- Northern Aquaculture Demonstration Facility, University of Wisconsin – Stevens Point, Bayfield, WI, United States
One of the most persistent challenges in walleye larviculture is the high rate of unobserved mortality occurring within the first 30 days post-hatch. Walleye larviculture is characterized by high mortality rates, but oftentimes, only a fraction of the total mortality in walleye larviculture is observed. Unobserved mortality is frequently attributed to a high rate of cannibalism, but decomposition of larvae and other factors may also be important contributors. Unobserved mortality can make walleye larviculture especially challenging as accurately estimating daily feed rations and forecasting larval production rely on accurate counts of surviving fish. Despite the importance of unobserved mortality, there is little empirical evidence available that assesses the temporal occurrence and causes of unobserved mortality in walleye larviculture. This study assessed when unobserved mortality occurs during walleye larviculture by examining survival at three timepoints (8, 15, and 30 days post-stocking). Tanks were cleaned daily and dead fry were enumerated to account for observed mortality. At the end of each treatment period, the tanks were drained, all remaining fry were enumerated, and the unobserved mortality rate was calculated. While observed mortality trended higher as trial duration increased, unobserved mortality did not increase significantly from the 8-day treatment suggesting that the bulk of unobserved mortality occurs during the first 8 days of walleye larviculture.
Introduction
Walleye (Sander vitreus) are an emerging aquaculture species with high value and demand in the northern United States and Canada, both as a food fish species and for stocking for sportfishing. Traditionally, walleye have been raised exclusively outdoors in pond systems, primarily for stocking efforts into natural waters for conservation purposes. More recently, walleye are gaining popularity in indoor (intensive) aquaculture settings for stocking and food fish production. The intensive rearing of larval walleye has numerous obstacles to overcome. These obstacles include training larval walleye to eat a commercially pelleted feed (Fischer et al., 2022), challenges with gas bladder inflation (Summerfelt, 2013), cannibalism (Cuff, 1977; Loadman et al., 1986; Reiger and Summerfelt, 1995), unobserved mortality (Summerfelt and Johnson, 2015), turbidity (Bristow et al., 1996; Rieger and Summerfelt, 1997), tank clinging (Rieger and Summerfelt, 1997), water quality (Phillips et al., 1998), and labor requirements. Although progress has been made on many of these bottlenecks, cannibalism and unobserved mortality remain persistent problems for walleye larviculture.
Unobserved mortality (defined as mortalities that are not accounted for through the larval period) has been attributed to several factors including cannibalism (Figure 1) and decomposition (Figure 2) with the bulk of walleye literature using cannibalism to explain any unaccounted larvae and discrepancies in survival (Kindschi and MacConnell, 1989; Peterson et al., 1997; Dabrowski et al., 2000). Cannibalism can be categorized as either type I where the victim is not consumed but dies from the cannibalism attempt or type II where the victim is completely consumed (Duk et al., 2017). Type II cannibalism mortalities are nearly impossible to observe as fish consumption does not leave any evidence. Type I cannibalism can also contribute to unobserved mortality if the victim fish disintegrate before they are accounted for. Cannibalism is common in cultured fish and can significantly impact aquaculture operations (Baras and Jobling, 2002; Duk et al., 2017). Walleye are particularly prone to type I cannibalism as they are capable of attacking conspecifics that are the same size or larger than themselves (Cuff, 1980; Kestemont et al., 2003). Cannibalism is not the only potential cause of unobserved mortality. Because of the general vulnerability of early larvae [4–12 days post-hatch (DPH)], routine mortality can occur from a wide variety of sources including sub-optimal rearing conditions, non-acceptance of feed, bacteria, lack of swim bladder inflation, and stress. Human error and missed mortalities when cleaning tanks can also contribute to unobserved mortality, especially when rapid decomposition of larval walleye makes identifying mortalities difficult. Similar challenges have been documented in early larviculture of closely related pikeperch (Sander lucioperca) in intensive systems (Steenfeldt, 2015).
