Corrigendum: Molecular Insights Into Lysyl Oxidases in Cartilage Regeneration and Rejuvenation
- 1Department of Orthopaedics and Traumatology, Stem Cells and Regenerative Medicine Laboratory, Li Ka Shing Institute of Health Sciences, The Chinese University of Hong Kong, Prince of Wales Hospital, Hong Kong, China
- 2The First Affiliated Hospital of Guangzhou University of Chinese Medicine, Lingnan Medical Research Center, Guangzhou University of Chinese Medicine, Guangzhou, China
- 3MOE Key Laboratory for Regenerative Medicine, School of Biomedical Sciences, The Chinese University of Hong Kong, Hong Kong, China
Articular cartilage remains among the most difficult tissues to regenerate due to its poor self-repair capacity. The lysyl oxidase family (LOX; also termed as protein-lysine 6-oxidase), mainly consists of lysyl oxidase (LO) and lysyl oxidase-like 1-4 (LOXL1-LOXL4), has been traditionally defined as cuproenzymes that are essential for stabilization of extracellular matrix, particularly cross-linking of collagen and elastin. LOX is essential in the musculoskeletal system, particularly cartilage. LOXs-mediated collagen cross-links are essential for the functional integrity of articular cartilage. Appropriate modulation of the expression or activity of certain LOX members selectively may become potential promising strategy for cartilage repair. In the current review, we summarized the advances of LOX in cartilage homeostasis and functioning, as well as copper-mediated activation of LOX through hypoxia-responsive signaling axis during recent decades. Also, the molecular signaling network governing LOX expression has been summarized, indicating that appropriate modulation of hypoxia-responsive-signaling-directed LOX expression through manipulation of bioavailability of copper and oxygen is promising for further clinical implications of cartilage regeneration, which has emerged as a potential therapeutic approach for cartilage rejuvenation in tissue engineering and regenerative medicine. Therefore, targeted regulation of copper-mediated hypoxia-responsive signalling axis for selective modulation of LOX expression may become potential effective therapeutics for enhanced cartilage regeneration and rejuvenation in future clinical implications.
Introduction
The lysyl oxidase family (LOX; also termed as protein-lysine 6-oxidase) has been traditionally defined as cuproenzymes that are essential for stabilization of extracellular matrix (ECM), particularly cross-linking of collagen and elastin (Rucker et al., 1998; Kagan and Li, 2003). LOX mainly comprises of five members that were originally considered copper-dependent amine oxidases, including lysyl oxidase and lysyl oxidase-like 1-4 (LOXL1-LOXL4), is a copper-containing amine oxidase belonging to a heterogeneous family of enzymes, which catalyzes oxidative deamination of the amino group in certain lysine and hydroxylysine residues of collagen molecules for stabilization of collagen fibrils (Kagan and Li, 2003). To date, LOX has been demonstrated to regulate a diverse range of cellular processes and biological functions (Smith-Mungo and Kagan, 1998), as well as certain pathogenesis of various diseases, particularly fibrotic diseases, ischemic cardiovascular diseases, and cancer progression, which is mainly mediated by ECM remodeling and elastogenesis (Schmelzer et al., 2019), epithelial-mesenchymal transition and intracellular signaling (Lopez et al., 2010; Busnadiego et al., 2013; Cox et al., 2013, 2015; Klingberg et al., 2013; Rimar et al., 2014; Schmelzer et al., 2019). The LOX has been demonstrated as crucial contributors for normal embryonic development of various tissue and organ systems, including cardiovascular (Martinez-Gonzalez et al., 2019), and respiratory systems (Maki et al., 2002; Maki, 2009; Maki et al., 2005), as well as essential for normal physiological and cellular properties, such as sprouting angiogenesis of endothelial cells (Lucero and Kagan, 2006; Bignon et al., 2011).
Cartilage is an avascular collagen-abundant tissue. Unlike bone, cartilage seems to lack efficient self-reparative/regenerative capacity, making arthritis common and costly, affecting the well-being and quality of life of millions of people worldwide (Huey et al., 2012; Mobasheri and Batt, 2016; Deng et al., 2019). Inflammatory arthritides, such as rheumatoid arthritis, and psoriatic arthritis, are among the most challenging auto-immune diseases and health problems worldwide (Mobasheri and Batt, 2016; Flores et al., 2019; Zhou B. et al., 2019). Of note, osteoarthritis (OA) is the most common form of arthritis, which is one of the most prevalent chronic immune diseases. OA is characterized by articular cartilage degeneration, subchondral bone remodeling, osteophyte formation and synovial changes (Yuan et al., 2014). OA is a multifactorial disease and various risk factors of OA have been reported, such as obesity (Aspden, 2011), body mass (Messier et al., 2005), and aging (Lotz and Loeser, 2012). Until now, the etiology and pathophysiology of OA have not been well documented. Various treatments, such as cellular therapies (Fu et al., 2014; Lee and Wang, 2017; Lin et al., 2017; Teo et al., 2019; Xu et al., 2019), administration of certain drugs or chemicals (Zhang et al., 2016, 2019; Yao et al., 2019), therapeutic surgeries (Chen Y. et al., 2015), and bio-fabrication approach (Tatman et al., 2015; Onofrillo et al., 2018; Lee et al., 2019), have been intensely studied and tested in various preclinical studies and clinical trials during recent decades (Lee and Wang, 2017; Zhang et al., 2019). However, several hurdles are required to be addressed for therapeutic optimization before clinical translation. And currently there are no effective clinical options treating OA (Mobasheri and Batt, 2016; Chen et al., 2018; Griffin and Scanzello, 2019).
Cross-linking is essential for the stabilization and mechanical support of collagen networks within native cartilage. Of note, the formation of lysine-derived, covalent pyridinoline (PYR) cross-links relies on the enzyme LOX, which is hypoxia-response element-directed upregulation during HIF1-transcriptional activation (van Vlimmeren et al., 2010; Gao et al., 2013). The activity of LOX is regulated by proteolytic cleavage of the LOX pro-peptides. Importantly, the activity of LOX is also mainly dependent on the presence of copper (Wang et al., 1996). The LOX family plays central roles in the musculoskeletal system, such as tendon (Danielsen, 1982; Robinson et al., 2005; Marturano et al., 2013), ligaments (Makris et al., 2014), and cartilage (Iftikhar et al., 2011; Makris et al., 2014).
Of note, copper, an important co-factor of various chaperones and enzymes, is vital for maintenance of integrity and homeostasis of cartilage tissues. However, until now, the underlying detailed mechanisms remain elusive. In the current review, we summarized the advances of LOXs family in cartilage homeostasis and regeneration, including embryogenesis, and potential involvement during pathophysiology of arthritis, as well as copper-mediated activation of LOXs and hypoxia-responsive signaling axis during recent decades, the main molecular modulation signaling network controlling LOXs expression, which is promising for potential clinical implications of cartilage regeneration in regenerative medicine and tissue engineering. Also, we propose potential links between the LOXs family and aging-related chronic inflammation and cartilage degeneration. Modulation of certain the LOXs family members may become a promising therapeutic approach for cartilage regeneration.
Lysyl Oxidases in Cartilage Functioning
Endochondral ossification is one of the two main forms of skeletal formation during embryogenesis. Mesenchymal chondroprogenitor cells differentiate into chondrocytes through cellular condensation processes, which are then surrounded by an abundant layer of extracellular matrix, including type II, IX, and XI collagens, which is the characteristics of cartilage (Mendler et al., 1989).
Generally, aging-induced cartilage degeneration in arthritis is becoming increasingly prevalent, which is accompanied with the changes in the components of ECM of cartilage (Loeser et al., 2016; Varela-Eirin et al., 2018). The expression of LO and LOXLs has been detected in chondrocytes near the joint cavity undergoing appositional growth, as well as in the epiphyseal plate of femur undergoing endochondral ossification. Strong expression of LO was observed in marrow cavity. And the localization of LOXL was mainly detected in chondrocytes of the reserve, proliferating cartilage, and hypertrophic zones, suggesting their vital functions during cartilage embryogenesis and potential roles for the normal function of adult cartilage (Hayashi et al., 2004; Thomassin et al., 2005). Further studies have indicated the expression of LO, LOXL2, and LOXL4 on chondrocytes of articular cartilage layers (Huang et al., 2010). Also, expression of LOXL2 has been detected in proliferating and hypertrophic chondrocytes of normal growth plate in vivo (Iftikhar et al., 2011). The general histologic structure of joint cartilage (mainly including growth plate and articular cartilage) has been presented in Figure 1.
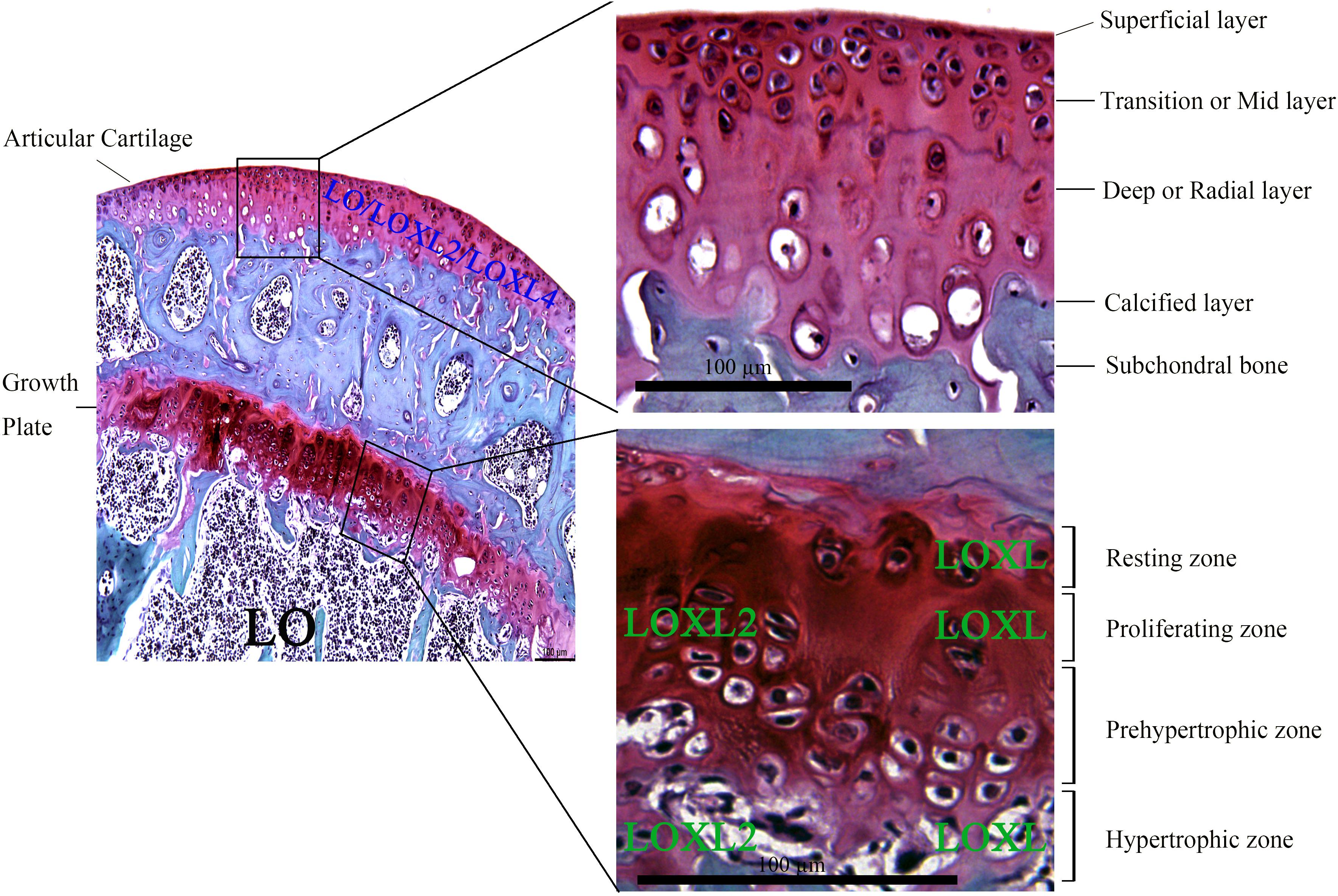
Figure 1. Histology of growth plate and articular cartilage through Safranin O-Fast green staining of the proximal tibia from adult C57BL/6 mice. Mature articular cartilage is mainly comprised of four zones based on histologic features: superficial layer, transition or mid (middle) layer, deep or radial layer, and calcified layer that is lined by subchondral bone. Growth plate is mainly characterized by several morphologically distinct zones, including resting zone, proliferating zone, prehypertrophic zone, and hypertrophic zone. LO is mainly expressed in marrow cavity, while LOXL2 expression is mainly localized in proliferating and hypertrophic zones of growth plate. LOXL was mainly found on chondrocytes of resting, proliferating and hypertrophic zones of growth plate. The expression of LO, LOXL2, and LOXL4 was descended from superficial to deep layers of articular cartilage. Lysyl oxidase: LO; lysyl oxidase like-2: LOXL2; lysyl oxidase like-4: LOXL4; Lysyl oxidase-like enzymes: LOXL. Scale bar: 100 μm.
