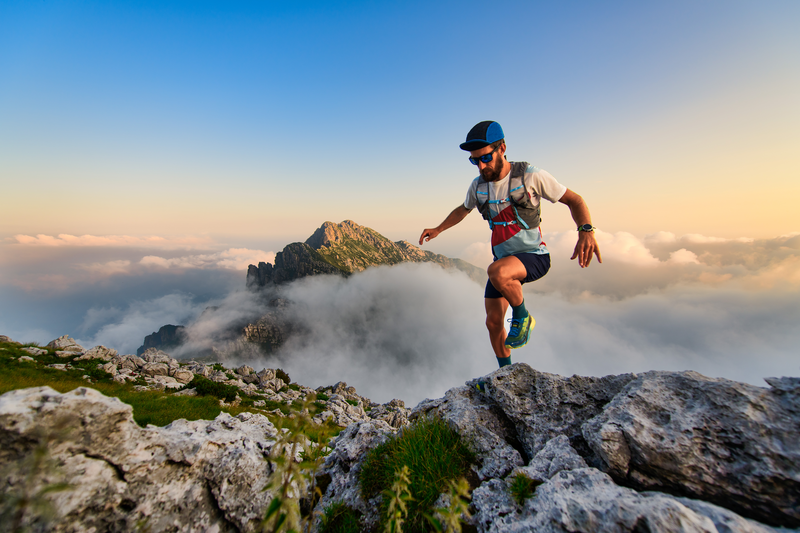
94% of researchers rate our articles as excellent or good
Learn more about the work of our research integrity team to safeguard the quality of each article we publish.
Find out more
PERSPECTIVE article
Front. Audiol. Otol. , 29 September 2023
Sec. Auditory Science
Volume 1 - 2023 | https://doi.org/10.3389/fauot.2023.1279818
This integrative review and perspective article synthesizes current knowledge about the co-morbidity of Type 1 Diabetes (T1D) and sensorineural hearing loss (SNHL), affecting an estimated 9.2 per 1,000 T1D patients annually. Combining data from clinical, preclinical, and mechanistic studies, the article elucidates the complex pathological mechanisms contributing to SNHL in T1D. It is established that T1D accelerates age-related SNHL and brings about detrimental changes in the auditory system, including damage to outer hair cells (OHCs), inner hair cells (IHCs), the stria vascularis (SV), and the spiral ligament (SL). Furthermore, T1D-associated peripheral neuropathy, microvascular damage, and chronic inflammation in the inner ear contribute to auditory deficits. Although some consistency exists between animal models and human conditions, notable discrepancies warrant the refinement of preclinical models to more accurately mirror human clinical scenarios. This perspective article highlights the need for targeted research to bridge existing knowledge gaps and accelerate the development of early-stage interventions for SNHL in T1D patients. Advancements in this field hold the promise of enhancing clinical prognosis and improving the quality of life of individuals having T1D.
Diabetes mellitus (DM) is a chronic metabolic disorder characterized by elevated blood glucose levels. It is a significant global public health concern, affecting both developed and developing countries to varying degrees (Lawrence et al., 2021; Mahase, 2022; Abraham et al., 2023; Wu et al., 2023). As of 2017, the global incidence of DM reached 22.9 million, with a steady increase in its prevalence, which now stands at 476.0 million (Lin et al., 2020; Mahase, 2022). There are two main types of diabetes: Type 1 (T1D) and Type 2 (T2D). T1D is characterized by autoimmune-mediated β-cell destruction, resulting in life-long absolute insulin deficiency. In contrast, T2D is marked by metabolically-mediated progressive β-cell dysfunction in the setting of insulin resistance, leading to relative insulin deficiency (Eizirik et al., 2020; Ingrosso et al., 2023). Central to the pathogenesis of DM is pancreatic β-cell dysfunction and death (Ingrosso et al., 2023). Globally, about 9 million people live with T1D, representing approximately 2% of all estimated cases of DM (Green et al., 2021).
T1D is accompanied by numerous co-morbidities, including sensorineural hearing loss (SNHL). Approximately 9.2 per 1,000 individuals with T1D develop debilitating SNHL each year (Kim et al., 2017). Both preclinical and clinical studies have shown the occurrence of SNHL in T1D. In a preclinical Akita mouse model of T1D, it was observed that the insulin-dependent Akita group showed significantly increased hearing thresholds at 16 and 32 kHz compared to the wild-type (WT) group, indicating that T1D causes hearing impairment (Lee et al., 2020). In a large prospective cohort clinical study with over 250,000 participants, it was found that individuals with DM were at an increased risk of SNHL, even after adjusting for multiple potential confounders, including demographic characteristics, occupational noise exposure, lifestyle risk factors, and other metabolic abnormalities (Kim et al., 2017). The risk of SNHL increased progressively with increasing A1c levels above 5% (Kim et al., 2017). A meta-analysis that explored auditory brainstem-evoked responses (ABR) showed that diabetic patients had a significantly slower nerve conduction into the auditory cortex than control patients suggesting peripheral diabetic neuropathy (Mujica-Mota et al., 2018).
