- 1School of Life Sciences, Shanghai University, Shanghai, China
- 2Department of Radiology, Union Hospital, Tongji Medical College, Huazhong University of Science and Technology, Wuhan, China
- 3Department of Radiology, The Affiliated Hospital of Qingdao University, Qingdao, China
Increasing awareness of chronic pain due to both injury and disease have encouraged drug companies and pharmaceutical researchers alike to design and fabricate better, more specific drugs for pain relief. However, overuse of clinically available pain medication has caused a multitude of negative repercussions, including drug tolerance, addiction, and other severe side effects, which can prolong suffering and reduce pain mediation. Applications of nanotechnology to the field of drug delivery has sought to enhance the treatment efficiency, lower side effects, and mitigate the formation of tolerance. The use of nanomaterials has several advantages for chronic pain relief, such as controlled release, prolonged circulation time, and limited side effects. With the development of nanotechnology, strategies for chronic pain relief have also bourgeoned utilizing a variety of nanomaterials and targeting surface modifications. In addition to using these materials as carriers for drug delivery, nanomaterials can also be designed to have inherent properties that relieve chronic pain. This minireview covers the current status of designed nanomaterials for pain relief and provides a discussion of future considerations for nanotechnology designed for relieving chronic pain.
Introduction
Chronic pain is characterized by enhanced responses to different external stimuli, also known as hyperalgesia, and is induced by inflammation following injury (Ji et al., 2014). When damage or inflammation occurs, mediators such as prostaglandins, cytokines, chemokines, neuropeptides, and nerve growth factor (NGF), are released (Zhang and An, 2007). These mediators maintain pain signaling that starts in the periphery and results in both peripheral and central sensitization, ultimately contributing to chronic pain. From the tremendous efforts of researchers in the fields of neurology and signaling, we have a deeper understanding of the mechanisms that drive pain. Additionally, chronic pain from a variety of sources, including injuries and disease, has promoted the development of targeted therapies (Mantyh et al., 2002; Binder, 2007; Said, 2007; Francis et al., 2008).
Current methods of pain relief and enhanced quality of life predominantly rely on surgery (Ducic et al., 2008), medication (Volkow et al., 2018), physical therapy (Ambrose and Golightly, 2015), and psychological therapy (De Williams et al., 2012). The use of medications, including opioid drugs (Sullivan and Howe, 2013; Ballantyne and Sullivan, 2015) and non-opioid drugs (Kaye et al., 2018), has increased significantly over the last several decades. Extensive use of medication has been associated with severe side effects, including drug addiction (Pohl and Smith, 2012), tolerance (Zhuo, 2016), abuse (Vowles et al., 2015; Volkow and McLellan, 2016), and even death. These significant drawbacks of clinically available drugs have shifted the focus of drug development on improving the targeting of drugs, reducing side effects, and prolonging the release of the active compounds (Gao and Ji, 2010). However, due to their rapid metabolism, these current formulations are challenging to manufacture reproducibly, and the required dosing can cause poorly tolerated physical side effects.
The integration of pharmacological sciences with nanotechnology has been a key step toward creating more effective drugs for chronic pain with fewer negative implications (Feynman, 1960). With the advent of nanotechnology, the field of drug delivery has undergone extensive development resulting in several nanomaterials being approved for clinical use (Ventola, 2017). Compared with traditional formulations, nanomaterials can be efficiently loaded with drugs (Farokhzad and Langer, 2009), protect the stability of protein-based drugs (Xu et al., 2019), sustain controlled release with prolonged circulation time (Blanco et al., 2015), and are also designed to be highly biocompatible (Nyström and Fadeel, 2012). In the field of chronic pain relief, nanomaterials have been developed explicitly for the targeted delivery and release of pain medication. Inspired by the first Food and Drug Administration (FDA)-approved nanodrug Doxil (Barenholz, 2012), nanotechnology is being applied to many biomedical applications, but with a limited focus on chronic pain relief. In this minireview, we cover the development of medications for chronic pain relief that employ nanotechnology, including targeted and non-targeted nanomaterials, and provide perspective for future applications of nanotechnology in pain relief (Figure 1).
