- Center for Hearing Research, Departments of Anatomy and Neurobiology, Biomedical Engineering, Cognitive Sciences and Otolaryngology–Head and Neck Surgery, University of California, Irvine, Irvine, CA, United States
Tinnitus is an auditory sensation in the absence of external sounds; its neural mechanisms remain unclear. An active loudness model suggests that tinnitus is a result of increased central noise while hyperacusis is that of increased central gain. To the extent that loudness reflects the system-level neural activities, this active model predicts that tinnitus increases loudness at thresholds but does not increase the slope of loudness function. To test this prediction, the present study compared loudness growth of various stimuli between tinnitus and non-tinnitus subjects. The stimuli were tested at frequencies without hearing loss and both tinnitus and non-tinnitus subjects had unremarkable hyperacusis. Consistent with the prediction, the loudness at threshold in tinnitus subjects were 2.3 times greater than that in non-tinnitus subjects; there was no significant difference in the slope of loudness growth between these two groups of subjects. The present result shows that traditional psychophysics, originally developed to investigate relationships between subjective sensation and physical stimulation, can also help delineate neural mechanisms underlying tinnitus and other disorders.
1. Introduction
Psychophysics studies the relationship between physical stimuli and their evoked sensations. In contrast, tinnitus is an auditory percept in the absence of external sounds. It is not surprising that we face a conceptual dilemma in studying the tinnitus sensation directly. This dilemma is best exemplified by the inconsistent subjective estimate of tinnitus, which can be unusually loud, but often matched to a soft external sound that is only 10–20 dB above the sound threshold (e.g., Moore, 2012). Alternatively, we can study the effect of tinnitus on perception of external sounds indirectly. This alternative approach would have the same psychophysical foundation while allowing examination of neural mechanisms underlying tinnitus.
An active loudness model took such an approach to decompose loudness growth into an additive noise source that determines loudness at thresholds and a nonlinear gain that determines the slope of loudness growth (Zeng, 2013). The active loudness model allows psychophysical investigation into the neural mechanisms underlying tinnitus and other related disorders. For example, if tinnitus increases the central noise, then one would predict increased loudness at the threshold. While previous studies found greater than normal loudness at the threshold in individuals with hearing loss (Buus and Florentine, 2002), no studies measured loudness at the sound threshold in tinnitus subjects. Also note that it is routine to use an external sound to match tinnitus loudness or measure the upper loudness level (e.g., Tyler and Conrad-Armes, 1983; Vernon and Meikle, 2003; Gu et al., 2010; Zeng et al., 2020), but few studies obtained the entire loudness function and none explicitly measured loudness at thresholds (Penner, 1986; Hebert et al., 2013; Erinc and Derinsu, 2022). On the other hand, if tinnitus increases the central gain, then one would predict a steepened loudness growth function. Although previous studies found steepened loudness growth in tinnitus subjects, these subjects had either hearing loss or hyperacusis that might confound the result (Penner, 1986; Hebert et al., 2013).
Conceptually, both the central gain and noise are well defined. First, the gain is the slope of an input-output function, which can be measured in terms of the relative change in evoked neural activities or behavioral outcomes such as loudness and reaction time in response to a given range of input. The increased central gain model is based on the observation that the peripheral output is reduced but the central output restored or even enhanced in response to apparent or hidden hearing loss (e.g., Norena, 2011; Schaette and McAlpine, 2011; Auerbach et al., 2014; Sedley, 2019). Second, the increased central noise may manifest itself in terms of both spontaneous and evoked activities. Similar to the central gain model, hearing loss reduces the spontaneous activity in the auditory nerve (Liberman and Kiang, 1978) but increases it in the central pathway (Kaltenbach and Afman, 2000). Different from the central gain model, the central noise may not change the gain in terms of the average spike rate but affect the evoked response in terms of impaired temporal coding, e.g., reduced phase locking to an external stimulus, bursting or oscillation activities independent of the external stimulation (e.g., Norena and Eggermont, 2003; Chambers et al., 2016; Knipper et al., 2020).
Here we used absolute magnitude estimation to measure systematically loudness growth in tinnitus and control subjects. Our hypothesis was that, compared with the control, tinnitus would increase loudness at the threshold but not the slope of loudness growth. The present study minimized the potential hearing loss confounding factor by collecting all data at frequencies with normal hearing. The study also controlled the hyperacusis factor by selecting tinnitus subjects with unremarkable hyperacusis.