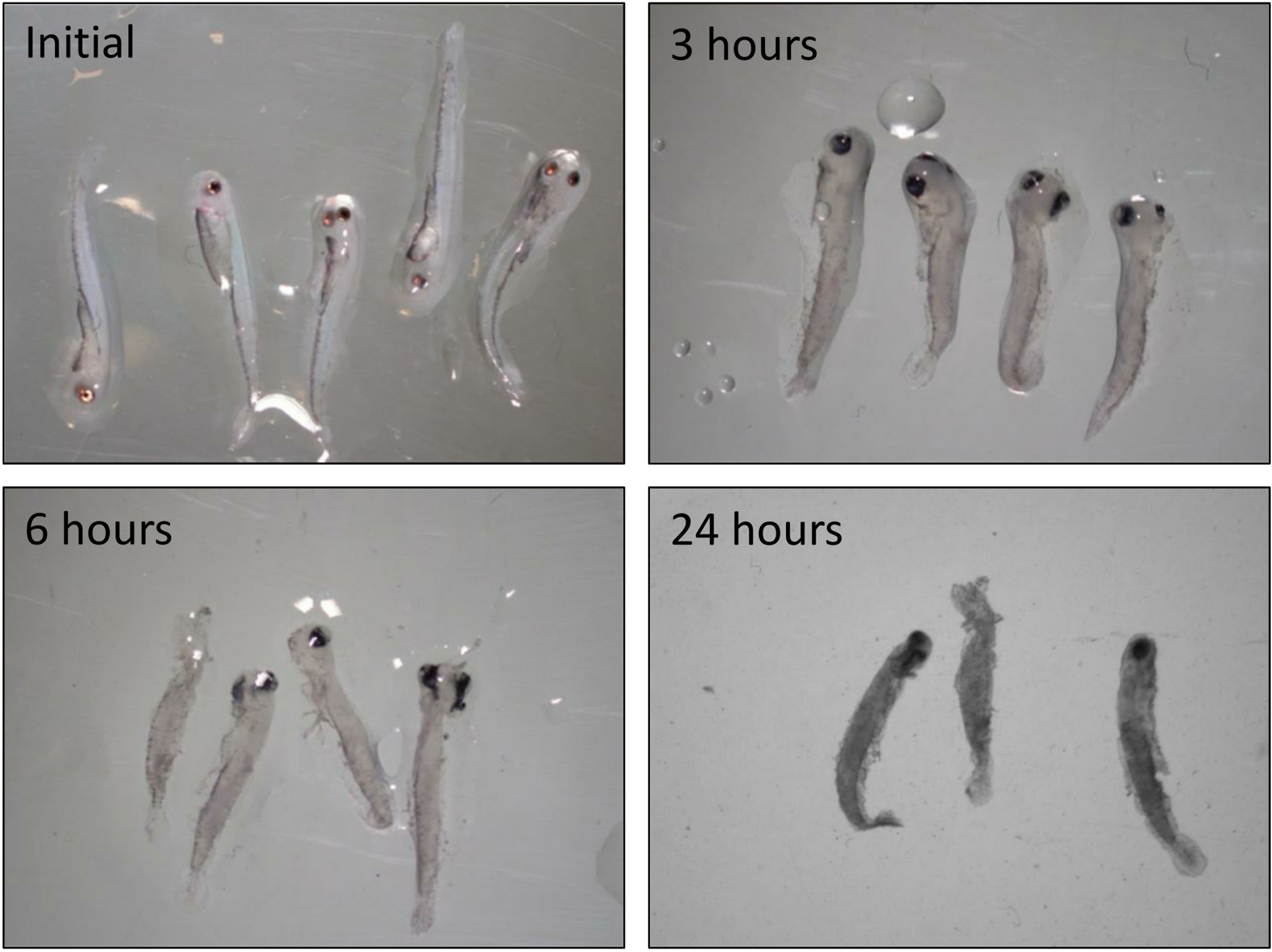
Figure 2 Decomposition of dead walleye larvae held in the bottom of a larviculture tank. Pictures were taken at the time of mortality (Initial), 3-h, 6-h, and 24-h post-mortality.
Unobserved mortality creates many practical challenges for walleye larviculture. Feeding rates are often reduced at regular intervals in the feeding schedule to account for unobserved mortality (Hauser et al., 2023). Because unobserved mortalities must be estimated, overestimating unobserved mortality for tanks with higher survival can cause excess mortality due to underfeeding. Conversely, underestimating unobserved mortalities can lead to the overfeeding of larvae. Overfeeding contributes to decreases in water quality and fungal growth within larviculture tanks. Fungal growth and poor water quality can also create a feedback loop as they increase the difficulty of cleaning tanks and accurately identifying mortalities trapped within fungal matter, causing a higher rate of both unobserved and observed mortalities. In many studies conducting walleye larviculture, unobserved mortalities lead to a large discrepancy between observed mortality and the final survival rate (Dabrowski et al., 2000; Garcia-Abiado et al., 2004; Summerfelt and Johnson, 2015; Fischer et al., 2022).