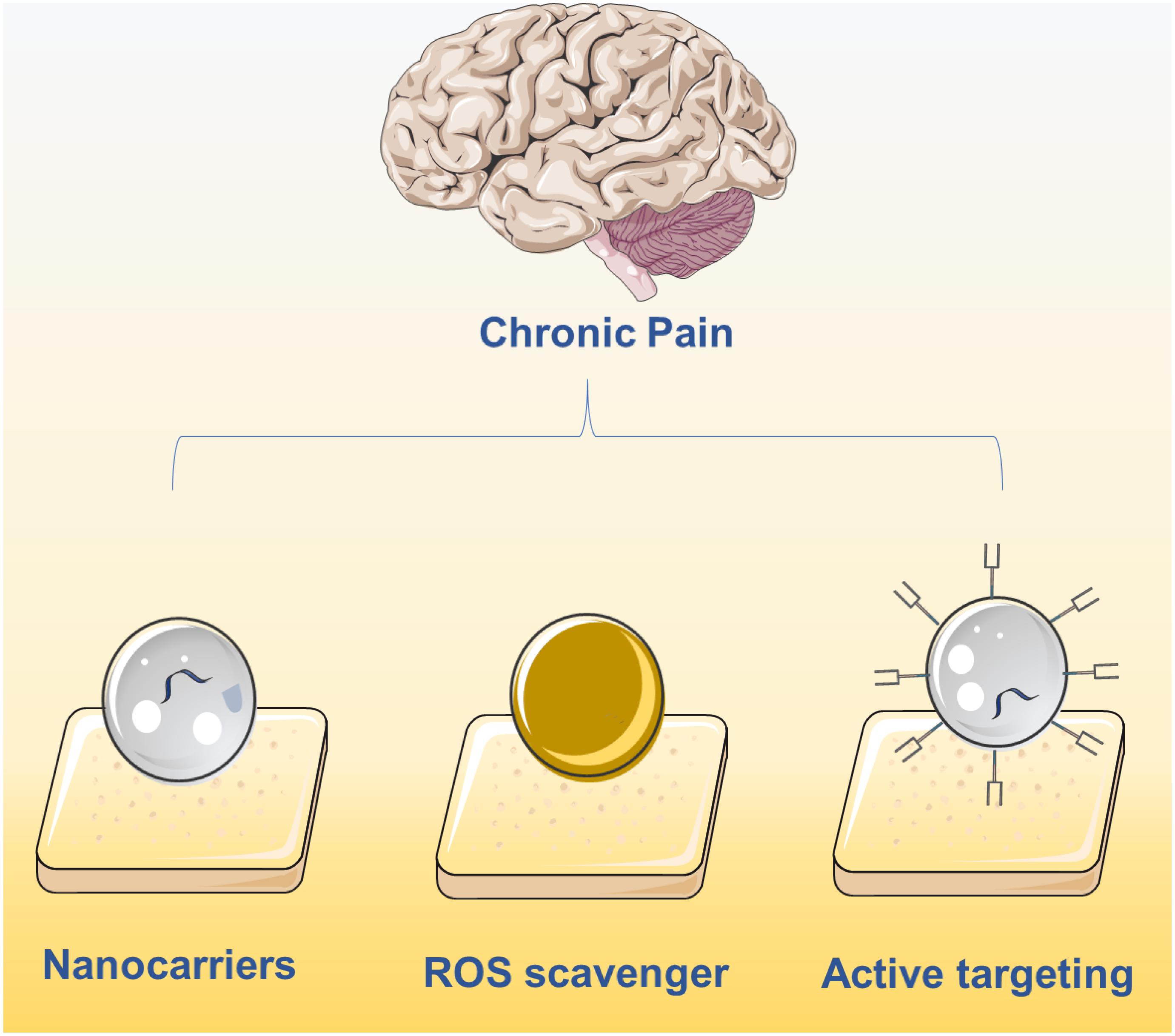
Figure 1. Current main strategies on chronic pain relief using nanotechnology including the delivery of drugs using nanocarriers, active targeting nanocarriers and ROS clearance via nanomaterials.
Non-Targeted Nanomaterials for Pain Relief
Nanomaterials can be designated as organic, inorganic, and metal-organic nanomaterials based on their components. All three categories of nanomaterials have been used as controlled release delivery systems to minimize side effects and promote treatment efficacy for pain medication. Nanomaterials can be used to encapsulate both free molecules and protein-based drugs to increase blood circulation time with sustained, controlled release, resulting in long-lasting pain relief with minimal side effects. In this section, we will present the development of organic and inorganic non-targeted nanomaterials, which have been broadly applied to several pain relief drugs.
