- 1Institute of Aquaculture, Nha Trang University, Nha Trang, Vietnam
- 2Department of Biological Sciences, University of Bergen, Bergen, Norway
- 3Institute of Marine Research (IMR), Bergen, Norway
- 4SPAROS Lda, Olhão, Portugal
- 5Instituto de Ciencias Marinas de Andalucía (ICMAN-CSIC), Puerto Real, Spain
- 6Centre of Marine Sciences of Algarve (CCMAR/CIMAR LA), University of Algarve, Faro, Portugal
Introduction: This study explored the effects of increased water temperatures and limited feeding on the growth, feed utilization, and nutrient retention in juvenile cobia (Rachycentron canadum).
Methods: Juvenile cobia, approximately 5.0 g in body weight, were distributed across two temperature conditions: 34°C, to mimic a global warming scenario, and 30°C, the current local summer average, as controls. The setup included eighteen 200-liter tanks, with 20 fish per tank. Within each temperature regime, triplicate tanks received one of three feeding levels (55%, 75%, and 95% of satiation as determined at 30°C) over a six-week period.
Results: The findings indicated that cobia exhibited the poorest growth performance at the elevated temperature (34°C) and the lowest feeding level (55%). These conditions also correlated with the lowest protein efficiency ratio and feed intake. The feed conversion ratio worsened with increased temperature and feeding levels, while protein production values decreased at higher temperatures. A lower feeding level caused a lower total lipid retention but led to increased retention of whole body essential amino acids.
Discussion: After six weeks, a 24-hour post-prandial analysis showed selective retention of some amino acids in muscle and plasma, but significantly higher retention in the liver at the higher temperature. Only a few amino acids' retention was influenced by feeding level. These results suggest that temperature and feeding levels alter the prioritization of amino acid metabolism and retention, as well as the utilization of energy and substrates across different organs of the cobia.
1 Introduction
The 2021 report by the Intergovernmental Panel on Climate Change (IPCC) forecasts a substantial increase in global temperatures, with estimates indicating a rise of 4 to 6°C by 2100 (IPCC, 2021). This warming trend poses a significant threat to Vietnam, a nation highly susceptible to the effects of climate change. In marine ecosystems, fish species, being ectotherms, exhibit varied sensitivities to temperature changes, which impact their survival and growth depending on their adaptability and inherent vulnerabilities. The cobia (Rachycentron canadum), an important species in Vietnam’s marine aquaculture industry, is prized for its excellent meat quality, rapid growth rate, and disease resistance. Known for its adaptability to diverse farming conditions, cobia is considered a promising candidate for global marine aquaculture expansion (Benetti et al., 2021).
The economic viability of cobia farming hinges on growth performance metrics such as weight gain and feed conversion ratio. Monitoring nitrogenous metabolites, like ammonia and urea, is crucial for assessing the metabolic health of cobia and the environmental impact of aquaculture practices (Fraser and Davies, 2009). Understanding the effects of climate change on cobia is vital due to the significant socio-economic implications. Ensuring high lean growth and minimal triacylglyceride accumulation while reducing nitrogen discharge into the environment is a key production challenge for cobia, which could be exacerbated by rising sea temperatures beyond the species’ optimal range. The impact of increased temperatures on marine species is a growing concern, especially under current global climate change trajectories. Temperature fluctuations can significantly affect the physiological processes of marine fish, influencing their growth rates, metabolic activities, and overall health (Volkoff and Rønnestad, 2020; Alfonso et al., 2021; Islam et al., 2022).
For cobia, studies have shown that elevated water temperatures can alter metabolic rates, potentially affecting growth performance, nitrogen utilization efficiency (Sun et al., 2006a; Sun and Chen, 2009; Nguyen et al., 2019; Yúfera et al., 2019), and feed intake and appetite-related neuropeptide expression in the brain (Nguyen et al., 2023). Feed availability is essential for effective feed management and fish welfare in aquaculture, as it allocates energy for growth and helps fish cope with thermal stress under climate change conditions. Proper feeding control is critical for optimizing growth rates and coping with thermal stress, particularly in the context of climate change. Reports on global warming have highlighted declines in catch composition and changes in the distribution of marine fish stocks, impacting food availability from both wild and aquaculture sources. Maintaining appropriate feeding levels is vital for optimizing body composition, including fillet quality, and ensuring economic efficiency. In cobia, inadequate food levels can significantly impact energy allocation, potentially limiting its ability to cope with thermal stress and affecting growth rates and basic maintenance (Costa-Bomfim et al., 2014). The quality and quantity of feed directly impact growth performance and influence water quality and the accumulation of nitrogenous wastes, such as ammonia and nitrate, in aquaculture systems (Munguti et al., 2020; Ramli et al., 2020).
Studies on the effects of feed availability on growth performance and feed utilization efficiency have been conducted on various tropical fish species, including juvenile grouper (Epinephelus coioides) (Luo et al., 2006), camouflage grouper (Epinephelus polyphekadion) (Al Zahrani et al., 2013), tilapia (Oreochromis niloticus) (Daudpota et al., 2016), and cobia (Sun et al., 2006b, 2006c). Understanding how cobia responds and adjusts its metabolism and physiology to elevated temperatures is crucial for optimizing daily feeding rations in future climate change scenarios. Elevated temperatures and feed availability significantly influence fish growth performance, feed utilization, body composition, and bioenergetics (Volkoff and Rønnestad, 2020). The effects of elevated temperatures beyond the optimal range, feeding availability, and feed intake on growth and bioenergetics have been studied in both freshwater and marine fish species (Sun and Chen, 2009; Imanpoor and Far, 2013; Mizanur et al., 2014). Periods of elevated temperatures have been shown to reduce growth and feed utilization in juveniles of turbot (Scophthalmus maximus) (Burel et al., 1996), spinefoot rabbitfish (Siganus rivulatus) (Saoud et al., 2008), and cobia (Sun et al., 2006a; Sun and Chen, 2009; 2014; Nguyen et al., 2019; Le et al., 2020). Additionally, elevated temperatures (34°C) have been reported to induce higher proteolitic activity and faster gut transit, but decreased digestion efficiency (Yúfera et al., 2019).
Several studies investigating the effects of elevated temperatures and feeding levels on cobia juveniles concluded that growth performance, feed conversion efficiency, fecal production, nitrogenous excretion, and energy budget showed increasing trends with higher feeding levels and temperatures within the range of 23 to 35°C (Sun et al., 2006a, 2006b, 2006c; Sun and Chen, 2009; 2014). Despite significant research on the digestive physiology of cobia, there has been little attention to amino acid and nitrogenous metabolite retention and their correspondence with growth performance and feed utilization.
Therefore, this study aims to investigate the effects of feed availability on the growth performance and nitrogenous metabolite production of cobia juveniles at elevated water temperatures. By examining how different feeding regimes interact with temperature stress, we seek to provide insights into optimizing cobia aquaculture for sustainability and resilience against climate variability. Additionally, changes at the biochemical level, particularly in the proximate body composition and free amino acids in plasma, muscle, and liver, were explored. The results will provide key information on how climate change may interact with other stressors to alter fish physiology, impacting aquaculture production and threatening immediate and future food security locally and globally (Pankhurst and Munday, 2011; Froehlich et al., 2018).
2 Materials and methods
2.1 Ethics
The studies involving animals were reviewed and approved by the Ethics Committee for Animal Research at the Institute of Aquaculture, Nha Trang University, Vietnam.
2.2 Fish feed preparation
The experimental feed was extruded at SPAROS Lda (Olhão, Portugal) with 46.5% protein and 10.3% lipid The ingredients and proximate composition of the diet (Table 1) were based on our previous studies on juvenile cobia (Nguyen et al., 2019; Nguyen et al., 2023). The dietary amino acid concentration is presented in Table 2.
2.3 Fish experiment
Juvenile cobias (1,200 juveniles with a mean body weight of 4.0 g) were purchased from a local hatchery in Nha Trang city, Vietnam, and transported to Center for Aquatic Animal Health and Breeding Studies (CAAHBS), Nha Trang University (NTU). Fish were acclimatized in two indoor fiberglass circular tanks (5 m3) with a density of 600 individuals per tank for one week. The fish were fed ad libitum by hand at 8:00 and 16:00 with a commercial diet (INVE, Ltd.) during the acclimatization period. The water temperature in one tank was increased at a rate of 1°C per day up to 34°C, while water temperature in the second tank was kept at 30°C.
2.4 Experimental design
From each acclimation tank, 180 fish were randomly distributed into 9 experimental tanks for each temperature (20 fish per tank and 18 tanks for two temperatures). There were 6 experimental treatments of 2 temperatures (30 or 34°C) and 3 feeding levels (55, 75 and 95% of satiation). The water temperature in each set of nine tanks was maintained at 30 ± 0.1°C and 34 ± 0.1°C, considered as control and elevated temperature treatments by thermal controllers (Chuan Kang Ltd., Taiwan), respectively. Temperatures of 30 and 34°C reflect the control and warming temperatures in the aquaculture ponds during the spring (Doan et al., 2018), which is also the rearing period for cobia. The experimental tanks were rectangular fiberglass tanks (80×50×60 cm), with 200L water, in a recirculating aquaculture system (RAS) with continuous aeration. Each experimental treatment was randomly assigned to three tanks. In each system, the input water from a two-chamber organic filtered fiberglass tank (1×1×2 m) flowed through plastic pipes to the experimental tanks (0.1 L/s). The output water from the experimental tanks was collected via perpendicular pipes (Ø 27 mm) in the middle of each tank, filtered for solids, passed throughout a biological filter set in a fiberglass tank (1×1×2 m) and pumped into the reservoir chamber of the RAS. A salinity of 29 ± 3.1 ppt, pH 7.8–8.3, oxygen 5.6 ± 0.5 mg/l and NH3 were maintained lower than 0.03 mg/l in the two experimental systems during the experimental period. The seawater in the RAS was renewed every 2–3 days depending on water quality analyses.