2. Methods
2.1. Participants
A total of 20 human listeners (female = 11) participated in the study. Nine served as a control, which had a mean age of 29 (±13 s.d.) years. Eleven had chronic tonal tinnitus (>6 months), with a mean tinnitus function index score of 49 (±28 s.d.). Table 1 provides 11 tinnitus individuals' demographics, tinnitus and hyperacusis characteristics. Although the tinnitus group had a significant older mean age of 50 (±17 s.d.) years than the control (two-tailed t-test, degree of freedom or df = 18, p = 0.005), there was no difference in thresholds at tested frequencies between the control and tinnitus groups (11 ± 3 vs. 12 ± 5 dB HL; two-tailed t-test, df = 18, p = 0.30). Note that one of the nine control and four of the 11 tinnitus subjects (T2, T5, T6 and T10 in Table 1) had >20 dB HL hearing loss at some non-tested audiometric frequencies, but the hearing loss at these non-tested frequencies should not affect loudness growth at the tested frequencies (e.g., Zeng and Turner, 1991). Also note that neither the control nor the tinnitus group had remarkable hyperacusis: KHQ mean ± s.d. = 10 ± 9 vs. 16 ± 9; two-tailed t-test, df = 17, p = 0.13, because the mean of both groups, as well as the highest score of 25 in the tinnitus group (Table 1), was lower than the recommended 28 cutoff score for hyperacusis (Khalfa et al., 2002). Subject T11 did not complete the KHQ questionnaire but was unlikely to have remarkable hyperacusis because T11 didn't self-report any hyperacusis and estimated loudness of a 90-dB SPL, 1000-Hz tone as 8 (within normal range, see Figure 1). The University of California Irvine Institutional Research Board approved the experimental protocol in the present study.
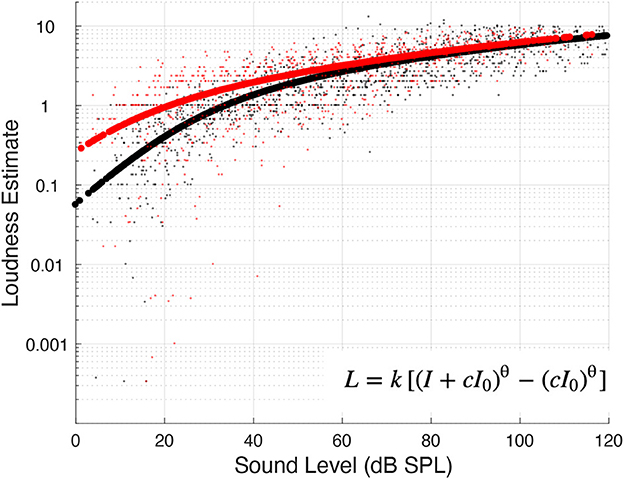
Figure 1. Loudness estimates and functions. Small black dots represent individual data from the control subjects, whereas small red dots from the tinnitus subjects. The thick black and red lines represent the fitted loudness function (see insert) for the control and tinnitus groups, respectively.
2.2. Stimuli
A total of 14 different stimuli were used in the study. Twelve stimuli varied on three dimensions: frequency (250, 1,000, or 4,000 Hz), tone vs. 1/2-octave band-pass noise, and modulated (100%, 41-Hz sinusoidal amplitude modulation) vs. unmodulated. In addition, modulated and unmodulated white noise was tested. All stimuli were 300 ms in duration including 20-ms cosine-squared onset and offset ramps. The stimuli were presented in the better ear via a sound card (Creative Labs EMU 0404, 24-bit, 96-kHz USB digital audio system, Creative Technology Ltd., Singapore), through circumaural headphones (Sennheiser HAD-200, Wedemark, Germany).