Notably, the activity of LOX is of pivotal importance for maintaining the tensile and elastic features of connective tissues in the musculoskeletal (Weiner and Traub, 1992; Herchenhan et al., 2015), cardiovascular and pulmonary systems (Ohmura et al., 2012; Nave et al., 2014). In cartilage, LOX is capable of modification of amino acids lysine and hydroxylysine into covalent PYR cross-linking (i.e., heterotypic collagen II/IX/XI) (Eyre et al., 2004), in particular the most abundant type of cross-links in native articular cartilage, which is tightly correlated with the tensile properties of native articular cartilage (Williamson et al., 2003; Eyre et al., 2008). Nevertheless, inactivation of LOXs induced by copper metabolic disorder or gene mutation would lead to dysfunction of connective tissues and collagen-containing organs (Kuivaniemi et al., 1982; Maki et al., 2002). To date, the discovery of crystal structures of copper-containing amine oxidase and lysyl oxidase-like 2 has been reported in the current literature (Figure 2) (Duff et al., 2003, 2004; Lunelli et al., 2005; Zhang X. et al., 2018).
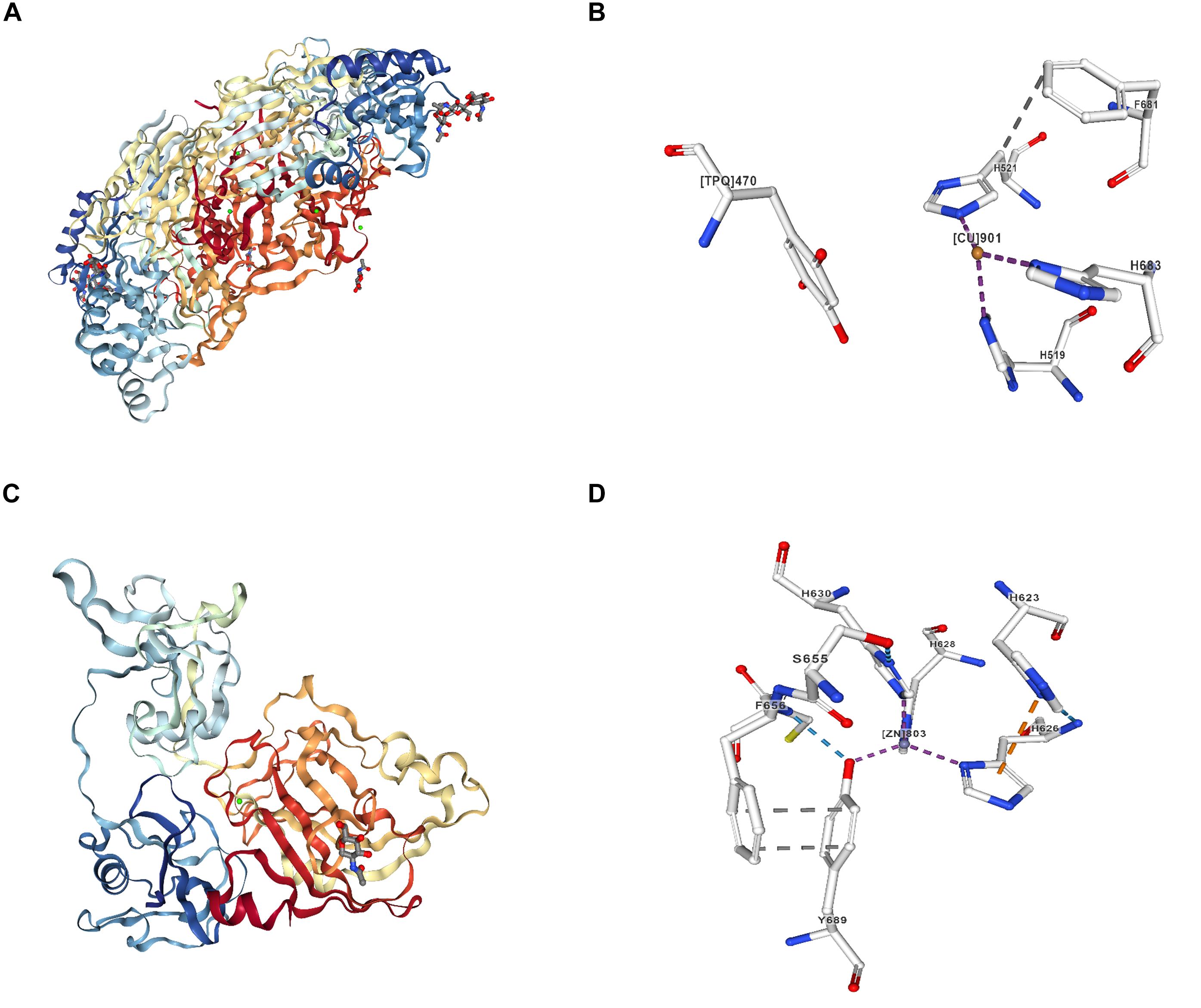
Figure 2. Crystal structures and ligand interactions of copper-containing amine oxidase and human lysyl oxidase-like 2 (http://www.rcsb.org/). (A) Copper- containing amine oxidase extracted from bovine serum (BSAO) was crystallized and its three-dimensional structure at 2.37A resolution. The biological unit of BSAO is a homodimer, formed by two monomers related to each other by a non-crystallographic 2-fold axis. Each monomer is composed of three domains. (B) Ligand interaction of copper iron [(CU)901] in copper-containing amine oxidase extracted from BSAO. (C) Crystal structure of human lysyl oxidase-like 2 (hLOXL2) at 2.4-Å resolution. (D) Ligand interaction of zinc iron ([ZN]803:A) in hLOXL2. The copper-binding site of hLOXL2 is occupied by zinc, which blocks lysyl tyrosylquinone (LTQ) generation and the enzymatic activity of hLOXL2. The LTQ precursor residues in the structure are distanced by 16.6 Å, corroborating the notion that the present structure may represent a precursor state and that pronounced conformational rearrangements would be required for protein activation.
To date, the LOX family has been reported essential for cartilage maturation, chondroprotection, and homeostasis maintenance of cartilage. LO and LOXL-3b have been demonstrated crucial for cartilage maturation during zebrafish development, respectively (Reynaud et al., 2008; van Boxtel et al., 2011). Further, studies have indicated the upregulation of LOXL2 in OA cartilage in response to injury, which may be considered as a naturally protective response that promotes anabolism while inhibiting specific catabolic response during OA pathophysiology (Alshenibr et al., 2017; Bais and Goldring, 2017). Likewise, a recent study further confirmed that systemic adenovirus-delivered LOXL2 expression or LOXL2 genetic overexpression both exhibited chondroprotective effects through inhibition of catabolic factors and IL-1β-induced NF-κB signaling in mice (Tashkandi et al., 2019). Also, Matrigel constructs of human chondrocytes from the knee joint and TMJ implanted in nude mice showed enhanced anabolic responses after LOXL2 transduction, including increased expression of sex determining region Y-box containing gene 9 (SOX9), aggrecan (ACAN), and COL2A1, whilst reduced the levels of extracellular matrix (ECM)-degrading enzymes matrix metalloproteinases and inhibited chondrocyte apoptosis (Alshenibr et al., 2017). Therefore, LOX-mediated collagen cross-links are essential for the functional integrity of articular cartilage and cartilage homeostasis. Appropriate modulation of the expression or activity of certain LOXs family members selectively may become potential promising strategy for cartilage repair.
The components of extracellular matrix are vital for the maintenance of phenotype and function of chondrocytes (Shi et al., 2017; Li et al., 2018; Zhang et al., 2020). The expression of a novel LOX-related gene, named LOXC, has been detected in cartilage in vivo, which modulates the formation of collagenous extracellular matrix (Ito et al., 2001). A series of further studies have confirmed LOX as a key enzyme responsible for the formation of collagen cross-links. Furthermore, hypoxia-induced endogenous LOX expression has been applied in the repair of de novo multiple musculoskeletal tissues (i.e., cartilage, meniscus, tendons, and ligaments) as important regenerative strategies, which is mainly mediated through mechanisms of hypoxia-induced enhanced PYR crosslinking and increased tensile properties of collagen-rich tissues (Makris et al., 2014).
Simultaneously, studies have demonstrated that combined treatment of copper sulfate and hydroxylysine would additively or synergistically enhance collagen cross-linking in engineered articular cartilage, improving the tensile and biomechanical properties of the neocartilage (Makris et al., 2013). LOXL2 promotes chondrogenic differentiation through regulation of SOX9 and SNAIL (Iftikhar et al., 2011). Also, LOX activity has been reported vital for phenotypic modulation of chondrocytes (Farjanel et al., 2005). Therefore, modulation of endogenous LOX activity or expression selectively has been demonstrated effective for promotion of tensile properties and cross-linking of cartilage, as well as phenotypic control of chondrocytes, which appears as promising clinically applicable approaches of regenerative medicine and tissue engineering (Makris et al., 2014; Hadidi et al., 2017) (Table 1).
Regulation of Hypoxia-Inducible Factor-1 in Chondrogenesis and Cartilage Homeostasis
The physiological oxygen tension between 2%∼9% in healthy individuals, which is termed as ‘physiologic normoxia’ (Simon and Keith, 2008). Generally, hypoxia-inducible factor 1 (HIF-1) is expressed in a variety of organs and tissues in healthy mammalians under physiologic normoxic conditions, including brain, kidney, liver, heart, and cartilage (Stroka et al., 2001; Coimbra et al., 2004). Articular cartilage is residing in a hypoxic microenvironment of avascular hypoxic zone in vivo under normal physiological conditions, ranging from 7∼10% oxygen tensions in the superficial zone, and 1% oxygen in the deep zones (Ferrell and Najafipour, 1992). Hypoxia-inducible factor 1α (HIF-1α) have been demonstrated essential for cartilage maturation (Duval et al., 2009; Stegen et al., 2019). Also, local activation of HIF-1α is necessary for survival and homeostatic function of chondrocytes, as well as normal joint development (Aro et al., 2012; Long, 2019). Thus, hypoxia and HIF-1 have been exploited to modulate chondrocyte phenotype and represent an efficient approach to improve cell properties before implantation for cartilage repair.
Notably, HIF-1 is of pivotal significance for survival and growth arrest of chondrocytes during cartilage development, as well as cartilage homeostasis of osteoarthritic cartilage (Pfander et al., 2006; Gelse et al., 2008). HIF-1 is conducive to the maintenance of chondrogenic specific markers (SOX9, type II collagen, and aggrecan) and inhibition of cartilage hypertrophy (Duval et al., 2012). HIF-1 has been demonstrated as a positive regulator of SOX9 activity, which is required for chondrogenesis and synthesis of cartilage ECM (Robins et al., 2005; Zhang et al., 2011). Nevertheless, dysregulation of HIF-1α signaling axis would lead to skeletal dysplasia by interfering with cellular bioenergetics and biosynthesis (Stegen et al., 2019). A recent study has indicated the increased expression of genes involved in matrix degradation, hypoxia-responsive, and inflammatory signaling in damaged cartilages comparing with healthy counterparts through whole genome microarray analysis, suggesting potential involvement of HIF-1α during the progression of OA pathophysiology (Yudoh et al., 2005; Aşık et al., 2019), which may be a natural protection response of the body since previous studies have demonstrated chondroprotection efficacy through activation of HIF-1 signaling axis (Gelse et al., 2008; Maes et al., 2012; Lin R. et al., 2019). Further comprehensive studies elucidating detailed roles of HIF-1α in certain stages of OA pathogenic progression, as well as detailed mechanisms on hypoxia-induced LOXs expression and activity, require further elucidation. Therefore, appropriate modulation of transcriptional activity of HIF-1α may become a potential feasible therapeutic approach for cartilage regeneration.
Chondroprotective Effects of Copper in Cartilage
Copper, an essential redox-active trace element, which is essential for most aerobic organisms (Tapiero et al., 2003; Solomon et al., 2014). Simultaneously, copper functions as a co-factor of various proteins and enzymes, including cytochrome C, superoxide dismutase, tyrosinase, ascorbate oxidase, lysyl oxidase, and amine oxidase, exhibiting diverse fundamental cellular functions in normal physiology, including energy generation, iron acquisition, oxygen transportation, cellular metabolism, peptide hormone maturation, blood clotting, neurotransmitter biosynthesis, and intracellular signal transduction (Huffman and O’Halloran, 2001; Hamza and Gitlin, 2002; Kim et al., 2008; Turski et al., 2012; Grubman and White, 2014; Wang et al., 2018; Miller et al., 2019). Generally, copper is able to exist in two oxidation states in the body of mammalians: Cu+ and Cu2+ (Lin and Kosman, 1990; Pushie et al., 2007; Solomon et al., 2014). The trafficking of copper into specific intracellular targets is delivered by metallochaperones (Hamza et al., 2001; Puig and Thiele, 2002; Chen G. F. et al., 2015). And there is no free copper in the cytoplasm under normal physiological conditions (Rae et al., 1999).
In general, moderate copper levels are essential for normal growth, development, health, such as the normal functioning of innate immune system (Djoko et al., 2015; Bost et al., 2016), and bone health (Eaton-Evans et al., 1996; Qu et al., 2018). Of note, copper is also vital for maintenance of integrity and homeostasis of cartilage tissues. Copper metabolic disorder correlates closely with ischemic cardiovascular diseases (Jiang et al., 2007; Kim et al., 2010), embryonic and neonatal abnormalities, and anemia (Cartwright and Wintrobe, 1964; Jensen et al., 2019), as well as the onset of osteoarthritis (Scudder et al., 1978; Yazar et al., 2005).
