- 1Chemistry Department, Acharya Narendra Dev College, University of Delhi, New Delhi, India
- 2Nucleic Acids Research Laboratory, CSIR-Institute of Genomics and Integrative Biology, New Delhi, India
The translational therapies to promote interaction between cell and signal come with stringent eligibility criteria. The chemically defined, hierarchically organized, and simpler yet blessed with robust intermolecular association, the peptides, are privileged to make the cut-off for sensing the cell-signal for biologics delivery and tissue engineering. The signature service and insoluble network formation of the peptide self-assemblies as hydrogels have drawn a spell of research activity among the scientists all around the globe in the past decades. The therapeutic peptide market players are anticipating promising growth opportunities due to the ample technological advancements in this field. The presence of the other organic moieties, enzyme substrates and well-established protecting groups like Fmoc and Boc etc., bring the best of both worlds. Since the large sequences of peptides severely limit the purification and their isolation, this article reviews the account of last 5 years' efforts on novel approaches for formulation and development of single molecule amino acids, ultra-short peptide self-assemblies (di- and tri- peptides only) and their derivatives as drug/gene carriers and tissue-engineering systems.
Introduction
The global trend is growing toward precise medicines and diagnoses through multi-centered approaches of drug delivery technology. The poor systemic bioavailability, solubility, absorption, and stability of large sized materials pose major challenges in the area of drug delivery. Novel natural biomaterials which can qualify to be biodegradable, biocompatible, non-toxic, renewable, and readily available to deliver therapeutic agents to precise targeted sites in a controlled manner is one of the most sort after research-field. Presently, nanotechnology (Lombardo et al., 2020) has provided a solution by opening up of newer avenues in terms of developing advanced controlled drug delivery and release systems that have met with huge success (Webber et al., 2016; Webber and Langer, 2017; Patra et al., 2018). The customizable nanoparticles with the manipulation in size, surface characteristics and materials used enhance the efficacy of drug delivery in a paramount manner along with the advantage of safer treatment (Eskandari et al., 2017; Rizvi and Saleh, 2018). A relatively newer area to deliver drugs across biological barriers for improved site-specific absorption is also being explored (Kou et al., 2018) i.e., transporter-targeted nanoparticles. A specific application based self-assembled materials, injectable biomaterials, are also being investigated to improve the advancing practices in healthcare (Sahoo et al., 2018b).
Uncomplicated to design and synthesize, biocompatible, embedded with appropriate opportunities for chemical alterations, demonstrating molecular selectivity and specific interaction with diverse types of biological systems- all these characteristics make peptides ideal and flexible candidates for constructing tuneable nanostructures with normal end functionalization (Sun L. et al., 2016; Yu et al., 2016; Galdiero and Gomes, 2017) (Figure 1). It is hypothesized that simple amino acids may be the first catalysts for formation of peptide bonds (Luisi, 2015). The evolutionary model put forward by Carny and Gazit on the mechanism of origin of life links the aptitude of short peptides to the creation of the present living systems (Carny and Gazit, 2005). According to this model, the properties like encapsulation, catalytic potential in chemical reactions and a highly ordered template for the assembly of nucleotides, which might be the early events, could lead to create the biological systems. Self-assembling peptides, a category of peptides, assemble spontaneously into ordered nanostructures. Peptides, even as short as dipeptides, are blessed to hold all the desirable molecular information to form well-ordered structures, when worked for nano-scale. The self-assembly of short peptides turning to β-sheet amyloid conformers brings extraordinary structural stability and multi-functionality like; self-replication, catalytic activities and information transfer, which is impossible for the corresponding non-aggregated peptides. The emergence and evolvement of such mutualistic networks may eventually lead to origin of life (Maury, 2018).
Peptide self-assemblies are the gait of 20 amino acids which can be manipulated in terms of number, type, sequence, and side chain groups. These nanostructures can be customized by incorporating modified amino acids in the peptide design to have superior assembling properties and enzymatic stability. This bottom-up science, inspired by the wonders of nature operating on the nanoscale, generates many biological nanostructures such as proteins and DNA/RNA, enabling functioning of life. Even the top-down method, with an advantage over the bottom-up approach supports to discover new peptide sequences aimed to a specific binding site on bio-macromolecules based on their structural properties. It is compelling the scientists to reach after the chemistry of these macromolecules and synthesize newer molecular self-assemblies. The peptide synthesis is a simple and affordable synthetic chemistry via conventional procedures, solution or solid phase. The cost of making peptide is found to be associated with the motif length, purity, chirality of amino acids as well as the expertise of the fabricator. The ability of natural and synthetic amino acid building blocks, both, to form ordered assemblies through self-association process with defined architectures and prominent physical properties has been reviewed by Chakraborty and Gazit (2018). The review by Lee et al., has summarized the building blocks of peptide self-assemblies categorized by their constituting amino acids, the bound chains/motifs, characteristics and regulatory factors (Lee et al., 2019). The study of ordered nanofibres from protein self-assembly in natural systems has been recognized as reductionist approach, wherein, core peptide building blocks could be derived from the parent protein (Guterman et al., 2016).
Regulators of Self-Assembly
The fundamental mechanisms for the self-assembled nanostructures of different types and structures of peptides are explored regularly (Mandal et al., 2014; Chen et al., 2016; Liao et al., 2016; Fan et al., 2017; Pandit et al., 2018; Mason and Buell, 2019). The constituent amino acid residues govern the supramolecular nanostructures and peptide secondary structures to adopt the conformations led by intra-/intermolecular interactions during peptide self-assembly. The multifunctional materials with highly ordered structures could be achieved by hierarchical architectures with anisotropy and suggested to be facilitated by rational self-control of weak intermolecular interactions regulating the self-assembly process at different steps (Yuan et al., 2019).
Inter/Intramolecular Non-Covalent Interactions
The design and nature of these ordered nanostructures is the outcome of the synergistic effect of various intermolecular non-covalent interactions, comprising H-bonding, π-π bonding, electrostatic forces, hydrophobic, and van der Waals' interactions. Molecular self-assembly, a more stable structure, is formed under thermodynamic equilibrium conditions by arrangements through various non-covalent weak interactions which can generate assemblies of excipient patterns (Mateescu et al., 2015). This alliance of non-covalent interactions strategizes the self-assembly process and determines the thermodynamic stability, though, kinetic parameters also play a critical factor in concluding the dynamic material (Wang et al., 2016a; Liu et al., 2017).
The hydrogen bonds have the competence to assist the growth of biomolecules in one direction with a long-range order to manifest one-dimensional (1D) nanostructures. The hydrogen bond stacking in case of cyclic peptides has been reported to provide the primary structure and comprehensive cylindrical morphology to the self-assembly (Rho et al., 2019). Besides the contribution from individual amino acids, the peptide backbone itself also provides significant stability through hydrogen bonds (Leite et al., 2015).
Electrostatic interactions portray remarkable performance in the self-assembly of peptides and stabilize the nanostructures. Electrostatic attraction between the positively charged peptide fibril and negatively charged small molecule drugs for controlled drug delivery (Mauri et al., 2017).
Amino acids based on amino acid residues can be categorized into hydrophobic and hydrophilic ones. Peptides can form highly ordered self-assembled superstructures due to their hydrophobic property. These hydrophobic interactions are observed to progress from open networks of secondary structures toward closed cylindrical nanostructures (β-sheets or random coils) (Fu et al., 2014).
van der Waals forces, contemplating entire intermolecular forces and relatively weaker to covalent bonds, play an integral role in supramolecular organization in nanotechnology. van der Waals interactions in plane are observed to control not only the molecular self-assembly structure but their phase transition as well (Gao et al., 2015). The dipole-dipole interactions also support in the self-assembly process and are reported to enhance the mechanical properties as dual non-covalent bonding strategy (Cao et al., 2019).
Ionic Interactions
The arrays of ionic interactions are also part of the driving energy of the self-assemblies in water along with the hydrophobic interactions and peptide-backbone hydrogen bonds. Self-assembling, ionic-complementary peptides are also being studied by researchers (Chen, 2005). The synergistic effect of both non-covalent and ionic interactions has been reported to provide better stability to the studied hydrogel (Xie et al., 2016).
Aromatic Interactions
π-π stacking between large π-conjugated surfaces provides an overall stability to supramolecular polymers bound together by non-covalent interactions (Cockroft et al., 2005). Various collagen like peptides mimic the fibril formation and assemble into higher order hierarchical structures through π-π stacking interactions (Chen and Zou, 2019). The hypothesis put forward by Gazit et al. regarding the lead role played by aromatic interactions in the self-assembly of peptide nanotubes/amyloid-like structures has been established time and again (Reches and Gazit, 2005). The aromatic-aromatic interactions have been reported to transform into β-sheet conformation from α-helix on being connected to an aromatic motif at C-terminal (Li J. et al., 2017). Though the importance of aromatic interactions in amyloid formation has been challenged (Lakshmanan et al., 2013) but, are significant in amyloid β-peptide (Genji et al., 2017).
In peptide self-assembly, nonpolar amino acids (aromatic and aliphatic amino acids) aggregate through π-π stacking and hydrophobic interactions, while the polar amino acids, depending on whether they have uncharged or charged residues, stabilize through either electrostatic interactions or hydrogen bonding. The weak bond-based injectable hydrogels based on hydrogen bonding, ionic, hydrophobic and π-π stacking interactions and host-guest chemistry have been reviewed by Ding and Wang (2017). These non-covalent interactions act as driving force in designing the gels (Dou and Feng, 2017). In a characteristic assembly, electrostatic repulsions control the nanofiber length. Addition of a covalent bond forming unit, which could conjugate to the peptide sequence by amide bond condensation altered the balance between hydrogen bond formation and compensated the repulsive electrostatic interactions. Thus formation of covalent bond reinforced hydrogen bonds between peptides enabling the fiber elongation, which otherwise energetically is not possible (Sato et al., 2017). The study on reversible covalent chemistry displayed that the difference in self-assembly modes at the non-covalent level could also be reflected at the covalent level (Komáromy et al., 2017).
It is difficult to predict the combo of all these molecular forces after the peptide self-assembly. Extra facts about the interaction of these forces are essential to plan much efficient, chemically stable peptide self-assemblies.
Secondary Structural Conformations
Most of the self-assembling peptides are supposed to be readily soluble in water due to the presence of amino acid molecules containing charged residues (alternating hydrophilic and hydrophobic regions), periodically repeated and discrete polar and non-polar surfaces. α-amino acids comprising the peptides have the inclination to adopt various secondary structural conformations like α-helices (Boyle, 2018), β-sheets (Leite et al., 2015), β-hairpins (Nagarkar et al., 2008) and even the folds (Yoo and Lee, 2017; Kulkarni et al., 2019) and this dynamic behavior of the self-assembling peptides at the molecular structural level influences the peptide-based self-assembly processes in water (Gopalan et al., 2015; Bera and Gazit, 2019; Kulkarni et al., 2019). The process continues and these structures assemble further spontaneously to form nanofibers which consequently aggregate into supramolecular scaffolds and can entrap large volumes of water. The hierarchical self-assembly process can potentially stabilize diverse β-sheet hydrogen bonded architectures. Among the secondary structures, hydrogen bonding between carbonyl oxygen and the amino group of every third residue in the helical turn stabilizes an α-helix (each helical turn consisting of 3.6 amino acid residues) while β-sheets originate with hydrogen bonding between two or more β-strands (along which, the backbone of the peptide stretches) (Kulkarni et al., 2019). The self-association phenomenon of aromatic side-chains in β-peptide oligomers supports the helical secondary structure formed by intramolecular backbone-side chain CH-π interactions and yields large vesicles due to the gain in the hydrophobic area (Mándity et al., 2014). In a study, Sarkar et al. (2015) have also demonstrated the effect of solvent interactions on the folding pattern resulting in a change in initial helical conformation and structural diversity of short aromatic γ-peptides. Another architecture, coiled-coil peptide self-assembly, though suffering from the drawback of having longer amino acid sequences compared to other self-assembling peptide systems such as β-sheet fibrillizing peptides or peptide amphiphiles has been reported to offer unique advantages as reviewed by Wu and Collier (2017). All these functional architectures are the result of molecular recognition process and self-assembly led by non-covalent interactions. Zhou et al. enlightened on the adoption of amino acid conformations in the complex interactive process in relation to peptide sequence. The study evidenced that peptides with high sequence similarity could self-assemble into diverse nanostructures though could acquire similar secondary structures while completely different sequences assembled into one type of nanostructures (Zhou et al., 2019).
Various molecular forces make peptides self-assemble in different supramolecular peptides. As per the observations, a peptide with an electrostatically charged/hydrophilic head and a hydrophobic tail would self-assemble in spherical micelles or vesicles which on elongation could lead the way into fibers or tubes, respectively. Peptides with a β-sheet show inclination to assemble into flat structures suchlike tapes or ribbons. Nonetheless, on increasing the concentration of the peptides, these tapes and ribbons could stack on one another and turn into more firmly packed fibers.
A relatively new innovation, the use of co-assembly, to produce nanostructures is also being explored. When an individual component is incompetent to have the basic properties required for the self-assembly, a co-assembly option provides the necessary support. The cooperativity movement is directed by non-covalent interactions, in particular, electrostatic. The combination of experimental justification and computational simulations provides a basic support to identify structural and functional components (Raymond and Nilsson, 2018).
Amphiphilic Peptides
It is an aqueous peptide self-assembly, characteristically spurred by the presence of amphiphilic character in the monomer units, contains hydrophilic and hydrophobic domains, which impulsively arrange to shield hydrophobic groups and minimize contact with bulk water. These molecules contain one or more alkyl chain tails along with a terminal peptidic head group. The fine tuning of balance among the hydrophilic block with polar amino acids and hydrophobic blocks could stabilize various supramolecular structures by hydrophobic, electrostatic, β-sheet hydrogen bonds and π-π stacking interactions (Mikhalevich et al., 2017; Qiu et al., 2018). A naïve investigation by Accardo et al. (2013) wherein use of an intrinsically disordered peptide as a polar head connected to alkyl chain led to some disorder-to-order transition upon their self-assembly in supramolecular aggregates. The group recommended this kind of ordered core and a “disordered” surface as a potential scaffold for future biomaterials. Furthermore, Tesauro et al. (2019) discussed the versatility of arrangements in side chains, option to load charges/functional groups and select physical and chemical patterns to acquire desired biostructures during the design of peptide amphiphile (PA) and suggested the option of using conformational preferences in structured and/or disordered peptides in the review.
The review by Cui et al. has emphasized on controlling the environment of PA self-assembly manufacturing and details of their applications (Cui et al., 2010). Addition of an ionizable/charged amino acid to the PA structure is suggestive to increase the number of charges per aggregate. A self-assembly model of PAs produced by a C16 alkyl tail linked to a chain of two lysines (C16K2) or three lysines (C16K3) stand reasonably accurate on the predicted behavior in terms of morphology, size and the state of protonation of the aggregates. The work by Zaldivar et al. revealed that the system followed a charge regulation mechanism and found to decrease electrostatic repulsions between charged lysines (Zaldivar et al., 2019). An atomic level study by Rad-Malekshahi et al. (2015a) analyzed the vesicle surface structure and dynamics of self-assembled nanovesicles along with the intermolecular forces between amphiphilic peptides to improve and tune the biophysical properties of the nanocarrier.
Impact of Chemical Modifications
Different chemical reactions are employed to construct self-assembled nanostructures (Rasale and Das, 2015). A general approach followed by peptide chemists is to incorporate modifications in the form of easy and reversible cleavable appropriate moieties/groups at the carbonyl–nitrogen bond (readily cleavable urethanes containing appropriate alkyl groups such as benzyl and tert-butyl liberating the amino groups), incorporating alkyl spacers of various lengths to modulate the chirality (Panda et al., 2019), π-clamping to tune the reactivity (Zhang C. et al., 2016), backbone amide modifications for peptidomimetic hydrogelators with enhanced stability and mechanical properties compared to peptide hydrogelators (Basavalingappa et al., 2019), enforcing a conformational constraint to prevent β-sheet structure (Bowerman and Nilsson, 2010), formulated sequence patterns to optimize charge distribution for conjugating bioactive cargo (Zhang H. et al., 2017), alternating d/l-chirality for 1D- to 2D-self-assembly (Insua and Montenegro, 2019), a racemic mixture of the mirror-image peptides to provide more rigidity to the gel (Nagy-Smith et al., 2017), alternative hydrophobic and hydrophilic residues for catalytic activity (Song et al., 2018), coordination with metal ions to inhibit amyloid-like structure (Ji et al., 2019), fine-tune assembly for hydrogel (Loic, 2017) and so on. N-Acetylation (compound attached to the amino; N-terminus) and C-amidation (compound attached to the carboxyl; C-terminus) is the most usual policy to stabilize nearly all categories of peptides. The peptides, without modification, perhaps may not be toxic, but the impact after the modification, though also unsettled, is being explored (Soleymani-Goloujeh et al., 2018). Amino acids and short peptides bearing moieties like 9-fluorenylmethoxycarbonyl (Fmoc) (Tao et al., 2016), (Chakraborty and Gazit, 2018), aromatic naphthalene-2-methoxycarbonyl (Nmoc) (Rasale et al., 2015), 9-anthracenemethoxycarbonyl (Amoc) (Gavel et al., 2018a), at N-terminal provide extra advantage to the fabrication of self-assemblies due to inherent hydrophobicity and aromaticity and are also, significant in gel formation (Orbach et al., 2012; Fleming et al., 2013; Singh et al., 2015). tert-butyloxycarbonyl (Boc) is also used to protect α-amino group in peptide synthesis (Ragnarsson and Grehn, 2013). Introduction of non-natural d-amino acid at the N-terminus, has shown unexpected effects on peptide secondary conformation and even the biological performance and so is another useful strategy to confer self-assembling properties (Melchionna et al., 2016). Small chemical modifications in peptides at the N- or C-terminus, intrinsic to self-assembly into ordered supramolecular architectures and their biomedical applications, have been reviewed by Rad-Malekshahi et al. (2015b). N- and C-terminal of the aromatic components as well as linker segment and peptide sequence have also been demonstrated to control the self-assembly of aromatic peptide amphiphiles (Fleming and Ulijn, 2014).
Recently, guiding principles to customize the kinetics and morphological changes in supramolecular peptide nanostructures have been introduced by Son et al. (2019) upon exposure of matrix metalloproteinase. These guiding principles enumerate systematic customization of enzyme-responsive peptide nanostructures by exchange of just a few amino acids, for general use in performance optimization of enzyme-responsive materials (Son et al., 2019). The advantages of enzyme-instructed self-assembly (EISA) in triggering the molecular self-assembly in situ, by overexpression, to prepare supramolecular biofunctional materials and hydrogels has been reviewed by Gao et al. (2019).
The focus has also turned to design self-assemblies of peptide-based conjugates. The multidisciplinary studies involving conjugated short peptides and single amino acids highlighted the role of conjugated material and broadened the horizon of self-assembling materials (Acar et al., 2017; Edwards-Gayle and Hamley, 2017). The reformed attempts to enhance in vivo half-life time and widespread applications of peptides are being made by conjugating them with nanoparticles (Jeong et al., 2018; Spicer et al., 2018; Wang et al., 2018; Jiang et al., 2019). A critical comparison of peptide materials with non-peptide materials has been attempted by Santis and Readnov to count on the contribution of peptide self-assemblies in real-life applications i.e., commercial products (De Santis and Ryadnov, 2015). The use of sequence-specific peptides as biological recognition elements has nicely been reviewed by Slocik and Naik (Slocik and Naik, 2017). The insertion of suitable spacers (charged or neutral) between the hydrophobic region and the peptide are reported to uphold flexibility, mobility, and sometimes increase the solubility of the molecule. The linker is supportive for creating functionalized nanofibrils and expand the modules of chemoselective bio-conjugation approaches in site-specific titivation of self-assembling peptides (Biscaglia et al., 2016; Scelsi et al., 2019). Cui and coworkers (Cui and Chen, 2017) worked on a themed issue envisioned to bring leading researchers working on peptides and peptide conjugates to assess the recent progress in utilizing peptide-based constructs and describe the challenges to interface with biology for specific biomedical applications.
The peptide-templated noble metal catalysts also play an important role in chemical biology (Wang W. et al., 2017). Metal coordination to natural and non-natural binding sites of different peptides has been reported to stimulate the peptide self-assembly (Zou et al., 2015). This knowledge of the forces to obtain an ordered organization can assist innovative peptide based materials for more assorted applications.
Applications of Peptide Self-Assemblies
Peptide Self- Assemblies as Drug Carriers
The rich chemistry of various non-covalent interactions has led to swift development of self-assemblies as drug carriers, particularly, in short peptides (Huang et al., 2013; Panda and Chauhan, 2014; Iglesias and Marchesan, 2017; Amit et al., 2018; Raza et al., 2018; Mishra and Jyoti Panda, 2019).
The impact of finite peptide nanostructures for the development of systemic therapeutic delivery vehicles is, in particular, of interest, as the length of the assembly plays important roles during cell uptake and tissue penetration (Mendes et al., 2013) (Figure 2). A strategy has been reported, where the length of charged peptide-amphiphile supramolecular assemblies could be controlled through covalent bond formation (Sato et al., 2017).
The extensive non-covalent interactions provide several advantages in developing self-assembled materials for drug-delivery (Leite et al., 2015). These are mainly at the three working stages, namely, drug loading, self-assembly–drug conjugate transport, and finally the cellular drug delivery (Doane and Burda, 2012; Habibi et al., 2016; Fan et al., 2017). Devadasu et al. have suggested that understanding of the drug and disease is of utmost importance before designing a delivery system (Devadasu et al., 2012). The self-assembled-ordered structures of peptides with extensive π-π and hydrogen bonding, also a precondition for semiconductor properties, has aspired the scientists to assemble such structures for biological semiconductors along-with biocompatible and drug release materials (Tao et al., 2017). A review by Sis and Webber has discussed about the basic designs in peptide self-assemblies and ways to mend the efficacy of drug delivery (Sis and Webber, 2019). Self-assembled photosensitizers resulting from amphiphilic dipeptide- or amino-acid-tuned for photodynamic therapy (PDT) have been reported (Liu et al., 2016). The tuneable size, surface charge and multi-responsiveness toward pH, detergents, and enzymes suggest the simple and efficient self-assembled method to deliver photosensitizers (Li L.L. et al., 2018).
Amphiphilic peptides have brought a paradigm shift toward self-assembled PA in drug delivery. The amphiphilic peptides provide a lot of option in both linear and cyclic peptide sequences, side chains loaded with charges, self–assembled as vesicles, micelles, nanofibers and nanotubes for delivery systems and for other biotechnological applications (Goel et al., 2015). An account on the versatility of cyclic peptides and the safety measures in terms of size control, length and bundle width of nanotubes during the successful delivery of active pharmaceutical ingredients has been presented in a mini-review by Hsieh and Liaw (2019). The impact of amino-acid side-chains or covalently linked hydrophobic chain in PA on their stimuli-responsive drug delivery applications has been reviewed by Song et al. (2017).
Another strategy is to use the self-assembly of amphiphilic drug molecules to do the drug-loading and then deliver the cargo as well on its own. In case of low water-solubility of the drug, hydrophilic segments may be conjugated to bestow amphiphilic behavior. The conjugation pushes the peptide sequences for one-dimensional elongation through β-sheet formation. Their architecture fundamentals administer the self-assembly of PA's into supramolecular systems to be applied in drug delivery (Lock et al., 2013).
Ample attention is also being directed to cell-penetrating peptides (CPPs), as carriers for intracellular transport cargoes such as siRNA, nucleic acids, proteins, various nano-particulate pharmaceutical carriers (e.g., liposomes, micelles), small molecule therapeutic agents as well as quantum dots and MRI contrast agents (Brasseur and Divita, 2010; Bechara and Sagan, 2013; Choi and David, 2014; Copolovici et al., 2014; Wang et al., 2014; Huang et al., 2015; Skotland et al., 2015; Dinca et al., 2016; Guo Z. et al., 2016; Kurrikoff et al., 2016; Lehto et al., 2016; Guidotti et al., 2017; Hoffmann et al., 2018; Panigrahi et al., 2018; Ramaker et al., 2018; Vánová et al., 2019), since these are internalized by cells in an exceedingly effective manner. The study by Ramaker et al. observed a statistically substantial dependence of CPPs' uptake efficiency on both net charge and peptide length; longer CPP possibly due to more ordered α-helical structure with high charge could ferry conjugated cargo across membranes more efficiently (Ramaker et al., 2018). The non-covalent approach for complexing CPPs to nucleic acids or viruses has shown better gene delivery both in vitro and in vivo (Alhakamy et al., 2013). Gallo et al. have provided a comprehensive list of recognized CPPs along with their reported applications (Gallo et al., 2019). Another review by Borrelli et al. has discussed biological properties of CPP upon conjugation with specific molecules with special emphasis on uses in cancer therapy (Borrelli et al., 2018).
Nanomedicines directly assembled from pharmaceutical ingredients, termed as small molecule nanomedicines (SMNs), have the potential to improve the drug delivery efficiency, biosafety and largely reduce the research and development cost. Xue et al. (2020) highlighted the recent advances in a section on drugs and photosensitizers with peptides and exhibited advantages of SMNs in the review article.
Tailored drug delivery vehicles are continuously gathering attention. Peptide–drug conjugates (PDCs) enable selective delivery of cytotoxic cargoes to target cells (Ma et al., 2017; Wang et al., 2017a; Wang W. et al., 2017; Vrettos et al., 2018). PDCs have exclusive and precise features to build one-component nanomedicines (OCNs) containing only one type of chemical substance. These OCNs do not require additional carriers. In fact, these are equipped with desired physicochemical features to involuntarily aggregate as well as accumulate at target sites (Su et al., 2015). Since peptides can be manufactured effortlessly in large quantities and need simple purification, their range of selection of peptide sequences as per the requisite physicochemical properties like stability, solubility, overall charge and availability of the characteristic groups for the conjugation with the therapeutic payload, these are considered as sought-after prodrugs (He et al., 2019).