When introducing nanomaterials into a clinical application, a major preliminary concern is the biocompatibility of the proposed nanomaterial. Consequently, nanomaterials that have already been approved by the FDA are generally the first to be considered by researchers. FDA approved nanomaterials are mainly organic in nature such as liposomes (Koudelka and Turánek, 2012), PLGA (Makadia and Siegel, 2011), and other carbon based polymer nanomaterials (Palazzolo et al., 2018). Several inorganic nanomaterials have also been approved, but remain in the minority (Urie et al., 2018).
Liposomes are especially attractive since they are derived from cellular-like lipids, making them extremely biocompatible, and are relatively well studied. A number of liposome formulations have been the focus of emerging clinical trials. For example, PEGylated-liposomes have been used to encapsulate and enhance the accumulation of zoledronic acid (ZOL), an inhibitor of the ras-dependent Erk-mediated pathway, for the treatment of neuropathic pain (Caraglia et al., 2013). Caraglia et al. proved that this liposomal based delivery system passed across the blood brain barrier (BBB), promoting its ability to release ZOL for efficient pain mitigation. Likewise, Smith et al. (2006) encapsulated the drug hydromorphone using the liposomes and tested its neuropathic pain relief ability in rats. As expected, liposomal delivery enabled prolonged pain relief with only a single injection.
Although liposomes have many advantages and benefit from established synthesis procedures, the next generation of nanomaterials is focused on a myriad of tunable features including size, surface properties, responsiveness, controlled circulation time, high loading efficiency, and the ability to target specific tissues. Durán-Lobato et al. (2015) compared PEG-modified lipid nanomaterials to chitosan-modified lipid nanomaterials and PLGA nanomaterials for their ability to deliver cannabinoids via oral administration. The three biocompatible nanomaterials varied in their performance, providing design criteria for the development of nanomaterials for pain relief drug delivery.
The overproduction of reactive oxygen species (ROS) at sites of inflammation can result in chronic pain; consequently, nanomaterials that consume ROS are a promising avenue for pain relief (Gwak et al., 2013). Along these lines, Liu et al. (2013) employed fullerol nanomaterials, which are known to consume ROS, to protect inflammatory sites and relieve the pain.
Integrating an FDA-approved nanomaterial PLGA with bupivacaine, Garcia et al. (2011) studied its drug release mechanism and ability to relieve pain. Likewise, Shen et al. (2013) synthesized a new combination of nanomaterial, naocurcumin, composed of PLGA and curcumin to attenuate morphine tolerance. Naocurcumin was orally administrated to mice revealing excellent biocompatibility and ability to mitigate tolerance to morphine. Apart from small molecule drug delivery, biomedicine and nanotechnology have also been focused on the delivery of biomolecules such as Ribonucleic Acid (RNA). Based on the ability of p38 small interfering RNA (p38 siRNA) to assuage neuropathic pain, Shin et al. (2018) used PLGA nanomaterials to encapsulate the p38 siRNA enhancing its stability and slow release for pain alleviation.
In addition to organic nanomaterials, inorganic nanomaterials have also been designed and applied to address chronic pain. Compared with their organic counterparts, inorganic nanomaterials are generally more stable, ensuring prolonged circulation, and can be designed to have more physicochemical properties, such as controlled drug release via stimulation by an external field. Recently, Song et al. (2015) published on a graphene oxide (GO) based nano-system for neuropathic pain relief. Specifically, these GO nanomaterials provided a large surface area to load lidocaine and thalidomide, enhancing the capacity of these drugs to relieve neuropathic pain. In a separate study of inorganic nanomaterials, Wu et al. (2017) demonstrated that ultra-small magnetic iron oxide particles exhibited dose-dependent analgesic effects for the treatment of chronic pain. Using hydroxyapatite nanomaterials, which are biocompatible and have been approved for use in bone-related diseases, Gu et al. (2012) encapsulated and efficiently delivered NR2B-siRNA into mice via intrathecal injection. These nanoparticles exhibited statistical levels of pain mitigation that motivate the future development of materials for siRNA delivery.