Supplementation of dietary copper has been reported to reduce the severity of osteochondrosis and other developmental cartilage lesions, which may result from enhanced collagen cross-linking and increased collagen II synthesis (Knight et al., 1990; Hurtig et al., 1993; Yuan et al., 2011). The chondroprotection efficacy of copper may be attributable to the anti-catabolic effects of Cu2+, which abrogates the degradation of cartilage matrix proteoglycan via inhibition of nitric oxide release (Pasqualicchio et al., 1996). A recent study has reported that copper-incorporated bioactive glass-ceramics facilitated the regeneration of cartilage and osteochondral interface effectively, which was mediated in part through activated HIF-1 signaling and inhibited inflammatory response, representing a feasible approach for treating osteoarthritis associated with osteochondral defects (Lin R. et al., 2019). However, until now, the detailed mechanisms of interactions between copper and chondrocytes, as well as copper trafficking within chondrocytes remain elusive. To date, copper has been identified as a cofactor of several identified major cartilage formation-associated enzymes (Rucker et al., 1998; Heraud et al., 2002; Makris et al., 2013), however, cellular and molecular mechanisms underlying intracellular copper transportation in chondrocytes are not yet clearly understood. A cartilage matrix glycoprotein, a membrane-associated protein synthesized by chondrocytes, has been demonstrated to bind copper and exert some oxidase activity similar with ceruloplasmin, which may function as an important copper transporter in chondrocytes and a potential chondrogenic marker (Fife et al., 1986, 1993; Harris, 2000; Ranganathan et al., 2011; La Mendola et al., 2012; Ishihara et al., 2014; Linder, 2016; Magri et al., 2018). The proposed cellular model of copper intracellular transportation has been presented in Figure 3 according to current literature (Okado-Matsumoto and Fridovich, 2001; Carr et al., 2005; Horng et al., 2005; Turski and Thiele, 2009; Ohrvik and Thiele, 2014; Urso and Maffia, 2015; Miller et al., 2019; Shi et al., 2019), illustrating the routes of copper trafficking and how it functions within chondrocytes and during ECM remodeling.
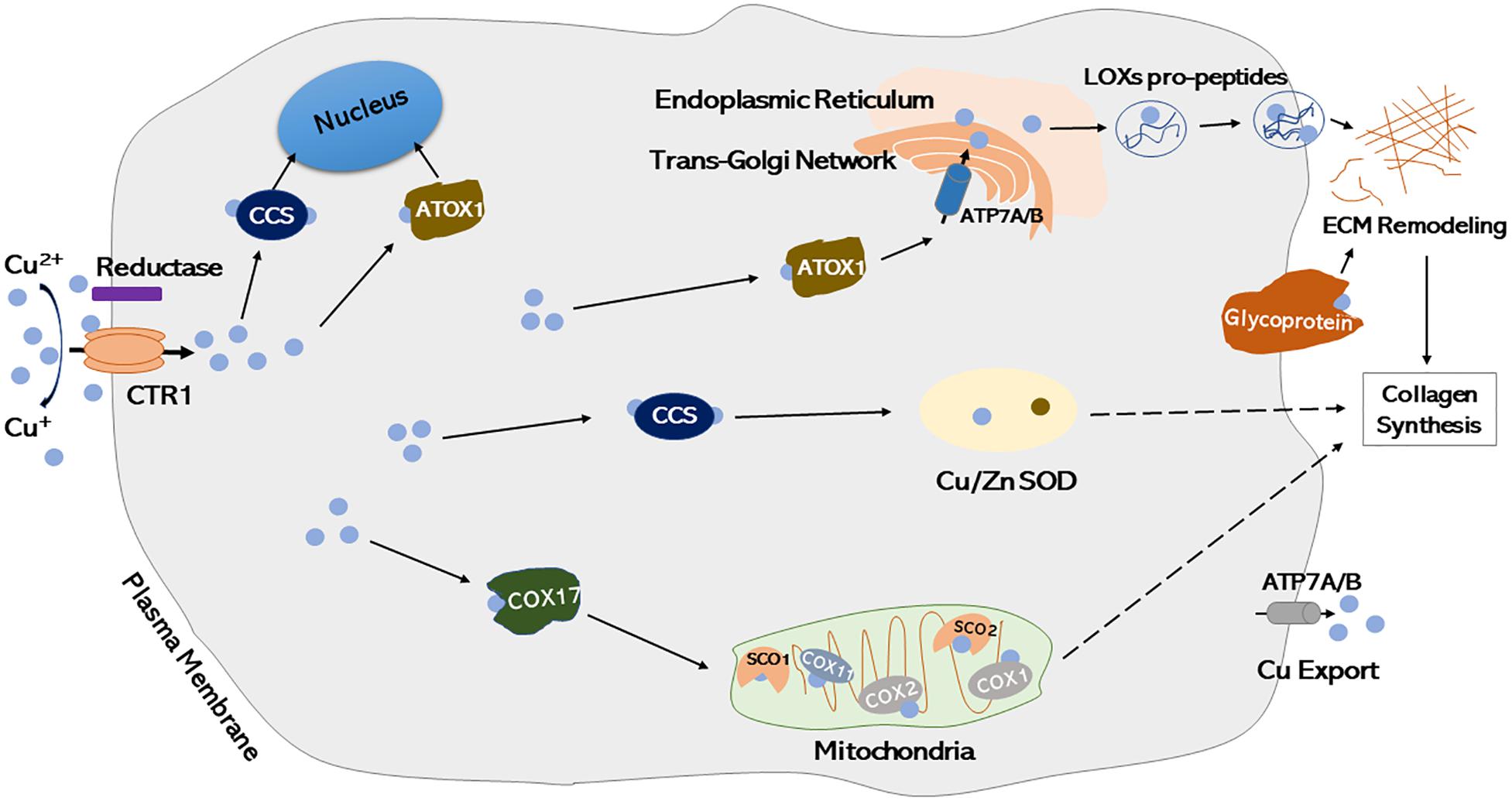
Figure 3. Proposed cellular models of intracellular copper trafficking in chondrocytes. Cu2+ is transformed to Cu+ by a putative metalloreductase, which then enters the cell through plasma membrane importer CTR1. Once inside the cell, copper is handed off to copper chaperones. CCS incorporated copper into the cytosolic protein Cu/Zn-SOD. ATOX1 delivers copper into secretory compartments of Golgi and Endoplasmic Reticulum through ATP7A/B. Copper can be incorporated into copper-dependent proteins, such as LOXs pro-peptides (secreted forms of LOXs), which is involved in ECM remodeling. Once inside the intermembrane space of mitochondria, copper is handed off to COX17 and then passed onto either SCO1, which then transfers copper to COX2 subunit of cytochrome oxidase, or COX11, which transfers copper to COX1 subunit of cytochrome oxidase. Copper exporter ATP7A/B exports copper out of the cell by translocating to plasma membrane when intracellular copper concentrations are high. Glycoprotein interacts with copper and regulates ECM remodeling. Cu, Copper; Copper-transporter 1, CTR1 (a major copper importer); CCS, Copper chaperone for superoxide dismutase; Cu/Zn-SOD, copper/zinc-superoxide dismutase; ATOX1, a copper chaperone, also known as HAH1; LOXs, lysyl oxidases; COX, cytochrome oxidase; SCO, synthesis of cytochrome c oxidase; ATP, copper transporting P-type ATPases [systemic copper absorption (ATP7A) and copper excretion (ATP7B)]; ECM, extracellular matrix.
Further, emerging evidence has indicated that copper stabilizes the HIF-1α protein through inhibiting prolyl hydroxylases-mediated prolyl hydroxylation in an iron-independent manner, which is required for transcriptional activation of HIF-1 of a series of target genes (Martin et al., 2005; Feng et al., 2009; Himoto et al., 2016; Liu et al., 2018; Chen et al., 2020), suggesting that appropriate copper levels may be required for normal cartilage function through regulation of HIF-1α transcriptional activity. However, detailed mechanisms of transcriptional processes initiating specific target genes expression of HIF-1 require further elucidation. Therefore, copper may modulate cartilage homeostasis through regulation of activity of HIF-1 transcription and chondrogenic-associated proteins and markers, such as LOXL2, and SOX9 (Robins et al., 2005; Amarilio et al., 2007; Schietke et al., 2010), as well as VEGF, which is essential for chondrocyte survival (Cramer et al., 2004; Maes et al., 2004, 2012) (Figure 4). Also, further extensive studies on regulation of HIF-1 expression (such as heat shock protein 90, HSP90), and specific targeting of certain transcriptional activation of certain HIF-1 target genes (Minet et al., 1999; Isaacs et al., 2002; Katschinski et al., 2004), as well as regulation of systemic copper metabolism are promising for potential therapeutic optimization (Isaacs et al., 2002; Kim et al., 2010).
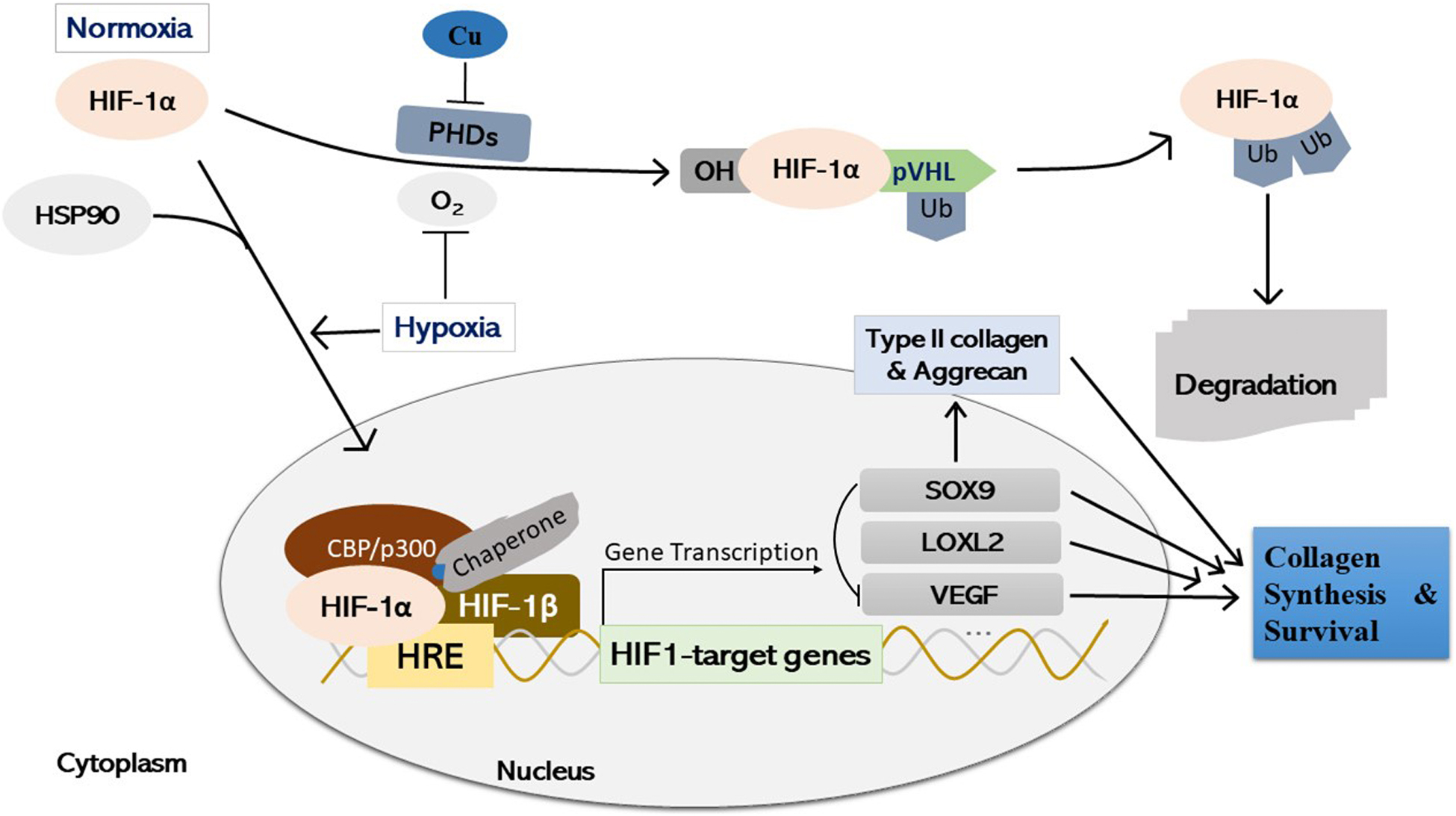
Figure 4. Schematic illustration of copper-mediated hypoxia-response element (HRE)-directed transcriptional fine-tuning of cartilage homeostasis-associated genes in chondrocytes. Under normoxia, HIF-1α undergoes PHDs-mediated prolyl hydroxylation, and prolyl OH HIF-1α is ligated by pVHL, an E3 ubiquitin ligase, and degraded by the proteasome finally. Copper stabilizes HIF-1α protein by inhibiting PHDs activity in an iron-independent manner. Under hypoxia or through interaction with HSP90, HIF-1α stabilizes and accumulates in the cell nucleus, where it forms a dimer with the HIF-1β subunit and a putative unidentified copper-chaperone. The dimer then forms a transcriptional complex with coactivator CBP/p300 through binding with HRE, regulating the expression of various downstream target genes, such as LOXL2, SOX9, and VEGF. Simultaneously, SOX9 is a negative regulator of VEGF, whilst the expression of SOX9 target genes (i.e., Type II collagen, and Aggrecan) is initiated, which is essential for cartilage synthesis and survival during both embryonic joint development and cartilage homeostasis. HIF-1α, hypoxia-inducible factor-1α; PHDs, prolyl hydroxylases; OH: enzymatic hydroxylation; pVHL, von Hippel-Lindau tumor suppressor protein; HRE, hypoxia-response element; Ub, ubiquitinated; SOX9, SRY (sex determining region Y)-box 9; HSP90, heat shock protein 90; LOXL2, lysyl oxidase-like 2; VEGF, vascular endothelial growth factor; Cu, copper.