Peptide-based hydrogels is another class of drug delivery vehicles programmed via drug encapsulation or conjugated covalently with therapeutics. Small peptide molecules, in general, have the desired state to form specific secondary structures in solution and then self-assemble into fibrillary network under various physical conditions (Fu et al., 2013; Tomasini and Castellucci, 2013; Zhang L. et al., 2015). An analysis of the structural/molecular features of different β-sheet peptide hydrogels and their relation to mechanical properties to design effective hydrogels has been reviewed by Rodriguez et al. (2016). The sticky-ended fibrillation designs, applied in DNA and coiled fibers, have inspired Sarkar et al. (2014) to develop a strategy to form staggered triple helical species assisted by interchain charged pairs. The comparison between the two classes of collagen mimetic peptides having same composition but different domain arrangements showed that the larger nucleation domains resulted in rapid fiber formation and gelation while short nucleation domains left the peptide soluble for longer period (Sarkar et al., 2014). The thixotropic supramolecular hydrogels formed in response to external environmental stimuli have wide ranges of potential biological applications (Levin et al., 2014; Seow and Hauser, 2014; Loic, 2017; Zanna and Tomasini, 2017; Mondal et al., 2020). The stability of peptide-based hydrogels, specially to enzymatic degradation has been reviewed by Yadav et al. (2020). The composition of hydrogel material with tuneable properties controls the release of sensitive drugs (Du et al., 2015; Raza et al., 2018). Various methods to design and control the self-assembly mechanism in hydrogels for drug-delivery have been elaborated in the literature (Altunbas and Pochan, 2011; Briuglia et al., 2014; Yu et al., 2015; Li et al., 2019b). A review by Zhang et al. has highlighted on tuning the unique features/morphology of the nanostructures functions formed during the gelation process by controlling the morphology-dependent variations (Zhang L. et al., 2015). The loaded drugs not only get physically trapped, but also bring morphological modifications in the peptide hydrogel (Kurbasic et al., 2017; Parisi et al., 2019), thus converting drug–peptide co-assembly into a supramolecular hydrogel. The attempts have also been made to design peptide-based supramolecular hydrogels for protein drug delivery and gene therapy (Li Y. et al., 2016; Youngblood et al., 2018). The incorporation of non-viral vectors within hydrogels to promote tissue regeneration is another challenging area (Rivas et al., 2019). A photo-cross-linking strategy, based on the ruthenium-complex-catalyzed conversion of tyrosine to dityrosine, to enhance the mechanical stability of nanofibers by 104-fold with a storage modulus of ~100 kPa (perhaps, one of the highest reported so far among the small peptide hydrogels), with potential to be used in tissue engineering and controlled drug release has been reported by Ding et al. (2013). The low molecular mass organic gelators (LMOGs) have gained much interest in recent years with impending applications in drug delivery and tissue engineering (Sagiri et al., 2014; Skilling et al., 2014; Li Z. et al., 2016). The work on nanostructures containing D-amino acids, for their role in biologics delivery is also catching attention, but has a long way to go (Wang H. et al., 2016). The covalent conjugation between a drug and a D-peptide impacts the gelation properties of a hydrogel including its biostability (Li et al., 2012). Insertion of D-amino acids is reported to twist heterochiral self-assembled peptide hydrogels. Its impact on the drug delivery along with other biological performances makes them versatile tools for therapy in future (Fichman and Gazit, 2014; Melchionna et al., 2016). The use of low molecular weight compounds in hydrogels for drug delivery is turning distinctive due to easy injectability, responsiveness to various stimuli and comfort of synthesis (Raeburn et al., 2013; Mayr et al., 2018). Even the presence of a single amino acid can influence the donor–acceptor charge-transfer interaction in a two-component co-assembled nanofibrous hydrogel (Nelli et al., 2017).
β-Hairpin hydrogels, a subgroup of hydrogels, are another exciting candidates as drug delivery vehicle. These are formed through a molecular self-assembly mechanism which occurs only after desired triggering of intramolecular peptide folding. A review by Worthington et al. (Worthington et al., 2017) has discussed the physical properties of this kind of hydrogel network and material properties which can be used for drug delivery.
Another class of self-assembling peptides, multi-domain peptides (MDPs), allows a wide range of modifications in β-sheet motif without disruption so that the materials for delivery can be trapped within the hydrophobic core of the nanofiber depending on the MDP design and cargo. (Kumar et al., 2015; Li I.C. et al., 2016; Moore and Hartgerink, 2017; Lopez-Silva et al., 2018; Chen and Zou, 2019) In a two-component system, in which a porphyrin cap is combined with a cyclic peptide the combination of various binding forces, e.g., hydrogen bonding, metal coordination, and dynamic covalent bonds, allows the delivery of encapsulated ligand (Ozores et al., 2017). These supramolecular injectable biomaterials, that can mimic the natural extracellular matrix nanostructure and show marked cellular infiltration, are ideal scaffolds for tissue engineering strategies. The self-assemble process of MDPs to a nanofibrous hydrogel requires the peptide sequence containing a core of alternating hydrophilic and hydrophobic amino acids and flanked by presence of charged amino acids which further modifies to nanofibers with bilayered β-sheets. These are further modified to a viscoelastic hydrogel processed via nanofiber elongation and cross-linking. The flexible short β-structure and the governing strong forces allow modifications to incorporate functionality, and so are the attractive choices for research (Li and Hartgerink, 2017; Carrejo et al., 2018).
As mentioned earlier, the peptide self-assemblies may have various morphologies and accordingly possess impressive range of applications though sometimes these might be undervalued. To overcome these limitations, assembly of multiple peptidic components can result in a broader range of applications as compared to the self-assemblies of either component. This epitome expands the conformational space of peptide self-assemblies in terms of structural and functional complexities (Makam and Gazit, 2018; Diaferia et al., 2019). For example, mixed dipeptide gelators are assumed to co-assemble to form fibers containing both the gelators randomly. But the pH triggered methodology, determined by the pKa of the gelator, a chemically programmed method, could alter the rate at which self-sorting occurs and forms self-assembled networks (Morris et al., 2013). These sophisticated multicomponent peptide assemblies are being seen as the next-generation bio-inspired materials (Draper and Adams, 2018; Raymond and Nilsson, 2018).
Tissue engineering is a special branch among self-assemblies whose perseverance is the replacement of damaged tissues with newly engineered tissues to restore the normal activity of the target organ, tissue or system. The rudimentary requirement of tissue engineering is a scaffold to sustain the cells and escort the regeneration of the new tissue (Figure 3). The self-assembling peptides are able to serve this resolute as scaffolds. The special focus in the review articles of hydrogels and porous scaffolds remains in controlled release of drugs from the tissue engineering platforms (Boekhoven and Stupp, 2014; Loo et al., 2015; Rambhia and Ma, 2015; Koutsopoulos, 2016; Banerjee et al., 2018; Lee, 2018; Inaba and Matsuura, 2019). One of the very important areas in tissue engineering is to explore the ways, to modify the properties of the materials, including mechanical and chemical functionality through changes at the sequence level to influence the cell behavior and modulate the scaffold stability (Zhou et al., 2014). Abbas et al. has reviewed the role of peptide and protein self-assembly in photodynamic and photothermal therapy (Abbas et al., 2017). There is a growing interest in the self-assemblies led by non-covalent hydrogels for three-dimensional cell scaffolding applications. The role of ultra-short molecules, namely, dipeptides and amino acids, holds special position in this area (Ryan and Nilsson, 2012). The collaboration between the molecular structure of the assembled materials and the mechanism of self-assembly grips the nerve on evolving biochemical and viscoelastic properties in this network (Maude et al., 2013). In one of the literature reports, Sarkar et al. (2018) have included an elaborated discussion on possible approaches to assimilate the functionality of peptide scaffolds that may be implanted in vivo at the site of ischemia for application in functional tissue regeneration. A review article by Rubert Pérez et al. (2015) and highlights the self-assembled peptides through solid-phase peptide methodologies, wherein the accurate amino acid sequence can be selected, for constructing bioactive matrices for regenerative medicine. Major advantages, promising applications and current limitations of peptidic materials has been reviewed by Pugliese and Gelain (2017).
Beyond the biological functions, designing peptides, with desired structures and functions by mimicking natural supramolecular systems, are in the air which can provide innovative designing principles to intricate “molecular robots” as next-generation peptide nanomaterials (Inaba and Matsuura, 2019). Handelman et al. (2016b) studied the reconstructive phase transition exhibited by some of the self-assembled ultrashort di- and tripeptide nanostructures to modify optoelectronic properties followed by the appearance of visible photoluminescence. The physics of light propagation (functional properties of linear and non-linear light propagation) in nanostructures of biological origin has been exploited to develop novel integrated nanophotonic devices (Handelman et al., 2016a).
Though the role of self-assembled peptides in drug and gene delivery is blossoming and there is a substantial flow in the research and review articles as mentioned above, but the short peptides (di- and tri-peptides) appear more promising and game changers, due to their simple structure, cost-effectiveness, non-toxic/non-antigenic nature and superior biocompatibility with enhanced bioactivity (Panda and Chauhan, 2014; Goel et al., 2015; Alam et al., 2016; Guo C. et al., 2016; Habibi et al., 2016; Hamley, 2017; Mishra and Jyoti Panda, 2019; Ni and Zhuo, 2019). Furthermore, the low molecular weight of short peptides allows purification via simple HPLC techniques. This review article has been conceptualized to express the potential of nanostructures resulting from single molecule of amino acids, di- and tri-peptides in the field of targeted biologics delivery and tissue engineering.
Single or Modified Single Amino Acids
Preparation of nano-shaped aggregates by self-assembly of simple building blocks is fascinating. It is quite startling that a self-assembly of a single amino acid, H-Phe-OH (Adler-Abramovich et al., 2012), was reported in 2012 much after the self-assembly of a dipeptide, H-Phe-Phe-OH (Reches and Gazit, 2003), in 2003. Single amino acid in nanodomain with a stable structure is rare. The chemical modifications of single amino acids have also been investigated for their potential in self-assembly (Chakraborty and Gazit, 2018). The aromatic α-amino acids; H-Phe-OH, H-His-OH, H-Tyr-OH, and H-Trp-OH, have been found to generate ordered self-assembled architects such as fibrils, ribbons, rods, and twisted nanosheets on varying the solvent systems (Singh et al., 2017).
The nanoparticles formed by a single amino acid derivative, a tryptophan derivative, Fmoc-Trp(Boc)-OH, with the molecular weight of 526.6 Da, showed biocompatibility and ability to release the encapsulated bioactive molecules to various cells by Dube et al. (2017). Protection to N-terminus with the Fmoc group and to the side chain with Boc group impacts the nature of peptide self-assembly and overall morphology. The spherical nanoparticles of Fmoc-Trp(Boc)-OH, with hollow interior at pH 6, showed no significant change in structure/morphology with change in pH and retained stability toward thermal perturbations upto 3 months. This asserted the overall impact of Fmoc and Boc groups. Efficient loading and release capabilities along with enhanced toxicity against cancer cells emphasize the role of self-assembly of a simple amino acid as a drug carrier (Tao et al., 2015; Zanna et al., 2015; Dube et al., 2017).
The study to unravel the stimulatory effect of H-Trp-OH on insulin absorption established that H-Trp-OH also possesses bio-enhancing effect as compared to other hydrophobic amino acids, viz., phenylalanine (Phe), proline (Pro) and isoleucine (Ile). The hypoglycemic reaction and surface plasmon resonance (SPR)-based assay showed enhancement in the oral absorption of insulin but without intermolecular interaction. Further, it showed the ability to enhance intestinal absorption of fluorescently labeled hydrophilic dextrans as well as GLP-1 and Exendin-4 peptide drugs (Kamei et al., 2018). H-Arg-OH, as single amino acid, has also shown the potential as insulin absorption enhancer and can be developed as oral delivery systems for insulin (Kamei et al., 2017). Likewise, the hydrophobic and π-π interactions between Fmoc-Lys-OH and pyrrole groups of Chlorin e6 (Ce6), a hydrophobic photosensitive drug, led to the formation of co-assembly, which showed an improved cellular uptake. The results advocate the promising potential of a non-toxic photosensitizer delivery system (Liu et al., 2016).
The pyrene conjugated H-Phe-OH derivative, Pyrene-Phe-OH, displayed its ability to gelify in aqueous solutions over a wide range of pH. The change in pH brought about significant alterations in its properties, namely, (i) a distinct change in morphology (nanoscale) from a chiral (left-handed helical) nano-fibers to achiral (non-helical) tape like nanofibers with increase in pH, and (ii) change in thixotropic (macroscale) property. The thixotropic behavior facilitated the encapsulation of vitamin B12 and an anticancer drug, doxorubicin (Dox), within the hydrogel and sustained release paving way to its drug-delivery applicability. The worth mentioning is that Pyrene-Val-OH was unable to form gel owing to absence of π-π interactions for self-association and the gelation process (Nanda et al., 2013).
The hydrophobic interactions hold good position in self-assembly to gel formation. Interestingly, in a research article, it has been shown that phenylketonuria formed due to defective phenylalanine hydroxylase, the H-Phe-OH level in brain increases and the self-assembly forms toxic amyloid fibrils by hydrophobic interactions. The administration of H-D-Phe-OH converts the fibrous formation route of H-Phe-OH to flakes formation and the flakes further restrict H-Phe-OH to form fiber. This slows down the toxic fibril formation (Singh V. et al., 2014), though doxycycline is observed to counteract these toxic effects (De Luigi et al., 2015).
The role of aromatic moieties is quite important in the self-assembly of ultrashort peptides, exclusively Fmoc-peptides, to form hydrogels (Orbach et al., 2012). Small angle neutron scattering (SANS) technique has been used to have a view on gel properties and cross-links of the fiber structures of low molecular weight gels formed by dipeptide gelators (Fleming et al., 2014; Mears et al., 2017). The presence of Fmoc protecting group enhances the hydrophobicity of H-Phe-OH, a hydrophobic amino acid, and lowers the solubility in water. The hydrophobicity of phenyl ring has been reported to contribute toward hydrogel formation ring while its aromaticity brings thermal stability to the supramolecular hydrogel system (Murali and Shanmugam, 2019). The study of the recipe of phenylalanine and Fmoc system for gel formation concluded that the covalent linkage between the two is highly imperative to provide accurate configuration and interactions. The methylene side chain serves in stacking process (in buffer conditions) to facilitate gel formation along with the non-covalent ionic interactions and hydrophobic stacking interactions during close proximity of Phe ring and Fmoc moiety in space. The gel showed enhanced dye diffusion and faster gel erosion at higher temperature suggesting further exploration of the molecule in drug delivery (Singh et al., 2015). The unidirectional hydrogen bonding of the carbamate group within Fmoc-Phe-OH assemblies directed the assembly into 1D fibrils while Fmoc-peptoid analogs displayed 2D/3D morphology due to the alteration in H-bonding (Rajbhandary et al., 2018).
Fmoc-Phe-OH derivatives, modified at the carboxylic acid position with diaminopropane (DAP), (non-fluorinated Fmoc-Phe-DAP, monofluorinated Fmoc-3F-Phe-DAP, Fmoc-F5-Phe-DAP, and co-assembly of Fmoc-Phe-DAP and Fmoc-F5-Phe-DAP) twisted to hydrogels on addition of physiologically relevant sodium chloride concentrations. The hydrogels exhibited encapsulation of non-steroidal anti-inflammatory drug, diclofenac, during the self-assembly process and in vivo drug release profile showed their usefulness as injectable materials. The release study of diclofenac, which decreased from Fmoc-F5-Phe-DAP to monofluorinated to the non-fluorinated gelator, demonstrated the role of the molecular structure of the gelators advocating the importance of aromatic benzyl side chain for π-π interactions between the gelator and cargo (Figure 4) (Raymond et al., 2019).
An amino acid derivative, N,N′-dibenzoyl-L-cystine (DBC), has been turned into a supramolecular hydrogel, as the carrier of salicylic acid (SA), by adjusting the pH of the solution. The DBC molecule with two amide groups and two carboxyl groups was able to oblige as hydrogen-bond donors and acceptors, respectively. The self-assembly of DBC molecules was facilitated by strong intermolecular hydrogen bonds linking neighboring amides and carboxylic acid as well as π-π stacking interactions amid aromatic rings, and hydrogen bonds among water molecules. The pH-responsive hydrogel along with thermo-reversibility and suitable mechanical properties is another addition to the controlled drug release system (Zhong et al., 2019).
Not much has been reported about fluorescent peptide nanotubes. Babar and Sarkar (Babar and Sarkar, 2017) synthesized stable, homogeneous nanotube type aggregates from individual amino acids, H-Trp-OH and H-Tyr-OH, retaining the characteristic fluorescence, which can be used as drug carriers. A simple approach, sonication followed by deposition onto brass stub, was used in the synthesis which could be extended to various nanotubes from single or mixture of amino acids. An inexpensive derivative of tyrosine, H-Tyr(t-Bu)-OH, is perhaps one of the lightest reported low molecular weight organogelator and only natural amino acid-derived gelator, decked with free -NH2 and -COOH groups. The analysis outcomes made this molecule more special as the gelation could be carried out in all classes of solvents; be it polar, protic, apolar, etc. The gelation in sunflower oil and diesel makes it an appropriate candidate for drug delivery (Aykent et al., 2019). Further investigations revealed the same capability of other enantiomer, H-D-Tyr(tBu)-OH to form the organogel while racemic H-DL-Tyr(tBu)-OH was unsuccessful to do so. The new work also exposed the role of tert-butyl moiety as H-Tyr-OH, H-Phe-OH and H-Tyr(Me)-OH could not form gel while H-Tyr(tBu)-OH could do so as mentioned above (Aykent et al., 2019).
Injectable self-assemblies of derivatives of H-Ala-OH, turned into hydrogels, were used to attach Dox, through an imine bond and the resulting Dox-Gel showed regression in tumor. The presence of rotationally-flexible, an aromatic N-protecting group on the H-Ala-OH accentuated the nanofiber creation. The intermolecular hydrogen-bonding in the derivatives, formed with the addition of carboxamide and hydrazide units at the carboxylic end of H-Ala-OH, H-Ala-CAM and H-Ala-HYD, respectively, along with the protonated H-Ala-HYD+, aided in the formation of gel. The imine bond between the hydrogel and drug was reversible which could cleave in the surrounding areas of the tumors and release the drug. The proposed work highlighted the use of self-assembly of molecules with molecular weight <300 Da in anticancer therapy (Singh M. et al., 2014).
A metallo-hydrogel was fabricated using H-Val-OH based ligand, L-3-methyl-2-(pyridine-4-ylmethylamino)-butanoic acid and Zn(II). The results revealed reversible gel-to-sol and xerogel-to-gel phase transitions and demonstrated a release of a polar drug stimulated by change in pH (Saha et al., 2014).
A two-component assembly of Fmoc-Tyr-OH and Fmoc-DOPA-OH (DOPA: 3,4-dihydroxyphenylalanine) resulted in a macroscopic structure with different characteristics from formed by modified amino acids hydrogels individually (Fichman et al., 2015). The amino acid H-Phe-OH in its zwitterionic state, under fibrillization conditions, is reported to be stabilized by hydrogen bonds and aromatic interactions network while organized into an ordered β-sheet-like layered assembly (Mossou et al., 2014). All coded amino acids are suggested to display a layer-like assembly (resembling supramolecular β-sheet structures) which is stabilized by α-amine to α-carboxyl H-bonds regardless of the presence of different side-chains that remarkably differ in their chemical properties though these side-chains govern the higher order organization of the layers. Overall, it recommends that the generic inclination of peptides and the proteins backbones to assemble as layered organizations might be ensued from their basic building block, the amino acid (Bera et al., 2018). Co-assembly, though an efficient strategy to form supramolecular structures, suffers in its tailor made functionality due to the lack of knowledge on structural correlation amid different amino acids. This makes the prediction on the resultant co-assemblies a difficult job. Bera et al. have demonstrated that the co-assembly of naturally occurring amino acids with similar chirality is strongly steered by their interlayer separation distances. a basic rule to predict the supramolecular co-assembly relationship with structure (Bera et al., 2019).
The role of disubstituted 1,2,3-triazoles, with the ability to mimic a trans- or a cis- configuration of the amide bond, prompted the researchers to use it as a co-assembly of isosteric amino acid-based hydrogelators. An amphiphilic N-stearoyl-L-glutamic acid (C18-Glu) and its analog with amide moiety replaced by 1,4-disubstituted 1,2,3-triazole unit (click-Glu) displayed distinctive nanostructures due to different hydrogen-bonding configurations. In C18-Glu, the polar protic environment (with intermolecular hydrogen-bonding between the amide NH-bond and the CO-group of the acid moiety next to the chiral center) favored nano-almond crunch-like structures while the non-polar environment preferred (with equal contribution from both intermolecular and intramolecular hydrogen-bonding) formation of nanofibers. The reverse was true for its analog click-Glu. The co-assembly, triggered by the formation of anticipated nanostructures in specific solvents, was applied for the release of an antibiotic, vancomycin (Bachl et al., 2015).
Dipeptides for Biologics Delivery
Since the beginning of the journey of the self-assembly, H-Phe-Phe-OH, the dipeptide, reported for the first time by Gazit and his group (Reches and Gazit, 2003), has taken the center-stage responding to its various roles. The core-recognition motif of the Alzheimer's disease associated β-amyloid polypeptide, has potential hydrophobic and hydrophilic moieties which are key parameters for molecular assembly. Other than self-assembly by itself, H-Phe-Phe-OH also functions as a co-assembling peptide for adjusting the self-assembly of various functional molecules, providing an easy and efficient method to modulate the morphology and property of these functional molecules. The potential applications of self-assembled dipeptides are catching up in the field of drug delivery and tissue engineering, as these moieties frame into variety of nanostructures, such as, spherical vesicles, nanotubes, and nanowires. The modes of interaction in general are π-π stackings, hydrophobic associations, electrostatic interactions, and hydrogen-bonding. Various morphologies based on free and protected H-Phe-Phe-OH molecule (Ribeiro et al., 2019) and its analogs/derivatives forming fibrils (Reches and Gazit, 2005), vesicles (Guo et al., 2012), tubes (Reches and Gazit, 2006), wires (Huang et al., 2014; Marchesan et al., 2015b), plates (Tamamis et al., 2009), sheets, flakes (Singh V. et al., 2014), necklaces (Yuran et al., 2012) could be demonstrated by manipulating the stimuli and physical/chemical conditions (Adler-Abramovich and Gazit, 2014; Datta et al., 2018, 2019). Concentration dependent ordered nanoarchitectures such as planar bilayers and other diverse shapes of vesicles, namely, discoid, toroid, ellipsoid, and pot-shaped, have been reported by Guo et al. (2012). Further, by chiral control at different stages, two elementary forms of the peptide self-assembly for the helical twisting of the β sheets were developed. These could turn into wide variety of hierarchical chiral nanostructures, viz., tube-like helical ribbons, twisted ribbons, big twists, nanoscrews and nanosprings (Wang et al., 2015). Even the rarely observed toroid nanostructures could be seen in H-Phe-Phe-OH and H-Phe-Phe-Phe-OH co-assembly (Guo C. et al., 2016) and triaromatic system, Z-Phe-Phe-OH (Z= benzyloxycarbonyl) (Brown et al., 2018).
In one of the studies by Wang et al. (2016b) involving H-Phe-Phe-OH dipeptide molecules, it has also been reported that the non-covalent interactions between trace solvents and peptides performing as solvent-bridged hydrogen bonding lead to directional hydrogen bonding between C-O and N–H in these molecules without inducing π-π stacking. It can promote long-range-ordered arrangement to form nanofibers /nanobelts preferentially along one dimension. The study on H-Gly-Pro-OH dipeptide has supported the ability of n → π* interactions between carbonyl groups for the stability of the structure (León et al., 2019). The nanotubes formation in self-assembly of a dipeptide, (S,S)-3-amino-2-(2-fluorophenyl)-3-phenylpropanoic acid and H-Ala-OH, has been observed to be supported by intermolecular N–H···O hydrogen bonds, Cπ-H···O, Cπ-H···F, and van der Waals interactions but no evidence of the presence of stacking interactions between the phenyl moieties has been observed by Bonetti et al. (2015).
The ability of peptides, even the ultrashort peptides as small as dipeptide, to form nano- and microstructures born with unique physical properties is the product of reductionist approach. It proposes the creation of well-ordered, amyloid-like β-sheet-rich assemblies comparable to supramolecular structures made of much larger proteins. This approach is exploited for the design and synthesis of peptide structures of technological utilization while establishing simple in vitro model systems to study the parent architecture. This has also resulted in the developing new bio-inspired configurations that could mimic the naturally-occurring architectures while keeping their functional properties intact, or even sometimes new functionalities are born. The similarity in the assembly mechanism between H-Phe-Phe-OH nanostructures and the aromatic amino acid containing amyloid fibrils inspired Brahmachari et al. to use the reductionist approach. Herein, a screening model was set up to identify molecules possibly capable of interfering with the aggregation process and their mode of action on the modulation of both the assembly and disassembly processes of H-Phe-Phe-OH assemblies (Brahmachari et al., 2017). Gazit is pioneer to demonstrate ultrashort peptides forming ordered assemblies by reductionist approach. His review on the reductionist approach educates on future of minimalistic peptide structures and the latitude of bioinspired self-assembly by the final reduction from very short peptides to extremely small metabolites (Gazit, 2018).
The analysis on temperature dependence of the kinetics of H-Phe-Phe-OH assembly, within the skeleton of crystallization theories, exposed that the transition state from solution to crystalline aggregates is enthalpically unfavorable-entropically favorable, qualitatively similar to longer sequences (Mason et al., 2017). Hydrophobic dipeptides, based on crystallization mode, could be categorized as self-assembled crystals composed of (i) elongated helical tubes; narrow hydrophobic channels and (ii) compact helical tubes; wide hydrophilic channels. The molecular mechanism build on density functional theory, advised by González-Díaz et al. recommends the position of the side chain, where it branches, during crystallization, to be the determining factor to drive the dipeptides into either hydrophobic or hydrophilic channels (González-Díaz et al., 2019). The structural transformation of a dipeptide self-assembly just by changing the types/ratios of the metal ion or the dipeptide to inhibit amyloid-like structure has been presented by Ji et al. (2019).
In a pair of dipeptide enantiomers, it is natural for both to exhibit opposite circular dichroism and handedness in self-assembly. In case, one of the amino acids is chiral, this chiral one exploits handedness. The situation is ambiguous, if both the amino acids are chiral. Fu et al. selected four dipeptides derived from L- and D-alanines and found that the chirality of the alanines at the terminals controls the handedness of their self-assemblies (Fu et al., 2013). The molecular hydrogelators, made of D-amino acids, have been shown to improve the selectivity of non-steroidal anti-inflammatory drugs (NSAIDs) (Li et al., 2012). It suggests that the chirality of the C-terminal amino acid commands the chiral orientation of the supramolecular helical nanostructures. In the study of dipeptides, viz., H-Phe-Phe-OH/H-Ala-Ala-OH, with NSAIDs, self-assembled as hydrogels, the peptides made of D-amino acids provided assistance to preserve the actions of NSAIDs (Li et al., 2013). Erdogan et al. (2015) observed morphological differences in H-Ala-Val-OH and H-Val-Ala-OH dipeptide molecules in the same solvent medium, though particular solvent property having impact on morphology difference could not be fixed. The two peptides differ in terms of the positional disorder of methyl group side chains in L-Val residues and torsion angles. The symmetrical intra- and intermolecular H-bonds, missing in H-Ala-Val-OH, but, present in H-Val-Ala-OH dipeptides were suggested to be accountable for long-range-ordered structures. The position and as well as number of methyl groups at the α carbons on N-terminus and C-terminus Fmoc-Phe(CH3)-Phe-OH or Fmoc-Phe-Phe(CH3)-OH has been observed to impact supramolecular nanostructure and the ability to form hydrogel (Arakawa et al., 2020).
An article dedicated to the role of dipeptides in diverse fields has been published by Panda and Mishra (2016). The bioinspired dipeptides in innovative emerging field of photoelectronics have been highlighted by Chen C. et al. (2015). Marchesan et al. discussed the preparation, characterization and pointed out the potential of H-Phe-Phe-OH motif in nanomedicine (Marchesan et al., 2015b). Multicomponent metallo-nanodrugs as coordination self-assemblies of Fmoc-His-OH and Z-His-Phe-OH in presence of Zn2+ ions, formed by the combined efforts of coordination and multiple non-covalent interactions were studied for cooperative coordination of photosensitizer Ce6. These multifunctional nanodrugs with enhanced tumor-specific delivery showed the potential for clinical translation (Li S. et al., 2018).