Targeted Nanomaterials for Pain Relief
Despite the advantages of using nanomaterials to encapsulate drugs for the alleviation of chronic pain, their limited treatment efficacy has drawn attention to the critical need to develop more promising solutions. Since the causes of chronic pain are variable, the requirements for drug type and dosing differ depending on the treatment intervention. Enhancing the concentration of the drug at the indented site of action is one way to increase the efficacy of the treatment and minimize off target effects. Modification of nanomaterials using targeting substances such as peptides and antibodies can help achieve this site-specific targeting. Additionally, the route of administration is a critical consideration for the use of these targeted materials in their corresponding chronic pain models. For example, exposed sites of chronic pain on the skin can be topically treated using a smear or spray, whereas an injection through the dorsal root ganglion is more suitable for chronic spinal nerve pain. Internal injury or disease may benefit from oral, intranasal, intramuscular, or intravenous injections, depending on the source and location of the pain. In the following section, we will highlight research focused on targeted strategies to promote efficient chronic pain treatment.
Surface modification of nanomaterials is a simple yet effective way to enhance the location-specific absorption of delivered drugs. For example, Hoekman et al. (2014) synthesized opioid fentanyl-encapsulated liposomes with integrin targeting motifs, revealing increased stability, enhanced analgesic ability, and reduced plasma drug exposure after aerosol administration. Likewise, Tosi et al. (2007) designed polyester-based nanoparticles consisting of PLGA and glycosylated heptapeptides for the delivery of the opioid agonist loperamide. Excitingly, these nanoparticles not only crossed the BBB via peptide targeting but also exhibited sustained release of loperamide. Lalani et al. (2015) modified PLGA nanomaterials with lactoferrin and transferrin as the ligands, ensuring brain targeting for better pain relief. Additionally, Patel et al. (2012) showed enhanced tizanidine HCl delivery to the brain using surface-modified thiolated chitosan nanomaterials when they were administered intranasally.
Pain evoked by cancer, as well as some cancer medications, has drawn substantial attention from researchers studying pain relief. To address cancer-induced bone pain, Gdowski et al. (2017) modified PLGA with the bone microenvironment targeting amino-bisphosphonate, to deliver cabazitaxel for efficient pain relief in a bone metastatic prostate cancer model. To mimic the endogenous response in inflammatory sites, Hua et al. designed opioid loaded liposomes with the capacity to target inflammatory environments and release the corresponding drugs for simultaneous pain relief (Hua and Cabot, 2013). Important research described by Ramírez-García et al. (2019) reveals that their novel pH-responsive nanomaterials with the ability to target neurokinin-1 receptors (NK1R) in the endosome display excellent capacity for preventing chronic pain. The accumulation of these pH-responsive nanomaterials in NK1R-containing endosomes resulted in sustained chronic pain relief. To date, targeted nanomaterial design is mostly focused on modification with targeting ligands, but other strategies for active targeting remain largely unexplored, which will be presented in the perspective section.
Discussion and Perspective
The urgent demand for chronic pain relief has highlighted the need for advancements in nanotechnology and pharmaceutical science, attracting attention from scientists in materials science, biomaterials, and chemistry. After decades of progress, the use of nanotechnology for chronic pain relief has been regarded as promising, prompting several clinical trials. However, the development of these smart nanomaterials is still in its infancy, with many unexplored opportunities. This minireview aims to highlight current difficulties in the pharmaceutical field and the advantages of applying nanotechnology to address these shortcomings. Herein, we have detailed some representative examples of organic and inorganic nanomaterials that facilitate controlled release, passive and active targeting, and the utilization of external energy fields. Based on these previous studies, we would like to provide some perspective thoughts on nanomaterial design for chronic pain relief (Figure 2):
(1) Oral administration of drugs poses a series of biological barriers requiring enhanced stability in the stomach, followed by the ability to be absorbed and be effective. Therefore, careful consideration of size, surface charge, and whether to modify the targeted ligands are necessary when designing a nanomaterial for this application. Moreover, non-conventional nanomaterials such as exosomes, cell membrane-derived vesicles (Van Dommelen et al., 2012) which have the targeting ability could also be investigated for oral drug delivery.
(2) Since each drug has its own kinetic profile, it is necessary to design appropriate nanomaterials to achieve a balance between drug release rate and drug action time. Additionally, combining interfering RNA with nanotechnology may prove to be an important strategy for pain mitigation.