Potential Links Between LOX and Aging-Associated Cartilage Degeneration
Aging, which is characterized by a chronic and low-grade inflammation, also termed as age-associated inflammation, which has been referred to as ‘inflamm-aging’ (Franceschi and Campisi, 2014; Huang et al., 2019; Josephson et al., 2019), is also a risk factor of osteoarthritis (Greene and Loeser, 2015). Inflammation is a normal process in healthy individuals. Acute inflammation initiates the regenerative response (Kyritsis et al., 2012). Whilst chronic inflammation is likely to cause various diseases (Akhurst et al., 2005; Liu et al., 2019). The signaling pathways that are implicated in chronic inflammation include NF-κB (Roman-Blas and Jimenez, 2008; Bamborough et al., 2010), signal transducer and activator of transcription (STAT) (He and Karin, 2011), mitogen-activated protein kinases (MAPKS) (Thalhamer et al., 2008). Interestingly, a recent study reported that LOXL3-mediated deacetylation/deacetylimination abolished the transcription activity of STAT3, thereby inhibiting differentiation of naïve CD4+ T cells toward Th17/Treg cells (regulatory T cells) during inflammatory responses (Ma et al., 2017).
Aging-associated destruction of joints and cartilage degradation in osteoarthritis is correlated with changes in extracellular matrix of articular cartilage, such as cartilage ECM stiffness, and in the levels and solubility profiles of matrix crosslinks, especially pentosidine, as well as reduced thickness of cartilage, proteolysis, advanced glycation and calcification (Eyre et al., 1988; Pokharna et al., 1995; Lotz and Loeser, 2012). Notably, rejuvenation has emerged as a promising therapeutic regenerative approach for improvement or restoration of the self-repair capacity of injured or aging tissue and organ systems (Leung et al., 2006; Nelson et al., 2008; Luria and Chu, 2014; Sarkar et al., 2020), which has been proposed as a conversion into an embryonic-like state recapitulating many events during embryogenesis, including the reactivation of embryonic signature genes, and cytoskeletal/ECM components, and lineage specification (Vortkamp et al., 1998; Jankowski et al., 2009; Luo et al., 2009; Adam et al., 2015; Caldwell and Wang, 2015; Hu et al., 2017; Ransom et al., 2018; Feng et al., 2019; Lin W. et al., 2019; Miao et al., 2019). Consistently, the process of cartilage repair has been considered as recapitulation of various events during developmental morphogenesis. Chondrocytes in osteoarthritic articular cartilage usually undergoes a gradual dissolution of anisotropic organization along with re-expression of phenotypic biomarkers of immature cartilage, so tissue maturation is a potential approach for restoration of normal structure and function (Caterson et al., 1990; Khan et al., 2008; Hunziker, 2009; Jiang et al., 2015; Zhang et al., 2017).
Interestingly, copper is also involved in inflammatory responses, including both innate and adaptive immunity (Percival, 1998; Failla, 2003). Increasing evidence has indicated the potential link between copper metabolic disorder and aging-related diseases, such as aging-induced cartilage degradation and dysfunction (Yazar et al., 2005; Lotz and Loeser, 2012; Tao et al., 2019). And previous studies have demonstrated the vital roles of LOX in normal chondrocyte function (Sanada et al., 1978; Ahsan et al., 1999), which may be correlated with the pathogenesis of aging-associated osteoarthritis (Pokharna et al., 1995). Moreover, LOXL1 is expressed in major organs in late fetal and neonatal mice, but it generally diminishes in aging animals, which may be associated with aortic fragility resulting from abnormal remodeling of collagen and elastic fibers (Hayashi et al., 2004; Behmoaras et al., 2008). The decreased expression of LOXs may attribute to reduction of HIF-1 activity in aging organisms (Rivard et al., 2000; Ceradini et al., 2004). Meanwhile, LOXL2 has recently been demonstrated as a potential chondroprotective factor in aging related joint osteoarthritis, mainly through inducing anabolic gene expression and attenuating catabolic genes (Bais and Goldring, 2017; Tashkandi et al., 2019). Thus, restoration of collagen and elastic fiber synthesis in juvenile ECM components though regulation of signaling pathways governing LOX expression may become a promising therapeutic approach for amelioration of aging-associated cartilage degeneration and enhanced cartilage regeneration.
Meanwhile, changes in cell-ECM interactions are important features of aging phenomenon (Sun et al., 2011). During the progression of aging-associated degeneration diseases, altered cell fate of adult stem cells, or dysfunction of terminally differentiated mature cells occurs, which may result from the changed ECM niche modified with aging-related proteins and reduced expression of ECM-synthesis-associated proteins (Goupille et al., 1998; Chan et al., 2006; Sakai et al., 2012; Wang et al., 2015; Jeon et al., 2017; Zhang Y. et al., 2018; Patil et al., 2019), which may be correlated with the LOX family members. Therefore, reverting aging-associated genes in ECM may become an important strategy for joint rejuvenation (Chan et al., 2006; Fuoco et al., 2014; Li et al., 2014; Sun et al., 2011; Wang et al., 2019; Zhou J. et al., 2019). Modulation of expression of LOXs family members through transcriptional regulation of HIF-1 may become promising therapeutic approaches for treating aging-induced cartilage degeneration as potential rejuvenating therapies.
Concluding Remarks
In summary, LOXs play pivotal roles in maintenance of cartilage function and chondrogenesis. Therapeutic modulation of LOX activity and expression selectively targeting copper-mediated hypoxia-responsive signaling pathways is promising for cartilage repair and OA attenuation. Meanwhile, further extensive basic and preclinical research is warranted for potential translational application of the LOX family in tissue-engineered neocartilage in tissue engineering and regenerative medicine in the future. Specific and moderate manipulation of activities of LOXs and transcriptional regulation of hypoxia-responsive transcription factors through copper bioavailability modulation or continuous hypoxia-conditioning may become effective interventions for enhanced cartilage regeneration, as well as promising rejuvenation therapeutics, which may exert further therapeutic implications in the upcoming clinical arena.
Author Contributions
WL and LX made literature review and contributed equally. GL conceptualized the study and critically revised the manuscript. All authors read and approved the final manuscript.
Funding
This work was partially supported by grants from the National Natural Science Foundation of China (81772322), Hong Kong Government Research Grant Council, General Research Fund (14120118, 14160917, 9054014 N_CityU102/15, C7030-18G, and T13-402/17-N), Hong Kong Innovation Technology Commission Funds (PRP/050/19FX and ITS/448/18), and the Science and Technology Foundation of Guangdong Province (2017A050506046). This study also received support from the SMART program, Lui Che Woo Institute of Innovative Medicine, The Chinese University of Hong Kong.
Conflict of Interest
The authors declare that the research was conducted in the absence of any commercial or financial relationships that could be construed as a potential conflict of interest.
References
Adam, R. C., Yang, H., Rockowitz, S., Larsen, S. B., Nikolova, M., Oristian, D. S., et al. (2015). Pioneer factors govern super-enhancer dynamics in stem cell plasticity and lineage choice. Nature 521, 366–370. doi: 10.1038/nature14289
Ahsan, T., Lottman, L. M., Harwood, F., Amiel, D., and Sah, R. L. (1999). Integrative cartilage repair: inhibition by β-aminopropionitrile. J. Orthop. Res. 17, 850–857. doi: 10.1002/jor.1100170610
Akhurst, B., Matthews, V., Husk, K., Smyth, M. J., Abraham, L. J., and Yeoh, G. C. (2005). Differential lymphotoxin-β and interferon gamma signaling during mouse liver regeneration induced by chronic and acute injury. Hepatology 41, 327–335. doi: 10.1002/hep.20520
Alshenibr, W., Tashkandi, M. M., Alsaqer, S. F., Alkheriji, Y., Wise, A., Fulzele, S., et al. (2017). Anabolic role of lysyl oxidase like-2 in cartilage of knee and temporomandibular joints with osteoarthritis. Arthritis Res. Ther. 19:179. doi: 10.1186/s13075-017-1388-8
Amarilio, R., Viukov, S. V., Sharir, A., Eshkar-Oren, I., Johnson, R. S., and Zelzer, E. (2007). HIF1α regulation of Sox9 is necessary to maintain differentiation of hypoxic prechondrogenic cells during early skeletogenesis. Development 134, 3917–3928. doi: 10.1242/dev.008441
Aro, E., Khatri, R., Gerard-O’Riley, R., Mangiavini, L., Myllyharju, J., and Schipani, E. (2012). Hypoxia-inducible factor-1 (HIF-1) but not HIF-2 is essential for hypoxic induction of collagen prolyl 4-hydroxylases in primary newborn mouse epiphyseal growth plate chondrocytes. J. Biol. Chem. 287, 37134–37144. doi: 10.1074/jbc.M112.352872
Aşık, M. D., Gürsoy, S., Akkaya, M., Kozacı, L. D., Doğan, M., and Bozkurt, M. (2019). Microarray analysis of cartilage: comparison between damaged and non-weight-bearing healthy cartilage. Connect. Tissue Res. doi: 10.1080/03008207.2019.1611797 [Epub ahead of print].
Aspden, R. M. (2011). Obesity punches above its weight in osteoarthritis. Nat. Rev. Rheumatol. 7, 65–68. doi: 10.1038/nrrheum.2010.123
Bais, M. V., and Goldring, M. B. (2017). LOXL2 as a protective in osteoarthritis cartilage. Aging 9, 2024–2025. doi: 10.18632/aging.101317
Bamborough, P., Morse, M. A., and Ray, K. P. (2010). Targeting IKKbeta for the treatment of rheumatoid arthritis. Drug News Perspect. 23, 483–490. doi: 10.1358/dnp.2010.23.8.1447844
Behmoaras, J., Slove, S., Seve, S., Vranckx, R., Sommer, P., and Jacob, M. P. (2008). Differential expression of lysyl oxidases LOXL1 and LOX during growth and aging suggests specific roles in elastin and collagen fiber remodeling in rat aorta. Rejuvenation Res. 11, 883–889. doi: 10.1089/rej.2008.0760
Bignon, M., Pichol-Thievend, C., Hardouin, J., Malbouyres, M., Brechot, N., Nasciutti, L., et al. (2011). Lysyl oxidase-like protein-2 regulates sprouting angiogenesis and type IV collagen assembly in the endothelial basement membrane. Blood 118, 3979–3989. doi: 10.1182/blood-2010-10-313296
Bost, M., Houdart, S., Oberli, M., Kalonji, E., Huneau, J. F., and Margaritis, I. (2016). Dietary copper and human health: current evidence and unresolved issues. J. Trace Elem. Med. Biol. 35, 107–115. doi: 10.1016/j.jtemb.2016.02.006
Busnadiego, O., Gonzalez-Santamaria, J., Lagares, D., Guinea-Viniegra, J., Pichol-Thievend, C., Muller, L., et al. (2013). LOXL4 is induced by transforming growth factor β1 through Smad and JunB/Fra2 and contributes to vascular matrix remodeling. Mol. Cell. Biol. 33, 2388–2401. doi: 10.1128/MCB.00036-13
Caldwell, K. L., and Wang, J. (2015). Cell-based articular cartilage repair: the link between development and regeneration. Osteoarthritis Cartilage 23, 351–362. doi: 10.1016/j.joca.2014.11.004
Carr, H. S., Maxfield, A. B., Horng, Y. C., and Winge, D. R. (2005). Functional analysis of the domains in Cox11. J. Biol. Chem. 280, 22664–22669. doi: 10.1074/jbc.M414077200
Cartwright, G. E., and Wintrobe, M. M. (1964). The question of copper deficiency in man. Am. J. Clin. Nutr. 15, 94–110. doi: 10.1093/ajcn/15.2.94
Caterson, B., Mahmoodian, F., Sorrell, J. M., Hardingham, T. E., Bayliss, M. T., Carney, S. L., et al. (1990). Modulation of native chondroitin sulphate structure in tissue development and in disease. J. Cell Sci. 97(Pt 3), 411–417.