2.3. Procedures
Sat in a double-walled, sound-attenuated booth, all subjects performed the experiment using an absolute magnitude estimation procedure (Hellman and Zwislocki, 1963; Zwislocki and Goodman, 1980). First, the threshold and upper loudness level was measured for each stimulus in each subject. The threshold was obtained using a three-interval, two-down-one-up, forced-choice procedure estimating 70.7% correct detection. The upper level was determined using an ascending method of limits by increasing the stimulus level in 5-dB steps from the threshold until either the subject reported the sound being the loudest tolerable or the maximum level of 120 dB SPL was reached. Second, a series of stimuli with different levels was generated from the threshold to a level closest to the upper level or 120 dB SPL. This series of stimuli was presented to the subject in a random order, not only allowing a second opportunity to eliminate any loud sounds that might cause discomfort, but also minimizing potential attentional interaction between the stimuli and tinnitus (Zeng et al., 2020). Third, in each run of formal data collection, the stimuli with different levels were presented in a random order to the subject, who had to assign a number (whole numbers, decimals, or fractions) to match each stimulus level. The subject was also given specific instructions: “Don't worry about running out of numbers—there will always be a smaller number than the smallest you use and a larger one than the largest you use. Do not worry about numbers you assigned to previous stimuli.” Three such runs were completed for each stimulus, with the average of the three runs being used as the loudness estimate for each stimulus, at each level.
2.4. Analysis
Due to time and funding restrictions during the Covid-19 pandemic, the control subjects completed 82% of possible stimulus conditions (103/126), while the tinnitus subjects, due to additional hearing loss at some tested frequencies, completed 51% of conditions (78/154). This limited data collection prevented meaningful analysis of subject-stimulus interactions.
Loudness (L) was fitted as a power function of sound intensity (I in linear units) using the following formula (Zwislocki, 1965; Eq. 212):
where I0 is the detection threshold (also in linear units), q is the slope, while c and k are two scaling constants. The loudness at threshold (I0) and the slope were the two parameters of interest in the final analysis.
3. Results
Figure 1 shows all individual data points from the control (black dots; n = 103) and tinnitus (red dots; n = 78) groups, as well as the fitted power function to each group (thick black line = control; thick red line = tinnitus). The fitted function found that at the threshold, the control group produced a loudness estimate of 0.26, whereas the tinnitus group produced 2.4 times greater loudness of 0.62. The slope of loudness function was similar between the two groups (0.09 for the control vs. 0.08 for the tinnitus).
The same power function was fitted to each stimulus on an individual basis. Figure 2A shows the distribution of individually predicted loudness at thresholds (circles = individual data, bars = mean + std) between the tinnitus (left bar with orange circles; n = 78) and control (right bar with blue circles; n = 103) groups. On average, the loudness at thresholds was 2.3 times greater in the tinnitus than the control group (0.77 ± 0.72 vs. 0.34 ± 0.24; two-tailed t-test, df = 179, p = 0.002). In contrast, there was no significant difference in the slope of loudness function between the tinnitus and control groups (Figure 2B; 0.20 ± 0.11 vs. 0.23 ± 0.11; two-tailed t-test, df = 179, p = 0.08).
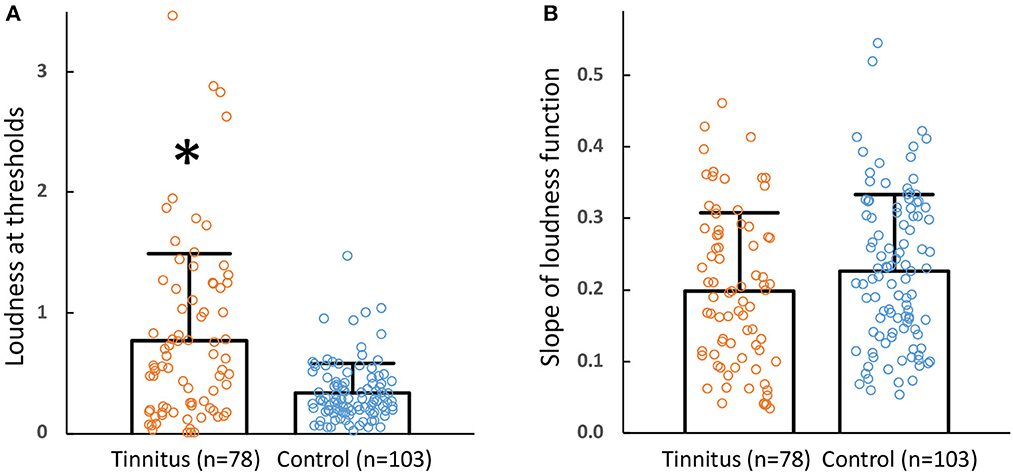
Figure 2. (A) Loudness at thresholds. Loudness at thresholds between tinnitus (left bar, mean; error bar, one standard deviation; orange circles, individual data) and control subjects (right bar, mean; error bar, one standard deviation; blue circles, individual data). The * symbol represents a significant difference between the two groups. (B) Slope of loudness functions. The format is the same as (A).