Another human study found that T1D is a factor that can damage hearing, the negative effect of which is compounded by aging (Horváth et al., 2023). In patients with T1D aged 19–39, the hearing threshold in middle to high frequencies (500 to 4000 Hz) was significantly higher when compared to controls of the same age; however, the hearing threshold in both groups was still below 25 dB at all frequencies. In patients with T1D aged 40–60, the hearing threshold in high frequencies (4,000–8,000 Hz) was significantly higher when compared to same-aged controls, requiring thresholds of 25 to 30 dB where controls had thresholds of 5–10 dB (Horváth et al., 2023). The hearing of the 19–39 aged T1D group was most like that of the 40–60 aged control group, and the most significant SNHL was demonstrated in the 40–60 aged T1D group; these results show that T1D could accelerate age-related SNHL (Horváth et al., 2023).
Several pathological mechanisms have been proposed to explain the association between T1D and SNHL, including microvascular damage, neuropathic complications, and chronic inflammation, known to affect other organ systems in patients with diabetes, that may also impact the structures of the inner ear (Figure 1). Among the proposed mechanisms are damage to outer hair cells (OHCs) and inner hair cells (IHCs), loss of spiral ganglion neurons (SGNs) through apoptosis-mediated cell death, thickening of the basement membrane (BM), and pathologies involving the stria vascularis (SV) and spiral ligament (SL) of the cochlear lateral wall (LW) (Fukushima et al., 2005; Kariya et al., 2010; Akinpelu et al., 2014; Kim et al., 2017; Lee et al., 2020) (Table 1).
Figure 1. Potential pathological mechanisms underlying sensorineural hearing loss in Type 1 diabetes (T1D). Taken and adapted from Horváth et al. (2023) distributed under the terms of the Creative Commons Attribution License, which permits unrestricted use, distribution, and reproduction in any medium, provided the original author and source are credited.
Table 1. A summary of changes in cochlear structures leading to sensorineural hearing loss (SNHL) in type 1 diabetes mellitus (T1D).
This perspective article aims to delve into the recent advancements regarding our understanding of the pathological mechanisms underlying SNHL in T1D (Figure 1). By gaining a better insight into these pathological underpinnings, we can lay the foundation for the development of novel, effective preventive, and therapeutic strategies for SNHL in individuals with T1D.
Sensory outer and inner hair cells within the organ of Corti (OC) play a crucial role in sound perception and signal transmission to the auditory cortex in the brain (Driver and Kelley, 2020; Helwany et al., 2023; White et al., 2023). Therefore, damage to these sensory cells has been implicated in SNHL. Human temporal bone studies have revealed significant loss of OHCs in individuals with T1D compared to the control group (Fukushima et al., 2005). Moreover, in the same study, an accelerated rate of age-related OHC loss was observed in patients with T1D compared to the control group, further highlighting the association between T1D and OHC damage.
Conversely, no loss of OHCs and IHCs has been observed in preclinical animal models of T1D, despite exhibiting SNHL. There were no histopathological changes in OHCs and IHCs in the Akita mouse model of T1D compared to the WT animals (Lee et al., 2020). These findings suggest that the OHC and IHC loss observed in human T1D cases may involve factors not adequately represented in the current animal models. This discrepancy highlights the necessity for refining animal models to better replicate the human clinical conditions of T1D, as OHC loss appears to be a contributor to SNHL in humans with T1D but not in the animal models investigated.
Spiral ganglion neurons (SGNs) are bipolar neurons that facilitate transferring auditory signals from sensory cells to the cochlear nucleus in the brainstem (Sun et al., 2021).
SGNs play a crucial role in auditory signal transmission, and their loss has been implicated in SNHL (Hoshino et al., 2019; Liu et al., 2019; Zhang et al., 2022). Notably, animal models of T1D have shown a significant reduction in SGNs. Lee et al demonstrated that in the Akita mouse, the most striking observation was a substantial decrease in the number of SGNs (Lee et al., 2020). At the ultramicroscopic level, SGNs in these mice showed abnormal mitochondria compared to WT, leading to disruptions in their normal structures. Histological analysis of the cochlea confirmed these findings, indicating a significant loss of SGNs in the Akita mice compared to the control animals.