Cobia are very voracious feeders and may ingest more feed than that required for metabolism and growth, resulting in low-cost effectiveness. Therefore, in this study the cobia were fed with three feeding rations (feeding levels), including 95%, 75% and 55% of satiety for a 6-week period. The 100% satiety levels were based on our previous studies of the species in similar conditions (Nguyen et al., 2019, 2023). The uneaten feed was collected daily and dried at 105°C in oven for the calculation of feed intake (FI) and feed conversion ratio (FCR).
2.5 Sampling procedure
The body weight and total length of the cobia juveniles were individually measured at the beginning and the end of the experiment. The fish were anesthetized with monophenyl ether glycol solution at the concentration of 150 – 200 ppm before any handling. A pooled sample of six fish from each tank was collected at the beginning and the end of the experiment and analyzed for proximate composition. Twenty-four hours after feeding, three fish from each tank were collected, pooled, and analyzed for their total amino acid composition. The pooled samples of the whole body were homogenized and dried at 105°C for 24-36h to a constant weight in an oven, and then preserved at -20°C for future analysis. Additionally, three fish were taken from each tank for blood collection using heparinized syringes from the caudal vein, followed by dissection to sample the visceral organs and liver. Plasma obtained from each tank, after centrifuging at 18,000 rpm for 10 minutes, and mixed with 10% sulfosalicylic acid (100% v/v) and kept at -80°C until further analysis. The weights of the visceral organs and liver were recorded to calculate the viscerosomatic index (VSI) and hepatosomatic index (HSI). Samples of lateral muscle, taken from a standardized area between the pectoral and anal fins of the same three fish, were prepared for N-metabolites. Pooled samples of liver and lateral muscle from each tank were stored in NUNC boxes at -20°C awaiting analysis. For analysis of muscle and liver N-metabolites, the frozen pooled samples were defrosted on ice, sectioned into small pieces for homogenization, and mixed with 600 µL of 10% sulfosalicylic acid in Eppendorf tubes. This mixture was vortexed and left at room temperature for an hour before being centrifuged at 8,000 rpm for 15 minutes. The supernatants were then transferred to new Eppendorf tubes and stored at -80°C until analysis. The body weight and total length of the remaining fish in each tank were measured to assess growth performance and accretion. All the samples were sent to the Institute of Marine Research (IMR, Bergen, Norway) for chemical analysis.
2.6 Chemical analyses
The analysis of the dietary crude composition, including dry matter, protein, lipids, ash, energy, and the whole-body content of lipid and protein, followed the methodology outlined by Nguyen et al. (2019). In summary, the procedure for dry matter analysis involved drying the sample at 105°C for 24 hours to remove moisture, then weighing to calculate dry matter and moisture content for the whole body, muscle, and feed samples. Crude protein was estimated using the Kjeldahl method, which involves digesting the sample in sulfuric acid, diluting, neutralizing, distilling, and titrating the resultant ammonium. The nitrogen content thus measured was multiplied by 6.25 to estimate crude protein, considering an average nitrogen content of 16% in proteins.
Crude lipids were measured by extracting dried samples with petroleum ether using a Soxhlet apparatus and calculating the ether extract as the weight difference pre- and post-extraction. This method assumes that all diethyl ether-soluble substances are lipids, which is a notable source of error. Ash content, indicative of inorganic minerals, was determined by incinerating samples at 550°C for 6 hours.
Moisture content was determined by drying samples at 105°C. Amino acid compositions of the diets and cobia whole body were assessed after hydrolyzing the samples in 6N HCl with norvaline and DDT for 22 hours, followed by derivatization with AccQTagTM and separation on a UPLC-system. Amino acid concentrations were calculated using external standards.
Free amino acids in deproteinized liver, plasma, and muscle samples were analyzed using a Biochrom 30 plus Amino Acid Analyser, equipped with a lithium column and post column ninhydrin derivatization, quantifying against standards from Sigma. This comprehensive suite of analyses provides detailed insights into the nutritional composition and bioavailability of the dietary components and the organism’s metabolic processing of these nutrients.
2.7 Calculations
The condition factor (CF) was determined as:
where W was the wet body weight in grams and L was the total length in centimeters.
The feed conversion ratio (FCR) for each tank was calculated by dividing the total diet consumed (kg) by the total biomass gain (kg):
Weight gain (ΔG) was assessed as the percentage increase in biomass relative to the initial biomass:
where W1 and W2 represented as the initial and the final body weigh in grams, respectively.
Specific growth rate (SGR) was calculated:
where ln indicates the natural logarithm of the initial (W1) and final (W2) body weights in grams.
Protein efficiency ratio (PER) was determined by the gained biomass per gram of protein consumed:
The protein productive value (PPV) measured how much of the consumed protein was retained:
The retention of indispensable amino acids (IR) was calculated by the formula:
2.8 Statistical analysis
The data is shown as the average for each tank, along with the standard error of the mean (S.E.M.). A two-way ANOVA was utilized to assess any differences or interactions between feeding levels and temperature, considering tanks as the unit of statistical analysis. Where significant differences were found (p < 0.05), the Tukey HSD test was applied to distinguish between the treatments. All statistical analyses were carried out using SPSS software version 22 (IBM Corp., 2013, IBM SPSS Statistics for Windows, Version 22.0, Armonk, NY, USA).
3 Results
3.1 Growth performance and feed utilization efficiency
Both temperature and feeding levels significantly affected the final body weight (BW), total length (TL), feed conversion ratio (FCR), specific growth rate (SGR), viscerosomatic index (VSI), protein efficiency ratio (PER), and feed intake (FI) (P<0.05; Table 3). Specifically, these values were significantly lower at 34°C compared to 30°C, irrespective of feeding level. For FCR and PER, the values at 34°C (95% feeding level) were 2.04 and 1.25, respectively, whereas at 30°C (55% feeding level), they were 1.16 and 1.89. Interestingly, the condition factor (CF) and hepatosomatic index (HSI) were influenced by feeding level (P<0.05) but not by water temperature (P>0.05). Conversely, protein productive value (PPV) was significantly affected by water temperature (P<0.05) but not by feeding level (P>0.05). Notably, interactions between feeding level and water temperature were observed for the final and gained BW, final and gained TL, VSI, and FI (P<0.05). Remarkably, the survival rate remained at 100% across all experimental treatments, indicating the resilience of cobia to the tested conditions.
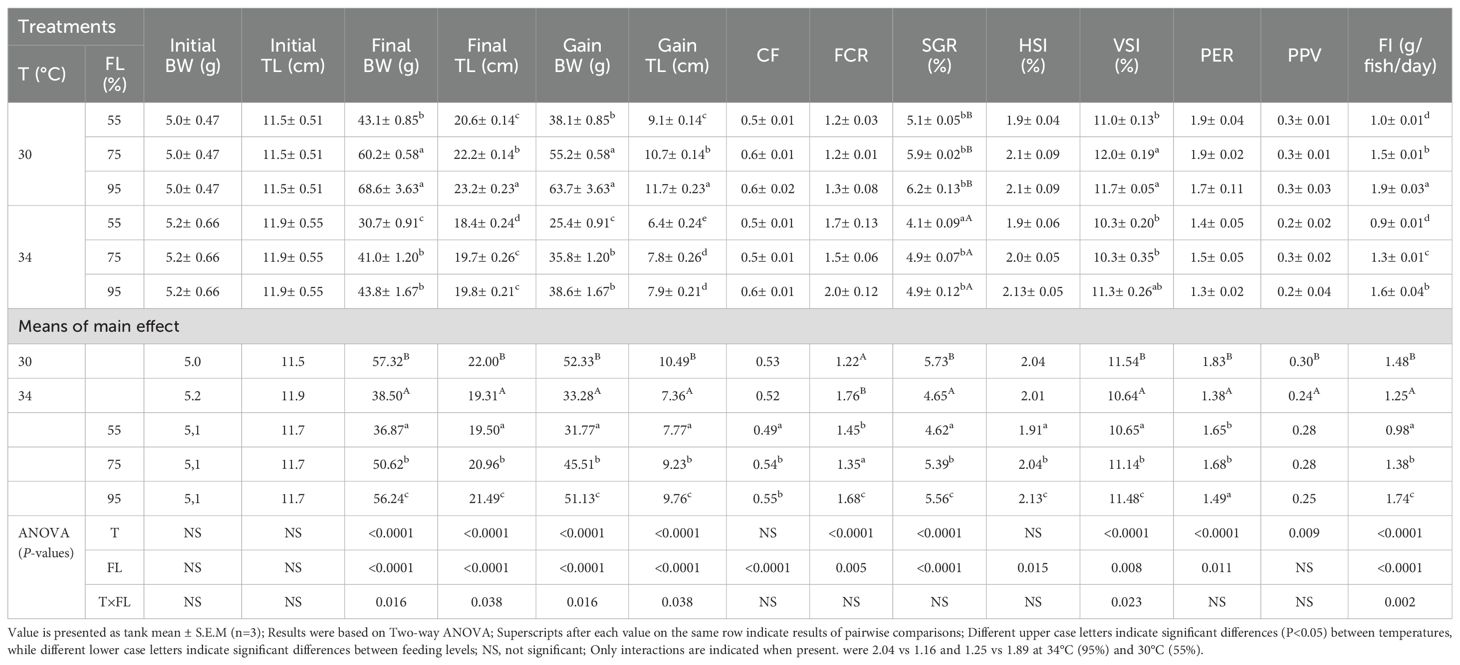
Table 3. Body weight (BW) and length (TL), specific growth rate (SGR), condition factor (CF), viscerosomatic index (VSI), hepatosomatic index (HSI), feed intake (FI), feed conversion ratio (FCR), protein efficient ratio (PER) and protein productive value (PPV) of the cobia fed the test diets with various feeding level (FL) at two temperatures (T: 30 and 34°C).
