Corrigendum: Middle ear anatomy and implant sizes: correlates and the need for uniform implant dimensions
- 1Department of Otolaryngology, Hannover Medical School, Hannover, Germany
- 2Department of Neuroradiology, Hannover Medical School, Hannover, Germany
- 3MED-EL Surgical Solutions, Rangendingen, Germany
- 4MED-EL Research Center, Hannover, Germany
Introduction: Conductive hearing loss describes an insufficient sound transfer of the middle ear, often caused by defects or absence of the ossicles. Depending on the specific middle ear dimensions and the kind of defect, surgeons can choose from a variety of passive implants to reconstruct the middle ear and hence restore sound transmission. However, the latter is only achieved if the optimal implant size is available and selected for each individual patient.
Methods: Anatomical dimensions relevant for middle ear reconstruction were assessed within high-resolution clinical imaging data of 50 patients (100 ears). The ranges of these dimensions were then compared to implant types and sizes available from different manufacturers.
Results: In general, total and partial prostheses seem to cover the whole range of anatomical variations. A lack of stapesplasty implants was found for particularly small anatomies. Various implant lengths of all types far exceed dimensions necessary for successful restoration of sound transmission. In some cases, implant lengths are not clearly specified by the manufacturer. Tympanic membrane and stapes axis were not in line for any of the investigated middle ears.
Conclusion: Clear specifications of implant lengths are crucial to allow for successful hearing restoration, and clinics often need to have more than one implant type to cover the entire range of anatomical variations they may encounter. There appears to be an unmet clinical need for smaller stapesplasty implants. Devices which allow for an angular adjustment between distal and proximal end appear to mimic the orientation of the ossicles more naturally.
1 Introduction
The function of the middle ear is the transmission of vibrations from the tympanic membrane to the stapes footplate. In doing so, the composition of tympanic membrane, ossicles and oval window compensate for impedance differences between the air-filled outer ear canal to the fluid-filled cochlea (Pickles, 2013). In patients with conductive hearing loss, sound transmission from outer to inner ear is compromised, requiring ossiculoplasty (Kartush, 1994; Mudhol et al., 2013; Young and Ng, 2023) to surgically reconstruct the functionally impaired middle ear structures. This can be done using either autologous grafts or alloplastic passive middle ear implants (PMEIs).
Various studies have been conducted to determine requirements for PMEIs and successful ossiculoplastic surgery, the goal of which is often stated as a postoperative air bone gap of less than 20dB (Yung and Vowler, 2006; Amith and Rs, 2017). While autologous grafts were found to be advantageous for slight defects (Amith and Rs, 2017), more pronounced ossicular chain destructions are typically treated with artificial prostheses made of metal and/or plastics (Beutner and Hüttenbrink, 2009). Obvious requirements for these manufactured PMEIs include factors such as biocompatibility and bio-stability, missing bone apposition, low weight for optimal sound conduction and the avoidance of radiological artifacts (Beutner and Hüttenbrink, 2009). Different materials such as hydroxyapatite, polytetrafluoroethylene, gold and titanium are commercially employed for PMEI manufacturing, all of which fulfill the requirements stated above and were shown to yield comparable sound transmission results (Morris et al., 2004; Ringeval et al., 2004; Mojallal et al., 2009).
One factor responsible for the variability in postoperative ossiculoplasty outcomes (Austin, 1969; Kartush, 1994; Yung and Vowler, 2006; Kamrava and Roehm, 2017; Neudert, 2020) is the condition of the middle ear: if the stapes superstructure is still present, partial prostheses can be implanted to only bridge the gap between tympanic membrane and stapes head. These devices were shown to yield favorable results in comparison to total prostheses which transfer incoming sound from the tympanic membrane directly to the stapes footplate (Yu et al., 2013; Kortebein et al., 2023). Furthermore, the absence of the malleus was shown to negatively affect implantation outcomes, which is likely owed to insufficient sound transfer from outer to middle ear (Yung and Vowler, 2006).
Another factor influencing the postoperative outcomes is the placement of the implant during surgery. If the malleus is present, the distal end of the total or partial prosthesis should be placed onto the malleus handle and not directly onto the tympanic membrane (Bance et al., 2004). Furthermore, cartilage slices are typically placed between malleus/tympanic membrane and prosthesis for mechanical stability, but the thickness of these slices should not exceed 0.5 mm in order to preserve optimal sound conduction properties (Mürbe et al., 2002; Morris et al., 2004).
Finally, the selection of the functional length of the implant was shown to play a significant role in ossiculoplasty outcomes (Morris et al., 2004; Merchant and Rosowski, 2013). The middle ear anatomy varies substantially between patients (aWengen et al., 1995; Todd and Creighton, 2013; Kamrava and Roehm, 2017), which is why PMEIs are typically available in different lengths or are length adjustable. The selection of the ideal length for an individual patient is crucial: a stapesplasty prosthesis should not be inserted more than 0.5 mm into the stapedotomy to avoid contact to the utricular macula (Mukherjee et al., 2011). In case of total or partial prostheses, the implant must be long enough to establish sufficient contact to the ossicles (Lord et al., 2000), but an implant which is too long creates a static force onto the stapes footplate, which causes the annular ligament (AL) surrounding the footplate to be elongated (Figure 1). It was shown that this elongation causes the AL to become stiffer (Gan et al., 2011; Lauxmann et al., 2014), which reduces the vibrational amplitudes of the stapes footplate during sound conduction (Koike et al., 2005) and hence negatively affects sound transmission especially in the low frequencies (Bance et al., 2004; Morris et al., 2004; Neudert et al., 2016; Schär et al., 2023). The implant length for an individual patient must hence be chosen such that it is long enough to bridge the gap between the affected middle ear structures, but not too long to avoid prestress of the AL surrounding the stapes footplate.
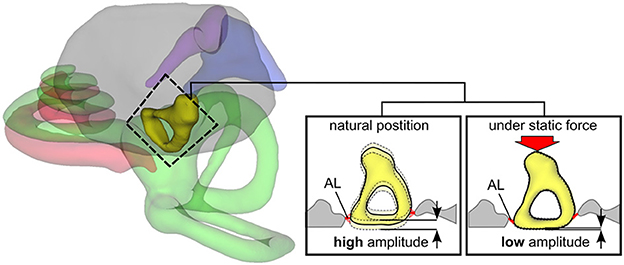
Figure 1. Static force onto the stapes causes pre-tension of the annular ligament (AL), resulting in substantially reduced vibrational amplitudes of the stapes during sound transmission.
Under consideration of these spatial constraints, the anatomical variations of the middle ear were assessed in clinical imaging data within the present study. Dimensions relevant for ossiculoplasty were compared to the ranges of PMEIs offered by different manufacturers. The goal was to assess if commercially available PMEI sizes (total, partial and stapes prostheses) sufficiently cover the anatomical variability of the middle ear.
2 Materials and methods
2.1 Imaging data
High-resolution cone-beam computed tomography (CBCT) imaging datasets of 100 ears (50 patients, 23 female, 27 male, 9–89 years old) were investigated within the present analysis. Informed consent was obtained from all patients by admission allowing the use of their anonymized data for research purposes. Each imaging dataset was obtained between 2017 and 2020 with a 3D Accuitomo 170 (Morita Group, Osaka, Japan) and had an isotropic voxel size of 250 μm. After initial investigation, 6 ears (3 patients) had to be excluded due to insufficient image quality, possibly owed to slight movements during CBCT acquisition, and an additional 5 ears (from four patients) were excluded because they were already implanted with a PMEI. Within all other imaging data, the middle ear anatomy was found to be normally developed and clearly distinguishable by an experienced ENT surgeon.