Ceradini, D. J., Kulkarni, A. R., Callaghan, M. J., Tepper, O. M., Bastidas, N., Kleinman, M. E., et al. (2004). Progenitor cell trafficking is regulated by hypoxic gradients through HIF-1 induction of SDF-1. Nat. Med. 10, 858–864. doi: 10.1038/nm1075
Chan, D., Song, Y., Sham, P., and Cheung, K. M. (2006). Genetics of disc degeneration. Eur. Spine J. 15, (Suppl. 3), S317–S325. doi: 10.1007/s00586-006-0171-3
Chen, G. F., Sudhahar, V., Youn, S. W., Das, A., Cho, J., Kamiya, T., et al. (2015). Copper transport protein antioxidant-1 promotes inflammatory neovascularization via chaperone and transcription factor function. Sci. Rep. 5:14780. doi: 10.1038/srep14780
Chen, X., Hu, J. G., Huang, Y. Z., Li, S., Li, S. F., Wang, M., et al. (2020). Copper promotes the migration of bone marrow mesenchymal stem cells via Rnd3-dependent cytoskeleton remodeling. J. Cell. Physiol. 235, 221–231. doi: 10.1002/jcp.28961
Chen, Y., Sun, Y., Pan, X., Ho, K., and Li, G. (2015). Joint distraction attenuates osteoarthritis by reducing secondary inflammation, cartilage degeneration and subchondral bone aberrant change. Osteoarthritis Cartilage 23, 1728–1735. doi: 10.1016/j.joca.2015.05.018
Chen, Y., Zhang, D., Ho, K. W., Lin, S., Suen, W. C., Zhang, H., et al. (2018). GPR120 is an important inflammatory regulator in the development of osteoarthritis. Arthritis Res. Ther. 20:163. doi: 10.1186/s13075-018-1660-6
Coimbra, I. B., Jimenez, S. A., Hawkins, D. F., Piera-Velazquez, S., and Stokes, D. G. (2004). Hypoxia inducible factor-1 α expression in human normal and osteoarthritic chondrocytes. Osteoarthritis Cartilage 12, 336–345. doi: 10.1016/j.joca.2003.12.005
Cox, T. R., Bird, D., Baker, A. M., Barker, H. E., Ho, M. W., Lang, G., et al. (2013). LOX-mediated collagen crosslinking is responsible for fibrosis-enhanced metastasis. Cancer Res. 73, 1721–1732. doi: 10.1158/0008-5472.CAN-12-2233
Cox, T. R., Rumney, R. M. H., Schoof, E. M., Perryman, L., Hoye, A. M., Agrawal, A., et al. (2015). The hypoxic cancer secretome induces pre-metastatic bone lesions through lysyl oxidase. Nature 522, 106–110. doi: 10.1038/nature14492
Cramer, T., Schipani, E., Johnson, R. S., Swoboda, B., and Pfander, D. (2004). Expression of VEGF isoforms by epiphyseal chondrocytes during low-oxygen tension is HIF-1 α dependent. Osteoarthritis Cartilage 12, 433–439. doi: 10.1016/j.joca.2004.02.003
Danielsen, C. C. (1982). Mechanical properties of reconstituted collagen fibrils. Influence of a glycosaminoglycan: dermatan sulfate. Connect. Tissue Res. 9, 219–225. doi: 10.3109/03008208209160265
Deng, Z., Gao, X., Sun, X., Amra, S., Lu, A., Cui, Y., et al. (2019). Characterization of articular cartilage homeostasis and the mechanism of superior cartilage regeneration of MRL/MpJ mice. FASEB J. 33, 8809–8821. doi: 10.1096/fj.201802132RR
Djoko, K. Y., Ong, C. L., Walker, M. J., and McEwan, A. G. (2015). The role of copper and zinc toxicity in innate immune defense against bacterial pathogens. J. Biol. Chem. 290, 18954–18961. doi: 10.1074/jbc.R115.647099
Duff, A. P., Cohen, A. E., Ellis, P. J., Kuchar, J. A., Langley, D. B., Shepard, E. M., et al. (2003). The crystal structure of Pichia pastoris lysyl oxidase. Biochemistry 42, 15148–15157. doi: 10.1021/bi035338v
Duff, A. P., Trambaiolo, D. M., Cohen, A. E., Ellis, P. J., Juda, G. A., Shepard, E. M., et al. (2004). Using xenon as a probe for dioxygen-binding sites in copper amine oxidases. J. Mol. Biol. 344, 599–607. doi: 10.1016/j.jmb.2004.09.075
Duval, E., Bauge, C., Andriamanalijaona, R., Benateau, H., Leclercq, S., Dutoit, S., et al. (2012). Molecular mechanism of hypoxia-induced chondrogenesis and its application in in vivo cartilage tissue engineering. Biomaterials 33, 6042–6051. doi: 10.1016/j.biomaterials.2012.04.061
Duval, E., Leclercq, S., Elissalde, J. M., Demoor, M., Galera, P., and Boumediene, K. (2009). Hypoxia-inducible factor 1α inhibits the fibroblast-like markers type I and type III collagen during hypoxia-induced chondrocyte redifferentiation: hypoxia not only induces type II collagen and aggrecan, but it also inhibits type I and type III collagen in the hypoxia-inducible factor 1α-dependent redifferentiation of chondrocytes. Arthritis Rheum. 60, 3038–3048. doi: 10.1002/art.24851
Eaton-Evans, J., Mcllrath, E., Jackson, W., McCartney, H., and Strain, J. J. (1996). Copper supplementation and the maintenance of bone mineral density in middle-aged women. J. Trace Elem. Exp. Med. 9, 87–94. doi: 10.1002/(sici)1520-670x(1996)9:3<87::aid-jtra1>3.0.co;2-e
Eyre, D. R., Dickson, I. R., and Van Ness, K. (1988). Collagen cross-linking in human bone and articular cartilage. Age-related changes in the content of mature hydroxypyridinium residues. Biochem. J. 252, 495–500. doi: 10.1042/bj2520495
Eyre, D. R., Pietka, T., Weis, M. A., and Wu, J. J. (2004). Covalent cross-linking of the NC1 domain of collagen type IX to collagen type II in cartilage. J. Biol. Chem. 279, 2568–2574. doi: 10.1074/jbc.M311653200
Eyre, D. R., Weis, M. A., and Wu, J. J. (2008). Advances in collagen cross-link analysis. Methods 45, 65–74. doi: 10.1016/j.ymeth.2008.01.002
Failla, M. L. (2003). Trace elements and host defense: recent advances and continuing challenges. J. Nutr. 133(5 Suppl. 1), 1443S–1447S. doi: 10.1093/jn/133.5.1443S
Farjanel, J., Seve, S., Borel, A., Sommer, P., and Hulmes, D. J. (2005). Inhibition of lysyl oxidase activity can delay phenotypic modulation of chondrocytes in two-dimensional culture. Osteoarthritis Cartilage 13, 120–128. doi: 10.1016/j.joca.2004.06.015
Feng, C., Chan, W. C. W., Lam, Y., Wang, X., Chen, P., Niu, B., et al. (2019). Lgr5 and Col22a1 mark progenitor cells in the lineage toward juvenile Articular chondrocytes. Stem Cell Rep. 13, 713–729. doi: 10.1016/j.stemcr.2019.08.006
Feng, W., Ye, F., Xue, W., Zhou, Z., and Kang, Y. J. (2009). Copper regulation of hypoxia-inducible factor-1 activity. Mol. Pharmacol. 75, 174–182. doi: 10.1124/mol.108.051516
Ferrell, W. R., and Najafipour, H. (1992). Changes in synovial PO2 and blood flow in the rabbit knee joint due to stimulation of the posterior articular nerve. J. Physiol. 449, 607–617. doi: 10.1113/jphysiol.1992.sp019104
Fife, R. S., Kluve-Beckerman, B., Houser, D. S., Proctor, C., Liepnieks, J., Masuda, I., et al. (1993). Evidence that a 550,000-dalton cartilage matrix glycoprotein is a chondrocyte membrane-associated protein closely related to ceruloplasmin. J. Biol. Chem. 268, 4407–4411.
Fife, R. S., Palmoski, M. J., and Brandt, K. D. (1986). Metabolism of a cartilage matrix glycoprotein in normal and osteoarthritic canine articular cartilage. Arthritis Rheum. 29, 1256–1262. doi: 10.1002/art.1780291011
Flores, R. R., Carbo, L., Kim, E., Van Meter, M., De Padilla, C. M. L., Zhao, J., et al. (2019). Adenoviral gene transfer of a single-chain IL-23 induces psoriatic arthritis-like symptoms in NOD mice. FASEB J. 33, 9505–9515. doi: 10.1096/fj.201900420R
Franceschi, C., and Campisi, J. (2014). Chronic inflammation (inflammaging) and its potential contribution to age-associated diseases. J. Gerontol. A Biol. Sci. Med. Sci. 69, (Suppl. 1), S4–S9. doi: 10.1093/gerona/glu057
Fu, W. L., Zhou, C. Y., and Yu, J. K. (2014). A new source of mesenchymal stem cells for articular cartilage repair: MSCs derived from mobilized peripheral blood share similar biological characteristics in vitro and chondrogenesis in vivo as MSCs from bone marrow in a rabbit model. Am. J. Sports Med. 42, 592–601. doi: 10.1177/0363546513512778
Fuoco, C., Sangalli, E., Vono, R., Testa, S., Sacchetti, B., Latronico, M. V., et al. (2014). 3D hydrogel environment rejuvenates aged pericytes for skeletal muscle tissue engineering. Front. Physiol. 5:203. doi: 10.3389/fphys.2014.00203
Gao, S., Zhou, J., Zhao, Y., Toselli, P., and Li, W. (2013). Hypoxia-response element (HRE)-directed transcriptional regulation of the rat lysyl oxidase gene in response to cobalt and cadmium. Toxicol. Sci. 132, 379–389. doi: 10.1093/toxsci/kfs327
Gelse, K., Muhle, C., Knaup, K., Swoboda, B., Wiesener, M., Hennig, F., et al. (2008). Chondrogenic differentiation of growth factor-stimulated precursor cells in cartilage repair tissue is associated with increased HIF-1α activity. Osteoarthritis Cartilage 16, 1457–1465. doi: 10.1016/j.joca.2008.04.006
Goupille, P., Jayson, M. I., Valat, J. P., and Freemont, A. J. (1998). Matrix metalloproteinases: the clue to intervertebral disc degeneration? Spine 23, 1612–1626. doi: 10.1097/00007632-199807150-00021
Greene, M. A., and Loeser, R. F. (2015). Aging-related inflammation in osteoarthritis. Osteoarthritis Cartilage 23, 1966–1971. doi: 10.1016/j.joca.2015.01.008
Griffin, T. M., and Scanzello, C. R. (2019). Innate inflammation and synovial macrophages in osteoarthritis pathophysiology. Clin. Exp. Rheumatol. 37, (Suppl. 120), 57–63.
Grubman, A., and White, A. R. (2014). Copper as a key regulator of cell signalling pathways. Expert Rev. Mol. Med. 16:e11. doi: 10.1017/erm.2014.11
Hadidi, P., Cissell, D. D., Hu, J. C., and Athanasiou, K. A. (2017). Temporal development of near-native functional properties and correlations with qMRI in self-assembling fibrocartilage treated with exogenous lysyl oxidase homolog 2. Acta Biomater. 64, 29–40. doi: 10.1016/j.actbio.2017.09.035
Hamza, I., Faisst, A., Prohaska, J., Chen, J., Gruss, P., and Gitlin, J. D. (2001). The metallochaperone Atox1 plays a critical role in perinatal copper homeostasis. Proc. Natl. Acad. Sci. U.S.A. 98, 6848–6852. doi: 10.1073/pnas.111058498
Hamza, I., and Gitlin, J. D. (2002). Copper chaperones for cytochrome c oxidase and human disease. J. Bioenerg. Biomembr. 34, 381–388. doi: 10.1023/a:1021254104012
Harris, E. D. (2000). Cellular copper transport and metabolism. Annu. Rev. Nutr. 20, 291–310. doi: 10.1146/annurev.nutr.20.1.291
Hayashi, K., Fong, K. S., Mercier, F., Boyd, C. D., Csiszar, K., and Hayashi, M. (2004). Comparative immunocytochemical localization of lysyl oxidase (LOX) and the lysyl oxidase-like (LOXL) proteins: changes in the expression of LOXL during development and growth of mouse tissues. J. Mol. Histol. 35, 845–855. doi: 10.1007/s10735-004-2340-1
He, G., and Karin, M. (2011). NF-κB and STAT3 - key players in liver inflammation and cancer. Cell Res. 21, 159–168. doi: 10.1038/cr.2010.183
Heraud, F., Savineau, C., and Harmand, M. F. (2002). Copper modulation of extracellular matrix synthesis by human articular chondrocytes. Scand. J. Rheumatol. 31, 279–284. doi: 10.1080/030097402760375179
Herchenhan, A., Uhlenbrock, F., Eliasson, P., Weis, M., Eyre, D., Kadler, K. E., et al. (2015). Lysyl oxidase activity is required for ordered collagen fibrillogenesis by tendon cells. J. Biol. Chem. 290, 16440–16450. doi: 10.1074/jbc.M115.641670
Himoto, T., Fujita, K., Nomura, T., Tani, J., Miyoshi, H., Morishita, A., et al. (2016). Roles of copper in hepatocarcinogenesis via the activation of hypoxia-inducible factor-1α. Biol. Trace Elem. Res. 174, 58–64. doi: 10.1007/s12011-016-0702-7
Horng, Y. C., Leary, S. C., Cobine, P. A., Young, F. B., George, G. N., Shoubridge, E. A., et al. (2005). Human Sco1 and Sco2 function as copper-binding proteins. J. Biol. Chem. 280, 34113–34122. doi: 10.1074/jbc.M506801200
Hu, D. P., Ferro, F., Yang, F., Taylor, A. J., Chang, W., Miclau, T., et al. (2017). Cartilage to bone transformation during fracture healing is coordinated by the invading vasculature and induction of the core pluripotency genes. Development 144, 221–234. doi: 10.1242/dev.130807
Huang, B., Wang, B., Yuk-Wai Lee, W., Pong, U. K., Leung, K. T., Li, X., et al. (2019). KDM3A and KDM4C regulate mesenchymal stromal cell senescence and bone aging via condensin-mediated heterochromatin reorganization. iScience 21, 375–390. doi: 10.1016/j.isci.2019.10.041
Huang, W., Sung, K. P., Xie, J., and Yang, J. J. (2010). Expression of lysyl oxidase family in different zones of articular cartilage. Chin. J. Trauma 26, 934–936.