However, cochlea from patients with T1D showed no significant difference in number of SGNs when compared to control (Fukushima et al., 2005). These discrepancies underscore the importance of developing improved models of T1D and to advance our understanding of the role of SGNs in T1D-related SNHL.
The cochlear lateral wall (LW), including the stria vascularis (SV) and spiral ligament (SL), plays an integral role in the maintenance of the endolymphatic potential (Liang et al., 2003, 2004; Hibino et al., 2010; Patuzzi, 2011). In Akita mice, the thickness of the SV was significantly decreased in the middle to basal turns of the cochlea compared to the WT group (Lee et al., 2020). Atrophy of the SV and loss of SL cells in the apical turns have also been observed in human cochlear studies (Fukushima et al., 2005).
The Akita mice also showed abnormal shapes in both the blood-labyrinth barrier and capillary formations, unlike the WT mice (Lee et al., 2020). The vessel walls of the SV in the cochlea were stained positively for cluster of differentiation 31 (CD31), which was significantly decreased in Akita mice compared to WT mice. This reduction in CD31 expression at the SV may be correlated with the altered capillary shapes observed in Akita mice. Similarly, human temporal bones studies have demonstrated changes in the cochlear spiral modiolar artery in patients with T1D (Kariya et al., 2010).
The enzyme Sodium-Potassium Adenosine Triphosphatase (Na+/K+-ATPase) is a critical player in specialized ear electrophysiological functions and is present in the SV and LW structures (Gratton et al., 1995; Liu and Rask-Andersen, 2022; Avillion et al., 2023). The Na+/K+-ATPase oligomer consists of several isoforms of multiple subunits (Ding et al., 2018; Stephenson et al., 2021). Notably, the α subunit within this oligomer contains binding sites for ATP and cations (Na+ and K+). The Akita mouse study examined the expression levels of Na+/K+-ATPase α1 in the cochlea of both WT and Akita mice (Lee et al., 2020). In the WT mice, the expression of Na+/K+-ATPase α1 was detected in type II and IV fibrocytes and the SV in the middle to basal turns. However, in the Akita mice, the expression in type II and IV fibrocytes and the SV in the middle to basal turns was decreased. Additionally, the expression of Na+/K+-ATPase α1 was marked in the SGNs of the WT mice but was significantly decreased in Akita mice. Additionally, the expression of Na+/K+-ATPase α1 was marked in the spiral ganglion neurons (SGNs) of the WT group but significantly decreased in the Akita group.
The caspase pathway plays a crucial role in the orchestration of apoptosis in the cochlea and can be initiated by extrinsic and intrinsic pathways. The caspase-dependent cell death pathway has been widely implicated in the programmed cell death of OHCs and IHCs (Tadros et al., 2008). In the Akita mouse study, higher expression of cleaved caspase-3 was observed in the SV and type I, II, and IV fibrocytes of Akita mice compared to the WT group (Lee et al., 2020). Cleaved caspase-3 was strongly expressed in the basal turn of the OC and nerve fibers. These results are consistent with the thinning of the SV, the loss of type I, II, and IV fibrocytes and SGNs, and the reduction in Na+/K+-ATPase α1, all exhibited in the Akita mouse model. However, no electrophysiological studies were performed to precisely determine the electrolyte influx changes and corresponding deterioration in SV. In summary, the expression level of Na+/K+-ATPase α1 and cleaved caspase-3 in the cochlea of Akita mice suggests their involvement in the observed structural changes, impairment of cellular function, and loss of cells. Nevertheless, further electrophysiological investigations are needed to gain a more comprehensive understanding of the electrolyte influx alterations in the stria vascularis and their implications.
T1D has been associated with SNHL, and numerous studies have shown pathological changes in cochlear structures including OHCs, IHCs, and SGNs (Figure 1). While significant progress has been made in linking T1D to SNHL, there are still knowledge gaps that warrant further investigations. Although certain concordant results have been observed between preclinical and clinical studies, contradictory findings emphasize the need of refining animal models to better replicate the human clinical conditions of T1D. There have been numerous animal models of various species aimed at accurately depicting T1D and its microvascular complications, the most successful of which being rodents and zebrafish for diabetic neuropathy and retinopathy, respectively (Singh et al., 2022; Yagihashi, 2023). A list of T1D rodent models to date has been summarized in Supplementary Table 1 (Lukens, 1948; Craighead et al., 1974; Goto et al., 1976; Like and Rossini, 1976; Nakhooda et al., 1977; Makino et al., 1980; Kawano et al., 1991; Yoshioka et al., 1997; Komeda et al., 1998; Lenzen et al., 2001; Zheng et al., 2004; Lee et al., 2020). Although these models were able to appropriately replicate diabetic neuropathy and retinopathy, hearing was not explicitly tested in these studies. As SNHL in T1D may have a similar pathogenesis to that of other diabetic microvascular complications, further preclinical research using rodents as animal models is warranted to explore this possibility.