2.2 Fiducial placement
Assessment of the middle ear anatomy was conducted using 3D Slicer [version 4.11, www.slicer.org, (Fedorov et al., 2012)] by placing a total of 10 fiducials per ear along various structures of interest. However, only 7 of these 10 fiducials are relevant for the present study: 4 of these fiducials were placed along the edge of the TM, 1 fiducial (F5) was placed onto the central malleus handle and 2 further points were placed along the stapes axis (F9: stapes head; F10: center of stapes footplate). Detailed depictions of these fiducials placed within the corresponding imaging data are given in the Supplementary Figure S1. All fiducials were first placed by an ENT resident and subsequently checked by an experienced ENT specialist. In addition, fiducials were placed again in 20 of the investigated ears by both the ENT specialist and an experienced neuroradiologist to investigate intra and inter observer variability respectively.
2.3 Computation of anatomical dimensions
The fiducials of the 89 ears included in the present investigation were then imported into Matlab (version R2018a, Mathworks, USA) to compute relevant dimensions for PMEIs. An overview of these dimensions and the fiducials used to compute the corresponding values is given in Figure 2. The TM diameters in horizontal (TMh) and vertical (TMv) direction were computed as the metric distances F1 to F2 and F3 to F4 respectively (Figure 2A). Furthermore, a plane was fitted to the fiducials F1-F4 by minimizing the perpendicular distances of these fiducials to the plane (least squares, see Figure 2B). This TM plane hence defines the spatial orientation of the TM in each ear. The normal vector of this plane in combination with the stapes axis, which was defined as the vector from stapes head (F9) to stapes footplate (F10), were then used to compute the angle α between TM and stapes (Figure 2B). A value of α = 0° hence corresponds to an exact alignment of stapes axis and TM normal direction. In addition, the metric distances from central malleus handle to stapes footplate (M-SFP) and stapes head (M-SH) were computed as the distances from F5 to F10 and F5 to F9 respectively (Figure 2C). Finally, the length of the stapes S (Figure 2D) was computed as the metric distance from stapes head (F9) to stapes footplate (F10).
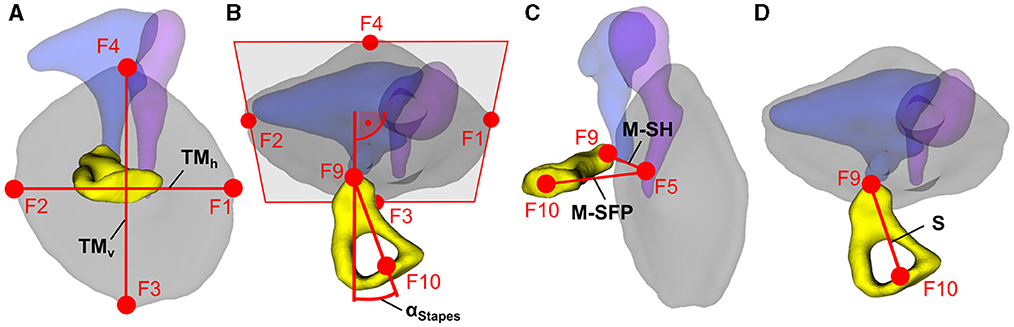
Figure 2. Fiducial coordinates were used to compute (A) the TM diameters in horizontal (TMh) and vertical direction (TMv), (B) the angle α between stapes and TM, (C) the distances between central malleus handle and stapes footplate (M-SFP) and stapes head (M-SH) respectively and (D) the length of the stapes (S).
It must be noted here that the TM could only be clearly distinguished on 41 of the 89 ears. The complete set of anatomical dimensions described above could hence only be computed for these 41 ears. For the other 48 cases, only those dimensions could be computed which were independent on F1–F4, i.e., M-SFP, M-SH and S.
2.4 Passive middle ear implants
Information on available total, partial and stapesplasty prostheses and the respective dimensions was acquired using the product catalogs of different implant manufacturers:
• Audio Technologies S.r.l. (Piacenza, Italy), www.audiotechnologies.it (version CAT400 rev.00 – Oct. 2015).
• Grace Medical, Inc. (Memphis, USA), www.gracemedical.com (version LIT0041 CID6009 Rev. 2018-08).
• Heinz Kurz GmbH (Dusslingen, Germany), www.kurzmed.com (version 03/2018-M9600320).
• MED-EL Medical Electronics (Innsbruck, Austria), www.medel.com (version M00130 r4.0).
• Medtronic ENT (Jacksonville, USA), www.medtronicent.com (version UC201402426k EN).
• Olympus Corporation (Tokyo, Japan), www.olympus-global.com (version E0492509EN · 800 · 11/17 · PR · HB).
• Spiggle & Theis (Overath, Germany), www.spiggle-theis.com (stapesplasty: version F_Nitinol_E_03; total/partial: version F_MEI_E_01).
Implants were grouped by manufacturer, implant type (total, partial, stapesplasty) and dependent on whether the implant length can be adjusted.
In order to allow for direct comparisons to the assessed anatomical dimensions, the minimal and maximal functional length each prosthesis was noted. In case of some partial and stapesplasty prostheses, the functional length was not stated by the manufacturer (e.g., in case of the stapesplasty prostheses by Audio Technologies). In these cases, a 1 mm offset was subtracted from the total implant length to account for the height of the stapes head attachment of partial prostheses or the loop diameter of stapesplasty prostheses respectively.
2.5 Statistical analyses
All statistical evaluations were conducted in Python (version 3.7, Python Software Foundation, USA) using the Scipy library (version 1.2.1). Normal distribution testing was conducted using the Shiparo-Wilk test with 5% significance level. Pearson's correlation tests were performed to investigate interrelations between specific parameters. Significances were tested with the two-sided Mann-Whitney-Wilcoxon test with Bonferroni correction with a 5% significance level.
3 Results
The derived distributions of the different anatomical dimensions evaluated within the present study are depicted in Figure 3. The median angle between stapes axis and TM normal direction α was found to be 15.4°, and α did not reach 0° in any of the investigated cases. Other median values were TMh = 8.84 mm and TMv = 9.59 mm for the horizontal and vertical TM diameters respectively, M-SFP = 4.32 mm and M-SH = 2.19 mm for the distances from central malleus handle to stapes footplate and stapes head respectively and S = 2.97 mm for the length of the stapes. The smallest stapes was found to only be 1.90 mm long, but the respective middle ear was confirmed to be normally developed after re-investigating the images. Only the TM diameters (TMh: p = 0.261; TMv: p = 0.651) and stapes length S (p = 0.171) were found to be normally distributed. Pearson correlation testing revealed significant positive correlations between TMh and TMv (R2 = 0.44, p < 0.001) as well as M-SFP and M-SH (R2 = 0.48, p < 0.001) and M-SFP and S (R2 = 0.14, p < 0.001).