It should be noted that the procedure for dry matter analysis involved drying the sample at 105°C for 24 hours to remove moisture, before weighing to calculate dry matter and moisture content for the whole body, muscle, and feed samples. The drying temperature of 105°C was selected for its efficiency in rapidly removing moisture, with expected minimal thermal degradation during the short drying period (24-36 hours). However, while this temperature effectively dries samples, lower temperatures (50-60°C) are generally recommended to preserve volatile compounds and prevent their loss. The study by Lantry and O’Gorman (2007) emphasizes that temperatures above 60°C can lead to the volatilization of compounds other than water, such as fats, which may affect energy density calculations. Although 105°C allowed for rapid drying, it is possible that the higher drying temperature caused some loss of volatile compounds, introducing a margin of error. However, the consistent use of 105°C across all samples may have minimized this potential error.
3.2 Proximate composition of whole body
The percentages of protein, lipid, and moisture in the whole body of cobia at both temperatures and different feeding level treatments are presented in Table 4. Body composition was not significantly different between the two temperatures (P>0.05). Additionally, there were no significant differences in protein content and moisture at the different feeding levels (P>0.05). However, a significant difference was observed in the percentage of lipid (P<0.05) at both temperatures: 3.98% (55% feeding level) and 4.60% (95% feeding level) at 34°C compared to 3.83% (55% feeding level) and 5.16% (95% feeding level) at 30°C. An interaction between feeding level and temperature was detected in moisture content (P<0.05), but no interaction was observed in protein and lipid content (P>0.05).
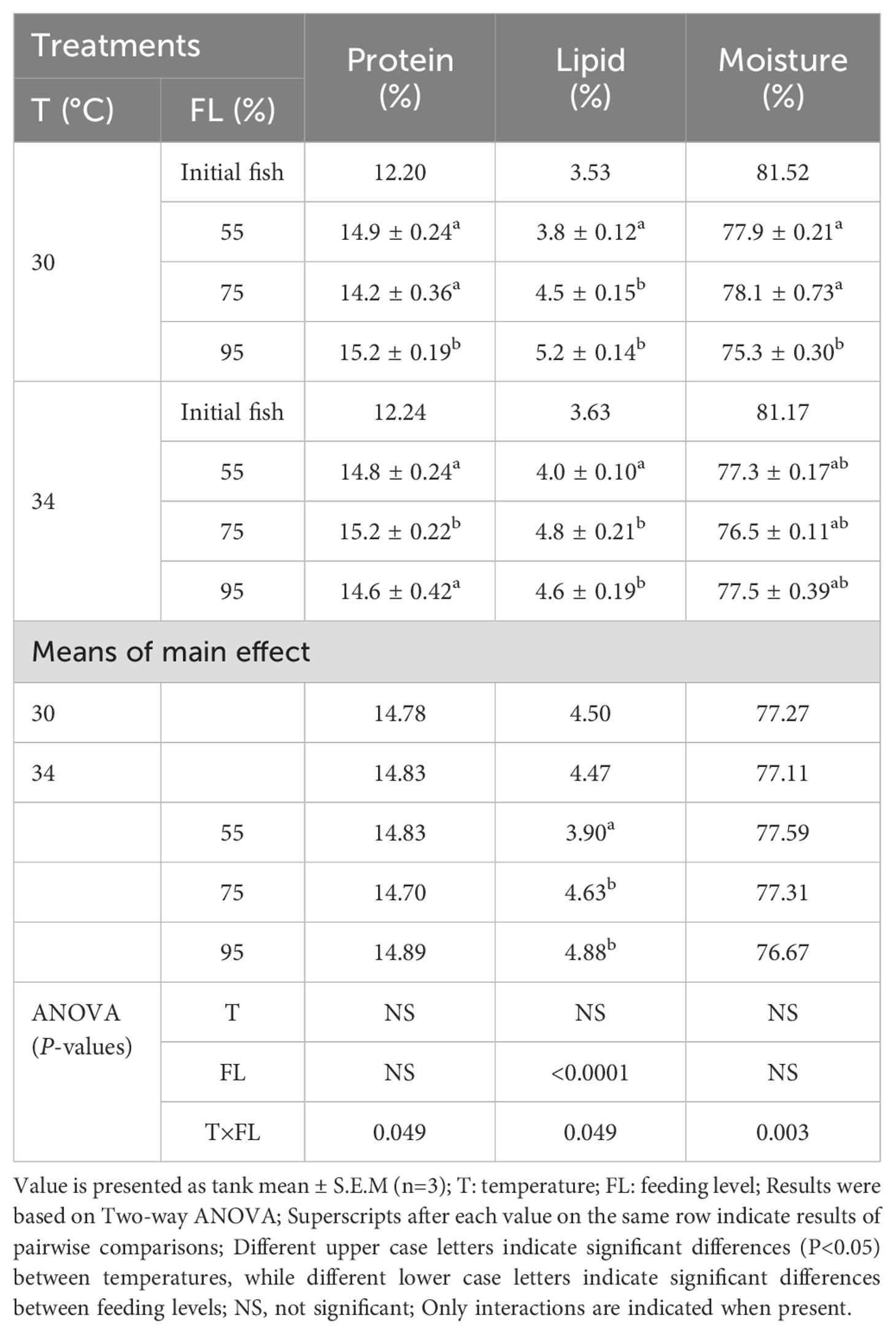
Table 4. Protein, lipid and moisture (%) in whole body of cobia fed three feed ratio levels at the two temperatures.
3.3 Retention of indispensable amino acids
The retention of indispensable amino acids (RIDAA) in cobia is presented in Table 5. RIDAA was influenced by both feeding level and temperature. Cobia fed at 90% of satiation level had poorer RIDAA compared to those fed at 75% or 50% satiation levels, with the latter two groups showing comparable RIDAA (P>0.05). Additionally, cobia reared at 30°C exhibited better RIDAA than those reared at 34°C (P<0.05). There were no significant interactions between feeding level and water temperature on the retention of indispensable amino acids in cobia (P>0.05).
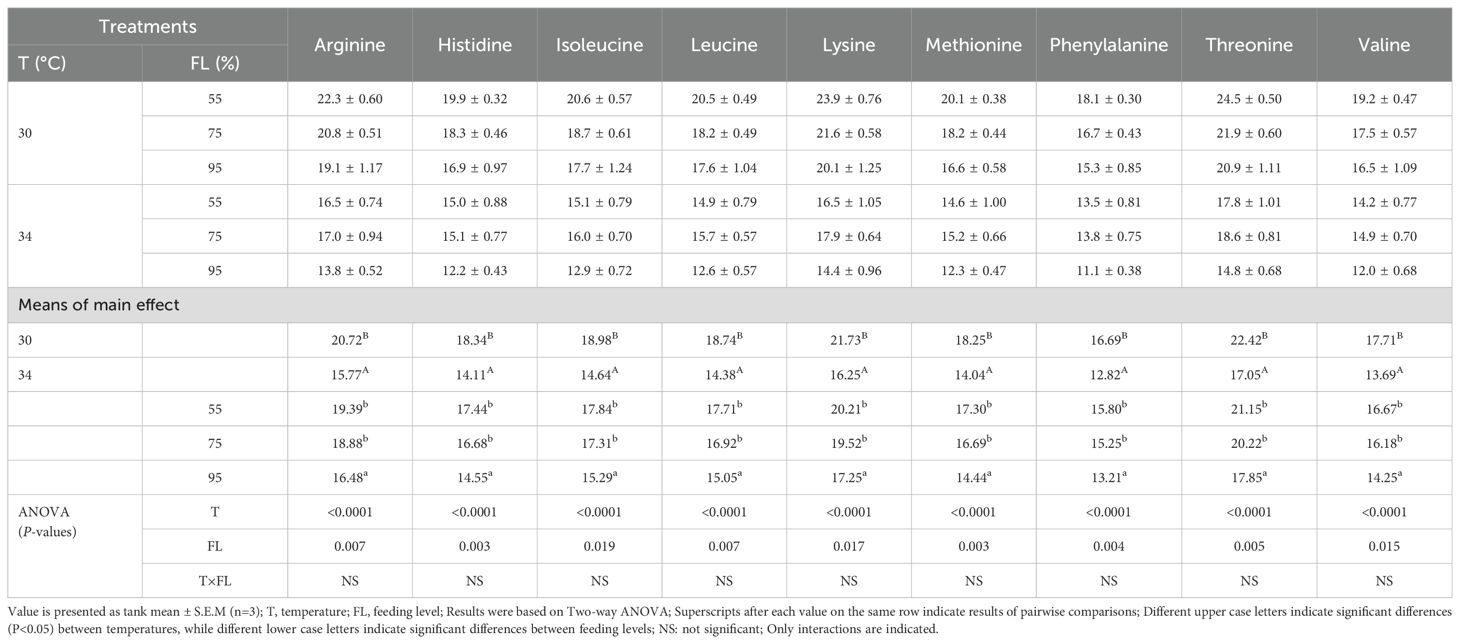
Table 5. Retention of indispensable amino acids (%) in juvenile cobia fed the different feeding levels at two temperatures for a period of 6 weeks.