Studies have implicated pathological changes in the stria vascularis (SV) as contributors to T1D-induced SNHL. However, to precisely determine the extent of damage, electrophysiological studies are needed. Additionally, time-dependent studies in animal models are essential to identify when cochlear pathological changes begin, leading to deterioration in hearing thresholds. The information available from these studies will enable us to determine the optimal therapeutic window for future novel interventions.
Genetics plays a crucial role in the pathophysiology of T1D and several T1D-associated genes have been identified in the affected individuals (Supplementary Table 2) (Eiberg et al., 2006; Todd et al., 2007; Barrett et al., 2009; Cooper et al., 2009; McKinnon et al., 2009; Bradfield et al., 2011; Evangelou et al., 2014; Onengut-Gumuscu et al., 2015; Törn et al., 2015; Duarte et al., 2017; Rigoli et al., 2018; Klak et al., 2020). Nevertheless, these studies did not explore a connection between these loci and SNHL. However, a missense mutation E864K in the WFS1 gene on chromosome 4p16 has been linked to Wolfram Syndrome 1, a condition characterized by non-autoimmune T1D and SNHL, among other deficits (Eiberg et al., 2006; Rigoli et al., 2018). This highlights the possibility that some T1D-associated variants may directly compromise the function of the inner ear, which warrants exploration in future studies. Furthermore, there is a need to determine the expression T1D-associated genes in the inner ear that has not been characterized so far in the current available studies.
Recent studies have shown the utility of gene and stem cell gene therapy in developing therapeutic strategies for SNHL (Mittal et al., 2017; Scheper et al., 2019; Eshraghi et al., 2020; Bergman et al., 2021; Zhang et al., 2022; Chou and Hsu, 2023). As technology develops further and novel interventions like these continue to arise, SNHL and deafness may become increasingly treatable conditions especially in individuals with T1D. A better knowledge about the pathological mechanisms of T1D-induced SNHL will lead to the development of early interventions for T1D-induced SNHL, resulting in better clinical outcomes and improved quality of life of affected individuals who are already having a debilitating metabolic disorder.
The original contributions presented in the study are included in the article/Supplementary material, further inquiries can be directed to the corresponding authors.
RM: Conceptualization, Investigation, Methodology, Project administration, Resources, Supervision, Visualization, Writing—original draft, Writing—review and editing. NC: Conceptualization, Formal analysis, Investigation, Methodology, Resources, Visualization, Writing—original draft, Writing—review and editing. JL: Conceptualization, Investigation, Methodology, Project administration, Resources, Supervision, Validation, Writing—original draft, Writing—review and editing. GL: Formal analysis, Investigation, Methodology, Validation, Writing—original draft, Writing—review and editing. CF: Conceptualization, Data curation, Investigation, Methodology, Project administration, Supervision, Writing—original draft, Writing—review and editing. KH: Conceptualization, Investigation, Methodology, Project administration, Resources, Supervision, Validation, Visualization, Writing—original draft, Writing—review and editing.
The author(s) declare that no financial support was received for the research, authorship, and/or publication of this article.
We are grateful to Dr. Valerie Gramling for critical reading of the manuscript.
The authors declare that the research was conducted in the absence of any commercial or financial relationships that could be construed as a potential conflict of interest.
The author(s) declared that they were an editorial board member of Frontiers, at the time of submission. This had no impact on the peer review process and the final decision.
All claims expressed in this article are solely those of the authors and do not necessarily represent those of their affiliated organizations, or those of the publisher, the editors and the reviewers. Any product that may be evaluated in this article, or claim that may be made by its manufacturer, is not guaranteed or endorsed by the publisher.