Observed mortality is straightforward to obtain by siphoning debris and dead fish from the bottom of the tank and enumerating mortalities. Currently, there is no way to effectively account for unobserved mortalities during the walleye larviculture period due to the practices required for raising fish at this life stage. Tanks are kept at a high turbidity (50–80 NTU) (Johnson et al., 2021; Hauser et al., 2023), which reduces the ability to visually inspect survival in the tanks. Walleye are reared in low light settings (two lumens) (Hauser et al., 2023), which further reduces the ability to observe mortality in the tanks. Larval walleye are also intolerant to handling and may die if moved or handled prior to scale development (Hauser et al., 2023).Their intolerance to handling makes draining and netting out fish to determine survival infeasible until approximately 30 days post-hatch, when their scales are more developed (larger than 40 mm in length) (Summerfelt and Johnson, 2015; Hauser et al., 2023). Presently, unobserved mortality totals are determined after fish are moved from the larval tanks at approximately 30 days post hatch, when total fry survival can be enumerated. Total survival is subtracted from the expected survival (initial stocking numbers minus observed mortalities) to obtain unobserved mortality totals.
This study’s objective is to determine at what stage most of the unobserved mortality occurs in the larval rearing period. Currently, there is little available information detailing when unobserved mortality occurs in larval walleye culture. There are several developmental milestones that may be critical points of high unobserved mortality. For example, 8 days post-hatch is a developmental milestone for air bladder inflation and feed acceptance (Rieger and Summerfelt, 1998). Larval walleye that fail to inflate their air bladders or accept feed by this time are more susceptible to mortality. Furthermore, walleye that do not accept feed are smaller than conspecifics that do and are therefore more likely to be cannibalized or to disintegrate before being counted as a mortality. Cannibalism has been previously documented from day 9 to day 15 (Peterson et al., 1997). Thirty days is typically when walleye are transferred from larval tanks and into a recirculating aquaculture system (RAS) for grow out. Moving fish prior to this can cause mortality due to the lack of scale production (Hauser et al., 2023). With these larviculture milestones in mind, we sought to assess when unobserved mortality occurs by raising larval walleye in larviculuture systems and sampling survival at distinct periods. We chose 8, 15, and 30 days post stocking as the trial lengths to determine which stage has the highest unobserved mortality. Determining when unobserved mortality is at its highest is important to walleye culture going forward and could help direct further efforts in reducing its overall percentage.
Methods
Intensively reared walleye broodstock (age 3–5) of Mississippi River strain origin were spawned at the University of Wisconsin-Stevens Point Northern Aquaculture Demonstration Facility (UWSP NADF) to provide eggs for this study. Eggs from two female walleye were collected and fertilized using the dry method (Piper, 1982) with milt from three different males. Post-fertilization, the eggs were water-hardened for 1 h. After the eggs were water-hardened, they were disinfected with iodine at 100 mg/L for 15 min. Once hardened and disinfected, eggs were then placed in a flow-through bell jar incubation system at 8.3°C. After 7 days, the temperature was increased by 0.5°C daily until hatch occurred at 15°C. Once hatched, fry swam out of the top of the bell jars and were directed into capture tanks (Supplementary Figure S1). They remained in the capture tanks for 4 days to allow yolk sac absorption and ensure that they are prepared for exogenous feeding Supplementary Figure S1.
Larval walleye were enumerated using Jensorter (FCM) with plate #1 (1.6 mm) installed and a setting of 9,2,2 with base A. Walleye larvae are photopositive at hatch, and only larvae congregated under the tank side lighting were collected (Supplementary Figure S2). Each tank was assigned a trial duration of 8, 15, or 30 days. A total of 1,000 larval walleye were stocked into six 240-L tanks (4 larvae/L) (Supplementary Figure S3) at 4 days post-hatch (DPH), and these tanks were used for the 8-day and 15-day trials. The three tanks in the 30-day trial were stocked with 1,200 fish (5 fry/L). Because the 30-day trial was stocked with a higher density, there could be a resulting density effect on mortality. To account for this, the results were compiled and analyzed as a proportion of the initial population. All treatment tanks were supplied with well water warmed to approximately 21°C at a flow of 4 L/min. Fish were fed Otohime (sizes B1, B2, C1 and C2; Marubeni Nisshin Feed Co., Ltd, Tokyo, Japan) 24 h/day for the duration of the trials (Table 1). Dim lighting was set to approximately two lumens in each of the tanks for a constant 24-h period. Turbidity in the tanks was kept at 50–80 (NTU) range using ball clay. Fish were raised in optimal flow-through conditions with dissolved oxygen maintained above 90% saturation (Summerfelt, 1996; Summerfelt and Johnson, 2015; Hauser et al., 2023).