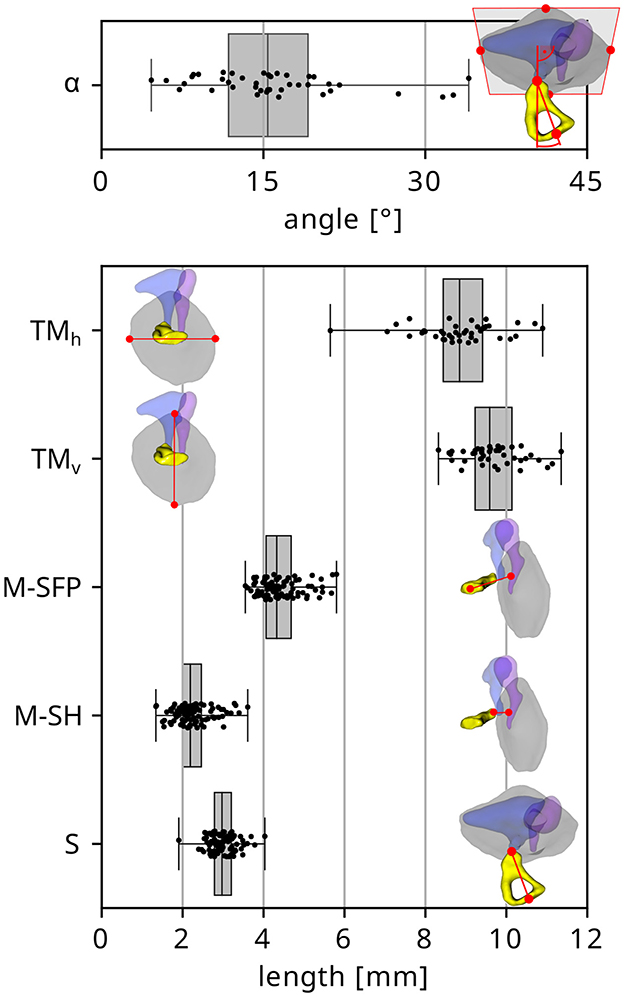
Figure 3. Distribution of the investigated anatomical dimensions (from top to bottom: stapes angle, horizontal and vertical TM diameter respectively, distance from central malleus handle to stapes footplate and stapes head respectively, stapes length).
In order to investigate the reproducibility of the conducted anatomical measurements, the fiducials described in Section 2.2 were placed again in 20 of the ears investigated within this study. This was done once by the same observer who conducted the initial assessments (obs1) and then repeated by a second, independent observer (obs2). The resulting intra and inter observer deviations are depicted as Bland-Altman and box plots in Figure 4. The results show noticeable differences in individual measurements both between measurements and observers. Comparisons of the ranges of derived anatomical dimensions revealed only one statistically significant difference, which was for the vertical tympanic membrane diameter TMv between observers 1 and 2. No statistically significant differences could be observed for any dimensions relevant for the investigated prostheses (i.e., M-SFP, M-SH or S).
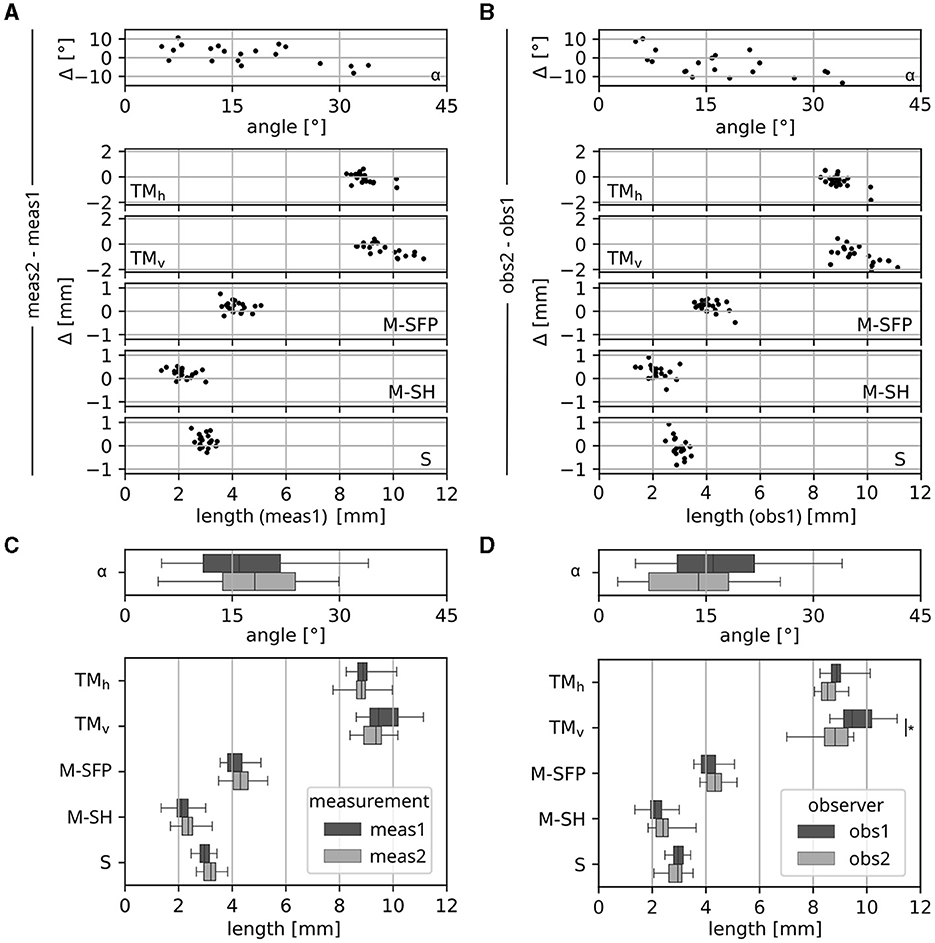
Figure 4. differences in dimensions as the result of 2 consecutive measurements (meas1 and meas2) by the same observer (obs1) as well as 2 measurements by two different observers (obs1 and obs2) in a subset of 20 ears, shown as (A, B) Bland-Altman plots to depict deviations in individual measurements as well as (C, D) box plots to show differences in the resulting ranges of anatomical dimensions, which were found to be not significant except for TMv between obs1 and obs2.
The subsequent step of the investigation was the extraction of the different types and lengths of available prostheses mentioned in Section 2.4. In total, 404 different implants were found and categorized. An overview these PMEIs is given in Table 1. The functional lengths offered by different manufacturers was then compared to the distributions of anatomical dimensions relevant for the respective PMEI type. In case of total prostheses, available implant lengths were compared to the distance from central malleus handle to stapes footplate M-SFP minus 0.5 mm to account for the recommended cartilage slice between implant and TM (Mürbe et al., 2002). For partial prostheses, the distance from central malleus handle to stapes head M-SH minus 0.5 mm cartilage thickness was used as the anatomical reference. In case of stapesplasty prostheses, the length of the stapes S plus 0.5 mm was used to account for the recommended insertion depth of the implant piston into the stapes footplate (Mukherjee et al., 2011).
Figure 5 shows the comparison of the corresponding anatomical reference ranges (box plot depiction on the top of each diagram, projected down in red) to the length ranges of total (Figures 5A, B), partial (Figures 5C, D) and stapesplasty implants (Figures 5E, F). Each implant type is separated into fixed (left column) and adjustable length type implants (right column). More detailed information of each individual implant offered by the different manufacturers are given in the Supplementary Figures S2–S22. For the total prostheses (Figures 5A, B), nearly all manufacturers offer implant lengths which cover the entire range of anatomical variation found within this study. In case of the adjustable length total prostheses by Medtronic, it must be noted that a minimal length is only stated for 3 of the 26 available implants. It is hence possible that the lack of implant lengths for very small anatomies depicted in Figure 5B is covered by some implants, but that is not distinguishable from the product catalog. It should also be noted that all manufacturers offer prostheses (adjustable and fixed) which noticeably exceed the anatomical distance they are supposed to cover.