(3) To improve therapeutic effects while minimizing side effects, a balance between the drug load and the release rate needs to be achieved. Similarly, improving the targeting efficiency of nanocarriers will reduce off-target effects by minimizing exposure to non-targeted organs during circulation.
(4) The phenomenon of protein coronas (Mahmoudi et al., 2016) has been attributed to the low efficacy of targeted nanomaterials, as serum proteins bind to the surface resulting in masking of the targeting epitopes and rapid clearance by phagocytic cells. Avoiding the formation of protein coronas is a critical avenue of research for the advancement of all nanomaterials for drug delivery. One proven method to mitigate corona formation is to modify the surface of nanomaterials with PEG.
(5) In pharmacology, the rapid development of multi-target drugs (Malek et al., 2015) has greatly improved the therapeutic outcome of chronic pain. Integrating nanotechnology with these enhanced formulations could allow for multi-drug co-delivery or their targeted release multiple sites. These methods have the capability to significantly reduce side effects, improve therapeutic outcomes, and reduce drug tolerization.
(6) Although nanomaterials have experienced rapid developmental growth over the past few decades, there is an urgency to ensure the safety of these materials and establish a unified evaluation standard.
(7) Although theranostic is a popular concept in the field of cancer, treatments for chronic pain treatment lack an intuitive imaging modality to monitor treatment progression and directly visualize therapeutic effects. Luminescent nanomaterials (Park et al., 2009) could be introduced into the field to achieve the integration of diagnosis and treatment, similar to their use in cancer therapeutics.
(8) The energy-conversion properties of inorganic nanomaterials can be employed to treat chronic pain, such as generating electrical signals when stimulated by light, (Tang et al., 2018) subsequently activating or inhibiting ion channels from playing an analgesic role. Additionally, magnetic nanomaterials (Zhang et al., 2016) can also use external magnetic fields to achieve targeted magnetic therapy.
(9) Microneedles (Kaushik et al., 2001) can be applied to the field of analgesia, where nanomaterials are integrated into the front end of the device to greatly reduce the pain caused by injecting drugs to patients.
(10) Inhalation of apposite nanomaterials that encapsulate drugs could be leveraged for chronic pain relief, especially lung-related pain.
As a collaboration between many fields of study, we believe that the application of nanotechnology to address chronic pain has a bright future. We hope that this field will develop steadily, with clinical relevance, to improve the quality of life for patients with chronic pain.
Author Contributions
All the authors contributed to the writing of the manuscript.
Conflict of Interest
The authors declare that the research was conducted in the absence of any commercial or financial relationships that could be construed as a potential conflict of interest.
Acknowledgments
We would like to thank Editage (www.editage.com) for English language editing.
References
Ambrose, K. R., and Golightly, Y. M. (2015). Physical exercise as non-pharmacological treatment of chronic pain: why and when. Best Pract. Res. Clin. Rheumatol. 29, 120–130. doi: 10.1016/j.berh.2015.04.022
Ballantyne, J. C., and Sullivan, M. D. (2015). Intensity of chronic pain—the wrong metric. N. Engl. J. Med. 373, 2098–2099. doi: 10.1056/nejmp1507136
Barenholz, Y. C. (2012). Doxil® —the first FDA-approved nano-drug: lessons learned. J. Control. Release 160, 117–134. doi: 10.1016/j.jconrel.2012.03.020
Binder, A. I. (2007). Cervical spondylosis and neck pain. BMJ 334, 527–531. doi: 10.1136/bmj.39127.608299.80
Blanco, E., Shen, H., and Ferrari, M. (2015). Principles of nanoparticle design for overcoming biological barriers to drug delivery. Nat. Biotechnol. 33:941. doi: 10.1038/nbt.3330
Caraglia, M., Luongo, L., Salzano, G., Zappavigna, S., Marra, M., Guida, F., et al. (2013). Stealth liposomes encapsulating zoledronic acid: a new opportunity to treat neuropathic pain. Mol. Pharm. 10, 1111–1118. doi: 10.1021/mp3006215
De Williams, A. C., Eccleston, C., and Morley, S. (2012). Psychological therapies for the management of chronic pain (excluding headache) in adults. Cochr. Data. Syst. Rev. 11:CD007407.