The Supplementary Material for this article can be found online at: https://www.frontiersin.org/articles/10.3389/fauot.2023.1279818/full#supplementary-material
Abraham, A. M., Jacob, J. J., and Varghese, A. (2023). Prevalence of hearing loss in type 2 diabetes mellitus and its association with severity of diabetic neuropathy and glycemic control. J. Assoc. Physicians India 71, 11–12. doi: 10.5005/japi-11001-0270
Akinpelu, O. V., Ibrahim, F., Waissbluth, S., and Daniel, S. J. (2014). Histopathologic changes in the cochlea associated with diabetes mellitus—a review. Otol. Neurotol. 35, 764. doi: 10.1097/MAO.0000000000000293
Avillion, M. P., Lopez, I. A., Matsui, H., Ishiyama, G., and Ishiyama, A. (2023). Differential expression of Na/K-ATPase in the human saccule of patients with and without otologic disease. Otol. Neurotol. Off. Publ. Am. Otol. Soc. Am. Neurotol. Soc. Eur. Acad. Otol. Neurotol. 44, e256–e261. doi: 10.1097/MAO.0000000000003834
Barrett, J. C., Clayton, D., Concannon, P., Akolkar, B., Cooper, J. D., Erlich, H. A., et al. (2009). Genome-wide association study and meta-analysis finds over 40 loci affect risk of type 1 diabetes. Nat. Genet. 41, 703–707. doi: 10.1038/ng.381
Bergman, J. E., Davies, C., Denton, A. J., Ashman, P. E., Mittal, R., and Eshraghi, A. A. (2021). Advancements in stem cell technology and organoids for the restoration of sensorineural hearing loss. J. Am. Acad. Audiol. 32, 636–645. doi: 10.1055/s-0041-1728677
Bradfield, J. P., Qu, H.-. Q, Wang, K., Zhang, H., Sleiman, P. M., et al. (2011). A genome-wide meta-analysis of six type 1 diabetes cohorts identifies multiple associated loci. PLoS Genet. 7, e1002293. doi: 10.1371/journal.pgen.1002293
Chou, C. W., and Hsu, Y. C. (2023). Current development of patient-specific induced pluripotent stem cells harbouring mitochondrial gene mutations and their applications in the treatment of sensorineural hearing loss. Hearing Res. 429, 108689. doi: 10.1016/j.heares.2023.108689
Cooper, J. D., Walker, N. M., Smyth, D. J., Downes, K., Healy, B. C., Todd, J. A., et al. (2009). Follow-up of (1715). SNPs from the Wellcome Trust Case Control Consortium genome-wide association study in type I diabetes families. Genes Immun 10, S85–94. doi: 10.1038/gene.2009.97
Craighead, J. E., Kanich, R. E., and Kessler, J. B. (1974). Lesions of the islets of Langerhans in encephalomyocarditis virus-infected mice with diabetes mellitus-like disease. I. Acute lesions. Am. J. Pathol. 74, 287–300.
Ding, B., Walton, J. P., Zhu, X., and Frisina, R. D. (2018). Age-related changes in Na, K-ATPase expression, subunit isoform selection and assembly in the stria vascularis lateral wall of mouse cochlea. Hear. Res. 367, 59–73. doi: 10.1016/j.heares.2018.07.006
Driver, E. C., and Kelley, M. W. (2020). Development of the cochlea. Dev. Camb. Engl. 147, dev162263. doi: 10.1242/dev.162263
Duarte, G. C. K., Assmann, T. S., Dieter, C., de Souza, B. M., and Crispim, D. (2017). GLIS3 rs7020673 and rs10758593 polymorphisms interact in the susceptibility for type 1 diabetes mellitus. Acta. Diabetol. 54, 813–821. doi: 10.1007/s00592-017-1009-7
Eiberg, H., Hansen, L., Kjer, B., Hansen, T., Pedersen, O., Bille, M., et al. (2006). Autosomal dominant optic atrophy associated with hearing impairment and impaired glucose regulation caused by a missense mutation in the WFS1 gene. J. Med. Genet. 43, 435–440. doi: 10.1136/jmg.2005.034892
Eizirik, D. L., Pasquali, L., and Cnop, M. (2020). Pancreatic β-cells in type 1 and type 2 diabetes mellitus: different pathways to failure. Nat. Rev. Endocrinol. 16, 349–362. doi: 10.1038/s41574-020-0355-7
Eshraghi, A. A., Jung, H. D., and Mittal, R. (2020). Recent advancements in gene and stem cell-based treatment modalities: potential implications in noise-induced hearing loss. Anat. Rec. 303, 516–526. doi: 10.1002/ar.24107
Evangelou, M., Smyth, D. J., Fortune, M. D., Burren, O. S., Walker, N. M., Guo, H., et al. (2014). A method for gene-based pathway analysis using genomewide association study summary statistics reveals nine new type 1 diabetes associations. Genet. Epidemiol. 38, 661–670. doi: 10.1002/gepi.21853
Fukushima, H., Cureoglu, S., Schachern, P. A., Kusunoki, T., Oktay, M. F., Fukushima, N., et al. (2005). Cochlear changes in patients with type 1 diabetes mellitus. Otolaryngol. Neck Surg. 133, 100–106. doi: 10.1016/j.otohns.2005.02.004
Goto, Y., Kakizaki, M., and Masaki, N. (1976). Production of spontaneous diabetic rats by repetition of selective breeding. Tohoku J. Exp. Med. 119, 85–90. doi: 10.1620/tjem.119.85
Gratton, M. A., Smyth, B. J., Schulte, B. A., and Vincent, D. A. (1995). Na,K-ATPase activity decreases in the cochlear lateral wall of quiet-aged gerbils. Hear. Res. 83, 43–50. doi: 10.1016/0378-5955(94)00188-V
Green, A., Hede, S. M., Patterson, C. C., Wild, S. H., Imperatore, G., Roglic, G., et al. (2021). Type 1 diabetes in 2017: global estimates of incident and prevalent cases in children and adults. Diabetologia 64, 2741–2750. doi: 10.1007/s00125-021-05571-8
Helwany, M., Arbor, T. C., and Tadi, P. (2023). “Embryology, ear,” in StatPearls. Treasure Island (FL): StatPearls Publishing, (2023). Available online at: http://www.ncbi.nlm.nih.gov/books/NBK557588/ (accessed July 2, 2023).