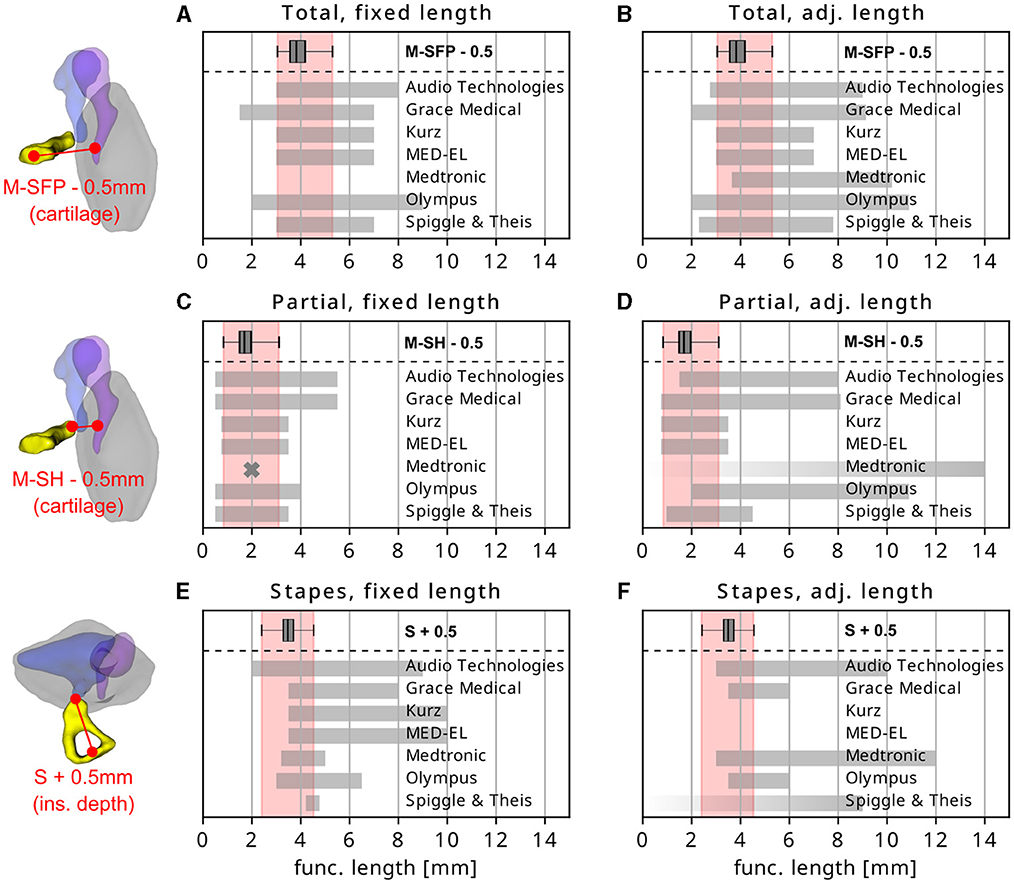
Figure 5. Comparison of available length ranges (in gray) of (A, B) total, (C, D) partial and (E, F) stapes prostheses offered by different manufacturers. Prosthesis groups are divided into (left column) fixed and (right column) adjustable length types. Each image also shows the relevant anatomical variations found within this study, the range of which is projected down in red. Fading edges of gray bars indicate that the respective length limit was not clearly stated by the manufacturer. Crosses indicate that only one specific length value is available.
The comparison of anatomical indication ranges and available implants lengths for partial prostheses is shown in Figures 5C, D. Although the different ranges of anatomies appear to be sufficiently covered overall, only few of the individual devices are available in all necessary sizes—especially regarding very small anatomies (cf. Supplementary Figures S2–S22): the ratio of implants covering the entire anatomical variability to the total number of prostheses is 1/25 for Audio Technologies, 3/49 for Grace Medical, 3/8 for Kurz, 4/4 for MED-EL, 0/27 for Medtronic, 0/15 for Olympus and 3/4 for Spiggle & Theis. Furthermore, some prosthesis types of Audio Technologies, Grace Medical, Medtronic and Olympus are only available in one particular size. Also, Medtronic offers only 2 types of fixed length partial prostheses which are both only available with a functional length of 2 mm, and the lower limit of the length adjustable partial prostheses is not stated for any of the 25 available implant types.
Finally, the comparison the anatomical variability of the stapes length and available stapesplasty implant sizes shows that most of the implant manufacturers do not offer a sufficient portfolio of implant lengths. Most of the available length ranges only cover about half of the derived anatomical variability, stapes pitons for smaller anatomies can only be found within the fixed length pistons offered by Audio Technologies. Other length adjustable devices offered by Audio Technologies, Medtronic and Olympus may be applicable in these cases, but the lower limit of the corresponding length ranges is not stated in the respective product catalogs. Spiggle & Theis only offers fixed length stapes prostheses from 4.25 to 4.75 mm, which only covers the top end of anatomical indications found within this study. The company also offers length adjustable stapesplasty prostheses but does not state the bottom limit of what these devices can be shortened to.
4 Discussion
4.1 Anatomical variations
The present study demonstrated that just like the inner ear (Meng et al., 2016; Timm et al., 2018), the middle ear anatomy shows substantial anatomical variations. While this has been demonstrated in previous studies as well (aWengen et al., 1995; Todd and Creighton, 2013; Kamrava and Roehm, 2017), the present results also highlight that there is not only variability in size but also in shape. Investigation of the horizontal and vertical tympanic membrane diameters, for instance, showed that only 44% of their variance is explained by correlation of the two measures. More relevant for PMEIs are the variations observed in between tympanic membrane and stapes footplate. The distance from central malleus handle to stapes footplate (M-SFP) was shown to correlate with both distance from malleus handle to stapes head (M-SH) and stapes length (S). However, Pearson coefficients were only R2 = 0.48 and R2 = 0.14 respectively. This shows that while the distance through the entire middle ear correlates with dimensions reflecting parts of this overall measure, their contribution to the overall distance varies in between subjects. Similar interrelations were derived by Todd and Creighton (2013) who investigated the correlation in size of malleus and incus. An additional degree of complexity is given by the fact that the central malleus handle is not in line with the stapes (Todd, 2008) and the angle between stapes and tympanic membrane axis (Beutner et al., 2011; Gostian et al., 2013). These factors cause the sum of the distance from malleus handle to stapes head and the stapes length to not correspond to the distance from malleus handle to stapes footplate (cf. Figure 3). So unlike cochlear implantation [where specific measurements like the basal cochlear parameters allow for accurate approximations of other parameters of interest (Schurzig et al., 2018, 2022; Breitsprecher et al., 2023)], tympanoplasty always requires the direct measurement of the dimension of interest itself, e.g., of the distance from central malleus handle to the stapes head M-SH for partial prostheses.