A controllable self-assembly of H-Phe-Phe-OH in an evaporative dewetting solution has been reported by Chen J. et al. (2015). The all-atom simulations of the small H-Phe-Phe-OH oligomers could accomplish the role of several driving forces and structural motifs that command initial assembly (Jeon et al., 2013). H-Phe-Phe-OH oligomers are associated by hydrophobic interactions between side chains while the H-Phe-Phe-OH zwitterionic peptides having charged termini form ordered, clustered, and compact shapes (electrostatic interactions steer their backbones into a more ordered state as compared to those of uncharged ones). From here on, the hydrophobic interactions of the side chains further lead to higher order oligomers just like in amphiphilic peptides. Therefore, the projected study concludes that the initial precursors of H-Phe-Phe-OH might first assemble into structures that form portions of the packed hydrophobic regions in the nanotube walls (Jeon et al., 2013; Chronopoulou et al., 2014). The aptness of the peptide prompted to explore the mechanical parameters of the H-Phe-Phe-OH self-assembled tubes. Atomic force microscope studies revealed these to be the stiffest (averaged point stiffness of 160 N/m) and with much higher Young's modulus (≈ 9 GPa) as compared to other biological nanostructures, significantly enough to provide mechanical strength to cytoskeleton (Kol et al., 2005). In peptides nanotubes, since the control over length of nanotubes in solution, posed a challenge, a slower co-assembly process along-with the adjustment in the molecular ratio of the H-Phe-Phe-OH assembly unit and its end-capped analog has been suggested (Adler-Abramovich et al., 2016).
The potential of H-Phe-Phe-OH microtubes as intracellular vehicle to release therapeutic compounds has been explored by Silva et al. (2013). The biological marker, rhodamine B, incorporated at the time of self-assembly, had the possibility to intercalate and showed its presence in the inner core to be accepted as intracellular drug delivery for hydrophilic molecules (Silva et al., 2013). These H-Phe-Phe-OH microtubes were further explored for delivering anti-cancer therapeutics, viz., 5-fluorouracil (5-FU) and anti-inflammatory cargo, flufenamic acid (FFA), by Emtiazi et al. (2017) post-conjugation with folic-acid and magnetic nanoparticles. These were found to show the potential as molecular carriers (Emtiazi et al., 2017).
The hydrophobic forces in these well-ordered tubular structures further facilitated to be soluble in a suitable solvent and self-assemble into well-organized films on various substrates. This technique was tried by Zohrabi et al. (2016) wherein FFA loaded inside the H-Phe-Phe-OH nanotubes were coated onto Au surfaces functionalized with 3-mercaptopropionic acid (MPA). The biocompatibility and in vitro release studies confirmed the potential of H-Phe-Phe-OH nanotubes as an alternate system for polymer coating in drugs eluting stents. Further, these H-Phe-Phe-OH nanotubes were also shown to possess anti-biofilm activity (Porter et al., 2018). Among the three H-Phe-Phe-OH variants at terminals, such as H-Phe-Phe-OH (l-enantiomer; carboxylic acid terminus), H-D-Phe-D-Phe-OH (d-enantiomer; carboxylic acid terminus), and H-Phe-Phe-NH2, investigated for this purpose, H-Phe-Phe-OH peptide nanotubes were found to be proficient to degrade the biofilm matrix, disrupt cell membranes and hold the potential as efficient drug carrier, though the precise link between these short self-assemblies and biofilm activity needs to be explored.
The peptide-porphyrin macrocycle conjugation has extended its use in biological systems, where the photophysical properties of these molecules and their ability to coordinate metals could be exploited. A review by Biscaglia and Gobbo has presented a survey on biomedical applications of these hybrid compounds, majorly in photodynamic therapy (Biscaglia and Gobbo, 2018). The reversible, biocatalytic and co-assembled nanofibers of Fmoc protected di-peptide (Fmoc-Thr-Leu-NH2) and its porphyrin derivative [TCPP, tetrakis(4-carboxyphenyl)porphyrin] advocate the role of Fmoc-moiety in biocatalysts (Wijerathne et al., 2019). The biocompatible photothermal nanodots formed by self-assembly of peptide-porphyrin conjugate; TPP-Phe-Phe-OH (TPP= tetraphenylporphyrin) have been found suitable for tumor ablation and stand good potential for biomedical photoactive applications. The peptide moieties could provide aqueous stability (through hydrophilic interactions) as well as a spatial barrier (through the strong π-π-stacking interactions between porphyrin groups) to inhibit the further growth of light-to-heat converted nanodots with totally inhibited fluorescence emission and singlet oxygen production (Zou et al., 2017).
Self-assembled cationic dipeptides (CDP), H-Phe-Phe-OH, nanocarriers have displayed the ability to encapsulate and transport drug molecules in vitro at physiological pH condition by the action of enzymes. A covalent bond formed via Schiff base between oligomeric glutaraldehyde and amino groups of CDP followed by aging yielded CDP nanocarriers (CDPNCs). The π-π interactions of aromatic rings have been proposed to be the driving force for the assembled nanocarriers. Remarkably, the auto-fluorescence due to n-π* transitions of C = N bonds offers visually traceable property in living cells. These highly biocompatible carriers with the ability to encapsulate small guest molecules and enzyme-sensitive nature exhibited a high cytotoxicity against tumor cell proliferation leading to be even used as in vivo applications in future (Zhang H. et al., 2015). Though the thermo-induced morphology changes modifying the optical properties in H-Phe-Phe-OH microtubes have been explored by Li et al. (2015), Semin et al. (2015), Nikitin et al. (2016), and Vasilev et al. (2016) the suitability of these H-Phe-Phe-OH based nanocarriers in the intracellular environment of tumor infected tissues was substantiated by Li Q. et al. (2016). Self-assembled hybrid nanospheres, pH- and glutathione (GSH)-responsive, based on H-Phe-Phe-OH and natural alginate dialdehyde as cross linker were used to deliver hydrophobic chemotherapeutic drugs. These nanospheres also contained Au3+, reduced to Au in situ by ADA, and formed H-Phe-Phe-ADA-Au hybrid nanospheres to facilitate ligand exchange reaction of GSH (Li Q. et al., 2016).
The impact of different degrees of hydrogen bonding and nitrogen substitution on mechanical properties of H-Phe-Phe-OH based peptides, indole-diphenylalanine, N-methyl indole-diphenylalanine, benzimidazolone-diphenylalanine and benzimidazole-diphenylalanine, capped at the N-terminus with heterocycles was executed by Martin et al. (2016). In conjugation with NSAIDs, this change altered the gelator properties. H-Phe-Phe-OH covalently conjugated to NSAIDs was found to form hydrogels and exhibit improved selectivity as compared to the native drug displaying its reputation in drug-delivery (Li et al., 2013). Moreover, the inclusion of ferrocene (Fc) moiety in H-Phe-Phe-OH hydrogel, a redox-active site, could change the morphology from nanosphere to nanofiber with the additional feature to reversibly control the self-assembly process of Fc–Phe-Phe-OH by altering the redox state of the Fc group (Wang et al., 2013b).
The study on impact of different N-terminal capping group to vary the aromaticity on hydrogel formation and properties with glyoxylamide mimics as self-assembly hydrogel by Aldilla et al. (2018) showed that the aromatic caps having the bulky indole side chain of tryptophan and another with electronegative substituent on the phenylalanine ring failed to form a hydrogel; might be because of hindrance in the intramolecular stacking. On the other hand, hydrogels with N-naphthalene sulfonyl cap, exhibited a β-sheet secondary structure with viscoelastic properties and topical delivery of ciprofloxacin and recommended as a drug delivery vehicle. Since H-Phe-Phe-OH forms nanofibers with remarkable optical and electrical properties, optical microscopy methods, fluorescence microscopy and super resolution single molecule localization microscopy have been suggested for the study of H-Phe-Phe-OH –based nanostructures (Pujals et al., 2017).
Kuang et al. made an attempt to establish the difference in properties between supramolecular nanofiber assemblies of small molecules and the individual molecules on interaction with the cells. The study of nanofibers formed by the self-assembly of two phenylalanine residues and a naphthyl group, with β-sheet-like-structure revealed the threshold concentration for the formation of nanofibers and suggested hydrophobicity to be responsible for higher cytotoxicity as compared to unassembled monomers (Kuang and Xu, 2013). A photo-responsive hydrogelator, wherein, H-Phe-Phe-OH was protected by 6-nitroveratryloxycarbonyl moiety; Nvoc-Phe-Phe-OH, has been designed and synthesized by Roth-Konforti et al. (2018). The photo-labile trigger, 4,5-dimethoxy-2-nitrobenzyl alcohol, served as a π-π stacking element in place of the aromatic Fmoc group. This stiff 3D-hydrogel displayed responsiveness to UV light irradiation and it completely disassembled subsequently on irradiation at room temperature to release the entrapped drug at the site of administration. The patterning of the photo-responsive peptide based hydrogel along with gradual release of insulin-FITC conferred linear correlation to the stimulus duration. Fmoc-Phe-Phe-OH gel remained unaffected by UV irradiation and showed insignificant FITC-insulin release post-UV application (Roth-Konforti et al., 2018).
The self-supporting gel, Fmoc-Phe-Phe-OH, an ultra-short peptide, is one of the most studied hydrogel. Fmoc-Phe-Phe-OH peptide has been reported by Aviv et al. to deliberate the mechanical rigidity and stability to hyaluronic acid (HA), a major component of the extracellular matrix, without the use of molecules to initiate chemical cross-linking. The Fmoc-Phe-Phe-OH/HA hydrogel, composed of two components, could allow fine-tuning of the hydrogel parameters, adjusted for injection and malleable and facilitating its use in drug-delivery and tissue engineering applications (Aviv et al., 2018). A review by Diaferia et al. describes the immense potential of the hydrogel specially in the field of drug delivery, tissue-engineering and catalytic behavior (Diaferia et al., 2019). Fmoc-Phe-Phe-OH based hydrogel nanoparticles have been explored as nano-carriers to encapsulate and deliver drugs/bioactive molecules. A scalable process for the assembly of Fmoc-Phe-Phe-OH peptide into hydrogel nanoparticles as ensuing drug delivery carriers has been outlined by Ischakov et al. (2013) The results of encapsulated Dox and 5-FU with different structural characteristics endorsed the assumption that the encapsulation ability of nanoparticles could be dependent upon the physicochemical nature of the molecules, thus affecting the release kinetics. Erdogan et al. have used freeze-quenching technique to prepare plasmonic nanoparticle-embedded organogels from Fmoc-Phe-Phe-OH and gold nanorods for efficient drug delivery. The controlled and enhanced release of the Dox was manipulated using laser illumination (Erdogan et al., 2016). The study by Truong et al. (2015) on Fmoc-Phe-Phe-OH self-assembled hydrogels with 5-FU and paclitaxel cautioned that it is the leaching time, not the exposure time which affects the overall cell viability and thus the cytotoxic effects could be observed only after the gel is completely dissolved. Since more stable gels are prone to leach their monomers slower into the neighboring biological environment, the overall concentration of these monomers and any potential adverse effects get reduced. Therefore, the factors controlling the stability of Fmoc-Phe-Phe-OH self-assembled hydrogels are more critical to continue this field (Truong et al., 2015). Argudo et al. have discussed the rules for chemical design through selection of the amino-acid sequence of Fmoc-dipeptides with the self assembling capability for 2D self-assembly at the air/water interface to form supramolecular structures (Argudo et al., 2018). The values of log P and -log S parameters have been proposed based on experimental results which can predict the possibility for a particular Fmoc-dipeptide to self-assemble at fluid interface. A cellular biosensing system, based on 3D culture model using Fmoc-Phe-Phe-OH dipeptide hydrogel employed as both, a 3D cell culture scaffold to provide a confinement in environment and an immobilized enzyme matrix through simple one-pot self-assembly is suggested for the detection of superoxide anion (O•-) by Lian et al. (2017).
A non-centro-symmetric β-sheet structure in Fmoc-Phe-Phe-OH nanofibrils, branded as biomimetic materials with mechanical properties equivalent to the biological gels, also displayed piezoelectric behavior which could enable these assemblies as scaffolds for tissue-engineering (Ryan et al., 2015). Fmoc-Phe-Phe-OH, hydrogel matrix, has been used by Lian et al. to construct a smart biointerface, followed by enzyme-based electrochemical biosensing and cell monitoring. The encapsulation of horseradish peroxidase (HRP) was achieved during self-assembly, which was subsequently used to detect the release of H2O2 from living cells (Lian et al., 2016).
The synergistic effect of the co-assembly of Fmoc-F5-Phe and Fmoc-Phe-Phe-OH has been reported to result in a ultra-rigid hydrogel with controllable mechanical properties optimum for tissue engineering (Figure 5) (Halperin-Sternfeld et al., 2017). The morphological transition from hybrid nanospheres to visible macroscopic films in the co-assembly of the cationic dipeptide (CDP), H-Phe-Phe-NH2 • HCl and a Keggin-type polyoxometalate (POM), phosphotungstic acid (PTA), upon photothermal treatment was observed which could be suitable for tissue engineering (Xing et al., 2015).
The in vivo studies of self-assembled, injectable, fibrous hydrogels obtained by the combination of dipeptide Fmoc-Phe-Phe-OH and poly-L-lysine have been reported to deliver the photosensitive drug Ce6 in a controlled manner at the tumor site and are suggested to work well under the strategy “once injection, multiple-treatments” by Abbas et al. (2018). Taking in and away plan of sheer forces has been observed to work well for the transmission from gel to sol state supporting the self-healing behavior.
Lately, the study by micro-second molecular dynamics simulations on the co-assembly of H-Phe-Phe-OH with different types of non-H-Phe-Phe-OH dipeptides manifested regular-shaped vesicles, single-/multi-cavity assemblies, and planar sheets, which otherwise, are hardly observed in self-assemblies of non-H-Phe-Phe-OH dipeptides. The balancing act between electrostatic repulsion, hydrophobic and aromatic stacking interactions attributes toward the formation of varied structures (Tang et al., 2020). Another novel, multi- component, organic- inorganic peptide-based hydrogel, with two building blocks, viz., Fmoc-Phe-Phe-OH and Fmoc-Arg-OH, and an inorganic material, hydroxyapatite (HAP) as 3D scaffolds was designed for bone tissue regeneration. Fmoc-Phe-Phe-OH served as rigid hydrogel that could mimic the ECM, arginine aided in tissue development and might also be helpful to fine tune the bioactivity of HAP, while HAP itself promoted the mechanical properties of the composite material. An optimum composition of the three resulted in a rigid and biocompatible hybrid suitable for cell adhesion and a bone tissue engineering scaffold (Ghosh et al., 2017).
A multicomponent dipeptide hydrogel from Fmoc-3F-Phe-Arg-NH2 and Fmoc-3F-Phe-Asp-OH, without covalent connection between Arg and Asp, co-assembled by aromatic, hydrophobic, and coulombic interactions displayed the feasibility in the design of innovative materials for tissue engineering (Liyanage et al., 2015).
It had been established that removal of the N-terminus charge via addition of a Boc group could not stop the formation of H-Phe-Phe-OH like structures (Levin et al., 2014). Boc-Phe-Phe-OH in different solvent conditions was observed to display tuneable structures of two different morphologies: tubular or spherical under different solvent conditions and the presence/absence of superparamagnetic iron oxide (SPIONs) core and studied for dual use (drug delivery and heat generation) (Majid et al., 2017). Also, molecular dynamics simulations had suggested that the N-terminus stabilizes the initial stage aggregates during the self-assembly formation while in the final tube structure, it does not rely on the charged termini regions. So when a mixture of H-Phe-Phe-OH and Boc-Phe-Phe-OH monomers, where the uncharged Boc group was added to the N-terminus of the dipeptide, leaving the aromatic residues free to interact, the fibers with varying morphologies and mechanical properties were grown depending on the ratio of the two monomeric dipeptides. Atomic force microscopy (AFM), fluorescence and FTIR spectroscopy showed a reduced Young's modulus and rigidity as well as reduction in hydrogen bonding along with an alteration in the electronic state of the aromatic residues in co-assembled fibers. So it was hypothesized that this reduction in the hydrogen bonding and a modification in π-π stacking between monomers altered the packing co-assembly. So, the study gives clue to develop various materials with different surface groups resulting in different interactions impacting the mechanical properties of scaffolds to be used in tissue-engineering (Creasey et al., 2016).
The co-assembly of H-Phe-Phe-OH and Boc-Phe-Phe-OH formed the fibers in aqueous conditions with reduced stiffness and curvier morphology. Creasey et al. proposed the disruption of the hydrogen bonding among neighboring N-termini and a variation of π-π stacking between monomers altered the packing of co-assembly and thus provided an option of post-assembly chemical functionality and supramolecular sites for drug delivery vehicles (Creasey et al., 2016). Just to briefly mention, a new prototype, an orthogonal strategy, for the cargo release from micro-compartments by modulating the intrinsic self-assembly state and opening the prospects to use the triggered self-assembly to finally control the transport of various molecular species across interfaces has been demonstrated with Boc-Phe-Phe-OH, which could trigger jet-like release within seconds (Levin et al., 2018). Among the short hybrid dipeptides composed of β(O)-δ5 -amino acids synthesized by Reja et al., the injectable hydrogel, Boc-β(O)-δ5-Phe-β(O)-δ5-Phe showed its utility in 2D-cell culture and could encapsulate proflavine solution in the gel matrix and release slowly (Reja et al., 2019).
Rhein (4,5-dihydroxyanthraquinone-2-carboxylic acid), a traditional Chinese anti-inflammatory compound, exhibiting planar aromatic structure, is branded to enhance intramolecular π-π stacking in dipeptide-based materials. The rhein-Phe-Phe-OH peptide gets muddled into uniform spherical nanoassemblies spontaneously, suggesting its competence as carrier for drug delivery. The virtual screening of rhein-Phe-Phe-OH from Traditional Chinese Medicine (TCM) database (based on MD simulations), showed its significant binding energy toward camptothecin (CPT), known for strong antitumor efficacy but limited in use due to poor solubility and stability (perhaps the first compound to be designed cooperatively by both experimental studies and computation simulations). In vitro and in vivo experimental evaluations validated the computation simulations (Sun et al., 2019).
Amoc-Leu-Phe-OH; an Amoc-capped dipeptide, wherein, Amoc moiety is supposed to provide optimal hydrophobicity, which in turn, could enhance self-assembling propensity demonstrated anti-inflammatory action using the rat air pouch model and antibacterial efficiency against Gram-positive and Gram-negative bacteria. The non-cytotoxic hydrogel has been suggested to be used in targeted drug/cell delivery and for wound healing applications by Gavel et al. (2018b).
Non-proteinogenic α,β-didehydro-α-amino acids, with peculiar conformational properties- planar conformation due to a double bond, between Cα and Cβ carbon atoms and fixed values of t ϕ and ψ torsion angles, have shown their ability to form hydrogels (Vilaça et al., 2015b) and applicable in drug delivery. A procedure for cheaper, less laborious and less time taken than other methods of dehydropeptide synthesis (α,β-didehydro-α-amino acid) through oxidation of the C-terminal residue in N-Boc-protected and N-Fmoc-protected peptides, both in solution and on the solid support for peptide synthesis has been reported by Wołczanski and Lisowski (Wołczanski and Lisowski, 2018). The ability to form secondary and super secondary structures and highly stable nanostructures has made α,β-didehydrophenylalanine a center of attraction for the researchers (Gupta and Chauhan, 2011). Self-assembled nanoparticles of dipeptide, methionine-dehydrophenylalanine (Met-ΔPhe), having an unsaturated analog of naturally occurring phenylalanine to entrap curcumin, an anticancer agent, have been synthesized and used by Alam et al. (2012). In this study, the role of ΔPhe in self-assembly of H-Met-ΔPhe-OH was compared with the self-assembly of H-Met-Phe-OH having natural L-phenylalanine. The comparison signified that the presence of ΔPhe in H-Met-ΔPhe-OH promoted the formation and stability of self-assembled dipeptide NPs remarkably, and enhanced entrapment efficiency of curcumin. Besides, the combination of dipeptide assembly with curcumin offered several additional advantages such as high biocompatibility of the former, inhibition of the tumor growth and increased longevity of melanoma tumor-bearing animals of the later. Self-assembly of the dipeptide not only demonstrated the entrapment of curcumin, which could not be used up to its potential due to its hydrophobic nature, leading to poor aqueous solubility, but also improved its bioavailability. Therefore, such dipeptide-NPs could also be used to improve the delivery of other effective hydrophobic drug molecules. Further work on the self-assembled nanotubes using two dipeptides with non-natural amino acids, one containing a flexible H-βPhe-Phe-OH amino acid (βPhe–Phe) and the other containing both H-βPhe-Phe-OH as well as a backbone constrained α,β-didehydrophenylalanine amino acid, H-βPhe–ΔPhe-OH, (βPhe–ΔPhe), was reported to have different properties than the native H-Phe–Phe-OH nanotubes. The study also addressed the effect of backbone length, introduction of conformational flexibility (caused by β-Phe) and constraint (produced by ΔPhe) on the self-assembly process and morphology. The dipeptide crystal, H-βPhe–Phe-OH, showed two conformations, viz., a gauche conformer forming the channel and a trans conformer trapped inside the channel formed by the gauche conformer, probably the first example presenting such self-entrapment. The nanotubes could efficiently encapsulate small hydrophobic drug molecules. The efficient delivery of anti-cancerous drug, mitoxantrone, entrapped in nanotubes as compared to free mitoxantrone can lead to development of more biocompatible and proteolytically stable drug delivery vehicles (Parween et al., 2014). Similarly, H-Phe–ΔPhe-OH based nanotubular system as sustained release nanocarriers for intravitreal delivery of the angiogenic drug was investigated. The sustained delivery potential, as pre-loaded and post-loaded options during nanotube formation assembly, was compared to the plain drug. It was speculated that in post-loading approach, there was a possibility of hydrophobic and aromatic π- π stacking interactions between the drug and the dipeptide. Additionally, certain amount of dipeptide might have released from the nanotube surface which could further entrap the drug within the nanotubes. The hydrophobic nature of the drug speculates its participation in the development of ordered nanotubes. Phenylalanine-α,β-didehydrophenylalanine (Phe-ΔPhe) dipeptide nanotubes were also explored by Khatri et al. to deliver cancer-testis antigens (CTAs). H-Phe-ΔPhe-CTA nanocomplex showed an inhibition in tumor growth in animal studies, opening the door for this dipeptide as in vivo delivery vehicle for CTAs (Khatri et al., 2019).
An arginine containing dipeptide, arginine-α,β-didehydrophenylalanine (Arg-ΔPhe), showed the capability to condense the plasmid DNA into positively charged nanoparticles of spherical morphology and deliver it inside the cells and distribute in cytosol and endosomes. The methodology offers simple synthesis route and characterization, viz., stability, biocompatibility and significant transfection efficiency, and made H-Arg-ΔPhe-OH a suitable contender for in vivo gene delivery applications (Khatri et al., 2017). The introduction of non-protein amino acid, namely N-methylated amino acid, D-amino acid and α,β,-didehydroamino acid, in peptides provides enhanced stability with regard to enzymatic degradation. Self-assembled nanoparticles of H-Arg-ΔPhe-OH were synthesized, characterized and derivatized with folic acid which showed high affinity for its cell surface receptors. The results exhibited significant cellular uptake and tumor regression toward different cancer cells compared to free drug, Dox /drug-loaded underivatized NPs both in vitro and in vivo. Iron oxide nanoparticles (IONP) conjugated H-Arg-ΔPhe-OH also showed heat-induced cytotoxicity on lung cancer cells which resulted in the death of these cells (Baskar et al., 2017). Further, it was demonstrated that the presence of arginine in the cationic dipeptide nanoparticles endorsed pH responsive behavior. FTIR and XRD analysis results for compatibility assessment ensured the retention of Dox activity and efficiency within the developed H-Arg-ΔPhe-Dox NPs. pH dependent release studies showed signs of maximum release at acidic pH and so, an excellent choice for site-specific drug delivery in tumors and tissues harboring acidic microenvironment like that of stomach (Singh et al., 2018). The H-Arg-ΔPhe-OH NPs, when conjugated with lactobionic acid (LA), a ligand for the asialoglycoprotein receptors, overexpressed in hepatocellular carcinoma (HCC) cell lines, achieved targeted delivery. H-Arg-ΔPhe-LA/miR NPs demonstrated the selective delivery of miR-199a-3p and so, the formulation could be used in HCC therapy (Varshney et al., 2018).
Spontaneous self-assembly of a dipeptide, leucine-α,β-didehydrophenylalanine (Leu-ΔPhe), into a stable hydrogel, was observed to enhance the antitumor activity of the drug, mitoxantrone. The amphipathic nature of the hydrogel, due to unprotected N- and C-terminals in H-Leu-ΔPhe-OH, could lend polar character. The side chains, bearing a strong hydrophobic character, offer the recipe for the entrapment of both hydrophilic and hydrophobic drugs in the gel matrix and release in a controlled manner (Thota et al., 2016).
Discrete nanoparticles synthesized by combination of different plasmid DNAs with cationic dipeptides. H-Lys-ΔPhe-OH and H-Arg-ΔPhe-OH, showed the potential of these dipeptides to protect DNA from enzymatic degradation and carried them to cellular cytoplasm and nucleus. The results showed that the DNA was packaged inside peptide NPs with no visible cytotoxic effect and exhibited enhanced cellular uptake. Therefore, these could be influential in non-viral mediated gene delivery applications (Panda et al., 2013).
Vilaça et al. synthesized naproxen N-conjugated dehydrodipeptide hydrogelators, having at least one aromatic amino acid (Vilaça et al., 2015b). Also, the evaluation of tryptophan N-capped naproxen and C-terminal dehydrophenylalanine (ΔPhe), dehydroaminobutyric acid (ΔAbu), and dehydroalanine (ΔAla), as protease resistant hydrogelators, demonstrated that Npx-Trp-Z-ΔPhe-OH (with C-terminal ΔPhe) was found to be the most suitable nanocarrier among the lot for the delivery of encapsulated low molecular weight drugs (Vilaça et al., 2015a). These results supported the development of a peptide construct, Npx-Ala-Z-ΔPhe-Gly-Arg-Gly-Asp-Gly-OH, which was also demonstrated to have properties of an efficient drug carrier (Vilaça et al., 2017). Further studies with tyrosine and aspartic acid residues, tyrosyldehydrophenylalanine [Npx-Tyr-Z-ΔPhe-OH] and aspartyldehydrophenyl-alanine [Npx-Asp-Z-ΔPhe-OH], showed that the incorporation of superparamagnetic iron oxide nanoparticles (SPIONs) could keep the magnetic behavior alive in spite of the strong diamagnetic contribution from the involved organic matrix. In vitro heat generation on magnetic excitation with AMF could convert the gel to a solution, acting as a remote trigger to release SPIONs and encapsulated drugs displaying an image-guided drug delivery through self-reporting mechanism (Carvalho et al., 2019). Recently, a newer dehydropeptide hydrogelator; Npx-Met-Z-ΔPhe-OH (containing naproxen and a thioether), was combined with two different architectures, core/shell manganese ferrite/gold nanoparticle and gold-decorated manganese ferrite nanoparticles (acclaiming the affinity of sulfur toward gold) and tested for the delivery of antitumor drug, curcumin by the group (Veloso et al., 2020). ΔPhe is known to endow proteolytic stability and add conformational restraints in the peptide backbone. The naproxen group is added to boost selectivity toward cyclooxygenase. The drug delivery was higher in both irradiated gels as compared to the non-irradiated gels, suggestive for multimodal cancer therapy by combining both the photothermia and controlled drug delivery.