Huey, D. J., Hu, J. C., and Athanasiou, K. A. (2012). Unlike bone, cartilage regeneration remains elusive. Science 338, 917–921. doi: 10.1126/science.1222454
Huffman, D. L., and O’Halloran, T. V. (2001). Function, structure, and mechanism of intracellular copper trafficking proteins. Annu. Rev. Biochem. 70, 677–701. doi: 10.1146/annurev.biochem.70.1.677
Hunziker, E. B. (2009). The elusive path to cartilage regeneration. Adv. Mater. 21, 3419–3424. doi: 10.1002/adma.200801957
Hurtig, M., Green, S. L., Dobson, H., Mikuni-Takagaki, Y., and Choi, J. J. E. V. J. (1993). Correlative study of defective cartilage and bone growth in foals fed a low-copper diet. Equine Vet. J. 25, 66–73. doi: 10.1111/j.2042-3306.1993.tb04857.x
Iftikhar, M., Hurtado, P., Bais, M. V., Wigner, N., Stephens, D. N., Gerstenfeld, L. C., et al. (2011). Lysyl oxidase-like-2 (LOXL2) is a major isoform in chondrocytes and is critically required for differentiation. J. Biol. Chem. 286, 909–918. doi: 10.1074/jbc.M110.155622
Isaacs, J. S., Jung, Y.-J., Mimnaugh, E. G., Martinez, A., Cuttitta, F., and Neckers, L. M. (2002). Hsp90 regulates a von Hippel Lindau-independent hypoxia-inducible factor-1α-degradative pathway. J. Biol. Chem. 277, 29936–29944. doi: 10.1074/jbc.m204733200
Ishihara, T., Kakiya, K., Takahashi, K., Miwa, H., Rokushima, M., Yoshinaga, T., et al. (2014). Discovery of novel differentiation markers in the early stage of chondrogenesis by glycoform-focused reverse proteomics and genomics. Biochim. Biophys. Acta 1840, 645–655. doi: 10.1016/j.bbagen.2013.10.027
Ito, H., Akiyama, H., Iguchi, H., Iyama, K., Miyamoto, M., Ohsawa, K., et al. (2001). Molecular cloning and biological activity of a novel lysyl oxidase-related gene expressed in cartilage. J. Biol. Chem. 276, 24023–24029. doi: 10.1074/jbc.M100861200
Jankowski, M. P., McIlwrath, S. L., Jing, X., Cornuet, P. K., Salerno, K. M., Koerber, H. R., et al. (2009). Sox11 transcription factor modulates peripheral nerve regeneration in adult mice. Brain Res. 1256, 43–54. doi: 10.1016/j.brainres.2008.12.032
Jensen, E. L., Gonzalez-Ibanez, A. M., Mendoza, P., Ruiz, L. M., Riedel, C. A., Simon, F., et al. (2019). Copper deficiency-induced anemia is caused by a mitochondrial metabolic reprograming in erythropoietic cells. Metallomics 11, 282–290. doi: 10.1039/c8mt00224j
Jeon, O. H., Kim, C., Laberge, R. M., Demaria, M., Rathod, S., Vasserot, A. P., et al. (2017). Local clearance of senescent cells attenuates the development of post-traumatic osteoarthritis and creates a pro-regenerative environment. Nat. Med. 23, 775–781. doi: 10.1038/nm.4324
Jiang, Y., Hu, C., Yu, S., Yan, J., Peng, H., Ouyang, H. W., et al. (2015). Cartilage stem/progenitor cells are activated in osteoarthritis via interleukin-1β/nerve growth factor signaling. Arthritis Res. Ther. 17:327. doi: 10.1186/s13075-015-0840-x
Jiang, Y., Reynolds, C., Xiao, C., Feng, W., Zhou, Z., Rodriguez, W., et al. (2007). Dietary copper supplementation reverses hypertrophic cardiomyopathy induced by chronic pressure overload in mice. J. Exp. Med. 204, 657–666. doi: 10.1084/jem.20061943
Josephson, A. M., Bradaschia-Correa, V., Lee, S., Leclerc, K., Patel, K. S., Muinos Lopez, E., et al. (2019). Age-related inflammation triggers skeletal stem/progenitor cell dysfunction. Proc. Natl. Acad. Sci. U.S.A. 116, 6995–7004. doi: 10.1073/pnas.1810692116
Kagan, H. M., and Li, W. (2003). Lysyl oxidase: properties, specificity, and biological roles inside and outside of the cell. J. Cell. Biochem. 88, 660–672. doi: 10.1002/jcb.10413
Katschinski, D., Le, L., Schindler, S., Thomas, T., Voss, A., Wenger, R. J. C. P., et al. (2004). Interaction of the PAS B domain with HSP90 accelerates hypoxia-inducible factor-1α stabilization. Cell. Physiol. Biochem. 14, 351–360. doi: 10.1159/000080345
Khan, I. M., Gilbert, S. J., Caterson, B., Sandell, L. J., and Archer, C. W. (2008). Oxidative stress induces expression of osteoarthritis markers procollagen IIA and 3B3(-) in adult bovine articular cartilage. Osteoarthritis Cartilage 16, 698–707. doi: 10.1016/j.joca.2007.10.004
Kim, B. E., Nevitt, T., and Thiele, D. J. (2008). Mechanisms for copper acquisition, distribution and regulation. Nat. Chem. Biol. 4, 176–185. doi: 10.1038/nchembio.72
Kim, B.-E., Turski, M. L., Nose, Y., Casad, M., Rockman, H. A., and Thiele, D. J. (2010). Cardiac copper deficiency activates a systemic signaling mechanism that communicates with the copper acquisition and storage organs. Cell Metab. 11, 353–363. doi: 10.1016/j.cmet.2010.04.003
Klingberg, F., Hinz, B., and White, E. S. (2013). The myofibroblast matrix: implications for tissue repair and fibrosis. J. Pathol. 229, 298–309. doi: 10.1002/path.4104
Knight, D. A., Weisbrode, S. E., Schmall, L. M., Reed, S. M., Gabel, A. A., Bramlage, L. R., et al. (1990). The effects of copper supplementation on the prevalence of cartilage lesions in foals. Equine Vet. J. 22, 426–432. doi: 10.1111/j.2042-3306.1990.tb04310.x
Kuivaniemi, H., Peltonen, L., Palotie, A., Kaitila, I., and Kivirikko, K. I. (1982). Abnormal copper metabolism and deficient lysyl oxidase activity in a heritable connective tissue disorder. J. Clin. Invest. 69, 730–733. doi: 10.1172/jci110503
Kyritsis, N., Kizil, C., Zocher, S., Kroehne, V., Kaslin, J., Freudenreich, D., et al. (2012). Acute inflammation initiates the regenerative response in the adult zebrafish brain. Science 338, 1353–1356. doi: 10.1126/science.1228773
La Mendola, D., Magri, A., Santoro, A. M., Nicoletti, V. G., and Rizzarelli, E. (2012). Copper(II) interaction with peptide fragments of histidine–proline-rich glycoprotein: speciation, stability and binding details. J. Inorg. Biochem. 111, 59–69. doi: 10.1016/j.jinorgbio.2012.02.027
Lee, C., O’Connell, C. D., Onofrillo, C., Choong, P. F. M., Di Bella, C., and Duchi, S. (2019). Concise review: human articular cartilage repair: sources and detection of cytotoxicity and genotoxicity in photo-crosslinkable hydrogel bioscaffolds. Stem Cells Transl. Med. 9, 302–315. doi: 10.1002/sctm.19-0192
Lee, W. Y., and Wang, B. (2017). Cartilage repair by mesenchymal stem cells: clinical trial update and perspectives. J. Orthop. Translat. 9, 76–88. doi: 10.1016/j.jot.2017.03.005
Leung, V. Y., Chan, D., and Cheung, K. M. (2006). Regeneration of intervertebral disc by mesenchymal stem cells: potentials, limitations, and future direction. Eur. Spine J. 15, (Suppl. 3), S406–S413. doi: 10.1007/s00586-006-0183-z
Li, A., Wei, Y., Hung, C., and Vunjak-Novakovic, G. (2018). Chondrogenic properties of collagen type XI, a component of cartilage extracellular matrix. Biomaterials 173, 47–57. doi: 10.1016/j.biomaterials.2018.05.004
Li, J., Hansen, K. C., Zhang, Y., Dong, C., Dinu, C. Z., Dzieciatkowska, M., et al. (2014). Rejuvenation of chondrogenic potential in a young stem cell microenvironment. Biomaterials 35, 642–653. doi: 10.1016/j.biomaterials.2013.09.099
Li, Y., Dai, G., Shi, L., Lin, Y., Chen, M., Li, G., et al. (2019). The potential roles of tendon stem/progenitor cells in tendon aging. Curr. Stem Cell Res. Ther. 14, 34–42. doi: 10.2174/1574888X13666181017112233
Lin, C. M., and Kosman, D. J. (1990). Copper uptake in wild type and copper metallothionein-deficient Saccharomyces cerevisiae, kinetics and mechanism. J. Biol. Chem. 265, 9194–9200.
Lin, R., Deng, C., Li, X., Liu, Y., Zhang, M., Qin, C., et al. (2019). Copper-incorporated bioactive glass-ceramics inducing anti-inflammatory phenotype and regeneration of cartilage/bone interface. Theranostics 9, 6300–6313. doi: 10.7150/thno.36120
Lin, S., Lee, W. Y. W., Xu, L., Wang, Y., Chen, Y., Ho, K. K. W., et al. (2017). Stepwise preconditioning enhances mesenchymal stem cell-based cartilage regeneration through epigenetic modification. Osteoarthritis Cartilage 25, 1541–1550. doi: 10.1016/j.joca.2017.05.008
Lin, W., Xu, L., Pan, Q., Lin, S., Feng, L., Wang, B., et al. (2019). Lgr5-overexpressing mesenchymal stem cells augment fracture healing through regulation of Wnt/ERK signaling pathways and mitochondrial dynamics. FASEB J. 33, 8565–8577. doi: 10.1096/fj.201900082RR
Linder, M. C. (2016). Ceruloplasmin and other copper binding components of blood plasma and their functions: an update. Metallomics 8, 887–905. doi: 10.1039/c6mt00103c
Liu, C. H., Abrams, N. D., Carrick, D. M., Chander, P., Dwyer, J., Hamlet, M. R. J., et al. (2019). Imaging inflammation and its resolution in health and disease: current status, clinical needs, challenges, and opportunities. FASEB J. 33, 13085–13097. doi: 10.1096/fj.201902024
Liu, X., Zhang, W., Wu, Z., Yang, Y., and Kang, Y. J. (2018). Copper levels affect targeting of hypoxia-inducible factor 1α to the promoters of hypoxia-regulated genes. J. Biol. Chem. 293, 14669–14677. doi: 10.1074/jbc.ra118.001764
Loeser, R. F., Collins, J. A., and Diekman, B. O. (2016). Ageing and the pathogenesis of osteoarthritis. Nat. Rev. Rheumatol. 12, 412–420. doi: 10.1038/nrrheum.2016.65
Long, F. (2019). Less is more: ditching mitochondria saves hypoxic cartilage. Dev. Cell 49, 656–658. doi: 10.1016/j.devcel.2019.05.030
Lopez, B., Gonzalez, A., Hermida, N., Valencia, F., de Teresa, E., and Diez, J. (2010). Role of lysyl oxidase in myocardial fibrosis: from basic science to clinical aspects. Am. J. Physiol. Heart Circ. Physiol. 299, H1–H9. doi: 10.1152/ajpheart.00335.2010
Lotz, M., and Loeser, R. F. (2012). Effects of aging on articular cartilage homeostasis. Bone 51, 241–248. doi: 10.1016/j.bone.2012.03.023
Lucero, H. A., and Kagan, H. M. (2006). Lysyl oxidase: an oxidative enzyme and effector of cell function. Cell. Mol. Life Sci. 63, 2304–2316. doi: 10.1007/s00018-006-6149-9
Lunelli, M., Di Paolo, M. L., Biadene, M., Calderone, V., Battistutta, R., Scarpa, M., et al. (2005). Crystal structure of amine oxidase from bovine serum. J. Mol. Biol. 346, 991–1004. doi: 10.1016/j.jmb.2004.12.038
Luo, J., Zhou, W., Zhou, X., Li, D., Weng, J., Yi, Z., et al. (2009). Regulation of bone formation and remodeling by G-protein-coupled receptor 48. Development 136, 2747–2756. doi: 10.1242/dev.033571
Luria, A., and Chu, C. R. (2014). Articular cartilage changes in maturing athletes: new targets for joint rejuvenation. Sports Health 6, 18–30. doi: 10.1177/1941738113514369
Ma, L., Huang, C., Wang, X. J., Xin, D. E., Wang, L. S., Zou, Q. C., et al. (2017). Lysyl oxidase 3 is a dual-specificity enzyme involved in STAT3 deacetylation and deacetylimination modulation. Mol. Cell 65, 296–309. doi: 10.1016/j.molcel.2016.12.002
Maes, C., Araldi, E., Haigh, K., Khatri, R., Van Looveren, R., Giaccia, A. J., et al. (2012). VEGF-independent cell-autonomous functions of HIF-1α regulating oxygen consumption in fetal cartilage are critical for chondrocyte survival. J. Bone Miner. Res. 27, 596–609. doi: 10.1002/jbmr.1487
Maes, C., Stockmans, I., Moermans, K., Van Looveren, R., Smets, N., Carmeliet, P., et al. (2004). Soluble VEGF isoforms are essential for establishing epiphyseal vascularization and regulating chondrocyte development and survival. J. Clin. Invest. 113, 188–199. doi: 10.1172/JCI19383