One limitation of the present study is the type of imaging that was employed. Although the resolution of 250 μm of the investigated images is quite high for clinical CBCT data, the images still lack the degree of clarity of the recent advances in photon-counting technology (Willemink et al., 2018; Nakamura et al., 2023) or even higher resolution imaging methods like synchrotron radiation phase-contrast imaging (Elfarnawany et al., 2017). Hence, the derived values are affected by assessment errors due to the imaging, which may reach values as high as the voxel size itself. Furthermore, the comparison of dimensions derived by different measurements and observers demonstrated a lack of reproducibility in conducting individual measurements (cf. Figures 4A, B), likely caused by the abovementioned lack of clarity in visualizing the ossicles in clinical CT imaging: the small size of the ossicles yields a blurry boundary when transitioning from bone to air, especially in case of the stapes. Small deviations in assessments between observers in combination with the small sizes of the ossicles hence cause noticeable relative deviations in anatomical dimensions between observers and measurements, exceeding the voxel size of the imaging. The employed clinical imaging technology is hence not sufficient for the assessment of patient specific ossicle dimensions, e.g., as part of the clinical planning prior to middle ear reconstruction. Hopefully, new technological advancements like photon-counting CT (Willemink et al., 2018; Nakamura et al., 2023) can help with these current, clinical limitations and enable more reliable quantitative assessments of patient specific middle ear dimensions in the future. An important finding regarding the present study is that no significant differences could be observed for the ranges of dimension relevant for the investigated prostheses (i.e., the distances from malleus handle to stapes footplate M-SFP and stapes head M-SH or the length of the stapes S) between measurements or observers (cf. Figures 4C, D). Thus, it can be assumed that the derived ranges of these middle ear dimensions are a sufficiently accurate foundation for the comparison with available PMEI lengths.
One further limitation of the present study is that all measurements were taken in normal middle ear anatomies. Consequently, the assessed middle ear dimensions may differ from those of malformed or diseased middle ears, e.g., because the tympanic membrane may be retracted. It is therefore unclear if the development of novel prostheses is justified exclusively based on the current study. It was, for instance, shown that the smallest stapes found within the patient cohort had a length of under 2 mm. The need for an accordingly small prothesis, however, can only be verified if such small dimensions can also be assessed within otosclerotic ears. Nevertheless, we believe that the assessed dimensions provide a good general foundation for what length ranges specific prostheses should cover. In the future, the anatomical part of the present study should be repeated on a large set of high-resolution imaging of pathologic middle ears to compare the spatial dimensions relevant for PMEIs.
4.2 PMEI specifications
Clear length specifications of PMEIs within the manufacturers' product catalogs and implant packaging are crucial for selecting the right implant for an individual patient. Unfortunately, in conducting the present study we found that these specifications are not always given. Especially in case of length adjustable implants, the bottom limit of this adjustability was often missing and—in case of some manufacturers—not stated for any available PMEIs. Furthermore, length specifications are often stated differently for different manufacturers. In fact, comparable length information across manufacturers was given only for total prostheses: the total length (TL) describes the distance from the top of the head plate to the bottom the prosthesis piston and corresponds to the anatomical distance which is supposed to be bridged by these devices, i.e., from the tympanic membrane [or malleus handle to improve audiological outcomes (Bance et al., 2004)] to the stapes footplate. In case of total prostheses, the functional length (FL), i.e., the length of the section bridging the gap of missing anatomical structures, hence corresponds to the TL of the implant.
Unfortunately, this is typically not the case for partial prostheses. In patients requiring the use of these devices, the distance which needs to be bypassed (i.e., the FL) corresponds to the distance from tympanic membrane/central malleus handle to the stapes head. This is relevant as partial prostheses typically have an adapter at the proximal end of the shaft to enable sufficient coupling of the prosthesis to the stapes head, or the prosthesis piston is hollow such that it can be placed over the stapes head and protect the implant against slipping out of place. The TL of the implant is hence strongly affected by the design of the individual prosthesis (as is depicted for TL1 and TL2 in Figure 6A) and does not correspond to the FL. If taking into account that prestressing the AL surrounding the stapes footplate entails substantial losses in sound transfer (Bance et al., 2004; Morris et al., 2004; Merchant and Rosowski, 2013; Neudert et al., 2016; Schär et al., 2023), length specifications of partial prostheses should hence always include the FL of the device such that surgeons can select an implant which matches the patient specific, intraoperative measurement.
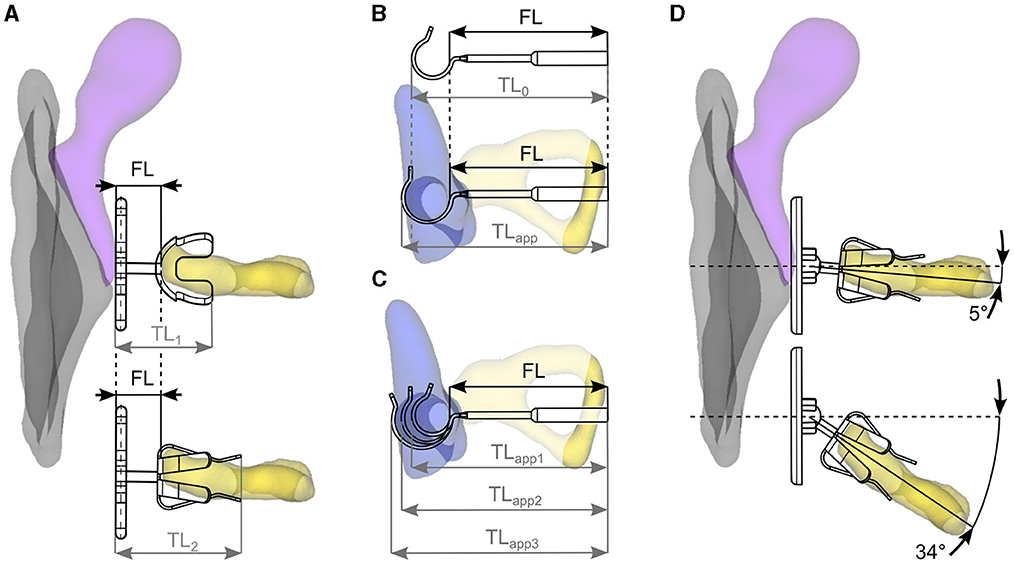
Figure 6. (A) Relevant for surgical reconstructions with passive prostheses is the functional length FL rather than total length TL as it describes the middle ear distance to be bridged independent of the prosthesis design. (B) The initial total length TL0 of a stapesplasty prosthesis may differ from the total length after application TLapp, and (C) the latter is further affected by the size of the long process of the incus, highlighting the importance of FL specifications for stapesplasty prostheses. (D) Accommodation of the derived extrema in angular deviations between tympanic membrane axis (dashed line) and stapes axis (solid line) by a partial prosthesis design containing a ball joint.