Different metal ions as well as anions modulating the self-assembly of the peptide amphiphiles have been investigated by Sharma et al. advocating an ion responsive behavior to the hydrogels. The group (Sharma et al., 2019) has reported various metal salts to influence self-assembly of histidine based dipeptide amphiphile accompanying a gel to sol transition. In another study, Fan et al. have shown the capability of a dipeptide, H-Trp-Phe-OH, nanoparticles conjugated with an aptamer to bind Dox, due to the π-π stacking interaction and Zn(II) chelation. The formulation exhibited the possibility to act as optical probe to monitor the drug uptake and release (Fan et al., 2016). As recommended by Gazit, the study on fluorophores derived from green fluorescent protein and dipeptide conjugates sets the stage for new frontiers in peptide optics and to load various aromatic drug molecules into these nanoparticles via aromatic stacking interactions (Gazit, 2016).
The nanotubes of H-Lys-Lys-OH functionalized with camptothecin (CPT) chromophore at the ε-amino position, Ac-Lys-Lys(CPT)-NH2 and NH2-Lys-Lys-(CPT)-NH2, were observed to protect the drug from lactone hydrolysis by sequestering the CPT segment within the hydrophobic nanotube walls, thereby increasing its stability. Herein, CPT conjugated dipeptide played the dual function, both as drug and precursor to the nanostructured carrier (Kim et al., 2015). Similar to this scheme, the research group Sun et al. worked for the delivery of 5-FU and synthesized di-lysine conjugated with 5-fluorouracil (5-FU), wherein hydrophobic π-π association of both uracil and Fmoc could drive β-sheet self-assembly (Sun Y. et al., 2016).
Fluorescein isothiocyanate (FITC) is known as sensitive fluorescent probe which can be detected easily in living cells. The attachment of fluorescein to N-terminal of H-Leu-Leu-OH dipeptide carried out by Kirkham et al. has found to be more cytocompatible as compared to the molecule itself. The strong π-π stacking interactions between the aromatic units in conjugate molecule, Fl-Leu-Leu-OH, formed could be responsible in the induction of self-assembly process. Fl-Leu-Leu-OH adopted a β-sheet structure as shown by FTIR spectroscopy, cryogenic transmission electron microscopy (cryo-TEM) and small-angle X-ray scattering (SAXS) studies. The conjugation improved the capacity of Fl-Leu-Leu-OH to be uptaken by cells without compromising cell viability as compared to FITC alone (Kirkham et al., 2016).
Use of a dipeptide, H-Asp-Phe-OH, to assist the uptake of a therapeutic polypeptide drug, calcitonin, broadly used to treat bone diseases, has been demonstrated by Cao et al. (2017) as co-assembly of the peptide-drug nanoparticles. The dipeptide (Asp-Phe) simply interacted with the Salmon calcitonin (sCT) through hydrophobic and electrostatic interactions leading to the supramolecular nanoparticles of sCT-Asp-Phe-OH. This self-assembly established long-lasting therapeutic effect and could control in vivo release of sCT, thereby, shun the need for multiple injections in patients (Cao et al., 2017).
The formation of NDI-Tyr-Phe-NH2 by thermodynamically driven enzymatic condensation of naphthalenediimide (NDI)-functionalized tyrosine (NDI-Tyr) and phenylalanine-amide (Phe-NH2) has been reported by Nalluri et al. (2014). The presence of dihydroxy/alkoxy naphthalene donors could produce proficient charge-transfer complexes. The fully reversible self-assemblies driven on minimized free-energy and fewer defects holds potential to develop self-healing materials.
Microvesicles, formed from a water-soluble, synthetic, amphiphilic dipeptide, containing a glutamic acid residue at the C-terminus and hydrophobic residue (Val/Leu/Nva) at N-terminal have been established to deliver and release the encapsulated anticancer drug and a fluorescent dye in response to a stimulus of the presence of calcium ions. The dye/drug-loaded vesicles have been found to be non-toxic using 2-(4,5-dimethyl-1,3-thiazol-2-yl)-3,5-diphenyl-2,3-dihydro-1H-tetrazol-4-ium bromide (MTT) based cytotoxicity assay. These biocompatible microvesicles were reported to have the capability to carry cyclic adenosine monophosphate (cAMP) at the target site suggesting the potential of these delivery vectors to carry drugs and other bioactive molecules (Naskar et al., 2011).
A dipeptide hydrogel, containing double Fmoc-group, self-assembled at very low concentration, exhibiting two-step self-assembly process, cytotoxic, suitable for 2D/3D cell scaffolding has been reported recently. The self-assembly of Fmoc-Lys(Fmoc)-Asp-H is reported to have gained advantage from (i) additional H-bonding from the carbonyl group, (ii) aromatic and hydrophobic interactions from the fluorenyl ring, and (iii) steric optimization from the methoxycarbonyl moiety. The balance between the hydrophobic and hydrophilic functionalities is essential for the self-assembly. So the hydrophobicity due to the additional Fmoc group is suggested to be balanced by the two hydrophilic carboxylic acid moieties present at the C-terminus as well as the Asp side chain (Chakraborty et al., 2020).
As linkers play an important role in speedy and effective delivery of the cytotoxic drugs, a lysosomal protease-cleavable dipeptide H-Val-Cit-OH, has commonly been used in the designing and synthesis of antibody-drug conjugates (ADCs) for delivering cytotoxic drugs to antigen bearing cells. Besides, H-Val-Ala-OH, another important dipeptide, has also been employed for synthesizing next-generation ADCs useful for drug loading and releasing purposes. The comparison studies of H-Val-Cit-OH and H-Val-Ala-OH based ADCs conjugated to the monomethyl auristatin E(MMAE) payload using xenograft model test showed their ability as patent payload for the anti-tumor activity (Wang et al., 2017b; Wang W. et al., 2017). It has also been reported that adding a hydrophilic group to the N-terminus of the valine residue in the H-Val-Cit-OH linker, viz., glutamic acid, prevents the premature cleavage of the linker in mouse plasma which otherwise is susceptible to extracellular carboxylesterase. This enhancement in the polarity of H-Val-Cit-OH linker offers higher in vivo stability to the antibody–drug conjugate in mouse plasma and allows the designing of ADC linkers (Anami et al., 2018).
An amphiphilic dendron, N-octadecanoyl-1,5-bis(L-glutamic acid)-L-glutamic diamide with potential to entrap Vitamin B1 (VB1) was found to shrink and turn into supergel triggered by monovalent to tetravalent metal ions. The mechanism of continuous, pH-responsive and thermally reversible shrinkage process has been proposed to involve protonation of carboxylic acid groups in presence of metal ions transforming morphology of the hydrogel from nanotubes to nanobelts followed by crosslinking between protonated carboxylic acid groups and metal ions again transforming morphology to nanofibres. During this transition process, the encapsulated water was released but immobilized due to cross-linked 3D structures and VB1 was released in water phase. The release rate was found to be tuneable upon the shrinking rate of OGAc/metal hydrogel arrangement (Qin et al., 2013). Since the best shrinkage capability was exhibited by Mg2+ ions, this research group used the proposed phase regulation property with change in pH in a further study. It was also observed that the addition of positively charged species accelerated the shrinkage and negatively charged additives suppressed the shrinkage of the supramolecular hydrogel. These pH change and ionic separation properties were exploited for the step-wise release of two-component drugs, pralidoxime iodide and phenol red, first the anionic drug, phenol red, was released through gel shrinkage and then on increasing pH, released the second cationic drug, pralidoxime iodide, through gel collapse, which suggests that the system still demands more sophisticated work to be carried out on the concept (Qin et al., 2014). Another pH- responsive hydrogel from the class of N-acetylglucosamine derivatives, especially, the heptylurea derivative with a p-methoxybenzylideneacetal protective group, showed release of trapped naproxen on addition of acid (Goyal et al., 2014).
Cyclic peptides have advantage to linear peptides in terms of structure rigidness and stability against proteolytic enzymes. This structure rigidity can reduce the freedom of possible structural conformations and so enhance the binding affinity of the ligands toward receptors. The cyclo-D-Trp-Tyr peptide nanotubes have been synthesized and assessed as carriers of caspase 3 silence shRNA. This could penetrate the intact cornea and deliver the CAP3 pRFP-C-RS DNA reducing the apoptosis in the wounded cornea triggered by corneal epithelial debridement (Lee et al., 2015). The injectable, in situ ambidextrous supergelator, cyclo-Phe-Glu(O-tert-But) with cytocompatibility has been reported to hold potential in drug delivery and regenerative medicine (Manchineella et al., 2017). A cationic, cyclic dipeptide, photoresponsive hydrogelator, with a diketopiperazine (DKP) derivative containing an unnatural amino acid bearing an azobenzene photoswitch (PAP–DKP); PAP-DKP-Lys favorably encapsulated oligonucleotides and released upon irradiation, thereby showing the potential for tuning the amino acid residue for the particular cargoes (Pianowski et al., 2016).
Tripeptides as Biologics Carriers
Amino acid chirality, considered a key tool to drive peptide self-assembly, exploited at the molecular and supramolecular levels for D- and L-amino acids positions in case of gelling tripeptides has been reported by Marchesan et al. (2015a), to establish a higher or lower supramolecular order. So, a suitable design of the chirality in sequence of self-assemblies has been suggested to assign and fine-tune the properties of reported tripeptide gel biomaterials. The amphiphilic tripeptides have been reported to generate diverse nanostructures with variable aspect-ratio and geometry as the amino acid is altered by Matson and Stupp. Hydrogen bonding between amide proton donors and adjacent carbonyl proton acceptors leading to β-sheet-type association in self-assembling oligopeptides has been found to favor the formation of high aspect-ratio nanostructures (Matson and Stupp, 2012). Sahoo et al. carried the gelation of tripeptides, by connecting a hydrophobic phenylalanine residue at the N-terminus, a variable amino acid to Ac-Phe-X-Asp-NH2 to alter the tendency for intermolecular hydrogen bonding [X= G/A/V/L/I residue], and a charged C-terminal aspartic acid residue. They found that even without a strong peptide-specific secondary structure, the tripeptides with last three of the mentioned amino acids formed hydrogels (Sahoo et al., 2017). Furthermore, the work on selected Ac-Phe-X-Asp-NH2 sequence with high aspect ratio; Ac-Phe-Ile-Asp-NH2, suggested the requirement of the non-aromatic and uncharged residue in this tripeptide, which in this case was isoleucine–to be at least hydrophobic as well to facilitated high aspect-ratio nanostructure and aid hydrogel formation (Sahoo et al., 2018a). The study by Yang et al., on structural transition in Fmoc-Phe-Phe-Cys-OH from parallel-aligned worm-like micelles to entangled, coiled micelles persuaded by the cross-linking of the disulfide bonding, elucidated the relationship between micelle chirality and gel properties and suggested a strategy to fabricate materials with controlled chirality by means of synchronized non-covalent and covalent self-assembly (Yang et al., 2019). A series of Fmoc- protected tripeptide containing β-amino acid from H-βAla-His-OH [L-carnosine (Car)] joined by covalent link to different Fmoc-L-amino acids with a wide range of side-chains having different hydrophobicities were observed by Das et al. The tripeptides were found to be proteolytically stable under physiological condition and produce helical structures and the gelation capability to depend on the hydrophobicity of the side chain moiety where the additional π-π stacking interaction by the phenyl group supported the process. This study also demonstrated that the Fmoc-Phe-βAla-His-OH containing Fmoc-tripeptide exhibited the highest gelation ability among the examined amino acid residues, may be the phenyl group provided π-π stacking interaction. The tyrosine residue, expected for similar associative interaction, showed less gelation ability due to intermolecular H-bonding through the phenolic –OH group (Das Mahapatra et al., 2017).
Two tripeptides, viz., H-Gly-His-Lys-OH and H-Phe-Phe-Asp-OH, co-assembled through non-covalent assimilation, easily formed complex selectively with copper ions and converted into a hydrogel formed of nanofibers from clear solution of nanotapes in response to complexation (Abul-Haija et al., 2017).
A tripeptide, H-Lys-Phe-Gly-OH, accomplished the morphology change in secondary structure with switching of nanostructures from nanospheres (vesicles) to nanotubes reversibly depending on the concentration. A transition in the secondary structure could change the morphology of nanostructures. At the low concentration, the tripeptide formed spherical structures with diameters of 50±10 nm, while, at the higher concentration, the tubular aggregates with diameters of 190±10 nm were observed by Moitra et al. (2014, 2017). Even a temperature-induced change from tubular to vesicular has been observed. The nanovesicles, formed at physiological pH and disrupted at pH 6, a fit condition for the drug-delivery, were loaded with a chemotherapeutic anticancer drug, Dox, which resulted in the intracellular release of the drug.
The self-assembled nanoparticles of Fmoc-Leu-Leu-Leu-OMe have been reported by Li et al. as carriers of hydrophobic porphyrin derivative, meso-tetra(p-hydroxyphenyl) porphine (m-THPP) (Li J. et al., 2018). The doping of peptide nanoparticles facilitated the solubility of hydrophobic porphyrin in aqueous media. Additionally, the doping preserved the intrinsic fluorescent property and reduced the risk of side effects due to overdose. The doping could enhance the two-photon fluorescence absorption ability of m-THPP. In vitro reactive oxygen species detection and the cytotoxicity test suggested high anticancer efficacy of the peptide nanoparticles by the two-photon irradiation (Li J. et al., 2018; Li et al., 2019a).
Fmoc-halogenated phenylalanine hydrogels have also been prepared and reported by Wang et al. (2013a). Out of various fluorinated analogs, Fmoc-4F-Phe-OH was found to be most suitable since the fluorenyl groups stacked more extensively. However, none of these hydrogels were found suitable to serve as a bioactive scaffold for NIH 3T3 cell culture in 2D environments. Thereafter, H-Arg-Gly-Asp-OH peptide was included in Fmoc-4F-Phe-OH to aid the cells' survival. This compound showed 3T3 cell adhesion and competent cell division. The results were suggestive of using similar bioactive scaffolds for drug delivery, tissue engineering and cell culture etc (Wang et al., 2013a). A self-assembled protected tripeptide, containing hydrophobic core, Boc-Pro-Phe-Gly-OMe, showed the ability to encapsulate hydrophobic drugs—eosin, aspirin and curcumin. The stabilization of the encapsulated nanostructures with Vitamin E-TPGS illustrated the controlled release of drugs endorsing their role as drug carriers (Yadav et al., 2015). Later, replacement of phenylalanine with dehydrophenylalanine, a constrained dehydroamino acid, self-assembled as hydrophobic matrix dehydrotripeptide, Boc-Pro-ΔPhe-Gly-OMe. The enhanced encapsulation competence of hydrophobic molecules showed soundness of constrained dehydroamino acids in drug-delivery applications (Figure 6) (Deka et al., 2017).
H-Phe-Phe-OH could promote self-assembly and gelation through π-π and hydrophobic interactions to construct gel materials. Also, an amino acid, 11-aminoundecanoic acid (AUDA), non-proteinaceous with long aliphatic chain, could add thixotropic property to the hydrogel. A combination of AUDA and H-Phe-Phe-OH residues as Boc-AUDA-Phe-Phe-COOH could promote both hydrogelation and thixotropic nature. The resultant hydrogel exhibited encapsulation and sustained release of an antibiotic (vancomycin) and vitamin B12 at physiological pH and temperature and endorsed itself for drug-delivery applications (Baral et al., 2014).
High-resolution scanning electron microscopic analysis revealed the architectural differences in the synthesized tripeptides, Boc-Phe-Gly-Glu-OH (L1) and Boc-Phe-Val-Glu-OH (L2), differing in hydrophobic groups and their corresponding Cu(II) conjugates (Das et al., 2018). The tripeptide with more hydrophobic glycine generated flower-like decorated branches and the less hydrophobic formed well-ordered spherical assemblies with nano-meric dimensions. The corresponding metallic conjugates self-assembled as nano-belt like structures in the former and nano-flake like in the later, which could be due to the metal–peptide interactions that controlled the self-assembly process, entailing that the metal–peptide interactions settle on the morphology of the assemblies. The observation on these non-cytotoxic metallo-peptides; Boc-Phe-Gly-Glu-Cu (L1M) and Boc-Phe-Val-Glu-Cu (L2M), could establish the role of metal ions- peptides coordination in removal of strong non-covalent interaction and the formation of supramolecular architectures with various morphologies (Das et al., 2018).
Fmoc-tripeptide hydrogels from Fmoc-Phe–Phe–Phe-OH with different chirality were synthesized by Chronopoulou et al. by a lipase-supported reaction in an aqueous phase using genipin as a crosslinker. The proposed crosslinker could modulate the physicochemical features of such hydrogels. Hydrogels displayed release of almost same amount of the entrapped drug, Dexamethasone (DXM), though the release kinetics varied. The D-amino acid based hydrogels were observed to be “dense” materials, so could detain the entrapped drugs for longer period than the corresponding L-counterparts and could also resist protease degradation. The results could establish the importance of chirality to induce the specific secondary structural features and also modulate physicochemical properties of the hydrogel. These biomaterials hold bright future in drug delivery and scaffolding uses in tissue engineering (Chronopoulou et al., 2014, 2015, 2017).
The prevalent challenges among cell-scaffolding materials are to upkeep with the vital physiognomies of the otherwise complex natural extracellular matrix (ECM) and incorporate the essential characteristics with minimal complexity into a scaffold. A biomimetic, nanofibrous hydrogel, co-assembled from two aromatic PAs, a structural unit, Fmoc-Phe-Phe-OH, and a functional unit, Fmoc-Arg-Gly-Asp-OH showed its supremacy to reconstruct a normal dermal tissue analog with enhanced mechanical strength. Fmoc-Phe-Phe-OH/Fmoc-Arg-Gly-Asp-OH hydrogel with elongated spindle-like morphology resembling myofibroblasts organized the nanofibrous 3D-networks and contracted the gel besides cell secretion of fibronectin and collagen I. As per in vitro results, fibronectin deposited ahead of denser collagen I, indicated construction of a normal dermal tissue within the scaffold. This simplified design by Zhou et al. (2014) supplies a pathway to create such versatile cell-scaffolds in skin engineering and similar bioactivities.
The policy to integrate D-amino acid residues indicates their potential use in drug delivery. The incorporation of D-amino acid residues in three chiral centers of homochiral tripeptide, Boc-Phe-Phe-Phe-COOH, could change the orientation of the corresponding aromatic ring of Phe residue(s) as well as the direction of π-π stacking interaction, which, in turn, could alter the mechanism of drug-delivery. The various combinations tried by Basu et al., hinted that the mechanical strength and proteolytic stability of a particular gelator could be modulated by changing the molecular chirality and placing the D-residue instead of L-residues in the appropriate position (at or toward the C terminus) (Basu et al., 2016).
A heterochiral supramolecular H-D-Leu-Phe-Phe-OH tripeptide hydrogel, loaded with an anti-metabolite and pyrimidine analog, hydrophobic antineoplastic drug, 5-fluorouracil (5-FU), formed hydrogel under physiological conditions and demonstrated a fast release. Interacting through π-π stacking between the H-Phe-Phe-OH and 5-FU rings as well as hydrogen bonding between the carboxylic acid group and N-H group of the 5-FU, this work examined the potential of a drug capable to interact non-covalently with a self-assembling tripeptide (Parisi et al., 2019). The outcome of the loading of the anti-inflammatory drugs, naproxen and ketoprofen, in the tripeptide hydrogel in earlier works could establish that the naphthalene unit engages more efficiently through non-covalent interactions than the benzene rings with the aromatic units of the peptide (Mayr et al., 2018). Though the release of 5-FU was found to be faster than that of naproxen, the role of π-π interaction and hydrogen bonding is concurrent with the hypothesized one. H-Phe-DAla-Phe-OH is an exception to the design rule, where the tripeptides of syndiotactic L-D-L stereochemistry adopt an amphipathic conformation, and the side chains in anisotactic configuration enabling the long-range self-organization turn into gel-forming fibers, as the molecule does not possess the required hydrophobicity and steric hindrance and so, it does not turn to hydrogel (Garcia et al., 2018). The attachment of p-aminobenzoyl moiety at the N-terminus of the tripeptide, (p-aminobenzoyl)- Phe-D-Ala- Phe-NH2, could address both the issues to yield a new gelator (Kieffer et al., 2019) that could be explored further.
The self-assembly of nanodrugs, as self-delivery of drugs, involving a photosensitizer (chlorine e6, Ce6) and Dox as carrier-free nanoparticles which combined photodynamic therapy with chemotherapy to inhibit tumor recurrence, has been tried (Zhang R. et al., 2016). Following the same working model, the supramolecular drug-drug delivery system (SDDDS) comprising of a tripeptide, H-Tyr-Ser-Val-OH (YSV) and Gefitinib (GEF), nanoparticles was studied. The low toxicity of the former and molecular targeting ability of the later led to the co-assembly, that required no chemical modification and performed better in drug efficacy both in vitro and in vivo as compared to individual drugs (Zhang Z. et al., 2017).
A phosphorylated and succinylated tripeptide, H-Gly-Tyr-Lys-OH, on conjugation with folic acid and Taxol, FA–Gly-Tyr-Lys–Taxol, self-assembled into a molecular hydrogel. The much higher weight percentage of Taxol in this gel compared to other similar gels, proposes it as injectable material to deliver Taxol for long period of time during chemotherapy (Wang and Yang, 2012).
A cytocompatible, photoresponsive hydrogelator with tetrazole-containing moiety, Tet(I)-Gly-Phe-Phe-OH, with fast responses to mild light irradiation, has shown the ability to modulate cellular microenvironment using a bio-orthogonal photoclick reaction. The mechanically strong hydrogel could entrap horse serum (HS) and human mesenchymal stem cells (hMSCs) for longer duration of time and its release could be modulated through photodegradation of the gel matrix (photo-triggered tetrazole-to-pyrazoline transformation). Further investigation holds bright chances to control the biological behavior of the live cells in spatially defined channels (He et al., 2013). Nap-Phe-Phe-Gly-OH, another tripeptide hydrogelator, was found to create surface-induced self-assembly that could inhibit human platelet aggregations. The comparison with Nap-Phe-Phe-OH, which could not inhibit the platelet aggregation, evidenced the status of Gly residue (Zheng et al., 2012). Further exploration on naphthaleneimide (NI) conjugates; NI-Gly-Phe-Phe-OH and NI-Phe-Phe-Gly-OH supramolecular hydrogels, articulated the good biocompatibility of NI-Gly-Phe-Phe-OH with cell lines. The observations could suggest that these supramolecular materials are promising candidates for their use in biomedical applications (Yeh et al., 2016).
Among the hydrogel biomaterials, a tripeptide, Ac-Phe-Phe-Ala-NH2, developed by Pospišil et al., self-assembled into non-covalent, transparent, stable and biocompatible nanofiber hydrogel scaffolds at physiological pH without use of any organic solvent. The intramolecular hydrogen bonding of cross-β-type structure aggregates and non-covalent interactions between molecules of hydrogelators were proposed to lead the creation of the 3D gel network. The physicochemical and biological properties (in vitro) of the hydrogel suggest it as a hopeful scaffold for tissue engineering applications (Pospišil et al., 2016).
Acknowledging the importance of various factors, viz., morphology, rigidity, periodicity and epitope spacing, which impacted the performance of nanostructures and in turn vividly sway cell adhesion and signaling, a peptide-based amphiphile with a rigid amphiphilic structure of the derivative was obtained. A novel compound, N-3β-(4-tert-butylbenzoylamine)-(7α,12α-dihydroxy-5β-cholan-24-oyl)-(S)-Arg-(S)-Gly-(S)-Asp (tert-but-PhC-RGD), containing the H-Arg-Gly-Asp-OH epitope, a recognition motif involved in cell adhesion processes, was synthesized. This unusual self-assembly, compared to conventional H-Arg-Gly-Asp-OH containing amphiphiles, formed fibers with a circular cross-section and tested positive as scaffolds for tissue regeneration. The circular dichorism results showed weak interactions among H-Arg-Gly-Asp-OH groups as compared to the usual twisted ribbons superstructure which stipulate specific interactions among the chiral groups of the amphiphiles. So, relatively free H-Arg-Gly-Asp-OH groups provided opportunities to interact with the environment and, thereby, were found vital to the applications (Travaglini et al., 2017).
A tri-β-peptide, Ac-βAla-Z-βLys-βAla-OH, self-assembled to form non-toxic, biocompatible and mechanically stable hydrogel, probably the first peptide hydrogel comprising exclusively of β-amino acids, with stiffness similar to brain tissue. This tripeptide contained a β-homo-lysine (K) residue (to enhance solubility of peptide in aqueous buffer) and a C14 acylated alkyl chain (a hydrophobic acyl tail to produce a peptide amphiphile to direct self-assembly into nano-cylinders) and formed stable hydrogels. The peptide monomers, driven by a 3-point hydrogen-bonding motif associated with the 14-helical structure of N-acetyl-β3–peptides, self-assembled into helices in a unique head to-tail fashion. The hydrogel could rapidly recover stiffness since high strain could break just the 3D fibrous network by upsetting the non-covalent interactions and transform to sol, without destroying the fibers, but reorganized to 3D network again on reducing the strain in a relatively short time. This superior self-healing property of the hydrogel with minimal post-operative damage could fasten the recovery (Del Borgo et al., 2013). Following the recipe, Motamed et al. (2016) had developed a new technique to synthesize peptide based self-assembly wherein helical N-acetyl-β3–peptides could spontaneously form fibers, ranging from nano- to macroscale. The β3-tripeptides were observed by Del Borgo et al. to devise distinct self-assembly units segregated by a linker and turn to fibrous assemblies. The linkers within the peptide sequence, whether rigid or flexible, could be composed of a bioactive α-peptide, could demonstrate fiber formation and create a hybrid α/β peptide scaffold regardless of amino acid sequence, or interruption of the sequence in the presented work (Del Borgo et al., 2018). A structural model developed for self-assembled N-acetyl-β3-peptides as stable three-stranded helical coiled coil could be used to explore the fibril structure for other materials (Christofferson et al., 2018) and be tailored for different applications (Kulkarni et al., 2019). A summary of this content is provided in Table 1.