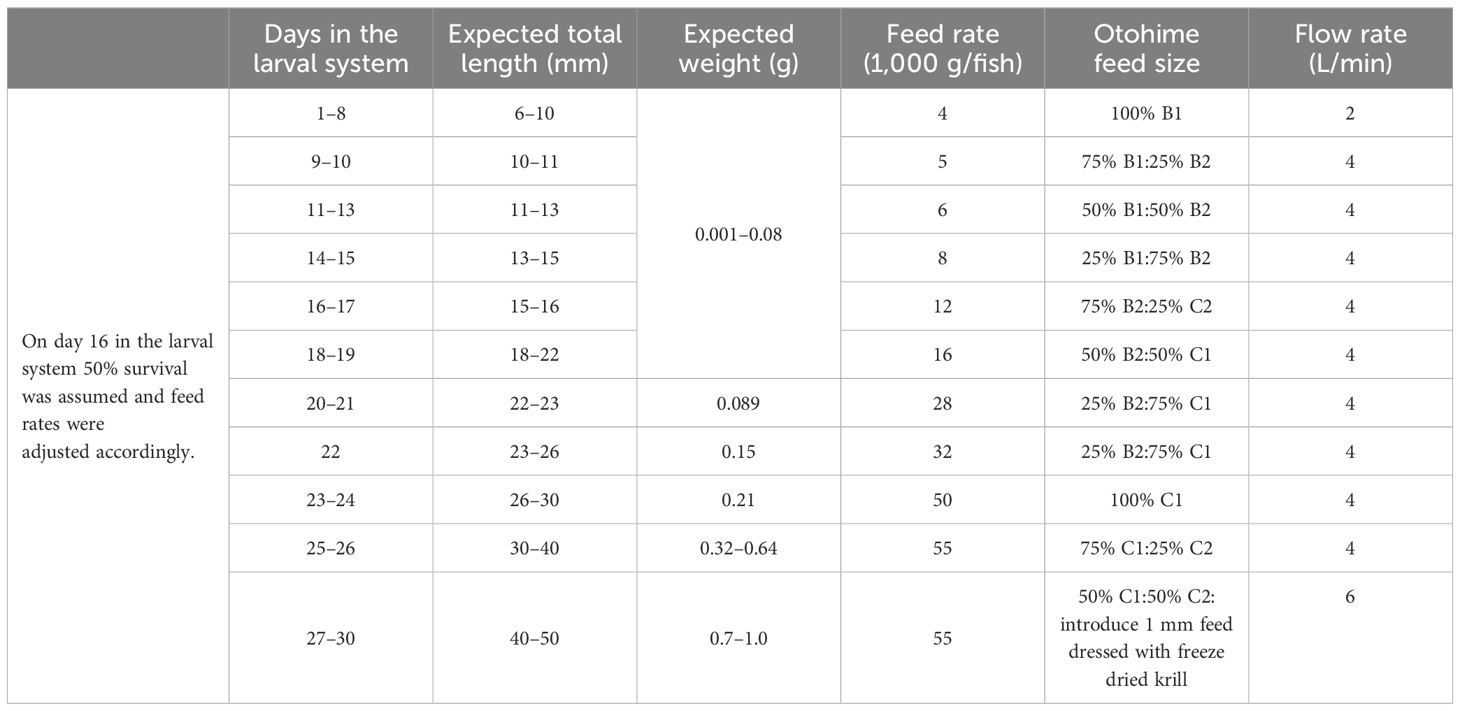
Table 1 Description of feed rates, feed sizes, flow rates, and approximate walleye size based on days in the larval system.
In the three, 8-day treatment tanks, fry were netted out and hand-counted after 8 days in the system (12 DPH). In the three, 15-day treatment tanks, surviving fry were counted after 15 days in the system (19 DPH). The remaining three, 30-day treatment tanks were maintained until day 30 in the system (34 DPH) and surviving fry were enumerated. Unobserved mortality was calculated as a percentage of the initial number of stocked larvae that could not be accounted for during the daily collection of observed mortality, using the equation: where P is tank population.
To qualitatively investigate the rate of decomposition in early walleye larviculture, we assessed decomposition rates of recently hatched larvae. Five fry were euthanized using MS-222 (Syndel, Ferndale, WA, USA) following manufacturer specifications at 4 DPH and placed into two 7.6-cm diameter polyvinyl chloride (PVC) sections with fine micron screen on both ends to allow for water exchange. The PVC sections were placed in separate larviculture tanks under the same conditions as experimental tanks. Pictures were taken at 3, 6, and 24 h to capture the rate of decomposition using a dissecting microscope.
Statistics were calculated with the use of program R (R Core Team, 2023). We used one-way ANOVA tests with a significance threshold of alpha = 0.05. If most of the unobserved mortalities occur prior to day 8, with no or limited mortality thereafter, we would not expect to see a difference in unobserved mortality between trial durations and fail to reject the null hypothesis. If unobserved mortalities continue to occur post day 8 but stops prior to day 15, the 15- and 30-day trials would have no significant difference but would be significantly different from the day-8 trials. If unobserved mortalities continue throughout the entire 30-day larviculture period, all trials would be significantly different. If unobserved mortalities primarily occur between day 8 and day 15, both the 15-day and 30-day trials would have no significant difference, but both would be significantly different from the 8-day trials. Our analysis relies on the assumption that unobserved mortality is equal among treatments at any given time. While it would be more optimal to observe mortality in distinct time periods, this would require handling larvae at their most fragile age and would therefore result in unacceptably high mortality in our latter treatments. Therefore, relying on the assumption of equal mortality is required.