3.4 Free amino acids and N-metabolites in plasma, muscle and liver tissues of cobia
The concentrations of free amino acids and nitrogen metabolites in cobia plasma at different temperatures and feeding levels are listed in Table 6 and Supplementary Table S1. Temperature significantly affected plasma concentrations of ornithine, methionine, valine, alpha-aminobutyric acid, citrulline, glycine, proline, glutamine, asparagine, threonine, urea, and phosphoserine (P<0.05). The plasma concentrations of ornithine, citrulline, proline, threonine, urea, and phosphoethanolamine were lower at the elevated temperature (34°C) compared to the control temperature (30°C). Feeding levels significantly affected the concentrations of alpha-aminobutyric acid, urea, and phosphoserine (P<0.05). However, there were no significant interactions between water temperature and feeding level on the concentrations of any analyzed free amino acid in plasma (P>0.05).
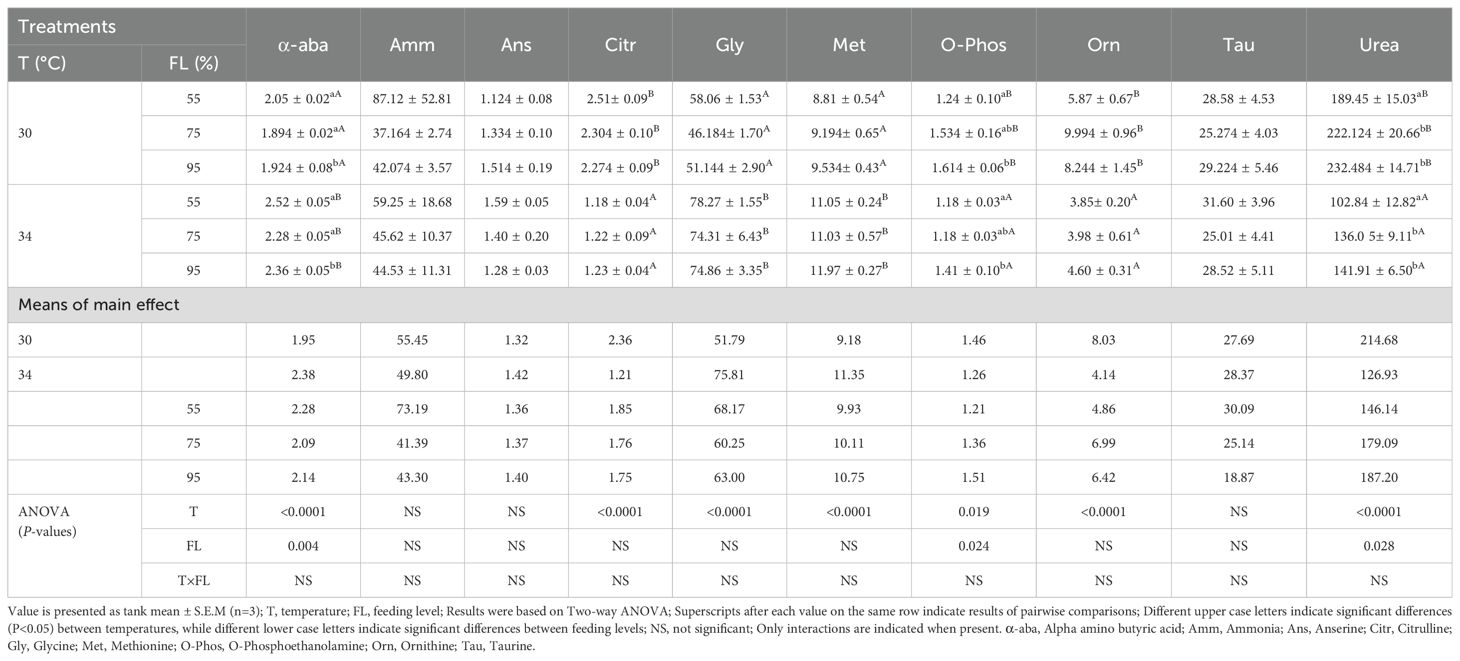
Table 6. Plasma free amino acids and N-metabolites (µmol.100 ml−1) in the cobia fed the three ratio levels at two temperatures during a period of 6 weeks as occurring 24 hour post-prandial.
The final concentrations of free amino acids and nitrogen metabolites in muscle at various temperatures and feeding levels are listed in Table 7 and Supplementary Table S2. Concentrations of ornithine, glycine, serine, and urea in muscle were lower at elevated temperatures (P<0.05). Conversely, changes in feeding levels significantly affected the concentrations of anserine, histidine, ornithine, phenylalanine, beta-alanine, tyrosine, leucine, isoleucine, cystathionine, methionine, valine, citrulline, alanine, glycine, proline, glutamine, glutamic acid, asparagine, serine, threonine, urea, phosphoethanolamine, and taurine (P<0.05). Interactions between water temperature and feeding level were observed in the muscle concentrations of anserine, ornithine, cystathionine, threonine, and hydroxyproline (P<0.05), but not in the other free amino acids in the muscle (P>0.05).
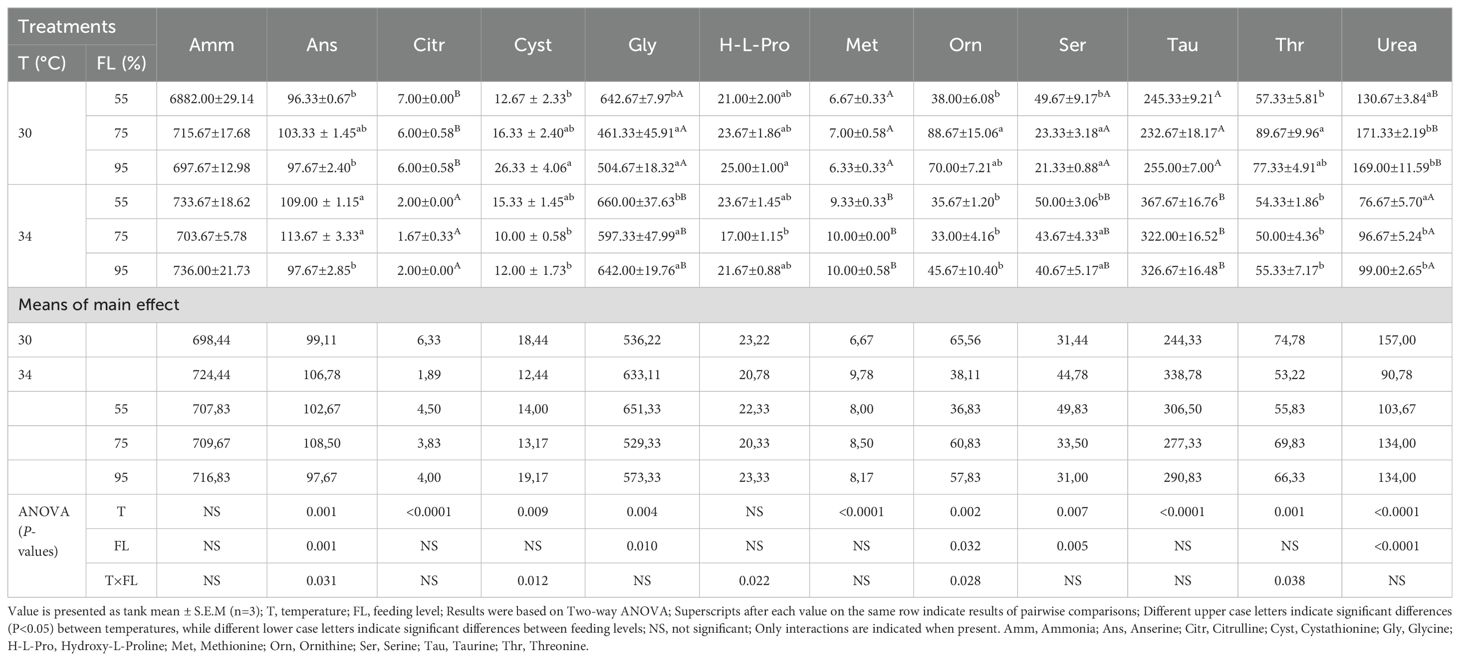
Table 7. Muscle free amino acids and N-metabolites (µmol/100g tissue) of the cobia fed different feeding levels at two temperatures for a period of 6 weeks as occurring 24 hour postprandial.