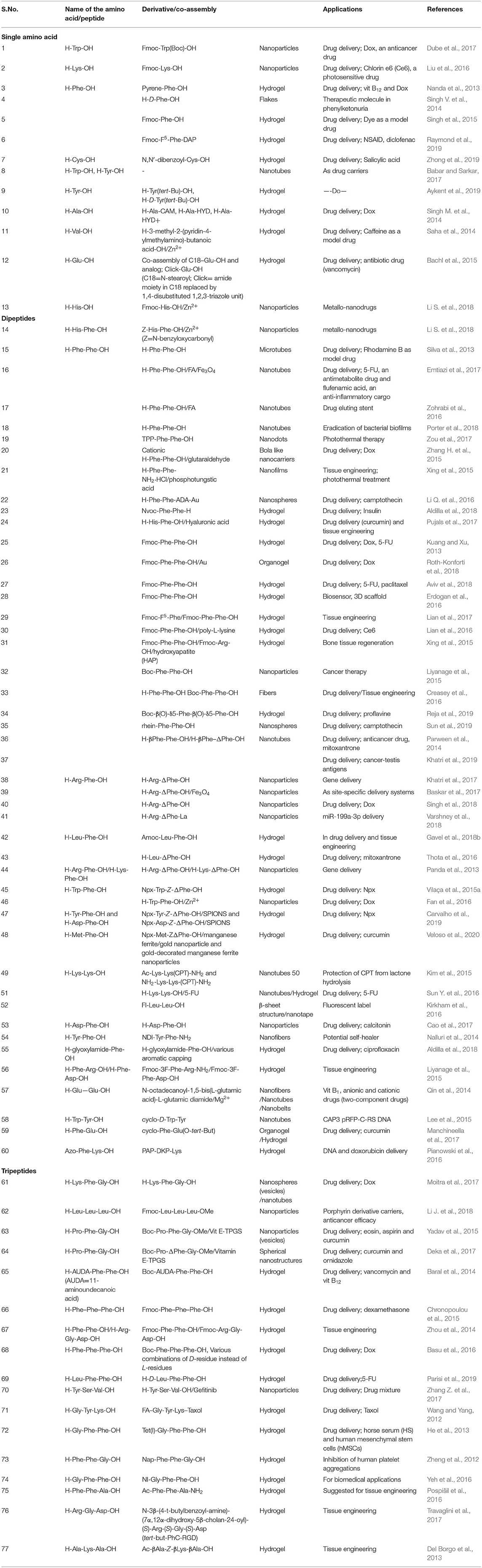
Table 1. Summary of the amino acid/peptide derivatives and their applications (Figure 7).
Conclusion AND Future Perspectives
Self-assembly by bioactive molecules is the talent to associate into ordered 3D structures via noncovalent interactions, through a bottom-up approach, without guidance by an external source (Dehsorkhi et al., 2014). In the last 20 years, remarkable expansion has revolutionized, the aptitude has navigated to the potentially useful nanostructure-based materials and has offered newer tools in the field of biological and biomedical sciences. Nanotechnology, the driving force behind this evolution and the revolutionary modifications, has contributed immensely toward the realization of targeted and controlled delivery of available therapeutics. Different materials/systems have been developed with this vision. Except for few, these materials hold their own merits and demerits. Biocompatibility, the nature of the interaction with surrounding tissues, is crucial. Certain materials have claimed to demonstrate biocompatibility, while other developed materials showing toxicity, are proved unsuitable for in vivo applications. Cationic lipid-based nanostructures, found to activate immune system, are such an example (Campani et al., 2018). Besides, these are also linked with certain technological issues, such as, reproducibility, stability, low drug-loading, uncontrolled leaching and encapsulation problems. Further polymeric systems have been developed, but the evaluation studies showed these materials also facing similar limitations. The surface functionalization was considered to improve drug or gene-targeting which in general, is complicated (Abd Ellah and Abouelmagd, 2017). Likewise, natural polymers, elicited undesirable immune reactions, illustrated batch to batch inconsistency (Ginjupalli et al., 2017) and so in vivo performance of these polymers became debatable.
Peptides and small molecule-based nanostructures can be virtuous alternatives for therapeutic delivery due to characteristics, like, good biocompatibility, easy on design/synthesis and also the functionalization. These distinctive and superior qualities make their self-assembled nanostructures smart tools in biomedical field. The masterstroke lies in the ability of these self-assembled small molecules to exhibit stimuli-responsiveness to the environment (internal/external stimuli), thus enabled to control and sustain the therapeutic release as per the requirement, an exciting prospect for multiple biomedical and bionanotechnology applications in drug delivery (Mart et al., 2006). Thus, virtues like, mild and rapid synthesis setting, non-requirement of specialized equipments, low production expenditure, easy dispersibility in aqueous medium and simple functionalization promote their use as future candidates for diverse applications such as, production of biomaterials, healthcare systems drug/gene delivery, tissue engineering, imaging, sensors, diagnosis, bioelectronics, and so on. Diverse types of structures/architectures can be generated simply by altering the physical or chemical conditions. Thus, this newer area of research is growing at an accelerating pace. Self-assemblies do result in the a variety of type of structures; nanoparticles, nanospheres, nanotubes, nanorods, nanotapes, nanofibers, nanogels, etc., yet there still exist several challenges to be addressed to make these self- assemblies- the materials of choice for research. The limited information on the influence of nanostructures on the organisms, control over size and composition during processing, tenability, behavior in aqueous environment, stability, up-scaling and degree of loading/entrapment of therapeutics are some obstacles which still need sincere attention of the researchers. Besides, follow-on these studies to establish biocompatibility and immunogenicity of these nanostructures are lacking. Nonetheless, it is viable to fine-tune the physicochemical properties by assimilating chemical modifications and so optimize the peptide functionality to minimize the toxicity without threatening their therapeutic activity (Lombardi et al., 2019). Hydrogel based drug delivery systems are of special mention as these are customized for modified release to the target site, reduce toxicity and side effects (Du et al., 2015; Li Y. et al., 2016).
Peptides offer a striking platform as non-viral gene delivery vectors which can minimize the disadvantages associated with viral vectors. Several groups have deliberated on non-viral gene delivery vehicles coupled with targeting peptides (Levine et al., 2013; Sharma et al., 2014; Kang et al., 2019; Wang et al., 2019). Though several functional peptide classes possess essential characteristics to overcome extracellular and intracellular barriers and make gene delivery feasible, still none of these functional peptides have been observed to contain all the essential characteristics required to overcome all of the barriers. Attempts are being made to develop novel multifunctional peptide vectors by combinatorial strategies and high-throughput screening (Jia et al., 2013; Raad et al., 2014; Riley and Vermerris, 2017).
Author Contributions
PK and AS conceived the idea to write a review article on peptide self-assembly. SG compiled the article and IS helped in the graphics and compilation. SG, AS, and PK also did editing.
Conflict of Interest
The authors declare that the research was conducted in the absence of any commercial or financial relationships that could be construed as a potential conflict of interest.
References
Abbas, M., Xing, R., Zhang, N., Zou, Q., and Yan, X. (2018). Antitumor photodynamic therapy based on dipeptide fibrous hydrogels with incorporation of photosensitive drugs. ACS Biomater. Sci. Eng. 4, 2046–2052. doi: 10.1021/acsbiomaterials.7b00624
Abbas, M., Zou, Q., Li, S., and Yan, X. (2017). Self-assembled peptide-and protein-based nanomaterials for antitumor photodynamic and photothermal therapy. Adv. Mater. 29:1605021. doi: 10.1002/adma.201605021
Abd Ellah, N. H., and Abouelmagd, S. A. (2017). Surface functionalization of polymeric nanoparticles for tumor drug delivery: approaches and challenges. Exp. Opin. Drug Deliv. 14, 201–214. doi: 10.1080/17425247.2016.1213238
Abul-Haija, Y. M., Scott, G. G., Sahoo, J. K., Tuttle, T., and Ulijn, R. V. (2017). Cooperative, ion-sensitive co-assembly of tripeptide hydrogels. Chem. Commun. 53, 9562–9565. doi: 10.1039/C7CC04796G
Acar, H., Srivastava, S., Chung, E. J., Schnorenberg, M. R., Barrett, J. C., LaBelle, J. L., et al. (2017). Self-assembling peptide-based building blocks in medical applications. Adv. Drug Deliv. Rev. 110, 65–79. doi: 10.1016/j.addr.2016.08.006
Accardo, A., Leone, M., Tesauro, D., Aufiero, R., Bénarouche, A., Cavalier, J.-F., et al. (2013). Solution conformational features and interfacial properties of an intrinsically disordered peptide coupled to alkyl chains: a new class of peptide amphiphiles. Mol. BioSyst. 9, 1401–1410. doi: 10.1039/c3mb25507g
Adler-Abramovich, L., and Gazit, E. (2014). Correction: the physical properties of supramolecular peptide assemblies: from building block association to technological applications. Chem. Soc. Rev. 43, 7236–7236. doi: 10.1039/C4CS90080D
Adler-Abramovich, L., Marco, P., Arnon, Z. A., Creasey, R. C., Michaels, T. C., Levin, A., et al. (2016). Controlling the physical dimensions of peptide nanotubes by supramolecular polymer coassembly. ACS Nano 10, 7436–7442. doi: 10.1021/acsnano.6b01587
Adler-Abramovich, L., Vaks, L., Carny, O., Trudler, D., Magno, A., Caflisch, A., et al. (2012). Phenylalanine assembly into toxic fibrils suggests amyloid etiology in phenylketonuria. Nat. Chem. Biol. 8:701. doi: 10.1038/nchembio.1002
Alam, S., Panda, J. J., and Chauhan, V. S. (2012). Novel dipeptide nanoparticles for effective curcumin delivery. Int. J. Nanomed. 7:4207. doi: 10.2147/IJN.S33015
Alam, S., Panda, J. J., Mukherjee, T. K., and Chauhan, V. S. (2016). Short peptide based nanotubes capable of effective curcumin delivery for treating drug resistant malaria. J. Nanobiotechnol. 14:26. doi: 10.1186/s12951-016-0179-8
Aldilla, V. R., Martin, A. D., Nizalapur, S., Marjo, C. E., Rich, A. M., Ho, K. K., et al. (2018). Glyoxylamide-based self-assembly hydrogels for sustained ciprofloxacin delivery. J. Mater. Chem. B, 6, 6089–6098. doi: 10.1039/C8TB01290C
Alhakamy, N. A., Nigatu, A. S., Berkland, C. J., and Ramsey, J. D. (2013). Noncovalently associated cell-penetrating peptides for gene delivery applications. Therap. Deliv. 4, 741–757. doi: 10.4155/tde.13.44
Altunbas, A., and Pochan, D. J. (2011). “Peptide-based and polypeptide-based hydrogels for drug delivery and tissue engineering,” in Peptide-Based Materials, ed T. Deming (Berlin; Heidelberg: Springer), 135–167. doi: 10.1007/128_2011_206
Amit, M., Yuran, S., Gazit, E., Reches, M., and Ashkenasy, N. (2018). Tailor-made functional peptide self-assembling nanostructures. Adv. Mater. 30:1707083. doi: 10.1002/adma.201707083
Anami, Y., Yamazaki, C. M., Xiong, W., Gui, X., Zhang, N., An, Z., et al. (2018). Glutamic acid–valine–citrulline linkers ensure stability and efficacy of antibody–drug conjugates in mice. Nat. Commun. 9:2512. doi: 10.1038/s41467-018-04982-3
Arakawa, H., Takeda, K., Higashi, S. L., Shibata, A., Kitamura, Y., and Ikeda, M. (2020). Self-assembly and hydrogel formation ability of Fmoc-dipeptides comprising α-methyl-L-phenylalanine. Polymer J. 30, 1–8. doi: 10.1038/s41428-019-0301-5
Argudo, P. G., Contreras-Montoya, R., de Cienfuegos, L. Á., Cuerva, J. M., Cano, M., Alba-Molina, D., et al. (2018). Unravelling the 2D self-assembly of Fmoc-dipeptides at fluid interfaces. Soft Matter. 14, 9343–9350. doi: 10.1039/C8SM01508B
Aviv, M., Halperin-Sternfeld, M., Grigoriants, I., Buzhansky, L., Mironi-Harpaz, I., Seliktar, D., et al. (2018). Improving the mechanical rigidity of hyaluronic acid by integration of a supramolecular peptide matrix. ACS Appl. Mater. Interfaces 10, 41883–41891. doi: 10.1021/acsami.8b08423
Aykent, G., Zeytun, C., Marion, A., and Özçubukçu, S. (2019). Simple tyrosine derivatives act as low molecular weight organogelators. Sci. Rep. 9:4893. doi: 10.1038/s41598-019-41142-z
Babar, D. G., and Sarkar, S. (2017). Self-assembled nanotubes from single fluorescent amino acid. Appl. Nanosci. 7, 101–107. doi: 10.1007/s13204-017-0551-5
Bachl, J., Mayr, J., Sayago, F. J., Cativiela, C., and Díaz, D. D. (2015). Amide–triazole isosteric substitution for tuning self-assembly and incorporating new functions into soft supramolecular materials. Chem. Commun. 51, 5294–5297. doi: 10.1039/C4CC08593K
Banerjee, J., Radvar, E., and Azevedo, H. (2018). “Self-assembling peptides and their application in tissue engineering and regenerative medicine,” in Peptides and Proteins as Biomaterials for Tissue Regeneration and Repair, eds M. A. Barbosa and M. C. L. Martins (Duxford: Elsevier), 245–281. doi: 10.1016/B978-0-08-100803-4.00010-3
Baral, A., Roy, S., Dehsorkhi, A., Hamley, I. W., Mohapatra, S., Ghosh, S., et al. (2014). Assembly of an injectable noncytotoxic peptide-based hydrogelator for sustained release of drugs. Langmuir 30, 929–936. doi: 10.1021/la4043638
Basavalingappa, V., Guterman, T., Tang, Y., Nir, S., Lei, J., Chakraborty, P., et al. (2019). Expanding the functional scope of the fmoc-diphenylalanine hydrogelator by introducing a rigidifying and chemically active urea backbone modification. Adv. Sci. 6:1900218. doi: 10.1002/advs.201900218
Baskar, G., Ravi, M., Panda, J. J., Khatri, A., Dev, B., Santosham, R., et al. (2017). Efficacy of dipeptide-coated magnetic nanoparticles in lung cancer models under pulsed electromagnetic field. Cancer Investig. 35, 431–442. doi: 10.1080/07357907.2017.1318894
Basu, K., Baral, A., Basak, S., Dehsorkhi, A., Nanda, J., Bhunia, D., et al. (2016). Peptide based hydrogels for cancer drug release: modulation of stiffness, drug release and proteolytic stability of hydrogels by incorporating D-amino acid residue (s). Chem. Commun. 52, 5045–5048. doi: 10.1039/C6CC01744D
Bechara, C., and Sagan, S. (2013). Cell-penetrating peptides: 20 years later, where do we stand? FEBS Lett. 587, 1693–1702. doi: 10.1016/j.febslet.2013.04.031
Bera, S., and Gazit, E. (2019). Self-assembly of functional nanostructures by short helical peptide building blocks. Protein Peptide Lett. 26, 88–97. doi: 10.2174/0929866525666180917163142
Bera, S., Mondal, S., Rencus-Lazar, S., and Gazit, E. (2018). Organization of amino acids into layered supramolecular secondary structures. Acc. Chem. Res. 51, 2187–2197. doi: 10.1021/acs.accounts.8b00131
Bera, S., Mondal, S., Tang, Y., Jacoby, G., Arad, E., Guterman, T., et al. (2019). Deciphering the rules for amino acid co-assembly based on interlayer distances. ACS Nano 13, 1703–1712. doi: 10.1021/acsnano.8b07775
Biscaglia, F., Frezza, E., Zurlo, E., and Gobbo, M. (2016). Linker dependent chirality of solvent induced self-assembled structures of porphyrin–α-helical peptide conjugates. Organ. Biomol. Chem. 14, 9568–9577. doi: 10.1039/C6OB01633B
Biscaglia, F., and Gobbo, M. (2018). Porphyrin–peptide conjugates in biomedical applications. Peptide Sci. 110:e24038. doi: 10.1002/pep2.24038
Boekhoven, J., and Stupp, S. I. (2014). 25th anniversary article: supramolecular materials for regenerative medicine. Adv. Mater. 26, 1642–1659. doi: 10.1002/adma.201304606
Bonetti, A., Pellegrino, S., Das, P., Yuran, S., Bucci, R., Ferri, N., et al. (2015). Dipeptide nanotubes containing unnatural fluorine-substituted β2, 3-diarylamino acid and L-alanine as candidates for biomedical applications. Organ. Lett. 17, 4468–4471. doi: 10.1021/acs.orglett.5b02132
Borrelli, A., Tornesello, A. L., Tornesello, M. L., and Buonaguro, F. M. (2018). Cell penetrating peptides as molecular carriers for anti-cancer agents. Molecules 23:20295. doi: 10.3390/molecules23020295
Bowerman, C. J., and Nilsson, B. L. (2010). A reductive trigger for peptide self-assembly and hydrogelation. J. Am. Chem. Soc. 132, 9526–9527. doi: 10.1021/ja1025535
Boyle, A. L. (2018). “Applications of de novo designed peptides,” in Peptide Applications in Biomedicine, Biotechnology and Bioengineering (Elsevier), 51–86. doi: 10.1016/B978-0-08-100736-5.00003-X
Brahmachari, S., Arnon, Z. A., Frydman-Marom, A., Gazit, E., and Adler-Abramovich, L. (2017). Diphenylalanine as a reductionist model for the mechanistic characterization of β-amyloid modulators. ACS Nano 11, 5960–5969. doi: 10.1021/acsnano.7b01662
Brasseur, R., and Divita, G. (2010). Happy Birthday Cell Penetrating Peptides: Already 20 Years. Amsterdam: Elsevier. doi: 10.1016/j.bbamem.2010.09.001
Briuglia, M.-L., Urquhart, A. J., and Lamprou, D. A. (2014). Sustained and controlled release of lipophilic drugs from a self-assembling amphiphilic peptide hydrogel. Int. J. Pharm. 474, 103–111. doi: 10.1016/j.ijpharm.2014.08.025
Brown, N., Lei, J., Zhan, C., Shimon, L. J., Adler-Abramovich, L., Wei, G., et al. (2018). Structural polymorphism in a self-assembled tri-aromatic peptide system. ACS nano, 12, 3253–3262. doi: 10.1021/acsnano.7b07723
Campani, V., Giarra, S., and De Rosa, G. (2018). Lipid-based core-shell nanoparticles: evolution and potentialities in drug delivery. OpenNano 3, 5–17. doi: 10.1016/j.onano.2017.12.001
Cao, J., Cai, Y., Yu, L., and Zhou, J. (2019). Dual physically crosslinked hydrogels based on the synergistic effects of electrostatic and dipole–dipole interactions. J. Mater. Chem. B 7, 676–683. doi: 10.1039/C8TB03032D
Cao, S., Liu, Y., Shang, H., Li, S., Jiang, J., Zhu, X., et al. (2017). Supramolecular nanoparticles of calcitonin and dipeptide for long-term controlled release. J. Controll. Rel. 256, 182–192. doi: 10.1016/j.jconrel.2017.04.014
Carny, O., and Gazit, E. (2005). A model for the role of short self-assembled peptides in the very early stages of the origin of life. FASEB J. 19, 1051–1055. doi: 10.1096/fj.04-3256hyp
Carrejo, N. C., Moore, A. N., Lopez Silva, T. L., Leach, D. G., Li, I.-C., Walker, D. R., et al. (2018). Multidomain peptide hydrogel accelerates healing of full-thickness wounds in diabetic mice. ACS Biomate. Sci. Eng. 4, 1386–1396. doi: 10.1021/acsbiomaterials.8b00031
Carvalho, A., Gallo, J., Pereira, D. M., Valentão, P., Andrade, P. B., Hilliou, L., et al. (2019). Magnetic dehydrodipeptide-based self-assembled hydrogels for theragnostic applications. Nanomaterials 9:541. doi: 10.3390/nano9040541
Chakraborty, P., and Gazit, E. (2018). Amino acid based self-assembled nanostructures: complex structures from remarkably simple building blocks. ChemNanoMat 4, 730–740. doi: 10.1002/cnma.201800147
Chakraborty, P., Tang, Y., Yamamoto, T., Yao, Y., Guterman, T., Zilberzwige-Tal, S., et al. (2020). Unusual two-step assembly of a minimalistic dipeptide-based functional hypergelator. Adv. Mater. 20:1906043. doi: 10.1002/adma.201906043
Chen, C., Liu, K., Li, J., and Yan, X. (2015). Functional architectures based on self-assembly of bio-inspired dipeptides: structure modulation and its photoelectronic applications. Adv. Colloid Interface Sci. 225, 177–193. doi: 10.1016/j.cis.2015.09.001
Chen, J., Qin, S., Wu, X., and Chu,; K, P. (2015). Morphology and pattern control of diphenylalanine self-assembly via evaporative dewetting. ACS Nano 10, 832–838. doi: 10.1021/acsnano.5b05936
Chen, J., and Zou, X. (2019). Self-assemble peptide biomaterials and their biomedical applications. Bioactive Mater. 4, 120–131. doi: 10.1016/j.bioactmat.2019.01.002
Chen, P. (2005). Self-assembly of ionic-complementary peptides: a physicochemical viewpoint. Colloids Surfaces A 261, 3–24. doi: 10.1016/j.colsurfa.2004.12.048
Chen, X., He, Y., Kim, Y., and Lee, M. (2016). Reversible, short α-peptide assembly for controlled capture and selective release of enantiomers. J. Am. Chem. Soc. 138, 5773–5776. doi: 10.1021/jacs.6b02401
Choi, Y. S., and David, A. E. (2014). Cell penetrating peptides and the mechanisms for intracellular entry. Curr. Pharm. Biotechnol. 15, 192–199. doi: 10.2174/1389201015666140617093331
Christofferson, A. J., Al-Garawi, Z. S., Todorova, N., Turner, J., Del Borgo, M. P., Serpell, L. C., et al. (2018). Identifying the coiled-coil triple helix structure of β-peptide nanofibers at atomic resolution. ACS Nano 12, 9101–9109. doi: 10.1021/acsnano.8b03131
Chronopoulou, L., Margheritelli, S., Toumia, Y., Paradossi, G., Bordi, F., Sennato, S., et al. (2015). Biosynthesis and characterization of cross-linked Fmoc peptide-based hydrogels for drug delivery applications. Gels 1, 179–193. doi: 10.3390/gels1020179
Chronopoulou, L., Sennato, S., Bordi, F., Giannella, D., Di Nitto, A., Barbetta, A., et al. (2014). Designing unconventional Fmoc-peptide-based biomaterials: structure and related properties. Soft Matter. 10, 1944–1952. doi: 10.1039/c3sm52457d
Chronopoulou, L., Toumia, Y., Cerroni, B., Pandolfi, D., Paradossi, G., and Palocci, C. (2017). Biofabrication of genipin-crosslinked peptide hydrogels and their use in the controlled delivery of naproxen. New Biotechnol. 37, 138–143. doi: 10.1016/j.nbt.2016.04.006
Cockroft, S. L., Hunter, C. A., Lawson, K. R., Perkins, J., and Urch, C. J. (2005). Electrostatic control of aromatic stacking interactions. J. Am. Chem. Soc. 127, 8594–8595. doi: 10.1021/ja050880n
Copolovici, D. M., Langel, K., Eriste, E., and Langel, U. (2014). Cell-penetrating peptides: design, synthesis, and applications. ACS Nano 8, 1972–1994. doi: 10.1021/nn4057269
Creasey, R. C., Louzao, I., Arnon, Z. A., Marco, P., Adler-Abramovich, L., Roberts, C. J., et al. (2016). Disruption of diphenylalanine assembly by a Boc-modified variant. Soft Matter 12, 9451–9457. doi: 10.1039/C6SM01770C
Cui, H., and Chen, X. (2017). Peptides and peptide conjugates in medicine. Adv. Drug Deliv. Rev. 110, 1–2. doi: 10.1016/j.addr.2017.04.004
Cui, H., Webber, M. J., and Stupp, S. I. (2010). Self-assembly of peptide amphiphiles: from molecules to nanostructures to biomaterials. Peptide Sci. 94, 1–18. doi: 10.1002/bip.21328
Das Mahapatra, R., Dey, J., and Weiss, R. G. (2017). L-Carnosine-derived Fmoc-tripeptides forming pH-sensitive and proteolytically stable supramolecular hydrogels. Langmuir 33, 12989–12999. doi: 10.1021/acs.langmuir.7b03018
Das, P., Pan, I., Cohen, E., and Reches, M. (2018). Self-assembly of a metallo-peptide into a drug delivery system using a “switch on” displacement strategy. J. Mater. Chem. B 6, 8228–8237. doi: 10.1039/C8TB01483C
Datta, D., Harikrishna, A., Nagaraj, R., and Chaudhary, N. (2019). Self-assembly of β-turn motif-connected tandem repeats of Aβ16-22 and its aromatic analogs. Peptide Sci. 111:e24099. doi: 10.1002/pep2.24099
Datta, D., Tiwari, O., and Ganesh, K. N. (2018). New archetypes in self-assembled Phe-Phe motif induced nanostructures from nucleoside conjugated-diphenylalanines. Nanoscale 10, 3212–3224. doi: 10.1039/C7NR08436F
De Luigi, A., Mariani, A., De Paola, M., Depaolini, A. R., Colombo, L., Russo, L., et al. (2015). Doxycycline hinders phenylalanine fibril assemblies revealing a potential novel therapeutic approach in phenylketonuria. Sci. Rep. 5:15902. doi: 10.1038/srep15902
De Santis, E., and Ryadnov, M. G. (2015). Peptide self-assembly for nanomaterials: the old new kid on the block. Chem. Soc. Rev. 44, 8288–8300. doi: 10.1039/C5CS00470E
Dehsorkhi, A., Castelletto, V., and Hamley, I. W. (2014). Self-assembling amphiphilic peptides. J. Peptide Sci. 20, 453–467. doi: 10.1002/psc.2633
Deka, S. R., Yadav, S., Kumar, D., Garg, S., Mahato, M., and Sharma, A. K. (2017). Self-assembled dehydropeptide nano carriers for delivery of ornidazole and curcumin. Colloids Surfaces B 155, 332–340. doi: 10.1016/j.colsurfb.2017.04.036
Del Borgo, M. P., Kulkarni, K., Tonta, M. A., Ratcliffe, J. L., Seoudi, R., Mechler, A. I., et al. (2018). β3-tripeptides act as sticky ends to self-assemble into a bioscaffold. APL Bioeng. 2:026104. doi: 10.1063/1.5020105
Del Borgo, M. P., Mechler, A. I., Traore, D., Forsyth, C., Wilce, J. A., Wilce, M. C., et al. (2013). Supramolecular self-assembly of N-acetyl-capped β-peptides leads to nano-to macroscale fiber formation. Angew. Chem. Int. Edn. 52, 8266–8270. doi: 10.1002/anie.201303175
Devadasu, V. R., Bhardwaj, V., and Kumar, M. R. (2012). Can controversial nanotechnology promise drug delivery? Chem. Rev. 113, 1686–1735. doi: 10.1021/cr300047q
Diaferia, C., Morelli, G., and Accardo, A. (2019). Fmoc-diphenylalanine as suitable building block for the preparation of hybrid materials and their potential applications. J. Mater. Chem. 7, 5142–5155. doi: 10.1039/C9TB01043B
Dinca, A., Chien, W.-M., and Chin, M. T. (2016). Intracellular delivery of proteins with cell-penetrating peptides for therapeutic uses in human disease. Int. J. Mol. Sci. 17:263. doi: 10.3390/ijms17020263
Ding, X., and Wang, Y. (2017). Weak bond-based injectable and stimuli responsive hydrogels for biomedical applications. J. Mater. Chem. B 5, 887–906. doi: 10.1039/C6TB03052A
Ding, Y., Li, Y., Qin, M., Cao, Y., and Wang, W. (2013). Photo-cross-linking approach to engineering small tyrosine-containing peptide hydrogels with enhanced mechanical stability. Langmuir 29, 13299–13306. doi: 10.1021/la4029639
Doane, T., and Burda, C. (2012). Nanoparticle mediated non-covalent drug delivery. Adv. Drug Deliv. Rev. 65:12. doi: 10.1016/j.addr.2012.05.012
Dou, X. Q., and Feng, C. L. (2017). Amino acids and peptide-based supramolecular hydrogels for three-dimensional cell culture. Adv. Mater. 29:1604062. doi: 10.1002/adma.201604062
Draper, E. R., and Adams, D. J. (2018). How should multicomponent supramolecular gels be characterised? Chem. Soc. Rev. 47, 3395–3405. doi: 10.1039/C7CS00804J
Du, X., Zhou, J., Shi, J., and Xu, B. (2015). Supramolecular hydrogelators and hydrogels: from soft matter to molecular biomaterials. Chem. Rev. 115, 13165–13307. doi: 10.1021/acs.chemrev.5b00299
Dube, T., Mandal, S., and Jyoti Panda, J. (2017). Nanoparticles generated from a tryptophan derivative: physical characterization and anti-cancer drug delivery. Amino Acids 49:8. doi: 10.1007/s00726-017-2403-8
Edwards-Gayle, C. J., and Hamley, I. W. (2017). Self-assembly of bioactive peptides, peptide conjugates, and peptide mimetic materials. Organ. Biomol. Chem. 15, 5867–5876. doi: 10.1039/C7OB01092C
Emtiazi, G., Zohrabi, T., Lee, L. Y., Habibi, N., and Zarrabi, A. (2017). Covalent diphenylalanine peptide nanotube conjugated to folic acid/magnetic nanoparticles for anti-cancer drug delivery. J. Drug Deliv. Sci. Technol. 41, 90–98. doi: 10.1016/j.jddst.2017.06.005
Erdogan, H., Babur, E., Yilmaz, M., Candas, E., Gordesel, M., Dede, Y., et al. (2015). Morphological versatility in the self-assembly of val-ala and ala-val dipeptides. Langmuir 31, 7337–7345. doi: 10.1021/acs.langmuir.5b01406
Erdogan, H., Yilmaz, M., Babur, E., Duman, M., Aydin, H. M., and Demirel, G. (2016). Fabrication of plasmonic nanorod-embedded dipeptide microspheres via the freeze-quenching method for near-infrared laser-triggered drug-delivery applications. Biomacromolecules 17, 1788–1794. doi: 10.1021/acs.biomac.6b00214
Eskandari, S., Guerin, T., Toth, I., and Stephenson, R. J. (2017). Recent advances in self-assembled peptides: implications for targeted drug delivery and vaccine engineering. Adv. Drug Deliv. Rev. 110, 169–187. doi: 10.1016/j.addr.2016.06.013
Fan, T., Yu, X., Shen, B., and Sun, L. (2017). Peptide self-assembled nanostructures for drug delivery applications. J. Nanomater. 2017:4562474. doi: 10.1155/2017/4562474
Fan, Z., Sun, L., Huang, Y., Wang, Y., and Zhang, M. (2016). Bioinspired fluorescent dipeptide nanoparticles for targeted cancer cell imaging and real-time monitoring of drug release. Nat. Nanotechnol. 11:388. doi: 10.1038/nnano.2015.312