Hibino, H., Nin, F., Tsuzuki, C., and Kurachi, Y. (2010). How is the highly positive endocochlear potential formed? The specific architecture of the stria vascularis and the roles of the ion-transport apparatus. Pflugers Arch. 459, 521–533. doi: 10.1007/s00424-009-0754-z
Horváth, M., Herold, Z., Küstel, M., Tamás, L., Prekopp, P., Somogyi, A., et al. (2023). Changes in the cochlear and retrocochlear parts of the auditory system in 19–39 and 40–60 years old patients with type 1 diabetes mellitus. PLoS ONE 18, e0285740. doi: 10.1371/journal.pone.0285740
Hoshino, T., Terunuma, T., Takai, J., Uemura, S., Nakamura, Y., Hamada, M., et al. (2019). Spiral ganglion cell degeneration-induced deafness as a consequence of reduced GATA factor activity. Genes Cells Devoted. Mol. Cell Mech. 24, 534–545. doi: 10.1111/gtc.12705
Ingrosso, D. M. F., Primavera, M., Samvelyan, S., Tagi, V. M., and Chiarelli, F. (2023). Stress and diabetes mellitus: pathogenetic mechanisms and clinical outcome. Horm. Res. Paediatr. 96, 34–43. doi: 10.1159/000522431
Kariya, S., Cureoglu, S., Fukushima, H., Morita, N., Baylan, M. Y., Maeda, Y., et al. (2010). Comparing the cochlear spiral modiolar artery in type-1 and type-2 diabetes mellitus: a human temporal bone study. Acta Med. Okayama. 64, 375–83. doi: 10.18926/AMO/41323
Kawano, K., Hirashima, T., Mori, S., Saitoh, Y., Kurosumi, M., Natori, T., et al. (1991). New inbred strain of Long-Evans Tokushima lean rats with IDDM without lymphopenia. Diabetes 40, 1375–1381. doi: 10.2337/diab.40.11.1375
Kim, M. B, Zhang, Y., Chang, Y., Ryu, S., Choi, Y., et al. (2017). Diabetes mellitus and the incidence of hearing loss: a cohort study. Int. J. Epidemiol. 46, 717–726. doi: 10.1093/ije/dyw243
Klak, M., Gomółka, M., Kowalska, P., Cicho,ń, J., Ambrozkiewicz, F., Serwańska-Swietek, M., et al. (2020). Type 1 diabetes: genes associated with disease development. Cent-Eur. J. Immunol. 45, 439–453. doi: 10.5114/ceji.2020.103386
Komeda, K., Noda, M., Terao, K., Kuzuya, N., Kanazawa, M., Kanazawa, Y., et al. (1998). Establishment of two substrains, diabetes-prone and non-diabetic, from Long-Evans Tokushima Lean (LETL) rats. Endocr. J. 45, 737–744. doi: 10.1507/endocrj.45.737
Lawrence, J. M., Divers, J., Isom, S., Saydah, S., Imperatore, G., Pihoker, C., et al. (2021). Trends in prevalence of type 1 and type 2 diabetes in children and adolescents in the US, 2001-2017. JAMA 326, 717–727. doi: 10.1001/jama.2021.11165
Lee, Y. Y., Kim, Y. J., Gil, E. S., Kim, H., Jang, J. H., and Choung, Y. H. (2020). Type 1 diabetes induces hearing loss: functional and histological findings in an akita mouse model. Biomedicines 8, 343. doi: 10.3390/biomedicines8090343
Lenzen, S., Tiedge, M., Elsner, M., Lortz, S., Weiss, H., Jörns, A., et al. (2001). The LEW.1AR1/Ztm-iddm rat: a new model of spontaneous insulin-dependent diabetes mellitus. Diabetologia 44, 1189–1196. doi: 10.1007/s001250100625
Liang, F., Hu, W., Schulte, B. A., Mao, C., Qu, C., Hazen-Martin, D. J., et al. (2004). Identification and characterization of an L-type Cav1.2 channel in spiral ligament fibrocytes of gerbil inner ear. Brain Res. Mol. Brain Res. 125, 40–46. doi: 10.1016/j.molbrainres.2004.03.003
Liang, F., Niedzielski, A., Schulte, B. A., Spicer, S. S., Hazen-Martin, D. J., Shen, Z., et al. (2003). A voltage- and Ca2+-dependent big conductance K channel in cochlear spiral ligament fibrocytes. Pflugers Arch. 445, 683–692. doi: 10.1007/s00424-002-0976-9
Like, A. A., and Rossini, A. A. (1976). Streptozotocin-induced pancreatic insulitis: new model of diabetes mellitus. Science. 193, 415–417. doi: 10.1126/science.180605