4. Discussion
Following a classic absolute magnitude estimation technique, the present study found that the average loudness at the threshold was 2.3 times greater in 11 tinnitus subjects than in nine control subjects, while the average slope of their loudness functions was not significantly different. It is worthwhile to note several contexts surrounding the present study. First, the present result was obtained under normal audiometric thresholds and with unremarkable hyperacusis in both tinnitus and control subjects. Second, the present study did not control for age, nor did it complete data collection for all stimuli in all subjects. Third, the small sample size (11 tinnitus and nine control subjects) limits the generalization of the present results.
The present study can be compared to previous studies. Penner (1986) used a similar magnitude estimation technique to measure loudness growth at 1 kHz and tinnitus frequency (3.5–8.0 kHz) in 10 tinnitus subjects. She found that the slope of loudness function was much greater at the tinnitus frequency than the 1 kHz (0.74 vs. 0.28), but this difference was due to hearing loss at the tinnitus frequency because eight of her 10 subjects had hearing loss (25–69 dB SPL) while the remaining two without hearing loss had similar slope (0.20 and 0.36, see her Table 1). Hebert et al. (2013) used a scaling method to measure loudness growth at 1 and 4 kHz in 63 tinnitus and 53 control subjects. Controlling for hearing loss, they found that the tinnitus subjects had steeper than normal slope at both frequencies. Because the greater slope was a result of increased sensitivity to loud sounds and 60% of them had a hyperacusis score >10, they interpreted the steeper slope as an increased central gain. While hyperacusis and tinnitus are co-morbid, Erinc and Derinsu (2022) were able to find 20 subjects with mostly hyperacusis and additionally 20 with mostly tinnitus. They also used the scaling method to measure loudness growth at 0.5 and 2 kHz and found that only the hypercusis subjects had increased sensitivity. The present result is consistent with the Erinc and Derinsu finding that tinnitus does not increase sound sensitivity after controlling for hearing loss and hyperacusis.
To the extent that loudness reflects system-level neural processes, the present result supports the hypothesis that tinnitus increases the central noise but not the central gain (Zeng, 2013). The previously observed steeper than normal slope of loudness growth in tinnitus subjects reflects more likely the co-morbid hyperacusis (Penner, 1986; Hebert et al., 2013), which is a result of the increased central gain (Norena, 2011; Schaette and McAlpine, 2011).
Although the present study favors the central noise model in tinnitus, it remains to be worked out what central sources are involved and how they influence the central noise or gain in response to peripheral changes and their eventual system-level outcomes (e.g., Li et al., 2013; Wang et al., 2023). A potential means of separating the central noise and gain contributions is to examine the central variance, which should grow linearly with the additive central noise but nonlinearly with the multiplicative central gain (Zeng, 2020a). Psychophysically, the difference limen in intensity may serve as an indirect measure of the central variance (Zeng, 2020b).
5. Conclusions
The present study found that compared with the normal control, tinnitus subjects had increased loudness at the threshold, but unchanged slope of the loudness growth. This finding supports the increased central noise hypothesis in tinnitus. The strength of the evidence is limited by the uneven age, incomplete dataset and small sample size in the present study.
Data availability statement
The raw data supporting the conclusions of this article will be made available by the authors, without undue reservation.
Ethics statement
The studies involving humans were approved by University of California Irvine Institutional Research Board. The studies were conducted in accordance with the local legislation and institutional requirements. Written informed consent for participation in this study was provided by the participants' legal guardians/next of kin.
Author contributions
KT: Investigation, Data curation, Formal analysis, Methodology, Software, Writing—review and editing. F-GZ: Investigation, Conceptualization, Funding acquisition, Project administration, Supervision, Writing—original draft.