Results
In the 8-day trial, unobserved mortalities accounted for 24.27% (± standard deviation of 4.22) of the initial population of larval walleye in the tanks (Figure 3). In the 15-day trial, unobserved mortalities accounted for 34.87% (± standard deviation of 10.59) of the initial population in the tanks (Figure 3). Day-30 trial saw unobserved mortality account for 18.56% (± standard deviation of 7.14) of the initial population (Figure 3). Fry survival (including observed and unobserved mortalities) in the 8-day trials averaged 35.5%, 15-day trial averaged 15.2%, and 30-day trial averaged 29.5% (Figure 3). One tank in the 15-day trial only had four fish survive the entire period (Supplementary Table S1). Although this is a high mortality rate and appears to be an outlier, it is not uncommon to see periodic high mortality in walleye larviculture tanks, and therefore, this sample was not excluded from analysis. There was no significant difference in the percentage of the initial population that had unobserved mortality (P value = 0.304) among treatments. There was also no significant difference in the percentage of observed mortality (P value = 0.053) among treatments.
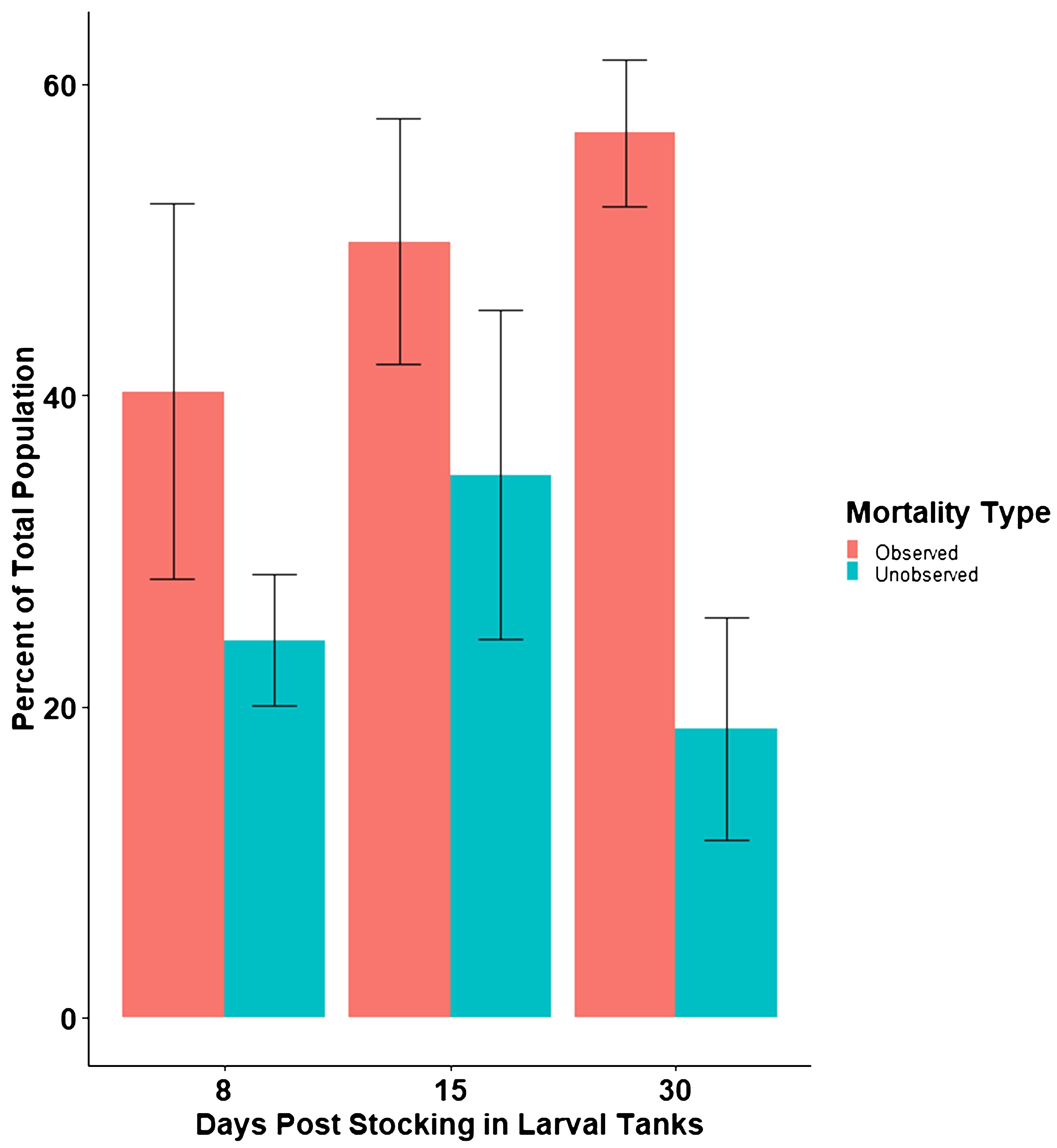
Figure 3 Observed and unobserved mortality presented as a percentage of the initially stocked population in each trial length. Bars represent averages and error bars show standard deviation.