Lin, X., Xu, Y., Pan, X., Xu, J., Ding, Y., Sun, X., et al. (2020). Global, regional, and national burden and trend of diabetes in 195 countries and territories: an analysis from 1990 to 2025. Sci. Rep. 10, 14790. doi: 10.1038/s41598-020-71908-9
Liu, W., and Rask-Andersen, H. (2022). Na/K-ATPase gene expression in the human cochlea: a study using mrna in situ hybridization and super-resolution structured illumination microscopy. Front. Mol. Neurosci. 15, 857216. doi: 10.3389/fnmol.2022.857216
Liu, W., Wang, X., Wang, M., and Wang, H. (2019). Protection of spiral ganglion neurons and prevention of auditory neuropathy. Adv. Exp. Med. Biol. 1130, 93–107. doi: 10.1007/978-981-13-6123-4_6
Lukens, F. D. W. (1948). Alloxan diabetes. Physiol. Rev. 28, 304–330. doi: 10.1152/physrev.1948.28.3.304
Mahase, E. (2022). Type 1 diabetes: Global prevalence is set to double by 2040 study estimates. BMJ 378, o2289. doi: 10.1136/bmj.o2289
Makino, S., Kunimoto, K., Muraoka, Y., Mizushima, Y., Katagiri, K., Tochino, Y., et al. (1980). Breeding of a non-obese, diabetic strain of mice. Jikken Dobutsu 29, 1–13. doi: 10.1538/expanim1978.29.1_1
McKinnon, E., Morahan, G., Nolan, D., and James, I. (2009). Diabetes Genetics Consortium: Association of MHC SNP genotype with susceptibility to type 1 diabetes: a modified survival approach. Diabetes Obes. Metab. 11, 92–100. doi: 10.1111/j.1463-1326.2008.01009.x
Mittal, R., Nguyen, D., Patel, A. P., Debs, L. H., Mittal, J., Yan, D., et al. (2017). Recent advancements in the regeneration of auditory hair cells and hearing restoration. Front. Mol. Neurosci. 10, 236. doi: 10.3389/fnmol.2017.00236
Mujica-Mota, M. A., Patel, N., and Saliba, I. (2018). Hearing loss in type 1 diabetes: Are we facing another microvascular disease? A meta-analysis. Int. J. Pediatr. Otorhinolaryngol. 113, 38–45. doi: 10.1016/j.ijporl.2018.07.005
Nakhooda, A. F., Like, A. A., Chappel, C. I., Murray, F. T., and Marliss, E. B. (1977). The spontaneously diabetic Wistar rat. Metabolic and morphologic studies. Diabetes 26, 100–112. doi: 10.2337/diab.26.2.100
Onengut-Gumuscu, S., Chen, W. M., Burren, O., Cooper, N. J., Quinlan, A. R., et al. (2015). Fine mapping of type 1 diabetes susceptibility loci and evidence for colocalization of causal variants with lymphoid gene enhancers. Nat. Genet. 47, 381–386. doi: 10.1038/ng.3245
Patuzzi, R. (2011). Ion flow in stria vascularis and the production and regulation of cochlear endolymph and the endolymphatic potential. Hear. Res. 277, 4–19. doi: 10.1016/j.heares.2011.01.010
Rigoli, L., Bramanti, P., Di Bella, C., and Luca, D. (2018). F: Genetic and clinical aspects of Wolfram syndrome 1, a severe neurodegenerative disease. Pediatr. Res. 83, 921–929. doi: 10.1038/pr.2018.17
Scheper, V., Schwieger, J., Hamm, A., Lenarz, T., and Hoffmann, A. (2019). BDNF-overexpressing human mesenchymal stem cells mediate increased neuronal protection in vitro. J. Neurosci. Res. 97, 1414–1429. doi: 10.1002/jnr.24488
Singh, R., Farooq, S. A., Mannan, A., Singh, T. G., Najda, A., Grazyna, Z., et al. (2022). Animal models of diabetic microvascular complications: Relevance to clinical features. Biomed. Pharmacother. 145, 112305. doi: 10.1016/j.biopha.2021.112305
Stephenson, R., Mangasarian, A., Ishiyama, G., Hosokawa, K., Hosokawa, S., Ishiyama, A., et al. (2021). Immunohistochemical location of Na+, K+-ATPase α1 subunit in the human inner ear. Hear. Res. 400, 108113. doi: 10.1016/j.heares.2020.108113
Sun, S., Siebald, C., and Müller, U. (2021). Subtype maturation of spiral ganglion neurons. Curr. Opin. Otolaryngol. Head Neck Surg. 29, 391–399. doi: 10.1097/MOO.0000000000000748