Fichman, G., and Gazit, E. (2014). Self-assembly of short peptides to form hydrogels: design of building blocks, physical properties and technological applications. Acta Biomaterialia 10, 1671–1682. doi: 10.1016/j.actbio.2013.08.013
Fichman, G., Guterman, T., Adler-Abramovich, L., and Gazit, E. (2015). Synergetic functional properties of two-component single amino acid-based hydrogels. CrystEngComm 17, 8105–8112. doi: 10.1039/C5CE01051A
Fleming, S., Debnath, S., Frederix, P. W., Hunt, N. T., and Ulijn, R. V. (2014). Insights into the coassembly of hydrogelators and surfactants based on aromatic peptide amphiphiles. Biomacromolecules 15, 1171–1184. doi: 10.1021/bm401720z
Fleming, S., Debnath, S., Frederix, P. W., Tuttle, T., and Ulijn, R. V. (2013). Aromatic peptide amphiphiles: significance of the Fmoc moiety. Chem. Commun. 49, 10587–10589. doi: 10.1039/c3cc45822a
Fleming, S., and Ulijn, R. V. (2014). Design of nanostructures based on aromatic peptide amphiphiles. Chem. Soc. Rev. 43, 8150–8177. doi: 10.1039/C4CS00247D
Fu, I. W., Markegard, C. B., Chu, B. K., and Nguyen, H. D. (2014). Role of hydrophobicity on self-assembly by peptide amphiphiles via molecular dynamics simulations. Langmuir 30, 7745–7754. doi: 10.1021/la5012988
Fu, Y., Li, B., Huang, Z., Li, Y., and Yang, Y. (2013). Terminal is important for the helicity of the self-assemblies of dipeptides derived from alanine. Langmuir 29, 6013–6017. doi: 10.1021/la400910g
Galdiero, S., and Gomes, P. A. (2017). Peptide-based drugs and drug delivery systems. Molecules 22:2185. doi: 10.3390/molecules22122185
Gallo, M., Defaus, S., and Andreu, D. (2019). 1988-2018: Thirty years of drug smuggling at the nano scale. Challenges and opportunities of cell-penetrating peptides in biomedical research. Arch. Biochem. Biophys. 661, 74–86. doi: 10.1016/j.abb.2018.11.010
Gao, H.-Y., Wagner, H., Held, P. A., Du, S., Gao, H.-J., Studer, A., et al. (2015). In-plane Van der Waals interactions of molecular self-assembly monolayer. Appl. Phys. Lett. 106:081606. doi: 10.1063/1.4907777
Gao, J., Zhan, J., and Yang, Z. (2019). Enzyme-Instructed Self-Assembly (EISA) and hydrogelation of peptides. Adv. Mater. 32:1805798. doi: 10.1002/adma.201805798
Garcia, A. M., Iglesias, D., Parisi, E., Styan, K. E., Waddington, L. J., Deganutti, C., et al. (2018). Chirality effects on peptide self-assembly unraveled from molecules to materials. Chemistry 4, 1862–1876. doi: 10.1016/j.chempr.2018.05.016
Gavel, P. K., Dev, D., Parmar, H. S., Bhasin, S., and Das, A. K. (2018a). Investigations of peptide-based biocompatible injectable shape-memory hydrogels: differential biological effects on bacterial and human blood cells. ACS Appl. Mater. Interfaces 10, 10729–10740. doi: 10.1021/acsami.8b00501
Gavel, P. K., Parmar, H. S., Tripathi, V., Kumar, N., Biswas, A., and Das, A. K. (2018b). Investigations of anti-inflammatory activity of a peptide-based hydrogel using rat air pouch model. ACS Appl. Mater. Interfaces 11, 2849–2859. doi: 10.1021/acsami.8b19228
Gazit, E. (2016). Peptide nanostructures: aromatic dipeptides light up. Nat. Nanotechnol. 11:309. doi: 10.1038/nnano.2015.321
Gazit, E. (2018). Reductionist approach in peptide-based nanotechnology. Ann. Rev. Biochem. 87, 533–553. doi: 10.1146/annurev-biochem-062917-012541
Genji, M., Yano, Y., Hoshino, M., and Matsuzaki, K. (2017). Aromaticity of phenylalanine residues is essential for amyloid formation by Alzheimer's amyloid β-peptide. Chem. Pharm. Bull. 65, 668–673. doi: 10.1248/cpb.c17-00203
Ghosh, M., Halperin-Sternfeld, M., Grigoriants, I., Lee, J., Nam, K. T., and Adler-Abramovich, L. (2017). Arginine-presenting peptide hydrogels decorated with hydroxyapatite as biomimetic scaffolds for bone regeneration. Biomacromolecules 18, 3541–3550. doi: 10.1021/acs.biomac.7b00876
Ginjupalli, K., Shavi, G. V., Averineni, R. K., Bhat, M., Udupa, N., and Upadhya, P. N. (2017). Poly (α-hydroxy acid) based polymers: a review on material and degradation aspects. Polymer Degradation Stabil. 144, 520–535. doi: 10.1016/j.polymdegradstab.2017.08.024
Goel, R., Gopal, S., and Gupta, A. (2015). Self-assembly of β-alanine homotetramer: formation of nanovesicles for drug delivery. J. Mater. Chem. B 3, 5849–5857. doi: 10.1039/C5TB00652J
González-Díaz, N. E., López-Rendón, R., and Ireta, J. (2019). Insight into the dipeptide self-assembly process using density functional theory. J. Phys. Chem. C 123, 2526–2532. doi: 10.1021/acs.jpcc.8b10340
Gopalan, R. D., Del Borgo, M. P., Mechler, A. I., Perlmutter, P., and Aguilar, M.-I. (2015). Geometrically precise building blocks: the self-assembly of β-peptides. Chem. Biol. 22, 1417–1423. doi: 10.1016/j.chembiol.2015.10.005
Goyal, N., Mangunuru, H. P., Parikh, B., Shrestha, S., and Wang, G. (2014). Synthesis and characterization of pH responsive D-glucosamine based molecular gelators. Beilstein J. Organic Chem. 10, 3111–3121. doi: 10.3762/bjoc.10.328
Guidotti, G., Brambilla, L., and Rossi, D. (2017). Cell-penetrating peptides: from basic research to clinics. Trends Pharm. Sci. 38, 406–424. doi: 10.1016/j.tips.2017.01.003
Guo, C., Arnon, Z. A., Qi, R., Zhang, Q., Adler-Abramovich, L., Gazit, E., et al. (2016). Expanding the nanoarchitectural diversity through aromatic di-and tri-peptide coassembly: Nanostructures and molecular mechanisms. ACS nano 10, 8316–8324. doi: 10.1021/acsnano.6b02739
Guo, C., Luo, Y., Zhou, R., and Wei, G. (2012). Probing the self-assembly mechanism of diphenylalanine-based peptide nanovesicles and nanotubes. ACS nano, 6, 3907–3918. doi: 10.1021/nn300015g
Guo, Z., Peng, H., Kang, J., and Sun, D. (2016). Cell-penetrating peptides: possible transduction mechanisms and therapeutic applications. Biomed. Rep. 4, 528–534. doi: 10.3892/br.2016.639
Gupta, M., and Chauhan, V. S. (2011). De novo design of α, β-didehydrophenylalanine containing peptides: from models to applications. Biopolymers 95, 161–173. doi: 10.1002/bip.21561
Guterman, T., Kornreich, M., Stern, A., Adler-Abramovich, L., Porath, D., Beck, R., et al. (2016). Formation of bacterial pilus-like nanofibres by designed minimalistic self-assembling peptides. Nat. Commun. 7, 1–10. doi: 10.1038/ncomms13482
Habibi, N., Kamaly, N., Memic, A., and Shafiee, H. (2016). Self-assembled peptide-based nanostructures: smart nanomaterials toward targeted drug delivery. Nano Today 11, 41–60. doi: 10.1016/j.nantod.2016.02.004
Halperin-Sternfeld, M., Ghosh, M., Sevostianov, R., Grigoriants, I., and Adler-Abramovich, L. (2017). Molecular co-assembly as a strategy for synergistic improvement of the mechanical properties of hydrogels. Chem. Commun. 53, 9586–9589. doi: 10.1039/C7CC04187J
Hamley, I. W. (2017). Small bioactive peptides for biomaterials design and therapeutics. Chem. Rev. 117, 14015–14041. doi: 10.1021/acs.chemrev.7b00522
Handelman, A., Apter, B., Turko, N., and Rosenman, G. (2016a). Linear and nonlinear optical waveguiding in bio-inspired peptide nanotubes. Acta Biomaterialia 30, 72–77. doi: 10.1016/j.actbio.2015.11.004
Handelman, A., Kuritz, N., Natan, A., and Rosenman, G. (2016b). Reconstructive phase transition in ultrashort peptide nanostructures and induced visible photoluminescence. Langmuir 32, 2847–2862. doi: 10.1021/acs.langmuir.5b02784
He, M., Li, J., Tan, S., Wang, R., and Zhang, Y. (2013). Photodegradable supramolecular hydrogels with fluorescence turn-on reporter for photomodulation of cellular microenvironments. J. Am. Chem. Soc. 135, 18718–18721. doi: 10.1021/ja409000b
He, R., Finan, B., Mayer, J. P., and DiMarchi, R. D. (2019). Peptide conjugates with small molecules designed to enhance efficacy and safety. Molecules 24:1855. doi: 10.3390/molecules24101855
Hoffmann, K., Milech, N., Juraja, S. M., Cunningham, P. T., Stone, S. R., Francis, R. W., et al. (2018). A platform for discovery of functional cell-penetrating peptides for efficient multi-cargo intracellular delivery. Sci. Rep. 8:12538. doi: 10.1038/s41598-018-30790-2
Hsieh, W.-H., and Liaw, J. (2019). Applications of cyclic peptide nanotubes (cPNTs). J. Food Drug Analysis 27, 32–47. doi: 10.1016/j.jfda.2018.09.004
Huang, R., Wang, Y., Qi, W., Su, R., and He, Z. (2014). Temperature-induced reversible self-assembly of diphenylalanine peptide and the structural transition from organogel to crystalline nanowires. Nanoscale Res. Lett. 9, 1–9. doi: 10.1186/1556-276X-9-653
Huang, Y.-W., Lee, H.-J., Tolliver, L. M., and Aronstam, R. S. (2015). Delivery of nucleic acids and nanomaterials by cell-penetrating peptides: opportunities and challenges. BioMed Res. Int. 2015:834079. doi: 10.1155/2015/834079
Huang, Z., Guan, S., Wang, Y., Shi, G., Cao, L., Gao, Y., et al. (2013). Self-assembly of amphiphilic peptides into bio-functionalized nanotubes: a novel hydrolase model. J. Mater. Chem. B 1, 2297–2304. doi: 10.1039/c3tb20156b
Iglesias, D., and Marchesan, S. (2017). “Short peptide self-assembled nanostructures for therapeutics innovative delivery,” in Nanostructures for Novel Therapy, eds D. Ficai and A. M. Grumezescu (Amsterdam: Elsevier), 227–250. doi: 10.1016/B978-0-323-46142-9.00009-8
Inaba, H., and Matsuura, K. (2019). Peptide nanomaterials designed from natural supramolecular systems. Chem. Record 19, 843–858. doi: 10.1002/tcr.201800149
Insua, I., and Montenegro, J. (2019). 1D to 2D self assembly of cyclic peptides. J. Am. Chem. Soc. 142, 300–307. doi: 10.1021/jacs.9b10582
Ischakov, R., Adler-Abramovich, L., Buzhansky, L., Shekhter, T., and Gazit, E. (2013). Peptide-based hydrogel nanoparticles as effective drug delivery agents. Bioorgan. Med. Chem. 21, 3517–3522. doi: 10.1016/j.bmc.2013.03.012
Jeon, J., Mills, C. E., and Shell, M. S. (2013). Molecular insights into diphenylalanine nanotube assembly: all-atom simulations of oligomerization. J. Phys. Chem. B 117, 3935–3943. doi: 10.1021/jp308280d
Jeong, W.-J., Bu, J., Kubiatowicz, L. J., Chen, S. S., Kim, Y., and Hong, S. (2018). Peptide–nanoparticle conjugates: a next generation of diagnostic and therapeutic platforms? Nano Converg. 5:38. doi: 10.1186/s40580-018-0170-1
Ji, W., Yuan, C., Zilberzwige-Tal, S., Xing, R., Chakraborty, P., Tao, K., et al. (2019). Metal-ion modulated structural transformation of amyloid-like dipeptide supramolecular self-assembly. ACS Nano 13, 7300–7309. doi: 10.1021/acsnano.9b03444
Jia, F., Liu, X., Li, L., Mallapragada, S., Narasimhan, B., and Wang, Q. (2013). Multifunctional nanoparticles for targeted delivery of immune activating and cancer therapeutic agents. J. Controll. Rel. 172, 1020–1034. doi: 10.1016/j.jconrel.2013.10.012
Jiang, Z., Guan, J., Qian, J., and Zhan, C. (2019). Peptide ligand-mediated targeted drug delivery of nanomedicines. Biomater. Sci. 7, 461–471. doi: 10.1039/C8BM01340C
Kamei, N., Khafagy, E.-S., Hirose, J., and Takeda-Morishita, M. (2017). Potential of single cationic amino acid molecule “Arginine” for stimulating oral absorption of insulin. Int. J. Pharmaceutics 521, 176–183. doi: 10.1016/j.ijpharm.2017.01.066
Kamei, N., Tamiwa, H., Miyata, M., Haruna, Y., Matsumura, K., Ogino, H., et al. (2018). Hydrophobic amino acid tryptophan shows promise as a potential absorption enhancer for oral delivery of biopharmaceuticals. Pharmaceutics 10:182. doi: 10.3390/pharmaceutics10040182
Kang, Z., Meng, Q., and Liu, K. (2019). Peptide-based gene delivery vectors. J. Mater. Chem. B 7, 1824–1841. doi: 10.1039/C8TB03124J
Khatri, A., Mishra, A., and Chauhan, V. S. (2017). Characterization of DNA condensation by conformationally restricted dipeptides and gene delivery. J. Biomed. Nanotechnol. 13, 35–53. doi: 10.1166/jbn.2017.2325
Khatri, A., Siddiqui, H., Panda, J., and Chauhan, V. (2019). Delivery of cancer-testis antigens using self-assembled dipeptide nanotubes inhibits tumor growth in mice melanoma model. J. Nanomed. Nanotechnol. 10:2. doi: 10.35248/2157-7439.19.10.533
Kieffer, M., Garcia, A. M., Haynes, C. J., Kralj, S., Iglesias, D., Nitschke, J. R., et al. (2019). Embedding and positioning of Two FeII4L4 cages in supramolecular tripeptide gels for selective chemical segregation. Angew. Chem. 58, 7982–7986. doi: 10.1002/anie.201900429
Kim, S. H., Kaplan, J. A., Sun, Y., Shieh, A., Sun, H. L., Croce, C. M., et al. (2015). The self-assembly of anticancer camptothecin–dipeptide nanotubes: a minimalistic and high drug loading approach to increased efficacy. Chem. A Eur. J. 21, 101–105. doi: 10.1002/chem.201404520
Kirkham, S., Hamley, I. W., Smith, A. M., Gouveia, R. M., Connon, C. J., Reza, M., et al. (2016). A self-assembling fluorescent dipeptide conjugate for cell labelling. Colloids Surfaces B Biointerfaces 137, 104–108. doi: 10.1016/j.colsurfb.2015.04.062
Kol, N., Adler-Abramovich, L., Barlam, D., Shneck, R. Z., Gazit, E., and Rousso, I. (2005). Self-assembled peptide nanotubes are uniquely rigid bioinspired supramolecular structures. Nano Lett. 5, 1343–1346. doi: 10.1021/nl0505896
Komáromy, D. v, Stuart, M. C., Monreal Santiago, G., Tezcan, M., Krasnikov, V. V., et al. (2017). Self-assembly can direct dynamic covalent bond formation toward diversity or specificity. J. Am. Chem. Soc. 139, 6234–6241. doi: 10.1021/jacs.7b01814
Kou, L., Bhutia, Y. D., Yao, Q., He, Z., Sun, J., and Ganapathy, V. (2018). Transporter-guided delivery of nanoparticles to improve drug permeation across cellular barriers and drug exposure to selective cell types. Front. Pharmacol. 9:27. doi: 10.3389/fphar.2018.00027
Koutsopoulos, S. (2016). Self-assembling peptide nanofiber hydrogels in tissue engineering and regenerative medicine: progress, design guidelines, and applications. J. Biomed. Mater. Res. Part A 104, 1002–1016. doi: 10.1002/jbm.a.35638
Kuang, Y., and Xu, B. (2013). Disruption of the dynamics of microtubules and selective inhibition of glioblastoma cells by nanofibers of small hydrophobic molecules. Angew. Chem. Int. Edn. 52, 6944–6948. doi: 10.1002/anie.201302658
Kulkarni, K., Habila, N., Del Borgo, M. P., and Aguilar, M.-I. (2019). Novel materials from the supramolecular self-assembly of short helical β3-peptide foldamers. Front. Chem. 7:70. doi: 10.3389/fchem.2019.00070
Kumar, V. A., Taylor, N. L., Shi, S., Wickremasinghe, N. C., D'Souza, R. N., and Hartgerink, J. D. (2015). Self-assembling multidomain peptides tailor biological responses through biphasic release. Biomaterials 52, 71–78. doi: 10.1016/j.biomaterials.2015.01.079
Kurbasic, M., Romano, C., Garcia, A., Kralj, S., and Marchesan, S. (2017). Assembly of a Tripeptide and anti-inflammatory drugs into supramolecular hydrogels for sustained release. Gels 3:29. doi: 10.3390/gels3030029
Kurrikoff, K., Gestin, M., and Langel, Ü. (2016). Recent in vivo advances in cell-penetrating peptide-assisted drug delivery. Exp. Opin. Drug Deliv. 13, 373–387. doi: 10.1517/17425247.2016.1125879
Lakshmanan, A., Cheong, D. W., Accardo, A., Di Fabrizio, E., Riekel, C., and Hauser, C. A. (2013). Aliphatic peptides show similar self-assembly to amyloid core sequences, challenging the importance of aromatic interactions in amyloidosis. Proc. Natl. Acad. Sci. U.S.A. 110, 519–524. doi: 10.1073/pnas.1217742110
Lee, J. H. (2018). Injectable hydrogels delivering therapeutic agents for disease treatment and tissue engineering. Biomaterials research 22, 27. doi: 10.1186/s40824-018-0138-6
Lee, S., Trinh, T. H., Yoo, M., Shin, J., Lee, H., Kim, J., et al. (2019). Self-assembling peptides and their application in the treatment of diseases. Int. J. Mol. Sci. 20:5850. doi: 10.3390/ijms20235850
Lee, Y.-H., Chang, S.-F., and Liaw, J. (2015). Anti-apoptotic gene delivery with cyclo-(d-Trp-Tyr) peptide nanotube via eye drop following corneal epithelial debridement. Pharmaceutics 7, 122–136. doi: 10.3390/pharmaceutics7030122
Lehto, T., Ezzat, K., Wood, M. J. A., and El Andaloussi, S. (2016). Peptides for nucleic acid delivery. Adv. Drug Deliv. Rev. 106, 172–182. doi: 10.1016/j.addr.2016.06.008
Leite, D., Barbu, E., Pilkington, G., and Lalatsa, A. (2015). Peptide self-assemblies for drug delivery. Curr. Top. Med. Chem. 15:120456. doi: 10.2174/1568026615666150605120456
León, I., Alonso, E. R., Cabezas, C., Mata, S., and Alonso, J. L. (2019). Unveiling the n → π* interactions in dipeptides. Commun. Chem. 2:3. doi: 10.1038/s42004-018-0103-2
Levin, A., Mason, T. O., Adler-Abramovich, L., Buell, A. K., Meisl, G., Galvagnion, C., et al. (2014). Ostwald's rule of stages governs structural transitions and morphology of dipeptide supramolecular polymers. Nat. Commun. 5:5219. doi: 10.1038/ncomms6219
Levin, A., Michaels, T. C., Mason, T. O., Müller, T., Adler-Abramovich, L., Mahadevan, L., et al. (2018). Self-assembly-mediated release of peptide nanoparticles through jets across microdroplet interfaces. ACS Appl. Mater. Interfaces 10, 27578–27583. doi: 10.1021/acsami.8b09511
Levine, R. M., Scott, C. M., and Kokkoli, E. (2013). Peptide functionalized nanoparticles for nonviral gene delivery. Soft Matter. 9, 985–1004. doi: 10.1039/C2SM26633D
Li, I.-C., and Hartgerink, J. D. (2017). Covalent capture of aligned self-assembling nanofibers. J. Am. Chem. Soc. 139, 8044–8050. doi: 10.1021/jacs.7b04655
Li, I. C., Moore, A. N., and Hartgerink, J. D. (2016). “Missing tooth” multidomain peptide nanofibers for delivery of small molecule drugs. Biomacromolecules 17, 2087–2095. doi: 10.1021/acs.biomac.6b00309
Li, J., Du, X., Hashim, S., Shy, A., and Xu, B. (2017). Aromatic–aromatic interactions enable α-Helix to β-sheet transition of peptides to form supramolecular hydrogels. J. Am. Chem. Soc. 139, 71–74. doi: 10.1021/jacs.6b11512
Li, J., Kuang, Y., Gao, Y., Du, X., Shi, J., and Xu, B. (2012). D-amino acids boost the selectivity and confer supramolecular hydrogels of a nonsteroidal anti-inflammatory drug (NSAID). J. Am. Chem. Soc. 135, 542–545. doi: 10.1021/ja310019x
Li, J., Kuang, Y., Shi, J., Gao, Y., Zhou, J., and Xu, B. (2013). The conjugation of nonsteroidal anti-inflammatory drugs (NSAID) to small peptides for generating multifunctional supramolecular nanofibers/hydrogels. Beilstein J. Organ Chem. 9, 908–917. doi: 10.3762/bjoc.9.104
Li, J., Wang, A., Ren, P., Yan, X., and Bai, S. (2019a). One-step co-assembly method to fabricate photosensitive peptide nanoparticles for two-photon photodynamic therapy. Chem. Commun. 55, 3191–3194. doi: 10.1039/C9CC00025A
Li, J., Wang, A., Zhao, L., Dong, Q., Wang, M., Xu, H., et al. (2018). Self-assembly of monomeric hydrophobic photosensitizers with short peptides forming photodynamic nanoparticles with real-time tracking property and without the need of release in vivo. ACS Appl. Mater. Interfaces 10, 28420–28427. doi: 10.1021/acsami.8b09933
Li, J., Xing, R., Bai, S., and Yan, X. (2019b). Recent advances of self-assembling peptide-based hydrogels for biomedical applications. Soft Matter. 15, 1704–1715. doi: 10.1039/C8SM02573H
Li, L. L., Qiao, Z. Y., Wang, L., and Wang, H. (2018). Programmable construction of peptide-based materials in living subjects: from modular design and morphological control to theranostics. Adv. Mater.20:1804971. doi: 10.1002/adma.201804971
Li, Q., Chen, M., Chen, D., and Wu, L. (2016). One-pot synthesis of diphenylalanine-based hybrid nanospheres for controllable pH- and GSH-responsive delivery of drugs. Chem. Mater. 28, 6584–6590. doi: 10.1021/acs.chemmater.6b02604
Li, Q., Jia, Y., Dai, L., Yang, Y., and Li, J. (2015). Controlled rod nanostructured assembly of diphenylalanine and their optical waveguide properties. ACS Nano 9, 2689–2695. doi: 10.1021/acsnano.5b00623
Li, S., Zou, Q., Li, Y., Yuan, C., Xing, R., and Yan, X. (2018). Smart peptide-based supramolecular photodynamic metallo-nanodrugs designed by multicomponent coordination self-assembly. J. Am. Chem. Soc. 140, 10794–10802. doi: 10.1021/jacs.8b04912
Li, Y., Wang, F., and Cui, H. (2016). Peptide-based supramolecular hydrogels for delivery of biologics. Bioeng. Transl. Med. 1, 306–322. doi: 10.1002/btm2.10041
Li, Z., Cao, J., Li, H., Liu, H., Han, F., Liu, Z., et al. (2016). Self-assembled drug delivery system based on low-molecular-weight bis-amide organogelator: synthesis, properties and in vivo evaluation. Drug Deliv. 23, 3168–3178. doi: 10.3109/10717544.2016.1157841
Lian, M., Chen, X., Lu, Y., and Yang, W. (2016). Self-assembled peptide hydrogel as a smart biointerface for enzyme-based electrochemical biosensing and cell monitoring. ACS Appl. Mater. Interfaces 8, 25036–25042. doi: 10.1021/acsami.6b05409
Lian, M., Xu, L., Zhu, X., Chen, X., Yang, W., and Wang, T. (2017). Seamless signal transduction from three-dimensional cultured cells to a superoxide anions biosensor via in situ self-assembly of dipeptide hydrogel. Anal. Chem. 89, 12843–12849. doi: 10.1021/acs.analchem.7b03371
Liao, H.-S., Lin, J., Liu, Y., Huang, P., Jin, A., and Chen, X. (2016). Self-assembly mechanisms of nanofibers from peptide amphiphiles in solution and on substrate surfaces. Nanoscale 8, 14814–14820. doi: 10.1039/C6NR04672J
Liu, K., Xing, R., Zou, Q., Ma, G., Möhwald, H., and Yan, X. (2016). Simple peptide-tuned self-assembly of photosensitizers towards anticancer photodynamic therapy. Angew. Chem. Int. Edn. 55, 3036–3039. doi: 10.1002/anie.201509810
Liu, Y., Zhang, L., and Wei, W. (2017). Effect of noncovalent interaction on the self-assembly of a designed peptide and its potential use as a carrier for controlled bFGF release. Int. J. Nanomed. 12:659. doi: 10.2147/IJN.S124523
Liyanage, W., Vats, K., Rajbhandary, A., Benoit, D. S., and Nilsson, B. L. (2015). Multicomponent dipeptide hydrogels as extracellular matrix-mimetic scaffolds for cell culture applications. Chem. Commun. 51, 11260–11263. doi: 10.1039/C5CC03162A
Lock, L. L., LaComb, M., Schwarz, K., Cheetham, A. G., Lin, Y.-A., Zhang, P., et al. (2013). Self-assembly of natural and synthetic drug amphiphiles into discrete supramolecular nanostructures. Faraday Discuss. 166, 285–301. doi: 10.1039/c3fd00099k
Loic, S. (2017). Amino Acids Modification to Improve and Fine-Tune Peptide-Based Hydrogels in Amino Acid-New Insights and Roles in Plant and Animal. London, UK: IntechOpen. doi: 10.5772/intechopen.68705
Lombardi, L., Falanga, A., Del Genio, V., and Galdiero, S. (2019). A new hope: self-assembling peptides with antimicrobial activity. Pharmaceutics 11:166. doi: 10.3390/pharmaceutics11040166
Lombardo, D., Calandra, P., Pasqua, L., and Magazù, S. (2020). Self-assembly of organic nanomaterials and biomaterials: the bottom-up approach for functional nanostructures formation and advanced applications. Materials 13:1048. doi: 10.3390/ma13051048
Loo, Y., Goktas, M., Tekinay, A. B., Guler, M. O., Hauser, C. A., and Mitraki, A. (2015). Self-assembled proteins and peptides as scaffolds for tissue regeneration. Adv. Healthcare Mater. 4, 2557–2586. doi: 10.1002/adhm.201500402
Lopez-Silva, T. L., Leach, D. G., Li, I.-C., Wang, X., and Hartgerink, J. D. (2018). Self-assembling multidomain peptides: design and characterization of neutral peptide-based materials with ph and ionic strength independent self-assembly. ACS Biomater. Sci. Eng. 5, 977–985. doi: 10.1021/acsbiomaterials.8b01348
Luisi, P. L. (2015). Chemistry constraints on the origin of life. Israel J. Chem. 55, 906–918. doi: 10.1002/ijch.201400177
Ma, L., Wang, C., He, Z., Cheng, B., Zheng, L., and Huang, K. (2017). Peptide-drug conjugate: a novel drug design approach. Curr. Med. Chem. 24, 3373–3396. doi: 10.2174/0929867324666170404142840
Majid, A., Patil-Sen, Y., Ahmed, W., and Sen, T. (2017). Tunable self-assembled peptide structure: a novel approach to design dual-use biological agents. Mater. Today Proc. 4, 32–40. doi: 10.1016/j.matpr.2017.01.190
Makam, P., and Gazit, E. (2018). Minimalistic peptide supramolecular co-assembly: expanding the conformational space for nanotechnology. Chem. Soc. Rev. 47, 3406–3420. doi: 10.1039/C7CS00827A
Manchineella, S., Murugan, N. A., and Govindaraju, T. (2017). Cyclic dipeptide-based ambidextrous supergelators: minimalistic rational design, structure-gelation studies, and in situ hydrogelation. Biomacromolecules 18, 3581–3590. doi: 10.1021/acs.biomac.7b00924
Mandal, D., Shirazi, A. N., and Parang, K. (2014). Self-assembly of peptides to nanostructures. Organ. Biomol. Chem. 12, 3544–3561. doi: 10.1039/C4OB00447G
Mándity, I. M., Monsignori, A., Fülöp, L., Forr,ó, E., and Fülöp, F. (2014). Exploiting aromatic interactions for β-peptide foldamer helix stabilization: a significant design element. Chem. Eur. J. 20, 4591–4597. doi: 10.1002/chem.201304448
Marchesan, S., Styan, K., Easton, C., Waddington, L., and Vargiu, A. V. (2015a). Higher and lower supramolecular orders for the design of self-assembled heterochiral tripeptide hydrogel biomaterials. J. Mater. Chem. B 3, 8123–8132. doi: 10.1039/C5TB00858A
Marchesan, S., Vargiu, A. V., and Styan, K. E. (2015b). The Phe-Phe Motif for Peptide Self-assembly in nanomedicine. Molecules 20, 19775–19788. doi: 10.3390/molecules201119658
Mart, R. J., Osborne, R. D., Stevens, M. M., and Ulijn, R. V. (2006). Peptide-based stimuli-responsive biomaterials. Soft Matter. 2, 822–835. doi: 10.1039/b607706d
Martin, A. D., Wojciechowski, J. P., Warren, H., and Thordarson, P. (2016). Effect of heterocyclic capping groups on the self-assembly of a dipeptide hydrogel. Soft Matter. 12, 2700–2707. doi: 10.1039/C6SM00025H
Mason, T. O., and Buell, A. K. (2019). “The kinetics, thermodynamics and mechanisms of short aromatic peptide self-assembly,” in Biological and Bio-Inspired Nanomaterials, eds S. Perrett, A. K. Buell, and T. Knowles (Singapore: Springer), 61–112. doi: 10.1007/978-981-13-9791-2_3
Mason, T. O., Michaels, T. C., Levin, A., Dobson, C. M., Gazit, E., Knowles, T. P., et al. (2017). Thermodynamics of polypeptide supramolecular assembly in the short-chain limit. J. Am. Chem. Soc. 139, 16134–16142. doi: 10.1021/jacs.7b00229
Mateescu, M., Ispas-Szabo, P., and Assaad, E. (2015). “The concept of self-assembling and the interactions involved,” in Controlled Drug Delivery, eds M. A. Mateescu, P. Ispas-Szabo, and E. Assaad (Cambridge, UK: Elsevier), 1–20.