Discussion
Unobserved mortality is a pressing challenge in walleye larviculture that adds uncertainty to production efforts and leads to difficulties adjusting feed rates. This study aimed to identify when unobserved mortality occurs temporally during walleye larviculture so that survival can be more accurately forecasted and feed regimes can be more precisely delivered. Our results suggest that the bulk of unobserved mortalities occur within the first 8 days in the larval system. Observed mortality trended higher as trial duration progressed, but unobserved mortality remained similar across all trials (Figure 3). Unobserved mortality was not significantly higher in the latter treatments than in the 8-day treatment (p = 0.304) indicating that the first 8 days account for the bulk of these occurrences.
Although this study was not explicitly designed to identify specific causes of unobserved mortality, the early occurrence of unobserved mortality provides some insight into potential causes. The small size (<14 mm) of walleye larvae prior to day 8 suggests that decomposition may play a substantial role. At these small sizes, dead larvae can be exceedingly difficult to differentiate from uneaten feed, debris, and fungus collected during routine tank cleaning. Decomposition occurs rapidly, and within 24 h of mortality, many may not be readily identifiable as dead larvae (Figure 2). Given that most of the observed mortality also occurs within the first 8 days (Figure 3) and decomposition of small dead larvae can be rapid (Figure 2), it is not surprising that a substantial proportion of these mortalities would be missed during routine sampling and enumeration. The high mortality rate early on in walleye larviculture is likely driven by individuals that fail to meet key developmental milestones such as feed acceptance and air bladder inflation that generally occur near day 8 (Rieger and Summerfelt, 1998). Larvae that never accept dry feed provided to them are likely exceptionally small when they die. Their small size combined with the effects of disintegration may make identifying these individuals nearly impossible. Furthermore, mortalities that occur soon after tank cleaning or those missed due to the dark turbid conditions in the larval tanks experience extended decomposition times, which can lead to further difficulty in identifying individual mortalities and in some cases, complete disintegration. Conversely, larvae that were beginning to grow and were successfully achieving critical developmental milestones but die thereafter would be easier to observe due to their larger size and the greater degree of decomposition required to render them unidentifiable.
Just because decomposition is a likely culprit for unobserved mortality does not mean that cannibalism does not also play a role. Type I cannibalism, where the victim is attacked but not consumed (Duk et al., 2017), may significantly contribute to early mortalities that decompose before being accounted for. Direct observations of early walleye larvae suggest that aggressive behavior that leads to wounding of other fish (type I cannibalism) contributes a greater proportion of mortality than direct consumption (type II cannibalism) of conspecifics (Reiger and Summerfelt, 1995). Anecdotally, we observed cannibalism attempts near the surface of the larviculture tanks during the photopositive stage (~1–10 DPH; Hauser et al., 2023). While we could not determine if these attempts lead to type I or type II, the small gape size of larvae at this stage leads us to suspect that relatively few resulted in type II cannibalism where the target was fully consumed. Other studies have similarly observed early cannibalism attempts (Peterson et al., 1997; Dabrowski et al., 2000; Johnson et al., 2021) but did not identify if they were type I or type II cannibalism. Because type II cannibalism is generally more frequent as fish grow and size differences develop (Duk et al., 2017), it is unlikely to play a substantial role in unobserved mortality prior to day 8. Further studies are needed to fully explore the relative influence of type II cannibalism on unobserved mortality during walleye larviculture, but given the temporal incidence of unobserved mortality, it appears unlikely that direct consumption of conspecifics is the largest contributor.
Intensive walleye larviculture is challenging, and unobserved mortality can make optimizing feeding regimes and forecasting production difficult. Because unobserved mortality appears to occur before day 8, particular attention to feed rates at this time can ensure fish are not being overfed resulting in dirty tanks. Excess feed leads to fungal problems and decreases water quality, so accurately estimating feed rates based on the true number of fish in the tank is critical. Going forward, an estimated “true” mortality rate could be developed based on observed mortality and an assumed unobserved mortality portion. Our study is likely insufficient to develop this estimation with any certainty, but this would be a fruitful goal of subsequent studies. One can also factor this information into their forecasting of production at the end of the larviculture period. Initially stocking fry in excess to account for unobserved mortality prior to day 8 can ensure that an operation will have a sufficient number of fry at the end of the larviculture period. However, there may be limits to this approach, as higher initial density may increase mortality rates and subsequent unobserved mortality. Unobserved mortality is just one of the many hurdles making walleye larviculture difficult, but understanding when it occurs and developing strategies to account for it can facilitate the success of walleye aquaculture going forward.