Ducic, I., Mesbahi, A. N., Attinger, C. E., and Graw, K. (2008). The role of peripheral nerve surgery in the treatment of chronic pain associated with amputation stumps. Plastic Reconst. Surgery 121, 908–914. doi: 10.1097/01.prs.0000299281.57480.77
Durán-Lobato, M., Martín-Banderas, L., Gonçalves, L. M., Fernández-Arévalo, M., and Almeida, A. J. (2015). Comparative study of chitosan-and PEG-coated lipid and PLGA nanoparticles as oral delivery systems for cannabinoids. J. Nanop. Res. 17:61.
Farokhzad, O. C., and Langer, R. (2009). Impact of nanotechnology on drug delivery. ACS Nano 3, 16–20.
Feynman, R. P. (1960). There’s Plenty of Room at the Bottom. Pasadena, CA: California Institute of Technology.
Francis, R., Aspray, T., Hide, G., Sutcliffe, A., and Wilkinson, P. (2008). Back pain in osteoporotic vertebral fractures. Osteopor. Int. 19, 895–903. doi: 10.1007/s00198-007-0530-x
Gao, Y.-J., and Ji, R.-R. (2010). Targeting astrocyte signaling for chronic pain. Neurotherapeutics 7, 482–493. doi: 10.1016/j.nurt.2010.05.016
Garcia, X., Escribano, E., Domenech, J., Queralt, J., and Freixes, J. (2011). In vitro characterization and in vivo analgesic and anti-allodynic activity of PLGA-bupivacaine nanoparticles. J. Nanopar. Res. 13, 2213–2223. doi: 10.1007/s11051-010-9979-1
Gdowski, A. S., Ranjan, A., Sarker, M. R., and Vishwanatha, J. K. (2017). Bone-targeted cabazitaxel nanoparticles for metastatic prostate cancer skeletal lesions and pain. Nanomedicine 12, 2083–2095. doi: 10.2217/nnm-2017-0190
Gu, Y. H., Yan, X. B., Huang, D., Han, R., and Wu, L. X. (2012). NR2B-siRNA Mediated by Hydroxyapatite Nanoparticles Relieves for Malin-Induced Pain of Mice. Pfaffikon: Advanced Materials Research, Trans Tech Publications.
Gwak, Y. S., Hassler, S. E., and Hulsebosch, C. E. (2013). Reactive oxygen species contribute to neuropathic pain and locomotor dysfunction via activation of CamKII in remote segments following spinal cord contusion injury in rats. PAIN® 154, 1699–1708. doi: 10.1016/j.pain.2013.05.018
Hoekman, J. D., Srivastava, P., and Ho, R. J. (2014). Aerosol-stable peptide-coated liposome nanoparticles: A proof-of-concept study with opioid fentanyl in enhancing analgesic effects and reducing plasma drug exposure. J. Pharm. Sci. 103, 2231–2239. doi: 10.1002/jps.24022
Hua, S., and Cabot, P. J. (2013). Targeted nanoparticles that mimic immune cells in pain control inducing analgesic and anti-inflammatory actions: a potential novel treatment of acute and chronic pain conditions. Pain Physician 16, E199–E216.
Ji, R.-R., Xu, Z.-Z., and Gao, Y.-J. (2014). Emerging targets in neuroinflammation-driven chronic pain. Nat. Rev. Drug Dis. 13, 533–548. doi: 10.1038/nrd4334
Kaushik, S., Hord, A. H., Denson, D. D., McAllister, D. V., Smitra, S., Allen, M. G., et al. (2001). Lack of pain associated with microfabricated microneedles. Anesth. Anal. 92, 502–504. doi: 10.1097/00000539-200102000-00041
Kaye, A. D., Cornett, E. M., Hart, B., Patil, S., Pham, A., Spalitta, M., et al. (2018). Novel pharmacological nonopioid therapies in chronic pain. Curr. Pain Head. Rep. 22:31.