Concentrations of free amino acids and N-metabolites in the liver at various temperatures and feeding levels are listed in Table 8 and Supplementary Table S3. The concentrations of all free amino acids were significantly affected by water temperature (P<0.05) except urea and ethanolamine (P>0.05). At elevated temperature there were lower concentrations of phosphoserine, phosphoethanolamine, aspartic acid, asparagine, glutamic acid, glutamine, alpha-aminoadipic acid, glycine, alanine, alpha aminobutyric acid, cystathionine, Ornithine, 1-methylhistidine, histidine, and taurine. However, the changes of feeding level influenced the concentration of gamma-amino-n-butyric acid, alpha-amino-n-butyric acid, alanine, glycine, alpha-aminoadipic, glutamine, glutamic, asparagine, serine, aspartic, urea, o-phospho-l-serine, taurine (P<0.05) but had no effect on the remainder free amino acids (Table 8, P>0.05). The interaction of feeding levels and elevated temperatures altered the concentration of asparagine, alpha-aminoadipic, methionine, gamma-amino-n-butyric acid, ornithine (P<0.05) and did not affect the others free amino acids (P>0.05).
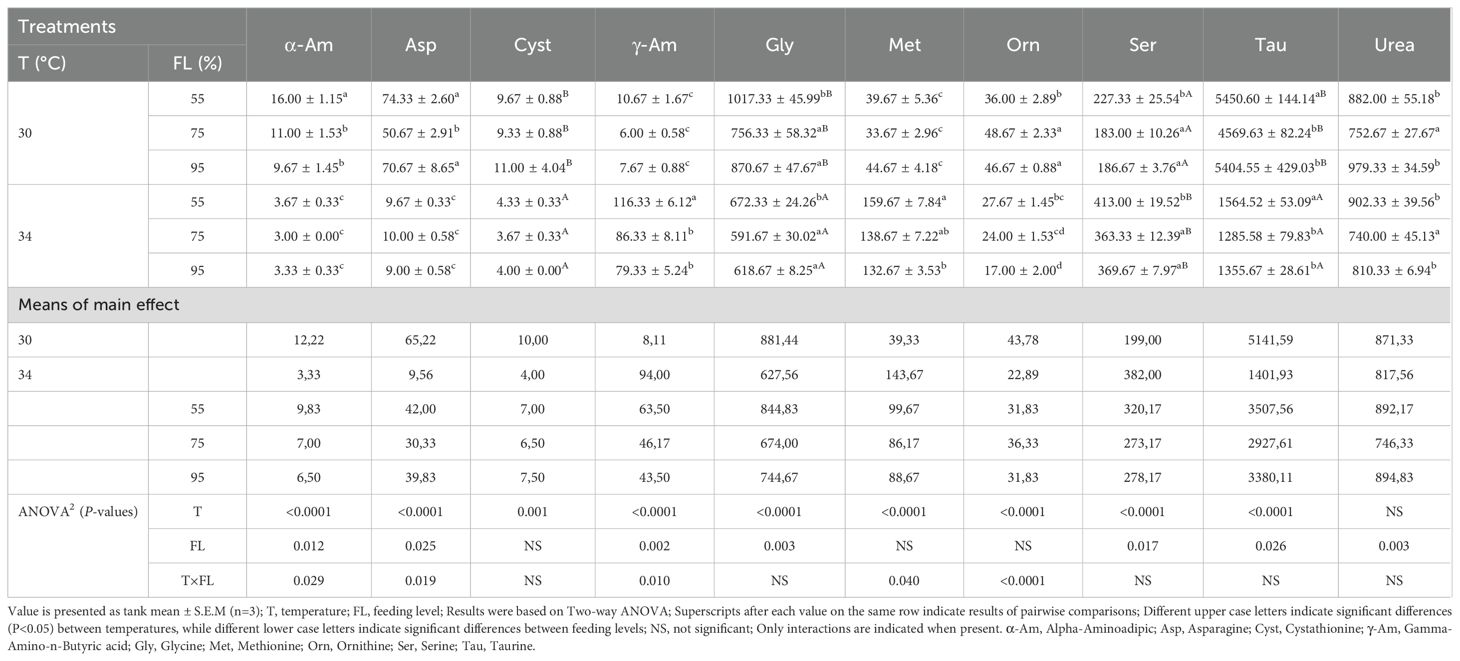
Table 8. Liver free amino acids and N-metabolites (µmol/100g tissue) in the cobia fed the different ratio levels at two temperatures for a period of 6 weeks as occurring 24 hour post-prandial.
4 Discussions
The significant effects of both water temperature and feeding levels on the growth performance and feed utilization of cobia, as observed in our study, align with the growing body of evidence suggesting the critical role of environmental factors on fish performance in aquaculture. The pronounced reduction in growth metrics such as body weight (BW), total length (TL), feed conversion ratio (FCR), specific growth rate (SGR), and protein efficiency ratio (PER) at elevated temperatures (34°C) underscores the thermal sensitivity of cobia. This sensitivity is indicative of metabolic stress, where higher temperatures may accelerate metabolism, leading to increased energy expenditure and, consequently, lower growth efficiency (O’brien et al., 2010; Gonzalez-Rivas et al., 2020).
As ectothermic aquatic animals, fish are strongly affected by the water temperature. Temperature affects metabolism, growth and survival rate of fish (Volkoff and Rønnestad, 2020). The juvenile stage is usually more sensitive than the adult stage to environmental fluctuations, especially to temperature deviations outside the optimum range (Sun and Chen, 2014). The effects of water temperature on fish growth have been examined in several fish species such as European sea bass (Dicentrarchus labrax) (Person-Le Ruyet et al., 2004), parrotfish (Oplegnathus fasciatus) (Kim et al., 2009), turbot (Van Ham et al., 2003), and also cobia (Sun et al., 2006a, 2006b, 2006c; Sun and Chen, 2009; 2014; Nguyen et al., 2019; Le et al., 2020). Generally, the growth rate of fish juveniles increases with increasing water temperature up to the optimal temperature, that is specific for each species, and then decreases at higher temperatures (Sun et al., 2006a, 2006b, 2006c; Sun and Chen, 2009; Pankhurst and Munday, 2011; Sun and Chen, 2014; Nguyen et al., 2019; Le et al., 2020). Part of the reason for this response is a decrease in voluntary feed intake at elevated water temperatures (Pankhurst and Munday, 2011; Kullgren et al., 2013; Froehlich et al., 2018; Nguyen et al., 2019; Le et al., 2020) although the underlying reason for this still remains unknown. Reduced growth and protein deposition were also reported for Atlantic salmon (Salmo salar) fed at increasing water temperatures (Hevrøy et al., 2015) of which most probably was due to reduced voluntary feed intake. Some of the orexigenic and anorexigenic neuropeptides involved in the control of appetite have been shown to be involved in the reduced feed intake (Nguyen et al., 2013; Rønnestad et al., 2017; Nguyen et al., 2023). For juvenile cobia (initial body weight ~22g) a range between 27 and 29°C seems to be the optimal temperature for maximum growth and feed conversion efficiency (Sun et al., 2006a). However, in one of their follow up studies these authors indicated 33°C as the optimal temperature for rearing cobia juveniles in the weight range from 10 to 200 g, since this temperature resulted in the highest growth and feed conversion ratio (Sun and Chen, 2014). When temperature increased above this optimal range a reduced feed consumption, higher feed conversion ratio and lower growth was observed. The current trial was set up using 30°C as the control temperature based on current practice and protocols for rearing cobia juvenile in Vietnam and 34°C as the elevated temperature scenario condition (Doan et al., 2018; Nguyen et al., 2019; Le et al., 2020; Nguyen et al., 2023). As the results presented in Table 4 show, temperature strongly affected growth rates and the feed consumption. This also resulted in that i.e. the final BW at 95% feeding level at 34°C (43.8 g) where similar to the 55% feeding level at 30°C (43.1g). The performance of juvenile cobia was lower at the elevated temperature, that is in line with our previous experiment with cobia reared at similar temperatures although the survival rate did not differ at 34°C, and this temperature is lower that the long term critical temperature for the species (Nguyen et al., 2019). However, Le et al. (2020) reported that the specific growth rate of juvenile cobia was reduced by 10% at 34°C compared to 29°C in an ad libitum feeding regime. A higher FCR in cobia cultured at elevated temperature indicate that a larger amount of feed is needed to support the same growth performance as those fish reared at the control temperature (Sun and Chen, 2014; Nguyen et al., 2019). In this sense, Yúfera et al. (2019) found that in cobia, the residence time of feed within the gut was longer at 30 than at 34°C, and that the small increase of digestive proteolytic activity observed at 34°C was not enough to compensate for the faster gut transit. This indicated that a larger amount of feed would be needed for the same growth parameters as those fish reared in the optimal temperature. This is also expected based on bioenergetics principles (Volkoff and Rønnestad, 2020). In the present study, growth performance and feed utilization were significantly influenced by elevated temperature. Besides the elevated temperature, the feeding levels in the juvenile cobia did significantly influence growth performance and feed utilization. Our findings are similar with the previous report (Sun and Chen, 2014). In addition, growth performance and feed utilization of juvenile cobia were significantly affected by the interaction between feeding levels and elevated temperature. These results were in agreement with the previous study on the juvenile cobia (Sun and Chen, 2014). Interestingly, Nguyen et al. (2019) reported that juvenile cobia gained 62.5g and 42.2g body weight, when fish were fed to near satiety (until most of the fish stopped ingesting feed) during six weeks at temperature of 30°C and elevated temperature of 34°C, respectively. However, our results show that after six weeks cobia had reached 63.6g and 38.6g when it was fed 95% of satiety at 30°C and 34°C, respectively. Furthermore, these values were reduced by 43% and 60% at feeding levels of 75% and 55%, respectively. In addition, this value was 36% lower at 34°C than at 30°C. These differences in the final body weight could be explained by the differences in the initial body weight that in the previous study was 3.7 – 3.8 g/fish and in the present study was 5.0 – 5.2 g/fish, highlighting the importance of initial fish weight when comparing growth performance. The temperature-induced reduction in growth performance and feed utilization suggest that finfish production may be negatively affected by the temperature rise under global warming scenarios (IPCC, 2014, 2021).