Magri, A., Grasso, G., Corti, F., Finetti, F., Greco, V., Santoro, A. M., et al. (2018). Peptides derived from the histidine-proline rich glycoprotein bind copper ions and exhibit anti-angiogenic properties. Dalton Trans. 47, 9492–9503. doi: 10.1039/c8dt01560k
Maki, J. M. (2009). Lysyl oxidases in mammalian development and certain pathological conditions. Histol. Histopathol. 24, 651–660. doi: 10.14670/HH-24.651
Maki, J. M., Rasanen, J., Tikkanen, H., Sormunen, R., Makikallio, K., Kivirikko, K. I., et al. (2002). Inactivation of the lysyl oxidase gene Lox leads to aortic aneurysms, cardiovascular dysfunction, and perinatal death in mice. Circulation 106, 2503–2509. doi: 10.1161/01.cir.0000038109.84500.1e
Maki, J. M., Sormunen, R., Lippo, S., Kaarteenaho-Wiik, R., Soininen, R., and Myllyharju, J. (2005). Lysyl oxidase is essential for normal development and function of the respiratory system and for the integrity of elastic and collagen fibers in various tissues. Am. J. Pathol. 167, 927–936. doi: 10.1016/S0002-9440(10)61183-2
Makris, E. A., MacBarb, R. F., Responte, D. J., Hu, J. C., and Athanasiou, K. A. (2013). A copper sulfate and hydroxylysine treatment regimen for enhancing collagen cross-linking and biomechanical properties in engineered neocartilage. FASEB J. 27, 2421–2430. doi: 10.1096/fj.12-224030
Makris, E. A., Responte, D. J., Paschos, N. K., Hu, J. C., and Athanasiou, K. A. (2014). Developing functional musculoskeletal tissues through hypoxia and lysyl oxidase-induced collagen cross-linking. Proc. Natl. Acad. Sci. U.S.A. 111, E4832–E4841. doi: 10.1073/pnas.1414271111
Martin, F., Linden, T., Katschinski, D. M., Oehme, F., Flamme, I., Mukhopadhyay, C. K., et al. (2005). Copper-dependent activation of hypoxia-inducible factor (HIF)-1: implications for ceruloplasmin regulation. Blood 105, 4613–4619. doi: 10.1182/blood-2004-10-3980
Martinez-Gonzalez, J., Varona, S., Canes, L., Galan, M., Briones, A. M., Cachofeiro, V., et al. (2019). Emerging roles of lysyl oxidases in the cardiovascular system: new concepts and therapeutic challenges. Biomolecules 9:610. doi: 10.3390/biom9100610
Marturano, J. E., Arena, J. D., Schiller, Z. A., Georgakoudi, I., and Kuo, C. K. (2013). Characterization of mechanical and biochemical properties of developing embryonic tendon. Proc. Natl. Acad. Sci. U.S.A. 110, 6370–6375. doi: 10.1073/pnas.1300135110
Mendler, M., Eich-Bender, S. G., Vaughan, L., Winterhalter, K. H., and Bruckner, P. (1989). Cartilage contains mixed fibrils of collagen types II, IX, and XI. J. Cell Biol. 108, 191–197. doi: 10.1083/jcb.108.1.191
Messier, S. P., Gutekunst, D. J., Davis, C., and DeVita, P. (2005). Weight loss reduces knee-joint loads in overweight and obese older adults with knee osteoarthritis. Arthritis Rheum. 52, 2026–2032. doi: 10.1002/art.21139
Miao, Q., Hill, M. C., Chen, F., Mo, Q., Ku, A. T., Ramos, C., et al. (2019). SOX11 and SOX4 drive the reactivation of an embryonic gene program during murine wound repair. Nat. Commun. 10:4042. doi: 10.1038/s41467-019-11880-9
Miller, K. A., Vicentini, F. A., Hirota, S. A., Sharkey, K. A., and Wieser, M. E. (2019). Antibiotic treatment affects the expression levels of copper transporters and the isotopic composition of copper in the colon of mice. Proc. Natl. Acad. Sci. U.S.A. 116, 5955–5960. doi: 10.1073/pnas.1814047116
Minet, E., Mottet, D., Michel, G., Roland, I., Raes, M., Remacle, J., et al. (1999). Hypoxia-induced activation of HIF-1: role of HIF-1α-Hsp90 interaction. FEBS Lett. 460, 251–256. doi: 10.1016/s0014-5793(99)01359-9
Mobasheri, A., and Batt, M. (2016). An update on the pathophysiology of osteoarthritis. Ann. Phys. Rehabil. Med. 59, 333–339. doi: 10.1016/j.rehab.2016.07.004
Nave, A. H., Mizikova, I., Niess, G., Steenbock, H., Reichenberger, F., Talavera, M. L., et al. (2014). Lysyl oxidases play a causal role in vascular remodeling in clinical and experimental pulmonary arterial hypertension. Arterioscler. Thromb. Vasc. Biol. 34, 1446–1458. doi: 10.1161/ATVBAHA.114.303534
Nelson, T. J., Behfar, A., and Terzic, A. (2008). Strategies for therapeutic repair: the “R(3)” regenerative medicine paradigm. Clin. Transl. Sci. 1, 168–171. doi: 10.1111/j.1752-8062.2008.00039.x
Ohmura, H., Yasukawa, H., Minami, T., Sugi, Y., Oba, T., Nagata, T., et al. (2012). Cardiomyocyte-specific transgenic expression of lysyl oxidase-like protein-1 induces cardiac hypertrophy in mice. Hypertens. Res. 35, 1063–1068. doi: 10.1038/hr.2012.92
Ohrvik, H., and Thiele, D. J. (2014). How copper traverses cellular membranes through the mammalian copper transporter 1, Ctr1. Ann. N. Y. Acad. Sci. 1314, 32–41. doi: 10.1111/nyas.12371
Okado-Matsumoto, A., and Fridovich, I. (2001). Subcellular distribution of superoxide dismutases (SOD) in rat liver: Cu, Zn-SOD in mitochondria. J. Biol. Chem. 276, 38388–38393. doi: 10.1074/jbc.M105395200
Onofrillo, C., Duchi, S., O’Connell, C. D., Blanchard, R., O’Connor, A. J., Scott, M., et al. (2018). Biofabrication of human articular cartilage: a path towards the development of a clinical treatment. Biofabrication 10:045006. doi: 10.1088/1758-5090/aad8d9
Pasqualicchio, M., Gasperini, R., Velo, G. P., and Davies, M. E. (1996). Effects of copper and zinc on proteoglycan metabolism in articular cartilage. Mediators Inflamm. 5, 95–99. doi: 10.1155/S0962935196000154
Patil, P., Dong, Q., Wang, D., Chang, J., Wiley, C., Demaria, M., et al. (2019). Systemic clearance of -positive senescent cells mitigates age-associated intervertebral disc degeneration. Aging Cell 18:e12927. doi: 10.1111/acel.12927
Percival, S. S. (1998). Copper and immunity. Am. J. Clin. Nutr. 67(5 Suppl.), 1064S–1068S. doi: 10.1093/ajcn/67.5.1064S
Pfander, D., Swoboda, B., and Cramer, T. (2006). The role of HIF-1α in maintaining cartilage homeostasis and during the pathogenesis of osteoarthritis. Arthritis Res. Ther. 8:104. doi: 10.1186/ar1894
Pokharna, H. K., Monnier, V., Boja, B., and Moskowitz, R. W. (1995). Lysyl oxidase and Maillard reaction-mediated crosslinks in aging and osteoarthritic rabbit cartilage. J. Orthop. Res. 13, 13–21. doi: 10.1002/jor.1100130105
Puig, S., and Thiele, D. J. (2002). Molecular mechanisms of copper uptake and distribution. Curr. Opin. Chem. Biol. 6, 171–180. doi: 10.1016/s1367-5931(02)00298-3
Pushie, M. J., Ross, A. R., and Vogel, H. J. (2007). Mass spectrometric determination of the coordination geometry of potential copper(II) surrogates for the mammalian prion protein octarepeat region. Anal. Chem. 79, 5659–5667. doi: 10.1021/ac070312l
Qu, X., He, Z., Qiao, H., Zhai, Z., Mao, Z., Yu, Z., et al. (2018). Serum copper levels are associated with bone mineral density and total fracture. J. Orthop. Translat. 14, 34–44. doi: 10.1016/j.jot.2018.05.001
Rae, T. D., Schmidt, P. J., Pufahl, R. A., Culotta, V. C., and O’Halloran, T. V. (1999). Undetectable intracellular free copper: the requirement of a copper chaperone for superoxide dismutase. Science 284, 805–808. doi: 10.1126/science.284.5415.805
Ranganathan, P. N., Lu, Y., Jiang, L., Kim, C., and Collins, J. F. (2011). Serum ceruloplasmin protein expression and activity increases in iron-deficient rats and is further enhanced by higher dietary copper intake. Blood 118, 3146–3153. doi: 10.1182/blood-2011-05-352112
Ransom, R. C., Carter, A. C., Salhotra, A., Leavitt, T., Marecic, O., Murphy, M. P., et al. (2018). Mechanoresponsive stem cells acquire neural crest fate in jaw regeneration. Nature 563, 514–521. doi: 10.1038/s41586-018-0650-9
Reynaud, C., Baas, D., Gleyzal, C., Le Guellec, D., and Sommer, P. (2008). Morpholino knockdown of lysyl oxidase impairs zebrafish development, and reflects some aspects of copper metabolism disorders. Matrix Biol. 27, 547–560. doi: 10.1016/j.matbio.2008.03.002
Rimar, D., Rosner, I., Nov, Y., Slobodin, G., Rozenbaum, M., Halasz, K., et al. (2014). Brief report: lysyl oxidase is a potential biomarker of fibrosis in systemic sclerosis. Arthritis Rheumatol. 66, 726–730. doi: 10.1002/art.38277
Rivard, A., Berthou-Soulie, L., Principe, N., Kearney, M., Curry, C., Branellec, D., et al. (2000). Age-dependent defect in vascular endothelial growth factor expression is associated with reduced hypoxia-inducible factor 1 activity. J. Biol. Chem. 275, 29643–29647. doi: 10.1074/jbc.M001029200
Robins, J. C., Akeno, N., Mukherjee, A., Dalal, R. R., Aronow, B. J., Koopman, P., et al. (2005). Hypoxia induces chondrocyte-specific gene expression in mesenchymal cells in association with transcriptional activation of Sox9. Bone 37, 313–322. doi: 10.1016/j.bone.2005.04.040
Robinson, P. S., Huang, T. F., Kazam, E., Iozzo, R. V., Birk, D. E., and Soslowsky, L. J. (2005). Influence of decorin and biglycan on mechanical properties of multiple tendons in knockout mice. J. Biomech. Eng. 127, 181–185. doi: 10.1115/1.1835363
Roman-Blas, J. A., and Jimenez, S. A. (2008). Targeting NF-κB: a promising molecular therapy in inflammatory arthritis. Int. Rev. Immunol. 27, 351–374. doi: 10.1080/08830180802295740
Rucker, R. B., Kosonen, T., Clegg, M. S., Mitchell, A. E., Rucker, B. R., Uriu-Hare, J. Y., et al. (1998). Copper, lysyl oxidase, and extracellular matrix protein cross-linking. Am. J. Clin. Nutr. 67(5 Suppl.), 996S–1002S. doi: 10.1093/ajcn/67.5.996S
Sakai, D., Nakamura, Y., Nakai, T., Mishima, T., Kato, S., Grad, S., et al. (2012). Exhaustion of nucleus pulposus progenitor cells with ageing and degeneration of the intervertebral disc. Nat. Commun. 3:1264. doi: 10.1038/ncomms2226
Sanada, H., Shikata, J., Hamamoto, H., Ueba, Y., Yamamuro, T., and Takeda, T. (1978). Changes in collagen cross-linking and lysyl oxidase by estrogen. Biochim. Biophys. Acta 541, 408–413. doi: 10.1016/0304-4165(78)90199-x
Sarkar, T. J., Quarta, M., Mukherjee, S., Colville, A., Paine, P., Doan, L., et al. (2020). Transient non-integrative expression of nuclear reprogramming factors promotes multifaceted amelioration of aging in human cells. Nat. Commun. 11:1545. doi: 10.1038/s41467-020-15174-3
Schietke, R., Warnecke, C., Wacker, I., Schodel, J., Mole, D. R., Campean, V., et al. (2010). The lysyl oxidases LOX and LOXL2 are necessary and sufficient to repress E-cadherin in hypoxia: insights into cellular transformation processes mediated by HIF-1. J. Biol. Chem. 285, 6658–6669. doi: 10.1074/jbc.M109.042424
Schmelzer, C. E. H., Heinz, A., Troilo, H., Lockhart-Cairns, M. P., Jowitt, T. A., Marchand, M. F., et al. (2019). Lysyl oxidase-like 2 (LOXL2)-mediated cross-linking of tropoelastin. FASEB J. 33, 5468–5481. doi: 10.1096/fj.201801860RR
Scudder, P. R., Al-Timimi, D., McMurray, W., White, A. G., Zoob, B. C., and Dormandy, T. L. (1978). Serum copper and related variables in rheumatoid arthritis. Ann. Rheum. Dis. 37, 67–70. doi: 10.1136/ard.37.1.67
Shi, Q., Qian, Z., Liu, D., Sun, J., Xu, J., and Guo, X. (2017). Maintaining the phenotype stability of chondrocytes derived from MSCs by C-type natriuretic peptide. Front. Physiol. 8:143. doi: 10.3389/fphys.2017.00143