Similar length specification issues occur in case of stapesplasty prostheses due to the loop at the distal end of these devices. This loop is supposed to be crimped around the long process of the incus during surgery, which can be done mechanically or using shape memory alloys which wrap around the incus when heat is applied to the structure. In either case, the initial loop diameter and implant size TL0 will only rarely correspond to the length after implantation TLapp (see Figure 6B). Furthermore, neither one of these dimensions corresponds to the distance FL needing to be bridged in these stapesplasty, which ranges from the proximal end of the long process of the incus to just below the stapes footplate. An additional factor making total length specifications of stapesplasty prostheses unsuitable is the variation of the diameter of the long process of the incus (Chien et al., 2009; Tóth et al., 2013). Studies have demonstrated that this diameter can range from under 500 μm to about 1.5 mm. Depending on the size of an individual incus, the TL of a stapesplasty prosthesis after implantation can vary noticeably between subjects, as is depicted in Figure 6C. The most straightforward and reliable approach for selecting an implant length ideally suited for a particular patient is hence to measure the distance between long incus process and stapes footplate, add the desired insertion depth of the implant piston and select an implant whose FL corresponds to this specific value, making a clear statement of the respective FL value within the implant specifications mandatory.
4.3 PMEI sizes
One of the most obvious findings within this study is that nearly all manufacturers offer implant lengths which far exceed the derived anatomical indication ranges (Figure 5). The maximum distance from central malleus handle to stapes footplate within the study cohort was 5.8 mm, resulting in a maximum required FL of a total prosthesis of 5.3 mm if considering a cartilage slice of 0.5 mm between prosthesis and tympanic membrane. As shown in Figures 5A, B, maximally available total prosthesis lengths exceed this value by far, with the largest fixed length protheses offered by Audio Technologies (e.g., with the 8 mm AUDIO-HA® Total Prosthesis) and Olympus (9 mm Wehrs II Incus-Stapes System). Since these devices cannot be shortened, their applicability is questionable if planning to avoid excessive pretension of the AL and corresponding losses in sound conduction. One potential indication may be patients with strongly lateralized tympanic membranes, requiring an accordingly longer prosthesis to bridge the gap between outer and inner ear (Sperling and Kay, 2000).
The same holds true for partial prostheses: the maximum anatomical distance to be bridged by these devices was found to be 3.6 mm, which corresponds to a maximum FL of 3.1 mm if a cartilage slice of 0.5 mm thickness is used. With a FL of 5.5 mm, the maximum fixed length partial prostheses offered by Audio Technologies and Grace Medical are substantially longer than the anatomical indication range and should hence not be employed If wanting to avoid excessive AL pretension. Another interesting observation was made when further inspecting the fixed length partial prostheses: Audio Technologies, Grace Medical, Medtronic and Olympus offer devices which are only available in one specific length. Although most of these specific length values lie close to the center of the derived anatomical variability and are hence made for average anatomies, studies could demonstrate clearly that even small preloads onto the stapes cause severe reductions in sound transmission. Unless devices are made for specific cases like the Goldenberg CAP Prosthesis which is clearly designed for particularly small anatomies, the derived anatomical variability suggests that implants should generally be available in different lengths.
For the fixed length stapesplasty prostheses it could be observed that Audio Technologies is the only manufacturer who offers devices which cover the entire anatomical indication range. This is owed to their Platinum Prosthesis for Stapedectomy (SPL 03.00) which is available in 2 mm and longer. All other manufacturers lack stapesplasty pistons which can—according to our study results—be safely implanted into smaller anatomies. In case of Spiggle & Theis it can only be stated that with 4.25–4.75 mm, their fixed length stapesplasty prostheses cover the smallest range of all investigated manufacturers and are only applicable in case of very long stapes lengths. The length adjustable prostheses offered by Spiggle & Theis may be applicable in all other cases but unfortunately, their product catalog is lacking the corresponding length specifications. It must be noted here that the present study was conducted with a target insertion depth of 0.5 mm of the prosthesis piston into the SFP. This value was postulated by Mukherjee et al. (2011) as the maximal insertion depth which will reliably transfer the piston movements into the perilymph without touching the utricular macula. However, another factor that must be considered in deciding on a target insertion depth are piston displacements not related to incoming sound, i.e., due to sneezing and/or atmospheric pressure changes. While inserting the stapesplasty piston 0.5 mm into the SFP was proposed to be sufficient for preventing piston dislocation due to sneezing (Fisch, 1994), research could also show that atmospheric pressure changes (e.g., when diving or flying) may displace the stapes piston by more than 0.5 (Hüttenbrink, 1988, 2003). Hence, a stapedotomy was suggested to be advisable at the posterior section of the SFP such that piston contact to the utriculus is avoided and the distance to the saccule is maximized (Hüttenbrink, 2003). In this case, the piston could be inserted beyond the 0.5 mm mark, which would shift the anatomical indication ranges (shaded areas in Figures 5E, F) toward larger values and hence create a larger overlap with currently available implant sizes. Another factor that must be addressed is that the reason for offering stapesplasty prostheses which far exceed the derived range of stapes length (plus an insertion depth of 0.5 mm and more) may have to do with manufacturers trying to not only offer devices for stapesplasty but also for malleovestibulopexy. In the latter case, the prosthesis must bridge the gap between malleus handle and SFP (i.e., a distance larger than the stapes length S), requiring accordingly longer prosthesis lengths.
A general finding regarding all length adjustable prostheses is that while the anatomical variation may be covered by nearly all manufacturers, that does not necessarily hold true for all devices offered within the respective product portfolios. This can be quantified by calculating the ratio of the number of implants covering the entire anatomical variability to all implants with sufficient length specifications. Length adjustable implants which do not cover the derived anatomical variability are offered by Audio Technologies (6/16), Medtronic (0/3) and Olympus (2/3) in case of total prostheses and Audio Technologies (0/6), Grace Medical (2/21), Olympus (0/3) and Spiggle & Theis (0/1) for partial prostheses. As mentioned before, no length adjustable stapesplasty implants were found which sufficiently cover the derived anatomical variability. These findings are highly relevant for hospitals wanting to reduce the number of locally stored implants by using length adjustable devices, as the ratios point out that the specific implant to be used must be chosen with care.
4.4 Angle between tympanic membrane and stapes
Within the present study, the angle between tympanic membrane and stapes α was found to lie between 4.6° and 34.0° with a median of 15.4°. A visual representation of this angular range is given in Figure 6D, and recent developments in partial prosthesis designs were aimed at accounting for this angular range by a more stable fit of the prosthesis onto the stapes (Schär et al., 2023) and a ball joint at prosthesis head (Beutner et al., 2011; Gostian et al., 2013). These ball joint prostheses are now commercially available (Clip Partial Flexibal Prosthesis by Kurz, mCLIP ARC Partial by MED-EL) and were shown to yield better results than partial prostheses without this joint (Stoppe et al., 2018; Schär et al., 2023).
5 Conclusion
The present study demonstrates that the middle ear anatomy shows substantial anatomical variations in size and shape. The resulting range of required implant lengths for full and partial prostheses is covered by nearly all manufacturers, but not by each implant. As for stapesplasty prostheses, there is an unmet clinical need for smaller devices to match the individual anatomy of every patient's middle ear. Passive implants with angular adjustment options between tympanic membrane and stapes appear to better adjust to the natural anatomic orientation of the ossicles.
Data availability statement
The raw data supporting the conclusions of this article will be made available by the authors, without undue reservation.
Ethics statement
The studies involving humans were approved by Ethics Committee of the Hannover Medical School. The studies were conducted in accordance with the local legislation and institutional requirements. Written informed consent for participation was not required from the participants or the participants' legal guardians/next of kin in accordance with the national legislation and institutional requirements.