Data availability statement
The raw data supporting the conclusions of this article will be made available by the authors, without undue reservation.
Ethics statement
The animal study was approved by University of Wisconsin – Stevens Point IACUC. The study was conducted in accordance with the local legislation and institutional requirements.
Author contributions
JN: Conceptualization, Data curation, Formal analysis, Investigation, Software, Visualization, Writing – original draft, Writing – review & editing. CB: Conceptualization, Data curation, Formal analysis, Investigation, Software, Visualization, Writing – original draft, Writing – review & editing. KH: Conceptualization, Investigation, Methodology, Resources, Supervision, Writing – original draft, Writing – review & editing, Formal analysis, Visualization. EH: Conceptualization, Investigation, Methodology, Resources, Visualization, Writing – original draft, Writing – review & editing. TF: Conceptualization, Investigation, Methodology, Resources, Visualization, Writing – original draft, Writing – review & editing, Data curation, Formal analysis, Software, Funding acquisition, Project administration, Supervision, Validation.
Funding
The author(s) declare financial support was received for the research, authorship, and/or publication of this article. This publication is a result of work sponsored by the North Central Regional Aquaculture Center Program under grant number SA75282130-A; P081068 from the U.S. Department of Agriculture. The U.S. Government and the North Central Regional Aquaculture Center are authorized to produce and distribute reprints for governmental purposes notwithstanding any copyright notation appearing herein.
Conflict of interest
The authors declare that the research was conducted in the absence of any commercial or financial relationships that could be construed as a potential conflict of interest.
Publisher’s note
All claims expressed in this article are solely those of the authors and do not necessarily represent those of their affiliated organizations, or those of the publisher, the editors and the reviewers. Any product that may be evaluated in this article, or claim that may be made by its manufacturer, is not guaranteed or endorsed by the publisher.
Supplementary material
The Supplementary Material for this article can be found online at: https://www.frontiersin.org/articles/10.3389/faquc.2024.1387495/full#supplementary-material
References
Baras E., Jobling M. (2002). Dynamics of intracohort cannibalism in cultured fish. Aquac Res. 33, 461–479. doi: 10.1046/j.1365-2109.2002.00732.x
Bristow B. T., Summerfelt R. C., Clayton R. D. (1996). Comparative performance of intensively cultured larval walleye in clear, turbid, and colored water. Progress. Fish-Cult. 58, 1–10. doi: 10.1577/1548-8640(1996)058<0001:CPOICL>2.3.CO;2
Cuff W. R. (1977). Initiation and control of cannibalism in larval walleyes. Progress. Fish-Cult. 39, 29–32. doi: 10.1577/1548-8659(1977)39[29:IACOCI]2.0.CO;2
Cuff W. R. (1980). Behavioral aspects of cannibalism in larval walleye, Stizostedion vitreum. Can. J. Zool 58, 1504–1507. doi: 10.1139/z80-207
Dabrowski K., Czesny S., Kolkovski S., Lynch W. E., Bajer P., Culver D. A. (2000). Intensive culture of walleye larvae produced out of season and during regular season spawning. N Am. J. Aquac 62, 219–224. doi: 10.1577/1548-8454(2000)062<0219:ICOWLP>2.3.CO;2
Duk K., Pajdak J., Terech-Majewska E., Szarek J. (2017). Intracohort cannibalism and methods for its mitigation in cultured freshwater fish. Rev. Fish Biol. Fish 27, 193–208. doi: 10.1007/s11160-017-9465-2
Fischer G. J., Firkus T. J., Holmes K., Blaufuss P. C., Amberg J. J., Hartleb C. F. (2022). Diet influences survival and growth of intensively reared larval saugeye (Sander vitreus × Sander canadensis). Aquacult. Fish Fish. 2, 450–457. doi: 10.1002/aff2.86
Garcia-Abiado M. A., Czesny S., Dabrowski K. (2004). Tank performance of larval saugeyes (Walleye × Sauger) produced out-of-season and during regular season spawning. N Am. J. Aquac 66, 48–52. doi: 10.1577/A03–001
Hauser E., Firkus T., Holmes K., Neibauer J., Branville C., Hartleb C., et al. (2023) Walleye Culture Guide. University of Wisconsin-Stevens Point Northern Aquaculture Demonstration Facility, Bayfield, Wisconsin. Available online at: https://www.researchgate.net/publication/368983337_Walleye_Culture_Guide.