Funding
The author(s) declare financial support was received for the research, authorship, and/or publication of this article. This work was partially supported by NIH (R01-DC015587).
Acknowledgments
We thank our subjects who participated in the present study.
Conflict of interest
F-GZ owns stock in Axonics, DiaNavi, Neocortix, Nurotron, Syntiant, Velox, and Xense.
The remaining author declares that the research was conducted in the absence of any commercial or financial relationships that could be construed as a potential conflict of interest.
The author(s) F-GZ declared that they were an editorial board member of Frontiers, at the time of submission. This had no impact on the peer review process and the final decision.
Publisher's note
All claims expressed in this article are solely those of the authors and do not necessarily represent those of their affiliated organizations, or those of the publisher, the editors and the reviewers. Any product that may be evaluated in this article, or claim that may be made by its manufacturer, is not guaranteed or endorsed by the publisher.
References
Auerbach, B. D., Rodrigues, P. V., and Salvi, R. J. (2014). Central gain control in tinnitus and hyperacusis. Front. Neurol. 5, 206. doi: 10.3389/fneur.2014.00206
Buus, S., and Florentine, M. (2002). Growth of loudness in listeners with cochlear hearing losses: recruitment reconsidered. J. Assoc. Res. Otolaryngol. 3, 120–139. doi: 10.1007/s101620010084
Chambers, A. R., Resnik, J., Yuan, Y., Whitton, J. P., Edge, A. S., Liberman, M. C., et al. (2016). Central gain restores auditory processing following near-complete cochlear denervation. Neuron 89, 867–879. doi: 10.1016/j.neuron.2015.12.041
Erinc, M., and Derinsu, U. (2022). Behavioural and electrophysiological evaluation of loudness growth in clinically normal hearing tinnitus patients with and without hyperacusis. Audiol. Neurootol. 27, 469–477. doi: 10.1159/000525692
Gu, J. W., Halpin, C. F., Nam, E. C., Levine, R. A., and Melcher, J. R. (2010). Tinnitus, diminished sound-level tolerance, and elevated auditory activity in humans with clinically normal hearing sensitivity. J. Neurophysiol. 104, 3361–3370. doi: 10.1152/jn.00226.2010
Hebert, S., Fournier, P., and Norena, A. (2013). The auditory sensitivity is increased in tinnitus ears. J. Neurosci. 33, 2356–2364. doi: 10.1523/JNEUROSCI.3461-12.2013
Hellman, R. P., and Zwislocki, J. (1963). Monaural loudness function at 1000 Cps and interaural summation. J. Acoust. Soc. Am. 35, 856. doi: 10.1121/1.1918619
Kaltenbach, J. A., and Afman, C. E. (2000). Hyperactivity in the dorsal cochlear nucleus after intense sound exposure and its resemblance to tone-evoked activity: a physiological model for tinnitus. Hear. Res. 140, 165–172. doi: 10.1016/S0378-5955(99)00197-5
Khalfa, S., Dubal, S., Veuillet, E., Perez-Diaz, F., Jouvent, R., and Collet, L. (2002). Psychometric normalization of a hyperacusis questionnaire. ORL J. Otorhinolaryngol. Relat. Spec. 64, 436–442. doi: 10.1159/000067570
Knipper, M., van Dijk, P., Schulze, H., Mazurek, B., Krauss, P., Scheper, V., et al. (2020). The neural bases of tinnitus: lessons from deafness and cochlear implants. J. Neurosci. 40, 7190–7202. doi: 10.1523/JNEUROSCI.1314-19.2020
Li, Y. T., Ibrahim, L. A., Liu, B. H., Zhang, L. I., and Tao, H. W. (2013). Linear transformation of thalamocortical input by intracortical excitation. Nat. Neurosci. 16, 1324–1330. doi: 10.1038/nn.3494
Liberman, M. C., and Kiang, N. Y. (1978). Acoustic trauma in cats. Cochlear pathology and auditory-nerve activity. Acta. Otolaryngol. 358, 1–63. doi: 10.1121/1.381736
Moore, B. C. J. (2012). “The psychophysics of tinnitus” in Tinnitus: Springer Handbook of Auditory Research, Eds. J.J. Eggermont, F.G. Zeng, A.R. Popper and R.R. Fay (New York, NY: Springer), 187–216.