Condition factor (CF), viserosomatic index (VSI), hepatosomatic index (HSI) are rough measurements of the energy reserves and nutritional state of the fish (Zhou et al., 2012). Our findings of unchanged condition factor (CF) and hepatosomatic index (HSI) with temperature, but their sensitivity to feeding level, suggest that nutritional strategies could partially mitigate the adverse effects of thermal stress. This is supported by Burraco et al. (2020), who emphasized the importance of tailored feeding regimens to bolster fish resilience against environmental stressors. The significant interaction between feeding level and temperature on growth metrics further highlights the complex interplay between these factors, echoing the need for integrated management approaches in aquaculture (Føre et al., 2018). In the present study, the HSI and CF values were not significantly influenced by the elevated temperature, however, fish cultured at the elevated temperature had lower VSI than fish reared at the control temperature. This result is in contrast with our previous finding (Nguyen et al., 2019) where elevated temperature significantly affected CF, VSI and HSI, while in the current study only the VSI value was affected by temperature. Moreover, the changes of CF, VSI and HSI values at the feeding rate 95% in the present study compared to the previous study of (Nguyen et al., 2019) where the fish were fed to satiety with the same feed and temperatures. Interestingly, their interaction did not affect CF and HSI and only VSI was significantly affected.
The elevated temperature not only affects growth performance, feed utilization, condition factor, viserosomatic index and hepatosomatic index but also has been shown to influence whole-body proximate composition (Nguyen et al., 2019; Le et al., 2020). The whole-body proximate composition analysis revealed significant lipid content variations with feeding level, irrespective of temperature, and points towards a metabolic flexibility of cobia in reallocating energy resources under different nutritional and thermal conditions. This flexibility, however, comes with the caveat of potentially compromised body composition at higher temperatures, necessitating careful consideration of lipid content in feed formulations under climate variability scenarios (De Silva and Soto, 2009). Sun and Chen (2009) and Le et al. (2020) reported that the whole-body proximate composition in juvenile cobia were strongly influenced by elevated temperature. However, in the present study these parameters were not significantly affected. These results can be explained by the differences in diet quality and/or feeding management between studies. The interaction between feeding ration and water temperature significantly affected the whole-body lipid and protein as well as moisture content in the juvenile cobia. These findings are in agreement with the previous study on cobia (Sun and Chen, 2009).
Furthermore, the protein efficiency ratio (PER) and protein productive value (PPV) of the juvenile cobia were significantly influenced by elevated temperature. These results were in agreement with the results of the previous report on juvenile cobia (Nguyen et al., 2019). Our findings indicated that the PER was affected by the feeding ration, but feeding ration did not significantly influence the PPV. Surprisingly, the PER and PPV of the juvenile cobia were not significantly affected by the interaction between feeding rations and temperature. These results suggest that protein digestion and retention of cobia juveniles were affected in the same way for different feeding rations at these two temperatures.
The retention of indispensable amino acids (IAAs) is significantly affected by both temperature and feeding levels (Table 5), with decreased retention observed at higher temperatures, indicating a thermal threshold beyond which nutritional interventions may not fully mitigate the negative impacts on amino acid metabolism (Hoseini et al., 2024). This finding is critical for formulating diets that are optimized not only for growth but also for maintaining amino acid balance, which is essential for overall health and stress resilience. Dispensable amino acids (DAAs) are primarily used as an energy substrate, while IAAs are preserved for growth (Rønnestad et al., 2001; Conceição et al., 2003; Rojas-García and Rønnestad, 2003; Nguyen et al., 2019). The higher retention of IAAs in cobia reared at 30°C may be attributed to their better performance in terms of growth and feeding efficiency compared to those reared at 34°C (Nguyen et al., 2019). Elevated temperatures reduce the retention of IAAs in cobia, likely due to the increased use of IAAs for energy maintenance (Nguyen et al., 2019), as observed in this study. DAA retention depends on factors such as feed consumption, feeding efficiency, growth and metabolism, and the digestion and assimilation of amino acids (Nguyen et al., 2019).
Moreover, the retention of IAAs in juvenile cobia was significantly influenced by feeding levels. In this study, the highest IAA retention was observed at the lower feeding level, although overall values were lower than those reported by Nguyen et al. (2019) for cobia fed at 100% feeding level. To our knowledge, this is the first study examining the effects of feeding levels on IAA retention in tropical finfish such as cobia. These results align with observations in colder water fish species such as Atlantic salmon, Senegal sole, European seabass, and Atlantic halibut (Rønnestad et al., 2001; Rojas-García and Rønnestad, 2003; Person-Le Ruyet et al., 2004; Kullgren et al., 2013; Hevrøy et al., 2015). Interestingly, there was no significant interaction between feeding levels and temperature on IAA retention in juvenile cobia.
Temperature significantly caused a modification of the concentration of free amino acids (FAAs) and N-metabolites in cobia across different tissues. Elevated temperature resulted in distinct metabolic responses between the different feeding levels, where lower feeding levels typically showed increased FAAs in the blood plasma (Table 6), probably as an adaptive mechanism to cope with thermal stress. Still, compared to the 100% feeding level at 34°C, in the study of Nguyen et al. (2019), the plasma concentration for most of the FAA were somewhat different, and with no particular correlation to whether FAA are indispensable or not, pointing to variability in the metabolic response.
In muscle tissue (Table 7), fish maintained at higher feeding levels at the elevated temperature had higher concentrations of key FAAs, reflecting strong metabolic adjustments to sustain muscle function and overall homeostasis. Conversely, in the liver (Table 8), some metabolites varied significantly with temperature, indicating a shift in nutrient utilization and metabolic pathways to manage stress. Again, compared to the 100% feeding level at 34°C in the study of Nguyen et al. (2019), the concentration for most FAA were again somewhat different, and with no particular correlation to whether FAA are indispensable or not, pointing to variability in the metabolic response.
The interactions between feeding levels and temperature had a more pronounced impact on muscle and liver FAAs, but not on plasma FAAs, highlighting that temperature is a predominant factor influencing plasma FAAs. This differential tissue response underscores the complexity of metabolic regulation in fish, suggesting potential avenues targeted nutritional strategies.
The distinct responses of plasma, muscle, and liver tissues to variations in temperature and feeding levels in our study contribute valuable insights into tissue-specific metabolic adjustments in cobia. The lower concentrations of specific amino acids and N-metabolites at elevated temperatures could reflect enhanced utilization or altered distribution among tissues, which might be compensated for by adjusting dietary amino acid profiles (Munguti et al., 2020; Ramli et al., 2020).
Our study focuses on juvenile cobia, providing crucial insights into their metabolic responses to elevated temperatures and varied feeding levels. While these findings are valuable for understanding the early life stages of cobia, we recognize that research on larger, near-harvest-size fish could yield more direct benefits to commercial aquaculture, especially in terms of feed conversion efficiency and nutrient retention. Future studies should extend this research to include larger fish sizes, offering a more comprehensive understanding of the feeding strategies required as cobia approach market size. This would help refine feeding practices and enhance production efficiency, aligning our scientific efforts with industry needs. Nevertheless, the insights gained from studying juvenile stages remain an important basis for optimizing early rearing conditions, contributing to the overall success of cobia farming throughout their growth phases. Our comprehensive analysis highlights the need for adaptive management strategies in cobia aquaculture to counteract the challenges posed by elevated temperatures. This includes optimizing feeding levels and schedules to improve feed utilization efficiency and growth performance, tailoring dietary compositions to ensure adequate nutrient intake and retention, and possibly employing selective breeding techniques to enhance thermal tolerance and nutrient utilization efficiency. The intricate effects of water temperature and feeding levels on cobia’s growth, feed utilization, body composition, and amino acid metabolism underscore the importance of developing nuanced, integrated aquaculture practices. By adapting to the multifaceted challenges of environmental variability, the aquaculture industry can enhance the sustainability and productivity of cobia farming.