Author contributions
TL: Writing – review & editing, Supervision, Resources, Project administration, Conceptualization. MB: Writing – original draft, Visualization, Validation, Methodology, Investigation, Data curation, Conceptualization. AW: Writing – review & editing, Writing – original draft, Validation, Supervision, Resources, Project administration, Methodology, Investigation, Formal analysis, Conceptualization. AG: Writing – review & editing, Visualization, Validation, Methodology, Investigation, Formal analysis, Data curation. NP: Writing – review & editing, Supervision, Resources, Project administration, Data curation, Conceptualization. US: Writing – review & editing, Visualization, Validation, Supervision, Resources, Investigation, Formal analysis, Data curation, Conceptualization. DS: Writing – review & editing, Writing – original draft, Visualization, Supervision, Project administration, Methodology, Investigation, Formal analysis, Conceptualization.
Funding
The author(s) declare that financial support was received for the research, authorship, and/or publication of this article. The study was supported by Deutsche Forschungsgemeinschaft (DFG Exc 2177). Publication of this manuscript was supported by “Zukunft.Niedersachsen” through the publication fund “NiedersachsenOPEN.”
Conflict of interest
DS and US are employed by MED-EL, one of the manufacturers of the investigated passive middle ear implants. However, the data collection and analysis was conducted independently by the Hannover Medical School, and all data is made available with the submission such that the validity of the results can be checked. Furthermore, it was derived within the present study that there is a lack of specific middle ear implant sizes (for stapesplasty) which also applies to MED-EL. The present research was hence conducted in a scientifically valid manner without any bias.
The remaining authors declare that the research was conducted in the absence of any commercial or financial relationships that could be construed as a potential conflict of interest.
The author(s) declared that they were an editorial board member of Frontiers, at the time of submission. This had no impact on the peer review process and the final decision.
Publisher's note
All claims expressed in this article are solely those of the authors and do not necessarily represent those of their affiliated organizations, or those of the publisher, the editors and the reviewers. Any product that may be evaluated in this article, or claim that may be made by its manufacturer, is not guaranteed or endorsed by the publisher.
Supplementary material
The Supplementary Material for this article can be found online at: https://www.frontiersin.org/articles/10.3389/fauot.2024.1418921/full#supplementary-material
References
Amith, N., and Rs, M. (2017). Autologous incus versus titanium partial ossicular replacement prosthesis in reconstruction of Austin type A ossicular defects: a prospective randomised clinical trial. J. Laryngol. Otol. 131, 391–398. doi: 10.1017/S0022215117000251
Austin, D. F. (1969). Types and indications of staging. Arch. Otolaryngol. 89, 235–242. doi: 10.1001/archotol.1969.00770020237005
aWengen, D. F., Nishihara, S., Kurokawa, H., and Goode, R. L. (1995). Measurements of the stapes superstructure. Ann. Otol. Rhinol. Laryngol. 104, 311–316. doi: 10.1177/000348949510400411
Bance, M., Morris, D. P., Vanwijhe, R. G., Kiefte, M., and Funnell, W. R. J. (2004). Comparison of the mechanical performance of ossiculoplasty using a prosthetic malleus-to-stapes head with a tympanic membrane-to-stapes head assembly in a human cadaveric middle ear model. Otol. Neurotol. 25, 903–909. doi: 10.1097/00129492-200411000-00008
Beutner, D., and Hüttenbrink, K. B. (2009). Passive and active middle ear implants. Laryngorhinootologie. 88, S32–47. doi: 10.1055/s-0028-1119493
Beutner, D., Luers, J. C., Bornitz, M., Zahnert, T., and Huttenbrink, K. B. (2011). Titanium clip ball joint: a partial ossicular reconstruction prosthesis. Otol. Neurotol. 32, 646–649. doi: 10.1097/MAO.0b013e318213867a
Breitsprecher, T., Mlynski, R., Volter, C., de Heyning, P., van Rompaey, V., Dazert, S., et al. (2023). Accuracy of preoperative cochlear duct length estimation and angular insertion depth prediction. Otol. Neurotol. 44, e566–e571. doi: 10.1097/MAO.0000000000003956
Chien, W., Northtop, C., Levine, S., Pilch, B., Peake, W., Rosowski, J. J., et al. (2009). Anatomy of the distal incus in humans. J. Assoc. Res. Otolaryngol. 10, 485–496. doi: 10.1007/s10162-009-0179-6
Elfarnawany, M., Riyahi Alam, S., Rohani, S. A., Zhu, N., Agrawal, S. K., and Ladak, H. M. (2017). Micro-CT versus synchrotron radiation phase contrast imaging of human cochlea. J. Microsc. 265, 349–357. doi: 10.1111/jmi.12507
Fedorov, A., Beichel, R., Kalpathy-Cramer, J., Finet, J., Fillion-Robin, J. C., et al. (2012). 3D Slicer as an image computing platform for the Quantitative Imaging Network. Magn. Reson. Imaging 30, 1323–1341. doi: 10.1016/j.mri.2012.05.001
Gan, R. Z., Yang, F., Zhang, X., and Nakmali, D. (2011). Mechanical properties of stapedial annular ligament. Med. Eng. Phys. 33, 330–339. doi: 10.1016/j.medengphy.2010.10.022
Gostian, A. O., Pazen, D., Luers, J. C., Huttenbrink, K. B., and Beutner, D. (2013). Titanium ball joint total ossicular replacement prosthesis – Experimental evaluation and midterm clinical results. Hear. Res. 301, 100–104. doi: 10.1016/j.heares.2012.10.009
Hüttenbrink, K. B. (1988). The mechanics of the middle-ear at static air pressures: the role of the ossicular joints, the function of the middle-ear muscles and the behaviour of stapedial prostheses. Acta Oto-Laryngologica 105, 1–35. doi: 10.3109/00016488809099007
Hüttenbrink, K. B. (2003). Biomechanics of stapesplasty: a review. Otol. Neurotol. 24, 548–557. doi: 10.1097/00129492-200307000-00004
Kamrava, B., and Roehm, P. C. (2017). Systematic review of ossicular chain anatomy: strategic planning for development of novel middle ear prostheses. Otolaryngol.–Head Neck Surg. 157, 190–200. doi: 10.1177/0194599817701717
Kartush, J. M. (1994). Ossicular chain reconstruction: capitulum to malleus. Otolaryngologic Clinics of North America 27, 689–715. doi: 10.1016/S0030-6665(20)30641-1
Koike, T., Shinozaki, M., Murakami, S., Homma, K., Kobayashi, T., and Wada, H. (2005). Effects of individual differences in size and mobility of the middle ear on hearing. JSME Int. J. Series C Mech. Syst. Mach. Elements Manufact. 48, 521–528. doi: 10.1299/jsmec.48.521
Kortebein, S., Russomando, A. C., Greda, D., Cooper, M., Ledbetter, L., and Kaylie, D. (2023). Ossicular chain reconstruction with titanium prostheses: a systematic review and meta-analysis. Otol. Neurotol. 44, 107–114. doi: 10.1097/MAO.0000000000003772
Lauxmann, M., Eiber, A., Haag, F., and Ihrle, S. (2014). Nonlinear stiffness characteristics of the annular ligament. J. Acoust. Soc. Am. 136, 1756–1767. doi: 10.1121/1.4895696
Lord, R. M., Mills, R. P., and Abel, E. W. (2000). An anatomically shaped incus prosthesis for reconstruction of the ossicular chain. Hear. Res. 145, 141–148. doi: 10.1016/S0378-5955(00)00085-X
Meng, J., Li, S., Zhang, F., Li, Q., and Qin, Z. (2016). Cochlear size and shape variability and implications in cochlear implantation surgery. Otol. Neurotol. 37, 1307–1313. doi: 10.1097/MAO.0000000000001189
Merchant, S. N., and Rosowski, J. J. (2013). “Surgical reconstruction and passive prostheses,” in The Middle Ear: Science, Otosurgery, and Technology, Herausgeber, eds. Puria, S., Fay, R. R., and A. N. Popper (New, York: Springer), 253–272.