Matson, J. B., and Stupp, S. I. (2012). Self-assembling peptide scaffolds for regenerative medicine. Chem. Commun. 48, 26–33. doi: 10.1039/C1CC15551B
Maude, S., Ingham, E., and Aggeli, A. (2013). Biomimetic self-assembling peptides as scaffolds for soft tissue engineering. Nanomedicine 8, 823–847. doi: 10.2217/nnm.13.65
Mauri, E., Chincarini, G. M., Rigamonti, R., Magagnin, L., Sacchetti, A., and Rossi, F. (2017). Modulation of electrostatic interactions to improve controlled drug delivery from nanogels. Mater. Sci. Eng. C 72, 308–315. doi: 10.1016/j.msec.2016.11.081
Maury, C. P. J. (2018). Amyloid and the origin of life: self-replicating catalytic amyloids as prebiotic informational and protometabolic entities. Cell. Mol. Life Sci. 75, 1499–1507. doi: 10.1007/s00018-018-2797-9
Mayr, J., Saldías, C., and Díaz, D. D. (2018). Release of small bioactive molecules from physical gels. Chem. Soc. Rev. 47, 1484–1515. doi: 10.1039/C7CS00515F
Mears, L. L., Draper, E. R., Castilla, A. M., Su, H., Dietrich, B., Nolan, M. C., et al. (2017). Drying affects the fiber network in low molecular weight hydrogels. Biomacromolecules 18, 3531–3540. doi: 10.1021/acs.biomac.7b00823
Melchionna, M., E Styan, K., and Marchesan, S. (2016). The unexpected advantages of using D-amino acids for peptide self-assembly into nanostructured hydrogels for medicine. Curr. Top. Med. Chem. 16, 2009–2018. doi: 10.2174/1568026616999160212120302
Mendes, A. C., Baran, E. T., Reis, R. L., and Azevedo, H. S. (2013). Self-assembly in nature: using the principles of nature to create complex nanobiomaterials. Wiley Interdisc. Rev. 5, 582–612. doi: 10.1002/wnan.1238
Mikhalevich, V., Craciun, I., Kyropoulou, M., Palivan, C. G., and Meier, W. (2017). Amphiphilic peptide self-assembly: expansion to hybrid materials. Biomacromolecules 18, 3471–3480. doi: 10.1021/acs.biomac.7b00764
Mishra, J., and Jyoti Panda, J. (2019). Short peptide-based smart targeted cancer nanotherapeutics: a glimmer of hope. Therap. Deliv. 10:5. doi: 10.4155/tde-2019-0005
Moitra, P., Kumar, K., Kondaiah, P., and Bhattacharya, S. (2014). efficacious anticancer drug delivery mediated by a ph-sensitive self-assembly of a conserved tripeptide derived from tyrosine kinase NGF receptor. Angew. Chem. Int. Edn. 53, 1113–1117. doi: 10.1002/anie.201307247
Moitra, P., Subramanian, Y., and Bhattacharya, S. (2017). Concentration dependent self-assembly of TrK-NGF receptor derived tripeptide: new insights from experiment and computer simulations. J. Phys. Chem. B 121, 815–824. doi: 10.1021/acs.jpcb.6b10511
Mondal, S., Das, S., and Nandi, A. K. (2020). A review on recent advances in polymer and peptide hydrogels. Soft Matter. 16, 1404–1454 doi: 10.1039/C9SM02127B
Moore, A. N., and Hartgerink, J. D. (2017). Self-assembling multidomain peptide nanofibers for delivery of bioactive molecules and tissue regeneration. Accounts Chem. Res. 50, 714–722. doi: 10.1021/acs.accounts.6b00553
Morris, K. L., Chen, L., Raeburn, J., Sellick, O. R., Cotanda, P., Paul, A., et al. (2013). Chemically programmed self-sorting of gelator networks. Nat. Commun. 4:1480. doi: 10.1038/ncomms2499
Mossou, E., Teixeira, S. C., Mitchell, E. P., Mason, S. A., Adler-Abramovich, L., Gazit, E., et al. (2014). The self-assembling zwitterionic form of L-phenylalanine at neutral pH. Acta Crystallograph. C 70, 326–331. doi: 10.1107/S2053229614002563
Motamed, S., Del Borgo, M., Kulkarni, K., Habila, N., Zhou, K., Perlmutter, P., et al. (2016). A self-assembling β-peptide hydrogel for neural tissue engineering. Soft Matter. 12, 2243–2246. doi: 10.1039/C5SM02902C
Murali, D. M., and Shanmugam, G. (2019). The aromaticity of the phenyl ring imparts thermal stability to a supramolecular hydrogel obtained from low molecular mass compound. N. J. Chem. 43, 12396–12409. doi: 10.1039/C9NJ01781J
Nagarkar, R. P., Hule, R. A., Pochan, D. J., and Schneider, J. P. (2008). De novo design of strand-swapped β-hairpin hydrogels. J. Am. Chem. Soc. 130, 4466–4474. doi: 10.1021/ja710295t
Nagy-Smith, K., Beltramo, P. J., Moore, E., Tycko, R., Furst, E. M., and Schneider, J. P (2017). Molecular, local, and network-level basis for the enhanced stiffness of hydrogel networks formed from coassembled racemic peptides: predictions from Pauling and Corey. ACS Central Sci. 3, 586–597. doi: 10.1021/acscentsci.7b00115
Nalluri, S. K. M., Berdugo, C., Javid, N., Frederix, P. W., and Ulijn, R. V. (2014). Biocatalytic self-assembly of supramolecular charge-transfer nanostructures based on n-type semiconductor-appended peptides. Angew. Chem. Int. Edn. 53, 5882–5887. doi: 10.1002/anie.201311158
Nanda, J., Biswas, A., and Banerjee, A. (2013). Single amino acid based thixotropic hydrogel formation and pH-dependent morphological change of gel nanofibers. Soft Matter. 9, 4198–4208. doi: 10.1039/c3sm27050e
Naskar, J., Roy, S., Joardar, A., Das, S., and Banerjee, A. (2011). Self-assembling dipeptide-based nontoxic vesicles as carriers for drugs and other biologically important molecules. Organ. Biomol. Chem. 9, 6610–6615. doi: 10.1039/c1ob05757j
Nelli, S. R., Lin, J.-H., Nguyen, T. N. A., Tseng, D. T.-H., Talloj, S. K., and Lin, H.-C. (2017). Influence of amino acid side chains on the formation of two component self-assembling nanofibrous hydrogels. New J. Chem. 41, 1229–1234. doi: 10.1039/C6NJ02820A
Ni, M., and Zhuo, S. (2019). Applications of self-assembling ultrashort peptides in bionanotechnology. RSC Adv. 9, 844–852. doi: 10.1039/C8RA07533F
Nikitin, T., Kopyl, S., Shur, V. Y., Kopelevich, Y., and Kholkin, A. (2016). Low-temperature photoluminescence in self-assembled diphenylalanine microtubes. Phys. Lett. A 380, 1658–1662. doi: 10.1016/j.physleta.2016.02.043
Orbach, R., Mironi-Harpaz, I., Adler-Abramovich, L., Mossou, E., Mitchell, E. P., Forsyth, V. T., et al. (2012). The rheological and structural properties of Fmoc-peptide-based hydrogels: the effect of aromatic molecular architecture on self-assembly and physical characteristics. Langmuir 28, 2015–2022. doi: 10.1021/la204426q
Ozores, H. L., Amorín, M., and Granja, J. R. (2017). Self-assembling molecular capsules based on α, γ-cyclic peptides. J. Am. Chem. Soc. 139, 776–784. doi: 10.1021/jacs.6b10456
Panda, J. J., and Chauhan, V. S. (2014). Short peptide based self-assembled nanostructures: implications in drug delivery and tissue engineering. Polymer Chem. 5, 4418–4436. doi: 10.1039/C4PY00173G
Panda, J. J., and Mishra, J. (2016). Self-assembled dipeptide-based nanostructures: tiny tots with great applications. Future Sci. 15:85. doi: 10.4155/tde.15.85
Panda, J. J., Varshney, A., and Chauhan, V. S. (2013). Self-assembled nanoparticles based on modified cationic dipeptides and DNA: novel systems for gene delivery. J. Nanobiotechnol. 11:18. doi: 10.1186/1477-3155-11-18
Panda, S. S., Shmilovich, K., Ferguson, A. L., and Tovar, J. D. (2019). Controlling supramolecular chirality in peptide– π-peptide networks by variation of the Alkyl spacer length. Langmuir 35, 14060–14073. doi: 10.1021/acs.langmuir.9b02683
Pandit, G., Roy, K., Agarwal, U., and Chatterjee, S. (2018). Self-assembly mechanism of a peptide-based drug delivery vehicle. ACS Omega 3, 3143–3155. doi: 10.1021/acsomega.7b01871
Panigrahi, B., Singh, R. K., Mishra, S., and Mandal, D. (2018). Cyclic peptide-based nanostructures as efficient siRNA carriers. Artif. Cells Nanomed. Biotechnol. 46, S763–S773. doi: 10.1080/21691401.2018.1511574
Parisi, E., Garcia, A. M., Marson, D., Posocco, P., and Marchesan, S. (2019). Supramolecular tripeptide hydrogel assembly with 5-fluorouracil. Gels 5:5. doi: 10.3390/gels5010005
Parween, S., Misra, A., Ramakumar, S., and Chauhan, V. S. (2014). Self-assembled dipeptide nanotubes constituted by flexible β-phenylalanine and conformationally constrained α, β-dehydrophenylalanine residues as drug delivery system. J. Mater. Chem. B 2, 3096–3106. doi: 10.1039/c3tb21856b
Patra, J. K., Das, G., Fraceto, L. F., Campos, E. V. R., del Pilar Rodriguez-Torres, M., Acosta-Torres, L. S., et al. (2018). Nano based drug delivery systems: recent developments and future prospects. J. Nanobiotechnol. 16:71. doi: 10.1186/s12951-018-0392-8
Pianowski, Z. L., Karcher, J., and Schneider, K. (2016). Photoresponsive self-healing supramolecular hydrogels for light-induced release of DNA and doxorubicin. Chem. Commun. 52, 3143–3146. doi: 10.1039/C5CC09633B
Porter, S. L., Coulter, S. M., Pentlavalli, S., Thompson, T. P., and Laverty, G. (2018). Self-assembling diphenylalanine peptide nanotubes selectively eradicate bacterial biofilm infection. Acta Biomaterialia 77, 96–105. doi: 10.1016/j.actbio.2018.07.033
Pospišil, T., Hamzić, L. F., Ahmed, L. B., Lovrić, M., Gajović, S., and Frkanec, L. (2016). Synthesis, characterization and in vitro biocompatibility assessment of a novel tripeptide hydrogelator, as a promising scaffold for tissue engineering applications. Biomater. Sci. 4, 1412–1416. doi: 10.1039/C6BM00287K
Pugliese, R., and Gelain, F. (2017). Peptidic biomaterials: from self-assembling to regenerative medicine. Trends Biotechnol. 35, 145–158. doi: 10.1016/j.tibtech.2016.09.004
Pujals, S., Tao, K., Terradellas, A., Gazit, E., and Albertazzi, L. (2017). Studying structure and dynamics of self-assembled peptide nanostructures using fluorescence and super resolution microscopy. Chem. Commun. 53, 7294–7297. doi: 10.1039/C7CC02176C
Qin, L., Duan, P., Xie, F., Zhang, L., and Liu, M. (2013). A metal ion triggered shrinkable supramolecular hydrogel and controlled release by an amphiphilic peptide dendron. Chem. Commun. 49, 10823–10825. doi: 10.1039/c3cc47004k
Qin, L., Xie, F., Duan, P., and Liu, M. (2014). A peptide dendron-based shrinkable metallo-hydrogel for charged species separation and stepwise release of drugs. Chem. A Eur. J. 20, 15419–15425. doi: 10.1002/chem.201404035
Qiu, F., Chen, Y., Tang, C., and Zhao, X. (2018). Amphiphilic peptides as novel nanomaterials: design, self-assembly and application. Int. J. Nanomed. 13, 5003–5022. doi: 10.2147/IJN.S166403
Raad, M. d, Teunissen, E. A., and Mastrobattista, E. (2014). Peptide vectors for gene delivery: from single peptides to multifunctional peptide nanocarriers. Nanomedicine 9, 2217–2232. doi: 10.2217/nnm.14.90
Rad-Malekshahi, M., Lempsink, L., Amidi, M., Hennink, W. E., and Mastrobattista, E. (2015b). Biomedical applications of self-assembling peptides. Bioconjugate Chem. 27, 3–18. doi: 10.1021/acs.bioconjchem.5b00487
Rad-Malekshahi, M., Visscher, K. M., Rodrigues, J. P., de Vries, R., Hennink, W. E., Baldus, M., et al. (2015a). The supramolecular organization of a peptide-based nanocarrier at high molecular detail. J. Am. Chem. Soc. 137, 7775–7784. doi: 10.1021/jacs.5b02919
Raeburn, J., Cardoso, A. Z., and Adams, D. J. (2013). The importance of the self-assembly process to control mechanical properties of low molecular weight hydrogels. Chem. Soc. Rev. 42, 5143–5156. doi: 10.1039/c3cs60030k
Ragnarsson, U., and Grehn, L. (2013). Dual protection of amino functions involving Boc. RSC Adv. 3, 18691–18697. doi: 10.1039/c3ra42956c
Rajbhandary, A., Brennessel, W. W., and Nilsson, B. L. (2018). Comparison of the self-assembly behavior of fmoc-phenylalanine and corresponding peptoid derivatives. Cryst. Growth Des. 18, 623–632. doi: 10.1021/acs.cgd.7b00709
Ramaker, K., Henkel, M., Krause, T., Röckendorf, N., and Frey, A. (2018). Cell penetrating peptides: a comparative transport analysis for 474 sequence motifs. Drug Deliv. 25, 928–937. doi: 10.1080/10717544.2018.1458921
Rambhia, K. J., and Ma, P. X. (2015). Controlled drug release for tissue engineering. J. Control. Release 219, 119–128. doi: 10.1016/j.jconrel.2015.08.049
Rasale, D. B., Biswas, S., Konda, M., and Das, A. K. (2015). Exploring thermodynamically downhill nanostructured peptide libraries: from structural to morphological insight. RSC Adv. 5, 1529–1537. doi: 10.1039/C4RA09490E
Rasale, D. B., and Das, A. K. (2015). Chemical reactions directed peptide self-assembly. Int. J. Mol. Sci. 16, 10797–10820. doi: 10.3390/ijms160510797
Raymond, D. L, Abraham, B., Fujita, T. J, Watrous, M., et al. (2019). Low molecular weight supramolecular hydrogels for sustained and localized in vivo drug delivery. ACS Appl. Bio Mater. 2:b00125. doi: 10.1021/acsabm.9b00125
Raymond, D. M., and Nilsson, B. L. (2018). Multicomponent peptide assemblies. Chem. Soc. Rev. 47, 3659–3720. doi: 10.1039/C8CS00115D
Raza, F., Zafar, H., Zhu, Y., Ren, Y., Ullah, A., Khan, A. U., et al. (2018). A review on recent advances in stabilizing peptides/proteins upon fabrication in hydrogels from biodegradable polymers. Pharmaceutics 10:16. doi: 10.3390/pharmaceutics10010016
Reches, M., and Gazit, E. (2003). Casting metal nanowires within discrete self-assembled peptide nanotubes. Science 300, 625–627. doi: 10.1126/science.1082387
Reches, M., and Gazit, E. (2005). Self-assembly of peptide nanotubes and amyloid-like structures by charged-termini-capped diphenylalanine peptide analogues. Israel J. Chem. 45, 363–371. doi: 10.1560/5MC0-V3DX-KE0B-YF3J
Reches, M., and Gazit, E. (2006). Designed aromatic homo-dipeptides: formation of ordered nanostructures and potential nanotechnological applications. Phys. Biol. 3:S10. doi: 10.1088/1478-3975/3/1/S02
Reja, R. M., Patel, R., Kumar, V., Jha, A., and Gopi, H. N. (2019). Divergent supramolecular gelation of backbone modified short hybrid δ-peptides. Biomacromolecules 20, 1254–1262. doi: 10.1021/acs.biomac.8b01684
Rho, J. Y., Cox, H., Mansfield, E. D., Ellacott, S. H., Peltier, R., Brendel, J. C., et al. (2019). Dual self-assembly of supramolecular peptide nanotubes to provide stabilisation in water. Nat. Commun. 10, 1–9. doi: 10.1038/s41467-019-12586-8
Ribeiro, A. C., Souza, G. A., Pereira, D. H., Cordeiro, D. S., Miranda, R. S., Custóio, R., et al. (2019). Phe–Phe Di-peptide nanostructure self-assembling modulated by luminescent additives. ACS Omega 4, 606–619. doi: 10.1021/acsomega.8b02732
Riley, M., and Vermerris, W. (2017). Recent advances in nanomaterials for gene delivery—a review. Nanomaterials 7:94. doi: 10.3390/nano7050094
Rivas, M., del Valle, L. J., Alemán, C., and Puiggalí, J. (2019). Peptide self-assembly into hydrogels for biomedical applications related to hydroxyapatite. Gels 5:14. doi: 10.3390/gels5010014
Rizvi, S. A., and Saleh, A. M. (2018). Applications of nanoparticle systems in drug delivery technology. Saudi Pharm. J. 26, 64–70. doi: 10.1016/j.jsps.2017.10.012
Rodriguez, L. M. D. L., Hemar, Y., Cornish, J., and Brimble, M. A. (2016). Structure–mechanical property correlations of hydrogel forming β-sheet peptides. Chem. Soc. Rev. 45, 4797–4824. doi: 10.1039/C5CS00941C
Roth-Konforti, M. E., Comune, M., Halperin-Sternfeld, M., Grigoriants, I., Shabat, D., and Adler-Abramovich, L. (2018). UV light–responsive peptide-based supramolecular hydrogel for controlled drug delivery. Macromol. Rapid Commun. 39:1800588. doi: 10.1002/marc.201800588
Rubert Pérez, C. M., Stephanopoulos, N., Sur, S., Lee, S. S., Newcomb, C., and Stupp, S. I. (2015). The powerful functions of peptide-based bioactive matrices for regenerative medicine. Ann. Biomed. Eng. 43, 501–514. doi: 10.1007/s10439-014-1166-6
Ryan, D. M., and Nilsson, B. L. (2012). Self-assembled amino acids and dipeptides as noncovalent hydrogels for tissue engineering. Polymer Chem. 3, 18–33. doi: 10.1039/C1PY00335F
Ryan, K., Beirne, J., Redmond, G., Kilpatrick, J. I., Guyonnet, J., Buchete, N.-V., et al. (2015). Nanoscale piezoelectric properties of self-assembled fmoc–FF peptide fibrous networks. ACS Appl. Mater. Interfaces 7, 12702–12707. doi: 10.1021/acsami.5b01251
Sagiri, S. S., Behera, B., Rafanan, R. R., Bhattacharya, C., Pal, K., Banerjee, I., et al. (2014). Organogels as matrices for controlled drug delivery: a review on the current state. Soft Mater. 12, 47–72. doi: 10.1080/1539445X.2012.756016
Saha, S., Bachl, J., Kundu, T., Díaz, D. D., and Banerjee, R. (2014). Dissolvable metallohydrogels for controlled release: evidence of a kinetic supramolecular gel phase intermediate. Chem. Commun. 50, 7032–7035. doi: 10.1039/C4CC02771J
Sahoo, J. K., Nazareth, C., VandenBerg, M. A., and Webber, M. J. (2017). Self-assembly of amphiphilic tripeptides with sequence-dependent nanostructure. Biomater. Sci. 5, 1526–1530. doi: 10.1039/C7BM00304H
Sahoo, J. K., Nazareth, C., VandenBerg, M. A., and Webber, M. J. (2018a). Aromatic identity, electronic substitution, and sequence in amphiphilic tripeptide self-assembly. Soft Matter. 14, 9168–9174. doi: 10.1039/C8SM01994K
Sahoo, J. K., VandenBerg, M. A., and Webber, M. J. (2018b). Injectable network biomaterials via molecular or colloidal self-assembly. Adv. Drug Deliv. Rev. 127, 185–207. doi: 10.1016/j.addr.2017.11.005
Sarkar, B., Nguyen, P. K., Gao, W., Dondapati, A., Siddiqui, Z., and Kumar, V. A. (2018). Angiogenic self-assembling peptide scaffolds for functional tissue regeneration. Biomacromolecules 19, 3597–3611. doi: 10.1021/acs.biomac.8b01137
Sarkar, B., O'Leary, L. E., and Hartgerink, J. D. (2014). Self-assembly of fiber-forming collagen mimetic peptides controlled by triple-helical nucleation. J. Am. Chem. Soc. 136, 14417–14424. doi: 10.1021/ja504377s
Sarkar, R., Debnath, M., Maji, K., and Haldar, D. (2015). Solvent assisted structural diversity: supramolecular sheet and double helix of a short aromatic γ-peptide. RSC Adv. 5, 76257–76262. doi: 10.1039/C5RA12831E
Sato, K., Ji, W., Palmer, L. C., Weber, B., Barz, M., and Stupp, S. I. (2017). Programmable assembly of peptide amphiphile via noncovalent-to-covalent bond conversion. J. Am. Chem. Soc. 139, 8995–9000. doi: 10.1021/jacs.7b03878
Scelsi, A., Bochicchio, B., and Pepe, A. (2019). Labeling of nanofiber-forming peptides by site-directed bioconjugation: effect of spacer length on self-assembly. Curr. Organic Synth. 16, 319–325. doi: 10.2174/1570179416666181127150142
Semin, S., Van Etteger, A., Cattaneo, L., Amdursky, N., Kulyuk, L., Lavrov, S., et al. (2015). Strong thermo-induced single and two-photon green luminescence in self-organized peptide microtubes. Small 11, 1156–1160. doi: 10.1002/smll.201401602
Seow, W. Y., and Hauser, C. A. (2014). Short to ultrashort peptide hydrogels for biomedical uses. Mater. Today 17, 381–388. doi: 10.1016/j.mattod.2014.04.028
Sharma, A. R., Kundu, S. K., Nam, J.-S., Sharma, G., Doss, P., George, C., et al. (2014). Next generation delivery system for proteins and genes of therapeutic purpose: why and how? BioMed. Res. Int. 2014:327950. doi: 10.1155/2014/327950
Sharma, P., Kaur, H., and Roy, S. (2019). Inducing differential self-assembling behavior in ultrashort peptide hydrogelators using simple metal salts. Biomacromolecules 20, 2610–2624. doi: 10.1021/acs.biomac.9b00416
Silva, R. F., Ara?jo, D. R., Silva, E. R., Ando, R., m, A., and Alves, W. A. (2013). L-diphenylalanine microtubes as a potential drug-delivery system: characterization, release kinetics, and cytotoxicity. Langmuir 29, 10205–10212. doi: 10.1021/la4019162
Singh, M., Kundu, S., Sreekanth, V., Motiani, R. K., Sengupta, S., Srivastava, A., et al. (2014). Injectable small molecule hydrogel as a potential nanocarrier for localized and sustained in vivo delivery of doxorubicin. Nanoscale 6, 12849–12855. doi: 10.1039/C4NR04064C