Tadros, S. F., D'Souza, M., Zhu, X., and Frisina, R. D. (2008). Apoptosis-related genes change their expression with age and hearing loss in the mouse cochlea. Apoptosis 13, 1303–1321. doi: 10.1007/s10495-008-0266-x
Todd, J. A., Walker, N. M., Cooper, J. D., Smyth, D. J., Downes, K., Plagnol, V., et al. (2007). Robust associations of four new chromosome regions from genome-wide analyses of type 1 diabetes. Nat. Genet. 39, 857–864. doi: 10.1038/ng2068
Törn, C., Hadley, D., Lee, H. S., Hagopian, W., Lernmark, Å., et al. (2015). Role of type 1 diabetes-associated SNPs on risk of autoantibody positivity in the TEDDY study. Diabetes 64, 1818–1829. doi: 10.2337/db14-1497
White, H. J., Helwany, M., Biknevicius, A. R., and Peterson, D. C. (2023). “Anatomy, head and neck, ear organ of corti,” in StatPearls. Treasure Island (FL): StatPearls Publishing. Available online at: http://www.ncbi.nlm.nih.gov/books/NBK538335/ (accessed July 2, 2023).
Wu, R., Burnside, M., Davies, H., Jefferies, C., Wheeler, B., Paul, R., et al. (2023). Prevalence and incidence of type 1 diabetes in children aged 0-14 years old in New Zealand in (2021). J. Paediatr. Child Health 59, 519–525. doi: 10.1111/jpc.16342
Yagihashi, S. (2023). Contribution of animal models to diabetes research: its history, significance, and translation to humans. J. Diabetes Investig. 14, 1015–1037. doi: 10.1111/jdi.14034
Yoshioka, M., Kayo, T., Ikeda, T., and Koizumi, A. (1997). A novel locus, Mody4, distal to D7Mit189 on chromosome 7 determines early-onset NIDDM in nonobese C57BL/6 (Akita) mutant mice. Diabetes 46, 887–894. doi: 10.2337/diab.46.5.887
Zhang, L., Chen, S., and Sun, Y. (2022). Mechanism and prevention of spiral ganglion neuron degeneration in the cochlea. Front Cell Neurosci. 15, 814891. doi: 10.3389/fncel.2021.814891
Keywords: sensorineural hearing loss, type 1 diabetes, sensory cells, inner ear, pathological mechanisms
Citation: Mittal R, Camick N, Lemos JRN, Lanzoni G, Fraker CA and Hirani K (2023) Hearing loss in type 1 diabetes mellitus: a need to understand precise pathological mechanisms and develop novel therapeutic approaches. Front. Audiol. Otol. 1:1279818. doi: 10.3389/fauot.2023.1279818
Received: 18 August 2023; Accepted: 11 September 2023;
Published: 29 September 2023.
Edited by:
A. Eliot Shearer, Harvard Medical School, United StatesReviewed by:
Kazuaki Homma, Northwestern University, United StatesCopyright © 2023 Mittal, Camick, Lemos, Lanzoni, Fraker and Hirani. This is an open-access article distributed under the terms of the Creative Commons Attribution License (CC BY). The use, distribution or reproduction in other forums is permitted, provided the original author(s) and the copyright owner(s) are credited and that the original publication in this journal is cited, in accordance with accepted academic practice. No use, distribution or reproduction is permitted which does not comply with these terms.
*Correspondence: Rahul Mittal, ci5taXR0YWwxMUBtZWQubWlhbWkuZWR1; Khemraj Hirani, a2hpcmFuaUBtZWQubWlhbWkuZWR1
†These authors have contributed equally to this work
Disclaimer: All claims expressed in this article are solely those of the authors and do not necessarily represent those of their affiliated organizations, or those of the publisher, the editors and the reviewers. Any product that may be evaluated in this article or claim that may be made by its manufacturer is not guaranteed or endorsed by the publisher.
Research integrity at Frontiers
Learn more about the work of our research integrity team to safeguard the quality of each article we publish.