5 Conclusion
In the present study a temperature of 30°C and 75-95% satiety feeding level, was the most favorable among the tested conditions for growth, as well as maintaining a balanced proximate composition and free amino acids concentrations in the liver, plasma, and muscle of cobia. Higher temperature and lower feeding levels resulted in poorer performance. At 34°C, the 75% feeding rate was more appropriate than other treatments at this temperature due to the growth rate and the FCR as well as the feed cost and production. A 25% reduction in feed administration can be a useful strategy to manage farms of cobia in the face of rising temperatures. It should be noted that he reduced performance with respect to body growth at elevated temperature and interaction with ration size was observed in juveniles in the size range of 5-69g. Ration size was correlated with increased performance at the optimal water temperature. At 30°C fish administered feed at 95% satiation levels had the fastest growth, while the protein retention was maximized at 75% feeding level. Our findings emphasize the intricate balance required in aquaculture practices to optimize growth performance of cobia in the face of environmental challenges.
Data availability statement
The original contributions presented in the study are included in the article/Supplementary Material. Further inquiries can be directed to the corresponding author.
Ethics statement
The animal study was approved by the Ethics Committee for Animal Research at the Institute of Aquaculture, Nha Trang University, Vietnam. The study was conducted in accordance with the local legislation and institutional requirements.
Author contributions
M-HL: Conceptualization, Data curation, Formal analysis, Investigation, Writing – original draft, Writing – review & editing. ME: Conceptualization, Data curation, Formal analysis, Investigation, Methodology, Writing – original draft, Writing – review & editing. LC: Conceptualization, Funding acquisition, Investigation, Writing – original draft, Writing – review & editing. MN: Conceptualization, Data curation, Formal analysis, Investigation, Methodology, Writing – original draft, Writing – review & editing. MY: Conceptualization, Investigation, Writing – original draft, Writing – review & editing. SE: Conceptualization, Investigation, Writing – original draft, Writing – review & editing. A-EJ: Conceptualization, Investigation, Writing – original draft, Writing – review & editing. HP: Conceptualization, Funding acquisition, Writing – original draft, Writing – review & editing. IR: Conceptualization, Funding acquisition, Investigation, Project administration, Supervision, Visualization, Writing – original draft, Writing – review & editing.
Funding
The author(s) declare financial support was received for the research, authorship, and/or publication of this article. This study has received funding from the European Union’s Horizon 2020 research and innovation programme under the Marie Skłodowska-Curie grant agreement No 691150 (WiseFeed). The work was also supported by project (No. QZA¬0485 SRV¬13/0010) in The Norwegian Programme for Capacity Development in Higher Education and Research for Development (NORHED).
Acknowledgments
Thanks are extended to master students at NTU and PhD candidate Pham Phuong Linh for technical assistance.
Conflict of interest
Author LC was employed by SPAROS Lda. The remaining authors declare that the research was conducted in the absence of any commercial or financial relationships that could be construed as a potential conflict of interest.
Publisher’s note
All claims expressed in this article are solely those of the authors and do not necessarily represent those of their affiliated organizations, or those of the publisher, the editors and the reviewers. Any product that may be evaluated in this article, or claim that may be made by its manufacturer, is not guaranteed or endorsed by the publisher.
Supplementary material
The Supplementary Material for this article can be found online at: https://www.frontiersin.org/articles/10.3389/faquc.2024.1476881/full#supplementary-material
References
Alfonso S., Gesto M., Sadoul B. (2021). Temperature increase and its effects on fish stress physiology in the context of global warming. J. Fish Biol. 98, 1496–1508. doi: 10.1111/jfb.14599
Al Zahrani A. W., Mohamed A. H., Serrano A. E. Jr., Traifalgar R. F. M. (2013). Effects of feeding rate and frequency on growth and feed utilization efficiency in the camouflage grouper (Epinephelus polyphekadion) fingerlings fed a commercial diet. Euro. J. Exp. Biol. 3, 596–601.
Benetti D. D., Suarez J., Camperio J., Hoenig R. H., Tudela C. E., Daugherty Z., et al. (2021). A review on cobia, Rachycentron canadum, aquaculture. J. World Aquaculture Soc. 52, 691–709. doi: 10.1111/jwas.12810
Burel C., Person-Le Ruyet J., Gaumet F., Le Roux A., Severe A., Boeuf G. (1996). Effects of temperature on growth and metabolism in juvenile turbot. J. Fish Biol. 49, 678–692. doi: 10.1111/j.1095-8649.1996.tb00064.x
Burraco P., Orizaola G., Monaghan P., Metcalfe N. B. (2020). Climate change and ageing in ectotherms. Global Change Biol. 26, 5371–5381. doi: 10.1111/gcb.v26.10
Conceição L. E., Grasdalen H., Dinis M. T. (2003). A new method to estimate the relative bioavailability of individual amino acids in fish larvae using 13C-NMR spectroscopy. Comp. Biochem. Physiol. Part B: Biochem. Mol. Biol. 134, 103–109. doi: 10.1016/S1096-4959(02)00193-8
Costa-Bomfim C., Pessoa W., Oliveira R., Farias J., Domingues E., Hamilton S., et al. (2014). The effect of feeding frequency on growth performance of juvenile cobia, R achycentron canadum (L innaeus 1766). J. Appl. Ichthyology 30, 135–139. doi: 10.1111/jai.12339
Daudpota A. M., Abbas G., Kalhoro I. B., Shah S. S. A., Kalhoro H., Hafeez-Ur-Rehman M., et al. (2016). Effect of feeding frequency on growth performance, feed utilization and body composition of juvenile Nile tilapia, Oreochromis niloticus (L.) reared in low salinity water. Pakistan J. Zoology 48, 171–177.
De Silva S. S., Soto D. (2009). “Climate change and aquaculture: potential impacts, adaptation and mitigation. Climate change implications for fisheries and aquaculture: overview of current scientific knowledge,” in FAO Fisheries and Aquaculture Technical Paper (Rome, Italy; FAO), Vol. 530. 151–212.
Doan N. X., Vu M. T., Nguyen H. T., Tran H. T., Pham H. Q., Dinh K. V. (2018). Temperature-and sex-specific grazing rate of a tropical copepod Pseudodiaptomus annandalei to food availability: Implications for live feed in aquaculture. Aquaculture Res. 49, 3864–3873. doi: 10.1111/are.2018.49.issue-12
Føre M., Frank K., Norton T., Svendsen E., Alfredsen J. A., Dempster T., et al. (2018). Precision fish farming: A new framework to improve production in aquaculture. Biosyst. Eng. 173, 176–193. doi: 10.1016/j.biosystemseng.2017.10.014
Fraser T. W., Davies S. J. (2009). Nutritional requirements of cobia, Rachycentron canadum (Linnaeus): a review. Aquaculture Res. 40, 1219–1234. doi: 10.1111/j.1365-2109.2009.02215.x
Froehlich H. E., Gentry R. R., Halpern B. S. (2018). Global change in marine aquaculture production potential under climate change. Nat. Ecol. Evol. 2, 1745–1750. doi: 10.1038/s41559-018-0669-1
Gonzalez-Rivas P. A., Chauhan S. S., Ha M., Fegan N., Dunshea F. R., Warner R. D. (2020). Effects of heat stress on animal physiology, metabolism, and meat quality: A review. Meat Sci. 162, 108025. doi: 10.1016/j.meatsci.2019.108025
Hevrøy E. M., Tipsmark C. K., Remø S. C., Hansen T., Fukuda M., Torgersen T., et al. (2015). Role of the GH-IGF-1 system in Atlantic salmon and rainbow trout postsmolts at elevated water temperature. Comp. Biochem. Physiol. Part A: Mol. Integr. Physiol. 188, 127–138. doi: 10.1016/j.cbpa.2015.06.030
Hoseini S. M., Barbieri E., Aydın B., Yousefi M. (2024). A review of dietary approaches for ammonia toxicity remediation in fish. Aquaculture Int. 32, 5639–5675. doi: 10.1007/s10499-024-01440-4
Imanpoor M., Far K. (2013). Effect of temperature and feeding frequency on growth performances of roach (Rutilus rutilus caspicus). World Appl. Sci. J. 28, 1233–1237. doi: 10.5555/20143096510Or
IPCC (2014). Mitigation of climate change. Contribution of working group III to the fifth assessment report of the intergovernmental panel on climate change. Cambridge University Press
IPCC (2021). Climate Change 2021: The Physical Science Basis. Contribution of Working Group I to the Sixth Assessment Report of the Intergovernmental Panel on Climate Change. Available online at: https://www.ipcc.ch/report/ar6/wg1/downloads/report/IPCC_AR6_WGI_Chapter_04.pdf.
