) pathogens. Molecules 24:2631. doi: 10.3390/molecules24142631
Mantzouridou, F., and Tsimidou, M. Z. (2010). Observations on squalene accumulation in Saccharomyces cerevisiae due to the manipulation of HMG2 and ERG6: observations on squalene accumulation capacity in yeast. FEMS Yeast Res. 10, 699–707. doi: 10.1111/j.1567-1364.2010.00645.x
Morehouse, B. R., Kumar, R. P., Matos, J. O., Olsen, S. N., Entova, S., and Oprian, D. D. (2017). Functional and structural characterization of a (+)-limonene synthase from Citrus sinensis. Biochemistry 56, 1706–1715. doi: 10.1021/acs.biochem.7b00143
Nevoigt, E. (2008). Progress in metabolic engineering of Saccharomyces cerevisiae. Microbiol. Mol. Biol. Rev. 72, 379–412. doi: 10.1128/MMBR.00025-7
Oliveira, G. R., Vargas-Sanchez, P. K., Fernandes, R. R., Ricoldi, M. S. T., Semeghini, M. S., Pitol, D. L., et al. (2019). Lycopene influences osteoblast functional activity and prevents femur bone loss in female rats submitted to an experimental model of osteoporosis. J. Bone Miner Metab. 37, 658–667. doi: 10.1007/s00774-018-0970-8
Oswald, M., Fischer, M., Dirninger, N., and Karst, F. (2007). Monoterpenoid biosynthesis in Saccharomyces cerevisiae. FEMS Yeast Res. 7, 413–421. doi: 10.1111/j.1567-1364.2006.00172.x
Paddon, C. J., Westfall, P. J., Pitera, D. J., Benjamin, K., Fisher, K., McPhee, D., et al. (2013). High-level semi-synthetic production of the potent antimalarial artemisinin. Nature 496, 528–532. doi: 10.1038/nature12051
Paramasivan, K., and Muttur, S. (2017). Progress in terpene synthesis strategies through engineering of Saccharomyces cerevisiae. Crit. Rev. Biotechnol. 37, 974–989. doi: 10.1080/07388551.2017.1299679
Rajput, J. D., Bagul, S. D., Pete, U. D., Zade, C. M., Padhye, S. B., and Bendre, R. S. (2018). Perspectives on medicinal properties of natural phenolic monoterpenoids and their hybrids. Mol. Div. 22, 225–245. doi: 10.1007/s11030-017-9787-y
Ren, Y., Liu, S., Jin, G., Yang, X., and Zhou, Y. J. (2020). Microbial production of limonene and its derivatives: achievements and perspectives. Biotechnol. Adv. 44:107628. doi: 10.1016/j.biotechadv.2020.107628
Rico, J., Pardo, E., and Orejas, M. (2010). Enhanced production of a plant monoterpene by overexpression of the 3-Hydroxy-3-Methylglutaryl Coenzyme A reductase catalytic domain in Saccharomyces cerevisiae. Appl. Environ. Microbiol. 76, 6449–6454. doi: 10.1128/AEM.02987-9
Rude, M. A., and Schirmer, A. (2009). New microbial fuels: a biotech perspective. Curr. Opin. Microbiol. 12, 274–281. doi: 10.1016/j.mib.2009.04.004
Sun, L., Li, S., Wang, F., and Xin, F. (2017). Research Progresses in the Synthetic Biology of Terpenoids. Biotechnol. Bull. 33, 64–75. doi: 10.13560/j.cnki.biotech.bull.1985.2017.01.007
Talman, A. M., Clain, J., Duval, R., Ménard, R., and Ariey, F. (2019). Artemisinin bioactivity and resistance in malaria parasites. Trends Parasitol. 35, 953–963. doi: 10.1016/j.pt.2019.09.005
Trombetta, D., Castelli, F., Sarpietro, M. G., Venuti, V., Cristani, M., Daniele, C., et al. (2005). Mechanisms of antibacterial action of three monoterpenes. Antimicrob Agents Chemother. 49, 2474–2478. doi: 10.1128/AAC.49.6.2474-2478.2005
Vining, K. J., Johnson, S. R., Ahkami, A., Lange, I., Parrish, A. N., Trapp, S. C., et al. (2017). Draft genome sequence of mentha longifolia and development of resources for mint cultivar improvement. Mol. Plant 10, 323–339. doi: 10.1016/j.molp.2016.10.018
Vranová, E., Coman, D., and Gruissem, W. (2013). Network analysis of the MVA and MEP Pathways for Isoprenoid Synthesis. Annu. Rev. Plant Biol. 64, 665–700. doi: 10.1146/annurev-arplant-050312-120116
Wang, C. (2013). Engineered heterologous FPP synthases-mediated Z,E-FPP synthesis in E. coli. Metab. Eng. 18, 53–59. doi: 10.1016/j.ymben.2013.04.002
Wang, F., Wen, T., Sang, W., Xu, F., and Zeng, N. (2017). Chemical constituents in essential oils of Schizonepeta tenuifolia Briq. and their pharmacological activities. Central South Pharm. 15, 312–318. doi: 10.7539/j.issn.1672-2981.2017.03.014
Wang, J., Wang, Y., and Meng, H. (2015). Research advances in synthetic biology of terpenoids. Sci. Sin. Vitae 45, 1040–1050. doi: 10.1360/N052015-00050