Koudelka, Š, and Turánek, J. (2012). Liposomal paclitaxel formulations. J. Control. Release 163, 322–334. doi: 10.1016/j.jconrel.2012.09.006
Lalani, J., Patil, S., Kolate, A., Lalani, R., and Misra, A. (2015). Protein-functionalized PLGA nanoparticles of lamotrigine for neuropathic pain management. AAPS Pharm. Sci.Tech. 16, 413–427. doi: 10.1208/s12249-014-0235-3
Liu, Q., Jin, L., Mahon, B. H., Chordia, M. D., Shen, F. H., and Li, X. (2013). A novel treatment of neuroinflammation against low back pain by soluble fullerol nanoparticles. Spine 38:1443. doi: 10.1097/brs.0b013e31828fc6b7
Mahmoudi, M., Bertrand, N., Zope, H., and Farokhzad, O. C. (2016). Emerging understanding of the protein corona at the nano-bio interfaces. Nano Today 11, 817–832. doi: 10.1016/j.nantod.2016.10.005
Makadia, H. K., and Siegel, S. J. (2011). Poly lactic-co-glycolic acid (PLGA) as biodegradable controlled drug delivery carrier. Polymers 3, 1377–1397. doi: 10.3390/polym3031377
Malek, N., Mrugala, M., Makuch, W., Kolosowska, N., Przewlocka, B., Binkowski, M., et al. (2015). A multi-target approach for pain treatment: dual inhibition of fatty acid amide hydrolase and TRPV1 in a rat model of osteoarthritis. Pain 156, 890–903. doi: 10.1097/j.pain.0000000000000132
Mantyh, P. W., Clohisy, D. R., Koltzenburg, M., and Hunt, S. P. (2002). Molecular mechanisms of cancer pain. Nat. Rev. Cancer 2, 201–209.
Nyström, A. M., and Fadeel, B. (2012). Safety assessment of nanomaterials: implications for nanomedicine. J. Control. Release 161, 403–408. doi: 10.1016/j.jconrel.2012.01.027
Palazzolo, S., Bayda, S., Hadla, M., Caligiuri, I., Corona, G., Toffoli, G., et al. (2018). The clinical translation of organic nanomaterials for cancer therapy: a focus on polymeric nanoparticles, micelles, liposomes and exosomes. Curr. Med. Chem. 25, 4224–4268. doi: 10.2174/0929867324666170830113755
Park, J.-H., Gu, L., Von Maltzahn, G., Ruoslahti, E., Bhatia, S. N., and Sailor, M. J. (2009). Biodegradable luminescent porous silicon nanoparticles for in vivo applications. Nat. Mater. 8, 331–336. doi: 10.1038/nmat2398
Patel, D., Naik, S., and Misra, A. (2012). Improved transnasal transport and brain uptake of tizanidine HCl-loaded thiolated chitosan nanoparticles for alleviation of pain. J. Pharm. Sci. 101, 690–706. doi: 10.1002/jps.22780
Pohl, M., and Smith, L. (2012). Chronic pain and addiction: challenging co-occurring disorders. J. Psych. Drugs 44, 119–124. doi: 10.1080/02791072.2012.684621
Ramírez-García, P. D., Retamal, J. S., Shenoy, P., Imlach, W., Sykes, M., Truong, N., et al. (2019). A pH-responsive nanoparticle targets the neurokinin 1 receptor in endosomes to prevent chronic pain. Nat. Nanotechnol. 14, 1150–1159. doi: 10.1038/s41565-019-0568-x
Shen, H., Hu, X., Szymusiak, M., Wang, Z. J., and Liu, Y. (2013). Orally administered nanocurcumin to attenuate morphine tolerance: comparison between negatively charged PLGA and partially and fully PEGylated nanoparticles. Mol. Pharm. 10, 4546–4551. doi: 10.1021/mp400358z
Shin, J., Yin, Y., Park, H., Park, S., Triantafillu, U. L., Kim, Y., et al. (2018). p38 siRNA-encapsulated PLGA nanoparticles alleviate neuropathic pain behavior in rats by inhibiting microglia activation. Nanomedicine 13, 1607–1621. doi: 10.2217/nnm-2018-0054
Smith, L. J., Valenzuela, J. R., Krugner-Higby, L. A., Brown, C., and Heath, T. D. (2006). A single dose of liposome-encapsulated hydromorphone provides extended analgesia in a rat model of neuropathic pain. Compar. Med. 56, 487–492.
Song, T., Gu, K., Wang, W., Wang, H., Yang, Y., Yang, L., et al. (2015). Prolonged suppression of neuropathic pain by sequential delivery of lidocaine and thalidomide drugs using PEGylated graphene oxide. J. Pharm. Sci. 104, 3851–3860. doi: 10.1002/jps.24598
Sullivan, M. D., and Howe, C. Q. (2013). Opioid therapy for chronic pain in the United States: promises and perils. PAIN® 154, S94–S100.