Islam M. J., Kunzmann A., Slater M. J. (2022). Responses of aquaculture fish to climate change-induced extreme temperatures: A review. J. World Aquaculture Soc. 53, 314–366. doi: 10.1111/jwas.12853
Kim S.-S., Galaz G. B., Pham M. A., Jang J.-W., Oh D.-H., Yeo I.-K., et al. (2009). Effects of dietary supplementation of a meju, fermented soybean meal, and Aspergillus oryzae for juvenile parrot fish (Oplegnathus fasciatus). Asian-Australasian J. Anim. Sci. 22, 849–856. doi: 10.5713/ajas.2009.80648
Kullgren A., Jutfelt F., Fontanillas R., Sundell K., Samuelsson L., Wiklander K., et al. (2013). The impact of temperature on the metabolome and endocrine metabolic signals in Atlantic salmon (Salmo salar). Comp. Biochem. Physiol. Part A: Mol. Integr. Physiol. 164, 44–53. doi: 10.1016/j.cbpa.2012.10.00
Lantry B. F., O’Gorman R. (2007). Drying temperature effects on fish dry mass measurements. J. Great Lakes Res. 33, 606–616. doi: 10.3394/0380-1330(2007)33[606:DTEOFD]2.0.CO;2
Le M. H., Dinh K. V., Nguyen M. V., Rønnestad I. (2020). Combined effects of a simulated marine heatwave and an algal toxin on a tropical marine aquaculture fish cobia (Rachycentron canadum). Aquaculture Res. 51, 2535–2544. doi: 10.1111/are.14596
Luo Z., Liu Y. J., Mai K. S., Tian L. X., Tan X. Y., Shi J. F. (2006). Effects of feeding levels on growth performance, feed utilization, body composition, and apparent digestibility coefficients of nutrients for grouper Epinephelus coioides juveniles. J. World Aquaculture Soc. 37, 32–40. doi: 10.1111/j.1749-7345.2006.00004.x
Mizanur R. M., Yun H., Moniruzzaman M., Ferreira F., Kim K.-W., Bai S. C. (2014). Effects of feeding rate and water temperature on growth and body composition of juvenile Korean rockfish, Sebastes schlegeli (Hilgendorf 1880). Asian-Australasian J. Anim. Sci. 27, 690–699. doi: 10.5713/ajas.2013.13508
Munguti J. M., Kirimi J. G., Obiero K. O., Ogello E. O., Kyule D. N., Liti D. M., et al. (2020). Aqua-feed wastes: Impact on natural systems and practical mitigations—A review. J. Agric. Sci. 13, 111. doi: 10.5539/jas.v13n1p111
Nguyen V. M., Espe M., Conceição L. E., Le M. H., Yúfera M., Engrola S. A., et al. (2019). The role of dietary methionine concentrations on growth, metabolism and N-retention in cobia (Rachycentron canadum) at elevated water temperatures. Aquaculture Nutr. 25, 495–507. doi: 10.1111/anu.2019.25.issue-2
Nguyen V. M., Jordal A.-E. O., Espe M., Buttle L., Lai V. H., Rønnestad I. (2013). Feed intake and brain neuropeptide Y (NPY) and cholecystokinin (CCK) gene expression in juvenile cobia fed plant-based protein diets with different lysine to arginine ratios. Comp. Biochem. Physiol. Part A: Mol. Integr. Physiol. 165, 328–337. doi: 10.1016/j.cbpa.2013.04.004
Nguyen M. V., Pham L. P., Jordal A., Espe M., Conceição L. E., Yúfera M., et al. (2023). Elevated sea temperature combined with dietary methionine levels affect feed intake and appetite-related neuropeptide expression in the brains of juvenile cobia (Rachycentron canadum). Front. Mar. Sci. 10. doi: 10.3389/fmars.2023.1183967
O’brien M., Rhoads R., Sanders S., Duff G., Baumgard L. (2010). Metabolic adaptations to heat stress in growing cattle. Domest. Anim. Endocrinol. 38, 86–94. doi: 10.1016/j.domaniend.2009.08.005
Pankhurst N. W., Munday P. L. (2011). Effects of climate change on fish reproduction and early life history stages. Mar. Freshw. Res. 62, 1015–1026. doi: 10.1071/MF10269
Person-Le Ruyet J., Mahé K., Le Bayon N., Le Delliou H. (2004). Effects of temperature on growth and metabolism in a Mediterranean population of European sea bass, Dicentrarchus labrax. Aquaculture 237, 269–280. doi: 10.1016/j.aquaculture.2004.04.021
Ramli N. M., Verreth J., Yusoff F. M., Nurulhuda K., Nagao N., Verdegem M. C. (2020). Integration of algae to improve nitrogenous waste management in recirculating aquaculture systems: A review. Front. bioengineering Biotechnol. 8, 1004. doi: 10.3389/fbioe.2020.01004
Rojas-García C., Rønnestad I. (2003). Assimilation of dietary free amino acids, peptides and protein in post-larval Atlantic halibut (Hippoglossushippoglossus). Mar. Biol. 142, 801–808. doi: 10.1007/s002270100675
Rønnestad I., Conceição L. E., Aragão C., Dinis M. T. (2001). Assimilation and catabolism of dispensable and indispensable free amino acids in post-larval Senegal sole (Solea Senegalensis). Comp. Biochem. Physiol. Part C: Toxicol. Pharmacol. 130, 461–466. doi: 10.1016/S1532-0456(01)00272-1
Rønnestad I., Gomes A. S., Murashita K., Angotzi R., Jönsson E., Volkoff H. (2017). Appetite-controlling endocrine systems in teleosts. Front. Endocrinol. 8, 73. doi: 10.3389/fendo.2017.00073
Saoud I. P., Mohanna C., Ghanawi J. (2008). Effects of temperature on survival and growth of juvenile spinefoot rabbitfish (Siganus rivulatus). Aquaculture Res. 39, 491–497. doi: 10.1111/j.1365-2109.2007.01903.x
Sun L., Chen H. (2009). Effects of ration and temperature on growth, fecal production, nitrogenous excretion and energy budget of juvenile cobia (Rachycentron canadum). Aquaculture 292, 197–206. doi: 10.1016/j.aquaculture.2009.04.041
Sun L., Chen H. (2014). Effects of water temperature and fish size on growth and bioenergetics of cobia (Rachycentron canadum). Aquaculture 426, 172–180. doi: 10.1016/j.aquaculture.2014.02.001
Sun L., Chen H., Huang L. (2006a). Effect of temperature on growth and energy budget of juvenile cobia (Rachycentron canadum). Aquaculture 261, 872–878. doi: 10.1016/j.aquaculture.2006.07.028
Sun L., Chen H., Huang L., Wang Z. (2006b). Growth, faecal production, nitrogenous excretion and energy budget of juvenile cobia (Rachycentron canadum) relative to feed type and ration level. Aquaculture 259, 211–221. doi: 10.1016/j.aquaculture.2006.05.024
Sun L., Chen H., Huang L., Wang Z., Yan Y. (2006c). Growth and energy budget of juvenile cobia (Rachycentron canadum) relative to ration. Aquaculture 257, 214–220. doi: 10.1016/j.aquaculture.2006.02.074
Van Ham E. H., Berntssen M. H., Imsland A. K., Parpoura A. C., Bonga S. E. W., Stefansson S. O. (2003). The influence of temperature and ration on growth, feed conversion, body composition and nutrient retention of juvenile turbot (Scophthalmus maximus). Aquaculture 217, 547–558. doi: 10.1016/S0044-8486(02)00411-8
Volkoff H., Rønnestad I. (2020). Effects of temperature on feeding and digestive processes in fish. Temperature. 7 (4), 307–320. doi: 10.1080/23328940.2020.1765950
Yúfera M., Nguyen M., Navarro-Guillén C., Moyano F. J., Jordal A.-E., Espe M., et al. (2019). Effect of increased rearing temperature on digestive function in cobia early juvenile. Comp. Biochem. Physiol. Part A: Mol. Integr. Physiol. 230, 71–80. doi: 10.1016/j.cbpa.2019.01.007
Keywords: Rachycentron canadum, elevated temperature, feeding levels, growth performance, amino acids, N-retention
Citation: Le M-H, Espe M, Conceição LEC, Nguyen MV, Yúfera M, Engrola S, Jordal A-EO, Pham HQ and Rønnestad I (2024) Impact of feed availability on growth performance and amino acid utilization of cobia (Rachycentron canadum) at elevated temperature. Front. Aquac. 3:1476881. doi: 10.3389/faquc.2024.1476881
Received: 06 August 2024; Accepted: 28 November 2024;
Published: 17 December 2024.
Edited by:
Elisabeth Ytteborg, Fisheries and Aquaculture Research (Nofima), NorwayReviewed by:
M. Refik Orhun, Southeast Fisheries Science Center (NOAA), United StatesDaniel Benetti, University of Miami, United States
Copyright © 2024 Le, Espe, Conceição, Nguyen, Yúfera, Engrola, Jordal, Pham and Rønnestad. This is an open-access article distributed under the terms of the Creative Commons Attribution License (CC BY). The use, distribution or reproduction in other forums is permitted, provided the original author(s) and the copyright owner(s) are credited and that the original publication in this journal is cited, in accordance with accepted academic practice. No use, distribution or reproduction is permitted which does not comply with these terms.
*Correspondence: Ivar Rønnestad, aXZhci5yb25uZXN0YWRAdWliLm5v
†ORCID: Minh Hoang Le, orcid.org/0000-0003-0908-1188
Luis E. C. Conceição, orcid.org/0000-0001-6102-2068
Minh V. Nguyen, orcid.org/0000-0003-1805-4018
Manuel Yũfera, orcid.org/0000-0003-2999-4611
Sofia Engrola, orcid.org/0000-0002-5244-5541
Hung Q. Pham, orcid.org/0000-0003-2470-518X
Ivar Rønnestad, orcid.org/0000-0001-8789-0197