Singh, P., Brar, S. K., Bajaj, M., Narang, N., Mithu, V. S., Katare, O. P., et al. (2017). Self-assembly of aromatic α-amino acids into amyloid inspired nano/micro scaled architects. Mater. Sci. Eng. C 72, 590–600. doi: 10.1016/j.msec.2016.11.117
Singh, P. K., Chibh, S., Dube, T., Chauhan, V. S., and Panda, J. J. (2018). Arginine-α, β-dehydrophenylalanine dipeptide nanoparticles for pH-responsive drug delivery. Pharm. Res. 35:35. doi: 10.1007/s11095-017-2299-8
Singh, V., Rai, R. K., Arora, A., Sinha, N., and Thakur, A. K. (2014). Therapeutic implication of L-phenylalanine aggregation mechanism and its modulation by D-phenylalanine in phenylketonuria. Sci. Rep. 4:3875. doi: 10.1038/srep03875
Singh, V., Snigdha, K., Singh, C., Sinha, N., and Thakur, A. K. (2015). Understanding the self-assembly of Fmoc–phenylalanine to hydrogel formation. Soft Matter. 11, 5353–5364. doi: 10.1039/C5SM00843C
Sis, M. J., and Webber, M. J. (2019). Drug delivery with designed peptide assemblies. Trends Pharmacol. Sci. 40, 747–762. doi: 10.1016/j.tips.2019.08.003
Skilling, K. J., Citossi, F., Bradshaw, T. D., Ashford, M., Kellam, B., and Marlow, M. (2014). Insights into low molecular mass organic gelators: a focus on drug delivery and tissue engineering applications. Soft Matter. 10, 237–256. doi: 10.1039/C3SM52244J
Skotland, T., Iversen, T., Torgersen, M., and Sandvig, K. (2015). Cell-penetrating peptides: possibilities and challenges for drug delivery in vitro and in vivo. Molecules 20, 13313–13323. doi: 10.3390/molecules200713313
Slocik, J. M., and Naik, R. R. (2017). Sequenced defined biomolecules for nanomaterial synthesis, functionalization, and assembly. Curr. Opin. Biotechnol. 46, 7–13. doi: 10.1016/j.copbio.2016.11.025
Soleymani-Goloujeh, M., Nokhodchi, A., Niazi, M., Najafi-Hajivar, S., Shahbazi-Mojarrad, J., Zarghami, N., et al. (2018). Effects of N-terminal and C-terminal modification on cytotoxicity and cellular uptake of amphiphilic cell penetrating peptides. Artif. Cells Nanomed. Biotechnol. 46, 91–103. doi: 10.1080/21691401.2017.1414823
Son, J., Kalafatovic, D., Kumar, M., Yoo, B., Cornejo, M. A., Contel, M., et al. (2019). Customizing morphology, size, and response kinetics of matrix metalloproteinase-responsive nanostructures by systematic peptide design. ACS Nano 13, 1555–1562. doi: 10.1021/acsnano.8b07401
Song, R., Wu, X., Xue, B., Yang, Y., Huang, W., Zeng, G., et al. (2018). Principles governing catalytic activity of self-assembled short peptides. J. Am. Chem. Soc. 141, 223–231. doi: 10.1021/jacs.8b08893
Song, Z., Chen, X., You, X., Huang, K., Dhinakar, A., Gu, Z., et al. (2017). Self-assembly of peptide amphiphiles for drug delivery: the role of peptide primary and secondary structures. Biomater. Sci. 5, 2369–2380. doi: 10.1039/C7BM00730B
Spicer, C. D., Jumeaux, C., Gupta, B., and Stevens, M. M. (2018). Peptide and protein nanoparticle conjugates: versatile platforms for biomedical applications. Chem. Soc. Rev. 47, 3574–3620. doi: 10.1039/C7CS00877E
Su, H., Koo, J. M., and Cui, H. (2015). One-component nanomedicine. J. Controll. Release 219, 383–395. doi: 10.1016/j.jconrel.2015.09.056
Sun, L., Zheng, C., and Webster, T. J. (2016). Self-assembled peptide nanomaterials for biomedical applications: promises and pitfalls. Int. J. Nanomed. 12, 73–86. doi: 10.2147/IJN.S117501
Sun, M., Zhang, X., Gao, Z., Liu, T., Luo, C., Zhao, Y., et al. (2019). Probing a dipeptide-based supramolecular assembly as an efficient camptothecin delivering carrier for cancer therapy: computational simulations and experimental validations. Nanoscale 11, 3864–3876. doi: 10.1039/C8NR07014H
Sun, Y., Kaplan, J. A., Shieh, A., Sun, H.-L., Croce, C. M., Grinstaff, M. W., et al. (2016). Self-assembly of a 5-fluorouracil-dipeptide hydrogel. Chem. Commun. 52, 5254–5257. doi: 10.1039/C6CC01195K
Tamamis, P., Adler-Abramovich, L., Reches, M., Marshall, K., Sikorski, P., Serpell, L., et al. (2009). Self-assembly of phenylalanine oligopeptides: insights from experiments and simulations. Biophys. J. 96, 5020–5029. doi: 10.1016/j.bpj.2009.03.026
Tang, Y., Yao, Y., and Wei, G. (2020). Expanding the structural diversity of peptide assemblies by coassembling dipeptides with diphenylalanine. Nanoscale 12, 3038–3049. doi: 10.1039/C9NR09317F
Tao, K., Levin, A., Adler-Abramovich, L., and Gazit, E. (2016). Fmoc-modified amino acids and short peptides: simple bio-inspired building blocks for the fabrication of functional materials. Chem. Soc. Rev. 45, 3935–3953. doi: 10.1039/C5CS00889A
Tao, K., Makam, P., Aizen, R., and Gazit, E. (2017). Self-assembling peptide semiconductors. Science 358:eaam9756. doi: 10.1126/science.aam9756
Tao, K., Yoskovitz, E., Adler-Abramovich, L., and Gazit, E. (2015). Optical property modulation of Fmoc group by pH-dependent self-assembly. RSC Adv. 5, 73914–73918. doi: 10.1039/C5RA16412E
Tesauro, D., Accardo, A., Diaferia, C., Milano, V., Guillon, J., Ronga, L., et al. (2019). Peptide-based drug-delivery systems in biotechnological applications: recent advances and perspectives. Molecules 24:351. doi: 10.3390/molecules24020351
Thota, C. K., Yadav, N., and Chauhan, V. S. (2016). A novel highly stable and injectable hydrogel based on a conformationally restricted ultrashort peptide. Sci. Rep. 6:31167. doi: 10.1038/srep31167
Tomasini, C., and Castellucci, N. (2013). Peptides and peptidomimetics that behave as low molecular weight gelators. Chem. Soc. Rev. 42, 156–172. doi: 10.1039/C2CS35284B
Travaglini, L., Giordano, C., D'Annibale, A., Gubitosi, M., di Gregorio, M. C., Schillén, K., et al. (2017). Twisted nanoribbons from a RGD-bearing cholic acid derivative. Colloids Surfaces B 159, 183–190. doi: 10.1016/j.colsurfb.2017.07.084
Truong, W. T., Su, Y., Gloria, D., Braet, F., and Thordarson, P. (2015). Dissolution and degradation of Fmoc-diphenylalanine self-assembled gels results in necrosis at high concentrations in vitro. Biomater. Sci. 3, 298–307. doi: 10.1039/C4BM00244J
Vánová, J., Hejtmánková, A., Kalbáčová, M. H., and Španielová, H. (2019). The utilization of cell-penetrating peptides in the intracellular delivery of viral nanoparticles. Materials 12:2671. doi: 10.3390/ma12172671
Varshney, A., Panda, J. J., Singh, A. K., Yadav, N., Bihari, C., Biswas, S., et al. (2018). Targeted delivery of microRNA-199a-3p using self-assembled dipeptide nanoparticles efficiently reduces hepatocellular carcinoma in mice. Hepatology 67, 1392–1407. doi: 10.1002/hep.29643
Vasilev, S., Zelenovskiy, P., Vasileva, D., Nuraeva, A., Shur, V. Y., and Kholkin, A. L. (2016). Piezoelectric properties of diphenylalanine microtubes prepared from the solution. J. Phys. Chem. Solids 93, 68–72. doi: 10.1016/j.jpcs.2016.02.002
Veloso, S. R., Martins, J., Hilliou, L., Amorim, C., Amaral, V., Almeida, B., et al. (2020). Dehydropeptide-based plasmonic magnetogels: a supramolecular composite nanosystem for multimodal cancer therapy. J. Mater. Chem. B 8, 45–64. doi: 10.1039/C9TB01900F
Vilaça, H., Castro, T., Costa, F. M., Melle-Franco, M., Hilliou, L., Hamley, I. W., et al. (2017). Self-assembled RGD dehydropeptide hydrogels for drug delivery applications. J. Mater. Chem. B 5, 8607–8617. doi: 10.1039/C7TB01883E
Vilaça, H., Hortelão, A. C., Castanheira, E. M., Queiroz, M.-J. o,. R, Hilliou, L., et al. (2015a). Dehydrodipeptide hydrogelators containing naproxen N-capped tryptophan: self-assembly, hydrogel characterization, and evaluation as potential drug nanocarriers. Biomacromolecules 16, 3562–3573. doi: 10.1021/acs.biomac.5b01006
Vilaça, H., Pereira, G., Castro, T., Hermenegildo, B., Shi, J., Faria, T., et al. (2015b). New self-assembled supramolecular hydrogels based on dehydropeptides. J. Mater. Chem. B 3, 6355–6367. doi: 10.1039/C5TB00501A
Vrettos, E. I., Mezo, G., and Tzakos, A. G. (2018). On the design principles of peptide–drug conjugates for targeted drug delivery to the malignant tumor site. Beilstein J. Organ. Chem. 14, 930–954. doi: 10.3762/bjoc.14.80
Wang, F., Wang, Y., Zhang, X., Zhang, W., Guo, S., and Jin, F. (2014). Recent progress of cell-penetrating peptides as new carriers for intracellular cargo delivery. J. Controll. Rel. 174, 126–136. doi: 10.1016/j.jconrel.2013.11.020
Wang, H., Feng, Z., and Xu, B. (2016). D-Amino acid-containing supramolecular nanofibers for potential cancer therapeutics. Adv. Drug Del. Rev. 4, 110–111. doi: 10.1016/j.addr.2016.04.008
Wang, H., Feng, Z., and Xu, B. (2019). Supramolecular assemblies of peptides or nucleopeptides for gene delivery. Theranostics 9:3213. doi: 10.7150/thno.31854
Wang, H., and Yang, Z. (2012). Molecular hydrogels of hydrophobic compounds: a novel self-delivery system for anti-cancer drugs. Soft Matter. 8, 2344–2347. doi: 10.1039/C2SM06923G
Wang, J., Hu, X., and Xiang, D. (2018). Nanoparticle drug delivery systems: an excellent carrier for tumor peptide vaccines. Drug Deliv. 25, 1319–1327. doi: 10.1080/10717544.2018.1477857
Wang, J., Liu, K., Xing, R., and Yan, X. (2016a). Peptide self-assembly: thermodynamics and kinetics. Chem. Soc. Rev. 45, 5589–5604. doi: 10.1039/C6CS00176A
Wang, J., Liu, K., Yan, L., Wang, A., Bai, S., and Yan, X. (2016b). Trace solvent as a predominant factor to tune dipeptide self-assembly. ACS Nano 10, 2138–2143. doi: 10.1021/acsnano.5b06567
Wang, W., Anderson, C. F., Wang, Z., Wu, W., Cui, H., and Liu, C.-J. (2017). Peptide-templated noble metal catalysts: syntheses and applications. Chem. Sci. 8, 3310–3324. doi: 10.1039/C7SC00069C
Wang, Y., Cheetham, A. G., Angacian, G., Su, H., Xie, L., and Cui, H. (2017a). Peptide–drug conjugates as effective prodrug strategies for targeted delivery. Adv. Drug Deliv. Rev. 110, 112–126. doi: 10.1016/j.addr.2016.06.015
Wang, Y., Fan, S., Zhong, W., Zhou, X., and Li, S. (2017b). Development and properties of valine-alanine based antibody-drug conjugates with monomethyl auristatin e as the potent payload. Int. J. Mol. Sci. 18:1860. doi: 10.3390/ijms18091860
Wang, Y., Huang, R., Qi, W., Wu, Z., Su, R., and He, Z. (2013b). Kinetically controlled self-assembly of redox-active ferrocene–diphenylalanine: from nanospheres to nanofibers. Nanotechnology 24:465603. doi: 10.1088/0957-4484/24/46/465603
Wang, Y., Qi, W., Huang, R., Yang, X., Wang, M., Su, R., et al. (2015). Rational design of chiral nanostructures from self-assembly of a ferrocene-modified dipeptide. J. Am. Chem. Soc. 137, 7869–7880. doi: 10.1021/jacs.5b03925
Wang, Y., Zhang, Z., Xu, L., Li, X., and Chen, H. (2013a). Hydrogels of halogenated Fmoc-short peptides for potential application in tissue engineering. Colloids Surfaces B 104, 163–168. doi: 10.1016/j.colsurfb.2012.11.038
Webber, M. J., Appel, E. A., Meijer, E. W., and Langer, R. (2016). Supramolecular biomaterials. Nat. Mater. 15, 13–26. doi: 10.1038/nmat4474
Webber, M. J., and Langer, R. (2017). Drug delivery by supramolecular design. Chem. Soc. Rev. 46, 6600–6620. doi: 10.1039/C7CS00391A
Wijerathne, N. K., Kumar, M., and Ulijn, R. V. (2019). Fmoc-dipeptide/porphyrin molar ratio dictates energy transfer efficiency in nanostructures produced by biocatalytic coassembly. Chem. A Eur. J. 2019:2819. doi: 10.1002/chem.201902819
Wołczanski, G., and Lisowski, M. (2018). A general method for preparation of N-Boc-protected or N-Fmoc-protected α, β-didehydropeptide building blocks and their use in the solid-phase peptide synthesis. J. Peptide Sci. 24:e3091. doi: 10.1002/psc.3091
Worthington, P., Langhans, S., and Pochan, D. (2017). beta-hairpin peptide hydrogels for package delivery. Adv. Drug Deliv. Rev. 110–111, 127–136. doi: 10.1016/j.addr.2017.02.002
Wu, Y., and Collier, J. H. (2017). α-Helical coiled-coil peptide materials for biomedical applications. Wiley Interdisc. Rev. 9:e1424. doi: 10.1002/wnan.1424
Xie, Y., Zhao, J., Huang, R., Qi, W., Wang, Y., Su, R., et al. (2016). Calcium-ion-triggered co-assembly of peptide and polysaccharide into a hybrid hydrogel for drug delivery. Nanoscale Res. Lett. 11:184. doi: 10.1186/s11671-016-1415-8
Xing, R., Jiao, T., Feng, L., Zhang, Q., Zou, Q., Yan, X., et al. (2015). Photothermally-induced molecular self-assembly of macroscopic peptide-inorganic hybrid films. Sci. Adv. Mater. 7, 1701–1707. doi: 10.1166/sam.2015.2411
Xue, X., Lindstrom, A., Qu, H., and Li, Y. (2020). Recent advances on small-molecule nanomedicines for cancer treatment. WIREs Nanomed. Nanobiotechnol. 2:e1607. doi: 10.1002/wnan.1607
Yadav, N., Chauhan, M. K., and Chauhan, V. S. (2020). Short to ultrashort peptide-based hydrogels as a platform for biomedical applications. Biomater. Sci. 8, 84–100. doi: 10.1039/C9BM01304K
Yadav, S., Rai, V., Mahato, M., Singh, M., Rekha Deka, S., and Kumar Sharma, A. (2015). Vitamin E–TPGS stabilized self-assembled tripeptide nanostructures for drug delivery. Curr. Top. Med. Chem. 15, 1227–1235. doi: 10.2174/1568026615666150330111348
Yang, X., Wang, Y., Qi, W., Xing, R., Yang, X., Xing, Q., et al. (2019). Disulfide crosslinking and helical coiling of peptide micelles facilitate the formation of a printable hydrogel. J. Mater. Chem. B 7, 2981–2988. doi: 10.1039/C8TB03121E
Yeh, M.-Y., Huang, C.-T., Lai, T.-S., Chen, F.-Y., Chu, N.-T., Tseng, D. T.-H., et al. (2016). Effect of peptide sequences on supramolecular interactions of naphthaleneimide/tripeptide conjugates. Langmuir 32, 7630–7638. doi: 10.1021/acs.langmuir.6b01809
Yoo, S. H., and Lee, H.-S. (2017). Foldectures: 3D molecular architectures from self-assembly of peptide foldamers. Accounts Chem. Res. 50, 832–841. doi: 10.1021/acs.accounts.6b00545
Youngblood, R. L., Truong, N. F., Segura, T., and Shea, L. D. (2018). It's all in the delivery: designing hydrogels for cell and non-viral gene therapies. Mol. Therap. 26, 2087–2106. doi: 10.1016/j.ymthe.2018.07.022
Yu, C.-Y., Huang, W., Li, Z.-P., Lei, X.-Y., He, D.-X., and Sun, L. (2016). Progress in self-assembling peptide-based nanomaterials for biomedical applications. Curr. Top. Med. Chem. 16, 281–290. doi: 10.2174/1568026615666150701114527
Yu, Z., Xu, Q., Dong, C., Lee, S. S., Gao, L., Li, Y., et al. (2015). Self-assembling peptide nanofibrous hydrogel as a versatile drug delivery platform. Curr. Pharm. Design 21, 4342–4354. doi: 10.2174/1381612821666150901104821
Yuan, C., Ji, W., Xing, R., Li, J., Gazit, E., and Yan, X. (2019). Hierarchically oriented organization in supramolecular peptide crystals. Nat. Rev. Chem. 3, 567–588. doi: 10.1038/s41570-019-0129-8
Yuran, S., Razvag, Y., and Reches, M. (2012). Coassembly of aromatic dipeptides into biomolecular necklaces. Acs Nano 6, 9559–9566. doi: 10.1021/nn302983e
Zaldivar, G., Vemulapalli, S., Udumula, V., Conda-Sheridan, M., and Tagliazucchi, M. (2019). Self-assembled nanostructures of peptide-amphiphiles: charge regulation by size regulation. J. Phys. Chem. C 123, 17606–17615. doi: 10.1021/acs.jpcc.9b04280
Zanna, N., Merlettini, A., Tatulli, G., Milli, L., Focarete, M. L., and Tomasini, C. (2015). Hydrogelation induced by Fmoc-protected peptidomimetics. Langmuir 31, 12240–12250. doi: 10.1021/acs.langmuir.5b02780
Zanna, N., and Tomasini, C. (2017). Peptide-based physical gels endowed with thixotropic behaviour. Gels 3:39. doi: 10.3390/gels3040039
Zhang, C., Welborn, M., Zhu, T., Yang, N. J., Santos, M. S., Van Voorhis, T., et al. (2016). π-Clamp-mediated cysteine conjugation. Nat. Chem. 8:120. doi: 10.1038/nchem.2413
Zhang, H., Fei, J., Yan, X., Wang, A., and Li, J. (2015). Enzyme-responsive release of doxorubicin from monodisperse dipeptide-based nanocarriers for highly efficient cancer treatment in vitro. Adv. Funct. Mater. 25, 1193–1204. doi: 10.1002/adfm.201403119
Zhang, H., Park, J., Jiang, Y., and Woodrow, K. A. (2017). Rational design of charged peptides that self-assemble into robust nanofibers as immune-functional scaffolds. Acta Biomaterialia 55, 183–193. doi: 10.1016/j.actbio.2017.03.041
Zhang, L., Wang, X., Wang, T., and Liu, M. (2015). Tuning soft nanostructures in self-assembled supramolecular gels: from morphology control to morphology-dependent functions. Small 11, 1025–1038. doi: 10.1002/smll.201402075
Zhang, R., Xing, R., Jiao, T., Ma, K., Chen, C., Ma, G., et al. (2016). Carrier-free, chemophotodynamic dual nanodrugs via self-assembly for synergistic antitumor therapy. ACS Appl. Mater. Interfaces 8, 13262–13269. doi: 10.1021/acsami.6b02416
Zhang, Z., Shi, L., Wu, C., Su, Y., Qian, J., Deng, H., et al. (2017). Construction of a supramolecular drug–drug delivery system for non-small-cell lung cancer therapy. ACS Appl. Mater. Interfaces 9, 29505–29514. doi: 10.1021/acsami.7b07565
Zheng, W., Gao, J., Song, L., Chen, C., Guan, D., Wang, Z., et al. (2012). Surface-induced hydrogelation inhibits platelet aggregation. J. Am. Chem. Soc. 135, 266–271. doi: 10.1021/ja308690y
Zhong, J., Fu, H., Jia, X., Lou, H., Wan, T., Luo, H., et al. (2019). A pH-/thermo-responsive hydrogel formed from N, N′-dibenzoyl-l-cystine: properties, self-assembly structure and release behavior of SA. RSC Adv. 9, 11824–11832. doi: 10.1039/C8RA09058K
Zhou, M., Ulijn, R. V., and Gough, J. E. (2014). Extracellular matrix formation in self-assembled minimalistic bioactive hydrogels based on aromatic peptide amphiphiles. J. Tissue Eng. 5:2041731414531593. doi: 10.1177/2041731414531593
Zhou, P., Wang, J., Wang, M., Hou, J., Lu, J. R., and Xu, H. (2019). Amino acid conformations control the morphological and chiral features of the self-assembled peptide nanostructures: young investigators perspective. J. Colloid Interface Sci. 548, 244–254. doi: 10.1016/j.jcis.2019.04.019
Zohrabi, T., Habibi, N., Zarrabi, A., Fanaei, M., and Lee, L. Y. (2016). Diphenylalanine peptide nanotubes self-assembled on functionalized metal surfaces for potential application in drug-eluting stent. J. Biomed. Mater. Res. Part A 104, 2280–2290. doi: 10.1002/jbm.a.35764
Zou, Q., Abbas, M., Zhao, L., Li, S., Shen, G., and Yan, X. (2017). Biological photothermal nanodots based on self-assembly of peptide–porphyrin conjugates for antitumor therapy. J. Am. Chem. Soc. 139, 1921–1927. doi: 10.1021/jacs.6b11382
Keywords: amphiphilicity, peptide, self-assembly, drug delivery, tissue engineering
Citation: Gupta S, Singh I, Sharma AK and Kumar P (2020) Ultrashort Peptide Self-Assembly: Front-Runners to Transport Drug and Gene Cargos. Front. Bioeng. Biotechnol. 8:504. doi: 10.3389/fbioe.2020.00504
Received: 29 January 2020; Accepted: 29 April 2020;
Published: 29 May 2020.
Edited by:
Stefano Leporatti, Italian National Research Council, ItalyReviewed by:
Remigiusz Marcin Bachor, University of Wrocław, PolandHakan Ceylan, Max Planck Institute for Intelligent Systems, Germany
Copyright © 2020 Gupta, Singh, Sharma and Kumar. This is an open-access article distributed under the terms of the Creative Commons Attribution License (CC BY). The use, distribution or reproduction in other forums is permitted, provided the original author(s) and the copyright owner(s) are credited and that the original publication in this journal is cited, in accordance with accepted academic practice. No use, distribution or reproduction is permitted which does not comply with these terms.
*Correspondence: Seema Gupta, c2VlbWFndXB0YSYjeDAwMDQwO2FuZGMuZHUuYWMuaW4=; Ashwani K. Sharma, YXNod2FuaSYjeDAwMDQwO2lnaWIuaW4=; Pradeep Kumar, cGt1bWFyJiN4MDAwNDA7aWdpYi5yZXMuaW4=