Wang, M., Wei, Y., Ji, B., and Nielsen, J. (2020). Advances in metabolic engineering of saccharomyces cerevisiae for cocoa butter equivalent production. Front. Bioeng. Biotechnol. 8:594081. doi: 10.3389/fbioe.2020.594081
Wang, P., Wei, Y., Fan, Y., Liu, Q., Wei, W., Yang, C., et al. (2015). Production of bioactive ginsenosides Rh2 and Rg3 by metabolically engineered yeasts. Metab. Eng. 29, 97–105. doi: 10.1016/j.ymben.2015.03.003
Weaver, B. A. (2014). How Taxol/paclitaxel kills cancer cells. Mol. Biol. Cell 25, 2677–2681. doi: 10.1091/mbc.e14-04-0916
Wei, W., Wang, P., Wei, Y., Liu, Q., Yang, C., Zhao, G., et al. (2015). Characterization of Panax ginseng UDP-glycosyltransferases catalyzing protopanaxatriol and biosyntheses of bioactive ginsenosides F1 and Rh1 in metabolically engineered yeasts. Mol. Plant 8, 1412–1424. doi: 10.1016/j.molp.2015.05.010
Wei, Y., Ji, B., Siewers, V., Xu, D., Halkier, B. A., and Nielsen, J. (2019). Identification of genes involved in shea butter biosynthesis from Vitellaria paradoxa fruits through transcriptomics and functional heterologous expression. Appl. Microbiol. Biotechnol. 103, 3727–3736. doi: 10.1007/s00253-019-09720-3
Wen, Z., Liu, L., Li, X., Zheng, Y., and Ma, D. (2019). Simultaneous determination of the contents of 8 components in Schizonepeta tenuifolia Briq in different producing areas by HPLC. J. Chin. Med. Mater. 423, 139–143.
Wojtunik-Kulesza, K. A., Kasprzak, K., Oniszczuk, T., and Oniszczuk, A. (2019). Natural monoterpenes: much more than only a scent. Chem. Biodiv. 21:e1900434.
Wu, T., Li, S., Zhang, B., Bi, C., and Zhang, X. (2018). Engineering Saccharomyces cerevisiae for the production of the valuable monoterpene ester geranyl acetate. Microb. Cell Fact. 17:85. doi: 10.1186/s12934-018-0930-y
Yan, X., Fan, Y., Wei, W., Wang, P., Liu, Q., Wei, Y., et al. (2014). Production of bioactive ginsenoside compound K in metabolically engineered yeast. Cell Res. 24, 770–773. doi: 10.1038/cr.2014.28
Yang, C., Li, C., Wei, W., Wei, Y., Liu, Q., Zhao, G., et al. (2020). The unprecedented diversity of UGT94-family UDP-glycosyltransferases in Panax plants and their contribution to ginsenoside biosynthesis. Sci. Rep. 10:15394. doi: 10.1038/s41598-020-72278-y
Zebec, Z., Wilkes, J., Jervis, A. J., Scrutton, N. S., Takano, E., and Breitling, R. (2016). Towards synthesis of monoterpenes and derivatives using synthetic biology. Curr. Opin. Chem. Biol. 34, 37–43. doi: 10.1016/j.cbpa.2016.06.002
Zhang, H., Liu, Q., Cao, Y., Feng, X., Zheng, Y., Zou, H., et al. (2014). Microbial production of sabinene—a new terpene-based precursor of advanced biofuel. Microb. Cell Fact. 13:20. doi: 10.1186/1475-2859-13-0
Zhang, H., Wang, Y., and Pfeifer, B. A. (2008). Bacterial hosts for natural product production. Mol. Pharm. 5, 212–225. doi: 10.1021/mp7001329
Keywords: monoterpenoids, biosynthetic pathway, Saccharomyces cerevisiae, protein engineering, structure biology, synthetic biology
Citation: Gao Q, Wang L, Zhang M, Wei Y and Lin W (2020) Recent Advances on Feasible Strategies for Monoterpenoid Production in Saccharomyces cerevisiae. Front. Bioeng. Biotechnol. 8:609800. doi: 10.3389/fbioe.2020.609800
Received: 24 September 2020; Accepted: 09 November 2020;
Published: 01 December 2020.
Edited by:
Evangelos Topakas, National Technical University of Athens, GreeceReviewed by:
Antonios Michael Makris, Centre for Research and Technology Hellas (CERTH), GreeceFarshad Darvishi, Alzahra University, Iran
Copyright © 2020 Gao, Wang, Zhang, Wei and Lin. This is an open-access article distributed under the terms of the Creative Commons Attribution License (CC BY). The use, distribution or reproduction in other forums is permitted, provided the original author(s) and the copyright owner(s) are credited and that the original publication in this journal is cited, in accordance with accepted academic practice. No use, distribution or reproduction is permitted which does not comply with these terms.
*Correspondence: Wei Lin, d2VpbGluQG5qdWNtLmVkdS5jbg==; Yongjun Wei, eW9uZ2p1bndlaUB6enUuZWR1LmNu
†These authors have contributed equally to this work