Shi, Y., Hu, X., Zhang, X., Cheng, J., Duan, X., Fu, X., et al. (2019). Superoxide dismutase 3 facilitates the chondrogenesis of bone marrow-derived mesenchymal stem cells. Biochem. Biophys. Res. Commun. 509, 983–987. doi: 10.1016/j.bbrc.2019.01.042
Simon, M. C., and Keith, B. (2008). The role of oxygen availability in embryonic development and stem cell function. Nat. Rev. Mol. Cell Biol. 9, 285–296. doi: 10.1038/nrm2354
Smith-Mungo, L. I., and Kagan, H. M. (1998). Lysyl oxidase: properties, regulation and multiple functions in biology. Matrix Biol. 16, 387–398. doi: 10.1016/s0945-053x(98)90012-9
Solomon, E. I., Heppner, D. E., Johnston, E. M., Ginsbach, J. W., Cirera, J., Qayyum, M., et al. (2014). Copper active sites in biology. Chem. Rev. 114, 3659–3853. doi: 10.1021/cr400327t
Stegen, S., Laperre, K., Eelen, G., Rinaldi, G., Fraisl, P., Torrekens, S., et al. (2019). HIF-1α metabolically controls collagen synthesis and modification in chondrocytes. Nature 565, 511–515. doi: 10.1038/s41586-019-0874-3
Stroka, D. M., Burkhardt, T., Desbaillets, I., Wenger, R. H., Neil, D. A., Bauer, C., et al. (2001). HIF-1 is expressed in normoxic tissue and displays an organ-specific regulation under systemic hypoxia. FASEB J. 15, 2445–2453. doi: 10.1096/fj.01-0125com
Sun, Y., Li, W., Lu, Z., Chen, R., Ling, J., Ran, Q., et al. (2011). Rescuing replication and osteogenesis of aged mesenchymal stem cells by exposure to a young extracellular matrix. FASEB J. 25, 1474–1485. doi: 10.1096/fj.10-161497
Tao, C., Wang, Y., Zhao, Y., Pan, J., Fan, Y., Liang, X., et al. (2019). Adipocyte-specific disruption of ATPase copper transporting α in mice accelerates lipoatrophy. Diabetologia 62, 2340–2353. doi: 10.1007/s00125-019-4966-2
Tapiero, H., Townsend, D. M., and Tew, K. D. (2003). Trace elements in human physiology and pathology. Biomed. Pharmacother. 57, 386–398. doi: 10.1016/s0753-3322(03)00012-x
Tashkandi, M., Ali, F., Alsaqer, S., Alhousami, T., Cano, A., Martin, A., et al. (2019). Lysyl oxidase-like 2 protects against progressive and aging related knee joint osteoarthritis in mice. Int. J. Mol. Sci. 20:4798. doi: 10.3390/ijms20194798
Tatman, P. D., Gerull, W., Sweeney-Easter, S., Davis, J. I., Gee, A. O., and Kim, D. H. (2015). Multiscale biofabrication of articular cartilage: bioinspired and biomimetic approaches. Tissue Eng. Part B Rev. 21, 543–559. doi: 10.1089/ten.TEB.2015.0142
Teo, A. Q. A., Wong, K. L., Shen, L., Lim, J. Y., Toh, W. S., Lee, E. H., et al. (2019). Equivalent 10-year outcomes after implantation of autologous bone marrow-derived mesenchymal stem cells versus autologous chondrocyte implantation for chondral defects of the knee. Am. J. Sports Med. 47, 2881–2887. doi: 10.1177/0363546519867933
Thalhamer, T., McGrath, M. A., and Harnett, M. M. (2008). MAPKs and their relevance to arthritis and inflammation. Rheumatology 47, 409–414. doi: 10.1093/rheumatology/kem297
Thomassin, L., Werneck, C. C., Broekelmann, T. J., Gleyzal, C., Hornstra, I. K., Mecham, R. P., et al. (2005). The Pro-regions of lysyl oxidase and lysyl oxidase-like 1 are required for deposition onto elastic fibers. J. Biol. Chem. 280, 42848–42855. doi: 10.1074/jbc.m506832200
Turski, M. L., Brady, D. C., Kim, H. J., Kim, B. E., Nose, Y., Counter, C. M., et al. (2012). A novel role for copper in Ras/mitogen-activated protein kinase signaling. Mol. Cell. Biol. 32, 1284–1295. doi: 10.1128/MCB.05722-11
Turski, M. L., and Thiele, D. J. (2009). New roles for copper metabolism in cell proliferation, signaling, and disease. J. Biol. Chem. 284, 717–721. doi: 10.1074/jbc.R800055200
Urso, E., and Maffia, M. (2015). Behind the link between copper and angiogenesis: established mechanisms and an overview on the role of vascular copper transport systems. J. Vasc. Res. 52, 172–196. doi: 10.1159/000438485
van Boxtel, A. L., Gansner, J. M., Hakvoort, H. W., Snell, H., Legler, J., and Gitlin, J. D. (2011). Lysyl oxidase-like 3b is critical for cartilage maturation during Zebrafish craniofacial development. Matrix Biol. 30, 178–187. doi: 10.1016/j.matbio.2010.12.002
van Vlimmeren, M. A., Driessen-Mol, A., van den Broek, M., Bouten, C. V., and Baaijens, F. P. (2010). Controlling matrix formation and cross-linking by hypoxia in cardiovascular tissue engineering. J. Appl. Physiol. 109, 1483–1491. doi: 10.1152/japplphysiol.00571.2010
Varela-Eirin, M., Loureiro, J., Fonseca, E., Corrochano, S., Caeiro, J. R., Collado, M., et al. (2018). Cartilage regeneration and ageing: Targeting cellular plasticity in osteoarthritis. Ageing Res. Rev. 42, 56–71. doi: 10.1016/j.arr.2017.12.006
Vortkamp, A., Pathi, S., Peretti, G. M., Caruso, E. M., Zaleske, D. J., and Tabin, C. J. (1998). Recapitulation of signals regulating embryonic bone formation during postnatal growth and in fracture repair. Mech. Dev. 71, 65–76. doi: 10.1016/s0925-4773(97)00203-7
Wang, A. M., Cao, P., Yee, A., Chan, D., and Wu, E. X. (2015). Detection of extracellular matrix degradation in intervertebral disc degeneration by diffusion magnetic resonance spectroscopy. Magn. Reson. Med. 73, 1703–1712. doi: 10.1002/mrm.25289
Wang, M. J., Chen, J., Chen, F., Liu, Q., Sun, Y., Yan, C., et al. (2019). Rejuvenating strategies of tissue-specific stem cells for healthy aging. Aging Dis. 10, 871–882. doi: 10.14336/AD.2018.1119
Wang, S. X., Mure, M., Medzihradszky, K. F., Burlingame, A. L., Brown, D. E., Dooley, D. M., et al. (1996). A crosslinked cofactor in lysyl oxidase: redox function for amino acid side chains. Science 273, 1078–1084. doi: 10.1126/science.273.5278.1078
Wang, T., Xiang, P., Ha, J. H., Wang, X., Doguer, C., Flores, S. R. L., et al. (2018). Copper supplementation reverses dietary iron overload-induced pathologies in mice. J. Nutr. Biochem. 59, 56–63. doi: 10.1016/j.jnutbio.2018.05.006
Weiner, S., and Traub, W. (1992). Bone structure: from angstroms to microns. FASEB J. 6, 879–885. doi: 10.1096/fasebj.6.3.1740237
Williamson, A. K., Chen, A. C., Masuda, K., Thonar, E. J., and Sah, R. L. (2003). Tensile mechanical properties of bovine articular cartilage: variations with growth and relationships to collagen network components. J. Orthop. Res. 21, 872–880. doi: 10.1016/S0736-0266(03)00030-5
Xu, L., Shunmei, E., Lin, S., Hou, Y., Lin, W., He, W., et al. (2019). Sox11-modified mesenchymal stem cells accelerate cartilage defect repair in SD rats. Cell Tissue Res. 376, 247–255. doi: 10.1007/s00441-018-02979-4
Yao, H., Xu, J. K., Zheng, N. Y., Wang, J. L., Mok, S. W., Lee, Y. W., et al. (2019). Intra-articular injection of magnesium chloride attenuates osteoarthritis progression in rats. Osteoarthritis Cartilage 27, 1811–1821. doi: 10.1016/j.joca.2019.08.007
Yazar, M., Sarban, S., Kocyigit, A., and Isikan, U. E. (2005). Synovial fluid and plasma selenium, copper, zinc, and iron concentrations in patients with rheumatoid arthritis and osteoarthritis. Biol. Trace Elem. Res. 106, 123–132. doi: 10.1385/bter:106:2:123
Yuan, X., Wang, J., Zhu, X., Zhang, Z., Ai, Y., Sun, G., et al. (2011). Effect of copper on levels of collagen and alkaline phosphatase activity from chondrocytes in newborn piglets in vitro. Biol. Trace Elem. Res. 144, 597–605. doi: 10.1007/s12011-011-9151-5
Yuan, X. L., Meng, H. Y., Wang, Y. C., Peng, J., Guo, Q. Y., Wang, A. Y., et al. (2014). Bone-cartilage interface crosstalk in osteoarthritis: potential pathways and future therapeutic strategies. Osteoarthritis Cartilage 22, 1077–1089. doi: 10.1016/j.joca.2014.05.023
Yudoh, K., Nakamura, H., Masuko-Hongo, K., Kato, T., and Nishioka, K. (2005). Catabolic stress induces expression of hypoxia-inducible factor (HIF)-1 α in articular chondrocytes: involvement of HIF-1 α in the pathogenesis of osteoarthritis. Arthritis Res. Ther. 7, R904–R914. doi: 10.1186/ar1765
Zhang, C., Yang, F., Cornelia, R., Tang, W., Swisher, S., and Kim, H. (2011). Hypoxia-inducible factor-1 is a positive regulator of Sox9 activity in femoral head osteonecrosis. Bone 48, 507–513. doi: 10.1016/j.bone.2010.10.006
Zhang, S., Hu, P., Liu, T., Li, Z., Huang, Y., Liao, J., et al. (2019). Kartogenin hydrolysis product 4-aminobiphenyl distributes to cartilage and mediates cartilage regeneration. Theranostics 9, 7108–7121. doi: 10.7150/thno.38182
Zhang, X., Cai, D., Zhou, F., Yu, J., Wu, X., Yu, D., et al. (2020). Targeting downstream subcellular YAP activity as a function of matrix stiffness with Verteporfin-encapsulated chitosan microsphere attenuates osteoarthritis. Biomaterials 232:119724. doi: 10.1016/j.biomaterials.2019.119724
Zhang, X., Wang, Q., Wu, J., Wang, J., Shi, Y., and Liu, M. (2018). Crystal structure of human lysyl oxidase-like 2 (hLOXL2) in a precursor state. Proc. Natl. Acad. Sci. U.S.A. 115, 3828–3833. doi: 10.1073/pnas.1720859115
Zhang, Y., Morgan, B. J., Smith, R., Fellows, C. R., Thornton, C., Snow, M., et al. (2017). Platelet-rich plasma induces post-natal maturation of immature articular cartilage and correlates with LOXL1 activation. Sci. Rep. 7:3699. doi: 10.1038/s41598-017-02297-9
Zhang, Y., Xiong, C., Kudelko, M., Li, Y., Wang, C., Wong, Y. L., et al. (2018). Early onset of disc degeneration in SM/J mice is associated with changes in ion transport systems and fibrotic events. Matrix Biol. 70, 123–139. doi: 10.1016/j.matbio.2018.03.024
Zhang, Z., Leong, D. J., Xu, L., He, Z., Wang, A., Navati, M., et al. (2016). Curcumin slows osteoarthritis progression and relieves osteoarthritis-associated pain symptoms in a post-traumatic osteoarthritis mouse model. Arthritis Res. Ther. 18:128. doi: 10.1186/s13075-016-1025-y
Zhou, B., Zhang, H., Su, X., Luo, Y., Li, X., Yu, C., et al. (2019). Therapeutic effects of a novel BAFF blocker on arthritis. Signal Transduct. Target. Ther. 4:19. doi: 10.1038/s41392-019-0051-z
Keywords: lysyl oxidase, cartilage, hypoxia-inducible factor, copper, transcription activity, regeneration, rejuvenation
Citation: Lin W, Xu L and Li G (2020) Molecular Insights Into Lysyl Oxidases in Cartilage Regeneration and Rejuvenation. Front. Bioeng. Biotechnol. 8:359. doi: 10.3389/fbioe.2020.00359
Received: 17 February 2020; Accepted: 31 March 2020;
Published: 30 April 2020.
Edited by:
Roberto Narcisi, Erasmus University Rotterdam, NetherlandsReviewed by:
Guus van den Akker, Maastricht University, NetherlandsRyan Michael Porter, University of Arkansas for Medical Sciences, United States
Copyright © 2020 Lin, Xu and Li. This is an open-access article distributed under the terms of the Creative Commons Attribution License (CC BY). The use, distribution or reproduction in other forums is permitted, provided the original author(s) and the copyright owner(s) are credited and that the original publication in this journal is cited, in accordance with accepted academic practice. No use, distribution or reproduction is permitted which does not comply with these terms.
*Correspondence: Gang Li, Z2FuZ2xpQGN1aGsuZWR1Lmhr; Weiping Lin, d2VpcGluZ2xpbkBsaW5rLmN1aGsuZWR1Lmhr
†These authors have contributed equally to this work