Mojallal, H., Stieve, M., Krueger, I., Behrens, P., Mueller, P., and Lenarz, T. (2009). A biomechanical ear model to evaluate middle-ear reconstruction. Int. J. Audiol. 48, 876–884. doi: 10.3109/14992020903085735
Morris, D. P., Bance, M., and Van Wijhe, R. G. (2004). How do cartilage and other material overlay over a prosthesis affect its vibration transmission properties in ossiculoplasty? Otolaryngol.–Head Neck Surg. 131, 423–428. doi: 10.1016/j.otohns.2004.02.055
Mudhol, R. S., Naragund, A. I., and Shruthi, V. S. (2013). Ossiculoplasty: revisited. Indian J. Otolaryngol. Head Neck Surg. 65, 451–454. doi: 10.1007/s12070-011-0472-7
Mukherjee, P., Uzun-Coruhlu, H., Curthoys, I. S., Jones, A. S., Bradshaw, A. P., and Pohl, D. (2011). Three-dimensional analysis of the vestibular end organs in relation to the stapes footplate and piston placement. Otol. Neurotol. 32, 367–372. doi: 10.1097/MAO.0b013e3182096ddd
Mürbe, D., Zahnert, T., Bornitz, M., and Hüttenbrink, K. (2002). Acoustic properties of different cartilage reconstruction techniques of the tympanic membrane. Laryngoscope 112, 1769–1776. doi: 10.1097/00005537-200210000-00012
Nakamura, Y., Higaki, T., Kondo, S., Kawashita, I., Takahashi, I., and Awai, K. (2023). An introduction to photon-counting detector CT (PCD CT) for radiologists. Jpn. J. Radiol. 41, 266–282. doi: 10.1007/s11604-022-01350-6
Neudert, M. (2020). Quality in middle ear surgery - a critical position determination. Laryngorhinootologie 99, S222–S271. doi: 10.1055/a-1021-6427
Neudert, M., Bornitz, M., Lasurashvili, N., Schmidt, U., Beleites, T., and Zahnert, T. (2016). Impact of prosthesis length on tympanic membrane's and annular ligament's stiffness and the resulting middle ear sound transmission. Otol. Neurotol. 37, e369–e376. doi: 10.1097/MAO.0000000000001064
Pickles, J. (2013). An Introduction to the Physiology of Hearing: Fourth Edition. Leiden: The Brill.
Ringeval, S., Fortunier, R., Forest, B., and Martin, C. (2004). Influence of the shape and material on the behaviour of a total ossicular replacement prosthesis. Acta Otolaryngol. 124, 789–792. doi: 10.1080/00016480410018016
Schär, M., Dobrev, I., Röösli, C., Huber, A. M., and Sim, J. H. (2023). Effects of preloads on middle-ear transfer function and acoustic reflex in ossiculoplasty with PORP. Hear. Res. 430, 108709. doi: 10.1016/j.heares.2023.108709
Schurzig, D., Repp, F., Timm, M., Batsoulis, C., Lenarz, T., and Kral, A. (2022). Virtual cochlear implantation for personalized rehabilitation of profound hearing loss. Hear. Res. 429, 108687. doi: 10.1016/j.heares.2022.108687
Schurzig, D., Timm, E., Batsoulis, C., Salcher, R., Sieber, D., Jolly, C., et al. (2018). A novel method for clinical cochlear duct length estimation toward patient-specific cochlear implant selection. OTO Open 2, 2473974X18800238. doi: 10.1177/2473974X18800238
Sperling, N. M., and Kay, D. (2000). Diagnosis and management of the lateralized tympanic membrane. Laryngoscope 110, 1987–1993. doi: 10.1097/00005537-200012000-00001
Stoppe, T., Borntiz, M., Lasurashvili, N., Sauer, K., Zahnert, T., Zaoui, K., et al. (2018). Function, applicability, and properties of a novel flexible total ossicular replacement prosthesis with a silicone coated ball and socket joint. Otol. Neurotol. 39. doi: 10.1097/MAO.0000000000001797
Timm, M. E., Majdani, O., Weller, T., Windeler, M., Lenarz, T., Buchner, A., et al. (2018). Patient specific selection of lateral wall cochlear implant electrodes based on anatomical indication ranges. PLoS ONE 13, e0206435. doi: 10.1371/journal.pone.0206435
Todd, N. W. (2008). The malleus-stapes offset. Laryngoscope 118, 110–115. doi: 10.1097/MLG.0b013e318155a299
Todd, N. W., and Creighton, F. (2013). Malleus and incus: correlates of size. Ann. Otol. Rhinol. Laryngol. 122, 60–65. doi: 10.1177/000348941312200111
Tóth, M., Moser, G., Rösch, S., Grabmair, S., and Rasp, G. (2013). Anatomic parameters of the long process of incus for stapes surgery. Otol. Neurotol. 34, 1564–1570. doi: 10.1097/MAO.0b013e3182a43619
Willemink, M. J., Persson, M., Pourmorteza, A., Pelc, N. J., and Fleischmann, D. (2018). Photon-counting CT: technical principles and clinical prospects. Radiology 289, 293–312. doi: 10.1148/radiol.2018172656
Yu, H., He, Y., Ni, Y., Wang, Y., Lu, N., and Li, H. (2013). PORP vs. TORP: a meta-analysis. Otol. Neurotol. 270, 3005–3017. doi: 10.1007/s00405-013-2388-1
Keywords: hearing loss, tympanoplasty, ossiculoplasty, stapesplasty, middle ear reconstruction, anatomical variability, sound conduction
Citation: Lenarz T, Becker M, Warnecke A, Giesemann A, Prenzler NK, Steinhardt U and Schurzig D (2024) Middle ear anatomy and implant sizes: correlates and the need for uniform implant dimensions. Front. Audiol. Otol. 2:1418921. doi: 10.3389/fauot.2024.1418921
Received: 17 April 2024; Accepted: 06 June 2024;
Published: 25 June 2024.
Edited by:
Mario Emilio Zernotti, Catholic University of Córdoba, ArgentinaReviewed by:
Ingo Todt, Bielefeld University, GermanyAndrew A. McCall, University of Pittsburgh, United States
Copyright © 2024 Lenarz, Becker, Warnecke, Giesemann, Prenzler, Steinhardt and Schurzig. This is an open-access article distributed under the terms of the Creative Commons Attribution License (CC BY). The use, distribution or reproduction in other forums is permitted, provided the original author(s) and the copyright owner(s) are credited and that the original publication in this journal is cited, in accordance with accepted academic practice. No use, distribution or reproduction is permitted which does not comply with these terms.
*Correspondence: Daniel Schurzig, c2NodXJ6aWcuZGFuaWVsJiN4MDAwNDA7bWgtaGFubm92ZXIuZGU=
†These authors have contributed equally to this work and share first authorship