Norena, A. J. (2011). An integrative model of tinnitus based on a central gain controlling neural sensitivity. Neurosci. Biobehav. Rev. 35, 1089–1109. doi: 10.1016/j.neubiorev.2010.11.003
Norena, A. J., and Eggermont, J. J. (2003). Changes in spontaneous neural activity immediately after an acoustic trauma: implications for neural correlates of tinnitus. Hear. Res. 183, 137–153. doi: 10.1016/S0378-5955(03)00225-9
Penner, M. J. (1986). Magnitude estimation and the “paradoxical” loudness of tinnitus. J. Speech. Hear. Res. 29, 407–412. doi: 10.1044/jshr.2903.407
Schaette, R., and McAlpine, D. (2011). Tinnitus with a normal audiogram: physiological evidence for hidden hearing loss and computational model. J. Neurosci. 31, 13452–13457. doi: 10.1523/JNEUROSCI.2156-11.2011
Sedley, W. (2019). Tinnitus: does gain explain? Neuroscience 407, 213–228. doi: 10.1016/j.neuroscience.2019.01.027
Tyler, R. S., and Conrad-Armes, D. (1983). The determination of tinnitus loudness considering the effects of recruitment. J. Speech. Hear. Res. 26, 59–72. doi: 10.1044/jshr.2601.59
Vernon, J. A., and Meikle, M. B. (2003). Tinnitus: clinical measurement. Otolaryngol. Clin. North. Am. 36, 293–305, vi. doi: 10.1016/S0030-6665(02)00162-7
Wang, X., Zhang, Y., Zhu, L., Bai, S., Li, R., Sun, H., et al. (2023). Selective corticofugal modulation on sound processing in auditory thalamus of awake marmosets. Cereb. Cortex. 33, 3372–3386. doi: 10.1093/cercor/bhac278
Zeng, F. G. (2013). An active loudness model suggesting tinnitus as increased central noise and hyperacusis as increased nonlinear gain. Hear. Res. 295, 172–179. doi: 10.1016/j.heares.2012.05.009
Zeng, F. G. (2020a). Tinnitus and hyperacusis: central noise, gain and variance. Curr. Opin. Physiol. 18, 123–129. doi: 10.1016/j.cophys.2020.10.009
Zeng, F. G. (2020b). A unified theory of psychophysical laws in auditory intensity perception. Front. Psychol. 11, 1459. doi: 10.3389/fpsyg.2020.01459
Zeng, F. G., Richardson, M., and Turner, K. (2020). Tinnitus does not interfere with auditory and speech perception. J. Neurosci. 40, 6007–6017. doi: 10.1523/JNEUROSCI.0396-20.2020
Zeng, F. G., and Turner, C. W. (1991). Binaural loudness matches in unilaterally impaired listeners. Q. J. Exp. Psychol. A 43, 565–583. doi: 10.1080/14640749108400987
Zwislocki, J. (1965). “Analysis of some auditory characteristics” in Handbook of Mathematical Psychology. Eds. R. Luce, R. R. Bush, and E. Galanter (New York, NY: John Wiley and Sons, Inc.), 79–97.
Keywords: tinnitus, hyperacusis, hearing loss, loudness, threshold, spontaneous activity, central noise, central gain
Citation: Zeng F-G and Turner KE (2023) Tinnitus as central noise revealed by increased loudness at thresholds. Front. Audiol. Otol. 1:1272880. doi: 10.3389/fauot.2023.1272880
Received: 04 August 2023; Accepted: 27 September 2023;
Published: 19 October 2023.
Edited by:
Jae-Jin Song, Seoul National University Bundang Hospital, Republic of KoreaReviewed by:
Philip Julian Sanders, The University of Auckland, New ZealandPrashanth Prabhu, All India Institute of Speech and Hearing (AIISH), India
Copyright © 2023 Zeng and Turner. This is an open-access article distributed under the terms of the Creative Commons Attribution License (CC BY). The use, distribution or reproduction in other forums is permitted, provided the original author(s) and the copyright owner(s) are credited and that the original publication in this journal is cited, in accordance with accepted academic practice. No use, distribution or reproduction is permitted which does not comply with these terms.
*Correspondence: Fan-Gang Zeng, ZnplbmdAdWNpLmVkdQ==