Tang, Z., Zhao, P., Ni, D., Liu, Y., Zhang, M., Wang, H., et al. (2018). Pyroelectric nanoplatform for NIR-II-triggered photothermal therapy with simultaneous pyroelectric dynamic therapy. Mat. Horiz. 5, 946–952. doi: 10.1039/c8mh00627j
Tosi, G., Costantino, L., Rivasi, F., Ruozi, B., Leo, E., Vergoni, A. V., et al. (2007). Targeting the central nervous system: in vivo experiments with peptide-derivatized nanoparticles loaded with Loperamide and Rhodamine-123. J. Control. Release 122, 1–9. doi: 10.1016/j.jconrel.2007.05.022
Urie, R., Ghosh, D., Ridha, I., and Rege, K. (2018). Inorganic nanomaterials for soft tissue repair and regeneration. Ann. Rev. Biomed. Eng. 20, 353–374. doi: 10.1146/annurev-bioeng-071516-044457
Van Dommelen, S. M., Vader, P., Lakhal, S., Kooijmans, S., van Solinge, W. W., Wood, M. J., et al. (2012). Microvesicles and exosomes: opportunities for cell-derived membrane vesicles in drug delivery. J. Control. Release 161, 635–644. doi: 10.1016/j.jconrel.2011.11.021
Ventola, C. L. (2017). Progress in nanomedicine: approved and investigational nanodrugs. Pharmacy Therapeutics 42:742.
Volkow, N., Benveniste, H., and McLellan, A. T. (2018). Use and misuse of opioids in chronic pain. Ann. Rev. Med. 69, 451–465. doi: 10.1146/annurev-med-011817-044739
Volkow, N. D., and McLellan, A. T. (2016). Opioid abuse in chronic pain—misconceptions and mitigation strategies. New Eng. J. Med. 374, 1253–1263. doi: 10.1056/nejmra1507771
Vowles, K. E., McEntee, M. L., Julnes, P. S., Frohe, T., Ney, J. P., and van der Goes, D. N. (2015). Rates of opioid misuse, abuse, and addiction in chronic pain: a systematic review and data synthesis. Pain 156, 569–576. doi: 10.1097/01.j.pain.0000460357.01998.f1
Wu, P.-C., Hsiao, H.-T., Lin, Y.-C., Shieh, D.-B., and Liu, Y.-C. (2017). The analgesia efficiency of ultrasmall magnetic iron oxide nanoparticles in mice chronic inflammatory pain model. Nanomed. Nanotechnol. Biol. Med. 13, 1975–1981. doi: 10.1016/j.nano.2017.05.005
Xu, C., Lei, C., and Yu, C. (2019). Mesoporous silica nanoparticles for protein protection and delivery. Front. Chem. 7:290.
Zhang, C., Bu, W., Ni, D., Zhang, S., Li, Q., Yao, Z., et al. (2016). Synthesis of iron nanometallic glasses and their application in cancer therapy by a localized Fenton reaction. Angew. Chem. Int. Ed. 55, 2101–2106. doi: 10.1002/anie.201510031
Zhang, J.-M., and An, J. (2007). Cytokines, inflammation and pain. Int. Anesthesiol. Clin. 45:27. doi: 10.1097/aia.0b013e318034194e
Keywords: nanotechnology, chronic pain, inflammation, drugs, targeting
Citation: Chen J, Jin T and Zhang H (2020) Nanotechnology in Chronic Pain Relief. Front. Bioeng. Biotechnol. 8:682. doi: 10.3389/fbioe.2020.00682
Received: 01 May 2020; Accepted: 02 June 2020;
Published: 19 June 2020.
Edited by:
Wei Tao, Harvard Medical School, United StatesReviewed by:
Jiang Ouyang, Central South University, ChinaChuang Liu, Ningbo Institute of Materials Technology & Engineering (CAS), China
Copyright © 2020 Chen, Jin and Zhang. This is an open-access article distributed under the terms of the Creative Commons Attribution License (CC BY). The use, distribution or reproduction in other forums is permitted, provided the original author(s) and the copyright owner(s) are credited and that the original publication in this journal is cited, in accordance with accepted academic practice. No use, distribution or reproduction is permitted which does not comply with these terms.
*Correspondence: Teng Jin, 394724768@qq.com; Hua Zhang, wozhanghua@126.com