Johnson J. A., Kelsey K., Summerfelt R. (2021). “Walleye Larviculture in Water Reuse Aquaculture Systems,” in Yellow Perch, Walleye, and Sauger: Aspects of Ecology, Management, and Culture. Eds. Bruner J. C., DeBruyne R. L. (Springer International Publishing, Cham), 141–190. doi: 10.1007/978–3-030–80678-1_6
Kestemont P., Jourdan S., Houbart M., Mélard C., Paspatis M., Fontaine P., et al. (2003). Size heterogeneity, cannibalism and competition in cultured predatory fish larvae: Biotic and abiotic influences. Aquaculture 227, 333–356. doi: 10.1016/S0044-8486(03)00513-1
Kindschi G. A., MacConnell E. (1989). Factors influencing early mortality of walleye fry reared intensively. Progress. Fish Cult. 51, 220–226. doi: 10.1577/1548-8640(1989)051<0220:CFIEMO>2.3.CO;2
Loadman N. L., Moodie G. E. E., Mathias J. A. (1986). Significance of cannibalism in larval walleye (Stizostedion vitreum). Can. J. Fish. Aquat. Sci. 43, 613–618. doi: 10.1139/f86-073
Peterson D., Carline R., Wilson T., Hendricks M. (1997). Production-scale methods for intensive culture of walleye fry. Progress. Fish Cult. 59, 14–19. doi: 10.1577/1548-8640(1997)059>0014:PSMFIC<2.3.CO;2
Phillips T. A., Summerfelt R. C., Clayton R. D. (1998). Feeding frequency effects on water quality and growth of walleye fingerlings in intensive culture. Progress. Fish-Cult. 60, 1–8. doi: 10.1577/1548-8640(1998)060<0001:FFEOWQ>2.0.CO;2
Piper R. G. (1982). Fish Hatchery Management. (Washington D.C., USA: U.S. Department of the Interior, Fish and Wildlife Service). Available at: https://books.google.com/books?id=FPiHkQiZL_AC.
R Core Team. (2023). R: A Language and Enviornment for Statistical Computing (R Foundation for Statistical Computing). Available online at: https://www.R-project.org/ (Accessed December 25, 2023).
Reiger P., Summerfelt R. (1995). The nature and significance of cannibalistic behavior in larviculture walleye. Progress. Fish-Cult.
Rieger P. W., Summerfelt R. C. (1997). The influence of turbidity on larval walleye, Stizostedion vitreum, behavior and development in tank culture. Aquaculture 159, 19–32. doi: 10.1016/S0044-8486(97)00187-7
Rieger P. W., Summerfelt R. C. (1998). Microvideography of gas bladder inflation in larval walleye. J. Fish Biol. 53, 93–99. doi: 10.1111/j.1095-8649.1998.tb00112.x
Steenfeldt S. (2015). “Culture methods of pikeperch early life stages,” in Biology and Culture of Percid Fishes: Principles and Practices, eds. Kestemont P., Dabrowski K., Summerfelt R. (Netherlands: Springer, Dordrecht), 295–312. doi: 10.1007/978–94-017–7227-3_10
Summerfelt R. C. (1996). “Walleye culture manual”, NCRAC culture series 101. (Iowa State University, Ames: North Central Regional Aquaculture Center Publications Office).
Summerfelt R. C. (2013). “Gas bladder inflation in larval fish aquaculture”, in Larval fish aquaculture, ed. Qin J. G. (Hauppage, NY, USA: Nova Science Publishers, Inc.), 125–142.
Keywords: larviculture, walleye (Sander vitreus), cannibalism, intensive rearing, aquaculture, decomposition
Citation: Neibauer J, Branville C, Holmes K, Hauser E and Firkus T (2024) Unobserved mortality occurs early in larval walleye (Sander vitreus) aquaculture. Front. Aquac. 3:1387495. doi: 10.3389/faquc.2024.1387495
Received: 17 February 2024; Accepted: 24 May 2024;
Published: 17 June 2024.
Edited by:
Youji Wang, Shanghai Ocean University, ChinaReviewed by:
Ivar Lund, Technical University of Denmark, DenmarkVikash Kumar, Central Inland Fisheries Research Institute (ICAR), India
Copyright © 2024 Neibauer, Branville, Holmes, Hauser and Firkus. This is an open-access article distributed under the terms of the Creative Commons Attribution License (CC BY). The use, distribution or reproduction in other forums is permitted, provided the original author(s) and the copyright owner(s) are credited and that the original publication in this journal is cited, in accordance with accepted academic practice. No use, distribution or reproduction is permitted which does not comply with these terms.
*Correspondence: Colton Branville, colton.d.branville@gmail.com; Tyler Firkus, tyfirkus@uwsp.edu
†These authors share first authorship